- 1The Water Institute at UNC, Department of Environmental Sciences and Engineering, Gillings School of Global Public Health, University of North Carolina at Chapel Hill, Chapel Hill, NC, United States
- 2Department of Civil and Environmental Engineering, Faculty of Engineering, Kyambogo University, Kampala, Uganda
- 3Department of Civil and Environmental Engineering, College of Engineering, Design, Art and Technology (CEDAT), Makerere University, Kampala, Uganda
- 4School of Engineering, University of Northern British Columbia (UNBC), Prince George, BC, Canada
Globally, about 2.7 billion people depend on onsite sanitation systems (OSS) (e.g., septic tanks) for their sanitation needs. Although onsite sanitation systems help in providing primary treatment for domestic wastewater, they don’t effectively remove nutrients, pathogens, and other inorganic contaminants. Previous studies have posited that the use of post treatment systems which incorporate biochar leads to improved contaminant removal efficiency. However, the mechanism through which contaminants are removed and factors potentially affecting the removal are still understudied. To fill this knowledge gaps, this review discusses factors which affect efficiency of biochar in removing contaminants found in onsite domestic wastewater, modifications applied to improve the efficiency of biochar in removing contaminants, mechanisms through which different contaminants are removed and constraints in the use of biochar for onsite wastewater treatment. It was noted that the removal of contaminants involves a combination of mechanisms which include adsorption, filtration, biodegradation, ion exchange, pore entrapment. The combination of these mechanisms is brought about by the synergy between the properties of biochar and microbes trapped in the biofilm on the surface of the biochar. Future areas of research such as the modification of biochar, use of biochar in the removal of antibiotic resistant genes (ARGs), application of wet carbonization methods and resistance of biochar to physical disintegration are also discussed. This study provides useful information that can be applied in the use of biochar for the treatment of wastewater and guide future design of treatment systems for optimized treatment performance.
1 Introduction
In many low- and middle-income countries, the sanitation needs of most people are met by on-site sanitation systems (OSS) due to their low capital and maintenance costs (Strande et al., 2014; Manga et al., 2019; Krueger et al., 2020; Manga et al., 2020). Although these systems are cost-effective, they are unsustainable due to their inability to properly treat human excreta. Furthermore, the liquid released from these systems into the surrounding soils is a source of environmental pollution and a threat to public health and environmental quality (Manga et al., 2022a; Capone et al., 2022). One such system is the septic tank discharging to a soak away pit, that is currently in use by about 1.7 billion people worldwide and is considered a minimum standard for use in public/congregated places in some developing nations (WHO and UNICEF, 2021). Limitations of these systems have brought about the need to explore subsequent post-treatment or polishing technologies required to achieve the desired effluent disposal or reuse standards. Various ecologically safe and cost-effective technologies such as constructed wetlands (CW) or subsurface wastewater infiltration systems (SWIS), have shown to be promising systems (Mburu et al., 2013). However, these systems are not effective in removing phosphorus from wastewater due to the fact that they make use of sand bed media and do not have sufficient capacity to bind phosphorus for a prolonged period (Brix and Arias, 2005). Different kinds of media such as oyster shell, limestone, calcite, zeolite, and biochar have been applied to improve the contaminant removal efficiency of these soil-based biofiltration systems (Gungor and Unlu, 2005; Gill et al., 2009; Wang et al., 2013; Zhang et al., 2015). Amongst the aforementioned amendment materials, biochar holds great potential to improve the removal of contaminants due to its ability to adsorb various organic and inorganic compounds, good cation exchange capacity and ability to serve as growth media for biofilm. Though a lot of investigations have been carried out on the use of biochar for centralized wastewater treatment, the use of biochar for the treatment of effluent from onsite containment units is still nascent and there are still some knowledge gaps. Mechanisms through which different contaminants can be removed and factors which affect the ability of biochar to adsorb contaminants found in onsite domestic wastewater are not well documented. The aim of this paper is to critically evaluate the applicability of biochar for the treatment of effluent from onsite containment units especially septic tanks. The review specifically discusses: 1) Factors which affect efficiency of biochar in removing contaminants in onsite domestic wastewater; 2) biochar modifications to improve contaminant removal efficiency; 3) mechanisms of different contaminants removal; and 4) constraints, gaps and areas of future research in the use of biochar for onsite wastewater treatment.
2 Composition of domestic onsite wastewater
Domestic on-site wastewater emanates from onsite wastewater treatment systems (OWTS) which are used for the treatment and disposal of household wastewater in places where centralized sewerage systems are not available (Babcock et al., 2014). Some of the most commonly used OWTS are septic systems, cesspools, aerobic treatment units (ATUs), above-ground mounded soil systems, subsurface drip irrigation systems, land application systems such as spray irrigation, and aquatic systems such as wetlands and lagoons. OWTS are multistage systems which make use of a combination of technology and natural processes to collect and treat wastewater. The treated effluent is released into the environment through a dispersal unit which makes use of a network of soil infiltration trenches (leach field) where the effluent is further treated as it percolates through the vadose zone prior to discharge to local groundwater (Lamichhane, 2007; Manga et al., 2022a).
Domestic on-site wastewater is made up of human faeces, urine, cleansing material, flush water and at times grey water and contains various pollutants which include organic matter (biochemical oxygen demand (BOD) and chemical oxygen demand (COD)), nutrients (i.e., nitrogen and phosphorous) (Table 1), pathogens, heavy metals, pharmaceutical, and personal care products (PPCPs) (Manga et al., 2016; Martikainen et al., 2018; Guruge et al., 2019; Farkas et al., 2020; Semiyaga et al., 2022; Tokwaro et al., 2023). Pathogens found in onsite domestic wastewater include bacterial pathogens (i.e., Escherichia coli, Legionella pneumophila, Leptospira spp, Salmonella spp, Shigella spp, Vibro cholera, and Yersinia enterocoliticia), viral pathogens (e.g., adenovirus, enterovirus, hepatitis A, norovirus, reovirus, rotavirus, and echovirus) and helminths (ascaris, hookworm, whipworm) (Table 2) (Lusk et al., 2017; Manga, 2017; Manga et al., 2023). Heavy metals in domestic wastewater emanate from detergents and body care products (Akpor et al., 2014). The most common heavy metals in domestic wastewater are lead (Pb), mercury (Hg), cadmium (Cd), chromium (Cr), copper (Cu), nickel (Ni), silver (Ag), zinc (Zn), manganese (Mn), and iron (Fe) (Table 3) (Chino et al., 1991; Manga et al., 2022b). Different kinds of PPCPs have been found in domestic onsite wastewater and they include ibuprofen, caffeine, erythromycin, ciprofloxacin, sulfamethoxazole, ketoprofen, carbamazepine (Table 3) (Miège et al., 2009).
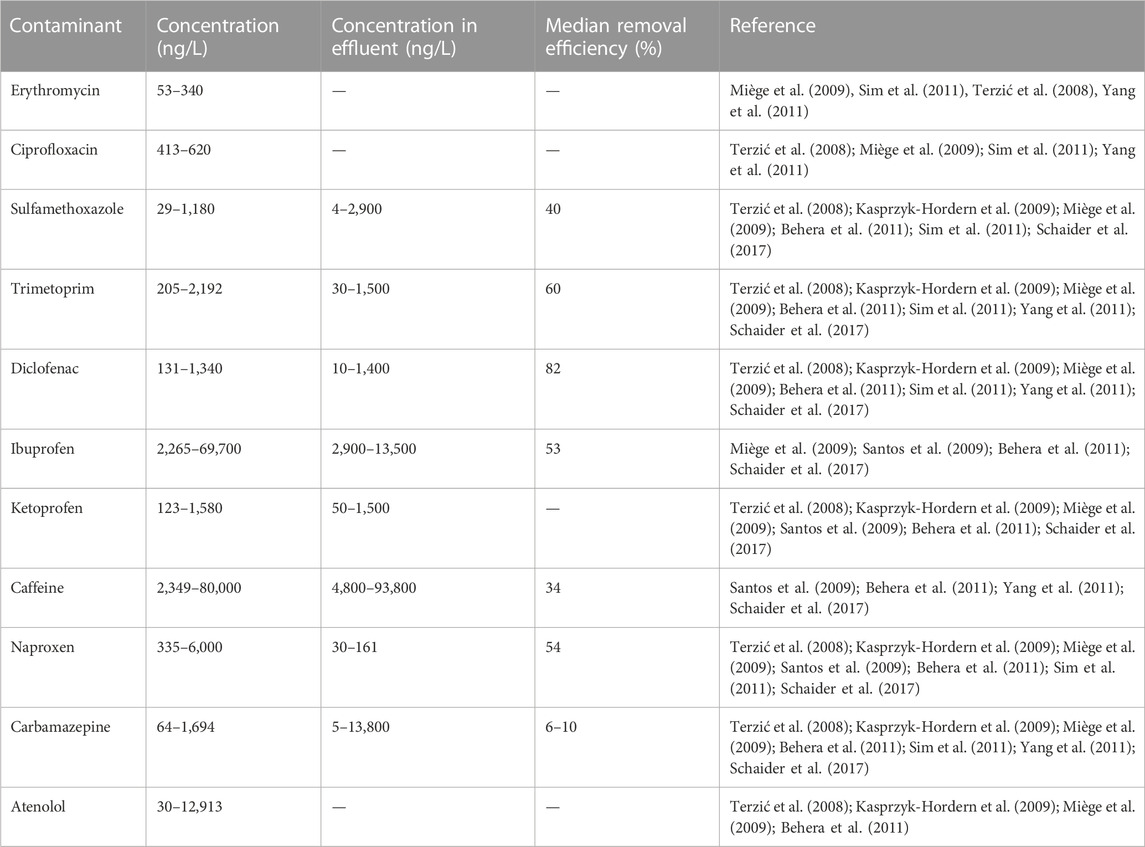
TABLE 3. Pharmaceutical and personal care products detected in domestic wastewater, concentrations in effluent of onsite systems, and removal efficiencies by onsite systems.
The quantities of each of the aforementioned contaminants in domestic wastewater stream usually varies with factors such as weather conditions, number of users, water use patterns (Oteng-Peprah et al., 2018). Majority of these pollutants are persistent in nature (especially PPCPs) and cannot be easily removed using conventional onsite treatment techniques, such as sand filter and soil infiltration treatment systems. These systems have been proven to be only partially effective in removing different kinds of organic micropollutants found in domestic wastewater including PPCPS. For instance, Gros et al. (2016) noted low to intermediate removal of PPCPs in soil beds that are used for onsite filtration (e.g., 46% removal for ibuprofen, 86% for diclofenac, 44% for metoprolol, and 73% for caffeine), while Blum et al. (2017) reported insignificant (>10%) removal of acetaminophen in soil beds A summary of the concentration and ranges of these pollutants in domestic wastewater, quality of effluent from onsite systems as well as removal efficiencies are presented in Tables 1–3.
Currently, the methods used for removing PPCPs from wastewater include electrochemical method (Barrios et al., 2016), microbial method (Ferreira et al., 2016), membrane process, chemical oxidation process and adsorption method (Acero et al., 2015). Amongst the aforementioned methods, adsorption is widely accepted because of its cost effectiveness, high efficiency, and wide processing range. The most commonly used adsorbents are activated carbon, silica gel, alumina, polyacrylamide, adsorbent resin, and zeolite (Esmaeeli et al., 2017; Qu et al., 2018; Peng et al., 2019; Jiang et al., 2020). Though activated carbon has very large specific surface area and in turn a large adsorption capacity, it is expensive while other adsorbents like silica gel, alumina, adsorbent resin have narrow adsorption surface and polyacrylamide is difficult to produce (Cheng et al., 2021). This necessitates the need for other treatment techniques which are efficient, economical, and environmentally friendly such as the use of biochar. When compared with other adsorbents, biochar presents a potential low-cost and effective adsorbent; the properties of biochar such as its large surface area, porous structure, enriched surface functional groups and mineral components make it possible for it to be used as proper adsorbent for contaminants in domestic wastewater. The specific properties of biochar including large specific surface area, porous structure, enriched surface functional groups and mineral components make it possible to be used as proper adsorbent to remove pollutants from aqueous solutions (Tan et al., 2015).
3 Biochar and factors influencing contaminant removal by biochar
3.1 Production of biochar
Biochar is a solid residue produced either by pyrolysis or gasification of biomass with former being the widely used technique. Pyrolysis refers to the thermal decomposition of organic materials in an inert atmosphere (oxygen-limited environment). The pyrolysis process can be represented by a generic chemical reaction (Eq. 1).
Depending on the operating conditions such as as temperature, heating rate, residence time, and pressure, pyrolysis process can be divided into three classes: Slow pyrolysis (carbonization), fast pyrolysis, and flash pyrolysis (Demibraş, 2001). Flash pyrolysis is distinguished from fast pyrolysis by relatively higher heating rates, and very low residence time (0.5–2 s). The choice of pyrolysis process used depends on the most preferred product. If the aim is the production of liquid and/or gaseous products, then fast pyrolysis is recommended but if the aim is more formation of biochar, then slow pyrolysis is to be used. The use of pyrolysis for biochar production from biomass is considered to be an affordable and environmentally friendly process (Ahmad et al., 2012). Biochar production is considered environmentally friendly since it stores carbon in a stable form thereby offsetting the release of greenhouse gases into the atmosphere (Xiang et al., 2020). In producing biochar from pyrolysis, process parameters such as temperature, residence time, heating rate are responsible for determining the yield of the biochar.
3.2 Factors for contaminant removal using biochar
3.2.1 Internal factors
The ability of biochar to remove contaminants present in wastewater is governed by some of its intrinsic properties (physicochemical and spectral properties of biochar) such as the feedstock type, specific surface area and porosity. The factors which influence the ability of biochar to remove contaminants can be external (related to pyrolysis conditions, feedstock type and properties of the solution to be treated). The later depends on the preparation process and pyrolysis conditions used the following sections will elaborate more about these factors:
3.2.1.1 Specific surface area and porosity
The specific surface area (SSA) and porosity of biochar are major parameters that influence its ability to adsorb contaminants (Liu et al., 2015). When biomass is being pyrolyzed, some of the volatile components are lost and this leads to the formation of micropores on the surface of the biochar (Bagreev et al., 2001). The pore size is very important for adsorption as biochar with small pore size cannot trap large sorbate, regardless of their charges or polarity (Ahmedna et al., 2004). When biochar is highly porous, it has high potential for filtration and this presents two major advantages when it is used as a biofilter for water/wastewater treatment: 1) Good water-holding capacity in the pores and 2) ability to harbor biofilm in pores without clogging (Li et al., 2016).
Biochar porosity and SSA vary considerably with pyrolysis temperature with high temperature ranges leading to larger pore size and larger surface area which in turn leads to increased adsorption efficiency. The aforementioned trend has been noted in quite a number of studies. Chen et al. (2014) noted that when pyrolysis temperature increased from 500°C to 900°C, the pore volume of biochar increased from 0.056 to 0.099 cm3/g while the surface area increased from 25.4 to 67.6 m2/g. However, in some cases, biochar produced at high temperature has lower surface area and porosity. Chun et al. (2004) observed that the surface area of wheat straw biochar reduced when the pyrolysis temperature decreased from 700°C to 600°C. The reduced surface area is due to the fact that at high temperature, the porous structure of biochar may be reduced by graphitization bringing about reduced surface area. Thus, it is necessary to optimize pyrolysis temperature to obtain biochar with the required adsorptive characteristics.
3.2.1.2 Feedstock type
Different kinds of organic materials can be used as feedstock to produce biochar; these organic materials can be plant-based, manure-based, or agricultural/food processing residual–based biomass (Table 4). The composition of the parent material influences the yield, elemental content, and the microstructure of the biochar. Generally, plant-based biochar has high lignin content, which results in high biochar yield. Animal manure and sludge have high inorganic content implying that large quantities of ash will be generated from the feedstock when it is pyrolyzed leading to significant reduction in the surface structure and reaction properties of the biochar (Zielinska et al., 2015).
Feedstock type and pyrolysis temperature influence the quantity of ash in biochar and in turn the use to which the biochar can be put (Ali et al., 2022a). Generally, biochar from sewage sludge and faecal sludge have higher ash contents than biochar from wood and agricultural/food processing residue. The high ash content of biochar from sewage sludge is principally due to the high mineral/inorganic matter content of sludge which is turned into ash after pyrolysis (Zielinska et al., 2015). The high ash content of biochar from sludge implies that it can be applied as soil amendment while biochar from wood and agricultural/food are better suited for the removal of contaminants in wastewater (Perez-Mercado et al., 2018). This implies that if biochar from faecal sludge and sewage sludge are to be utilized for wastewater filtration, they will need to be co-pyrolyzed with other biomass with lower ash content.
Pyrolysis process leads to a change in elemental composition of the raw material, which is usually reflected by an increase in carbon (C) content and a decrease in hydrogen (H), and oxygen (O) contents. This results in an increase in the aromatic properties and a decrease in the polarity of the biochar. Generally, livestock manure and sewage sludge biochar have relatively higher nitrogen (N) and sulfur (S) contents than plant-based biochar which has higher C contents. The higher carbon content of plant-based biochar is due to the fact that they are lignocellulosic materials which have high lignin content that promote carbonization and increase the biochar carbon content (Tomczyk et al., 2020). Also, due to the fact that wood-based biochar maintains its plant cell structure and contains interconnected pores (5–10 µm diameter), it allows for better retention and entrapment of bacteria (Abit et al., 2012). This fact was corroborated by Lau et al. (2017) who noted that biochar produced from forestry wood waste removed 92%–99% E. coli from synthetic storm water.
3.2.2 External conditions
Pyrolysis process parameters such as temperature, heating rate, residence time as well as absorbent dose and solution pH influence the intrinsic properties of biochar and in turn its ability to adsorb contaminants (Gopinath et al., 2021).
3.2.2.1 Temperature
The temperature at which pyrolysis is carried out influences the ability of biochar to remove contaminants as it affects the yield, specific surface area, types, and abundance of the surface functional groups on biochar (Zhou et al., 2019). Various researchers have conducted experiments to determine the effect of temperature on adsorption properties of biochar. Hossain et al. (2011) carried out the pyrolysis of dried sewage sludge under different temperatures, which ranges from 300°C to 700°C at heating rate of 10°C/min under nitrogen gas atmosphere in a horizontal tubular reactor. It was observed that biochar yield decreased from 72.3% to 63.7%, 57.9%, and 52.4% when temperature was increased from 300 to 400, 500 and then 700°C, respectively. This is because, when temperature increases, the decomposition of the organic material occurs at a faster rate and certain parts of the raw biomass are lost (Qurat-ul-Ain et al., 2021). In addition, Konczak et al. (2019) reported that the specific surface area increased from 69.7 to 75.5 and 89.2 m2/g, when the pyrolysis temperature increased from 500 to 600°C and 700°C, respectively. This is due to removal of volatile matter leading to the opening of pores and in turn providing additional surface area. Also, pyrolysis temperature influences the percentage of carbon (C), oxygen (O), and hydrogen (H) contents in biochar as increase in temperature leads to an increase in the percentage of carbon but decreases the oxygen and hydrogen content in the mixture (Weber and Quicker, 2018). The considerable changes in H and O contents have been attributed to the cleavage of heterocyclic compounds and nitrile group at elevated temperature (Konczak et al., 2019).
3.2.2.2 Adsorbent dose
The amount of biochar applied has significant influence on the adsorption efficiency and in turn application of the optimum dose for contaminants removal, which is vital for cost-effective application. Increase in adsorption dosage enhances the adsorption efficiency of both organic and inorganic pollutants due to the availability of numerous bonding or sorption sites. Chen et al. (2011) investigated the removal of toxic metals (Cd, Cu, Pb, and Zn) from wastewater using biochar made from hardwood and corn straw. They observed that the adsorption efficiency of toxic metals by biochar improved when biochar concentration increased from 0.5 to 5 g/L. The increase in adsorption efficiency was attributed to increase in the number of active sites and surface area brought about by increase in amount of biochar. This observation is similar to results obtained by Lalhruaitluanga et al. (2010); Tsai and Chen (2013); Lu et al. (2017); Wang et al. (2018) observed similar results when they noted that increasing the biochar amount increased adsorption of organic pollutants. This shows that high concentration of biochar has a positive impact on the adsorption of pollutants and it would be therefore useful to find the optimum dose, which is a key factor to minimize the biochar production costs in view of its industrial application, (Ambaye et al., 2021). However, the adsorption efficiency can be negatively correlated with adsorbent dose when its optimized dose is exceeded (Saltalı et al., 2007). This can be attributed to the unsaturation of sorption sites and also overcrowding of adsorbent molecules, which may lead to overlapping (Merrikhpour and Jalali, 2012).
3.2.2.3 Residence time
Residence time which is the amount of time the water molecules spend within the adsorbent is another important factor to consider during adsorption process. In general, the adsorption process has two-stages; a first stage which is rapid and quantitatively significant and a second stage which is slower. In the first stage, a larger surface of adsorbent is easily available but the pores of the biochar get clogged with time, leading to reduced adsorption and higher residence time in the second stage. Kizito et al. (2015) investigated the effect of contact time on the adsorption of ammonium ion (NH4+) and observed that NH4+ adsorption increased rapidly with time in the first 6–8 h and became significantly slower until equilibrium was reached. A similar observation was also made by Saltalı et al. (2007).
3.2.2.4 Solution pH
The solution pH is an essential parameter in the adsorption process and its influence depends on the biochar type and target contaminants. The pH influences both the surface charge and the degree of ionization and speciation of the biochar (Kołodyńska et al., 2012; Regmi et al., 2012). Changes in solution pH bring about changes on the surface functional groups found on biochar; when the solution is acidic, most of the functional groups on biochar are protonated and are present in positively charged form favoring adsorption of anions (Oh et al., 2012; Abdel-Fattah et al., 2014). With an alkaline solution, the surface of biochar is negatively charged, and the cations can easily be captured by biochar surface. The aforementioned trends have been noted in studies of different heavy metals adsorption on various kinds of biochar. Tong et al. (2011) investigated Cu (II) ion adsorption on three different types of biochar and observed that the adsorption of Cu (II) ion was increased when the solution pH was increased from 3.5 to 6.0. In another study by Chen et al. (2011) where biochar made from hardwood and corn straw were used to adsorb metals like copper, zinc, and lead, it was noted that when the pH increased from 2.0 to 5.0, the adsorption capacity of the metallic cations also increased. But at pH above 5.0, there was a decrease in the adsorption capacity due to hydroxide complex formation (Ambaye et al., 2021) Similar results were reported by Lu et al. (2012) who noted enhanced Pb adsorption on sludge derived biochar at increased pH.
3.2.2.5 Heating rate
The heating rate during pyrolysis usually affects the structural and physicochemical properties of the resulting biochar most especially the pore structure (Yaman, 2004). When the heating rate is low and residence time is long and the pyrolysis process will occur slowly leading to full carbonization of the organic matter content. This brings about perforation in the pore wall structure of the biochar and subsequent decrease in the number of micropores in the biochar. When heating rate is high, large amount of volatile matter will escape from the feedstock within a short time leading to formation of a loose, and well-developed pore structure (Pan et al., 2021).
4 Modification of biochar for enhanced contaminant removal
When biochar is produced without any modification, it has poor surface functional groups (only
4.1 Physical activation
Water vapour and carbon dioxide (CO2) are the most commonly used oxidizing agents for physical activation of biochar (Sajjadi et al., 2018). Biochar is subjected to extremely high temperatures (800°C–1,100°C) and active atmosphere pressure with vapour, CO2 or a mixture. The process increases the porosity and specific surface area of the biochar. The chemical reactions governing physical modification by steam using CO2 and H2O as reagents, are expressed in Equations (2)–(4) (Bansal et al., 1988).
In the study by Kołtowski et al. (2017), significant changes in physical properties such as specific surface area, micro-pores, and surface chemical characteristics (such as functional groups, polarity, and hydrophobicity) were observed after physical activation. The activation of cotton shell biochar using steam and CO2 independently and then in combination was investigated by Yang et al. (2010), where results showed that the specific surface area of the activated carbon increased by over 2000 m2/g irrespective of the activation agent used.
4.2 Chemical activation of biochar
In chemical activation, biochar is impregnated with either acids (sulfuric acid, H2SO4; hydrochloric acid, HCl; phosphoric acid, H3PO4; nitric acid HNO3; and carbon dioxide, CO2), alkalis (potassium hydroxide, KOH; sodium hydroxide, NaOH and potassium carbonate K2CO3) or oxidizing agents (hydrogen peroxide, H2O2, and potassium permanganate KMnO4). Acidic activation is purposely done to introduce acidic surface functional groups to biochar. Depending on the type and concentration of the acidic agent, SSA and pore structure of biochar can be altered. Peng et al. (2016) reported that 1 M of HCl enhanced the surface area of biochar by more than 50%. Another study by Vithanage et al. (2015) reported a significant increase of more than 2,400% (i.e., 2.3 to 571 m2/g) when biochar was activated by 30% H2SO4 and oxalic acids. Feedstock type and method of preparation have a strong effect on the properties of biochar modified by alkali. Similarly, it is reported that the type and proportion of alkali activator have profound effect on the characteristics of biochar (Shen and Zhang, 2019). The activation of biochar by oxidizing agents such as H2O2 and KMnO4 is reported to enhance oxygen-containing surface function groups. In a study by Wang et al. (2015) where hickory-wood biochar was activated by impregnating it with KMnO4, it was observed that there was an increase in the surface area from 101 to 205 m2/g. Also, the adsorption capacity towards Pb (II), Cu (II), and Cd(II) was enhanced after the modification. In another study by Xue et al. (2012) where biochar from agricultural residue was modified with H2O2, it was noted that when compared to the control group without modification, the modified one had higher oxygen functional groups particularly the carboxyl group and this lead to enhanced lead adsorption capacity (22.82 mg/g for the modified biochar and 0.88 mg/g for unmodified biochar).
4.3 Impregnation methods
In impregnation, metal salts or oxides, hydroxides and nanoparticles are being applied for biochar surface modification to enhance contaminant removal capacity due to their high abundance, cost-effectiveness, environmental friendliness, and chemical stability (Li et al., 2017). Biochar impregnation can be conducted by 1) soaking or suspending the biomass followed by pyrolysis and 2) pyrolysis of the biochar followed by impregnation (Ahmed et al., 2016). In a study by Chen et al. (2011), biochar was produced at different temperatures and impregnated with magnetite (Fe2O3) for the removal of phosphorus. It was observed that the modified biochar showed higher phosphorus adsorption (∼99% removal) than unmodified biochar. In another study by Li et al. (2017), it was noted that adding ferric chloride (FeCl3) to biochar increased the phosphorus adsorption rate from 1 to 16.58 mg/g of biochar. Zhou et al. (2014) observed that when biochar surface was loaded with zerovalent iron (ZVI)–silver (Ag) nanoparticles (NPs) complex, the growth of E. coli was completely hindered. Silver nanoparticles (Ag-NPs) serve as antimicrobial particles due to a range of mechanisms such as disruption of cell wall and cytoplasmic membrane, denaturation of ribosomes, interruption of ATP production, and interference of DNA replication (Yin et al., 2020). Biochar composites impregnated with nanomaterials have also been found to improve the removal of PPCPs. For instance, the adsorption capacities of a group of PPCPs [salicylic acid (683 mg g−1), naproxen (533 mg g−1), and ketoprofen (444 mg g−1)] were 11–45 times higher in iron oxide (Fe2O3)-impregnated biochar when compared with those of unmodified biochar (Baccar et al., 2012; Karunanayake et al., 2017; Ahmed & Hameed, 2018; Anfar et al., 2020). Higher adsorption capacities of caffeine, ibuprofen, and acetylsalicylic acid were observed in biochar impregnated with iron oxide nanoparticle (Liyanage et al., 2020).
5 Mechanisms of contaminants removal from domestic wastewater using biochar
When biochar is applied as a support media for the treatment of domestic wastewater, several physical, chemical, and biological mechanisms are responsible for pollutant removal. However, the dominant removal mechanism depends on several factors which include the biochar’s physicochemical properties, system configuration and operational condition (Quipse et al., 2022). These mechanisms include adsorption, biodegradation, ion exchange, and pore filling/entrapment.
5.1 Adsorption
The mechanism used by biochar for adsorption is dependent on the heterogeneity of the adsorbent surface and the nature of the pollutant (adsorbate). Adsorption to the biochar surface occurs through van der Waals forces and hydrogen bonds with functional groups, filtration, electrostatic interaction, ion exchange, and surface complexation (Li et al., 2019; Cheng et al., 2021). When organic contaminants are to be removed, electrostatic attraction, hydrophobic sorption, hydrogen bond, π-π electron-donor acceptor interactions and pores fillings facilitate the adsorption process. For the removal of inorganic pollutants (heavy metals), the adsorption process occurs through electrostatic attraction, ion exchange, complexation, and co-precipitation. In complexation and co-precipitation, the metals present in the domestic wastewater form complexes with oxide minerals or free carboxyl and hydroxyl functional groups available on the biochar surface after which they settle (physical sorption) or form layers (precipitation) on the biochar surface (Li et al., 2014). Pharmaceutical and personal care products which are emerging contaminants are usually adsorbed by biochar mainly by mechanisms of electrostatic attraction, hydrophobic effects, aromatic p-donor and cationic p-acceptor conjugation, hydrogen bonding and pore filling (Zhang et al., 2019; Xiang et al., 2020).
Pore filling is another mechanism through which contaminants are removed from onsite wastewater by adsorption. The pore network of biochar is usually made of micropores <2 nm, mesopores ∼2–50 nm, and macropores >50 nm; the micropores and small mesopores (2–20 nm) contribute majorly to the surface area of the biochar and mostly influence its uptake for organic compounds (Pignatello et al., 2006). Pore filling is usually applied for the removal of organic contaminants in domestic wastewater and this is a function of the total micropore and mesopore volume on biochar pore surface (Nguyen et al., 2007; Hao et al., 2013). The pore fling mechanism depends upon nature, type of the biochar, as well as the polarity of the organic contaminant. Kasozi et al. (2010) studied the removal of organic contaminants from wastewater using biochar made from oak, gamma-grass and loblolly pine; it was noted that the biochars’ strong affinity for catechol (PPCP) was mainly due to micropore filling.
5.2 Biodegradation
The synergy between the properties of biochar and the metabolism of microbial organisms attached to the surface of biochar play a significant role in its removal of contaminants (Faulwetter et al., 2009). Biochar’s distinct physicochemical characteristics such as large surface area and excellent pore structure make it support several microbial communities (Wang et al., 2020; Deng et al., 2021). The pH of biochar and the nature of dissolved oxygen carbon (DOC) are also important parameters for microbial growth on biochar. There is limited evidence in literature documenting the effects of biochar on the composition, activities and diversities of microorganisms during the treatment of household wastewater. Dalahmeh et al. (2014) evaluated the effect of bark, charcoal and sand filters on microbial diversity and potential respiration during the treatment of greywater. It was observed in the study that charcoal filters enhanced the development of diverse microbial communities. For the bacterial community composition, 33%, 25%, 13%, and 11% were reported for classes g-proteobacteria, a-proteobacteria, b-proteobacteria and Clostridia, respectively. There was a negative correlation between potential respiration and depth in both charcoal and bark filters due to fast mineralization of easily biodegradable matter in the topmost layer and subsequent oxygen depletion in the layers underneath. This is an indication that charcoal filters should be prepared to be more shallow in comparison with sand filters in greywater treatment systems; Deng et al. (2021) revealed that the use of biochar as a substrate in constructed wetlands was found to support many functional microbes such as nitrifying and denitrifying bacteria. This is because biochar has large SSA and several pores making it easier to trap huge amounts of organic matter which supports reproduction and metabolism in microorganisms (Gul et al., 2015; Ji et al., 2020; Jia et al., 2020; Yuan et al., 2020). Other researchers also observed an enhancement of functional microorganisms in relation with organic matter degradation and nitrogen removal due to biochar amendments in constructed wetlands (Guo et al., 2020; Ji et al., 2020; Liang et al., 2020; Deng et al., 2021). The addition of biochar has the potential to increase the proportion of microorganism groups. With this, microbial activity is enhanced and the stress in removing toxic substances is eliminated (Wang et al., 2020). The overall effect of biochar incorporation is that there is regulation of microbial activities which enhances the removal of pollutants.
5.3 Ion exchange
Ion exchange is a reversible reaction which occurs between ions in the liquid phase and ions in the solid phase. During ion exchange, when ions in the liquid phase get adsorbed by the ion exchange solid, the ion exchange solid releases equivalent ions back into the solution to maintain the electrical neutrality of the aqueous solution. (Cheng et al., 2021). Ion exchange is the main mechanism for removing heavy metals from wastewater by biochar and the ion exchange capacity is usually influenced by pyrolysis temperature as temperatures greater than 350°C decreases the ion exchange capacity of biochar. Several studies have noted that higher ion exchange capacity leads to higher adsorption efficiency for metals (El-Shafey, 2010; Trakal et al., 2016). Though ion exchange is the main mechanism for removing metals from onsite domestic wastewater, PPCPs cannot be easily removed by ion exchange. This is due to the fact that they cannot be easily converted into ionic forms. (Cheng et al., 2021).
5.4 Synergetic removal of contaminants from onsite domestic wastewater
Synergetic removal of PPCPs, organic matter and nutrients from wastewater using biochar filters involves the combination of adsorption and biodegradation processes. When adsorption and biodegradation processes are used together, biochar filters can be made self-sustaining through bio-regeneration; microbes (naturally present in water or externally introduced to suit target contaminants) can be immobilized on the surface of porous carbon forming biofilms which then metabolize and degrade the adsorbed contaminants in a bio-regeneration process. This bio regeneration process frees up clogged pores of the biochar, rejuvenating its adsorptive capacity thereby increasing its lifespan (Simpson, 2008; Jin et al., 2013). Also, nutrients such as nitrogen which are retained on the surface of biochar filters can help to enhance the biodegradation process. A study conducted by Jefferson et al. (2001) showed increased biological treatment (increased COD removal and oxygen uptake) after micronutrients (Zn) and macronutrients (N, P) were added.
Figure 1 shows the various mechanisms through which contaminants are removed from domestic onsite wastewater by biochar.
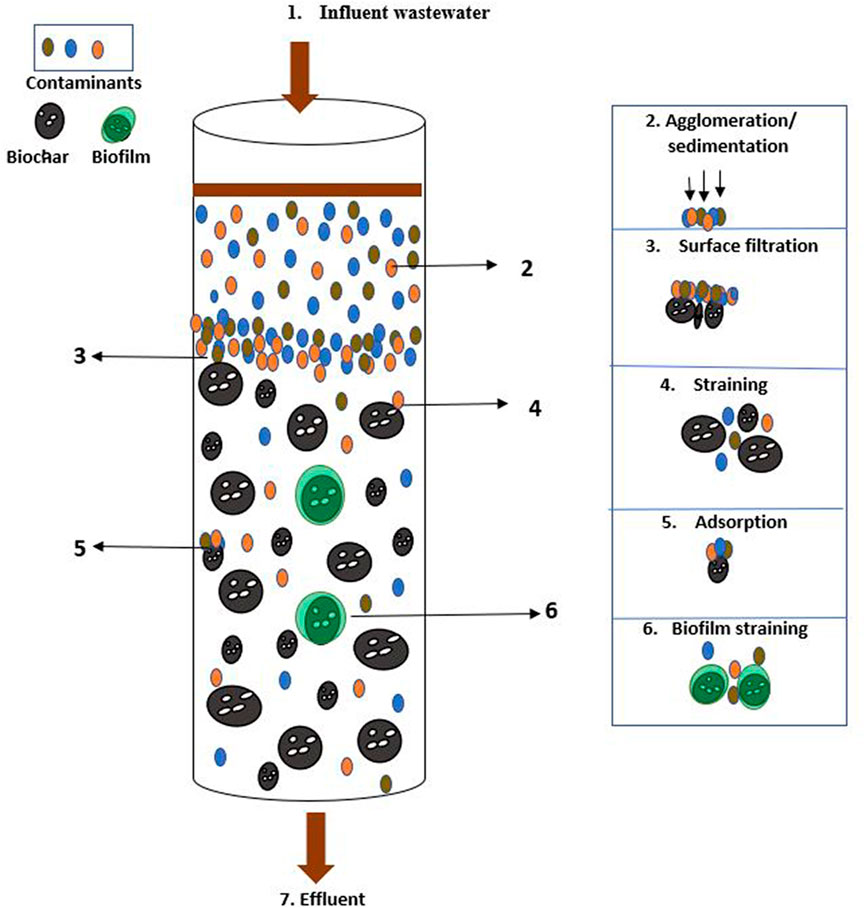
FIGURE 1. The various mechanisms through which contaminants are removed from onsite domestic wastewater by biochar (Modified from Quipse et al., 2022).
6 Previous studies on the use of biochar for the treatment of onsite domestic wastewater
Previous studies have successfully incorporated biochar into on-site sanitation facilities for the treatment of domestic wastewater. Mwenge and Seodigeng (2019) carried out experiments in laboratory plastic columns where activated biochar from agricultural wastes sandwhiched between two gravel layers was used as filter for the removal of TSS, NH3-N, NO3-N, and COD in kitchen, floor cleaning, showering and laundry greywater. Two different loadings of biochar—200 and 400 g—were used and it was observed that as the amount of biochar used increased, removal efficiency of contaminants across the wastewater types increased. Also, the initial concentration of pollutants used in the biochar had effect on the removal efficiency as it was noted that kitchen wastewater which had the highest pollutant concentration had the highest removal rate for most of the contaminants. The results of the study showed that the biochar column had efficiency with TSS (95% on kitchen greywater), NH4-N (85% on bathroom greywater), NO3-N (76% on kitchen greywater) and COD (63% on kitchen greywater).
Sidibe (2014) determined the effectiveness of salix leaves biochar filter for the removal of COD, Salmonella spp. And male-specific phages from artificial greywater in a laboratory filtering columns. The hydraulic loading rate was 0.032 m3 m-2 day-1 and the organic loading rate was 76 mg BOD5 m-2 day-1 (240 g COD m-2 day-1). It was noted that the reduction of COD in 60 cm high biochar filter was 90%, 3
Dalahmeh et al. (2016) used pilot scale sand bed biochar filter for the treatment of household grey water and reported the removal efficiency of biochar filter for BOD5, TSS, and E. coli to be 93%, 85% and 1log10 reduction, respectively. Niwagaba et al. (2014) constructed a 60 cm high gravel biochar filter for greywater treatment and reported high removal efficiency of BOD5, TSS and faecal coliforms (FC) which are 96%, 85.2%, and 95%, respectively after 36 h of operation. In contrast to the study of Dalahmeh et al. (2016), it can be seen that the study by Niwagaba et al. (2014) achieved high removal of faecal coliform. These differences can be a direct influence of the properties of the bacteria, the features of the biofilm-supporting materials (sand versus gravel), environmental factors as pH and temperature, the presence of other bacteria, the nutrients (quality and quantity) in the media which together play a role in the removal of faecal coliform (Van Houdt & Michiels, 2010).
Perez-mercado et al. (2018) studied the effect of different biochar filters (pine-spruce, willow and activated biochar) on the removal of COD, total phosphorus and nitrate phosphorus from onsite wastewater before irrigation. All types of biochar and all particle sizes of pine-spruce biochar achieved a high degree of removal of organic material (COD >90%). Willow biochar and activated biochar showed higher removal of total and phosphate phosphorus (>70%) than in pine-spruce biochar. It was noted that the particle size influenced the COD removal efficiency as the larger particle size (2.8 mm) had significantly lower removal efficiency (94%) than the smaller particle sizes (0.7 and 1.4 mm with greater than 99% removal efficiency). The lower removal efficiency of the larger particle size may be attributed to the fact that the larger particle size allows for larger macropores between the particles and it is likely that there is rapid passage through the filter which gives less contact time between the filter and the contaminants in the wastewater and thus less treatment efficiency.
In a study by Kholoma et al. (2016), biochar (about 0.2 m) was placed on top of a sand layer (0.8 m) in a filtration unit which was used for reclaiming domestic wastewater containing 1.9 mg P/L. It was observed that the phosphorus removal efficiency improved by 25.6% in the biochar-amended sand column when compared to the column with only sand. Gupta et al. (2016) mixed oak tree biochar with horizontal flow constructed wetland filter media used for treating synthetic wastewater. The application of biochar brought about increased contaminant removal with removal rates of 91.3% for COD, 58.3% for TN, 58.3% for NH3, 92% for NO3-N, 79.5% for TP, and 67.7% for PO4. . In a pilot-scale study by Bolton et al. (2019), the addition of 100% chipped hemp fibre biochar filter after a horizontal flow gravel wetland for the treatment of domestic wastewater brought about better phosphorus (P) removal in the long term (5 months); 97% P removal was noted compared with 87% in the control (Bolton et al., 2019). The aforementioned studies show that using biochar as a filter media can improve the removal efficiency of phosphorus by constructed wetlands (constructed wetlands typically do not have high removal efficiency for phosphorus).
Forbis-Strokes et al. (2018) applied wastewater containing high concentrations of nitrogen to both unsaturated biochar trickling filters and saturated filters for 1 year and observed that the ammonium ion (NH4 +) removal rate was higher in unsaturated biochar nitrifying column (0.075–0.100 kg N/m3. day) than that of the control gravity column (0.041–0.094 kg N/m3. day). In another trickling filter study, when biochar was added to the sand column, the total nitrogen removal efficiency of the system increased from 8% to 42% (Tait et al., 2015). From all the aforementioned studies, it is seen that the addition of biochar to the treatment units improves the quality of the effluent from these systems making it a suitable alternative for the treatment of onsite domestic wastewater. Biochar-amended onsite sanitation systems have also been applied for enhanced PPCP adsorption (Cheng et al., 2021). Williams et al. (2015) noted that when eucalyptus wood and wheat residue biochar were applied to the soil matrix (0.5 t/ha), the removal of selected active pharmaceutical ingredients (propranonol and carbamazepine) increased by threefold. In another case study, various filtration matrices - (a) 100% silica sand, (b) 100% ZVI, (c) biochar-amended sand (50% v/v), and (d) 10% ZVI +40% biochar +50% sand (v/v)- were compared for their PPCP removal performance. It was observed that the column with a combination of ZVI, biochar, and sand showed the highest PPCP removal efficiency (>97% removal of all PPCPs at 10 μg/L of each chemical) within the top 10 cm of the column while the 100% sand control column did not show effective removal for the tested PPCPs (0.3 μg/L in the effluent) (Liu et al., 2019). Table 6 is a summary of studies which have investigated the suitability of biochar for greywater treatment.
7 Management of spent biochar, constraints, gaps and areas of future research in the use of biochar for wastewater treatment
7.1 Management of spent biochar
Since biochar is usually applied for the removal of toxic pollutants, its disposal needs to be carefully considered. The management option depends on the contaminant removed by the biochar and some of the management options include: Use as a soil conditioner, re-use in wastewater treatment, energy source and landfilling. The following sections discusses these mentioned management options:
7.1.1 Spent biochar as a soil conditioner
If biochar is loaded with nutrients such as ammonium, nitrate, and phosphate, without other toxic pollutants, it can be used as slow-release organic fertilizer into surrounding fields and degraded lands to improve soil fertility and consequently improve crop yield and quality (Yao et al., 2011; Zhang et al., 2013). This is a practical solution which can be used to promote organic farming, and even alleviate agricultural non-point pollution runoff leading to multiple agronomic and environmental benefits (Werner et al., 2018; Kizito et al., 2019; Zheng et al., 2019). However, if biochar is used to adsorb toxic pollutants such as heavy metals and organic pollutants, it cannot be applied to the soil and there will be need to investigate its desorption/regeneration.
7.1.2 Re-use of spent biochar in wastewater treatment
The desorption/regeneration properties of biochar have been investigated by several studies in order to determine the economic feasibility of reusing it as an adsorbent. Zhang et al. (2013) reported that the adsorbed uranyl ion U(VI) could be effectively desorbed from the spent biochar using 0.05 mol/L HCL in four times of adsorption–desorption cycles. In another study, food waste biochar which was loaded with dye was desorbed using ethanol. The results showed that the biochar could be used repeatedly without much loss in the total adsorption capacity of the dyes (Parshetti et al., 2014). Despite the feasibility of reuse, the wide source of waste biomass for producing biochar may make the recovery process economically unnecessary. Therefore, the assessment of the economic feasibility of desorption/regeneration process is needed in the future adsorption process.
7.1.3 Spent biochar as energy source
Apart from regeneration, spent biochar can be used for energy conversion, storage devices, capacitors, and catalyst/catalyst support. Studies have shown that metal impregnated biochar can replace carbon nanotubes and might also be used for tar removal or as supercapacitors (Qian and Chen, 2013). Also, spent biochar can be used for energy production by combustion (Kaetzl et al., 2019).
7.1.4 Landfilling of spent biochar
After re-use, spent biochar can be safely disposed into landfills and can be incinerated. However, knowledge on the disposal of spent biochar using these methods is limited. The stability, potential pollution, effect on the carbon sequestration, and economical feasibility of using these methods to deal with the spent biochar remain unclear necessitating the need for further research in this area.
7.2 Constraints, gaps and areas of future research in the use of biochar for onsite wastewater treatment
Although biochar presents a cost effective and innovative technique for the treatment of onsite domestic wastewater, it still has some constraints which affect its applicability. Biochar is known to physically disintegrate in aqueous solution (Bangham and Razouk, 1938); the physical breakdown occurs through several mechanisms. High oxygen to carbon (O:C) ratio biochar. Can dissolve readily when it is exposed to rewetting or saturation cycles (Parr et al., 1931). Water and water vapor absorption may put stress on the physical structure of biochar due to exothermic graphite sheet swelling. The aforementioned mechanisms lead to the swelling and expansion of biochar’s physical structure which paves way for further fragmentation (Théry-parisot et al., 2010). Moreover, the mechanical strength of biochar reduces with age (Spokas et al., 2014). Consequently, the prolonged exposure of biochar to stress may lead to the formation of fragments during wastewater treatment necessitating the need to develop biochar which can withstand physical breakdown in order to maximize the long-term biochar potential for wastewater treatment.
Biochar is a promising material for on-site sanitation treatment due to its excellent properties. Nevertheless, research on this technology is still in its infancy. Further research is required to optimize the usage of biochar to minimise the release of harmful substances into the atmosphere. It is also important to identify the optimum biochar dose and the number of times each dose can be used for faecal sludge management to enhance adsorption of pollutants. Even though there are similarities between biochar and modified carbon, the physicochemical properties of biochar are known to be highly heterogeneous, depending on the type of feedstock and pyrolysis operating conditions. Thus, validated standardized protocols for biochar technology and its subsequent adoption in on-site sanitation treatment systems is still lacking (Gwenzi et al., 2017). Advanced pyrolysis techniques with precise reaction condition control, high energy efficiency, and low environmental impact at a reduced cost are required for increased biochar usage.
In addition, biochar production through pyrolysis technology is inefficient and energy intensive making it less attractive option now. Homagain et al. (2016) carried out life cycle and cost assessment (LCCA) for the production of biochar from wood and posited that biochar production by pyrolysis is a costly investment. This is especially true in a situation where biochar has to be activated/modified to improve its functionality; if pyrolysis is to be used for the production of biochar, then the substrate has to be pyrolyzed first before it is activated and this methodology usually requires multiple treatment steps, high electricity and energy inputs, and corrosive chemicals, which makes it a tedious, expensive, and complex method (Zhou et al., 2019). Thus, efforts should be geared towards more techniques which can improve the functionality of biochar such as simultaneous pyrolysis and activation. One notable option is through the use of wet carbonization methods such as the use of hydrothermal carbonization (HTC) which is used for producing hydrochar. HTC has an added advantage of eliminating pre-drying, which is highly energy intensive and a huge financial load in biomass pre-treatment when performed under pyrolysis. Additionally, a higher conversion efficiency (40%–70%), and lower operating temperatures make HTC a more suitable technology than pyrolysis (Kambo & Dutta, 2015) on sustainable point of view. Also, the use of hydrothermal carbonization enables the addition of active reagents to the liquid media during carbonization and activation, to form functional biochars in a one-step reaction (Fu et al., 2018).
In developing economies, environmental groups may consider biochar technology to be futuristic and overly ambitious since there is lack of pilot-scale and industrial level adoption. Presently, studies on biochar for onsite sanitation including treatment of faecal sludge and greywater have been investigated using only laboratory-scale. The effects of biochar for onsite sanitation on the environment is not properly understood. The conditions of real environment are more complicated than the conditions created in the laboratory, resulting in high uncertainties in biochar application on the environment. Thus, more in situ experiments are required to ascertain the real effect of biochar on the environment before full-scale applications.
Physical and chemical modification which are predominantly used for biochar activation are energy intensive, expensive and are associated with environmental concerns. Thus, the design of low-cost, highly effective biochar-based adsorbent for on-site sanitation and faecal sludge treatment using biological modification needs to be explored. Due to its high surface area and porous structure, biochar can be employed as a scaffolding material for colonizing and growing biofilms. The microorganisms may adhere to the surface of biochar and develop an extracellular biofilm. In this modification system, while biochar adsorbs other contaminants such as heavy metals due to its porous structure, high surface area and different functional groups, the microorganisms will enhance the degradation of organic contaminants. This synergistic removal effect makes biologically modified biochar effective for on-site sanitation treatment systems. Simultaneously, both organic and inorganic pollutants can be removed via biodegradation and sorption processes, respectively.
Finally, the use of biochar for wastewater treatment is widely reported in the literature but its effect in mitigating antimicrobial resistance in household wastewater is largely unknown; the effect of biochar in mitigating the migration of antibiotic resistant genes (ARGs) is still unexplored. Emerging microbial pathogens with ARGs have become a major public health problem. These genes are dangerous because they can easily proliferate through horizontal gene transfer (unresistant bacteria obtain the necessary gene element from the mobile genetic element and become resistant) and when released into waterbodies/environment, they can alter the microbial community structure and in turn affect the quality of water (Feng et al., 2020). Thus, there is urgent need for detailed investigations to ascertain the potential of biochar in mitigating ARGs present in onsite wastewater. There is need to explore how intrinsic properties of biochar can influence its ability to remove ARGs in onsite wastewater. Also, modifications such as the use of transition metals to increase the amount of persistent free radicals (PFRs) present on biochar surface should be investigated.
8 Conclusion
A critical review of biochar production technologies, properties and its application for the treatment of onsite domestic wastewater is presented in this paper. It is observed that the application of biochar to onsite wastewater treatment systems brings about improvement in the treatment efficiency of these systems. The removal of different contaminants by biochar is achieved through the combination of mechanisms which include adsorption, ion exchange, filtration, electrostatic attraction, biodegradation and pore-filling. The synergistic removal of contaminants is brought about by the properties of biochar together with the microbes found in biofilm trapped on biochar surface. There are still other areas where further research is required in the application of biochar for the treatment of onsite domestic wastewater. There is need to research on the validation of standardized protocols for biochar production and scaling up of biochar production from laboratory scale to full-scale. Further research is also needed to enhance biochar properties and its subsequent potential for the removal of AMR/drug resistance and emerging pollutants in domestic wastewater.
Author contributions
CM: Conceptualization; methodology; software; formal analysis; investigation; writing-original draft preparation; visualization. PO: Conceptualization; methodology; software; formal analysis; investigation; writing-original draft preparation; visualization. AN: Writing-review and editing. SL: Formal analysis; investigation; writing-review and editing. SS: Formal analysis; investigation; writing-review and editing; visualization. OI: Conceptualization; formal analysis; investigation; writing-review and editing; visualization; supervision. MM: Conceptualization; methodology; software; formal analysis; investigation; resources; writing-original draft preparation; writing-review and editing; visualization; supervision; project administration. All authors read and approved the final manuscript.
Conflict of interest
The authors declare that the research was conducted in the absence of any commercial or financial relationships that could be construed as a potential conflict of interest.
Publisher’s note
All claims expressed in this article are solely those of the authors and do not necessarily represent those of their affiliated organizations, or those of the publisher, the editors and the reviewers. Any product that may be evaluated in this article, or claim that may be made by its manufacturer, is not guaranteed or endorsed by the publisher.
References
Abdel-Fattah, T. M., Mahmoud, M. E., Ahmed, S. B., Huff, M. D., Lee, J. W., and Kumar, S. (2014). Biochar from woody biomass for removing metal contaminants and carbon sequestration. J. Ind. Eng. Chem. 22, 103–109. doi:10.1016/j.jiec.2014.06.030
Abdel-Shafy, H. I., and El-Khateeb, M. A. (2013). Integration of septic tank and constructed wetland for the treatment of wastewater in Egypt. Desalin. Water Treat. 51, 3539–3546. doi:10.1080/19443994.2012.749585
Abit, S. M., Bolster, C. H., Cai, P., and Walker, S. L. (2012). Influence of feedstock and pyrolysis temperature of biochar amendments on transport of Escherichia coli in saturated and unsaturated soil. Environ. Sci. Technol. 46 (15), 8097–8105. doi:10.1021/es300797z
Acero, J. L., Benitez, F. J., Real, F. J., and Rodriguez, E. (2015). Elimination of selected emerging contaminants by the combination of membrane filtration and chemical oxidation processes. Water Air Soil Pollut. 226, 139. doi:10.1007/s11270-015-2404-8
Achaw, O., and Afrane, G. (2008). The evolution of the pore structure of coconut shells during the preparation of coconut shell-based activated carbons. Microporous Mesoporous Mater. 112, 284–290. doi:10.1016/j.micromeso.2007.10.001
Agoro, M. A., Okoh, O. O., Adefisoye, M. A., and Okoh, A. I. (2018). Physicochemical properties of wastewater in three typical South African sewage works. Pol. J. Environ. Stud. 27 (2), 491–499. doi:10.15244/pjoes/74156
Ahmad, M., Soo, S., Dou, X., Mohan, D., Sung, J., Yang, J. E., et al. (2012). Effects of pyrolysis temperature on soybean stover- and peanut shell-derived biochar properties and TCE adsorption in water. Bioresour. Technol. 118, 536–544. doi:10.1016/j.biortech.2012.05.042
Ahmed, M. B., Zhou, J. L., Ngo, H. H., Guo, W., and Chen, M. (2016). Progress in the preparation and application of modified biochar for improved contaminant removal from water and wastewater. Bioresour. Technol. 214, 836–851. doi:10.1016/j.biortech.2016.05.057
Ahmed, M. J., and Hameed, B. H. (2018). Adsorption behavior of salicylic acid on biochar as derived from the thermal pyrolysis of barley straws. J. Clean. Prod. 195, 1162–1169. doi:10.1016/j.jclepro.2018.05.257
Ahmedna, M., Marshall, W. E., Husseiny, A. A., Rao, R. M., and Goktepe, I. (2004). The use of nutshell carbons in drinking water filters for removal of trace metals. Water Res. 38, 1062–1068. doi:10.1016/j.watres.2003.10.047
Akpor, O. B., Ohiobor, G. O., and Olaolu, T. D. (2014). Heavy metal pollutants in wastewater effluents: Sources, effects and remediation. Adv. Biosci. Bioeng. 2, 37–43. doi:10.11648/j.abb.20140204.11
Akunna, J. C., O’Keeffe, J. M., and Allan, R. (2017). Reviewing factors affecting the effectiveness of decentralised domestic wastewater treatment systems for phosphorus and pathogen removal. Desalin. Water Treat. 91, 40–47. doi:10.5004/dwt.2017.20750
Ali, L., Palamanit, A., Techato, K., Baloch, K. A., and Jutidamrongphan, W. (2022b). Valorization of rubberwood sawdust and sewage sludge by pyrolysis and co-pyrolysis using agitated bed reactor for producing biofuel or value-added products. Environ. Sci. Pollut. Res. 29 (1), 1338–1363. doi:10.1007/s11356-021-15283-6
Ali, L., Palamanit, A., Techato, K., Ullah, A., Chowdhury, M. S., and Phoungthong, K. (2022a). Characteristics of biochars derived from the pyrolysis and Co-pyrolysis of rubberwood sawdust and sewage sludge for further applications. Sustainability 14 (7), 3829. doi:10.3390/su14073829
Ambaye, T. G., Vaccari, M., van Hullebusch, E. D., Amrane, A., and Rtimi, S. (2021). Mechanisms and adsorption capacities of biochar for the removal of organic and inorganic pollutants from industrial wastewater. Int. j. sci. environ. technol. 18 (10), 3273–3294.
Amin, N., Liu, P., Foster, T., Rahman, M., Miah, M. R., Ahmed, G. B., et al. (2020). Pathogen flows from on-site sanitation systems in low-income urban neighborhoods, dhaka: A quantitative environmental assessment. Int. J. Hyg. Environ. Health 230, 113619. doi:10.1016/j.ijheh.2020.113619
Anfar, Z., Zbair, M., Ait Ahsiane, H., Jada, A., and El Alem, N. (2020). Microwave assisted green synthesis of Fe2O3/biochar for ultrasonic removal of nonsteroidal anti-inflammatory pharmaceuticals. RSC Adv. 10 (19), 11371–11380. doi:10.1039/d0ra00617c
Aworn, A., Thiravetyan, P., and Nakbanpote, W. (2008). Preparation and characteristics of agricultural waste activated carbon by physical activation having micro- and mesopores. J. Anal. Appl. Pyrolysis 82 (2), 279–285. doi:10.1016/j.jaap.2008.04.007
Awuah, E., Amankwah-Kuffuor, R., Gyasi, S. F., Lubberding, H. J., and Gijzen, H. J. (2014). Characterization and management of domestic wastewater in two suburbs of kumasi, Ghana. Res. J. Environ. Sci. 8 (6), 318–330. doi:10.3923/rjes.2014.318.330
Azevedo, D. C. S., Araujo, J. C. S., Bastos-Neto, M., Torres, A. E. B., Jaguaribe, E. F., and Cavacante, C. L. (2007). Microporous activated carbon prepared from coconut shells using chemical activation with zinc chloride. Microporous Mesoporous Mater. 100, 361–364. doi:10.1016/j.micromeso.2006.11.024
Babcock, R. W., Lamichhane, K. M., Cummings, M. J., and Cheong, G. H. (2014). Condition assessment survey of onsite sewage disposal systems (OSDSs) in Hawaii. Water Sci. Technol. 70 (6), 1083–1089. doi:10.2166/wst.2014.336
Baccar, R., Sarrà, M., Bouzid, J., Feki, M., and Blánquez, P. (2012). Removal of pharmaceutical compounds by activated carbon prepared from agricultural by-product. Chem. Eng. J. 211, 310–317. doi:10.1016/j.cej.2012.09.099
Bagreev, A., Bandosz, T., and Locke, D. (2001). Pore structure and surface chemistry of adsorbents obtained by pyrolysis of sewage sludge-derived fertilizer. Carbon 39 (13), 1971–1979. doi:10.1016/s0008-6223(01)00026-4
Bangham, D. H., and Razouk, R. I. (1938). The swelling of charcoal Part V. The saturation and immersion expansions and the heat of wetting. Proc. R. Soc. Lond. 166 (927), 572–586. doi:10.1098/rspa.1938.0112
Bansal, R. C., Donnet, J. B., and Stoeckli, F. (1988). Active carbon. New York: Marcel Dekker). doi:10.1080/01932699008943255
Barrios, J. A., Cano, A., Becerril, J. E., and Jimenez, B. (2016). Influence of solids on the removal of emerging pollutants in electrooxidation of municipal sludge with boron-doped diamond electrodes. J. Electroanal. Chem. 776, 148–151. doi:10.1016/j.jelechem.2016.07.018
Behera, S. K., Kim, H. W., Oh, J. E., and Park, H. S. (2011). Occurrence and removal of antibiotics, hormones and several other pharmaceuticals in wastewater treatment plants of the largest industrial city of Korea. Sci. Total Environ. 409, 4351–4360. doi:10.1016/j.scitotenv.2011.07.015
Berger, C. (2012). Biochar and activated carbon filters for greywater treatment – comparison of organic matter and nutrients removal. Uppsala, Sweden: Swedish University of Agricultural Sciences.
Blum, K. M., Andersson, P. L., Renman, G., Ahrens, L., Gros, M., Wiberg, K., et al. (2017). Non-target screening and prioritization of potentially persistent, bioaccumulating and toxic domestic wastewater contaminants and their removal in on-site and large-scale sewage treatment plants. Sci. Total Environ. 575, 265–275. doi:10.1016/j.scitotenv.2016.09.135
Boano, F., Costamagna, E., Caruso, A., Fiore, S., Chiappero, M., Galvão, A., et al. (2021). Evaluation of the influence of filter medium composition on treatment performances in an open-air green wall fed with greywater. J. Environ. Manag. 300, 113646. doi:10.1016/j.jenvman.2021.113646
Bolton, L., Joseph, S., Greenway, M., Donne, S., Munroe, P., and Marjo, C. E. (2019). Phosphorus adsorption onto an enriched biochar substrate in constructed wetlands treating wastewater. Ecol. Eng. 142, 100005. doi:10.1016/j.ecoena.2019.100005
Bouchelta, C., Medjram, M. S., Bertrand, O., and Bellat, J. P. (2008). Preparation and characterization of activated carbon from date stones by physical activation with steam. J. Anal. Appl. Pyrolysis 82 (1), 70–77. doi:10.1016/j.jaap.2007.12.009
Boutilier, L., Jamieson, R., Gordon, R., Lake, C., and Hart, W. (2010). Performance of surface-flow domestic wastewater treatment wetlands. Wetlands 30, 795–804. doi:10.1007/s13157-010-0067-1
Brix, H., and Arias, C. A. (2005). The use of vertical flow constructed wetlands for on-site treatment of domestic wastewater: New Danish guidelines. Ecol. Eng. 25 (5), 491–500. doi:10.1016/j.ecoleng.2005.07.009
Cagnon, B., Py, X., Guillot, A., Stoeckli, F., and Chambat, G. (2009). Contributions of hemicellulose, cellulose and lignin to the mass and the porous properties of chars and steam activated carbons from various lignocellulosic precursors. Bioresour. Technol. 100, 292–298. doi:10.1016/j.biortech.2008.06.009
Cantrell, K. B., Hunt, P. G., Uchimiya, M., Novak, J. M., and Ro, K. S. (2012). Impact of pyrolysis temperature and manure source on physicochemical characteristics of biochar. Bioresour. Technol. 107, 419–428. doi:10.1016/j.biortech.2011.11.084
Capone, D., Barker, T., Cumming, O., Flemister, A., Geason, R., Kim, E., et al. (2022). Persistent Ascaris transmission is possible in urban areas even where sanitation coverage is high. Environ. Sci. Technol. 56 (22), 15969–15980. doi:10.1021/acs.est.2c04667
Chen, T., Zhang, Y. X., Wang, H. T., Lu, W. J., Zhou, Z. Y., Zhang, Y. C., et al. (2014). Influence of pyrolysis temperature on characteristics and heavy metal adsorptive performance of biochar derived from municipal sewage sludge. Bioresour. Technol. 164, 47–54. doi:10.1016/j.biortech.2014.04.048
Chen, X., Chen, G., Chen, L., Chen, Y., Lehmann, J., McBride, M. B., et al. (2011). Adsorption of copper and zinc by biochars produced from pyrolysis of hardwood and corn straw in aqueous solution. Bioresour. Technol. 102 (19), 8877–8884. doi:10.1016/j.biortech.2011.06.078
Cheng, N., Wang, B., Wu, P., Lee, X., Xing, Y., Chen, M., et al. (2021). Adsorption of emerging contaminants from water and wastewater by modified biochar: A review. Environ. Pollut. 273, 116448. doi:10.1016/j.envpol.2021.116448
Chino, M., Moriyama, K., Saito, H., and Morn, T. (1991). The amount of heavy metals derived from domestic sources in Japan. Water, air, soil Pollut. 57 (1), 829–837. doi:10.1007/bf00282946
Chun, Y., Sheng, G., Chiou, C. T., and Xing, B. (2004). Compositions and sorptive properties of crop residue-derived chars. Environ. Sci. Technol. 38, 4649–4655. doi:10.1021/es035034w
Dalahmeh, S. S., Jönsson, H., Hylander, L. D., Hui, N., Yu, D., and Pell, M. (2014). Dynamics and functions of bacterial communities in bark, charcoal and sand filters treating greywater. Water Res. 54, 21–32. doi:10.1016/j.watres.2014.01.019
Dalahmeh, S. S., Lalander, C., Pell, M., Vinnerås, B., and Jönsson, H. (2016). Quality of greywater treated in biochar filter and risk assessment of gastroenteritis due to household exposure during maintenance and irrigation. J. Appl. Microbiol. 121 (5), 1427–1443. doi:10.1111/jam.13273
de Letona Sánchez, M. L., Macias-Garcia, A., Díaz-Díez, M. A., Cuerda-Correa, E. M., Ganan-Gomez, J., and Nadal-Gisbert, A. (2006). Preparation of activated carbons previously treated with hydrogen peroxide: Study of their porous texture. Appl. Surf. Sci. 252 (17), 5984–5987. doi:10.1016/j.apsusc.2005.11.022
Demiral, H., and Gündüzog, G. (2010). Removal of nitrate from aqueous solutions by activated carbon prepared from sugar beet bagasse. Bioresour. Technol. 101, 1675–1680. doi:10.1016/j.biortech.2009.09.087
Demirbaş, A. (2001). Biomass resource facilities and biomass conversion processing for fuels and chemicals. Energy Convers. Manag. 42 (11), 1357–1378. doi:10.1016/s0196-8904(00)00137-0
Deng, S., Chen, J., and Chang, J. (2021). Application of biochar as an innovative substrate in constructed wetlands/biofilters for wastewater treatment: Performance and ecological benefits. J. Clean. Prod. 293, 126156. doi:10.1016/j.jclepro.2021.126156
Din, A. T. M., Hameed, B. H., and Ahmad, A. L. (2009). Batch adsorption of phenol onto physiochemical-activated coconut shell. J. Haz 161, 1522–1529. doi:10.1016/j.jhazmat.2008.05.009
Dowd, S. E., Pillai, S. D., Wang, S., and Corapcioglu, M. Y. (1998). Delineating the specific influence of virus isoelectric point and size on virus adsorption and transport through sandy soils. Appl. Environ. Microbiol. 64 (2), 405–410. doi:10.1128/aem.64.2.405-410.1998
El-Khateeb, A. Y., Elsherbiny, E. A., Tadros, L. K., Ali, S. M., and Hamed, H. B. (2013). Phytochemical analysis and antifungal activity of fruit leaves extracts on the mycelial growth of fungal plant pathogens. J. Plant Pathology Microbiol. 4 (9), 1–6. doi:10.4172/2157-7471.1000199
El-Shafey, E. I. (2010). Removal of Zn(II) and Hg(II) from aqueous solution on a carbonaceous sorbent chemically prepared from rice husk. J. Hazard Mater 175, 319–327. doi:10.1016/j.jhazmat.2009.10.006
Eregno, F. E., and Heistad, A. (2019). On-site treated wastewater disposal systems—the role of stratified filter medias for reducing the risk of pollution. Environ. Int. 124, 302–311. doi:10.1016/j.envint.2019.01.008
Esmaeeli, F., Gorbanian, S., and Moazezi, N. (2017). Removal of estradiol valerate and progesterone using powdered and granular activated carbon from aqueous solutions. Int. J. Environ. Res. 11, 695–705. doi:10.1007/s41742-017-0060-0
Fakkaew, K., Koottatep, T., and Polprasert, C. (2018). Faecal sludge treatment and utilization by hydrothermal carbonization. J. Environ. Manag. 216, 421–426. doi:10.1016/j.jenvman.2017.09.031
Falabi, J. A., Gerba, C. P., and Karpiscak, M. M. (2002). Giardia and Cryptosporidium removal from wastewater by a duckweed (Lemna gibba L.) covered pond. Lett. Appl. Microbiol. 34, 384–387. doi:10.1046/j.1472-765x.2002.01104.x
Farkas, K., Walker, D. I., Adriaenssens, E. M., McDonald, J. E., Hillary, L. S., Malham, S. K., et al. (2020). Viral indicators for tracking domestic wastewater contamination in the aquatic environment. Water Res. 181, 115926. doi:10.1016/j.watres.2020.115926
Faulwetter, J. L., Gagnon, V., Sundberg, C., Chazarenc, F., Burr, M. D., Brisson, J., et al. (2009). Microbial processes influencing performance of treatment wetlands: A review. Ecol. Eng. 35, 987–1004. doi:10.1016/j.ecoleng.2008.12.030
Feng, D., Guo, D., Zhang, Y., Sun, S., Zhao, Y., Shang, Q., et al. (2020). Functionalized construction of biochar with hierarchical pore structures and surface O-/N-containing groups for phenol adsorption. Chem. Eng. J. 410, 127707. doi:10.1016/j.cej.2020.127707
Ferreira, L., Rosales, E., Danko, A. S., Sanroman, M. A., and Pazos, M. M. (2016). Bacillus thuringiensis a promising bacterium for degrading emerging pollutants. Process Saf. Environ. Prot. 101, 19–26. doi:10.1016/j.psep.2015.05.003
Forbis-Stokes, A. A., Rocha-Melogno, L., and Deshusses, M. A. (2018). Nitrifying trickling filters and denitrifying bioreactors for nitrogen management of high-strength anaerobic digestion effluent. Chemosphere 204, 119–129. doi:10.1016/j.chemosphere.2018.03.137
Fu, M., Mo, C., Li, H., Zhang, Y., Huang, W., and Wong, M. H. (2018). Comparison of physicochemical properties of biochars and hydrochars produced from food wastes. J. Clean. Prod. 236, 117637. doi:10.1016/j.jclepro.2019.117637
Gañán, J., González, J. F., González-García, C. M., Ramiro, A., Sabio, E., and Román, S. (2006). Carbon dioxide-activated carbons from almond tree pruning: Preparation and characterization. Appl. Surf. Sci. 252 (17), 5993–5998. doi:10.1016/j.apsusc.2005.11.025
García, J. A., Paredes, D., and Cubillos, J. A. (2013). Effect of plants and the combination of wetland treatment type systems on pathogen removal in tropical climate conditions. Ecol. Eng. 58, 57–62. doi:10.1016/j.ecoleng.2013.06.010
Garcia, M. L., Lapa, K. R., Foresti, E., and Zaiat, M. (2008). Effects of bed materials on the performance of an anaerobic sequencing batch biofilm reactor treating domestic sewage. J. Environ. Manage. 88 (4), 1471–1477. doi:10.1016/j.jenvman.2007.07.015
Gill, L. W., O’Luanaigh, N., Johnston, P. M., Misstear, B. D. R., and O’Suilleabhain, C. (2009). Nutrient loading on subsoils from on-site wastewater effluent, comparing septic tank and secondary treatment systems. Water Res. 43 (10), 2739–2749. doi:10.1016/j.watres.2009.03.024
Gopinath, A., Divyapriya, G., Srivastava, V., Laiju, A. R., Nidheesh, P. V., and Kumar, M. S. (2021). Conversion of sewage sludge into biochar: A potential resource in water and wastewater treatment. Environ. Res. 194, 110656. doi:10.1016/j.envres.2020.110656
Gros, M., Blum, K. M., Jernstedt, H., Renman, G., Rodriguez-Mozaz, S., Haglund, P., et al. (2016). Screening and prioritization of micropollutants in wastewaters from on-site sewage treatment facilities. J. Hazard. Mater. 328, 37–45. doi:10.1016/j.jhazmat.2016.12.055
Gul, S., Whalen, J. K., Thomas, B. W., Sachdeva, V., and Deng, H. (2015). Physico-chemical properties and microbial responses in biochar-amended soils: Mechanisms and future directions. Agric. Ecosyst. Environ. 206, 46–59. doi:10.1016/j.agee.2015.03.015
Gungor, K., and Unlu, K. (2005). Nitrite and nitrate removal efficiencies of soil aquifer treatment columns. Turkish J. Eng. Environ. Sci. 29, 159–170.
Guo, F., Zhang, J., Yang, X., He, Q., Ao, L., and Chen, Y. (2020). Impact of biochar on greenhouse gas emissions from constructed wetlands under various influent chemical oxygen demand to nitrogen ratios. Bioresour. Technol. 303, 122908. doi:10.1016/j.biortech.2020.122908
Gupta, P., Tae-woong, A., and Lee, S.-M. (2016). Use of biochar to enhance constructed wetland performance in wastewater reclamation. Environ. Eng. Res. 21 (1), 36–44. doi:10.4491/eer.2015.067
Guruge, K. S., Goswami, P., Tanoue, R., Nomiyama, K., Wijesekara, R. G. S., and Dharmaratne, T. S. (2019). First nationwide investigation and environmental risk assessment of 72 pharmaceuticals and personal care products from Sri Lankan surface waterways. Sci. Total Environ. 690, 683–695. doi:10.1016/j.scitotenv.2019.07.042
Gwenzi, W., Chaukura, N., Noubactep, C., and Mukome, F. N. D. (2017). Biochar-based water treatment systems as a potential low-cost and sustainable technology for clean water provision. J. Environ. Manag. 197, 732–749. doi:10.1016/j.jenvman.2017.03.087
Hao, F., Zhao, X., Ouyang, W., Lin, C., Chen, S., Shan, Y., et al. (2013). Molecular structure of corncob-derived biochars and the mechanism of atrazine sorption. Agron. J. 105 (3), 773–782. doi:10.2134/agronj2012.0311
Homagain, K., Shahi, C., Luckai, N., and Sharma, M. (2016). Life cycle cost and economic assessment of biochar-based bioenergy production and biochar land application in Northwestern Ontario, Canada. For. Ecosyst. 1, 21–10. doi:10.1186/s40663-016-0081-8
Horn, T. B., Zerwes, F. V., Kist, L. T., and Machado, Ê. L. (2014). Constructed wetland and photocatalytic ozonation for University sewage treatment. Ecol. Eng. 63, 134–141. doi:10.1016/j.ecoleng.2013.12.012
Hossain, M. K., Strezov Vladimir, V., Chan, K. Y., Ziolkowski, A., and Nelson, P. F. (2011). Influence of pyrolysis temperature on production and nutrient properties of wastewater sludge biochar. J. Environ. Manag. 92, 223–228. doi:10.1016/j.jenvman.2010.09.008
Humphries, B., Weaver, L., Burbery, L., Webber, J., Morgan, L., and Gregor, J. (2020). Microbial pathogen removal from domestic effluent using coral sand in Kiribati. J. Appl. Microbiol. 128, 1208–1220. doi:10.1111/jam.14555
Hussain, A., Janson, A., Matar, J. M., and Adham, S. (2021). Membrane distillation: Recent technological developments and advancements in membrane materials. Emergent Mater. 5, 347–367. doi:10.1007/s42247-020-00152-8
Intani, K., Latif, S., Cao, Z., and Müller, J. (2018). Characterisation of biochar from maize residues produced in a self-purging pyrolysis reactor. Bioresour. Technol. 265, 224–235. doi:10.1016/j.biortech.2018.05.103
Jefferson, B., Burgess, J. E., Pichon, A., Harkness, J., and Judd, S. J. (2001). Nutrient addition to enhance biological treatment of greywater. Water Res. 35 (11), 2702–2710. doi:10.1016/S0043-1354(00)00553-4
Ji, B., Chen, J., Mei, J., Chang, J., Li, X., Jia, W., et al. (2020). Roles of biochar media and oxygen supply strategies in treatment performance, greenhouse gas emissions, and bacterial community features of subsurface-flow constructed wetlands. Bioresour. Technol. 302, 122890. doi:10.1016/j.biortech.2020.122890
Jia, L., Liu, H., Kong, Q., Li, M., Wu, S., and Wu, H. (2020). Interactions of high-rate nitrate reduction and heavy metal mitigation in iron-carbon-based constructed wetlands for purifying contaminated groundwater. Water Res. 169, 115285. doi:10.1016/j.watres.2019.115285
Jiang, N., Shang, R., Heijman, S. G. J., and Rietveld, L. C. (2020). Adsorption of triclosan, trichlorophenol and phenol by high-silica zeolites: Adsorption efficiencies and mechanisms. Separ. Purif. Technol. 235, 116152. doi:10.1016/j.seppur.2019.116152
Jin, P., Jin, X., Wang, X., Feng, Y., and C, X. (2013). “Biological activated carbon treatment process for advanced water and wastewater treatment,” in Biomass now: Cultivation and utilization, 153–192. doi:10.5772/52021
Jung, C., Park, J., Lim, K. H., Park, S., Heo, J., Her, N., et al. (2013). Adsorption of selected endocrine disrupting compounds and pharmaceuticals on activated biochars. J. Hazard. Mater. 263, 702–710. doi:10.1016/j.jhazmat.2013.10.033
Kaetzl, K., Lübken, M., Uzun, G., Gehring, T., Nettmann, E., Stenchly, K., et al. (2019). On-farm wastewater treatment using biochar from local agro-residues reduces pathogens from irrigation water for safer food production in developing countries. Sci. Total Environ. 682, 601–610. doi:10.1016/j.scitotenv.2019.05.142
Kaliakatsos, A., Kalogerakis, N., Manios, T., and Venieri, D. (2019). Efficiency of two constructed wetland systems for wastewater treatment: Removal of bacterial indicators and enteric viruses. J. Chem. Technol. Biotechnol. 94, 6001–2130. doi:10.1002/jctb.6001
Kambo, H. S., and Dutta, A. (2015). A comparative review of biochar and hydrochar in terms of production, physico-chemical properties and applications. Renew. Sustain. Energy Rev. 45, 359–378. doi:10.1016/j.rser.2015.01.050
Karim, M. R., Manshadi, F. D., Karpiscak, M. M., and Gerba, C. P. (2004). The persistence and removal of enteric pathogens in constructed wetlands. Water Res. 38, 1831–1837. doi:10.1016/j.watres.2003.12.029
Karunanayake, A. G., Todd, O. A., Crowley, M. L., Ricchetti, L. B., Pittman, C. U., Anderson, R., et al. (2017). Rapid removal of salicylic acid, 4-nitroaniline, benzoic acid and phthalic acid from wastewater using magnetized fast pyrolysis biochar from waste Douglas fir. Chem. Eng. J. 319, 75–88. doi:10.1016/j.cej.2017.02.116
Kasozi, G. N., Zimmerman, A. R., Nkedi-Kizza, P., and Gao, B. (2010). Catechol and humic acid sorption onto a range of laboratory-produced black carbons (Biochars). Environ. Sci. Technol. 44 (16), 6189–6195. doi:10.1021/es1014423
Kasprzyk-Hordern, B., Dinsdale, R. M., and Guwy, A. J. (2009). The removal of pharmaceuticals, personal care products, endocrine disruptors and illicit drugs during wastewater treatment and its impact on the quality of receiving waters. Water Res. 43, 363–380. doi:10.1016/j.watres.2008.10.047
Kauppinen, A., Martikainen, K., Matikka, V., Veijalainen, A. M., Pitkänen, T., Heinonen-Tanski, H., et al. (2014). Sand filters for removal of microbes and nutrients from wastewater during a one-year pilot study in a cold temperate climate. J. Environ. Manag. 133, 206–213. doi:10.1016/j.jenvman.2013.12.008
Kholoma, E., Renman, G., and Renman, A. (2016). Phosphorus removal from wastewater by field-scale fortified filter beds during a one-year study. Environ. Technol. 37 (23), 2953–2963. doi:10.1080/09593330.2016.1170888
Kizito, S., Luo, H., Lu, J., Bah, H., Dong, R., and Wu, S. (2019). Role of nutrient-enriched biochar as a soil amendment during maize growth: Exploring practical alternatives to recycle agricultural residuals and to reduce chemical fertilizer demand. Sustainability 11 (11), 3211. doi:10.3390/su11113211
Kizito, S., Wu, S., Kirui, W. K., Lei, M., Lu, Q., Bah, H., et al. (2015). Evaluation of slow pyrolyzed wood and rice husks biochar for adsorption of ammonium nitrogen from piggery manure anaerobic digestate slurry. Sci. Total Environ. 505, 102–112. doi:10.1016/j.scitotenv.2014.09.096
Kołodyńska, D., Wnętrzak, R., Leahy, J. J., Hayes, M. H. B., Kwapiński, W., and Hubicki, Z. (2012). Kinetic and adsorptive characterization of biochar in metal ions removal. Chem. Eng. J. 197, 295–305. doi:10.1016/j.cej.2012.05.025
Kołtowski, M., Hilber, I., Bucheli, T. D., Charmas, B., Skubiszewska-Zięba, J., and Oleszczuk, P. (2017). Activated biochars reduce the exposure of polycyclic aromatic hydrocarbons in industrially contaminated soils. Chem. Eng. J. 310, 33–40. doi:10.1016/j.cej.2016.10.065
Konczak, M., Oleszczuk, P., and Rozyło, K. (2019). Application of different carrying gases and ratio between sewage sludge and willow for engineered (smart) biochar production. J. CO2Util. 29, 20–28. doi:10.1016/j.jcou.2018.10.019
Krueger, B. C., Fowler, G. D., Templeton, M. R., and Moya, B. (2020). Resource recovery and biochar characteristics from full-scale faecal sludge treatment and co-treatment with agricultural waste. Water Res. 169, 115253. doi:10.1016/j.watres.2019.115253
Lalander, C., Dalahmeh, S., Jönsson, H., and Vinnerås, B. (2013). Hygienic quality of artificial greywater subjected to aerobic treatment: A comparison of three filter media at increasing organic loading rates. Environ. Technol. 34 (18), 2657–2662. doi:10.1080/09593330.2013.783603
Lalhruaitluanga, H., Jayaram, K., Prasad, M. N. V., and Kumar, K. K. (2010). Lead (II) adsorption from aqueous solutions by raw and activated charcoals of melocanna baccifera roxburgh (bamboo)—A comparative study. J. Hazard. Mater. 175 (1-3), 311–318. doi:10.1016/j.jhazmat.2009.10.005
Lamichhane, K. M. (2007). On-site sanitation: A viable alternative to modern wastewater treatment plants. Water Sci. Technol. 55 (1–2), 433–440. doi:10.2166/wst.2007.044
Lau, A. Y., Tsang, D. C., Graham, N. J., Ok, Y. S., Yang, X., and Li, X. D. (2017). Surface-modified biochar in a bioretention system for Escherichia coli removal from stormwater. Chemosphere 169, 89–98. doi:10.1016/j.chemosphere.2016.11.048
Li, F., Cao, X., Zhao, L., Wang, J., and Ding, Z. (2014). Effects of mineral additives on biochar formation: carbon retention, stability, and properties. Environ. Sci. Tech. 48 (19), 11211–11217.
Li, F., Shen, K., Long, X., Wen, J., Xie, X., Zeng, X., et al. (2016). Preparation and characterization of biochars from eichornia crassipes for cadmium removal in aqueous solutions. PLoS ONE 11 (2), e0148132–e0148139. doi:10.1371/journal.pone.0148132
Li, H., Dong, X., da Silva, E. B., de Oliveira, L. M., Chen, Y., and Ma, L. Q. (2017). Mechanisms of metal sorption by biochars: Biochar characteristics and modifications. Chemosphere 178, 466–478. doi:10.1016/j.chemosphere.2017.03.072
Li, W., Yang, K., Peng, J., Zhang, L., Guo, S., and Xia, H. (2008). Effects of carbonization temperatures on characteristics of porosity in coconut shell chars and activated carbons derived from carbonized coconut shell chars. Industrial Crops Prod. 8, 190–198. doi:10.1016/j.indcrop.2008.02.012
Li, Y., Xu, D., Guan, Y., Yu, K., and Wang, W. (2019). Phosphorus sorption capacity of biochars from different waste woods and bamboo. Int. J. Phytoremediation 21 (2), 145–151. doi:10.1080/15226514.2018.1488806
Liang, Y., Wang, Q., Huang, L., Liu, M., Wang, N., and Chen, Y. (2020). Insight into the mechanisms of biochar addition on pollutant removal enhancement and nitrous oxide emission reduction in subsurface flow constructed wetlands: Microbial community structure, functional genes and enzyme activity. Bioresour. Technol. 307, 123249. doi:10.1016/j.biortech.2020.123249
Liu, W., Jiang, H., and Yu, H. (2015). Development of biochar-based functional materials: Toward a sustainable platform carbon material. Chem. Rev. 115, 12251–12285. doi:10.1021/acs.chemrev.5b00195
Liu, Y., Blowes, D. W., Ptacek, C. J., and Groza, L. G. (2019). Removal of pharmaceutical compounds, artificial sweeteners, and perfluoroalkyl substances from water using a passive treatment system containing zero-valent iron and biochar. Sci. Total Environ. 691, 165–177. doi:10.1016/j.scitotenv.2019.06.450
Liu, P., Liu, W., Jiang, H., Chen, J., Li, W., and Yu, H. (2012). Modification of bio-char derived from fast pyrolysis of biomass and its application in removal of tetracycline from aqueous solution. Bioresour. Technol. 121, 235–240. doi:10.1016/j.biortech.2012.06.085
Liyanage, A. S., Canaday, S., Pittman, C. U., and Mlsna, T. (2020). Rapid remediation of pharmaceuticals from wastewater using magnetic Fe3O4/Douglas fir biochar adsorbents. Chemosphere 258, 127336. doi:10.1016/j.chemosphere.2020.127336
Lowe, K. S., Tucholke, M. B., Tomaras, J. M. B., Conn, K., Hoppe, C., Drewes, J. E., et al. (2009). Influent constituent characteristics of the modern waste stream from single source. Alexandria, VA, United States: WERF and IWA publishers. doi:10.2166/9781780403519
Lu, K., Yang, X., Gielen, G., Bolan, N., Ok, Y. S., Niazi, N. K., et al. (2017). Effect of bamboo and rice straw biochars on the mobility and redistribution of heavy metals (Cd, Cu, Pb and Zn) in contaminated soil. J. Environ. Manag. 186, 285–292. doi:10.1016/j.jenvman.2016.05.068
Lu, H., Zhang, W., Yang, Y., Huang, X., Wang, S., Qiu, R., et al. (2012). Relative distribution of Pb2+ sorption mechanisms by sludge-derived biochar. Water Res. 46, 854–862.
Lusk, M. G., Toor, G. S., Yang, Y. Y., Mechtensimer, S., De, M., and Obreza, T. A. (2017). A review of the fate and transport of nitrogen, phosphorus, pathogens, and trace organic chemicals in septic systems. Crit. Rev. Environ. Sci. Technol. 47 (7), 455–541. doi:10.1080/10643389.2017.1327787
Manga, M., Bartram, J., and Evans, B. E. (2020). Economic cost analysis of low-cost sanitation technology options in informal settlement areas (case study: Soweto, Johannesburg). Int. J. Hyg. Environ. Health 223, 289–298. doi:10.1016/j.ijheh.2019.06.012
Manga, M., Camargo-Valero, M. A., and Evans, B. E. (2019). Inactivation of viable Ascaris eggs during faecal sludge co-composting with chicken feathers and market waste. Desalination Water Treat. 163, 347–357. doi:10.5004/dwt.2019.24494
Manga, M., Evans, B. E., Camargo-Valero, M. A., and Horan, N. J. (2016). Effect of filter media thickness on the performance of sand drying beds used for faecal sludge management. Water Sci. Technol. 74, 2795–2806. doi:10.2166/wst.2016.451
Manga, M., Evans, B. E., Ngasala, T. M., and Camargo-Valero, M. A. (2022b). Recycling of faecal sludge: Nitrogen, carbon and organic matter transformation during Co-composting of faecal sludge with different bulking agents. Int. J. Environ. Res. Public Health 19 (17), 10592. doi:10.3390/ijerph191710592
Manga, M., Kolsky, P., Rosenboom, J. W., Ramalingam, S., Sriramajayam, L., Bartram, J., et al. (2022a). Public health performance of sanitation technologies in Tamil Nadu, India: Initial perspectives based on E. coli release. Int. J. Hyg. Environ. Health 243, 113987. doi:10.1016/j.ijheh.2022.113987
Manga, M., Muoghalu, C., Camargo-Valero, M. A., and Evans, B. E. (2023). Effect of turning frequency on the survival of fecal indicator microorganisms during aerobic composting of fecal sludge with sawdust. Int. J. Environ. Res. Public Health 20 (3), 2668. doi:10.3390/ijerph20032668
Manga, M. (2017). The feasibility of Co-composting as an upscale treatment method for faecal sludge in urban Africa. Leeds, UK: School of Civil Engineering, University of Leeds. Available online: https://etheses.whiterose.ac.uk/16997 (accessed on June 3, 2022).
Martikainen, K., Veijalainen, A.-M., Torvinen, E., Heinonen-Tanski, H., Kauppinen, A., Pitkänen, T., et al. (2018). Efficiency of private household sand filters in removing nutrients and microbes from wastewater in Finland. Water 10 (8), 1000. doi:10.3390/w10081000
Mburu, N., Tebitendwa, S. M., van Bruggen, J. J. A., Rousseau, D. P. L., and Lens, P. N. L. (2013). Performance comparison and economics analysis of waste stabilization ponds and horizontal subsurface flow constructed wetlands treating domestic wastewater: A case study of the juja sewage treatment works. J. Environ. Manag. 128, 220–225. doi:10.1016/j.jenvman.2013.05.031
Merrikhpour, H., and Jalali, M. (2012). Waste calcite sludge as an adsorbent for the removal of cadmium, copper, lead, and zinc from aqueous solutions. Clean Technol. Environ. Policy 14 (5), 845–855. doi:10.1007/s10098-012-0450-0
Miège, C., Choubert, J. M., Ribeiro, L., Eusèbe, M., and Coquetry, M. (2009). Fate of pharmaceuticals and personal care products in wastewater treatment plants–conception of a database and first results. Environ. Pollut. 157, 1721–1726. doi:10.1016/j.envpol.2008.11.045
Moges, M. E., Eregno, F. E., and Heistad, A. (2015). Performance of biochar and filtralite as polishing step for on-site greywater treatment plant. Manag. Environ. Qual. Int. J. 26 (4), 607–625. doi:10.1108/meq-07-2014-0101
Mohan, D., Rajput, S., Singh, V. K., Steele, P. H., and Pittman, C. U. (2011). Modeling and evaluation of chromium remediation from water using low cost bio-char, a green adsorbent. J. Hazard. Mater. 188 (1–3), 319–333. doi:10.1016/j.jhazmat.2011.01.127
Morató, J., Codony, F., Sánchez, O., Pérez, L. M., García, J., and Mas, J. (2014). Key design factors affecting microbial community composition and pathogenic organism removal in horizontal subsurface flow constructed wetlands. Sci. Total Environ. 481, 81–89. doi:10.1016/j.scitotenv.2014.01.068
Mwenge, P., and Seodigeng, T. (2019). Greywater treatment using activated biochar produced from agricultural waste. Int. J. Chem. Mol. Eng. 13 (3), 140–145.
Ndung’u, P. W. (2020). Adsorptive ability of banana stalks biochar in greywater remediation for reuse: A case of university of embu. Kenya: University of Embu.
Nguyen, T. H., Cho, H. H., Poster, D. L., and Ball, W. P. (2007). Evidence for a pore-filling mechanism in the adsorption of aromatic hydrocarbons to a natural wood char. Environ. Sci. Technol. 41 (4), 1212–1217. doi:10.1021/es0617845
Nicholas, H. L., Mabbett, I., Apsey, H., and Robertson, I. (2022). Physico-chemical properties of waste derived biochar from community scale faecal sludge treatment plants. Gates Open Res. 6 (96), 96. doi:10.12688/gatesopenres.13727.1
Niwagaba, C. B. ., Dinno, P., Wamala, I., Dalahmeh, S. . S., Lalander, C., and Jonsson, H. (2014). Experiences on the implementation of a pilot grey water treatment and reuse based system at a household in the slum of Kyebando-Kisalosalo, Kampala. J. Water Reuse Desalination 4, 294–307. doi:10.2166/wrd.2014.016
Oh, T. K., Choi, B., Shinogi, Y., and Chikushi, J. (2012). Effect of pH conditions on actual and apparent fluoride adsorption by biochar in aqueous phase. Water, Air, Soil Pollut. 223, 3729–3738. doi:10.1007/s11270-012-1144-2
Oladoja, N. A., and Ademoroti, C. M. A. (2006). The use of fortified soil-clay as on-site system for domestic wastewater purification. Water Res. 40 (3), 613–620. doi:10.1016/j.watres.2005.11.031
Oteng-Peprah, M., Acheampong, M. A., and DeVries, N. K. (2018). Greywater characteristics, treatment systems, reuse strategies and user perception—A review. Water, Air, & Soil Pollut. 229 (256), 1–6. doi:10.1007/s11270-018-3909-8
Parr, S. W., Hopkins, H. C., and Mitchell, D. R. (1931). Fusain. Ind. Eng. Chem., Anal. Ed. 3 (1), 64–65.
Pan, X., Gu, Z., Chen, W., and Li, Q. (2021). Preparation of biochar and biochar composites and their application in a fenton-like process for wastewater decontamination: A review. Sci. Total Environ. 754, 142104. doi:10.1016/j.scitotenv.2020.142104
Parshetti, G. K., Chowdhury, S., and Balasubramanian, R. (2014). Hydrothermal conversion of urban food waste to chars for removal of textile dyes from contaminated waters. Bioresour. Technol. 161, 310–319. doi:10.1016/j.biortech.2014.03.087
Patnukao, P., and Pavasant, P. (2008). Activated carbon from Eucalyptus camaldulensis Dehn bark using phosphoric acid activation. Bioresour. Technol. 99 (17), 8540–8543. doi:10.1016/j.biortech.2006.10.049
Peng, P., Lang, Y., and Wang, X. (2016). Adsorption behavior and mechanism of pentachlorophenol on reed biochars: pH effect pyrolysis temperature, hydrochloric acid treatment and isotherms. Ecol. Eng. 90, 225–233. doi:10.1016/j.ecoleng.2016.01.039
Peng, S. S., Zhang, D. Q., Huang, H. H., Jin, Z. J., and Peng, X. H. (2019). Ionic polyacrylamide hydrogel improved by graphene oxide for efficient adsorption of methylene blue. Res. Chem. Intermed. 45, 1545–1563. doi:10.1007/s11164-018-3689-1
Perez-Mercado, L. F., Lalander, C., Berger, C., and Dalahmeh, S. S. (2018). Potential of biochar filters for onsite wastewater treatment: Effects of biochar type, physical properties and operating conditions. Water 10 (12), 1835. doi:10.3390/w10121835
Perez-Mercado, L. F., Lalander, C., Joel, A., Ottoson, J., Dalahmeh, S., and Vinnerås, B. (2019). Biochar filters as an on-farm treatment to reduce pathogens when irrigating with wastewater-polluted sources. J. Environ. Manage. 248, 1–10.
Petrov, N., Budinova, T., Razvigorova, M., Parra, J., and Galiatsatou, P. (2008). Conversion of olive wastes to volatiles and carbon adsorbents. Biomass Bioenergy 32 (12), 1303–1310. doi:10.1016/j.biombioe.2008.03.009
Pignatello, J., Kwon, S., and Lu, Y. (2006). Effect of natural organic substances on the surface and adsorptive properties of environmental black carbon (char): Attenuation of surface activity by humic and fulvic acids. Environ. Sci. Technol. 40 (24), 7757–7763. doi:10.1021/es061307m
Pipíška, M., Krajčíková, E. K., Hvostik, M. V., Ďuriška, L., Černičková, I., Kaňuchová, M., et al. (2022). Biochar from wood chips and corn cobs for adsorption of thioflavin T and erythrosine B. Materials 15 (4), 1492. doi:10.3390/ma15041492
Pishgar, R., Morin, D., Young, S. J., Schwartz, J., and Chu, A. (2021). Characterization of domestic wastewater released from ‘green’ households and field study of the performance of onsite septic tanks retrofitted into aerobic bioreactors in cold climate. Sci. Total Environ. 755, 142446. doi:10.1016/j.scitotenv.2020.142446
Qian, L., and Chen, B. (2013). Dual role of biochars as adsorbents for aluminum: The effects of oxygen-containing organic components and the scattering of silicate particles. Environ. Sci. Technol. 47, 8759–8768. doi:10.1021/es401756h
Qu, R. J., Li, C. G., Liu, J. Q., Xiao, R. Y., Pan, X. X., Zeng, X. L., et al. (2018). Hydroxyl radical based photocatalytic degradation of halogenated organic contaminants and paraffin on silica gel. Environ. Sci. Technol. 52, 7220–7229. doi:10.1021/acs.est.8b00499
Quiñónez-Díaz, M. D. J., Karpiscak, M. M., Ellman, E. D., and Gerba, C. P. (2001). Removal of pathogenic and indicator microorganisms by a constructed wetland receiving untreated domestic wastewater. J. Environ. Sci. 36, 1311–1320. doi:10.1081/ese-100104880
Quispe, J. B., Campos, L. C., Mašek, O., and Bogush, A. (2022). Use of biochar-based column filtration systems for greywater treatment: A systematic literature review. J. Water Process Eng. 48, 102908. doi:10.1016/j.jwpe.2022.102908
Qurat-ul-Ain, Q., Shehzad, R. A., Yaqoob, U., Sharif, A., Sajid, Z., Rafiq, S., et al. (2021). Designing of benzodithiophene acridine based Donor materials with favorable photovoltaic parameters for efficient organic solar cell. Comput. Theor. Chem. 1200, 113238. doi:10.1016/j.comptc.2021.113238
Rahman, S., Navarathna, C. M., Krishna, N., Alchouron, J., Reneau, P., Stokes, S., et al. (2021). High capacity aqueous phosphate reclamation using Fe/Mg-layered double hydroxide (LDH) dispersed on biochar. J. Colloid Interface Sci. 597, 182–195. doi:10.1016/j.jcis.2021.03.114
Regmi, P., Moscoso, J. L. G., Kumar, S., Cao, X., Mao, J., and Schafran, G. (2012). Removal of copper and cadmium from aqueous solution using switchgrass biochar produced via hydrothermal carbonization process. J. Environ. Manag. 109, 61–69. doi:10.1016/j.jenvman.2012.04.047
Reinoso, R., Torres, L. A., and Bécares, E. (2008). Efficiency of natural systems for removal of bacteria and pathogenic parasites from wastewater. Sci. Total Environ. 395, 80–86. doi:10.1016/j.scitotenv.2008.02.039
Román, S., González, J. F., González-García, C. M., and Zamora, F. (2008). Control of pore development during CO2 and steam activation of olive stones. Fuel Process. Technol. 89 (8), 715–720. doi:10.1016/j.fuproc.2007.12.015
Sajjadi, B., Chen, W. Y., and Egiebor, N. O. (2019). A comprehensive review on physical activation of biochar for energy and environmental applications. Rev. Chem. Eng. 35 (6), 735–776.
Saltalı, K., Sarı, A., and Aydın, M. (2007). Removal of ammonium ion from aqueous solution by natural Turkish (Yıldızeli) zeolite for environmental quality. J. Hazard. Mater. 141, 258–263. doi:10.1016/j.jhazmat.2006.06.124
Santos, J. L., Aparicio, I., Callejon, M., and Alonso, E. (2009). Occurrence of pharmaceutically active compounds during 1-year period in wastewaters from four wastewater treatment plants in Seville (Spain). J. Hazard. Mater 164, 1509–1516. doi:10.1016/j.jhazmat.2008.09.073
Schaider, L. A., Balan, S. A., Blum, A., Andrews, D. Q., Strynar, M. J., Dickinson, M. E., et al. (2017). Fluorinated compounds in US fast food packaging. Environ. Sci. Technol. Lett. 4 (3), 105–111. doi:10.1021/acs.estlett.6b00435
Semiyaga, S., Nakagiri, A., Niwagaba, C. B., and Manga, M. (2022). “Application of anaerobic digestion in decentralized faecal sludge treatment plants,” in Anaerobic biodigesters for human waste treatment (Singapore: Springer), 263–281. doi:10.1007/978-981-19-4921-0_14
Shen, Y., and Zhang, N. (2019). Facile synthesis of porous carbons from silica-rich rice husk char for volatile organic compounds (VOCs) sorption. Bioresour. Technol. 282, 294–300. doi:10.1016/j.biortech.2019.03.025
Sidibe, M. (2014). Comparative study of bark, bio-char, activated charcoal filters for upgrading greywater from a hygiene aspect. Uppsala, Sweden: Swedish University of Agricultural Sciences.
Sim, W. J., Lee, J. W., Lee, E. S., Shin, S. K., Hwang, S. R., and Oh, J. E. (2011). Occurrence and distribution of pharmaceuticals in wastewater from households, livestock farms, hospitals and pharmaceutical manufactures. Chemosphere 82, 179–186. doi:10.1016/j.chemosphere.2010.10.026
Simpson, D. R. (2008). Biofilm processes in biologically active carbon water purification. Water Res. 42, 2839–2848. doi:10.1016/j.watres.2008.02.025
Singh, K. P., Malik, A., Sinha, S., and Ojha, P. (2008). Liquid-phase adsorption of phenols using activated carbons derived from agricultural waste material. J. Hazard. Mater. 150, 626–641. doi:10.1016/j.jhazmat.2007.05.017
Spokas, K. A., Novak, J. M., Masiello, C. A., Johnson, M. G., Colosky, E. C., Ippolito, J. A., et al. (2014). Physical disintegration of biochar: An overlooked process. Environ. Sci. Technol. Lett. 1 (8), 326–332. doi:10.1021/ez500199t
Stott, R., May, E., and Mara, D. D. (2003). Parasite removal by natural wastewater treatment systems: Performance of waste stabilisation ponds and constructed wetlands. Water Sci. Technol. 48, 97–104. doi:10.2166/wst.2003.0095
Strande, L., Ronteltap, M., and Brdjanovic, D. (2014). Faecal sludge management: Systems approach for implementation and operation. London: IWA Publishing.
Stylianou, M., Christou, A., Dalias, P., Polycarpou, P., Michael, C., Agapiou, A., et al. (2020). Physicochemical and structural characterization of biochar derived from the pyrolysis of biosolids, cattle manure and spent coffee grounds. J. Energy Inst. 93 (5), 2063–2073. doi:10.1016/j.joei.2020.05.002
Tait, D. R., Shepherd, B. O., Befus, K. M., and Erler, D. V. (2015). Nutrient and greenhouse gas dynamics through a range of wastewaterloaded carbonate sand treatments. Ecol. Eng. 82, 126–137. doi:10.1016/j.ecoleng.2015.04.082
Tan, X., Liu, Y., Zeng, G., Wang, X., Hu, X., Gu, Y., et al. (2015). Application of biochar for the removal of pollutants from aqueous solutions. Chemosphere 125, 70–85. doi:10.1016/j.chemosphere.2014.12.058
Terzić, S., Senta, I., Ahel, M., Gros, M., Petrović, M., Barcelo, D., et al. (2008). Occurrence and fate of emerging wastewater contaminants in Western Balkan Region. Sci. Total Environ. 399, 66–77. doi:10.1016/j.scitotenv.2008.03.003
Théry-parisot, I., Chabal, L., and Chrzavzez, J. (2010). Anthracology and taphonomy, from wood gathering to charcoal analysis. A review of the taphonomic processes modifying charcoal assemblages, in archaeological contexts. Palaeogeogr. Palaeoclimatol. Palaeoecol. 291 (1–2), 142–153. doi:10.1016/j.palaeo.2009.09.016
Tokwaro, R., Semiyaga, S., Niwagaba, C. B., Nakagiri, A., Sempewo, J. I., Moughalu, C. C., et al. (2023). Application of black soldier fly larvae in decentralized treatment of faecal sludge from pit latrines in informal settlements in Kampala City. Front. Environ. Sci. 11, 138. doi:10.3389/fenvs.2023.1118635
Tomczyk, A., Sokołowska, Z., and Boguta, P. (2020). Biochar physicochemical properties: Pyrolysis temperature and feedstock kind effects. Rev. Environ. Sci. Biotechnol. 19 (1), 191–215. doi:10.1007/s11157-020-09523-3
Tong, X. J., Li, J. Y., Yuan, J. H., and Xu, R. K. (2011). Adsorption of Cu (II) by biochars generated from three crop straws. Chem. Eng. J. 172, 828–834. doi:10.1016/j.cej.2011.06.069
Torrens, A., Molle, P., Boutin, C., and Salgot, M. (2009). Removal of bacterial and viral indicator in vertical flow constructed wetlands and intermittent sand filters. Desalination 246, 169–178. doi:10.1016/j.desal.2008.03.050
Trakal, L., Veselská, V., Šafařík, I., Vítková, M., Číhalová, S., and Komárek, M. (2016). Lead and cadmium sorption mechanisms on magnetically modified biochars. Biores Technol. 203, 318–324. doi:10.1016/j.biortech.2015.12.056
Tsai, W. T., and Chen, H. R. (2013). Adsorption kinetics of herbicide paraquat in aqueous solution onto a low-cost adsorbent, swine-manure derived biochar. Int. J. Environ. Sci. Technol. 10 (6), 1349–1356. doi:10.1007/s13762-012-0174-z
Van Houdt, R., and Michiels, C. W. (2010). Biofilm formation and the food industry, a focus on the bacterial outer surface. J. Appl. Microbiol. 109 (4), 1117–1131. doi:10.1111/j.1365-2672.2010.04756.x
Vithanage, M., Rajapaksha, A. U., Zhang, M., Thiele-Bruhn, S., Lee, S. S., and Ok, Y. S. (2015). Acid-activated biochar increased sulfamethazine retention in soils. Environ. Sci. Pollut. Res. 22, 2175–2186. doi:10.1007/s11356-014-3434-2
Wang, H., Xu, J., and Sheng, L. (2020). Preparation of straw biochar and application of constructed wetland in China: A review. J. Clean. Prod. 273, 123131–131. doi:10.1016/j.jclepro.2020.123131
Wang, H., Gao, B., Wang, S., Fang, J., Xue, Y., and Yang, K. (2015). Removal of Pb (II), Cu (II), and Cd (II) from aqueous solutions by biochar derived from KMnO 4 treated hickory wood. Bioresour. Technol. 197, 356–362. doi:10.1016/j.biortech.2015.08.132
Wang, P., Liu, X., Wu, X., Xu, J., Dong, F., and Zheng, Y. (2018). Evaluation of biochars in reducing the bioavailability of flubendiamide in water/sediment using passive sampling with polyoxymethylene. J. Hazard Mater 344, 1000–1006. doi:10.1016/j.jhazmat.2017.12.003
Wang, Z., Dong, J., Liu, L., Zhu, G., and Liu, C. (2013). Study of oyster shell as a potential substrate for constructed wetlands. Water Sci. Technol. 67 (10), 2265–2272. doi:10.2166/wst.2013.105
Wang, Z., Tian, Q., Guo, J., Wu, R., Zhu, H., and Zhang, H. (2021). Co-pyrolysis of sewage sludge/cotton stalks with K2CO3 for biochar production: Improved biochar porosity and reduced heavy metal leaching. Waste Manag. 135, 199–207. doi:10.1016/j.wasman.2021.08.042
Weber, K., and Quicker, P. (2018). Properties of biochar. Fuel 217, 240–261. doi:10.1016/j.fuel.2017.12.054
Wei, S. U., Ya-ping, Z., Liu-fang, W. E. I., Yan, S. U. N., and Li, Z. (2007). Effect of microstructure and surface modification on the hydrogen adsorption capacity of active carbons. New Carbon Mater. 22 (2), 2323–2325. doi:10.1016/j.carbon.2007.06.038
Werner, S., Katzl, K., Wichern, M., Buerkert, A., Steiner, C., and Marschner, B. (2018). Agronomic benefits of biochar as a soil amendment after its use as wastewater filtration medium. Environ. Pollut. 233, 561–568. doi:10.1016/j.envpol.2017.10.048
WHOUNICEF (2021). Progress on household drinking water, sanitation and hygiene 2000-2020: Five years into the SDGs. Geneva, Switzerland: World Health Organization (WHO) and United Nations Children's Fund (UNICEF).
Williams, M., Martin, S., and Kookana, R. S. (2015). Sorption and plant uptake of pharmaceuticals from an artificially contaminated soil amended with biochars. Plant Soil 395 (1-2), 75–86. doi:10.1007/s11104-015-2421-9
Winward, G. P., Avery, L. M., Frazer-Williams, R., Pidou, M., Jeffrey, P., Stephenson, T., et al. (2008). A study of the microbial quality of grey water and an evaluation of treatment technologies for reuse. Ecol. Eng. 32, 187–197. doi:10.1016/j.ecoleng.2007.11.001
Xiang, W., Zhang, X., Chen, J., Zou, W., He, F., Hu, X., et al. (2020). Biochar technology in wastewater treatment: A critical review. Chemosphere 252, 126539. doi:10.1016/j.chemosphere.2020.126539
Xue, Y., Gao, B., Yao, Y., Inyang, M., Zhang, M., Zimmerman, A. R., et al. (2012). Hydrogen peroxide modification enhances the ability of biochar (hydrochar) produced from hydrothermal carbonization of peanut hull to remove aqueous heavy metals: Batch and column tests. Chem. Eng. J. 200, 673–680. doi:10.1016/j.cej.2012.06.116
Yaman, S. (2004). Pyrolysis of biomass to produce fuels and chemical feedstocks. Energy Convers. Manag. 45, 651–671. doi:10.1016/s0196-8904(03)00177-8
Yang, K., Peng, J., Srinivasakannan, C., Zhang, L., Xia, H., and Duan, X. (2010). Preparation of high surface area activated carbon from coconut shells using microwave heating. Bioresour. Technol. 101 (15), 6163–6169. doi:10.1016/j.biortech.2010.03.001
Yang, X., Flowers, R. C., Weinberg, H. S., and Singer, P. C. (2011). Occurrence and removal of pharmaceuticals and personal care products (PPCPs) in an advanced wastewater reclamation plant. Water Res. 45, 5218–5228. doi:10.1016/j.watres.2011.07.026
Yao, Y., Gao, B., Inyang, M., Zimmerman, A. R., Cao, X., Pullammanappallil, P., et al. (2011). Removal of phosphate from aqueous solution by biochar derived from anaerobically digested sugar beet tailings. J. Hazard. Mater. 190, 501–507. doi:10.1016/j.jhazmat.2011.03.083
Yin, I. X., Zhang, J., Zhao, I. S., Mei, M. L., Li, Q., and Chu, C. H. (2020). <p>The antibacterial mechanism of silver nanoparticles and its application in dentistry</p>. Int. J. Nanomedicine 15, 2555–2562. doi:10.2147/IJN.S246764
Yuan, Y., Yang, B., Wang, H., Lai, X., Li, F., Salam, M. M. A., et al. (2020). The simultaneous antibiotics and nitrogen removal in vertical flow constructed wetlands: Effects of substrates and responses of microbial functions. Bioresour. Technol. 310, 123419. doi:10.1016/j.biortech.2020.123419
Zhang, M., Muhammad, R., Zhang, L., Xia, H., Cong, M., and Jiang, C. (2019). Investigating the effect of biochar and fertilizer on the composition and function of bacteria in red soil. Appl. Soil Ecol. 139, 107–116. doi:10.1016/j.apsoil.2019.03.021
Zhang, Y., Cheng, Y., Yang, C., Luo, W., Zeng, G., and Lu, L. (2015). Performance of system consisting of vertical flow trickling filter and horizontal flow multi-soil-layering reactor for treatment of rural wastewater. Bioresour. Technol. 193, 424–432. doi:10.1016/j.biortech.2015.06.140
Zhang, Z. B., Cao, X. H., Liang, P., and Liu, Y. H. (2013). Adsorption of uranium from aqueous solution using biochar produced by hydrothermal carbonization. J. Radioanalytical Nucl. Chem. 295 (2), 1201–1208. doi:10.1007/s10967-012-2017-2
Zhang, C. M., Xu, L. M., Xu, P. C., and Wang, X. C. (2016). Elimination of viruses from domestic wastewater: Requirements and technologies. World J. Microbiol. Biotechnol. 32, 1–9.
Zhao, M., Dai, Y., Zhang, M., Feng, C., Qin, B., Zhang, W., et al. (2020). Mechanisms of Pb and/or Zn adsorption by different biochars: Biochar characteristics, stability, and binding energies. Sci. Total Environ. 717, 136894. doi:10.1016/j.scitotenv.2020.136894
Zheng, Y., Wang, B., Wester, A. E., Chen, J., He, F., Chen, H., et al. (2019). Reclaiming phosphorus from secondary treated municipal wastewater with engineered biochar. Chem. Eng. J. 362, 460–468. doi:10.1016/j.cej.2019.01.036
Zhou, J., Wang, X. C., Ji, Z., Xu, L., and Yu, Z. (2015). Source identification of bacterial and viral pathogens and their survival/fading in the process of wastewater treatment, reclamation, and environmental reuse. World J. Microbiol. Biotechnol. 31, 109–120. doi:10.1007/s11274-014-1770-5
Zhou, X., Wu, S., Wang, R., and Wu, H. (2019). Nitrogen removal in response to the varying C/N ratios in subsurface flow constructed wetland microcosms with biochar addition. Environ. Sci. Pollut. Res. Int. 26 (4), 3382–3391. doi:10.1007/s11356-018-3871-4
Zhou, Y., Gao, B., Zimmerman, A. R., and Cao, X. (2014). Biochar-supported zerovalent iron reclaims silver from aqueous solution to form antimicrobial nanocomposite. Chemosphere 117 (1), 801–805. doi:10.1016/j.chemosphere.2014.10.057
Keywords: biochar, biomass, greywater, organic waste, wastewater treatment, pyrolysis, biofiltration, wastewater reuse
Citation: Muoghalu CC, Owusu PA, Lebu S, Nakagiri A, Semiyaga S, Iorhemen OT and Manga M (2023) Biochar as a novel technology for treatment of onsite domestic wastewater: A critical review. Front. Environ. Sci. 11:1095920. doi: 10.3389/fenvs.2023.1095920
Received: 11 November 2022; Accepted: 06 February 2023;
Published: 23 February 2023.
Edited by:
Kangning Xu, Beijing Forestry University, ChinaReviewed by:
Santiago Septien Stringel, University of KwaZulu-Natal, South AfricaHonghong Lyu, Hebei University of Technology, China
Copyright © 2023 Muoghalu, Owusu, Lebu, Nakagiri, Semiyaga, Iorhemen and Manga. This is an open-access article distributed under the terms of the Creative Commons Attribution License (CC BY). The use, distribution or reproduction in other forums is permitted, provided the original author(s) and the copyright owner(s) are credited and that the original publication in this journal is cited, in accordance with accepted academic practice. No use, distribution or reproduction is permitted which does not comply with these terms.
*Correspondence: Musa Manga, bW1hbmdhQGVtYWlsLnVuYy5lZHUmI3gwMjAwYTs=, bXVzYS5tYW5nYUBtYWsuYWMudWc=
†These authors have contributed equally to this work and share first authorship