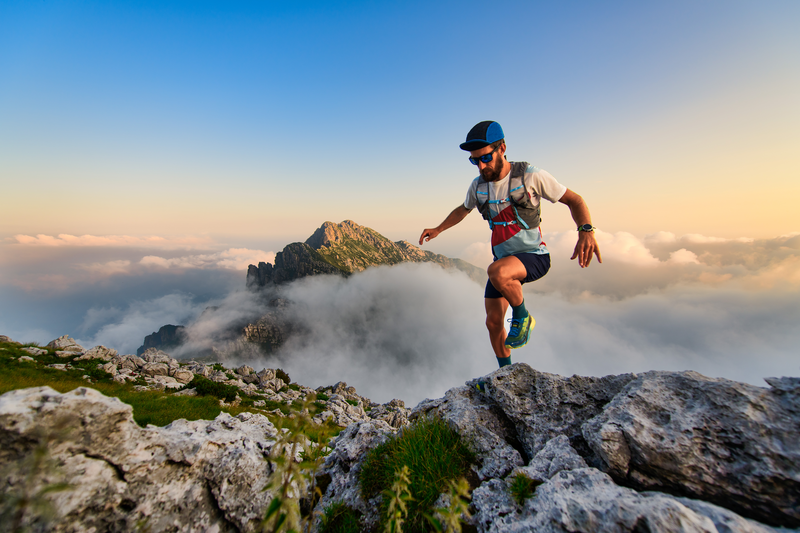
94% of researchers rate our articles as excellent or good
Learn more about the work of our research integrity team to safeguard the quality of each article we publish.
Find out more
REVIEW article
Front. Environ. Sci. , 27 January 2023
Sec. Freshwater Science
Volume 11 - 2023 | https://doi.org/10.3389/fenvs.2023.1084888
This article is part of the Research Topic Hydrological Connectivity and Sustainable Watershed Management in a Changing Environment View all 13 articles
Phytoplankton is an important primary producer of the estuarine ecosystem, which is essential for the biogeochemical cycle of water elements and nutrient transfer. The Pearl River estuary (PRE) is a dynamically complex estuary, and the environment of PRE is significantly impacted by anthropogenic activities, variation of phytoplankton community structure in the PRE are complex. This review aims to compare phytoplankton species, species diversity, and abundance variation characteristics from the 1980s and 2020s, evaluate the overall trend of phytoplankton in the PRE, and discuss the main environmental factors affecting phytoplankton growth in the PRE. The data from the past 40 years in PRE showed that the number of phytoplankton species significantly decreased (p < 0.05). There was no significant difference in the abundance of phytoplankton at the 10-year scale, however, the fluctuation range of the abundance has increased. Under the conditions of a decreasing species number and no significant difference in abundance, the species diversity of phytoplankton showed a downward trend. In addition, the dominant phytoplankton species in the nearshore waters were relatively homogenous, and the abundance of phytoplankton in the nearshore waters was higher than that in the open waters, which suggested that human activities have a great influence. This review can form the basis for facilitating health management in the PRE ecosystem. Further, relevant guidelines can be developed and implemented for promoting the ecological health of the Guangdong-Hong Kong-Macao Greater Bay Area and ensuring its sustainable development.
Figure 1 phytoplankton account for 1% of the photosynthetic biomass on Earth, and it is an important primary producer in aquatic ecosystems. Meanwhile, phytoplankton are the base of aquatic food webs and of global importance for ecosystem functioning and services (Winder and Sommer, 2012). Therefore, it plays a vital role in the biogeochemical processes of water elements and the process of nutrient transfer. Phytoplankton have important applications in the assessment of water quality and ecosystem health (Villegas and Giner, 1973; Guo et al., 2020). For example, the balance between phytoplankton, the environment, and the quality of water health can be evaluated using phytoplankton diversity and biological integrity index (Tan et al., 2017; Liu et al., 2020).
FIGURE 1. Graphical Abstract (Guo and Shen, 2003, Harrison et al., 2008, Huang et al., 2021).
The eutrophic conditions lead to phytoplankton blooms, Phytoplankton proliferate and cover the water surface in eutrophic water, blocking the transmission of light to the bottom, inhibiting the growth and proliferation of aquatic plants at the bottom, and reducing the release of oxygen. In addition, when the nutrients are exhausted, a large area of phytoplankton death will occur, and a large amount of oxygen will be consumed when they are decomposed by bacterial communities. The result of both actions is a decrease in the concentration of dissolved oxygen in the water (Anderson et al., 2002). Reduced concentrations of dissolved oxygen can cause the death of aquatic animals, especially fish. Some algae give off a fishy odor, which makes the water smell bad. The final development of eutrophication will reduce the water storage capacity due to organic residue deposition, destroy the ecological structure of water, break the biological chain, species tend to be single, and water function degradation (Anderson et al., 2002; Qi and Liu, 2004; Salk et al., 2022).
The Pearl River is the third longest river in China, with an annual runoff more than 349.2 billion cubic meters, which size is second only to that of the Yangtze River in China (Qiu et al., 2010). It comprises eight outlets and three main tributaries of the Pearl River—the North River, the East River, and the West River, which empty into the Pearl River Estuary (PRE) (Luo et al., 2007; Zhang, et al., 2009). The PRE in southern China is characterized by a typical subtropical climate, rich in natural resources, and the main water source for Zhuhai and Macao, therefore, it is recognized as the central development hub of the Guangdong-Hong Kong-Macao Greater Bay Area with salient natural geographical advantages. However, the PRE has faced substantial environmental pressure under the influence of frequent human activities and intensified global climate change in recent decades. The water developed from a mesotrophic system to undergoing local eutrophication since the 1900s, and this trend is currently being exacerbated (Jia et al., 2002; Zhang et al., 2009; Niu et al., 2020). Jia et al. (2002) revealed the increasing trend of eutrophication in the PRE over the past century through the sedimentary column. The sedimentary budget of organic carbon, biogenic silica and total nitrogen, which are related to the primary productivity of aquatic ecosystems, basically maintained in a relatively low and stable range before the early 20th century, whereas the sedimentary budget of each component showed an obvious increasing trend after 1920. Furthermore, Guo et al. (2022) showed that the overall water quality of the coastal waters of Zhuhai city was extremely poor in November 2017 and March 2018. The input of nutrients, especially nitrogen, has resulted in aquatic eutrophication in this estuary (Huang et al., 2003; Zhu et al., 2018).
Huge environmental pressure causes frequent occurrences of red tides in the PRE. Such tides severely affect the water supply security and threaten the sustainable utilization of natural resources in the Guangdong-Hong Kong-Macao Greater Bay Area. Thus, it is essential to study the phytoplankton population ecology to understand the health of the PRE ecosystem and its underlying drivers. Estuarine habitats are unique, while phytoplankton composition and distribution patterns are both complex. However, there is no review previously published about phytoplankton in the PRE, the existing ecological surveys and published data in the PRE are not continuous. Therefore, the phytoplankton status and change trend in the PRE are both poorly understood. New techniques for phytoplankton monitoring and evaluation, such as remote sensing inversions, may close this gap; however, they require substantial data support (Peng, 2021).
This review focuses on the species composition, dominant species, spatiotemporal distribution, and species diversity trends of phytoplankton in the PRE and summarizes the main findings on the phytoplankton community in the region. Our summary can facilitate health management in the PRE ecosystem and provide relevant guidelines for promoting the ecological health and sustainable development of the Guangdong-Hong Kong-Macao Greater Bay Area.
This manuscript refers only to published data, the graph and significance difference analysis (Student t test) of phytoplankton species and abundance in different periods were performed by R package ggplot2, RColorBrewer, ggsignif and stats. The graphical abstract was drawn with CorelDraw X8.
Estuaries are transitional zones between rivers and oceans, where brackish and fresh water interact and the evolution mechanism of biogeochemical processes is complex, thus forming a unique complex ecological environment (Lu and Gan, 2015; Niu et al., 2020). Estuarine phytoplankton groups, such as saltwater, freshwater, and semi-saline water species, are also unique. Eight phytoplankton taxa, namely Diatom, Dinoflagellate, Chlorophyta, Cyanobacteria, Chrysophyta, Cryptophyta, and Xanthophyta, have been reported in the PRE since the 1980s (Huang et al., 2004; Li et al., 2014). Similar to the phytoplankton community structure in the 1980s, diatom was determined as the dominant group (especially in the dry season), followed by Chlorophyta (Song, 1991; Xu et al., 2022). During the upstream period of saltwater intrusion, the dominant taxa in the PRE significantly changed, whereas diatoms predominated in the upper estuary, and dinoflagellates predominated in the lower estuary (Li et al., 2010). According to the published data (Figure 2), the data from the past 40 years in PRE showed that the number of phytoplankton species significantly decreased (p < 0.05). Previous studies identified ∼300 species of phytoplankton in the PRE in the early 1980s, mainly represented by the widespread and warm-water species (Liu, 2008). Compared with the 1980s, the overall number of phytoplankton species in the PRE exhibited a downward trend because only 84 phytoplankton species were identified during two voyage surveys in the PRE during 2010–2011 (Song, 1991). Moreover, in 2020, only 50 species of phytoplankton were identified at 14 survey stations in the PRE in the summer and winter (Xu S. et al., 2022). During the saltwater intrusion period, less than 80 species were detected (Xiong and Liu, 2018). The number of species in the PRE varies between seasons; an upward trend exists from spring to summer, where the number of species peaks in the summer and decreases as autumn approaches (Zhou et al., 2016).
FIGURE 2. Variation characteristics of phytoplankton species number in the Pearl River Estuary during the flood seasons from the 1980s–2020s. Asterisks indicate significant differences (*p < 0.05, **p < 0.01) (Song, 1991; Dai et al., 2004; Huang et al., 2004; Liu, 2008; Dong et al., 2009; Li et al., 2010; Feng et al., 2012; Wang et al., 2013; Zhou et al., 2016; Xu et al., 2017; Xiong and Liu, 2018; Xu et al., 2022).
As mentioned, the dominant species in the PRE and adjacent waters are mainly represented by diatoms, which are affected by the interleaving of two heterogeneous water masses, tidal current, and runoff, thereby causing severe water disturbance and distinct seasonal succession of dominant species in the PRE (Li et al., 2007). In the wet season, the dominant species are represented by Aulacoseira granulata and Aulacoseira granulata var. angustissima. Meanwhile, A. granulata is a common dominant species in the upper reaches of the PRE (Wang et al., 2012; 2013). Skeletonema costatum is arguably the dominant species in the PRE during the dry season, especially during the upstream salt-tide period. Skeletonema costatum, characterized by ectothermic and halotolerant organisms, is also the dominant species in the PRE, with a relative abundance of > 80%. Moreover, in some years, Skeletonema costatum was reported as the dominant species during wet seasons (Huang et al., 2004; Feng et al., 2012). In addition, the species of Cerochaete and Rhombic were also reported to be more abundant in the dry season. Similarly, Nitzschia pungens, Nitzschia delicatissima, Thalassiosira sp., Chaetoceros affinis etc., were found to be dominant species in the dry season (Dai et al., 2004; Wang et al., 2013). Compared with that in the wet season, the number of red tide species in estuaries increased in the dry season, and dinoflagellates were the species that increased the most (Dong et al., 2009). The statistics from the 2000–2014 period revealed that the the dominant red tide algae in the PRE were Cochlodinium polykrikoides, Cochlodinium geminatum, Gyrodinium instriatum, and Akashiwo sanguinea (Liu, 2008; Wei et al., 2012; Huan et al., 2016).
The abundance distribution of phytoplankton in the PRE exhibits distinct spatiotemporal variability. More specifically, the interactions between multiple environmental factors complicates the distribution of phytoplankton in the PRE. Consequently, the abundance of phytoplankton is higher in summer than in winter and higher in the wet season than in the dry season (Si, 2018; Xiong and Liu, 2018; Wang et al., 2021). The abundance of phytoplankton in the PRE has been mainly reported downstream in the wet season. Given the high dilution intensity in the upper estuary, a large amount of suspended matter is formed, thereby limiting the access of phytoplankton to nutrients and light. Consequently, phytoplankton growth is also hindered. By contrast, the downstream of the river water is largely devoid of suspended matter, the dilution intensity is small, and phytoplankton bloom, so the abundance is relatively high (Yin et al., 2004; Feng et al., 2012). The high value of phytoplankton abundance in the dry season was clustered in the middle of the estuary; previous studies also showed that the spatial distribution of chlorophyll a in the dry season was characterized by low variability due to the strong mixing of water bodies (Lu and Gan, 2015). Ma and Zhao (2021) determined that the spatial distribution of phytoplankton in the PRE was irregular in the summer. Li et al. (2010) analyzed the phytoplankton community in the PRE during brackish tides and found that phytoplankton exhibited a decreasing trend from the upper to the lower parts of the estuary during both high and low tides. Furthermore, historical data indicated that the abundance of phytoplankton in the PRE increased at small scale. The abundance of phytoplankton in the wet season was significantly higher during 2010–2019 than that in the 1980s, while there was no significant difference in the abundance of phytoplankton at the 10-year scale (Figure 3), however, the fluctuation range of the abundance has increased, and the inter-annual variation range of phytoplankton in the dry season was somewhat narrow (Song, 1991; Liu, 2008; Xu et al., 2017; Niu et al., 2020).
FIGURE 3. Variation characteristics of phytoplankton abundance in the Pearl River Estuary during the flood and dry seasons from the 1980s–2020s (Lin et al., 1994; Huang and Wang, 1997; Huang et al., 2004; Liu, 2008; Li et al., 2010; Feng et al., 2012; Wang et al., 2012; Li et al., 2022; Zhang et al., 2015; Li et al., 2019; Li et al., 2022; Xu et al., 2022).
The diversity of phytoplankton species in the PRE is greatly affected by anthropogenic activities. Indicatively, Wang et al. (2012) examined the phytoplankton in the Pearl River Delta River network and reported that the diversity of dominant species was low in the survey stations near Guangzhou. However, the authors found that the diversity of dominant species that were spatially distant from Guangzhou was high in the survey stations, while the frequent human activities in Guangzhou weakened the diversity of phytoplankton. Generally, the diversity of phytoplankton species in the PRE peaked in summer, was low in the estuary, and gradually increased outside the estuary. Overall, it has been shown that the greater the distance from the shore, the higher the salinity, and the higher the diversity (Dai et al., 2004). The diversity of phytoplankton species was found to be higher offshore than inshore because the abundance of phytoplankton is high near the PRE. However, the species distribution is uneven in the region, and the dominant species is concentrated and homogenous, thereby inducing weak diversity near the PRE (Feng et al., 2012). From the late 1990s to the early 2000s, phytoplankton diversity in the PRE was found to be slightly average (Liu, 2008). Zhang et al. (2015) focused on the PRE and its adjacent waters from 2006 to 2007. They clearly identified the dominant phytoplankton species in the PRE, while also showing that the overall diversity was low; unlike in the previous period, it exhibited a downward trend. Compared with that 20 years ago, the diversity of phytoplankton in the PRE has significantly decreased in recent years (Xu et al., 2022). Because it is associated with the diversity of phytoplankton species in the PRE, the diversity of phytoplankton species in the upper estuary is also highly important. Therefore, Cui (2019) investigated the Pearl River Basin and found that compared with that in the 1980s, the phytoplankton diversity in the Dongjiang and Beijing rivers exhibited downward trends in the 1990, with the exception of Xijiang River. The phytoplankton diversity input from the upper reaches of the Pearl River to the estuary was weak.
Estuaries are affected by the interaction between freshwater discharge and brackish water invasion, while the environmental factors controlling phytoplankton growth are highly complex. The main nutrients are nitrogen, phosphorus, and silicon (Yin et al., 2000; Xu S. et al., 2022). The concentration of phosphorus in the PRE is low, and the contribution of runoff to PO4-P formation is not significant; PO4-P mainly originates from the decomposition of sediment organic matter and the release of sediment, which is exacerbated by the incomplete treatment of domestic sewage discharge and excessive use of nitrogen fertilizers. Therefore, the PRE is characterized by high nitrogen and low phosphorus content. Spatially, nitrogen and phosphorus pollution trends are more prominent in the east but inconspicuous in the west. Dissolved inorganic nitrogen (DIN) in the PRE indicated that upstream of the mouth is dominated by NH4-N, and the concentration of NO3-N was the highest at the mouth, accounting for 51.2%–80.4% (Niu et al., 2020b; Li et al., 2022). Generally, the level of nitrogen and phosphorus nutrients is one of the key drivers of red tides in the PRE. Huang et al. (2016) determined that there was a highly significant positive correlation between NO3-N and the accumulated red tide area in the PRE. In this context, Li et al. (2022) argued that the large increase in PO4-P may be the cause of the large increase in the abundance of Skeletonema costatum, and the transformation of dominant species was mainly associated with the decrease in the concentration of PO4-P. Previously, Yin and Harrison (2008) had suggested that the excessive nitrogen enrichment in the PRE does not induce the high biomass of phytoplankton, and that limited phosphorus is one of the main drivers of this phenomenon. The surface distribution of nutrients in the PRE is mainly affected by terrestrial input and biological consumption. Given the influence of estuarine diluted water in the summer, the dilution of phosphorus in the PRE basin surface waters outside the estuary waters because of the water level change prevents the upper nutrient exchange between water and bottom water, which lead to the surface of phosphorus are exhausted, and phytoplankton growth is limited by phosphorus (Yin et al., 2011; Li et al., 2017). Additionally, phosphorus limitation has predetermined the miniaturization of phytoplankton in the PRE (Dai et al., 2008). Li et al. (2007) examined the characteristics of phytoplankton community structure in the PRE and found that, compared with the surrounding waters, the PRE was dominated by small phytoplankton. Si (2018) investigated the PRE in 2017 and found that the biomass of picophytoplankton was notably dominant, among which Prochlorococcus and Synechococcus contributed the most. A previous study demonstrated that phytoplankton communities with large particle sizes are dominant in nutrient rich environmentsand are more susceptible to phosphorus limitation (Litchman et al., 2007). However, other studies showed that ultra-micro phytoplankton with small particle sizes have a higher specific surface area. Thus, they can adapt to the poor nutrient niche and reproduce rapidly, thereby manifesting advantages in the water (Moutin et al., 2002; Zhou et al., 2016). In the winter, given the strong vertical mixing and low bioavailability, the phosphorus in the bottom sediment is replenished in the surface water, thereby elevating all the forms of phosphorus above the levels recorded in the summer. This increase alleviates the phosphorus restriction in phytoplankton growth, thereby causing more frequent winter blooms (Li et al., 2017; Ye et al., 2020).
Notably, the N/P ratio is crucial for phytoplankton growth, while the Redfield ratio (N: p = 16:1) can be used as a quantitative indicator to evaluate water eutrophication (Sañudo-Wilhelmy et al., 2004). Niu et al. (2020) elucidated the driving factors of the phytoplankton environment in the PRE from 1974 to 2017 and found that DIN and PO4-P both increased. Given the influence of the non-point source inputs from the PRE, the N/P at most survey stations in the PRE was substantially higher than the ratio optimal for phytoplankton growth. The increase in N/P is mainly driven by the enhanced use of nitrogen compared with that of phosphorus, where nitrogen has higher mobility and more dispersed sources than phosphorus (Peñuelas et al., 2012; Yu and Gan, 2022).
The estuarine biogeochemistry process is generally complex, while the main factors limiting phytoplankton growth differ depending on the season. Some studies have shown that Portland considerably limits phytoplankton growth in spring and summer. Phytoplankton quickly multiply in spring and summer as silicates, which are closely associated with the growth in most dominant species and represents one of the important conditions, showing the advantages of diatoms (Yin and Harrison, 2007; Dai et al., 2008). However, when silicate and phosphate contents are both very low, a dinoflagellate red tide can easily occur.
Brackish and freshwater species normally coexist in an estuary; therefore, salinity plays a decisive role in phytoplankton types in the PRE. In recent years, the inter-annual variability of salinity in the PRE has not been significant in the dry season. Despite the insignificance, the frequency, intensity, duration, and range of salt-tide invasion have all exhibited upward trends; salinity in the wet season also showed an upward trend (Zhang et al., 2017). Given the dilution effect of diluting water from the Pearl River in the summer, the salinity of the PRE was found to be higher in dry season than in wet season (Niu et al., 2020). Moreover, the spatial gradient of salinity is negatively correlated with the phytoplankton community in the PRE; as the salinity increases, the number of phytoplankton species decreases (Xiong and Liu, 2018). During the period of upstream saltwater intrusion, the salinity of the upper part of the estuary increases. Some species show weak adaptability to salinity, evident by the decrease in Chlorophyta species, which accounts for a high proportion in the estuary, and the dominant species change from freshwater and brackish water species to marine brackish water species (Wang et al., 2013; Zhang et al., 2017). Spatially, the salinity in the PRE is high in the south and east and low in the north and west. Zhang et al. (2015) showed that the vertical distribution of salinity in the PRE exhibited an increasing trend from the surface layer to the bottom layer and is stratified in the water column. The occurrence of haloclines in the summer hindered the exchange of nutrients between the bottom layer and the surface water, while the phytoplankton had limited access to nutrients, especially phosphate, thereby exacerbating the phosphorus limitations in the PRE.
Hydrodynamic conditions represent another important factor for phytoplankton explosion in estuaries (Zhang et al., 2009). Streamflow and tides both affect phytoplankton growth by altering salinity, salt, and light in the PRE (Lu and Gan, 2015; Niu et al., 2020). Because it is exposed to a monsoon climate, the water dynamics of the PRE exhibit clear seasonality. Ma and Zhao (2021) argued that runoff was the main driver of phytoplankton spatial distribution in the PRE. In particular, during the wet season, the river runoff is large and the water column is stratified, thereby forming the thermohalocline. The vertical exchange of nutrients is hindered, limiting the growth of phytoplankton in the PRE. Niu et al. (2020b) showed that the annual average sediment load in the PRE was mainly carried by runoff during 1974–2016. Moreover, they found that a large amount of suspended matter affected the growth of phytoplankton by influencing their photosynthesis. Furthermore, the runoff decreased during the dry season, while the strong vertical mixing of water created a large amount of suspended matter, thereby potentially inducing limited light conditions for phytoplankton.
Phytoplankton is an important factor to consider in environmental water quality assessment. Specifically, by monitoring the dynamic changes in phytoplankton, the optimal conditions of the PRE water ecological system and how these conditions are related to other estuarine ecosystems can be elucidated. Thus far, this interaction is more complex, fragile, and less understood because anthropogenic activities affecting aquatic systems have caused the eutrophication of the PRE. Furthermore, the lack of continuous phytoplankton monitoring in the PRE heightens both the complexity and poor understanding of these processes. Numerous scholars have previously used chlorophyll-a instead of phytoplankton monitoring, especially to study phytoplankton community composition. However, such an approach is not conducive for in-depth analysis of the water ecological health status of the PRE. Moreover, the sampling time of the phytoplankton community in the PRE was somewhat arbitrary in previous studies, causing a divergence in survey results. Consequently, there is no holistic study which summarizes, elucidates, and discusses the existing research results, while interpreting the ecological characteristics of the PRE.
A remote sensing approach to phytoplankton analysis is more beneficial compared to traditional methods for determining the distribution and dynamics of phytoplankton. Although such studies are becoming increasingly popular, remote sensing of phytoplankton has some limitations. In particular, for efficient inversion using remote sensing observations, the empirical model must be optimized and a large number of monitoring data must be provided. In other words, the monitoring of phytoplankton in the PRE should be considerably strengthened.
Moreover, the ongoing miniaturization of phytoplankton in the PRE should be further analyzed. In practice, the function of phytoplankton is closely related to its morphology. Phytoplankton, a key primary producer of estuarine ecosystems, plays an important role in the estuarine food chain. Overall, the existing literature demonstrated that phytoplankton in the PRE tend to be miniaturized. However, it is poorly understood whether the change in phytoplankton body size yields a change or loss in the functional needs, thereby prompting further studies on this topic. Finally, the environmental implications of phytoplankton miniaturization in the PRE and the physiological and functional changes in phytoplankton should be elucidated in detail in future studies.
In conclusion, the PRE data from the past 40 years showed that the number of phytoplankton species significantly decreased (p < 0.05). The dominant phytoplankton species in the nearshore waters were relatively homogenous, and the abundance of phytoplankton in the nearshore waters was higher than that in the open waters. There was no significant difference in the abundance of phytoplankton at the 10-year scale, however, the fluctuation range of the abundance has increased. Under the conditions of a decreasing species number and no significant difference in abundance, the species diversity of phytoplankton showed a downward trend.
WC was responsible for the structure and writing of the article; FG, WH, and JW, contributed to writing the article; MZ was responsible for drawing figures and proofreading; QW was responsible for proofreading.
This study was supported by the Special Foundation for National Science and Technology Basic Research Program of China (2019FY101900) and Guangdong Natural Science Foundation (2019A1515110131).
We thank all the members of the laboratory for their help in writing this article and colleagues for their active comments on this article.
The authors declare that the research was conducted in the absence of any commercial or financial relationships that could be construed as a potential conflict of interest.
All claims expressed in this article are solely those of the authors and do not necessarily represent those of their affiliated organizations, or those of the publisher, the editors and the reviewers. Any product that may be evaluated in this article, or claim that may be made by its manufacturer, is not guaranteed or endorsed by the publisher.
Anderson, D. M., Glibert, P. M., and Burkholder, J. M. (2002). Harmful algal blooms and eutrophication: Nutrient sources, composition, and consequences. Estuaries 25 (4), 704–726. doi:10.1007/bf02804901
Cui, W. Z. (2019). Temporal and spatial variation of water environment and its impact on the ecosystem of the Pearl River estuary [doctoral dissertation]. Nanjing: Hohai University.
Dai, M., Li, C. H., Jia, X. P., Zhang, H. H., and Chen, R. W. (2004). Ecological characteristics of phytoplankton in coastal area of Pearl River estuary. Chin. J. Appl. Ecol. 15, 1389–1394.
Dai, M., Zhai, W., Cai, W., Callahan, J., Huang, B., Shang, S., et al. (2008). Effects of an estuarine plume-associated bloom on the carbonate system in the lower reaches of the Pearl River Estuary and the coastal zone of the northern South China Sea. Contin. Shelf Res. 28, 1416–1423. doi:10.1016/j.csr.2007.04.018
Dong, Y. H., Cai, J. D., and Qian, H. L. (2009). Nutrient ratios and its relationship with phytoplankton in the Pearl River estuary. Mar. Sci. Bull. doi:10.3969/j.issn.1001-6392.2009.01.001
Feng, J. P., Jiang, S., Qiao, Y. M., Li, W. X., Guan, X., and Feng, J. H. (2012). Study on characteristics of phytoplankton community in Guangzhou segment of the Pearl River estuary. Ecol. Sci. 31, 284–288. doi:10.3969/j.issn.1008-8873.2012.03.010
Guo, F., Gu, J. G., Zhao, J., Yu, H. Z., and Xiao, L. J. (2020). Effects of phytoplankton classifications on the relationship between phytoplankton community and environment in summer subtropical reservoirs, Southern China. Environ. Sci. 41, 5050–5059. doi:10.13227/j.hjkx.202005106
Guo, K., Long, C., Dang, E., and Zhang, L. (2022). Assessment of water quality and eutrophication in the coastal area of Zhuhai city. Mar. Environ. Sci. 02, 222–229. doi:10.13634/j.cnki.mes.2022.02.002
Guo, P. Y., and Shen, H. T. (2003). Research advance in estuarine phytoplankton ecology. Chin. J. Appl. Ecol. 01, 139–142.
Harrison, P. J., Yin, K., Lee, J. H. W., Gan, J., and Liu, H. (2008). Physical–biological coupling in the pearl River Estuary. Cont. Shelf Res. 28 (12), 1405–1415. doi:10.1016/j.csr.2007.02.011
Huang, F., Lin, X., Hu, W., Zeng, F., He, L., and Yin, K. (2021). Nitrogen cycling processes in sediments of the Pearl River Estuary: Spatial variations, controlling factors, and environmental implications. CATENA 206, 105545. doi:10.1016/j.catena.2021.105545
Huang, L., Jian, W., Song, X., Huang, X., Liu, S., Qian, P., et al. (2004). Species diversity and distribution for phytoplankton of the Pearl River Estuary during rainy and dry seasons. Mar. Pollute. Bull. 49, 588–596. doi:10.1016/j.marpolbul.2004.03.015
Huang, L. Q., Pang, R., S., Zhou, Q. L., and Leng, K. M. (2016). Variation trends of nitrogen and phosphorus and the relationship with HABs in Shenzhen coastal waters. Mar. Environ. Sci. 06, 908–914. doi:10.13634/j.cnki.mes.2016.06.017
Huang, L., and Wang, H. (1997). Basic organisms composition and environment status of the Zhujiang estuary and adjacent waters. Mar. Environ. Sci. 16 (3), 1–7. doi:10.1007/BF02951625
Huang, X. P., Huang, L. M., and Yue, W. Z. (2003). The characteristics of nutrients and eutrophication in the Pearl River estuary, South China. Mar. Pollute. Bull. 47 (1-6), 30–36. doi:10.1016/s0025-326x(02)00474-5
Jia, G. D., Peng, P. A., and Fu, J. M. (2002). Sedimentary records of accelerated eutrophication for the last 100 years at the pearl river estuary. Quat. Sci. 22 (2), 158–165. doi:10.3321/j.issn:1001-7410.2002.02.009
Li, G., Lin, Q., Lin, J., Song, X., Tan, Y., and Huang, L. (2014). Environmental gradients regulate the spatial variations of phytoplankton biomass and community structure in surface water of the Pearl River Estuary. Acta Ecol. Sin. 34, 129–133. doi:10.1016/j.chnaes.2014.01.002
Li, J., Chen, Z., Jing, Z., Zhou, L., Li, G., Ke, Z., et al. (2019). Synechococcus bloom in the Pearl River Estuary and adjacent coastal area–With special focus on flooding during wet seasons. Sci. Total Environ. 692, 769–783. doi:10.1016/j.scitotenv.2019.07.088
Li, K. Z., Huang, L. M., Zhang, J. L., Yin, J. Q., and Luo, L. (2010). Characteristics of phytoplankton community in the PREPEARL RIVER ESTUARY during saline water intrusion period. J. Trop. Oceanogr. 29, 62–68. doi:10.3969/j.issn.1009-5470.2010.01.010
Li, L., Ma, F. F., Zhai, X. H., Yang, W. S., Ye, P. H., and Liu, Y. (2022). Analysis on the key factors for the population evolution and early warning of harmful algal blooms based on an algal bloom in Shenzhen Bay, the South China Sea. Ecol. Sci. 41, 82–89.
Li, R., Xu, J., Li, X., Shi, Z., and Harrison, P. J. (2017). Spatiotemporal variability in phosphorus species in the pearl River Estuary: Influence of the river discharge. Sci. Rep. 7, 13649. doi:10.1038/s41598-017-13924-w
Li, T., Liu, S., Huang, L. M., and Zhang, J. M. (2007). Comparative study on the community structure of phytoplankton in autumn in different oceanic function of zones of Guangdong seacoast. Mar. Sci. Bull. 26, 50–59. doi:10.3969/j.issn.1001-6392.2007.02.008
Litchman, E., Klausmeier, C. A., Schofield, O. M., and Falkowski, P. G. (2007). The role of functional traits and trade-offs in structuring phytoplankton communities: Scaling from cellular to ecosystem level. Ecol. Lett. 10, 1170–1181. doi:10.1111/j.1461-0248.2007.01117.x
Liu, K. R. (2008). The tendency of biodiversity variation of phytoplankton in the Pearl River Estuary [masters dissertation]. Dalian: Dalian Maritime University.
Liu, Y. Y., AYI, Q. L., Zhang, S. R., Wu, X. R., Wan, B. N., Zhang, X. P., et al. (2020). Comparative study on the suitability of periphytic algae and phytoplankton in river health assessment. Acta Ecol. Sin. 11, 3833–3843. doi:10.5846/stxb201812062679
Lu, Z. M., and Gan, J. P. (2015). Controls of seasonal variability of phytoplankton blooms in the Pearl River Estuary. Deep Sea Res. II 117, 86–96. doi:10.1016/j.dsr2.2013.12.011
Luo, X. L., Zeng, E. Y., Ji, R. Y., and Wang, C. P. (2007). Effects of in-channel sand excavation on the hydrology of the Pearl River Delta, China. J. Hydrol. 343 (3-4), 230–239. doi:10.1016/j.jhydrol.2007.06.019
Ma, B., and Zhao, H. (2021). Distribution characteristics of chlorophyll a and nutrients in the PREPEARL RIVER ESTUARY in summer and their relationship with environmental factors. Mar. Environ. Sci. 05, 707–716. doi:10.13634/j.cnki.mes.2021.05.008
Moutin, T., Thingstad, T. F., Van Wambeke, F., Marie, D., Slawyk, G., Raimbault, P., et al. (2002). Does competition for nanomolar phosphate supply explain the prePearl River Estuarydominance of the cyanobacterium Synechococcus? Limnol. Oceanogr. 47, 1562–1567. doi:10.4319/lo.2002.47.5.1562
Niu, L., Luo, X., Hu, S., Liu, F., Cai, H., Ren, L., et al. (2020). Impact of anthropogenic forcing on the environmental controls of phytoplankton dynamics between 1974 and 2017 in the Pearl River Estuary, China. Ecol. Indic. 116, 106484. doi:10.1016/j.ecolind.2020.106484
Niu, L., van Gelder, P., Luo, X., Cai, H., Zhang, T., and Yang, Q. (2020b). Implications of nutrient enrichment and related environmental impacts in the pearl River Estuary, China: Characterizing the seasonal influence of riverine input. Water 12, 3245. doi:10.3390/w12113245
Peng, Y. S. (2021). Remote sensing inversion of phytoplankton particle size classes and its spatial-temporal characteristics in the Pearl River Estuary [master’s dissertation]. Nanchang: East China University.
Peñuelas, J., Sardans, J., Rivas-Ubach, A., and Janssens, I. A. (2012). The human-induced imbalance between C, N and P in Earth’s life system. Glob. Change Biol. 18, 3–6. doi:10.1111/j.1365-2486.2011.02568.x
Qi, M., and Liu, F. (2004). Ecological hazards of urban water eutrophication and its prevention measures. Environ. Sustain. Dev. 01, 44–46. doi:10.19758/j.cnki.issn1673-288x.2004.01.022
Qiu, D., Huang, L., Zhang, J., and Lin, S. (2010). Phytoplankton dynamics in and near the highly eutrophic pearl River Estuary, south China sea. Cont. Shelf Res. 30, 177–186. doi:10.1016/j.csr.2009.10.015
Salk, K. R., Venkiteswaran, J. J., Couture, R. M., Higgins, S. N., Paterson, M. J., and Schiff, S. L. (2022). Warming combined with experimental eutrophication intensifies lake phytoplankton blooms. Limnol. Oceanogr. 67 (1), 147–158. doi:10.1002/lno.11982
Sañudo-Wilhelmy, S. A., Tovar-Sanchez, A., Fu, F. X., Capone, D. G., Carpenter, E. J., and Hutchins, D. A. (2004). The impact of surface-adsorbed phosphorus on phytoplankton Redfield stoichiometry. Nature 432, 897–901. doi:10.1038/nature03125
Si, Y. Y. (2018). Study on Mesozooplankton grazing and reproduction in Pearl River Estuary and north-central south China Sea [master’s dissertation]. Xiamen: Xiamen University.
Tan, Q., Ma, Q., Li, B. B., Lu, H. J., Fu, M., and Yao, W. (2017). Ecological health assessment of the upper reaches of the Yangtze River, based n biotic integrity index of phytoplankton. Freshw. Fish. 03, 97–104. doi:10.13721/j.cnki.dsyy.2017.03.015
Villegas, I., and de Giner, G. (1973). Phytoplankton as a biological indicator of water quality. Water Res. 7 (3), 479–487. doi:10.1016/0043-1354(73)90028-6
Wang, C., Jia, H., Wei, J., Yang, W., Gao, Y., Liu, Q., et al. (2021). Phytoplankton functional groups as ecological indicators in a subtropical estuarine river delta system. Ecol. Indic. 126, 107651. doi:10.1016/j.ecolind.2021.107651
Wang, C., Lai, Z. N., Li, Y. F., Li, X. H., Sovan, L., Hong, Y., et al. (2012). Population ecology of Aulacoseira granulata in Xijiang river. Acta Ecol. Sin. 32, 4793–4802. doi:10.5846/stxb201111031657
Wang, C., Li, X., Lai, Z. N., Zeng, Y. Y., Gao, Y., Liu, Q. F., et al. (2013). Temporal and spatial pattern of the phytoplankton biomass in the Pearl River Delta. Acta Ecol. Sin. 33, 5835–5847. doi:10.5846/stxb201305191112
Winder, M., and Sommer, U. (2012). Phytoplankton response to a changing climate. Hydrobiol. 698 (1), 5–16. doi:10.1007/s10750-012-1149-2
Wei, G., Q., Wang, H., Cai, W. X., and Yi, B. (2012). 10-year retrospective analysis on the harmful algal blooms in the Pearl River Estuary. Mar. Sci. Bull., 1001–6392. 2012.4.017. doi:10.11840/j.issn
Xiong, Y. J., and Liu, J. F. (2018). Can saltwater intrusion affect a phytoplankton community and its net primary production? A study based on satellite and field observations. Estuaries Coasts 41, 2317–2330. doi:10.1007/s12237-018-0427-2
Xu, M. N., Wu, Y., Zhang, X., Tang, J. M., Tan, E., Zheng, Z. Z., et al. (2022). Diel change in inorganic nitrogenous nutrient dynamics and associated oxygen stoichiometry along the Pearl River Estuary. Water Res. 222, 118954. doi:10.1016/j.watres.2022.118954
Xu, S., Liu, Y., Fan, J., Xiao, Y., Qi, Z., and Lakshmikandan, M. (2022). Impact of salinity variation and silicate distribution on phytoplankton community composition in Pearl River Estuary, China. Ecohydrol. Hydrobiol. 22, 466–475. doi:10.1016/j.ecohyd.2022.01.004
Xu, S., Yang, Y., Su, L., Gong, Y., and Chen, Z. (2017). Community structure of phytoplankton in the Nansha sea area of pearl river estuary. South China Fisher. Sci 13 (4), 26–33. doi:10.3969/j.issn.2095-0780.2017.04.004
Ye, H., Yang, C., Tang, S., and Chen, C. (2020). The phytoplankton variability in the Pearl River Estuary based on VIIRS imagery. Contin. Shelf Res. 207, 104228. doi:10.1016/j.csr.2020.104228
Yin, K. D., Harrison, P. J., Broom, M., and Chung, C. H. (2011). Ratio of nitrogen to phosphorus in the Pearl River and effects on the estuarine coastal waters: Nutrient management strategy in Hong Kong. Phys. Chem. Earth Parts A B C. 36, 411–419. doi:10.1016/j.pce.2010.04.014
Yin, K. D., and Harrison, P. J. (2007). Influence of the Pearl River Estuary and vertical mixing in Victoria Harbor on water quality in relation to eutrophication impacts in Hong Kong waters. Mar. Pollut. Bull. 54, 646–656. doi:10.1016/j.marpolbul.2007.03.001
Yin, K. D., and Harrison, P. J. (2008). Nitrogen over enrichment in subtropical Pearl River estuarine coastal waters: Possible causes and consequences. Contin. Shelf Res. 28, 1435–1442. doi:10.1016/j.csr.2007.07.010
Yin, K. D., Qian, P. Y., Chen, J. C., Hsieh, D. P., and Harrison, P. J. (2000). Dynamics of nutrients and phytoplankton biomass in the pearl River estuary and adjacent waters of Hong Kong during summer: Preliminary evidence for phosphorus and silicon limitation. Mar. Ecol. Prog. Ser. 194, 295–305. doi:10.3354/meps194295
Yin, K. D., Zhang, J., Qian, P., Jian, W., Huang, L., Chen, J., et al. (2004). Effect of wind events on phytoplankton blooms in the Pearl River Estuary during summer. Contin. Shelf Res. 24, 1909–1923. doi:10.1016/j.csr.2004.06.015
Yu, L., and Gan, J. (2022). Reversing impact of phytoplankton phosphorus limitation on coastal hypoxia due to interacting changes in surface production and shoreward bottom oxygen influx. Water Res. 212, 118094. doi:10.1016/j.watres.2022.118094
Zhang, J. P., Huang, X. P., Jiang, Z. J., and Huang, D. J. (2009). Seasonal variations of eutrophication and the relationship with environmental factors in the Zhujiang Estuary in 2006–2007. Acta Oceanol. Sin. 31, 113–120. doi:10.3321/j.issn:0253-4193.2009.03.013
Zhang, M., Cui, B., Zhang, Z., and Jiang, X. (2017). Salinity-oriented environmental flows for keystone species in the Modaomen Estuary, China. Front. Earth Sci. 11, 670–681. doi:10.1007/s11707-016-0609-9
Zhang, W., Sun, J., Lie, H. T., Jiang, G., Q., and Tao, J. H. (2015). Seasonal and spatial variations of nutrient and the response of phytoplankton in PREPEARL RIVER ESTUARY and adjacent sea areas. Acta Ecol. Sin. 12, 4034–4044. doi:10.5846/stxb201308262158
Zhang, W., Yan, Y., Zheng, J., Li, L., Dong, X., and Cai, H. (2009). Temporal and spatial variability of annual extreme water level in the Pearl River Delta region, China. Glob. Planet. Change 69 (1-2), 35–47. doi:10.1016/j.gloplacha.2009.07.003
Zhou, W., Jie, G., Liao, J., Shi, R., and Long, A. (2016). Characteristics of phytoplankton biomass, primary production and community structure in the modaomen channel, PRE, with special reference to the influence of saltwater intrusion during neap and spring tides. PLoS ONE 11 (12), e167630. doi:10.1371/journal.pone.0167630
Keywords: phytoplankton, the Pearl river estuary, estuarine ecosystem, species characteristics, spatio-temporal variation
Citation: Chen W, Guo F, Huang W, Wang J, Zhang M and Wu Q (2023) Advances in phytoplankton population ecology in the Pearl river estuary. Front. Environ. Sci. 11:1084888. doi: 10.3389/fenvs.2023.1084888
Received: 31 October 2022; Accepted: 12 January 2023;
Published: 27 January 2023.
Edited by:
Hu Liu, Northwest Institute of Eco-Environment and Resources (CAS), ChinaReviewed by:
Peng Zhang, Guangdong Ocean University, ChinaCopyright © 2023 Chen, Guo, Huang, Wang, Zhang and Wu. This is an open-access article distributed under the terms of the Creative Commons Attribution License (CC BY). The use, distribution or reproduction in other forums is permitted, provided the original author(s) and the copyright owner(s) are credited and that the original publication in this journal is cited, in accordance with accepted academic practice. No use, distribution or reproduction is permitted which does not comply with these terms.
*Correspondence: Wenlong Chen, NDg3MDgyMDlAcXEuY29t
Disclaimer: All claims expressed in this article are solely those of the authors and do not necessarily represent those of their affiliated organizations, or those of the publisher, the editors and the reviewers. Any product that may be evaluated in this article or claim that may be made by its manufacturer is not guaranteed or endorsed by the publisher.
Research integrity at Frontiers
Learn more about the work of our research integrity team to safeguard the quality of each article we publish.