- 1Aquatic Ecology and Fisheries Laboratory, Department of Natural and Applied Sciences, University of Wisconsin-Green Bay, Green Bay, WI, United States
- 2Department of Research, Education and Development, RED YAKU, Lima, Peru
- 3Programa de Pós-Graduação em Ciências Ambientais—CIAMB, Universidade Federal de Goiás, Goiás, Brazil
- 4School of Geosciences, Yangtze University, Wuhan, China
The Peruvian Amazon is known for harboring the greatest biodiversity on the planet, with a world record for biodiversity per unit area. Previous studies suggested that the high ecological value depends on correlations between ecosystem functionality and seasonal inundation control vegetation patches. However, the knowledge on how river morphodynamics and its complex erosion-depositional processes influence the aquatic mosaic and fishing activity in the region is still incipient. This study examines the hydrogeomorphology of the Peruvian tropical wetland of Pacaya Samiria, located in Western Amazonia, and its role in the distribution of aquatic habitats. By using remote sensing techniques, the hydrogeomorphological connectivity that bounds the Pacaya Samiria National Reserve is characterized by ancient to modern river processes. Additionally, river signatures developed by the Ucayali, Marañon, Huallaga, Pacaya, and Samiria Rivers overlap with fish extraction and dominant vegetation to describe how geomorphology is associated with the spatial distribution of fishing zones. Results indicated that paleochannels regulate wetland drainage within the Ucamara Depression, supporting stational water stagnation, vegetation cover, and formation of carbon rich detritus, relevant aspects to understand fish traits. Moreover, the Ucayali River dominates river dynamics in the Pacaya Samiria wetland, thus playing a pivotal role in shaping the complexity of streams and lakes. Furthermore, underfit-scavenger meandering rivers are observed in areas where paleochannels from large rivers are found. A geomorphological characterization of drainage patterns in freshwater environments, such as Amazonian wetlands, is crucial to develop sound management strategies. This methodological approach is expected to support decision-making in conservation actions in Amazonian environments based on understanding wetland connectivity and hydrogeomorphological behavior and their influence on commercial fisheries.
1 Introduction
The Amazon Basin is composed of a mosaic of interconnected freshwater and terrestrial ecosystems. The complexity of these connections depends not only on the hydrological patterns but also on the geomorphic features in the floodplain, creating a heterogeneous network of wetlands, lakes, channels, and alluvial deposits that varies in space and time (Alsdorf, 2003; Park and Latrubesse, 2017). Seasonally based flooding patterns of both hydrological and hydraulic regimes also create a diverse mosaic of vegetation and habitats, supporting the functioning of ecosystems. As a result of the constant development of the floodplains caused by seasonal alluvial flows, previous studies suggest that the diversity of the vegetation from different ages generated the high biological diversity in the Amazon rainforest (Salo et al., 1986). Therefore, dynamic flow regimes establish critical interactions between the main channel and adjacent floodplain habitats, such as “tipishcas” or oxbow lakes, thereby providing fish and other aquatic species access to shelter, food, and spawning grounds (Junk et al., 1989; Couto et al., 2017; Virgilio et al., 2020). According to Castello et al. (2013), this dynamic interaction explains the high ecological value of the Amazonian ecosystems but also makes them more sensitive to anthropogenic pressures. Flood frequency and duration of inundation are relevant to characterize the hydrological connectivity and the ecological features of Amazonian ecosystems (Wilson et al., 2007; Castello et al., 2013; Reis et al., 2019). Reis et al. (2019) also indicate that the predominance of local rainfall in flooding patterns of complex wetlands makes them more sensitive to climate change and external pressures at a regional scale but less vulnerable to alterations in the local hydrology of the river. However, this is the opposite for systems dominated by floodplain inundation that depend on the overflow from large rivers and lateral connectivity. Moreover, Arantes et al. (2019) demonstrated that the biomass distribution of fish functional diversity in floodplains is associated with the vegetation cover, resulting that biomass being most significant in areas of the flooded forest when compared to other habitats.
The Peruvian Amazon is one of the regions that harbor the largest biodiversity on the planet, with a world record for biodiversity per unit area (OTCA, 2009). Some theories explain that this biological value depends on the correlations of ecosystems with the dynamics of rivers because it is related to flooding patterns (Ferreira and Stohlgren, 1999), the geomorphology of the riverbed, the altitudinal climatic changes, and the influence of the upper part on the river channel (Naiman et al., 1993). A dynamic fluvial system helps to modify the flora, unlike a more stable environment where the time scale of these processes is much longer. Kalliola et al. (1992); Kalliola et al. (1993a); Kalliola et al. (1993b) and Puhakka et al. (1992) explained that the vegetation patterns and the diversity of the species are usually controlled by other environmental factors, such as 1) the dynamics of the river that defines the type of vegetation and habitats; 2) the influence of the change of season in the plain alluvial that generates regions of different elevations, age, and soil properties (deposition processes along the point bars, ridges, depressions, flooded basins, and abandoned channels); and 3) the alluvial processes that create new habitats, modify existing ones and produce a diversity of vegetation. Additionally, the seasonal intermittency of discharge into rivers produces variations in water cycles, which are associated with the frequency of floods, erosion of riverbanks, changes in fishing activity, and changes in the planimetric and altimetric patterns of rivers (Salonen et al., 2012; Abad et al., 2013).
The primary objective of this study was to explore the ancient and modern river geomorphology across the Pacaya-Samiria wetland system (the second largest protected area in Peru), considering the high geomorphological complexity observed in this region (Dumont, 1991; Dumont, 1992). Based on it, the second objective was to relate the landscape dynamism to water fluxes, peat vegetation, and fish extraction. This study hypothesizes that the characterization of wetland landscapes will help understand the gradients in habitats and support decision-making in conservation and planning strategies for Amazonian wetlands.
2 Study area
The Pacaya Samiria National Reserve (PSNR) is in the west portion of the Amazon basin (Figure 1A), a region where the Amazon River is born due to the confluence of the Ucayali (meandering) and Marañon (anabranching) rivers (Garcia et al., 2022; Guerrero et al., 2022), as illustrated in Figure 1A. The PSNR is a protected Ramsar Site of 20,800 km2 (one of the largest flooded forests within the Amazon basin) composed of a tropical seasonally flooded forest dominated by rainfall and upland run-off (Castello et al., 2013), where biodiversity indicators (e.g., terrestrial mammal richness, tree a-diversity) are higher compared to other regions in the Amazon basin (Hoorn et al., 2010).
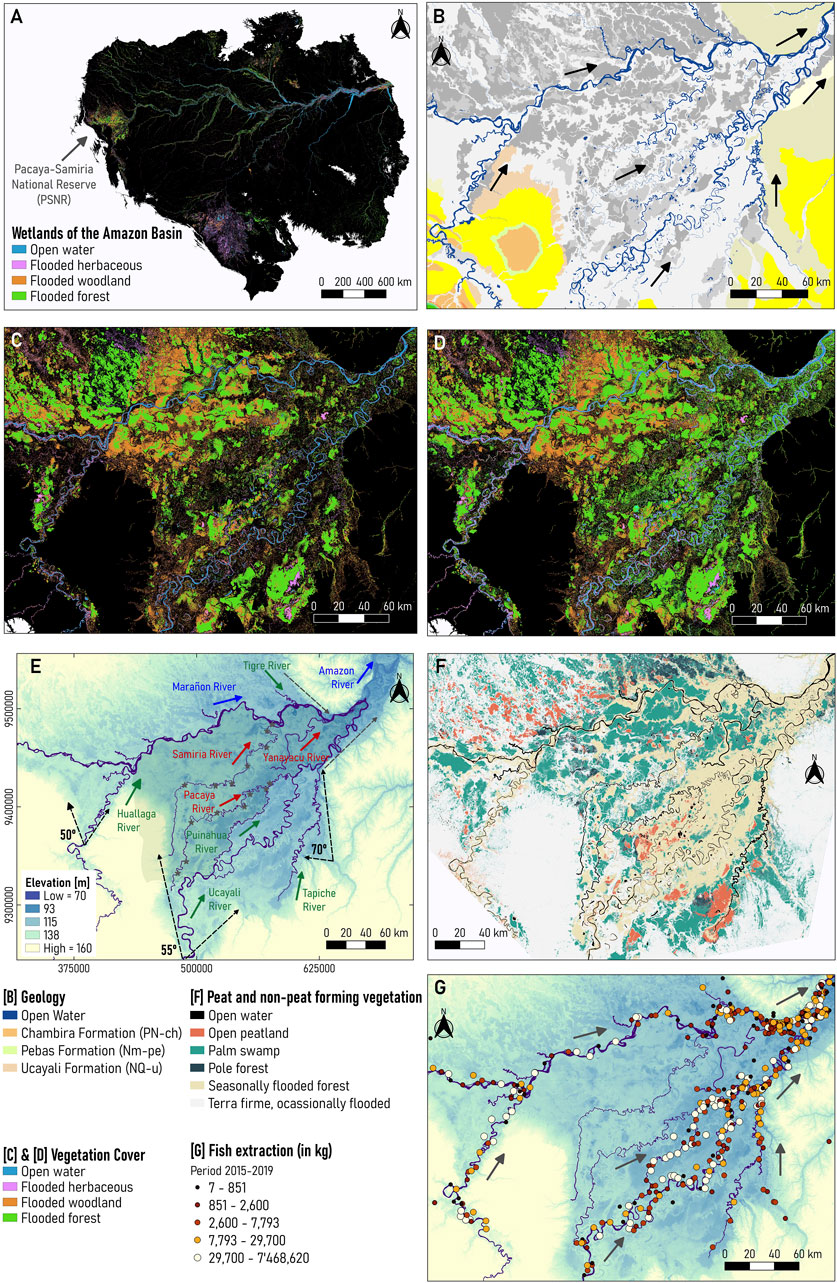
FIGURE 1. (A) Location of the Pacaya-Samiria (P–S) wetland in the Amazon Basin, (B) Geology (dataset obtained from the Geological Mining and Metallurgical Institute (INGEMMET) of Peru, (C) Low-water stage in 1995, (D) high-water stage 1996 (datasets obtained from Hess et al., 2015), (E) Main rivers and tributaries with DEM, (F) Peat and non-peat forming vegetation (dataset obtained from Draper et al., 2014), (G) Fish extraction from 2015–2017 (dataset provided by Wildlife Conservation Society, 2022).
From the geological point of view, the Pacaya-Samiria (P-S) wetland develops in the subsiding Ucamara depression of the Marañon Basin in the Peruvian Andean forelands. It is a very flat subsiding area of 330,000 km2, consisting of a complex network of rivers and permanent and semi-permanent swamps and lakes, and paleochannels. The depression is approximately delimited on the north by the Marañon River and to the southern and eastern borders by morphostructural boundaries and the Ucayali River. The Ucamara depression was an active sediment sink that was filled up by with sediment sourced from the Andes by avulsed alluvial belts of the Ucayali and Marañon (Dumont, 1992; Latrubesse, 2015). The thickness of the Quaternary deposits is unknown, but more than 15 m are exposed in bank outcrops of Quaternary terraces in the Ucayali River. Figure 1B shows that the P-S wetland overlies with the Chambira (continental) formation in the Ucayali Basin of Peru, to later overlap with the Solimões (previously known as Pebas) formation in the confluence between the Ucayali and Marañon Rivers (da Silva-Caminha et al., 2020; Guerrero et al., 2022).
Figure 1C shows that the P-S wetland has a low-water stage from October to November, whereas flooding (high-water stage) occurs between May and July (Hess et al., 2015; Reis et al., 2019). In this ecosystem, bordering rivers are narrow (Reis et al., 2019) and there is a direct correlation between flooded area percentage (open water, inundated non-forest, and inundated forest) (Jensen et al., 2018) and river level measurements. As illustrated in Figures 1C, D, the P-S wetland cover is composed of late-successional vegetation, such as forests and woodlands, that are inundated most of the year. During the high-water stage, areas along the streams (bare soil) inundate, allowing early successional vegetation, such as aquatic macrophytes, to grow.
Figure 1E shows that the P-S wetland includes different river systems: self-formed meandering (Ucayali, Huallaga, Tigre, Tapiche, Puinahua), underfit-scavenger-formed meandering (Yanayacu, Pacaya, Samiria), and anabranching (Marañon, Amazonas). The Huallaga, Ucayali, and Tapiche Rivers enter the P-S wetland with an increase in the valley angle―50, 55, and 70°, respectively, thus avulsion processes and meandering migration rates are enhanced (Abad et al., 2022a; Abad et al., 2022b). As observed in Figure 1E, there is a reduction in valley angle, promoting the birthplace of the Amazon River as the confluence of the Marañon and Ucayali Rivers (Garcia et al., 2022; Guerrero et al., 2022).
Previous studies have described the complex geomorphology within the P-S wetland, composed of interconnected rivers and swamps (Dumont and Garcia, 1991; Kalliola et al., 1992). The Marañon, the Ucayali, and the Tapiche are white-water rivers (high concentrations of solutes and sediment) that flow from west to east and south to north, respectively, while the Pacaya, Yanayacu and the Samiria are black-water rivers (high concentration of dissolved organic carbon) with similar geomorphic patterns that resulted from the accumulation of rainfall and flooding on fossil fluvial landforms (Dumont 1991; Duncan and Fernandes, 2010). Dumont. (1991) described that the composition of fluvial landforms along the Pacaya River is similar to those Ucayali River present, thus suggesting that the Ucayali River has flowed northward forming the Pacaya River valley to shift southeastward later. Likewise, the Samiria River presents similar fluvial landforms to the Ucayali River, but that are more preserved than Pacaya’s. These landforms are also surrounded by the Marañon River, indicating that Samiria was formerly connected to the Marañon River. Dumont. (1991) and Räsänen et al. (1992) indicated that the Samiria, Pacaya, and Tapiche are then underfit-scavenger rivers that rely on relic belts left by the Ucayali River. Additionally, Dumont. (1991) suggested that the Ucayali and Marañon Rivers have been joined on the western side of the P-S wetland and later the junction migrated eastward due to avulsion shifting dynamics.
Puhakka et al. (1992) described that sediment deposited in adjacent bars in meandering rivers tends to inundate during the high-flow season then allowing plant colonization in the following months. Herein, long-term aggradation and deposition of meandering river sediments result in alternated vegetation mosaics (Kalliola et al., 1992). Likewise, other colonized areas include poorly drained swales between the ridges. In meandering systems, such as the Ucayali and Huallaga Rivers, banks in chute islands (result from chute cutoff) are prone to erosion thus limiting vegetation succession, whereas river margin areas are more stable and can extend many kilometers downstream allowing vegetation grows in aged zones. Studies of vegetational successions in tropical and subtropical anabranching rivers are less known (Wittman et al., 2006; Peixoto et al., 2009; Montero and Latrubesse, 2013; Stevaux et al., 2013; Marchetti et al., 2013; Marchetti et al., 2020; among others). In anabranching systems, such as the Marañon River, braided channels are constantly dividing and rejoining; thereby, developing an unstable system composed of young successional vegetation. Additionally, Draper et al. (2014), Lähteenoja et al. (2012), and Lähteenoja and Page. (2011) suggested linkages between vegetation history with carbon stocks in peatland environments across the P-S wetland in the Pastaza-Marañon foreland. Figure 1F shows larger open peatland areas across the Ucayali River, and pole forests and palm swamps along the Marañon River. High carbon density has been found in below-ground pole forests, followed by palm swamps and pole forests (Draper et al., 2014), which suggests that the Marañon valley has greater carbon stocks than the Ucayali.
Gradients in land cover, because of inundation timing and consequent vegetation succession, are often associated with animal functional diversity and biomass in Amazonian floodplains (Arantes et al., 2019). The interconnected system between the Marañon and Ucayali Rivers combined with the exchange of water and sediment (Anderson et al., 2018) supports a high alpha and beta diversity of a myriad of fish species due to the habitat specialization and geographic isolation conditions (Albert et al., 2011; Aldea-Guevara et al., 2013). In the Marañon and Ucayali basins, more than 1,300 fish species have been estimated (Anderson et al., 2018; Latrubesse et al., 2021). The Ucayali basin encompasses more than 650 species of fish, 90 to 110 species of floodplain trees and more than 80 species of floodplain birds, whilst the Marañon basin sustains around 700 species of fish, the highest tree diversity among Amazon sub-basins, and more than 50% of flooded-habitat birds identified in the Amazon (Latrubesse et al., 2021). In addition, the Amazon basin reaches the highest diversity in the Marañon basin and Andean-foreland rivers have the highest tree diversity compared to any floodplain forests on Earth, including up to 30% endemic tree species (Wittmann et al., 2013).
Figure 1G shows about 1,112 fish extraction zones georeferenced across the Loreto region, of which 28% are in the PSNR along the Marañon, Huallaga, and Ucayali rivers (Wildlife Conservation Society, 2022). More than 60 billion kilograms were extracted in a 5-year period (from 2015 to 2017) in Loreto, and only 40% of the total fish extraction was extracted from the Ucayali River. Moreover, Figure 1F indicates a higher concentration of fishing areas near the San Lorenzo community (Marañon) but a lower fish extraction capacity. In contrast, locations near the Requena community (Ucayali) show a higher fish extraction capacity but a lower concentration of fishing zones. Note that this information was derived from the landings of commercial fishing registered in the main ports. Although the Regional Directorate of Production of Loreto (Dirección Regional de la Producción de Loreto, in Spanish) collected all data, there may be underestimates of the total fish volume of landings because not all ports have enough personnel to collect that information (Wildlife Conservation Society, 2022).
3 Materials and methods
3.1 Modern river dynamics
Modern meandering and anabranching river dynamics of the Ucayali and Marañon rivers were previously characterized by Gutierrez and Abad. (2014), Gutierrez et al. (2014), Frias et al. (2015), Mendoza et al. (2016) and Abad et al. (2022a). Figure 2 shows the planform configuration for upstream- (A1) and downstream-skewed (A2) meandering channels (Abad and Garcia, 2009a; Abad and Garcia, 2009b). The main metrics for rivers include W (channel width), A (amplitude), R (radius of curvature), L (wavelength, straight line between inflection points), λ (arc-wavelength), and sinuosity S (S = λ/L). Figure 2B shows the planform configuration of anabranching rivers (based on Garcia et al., 2022) which is composed of the main channel and several secondary channels, as well as islands and distinct size angles of bifurcation and confluence of channels.
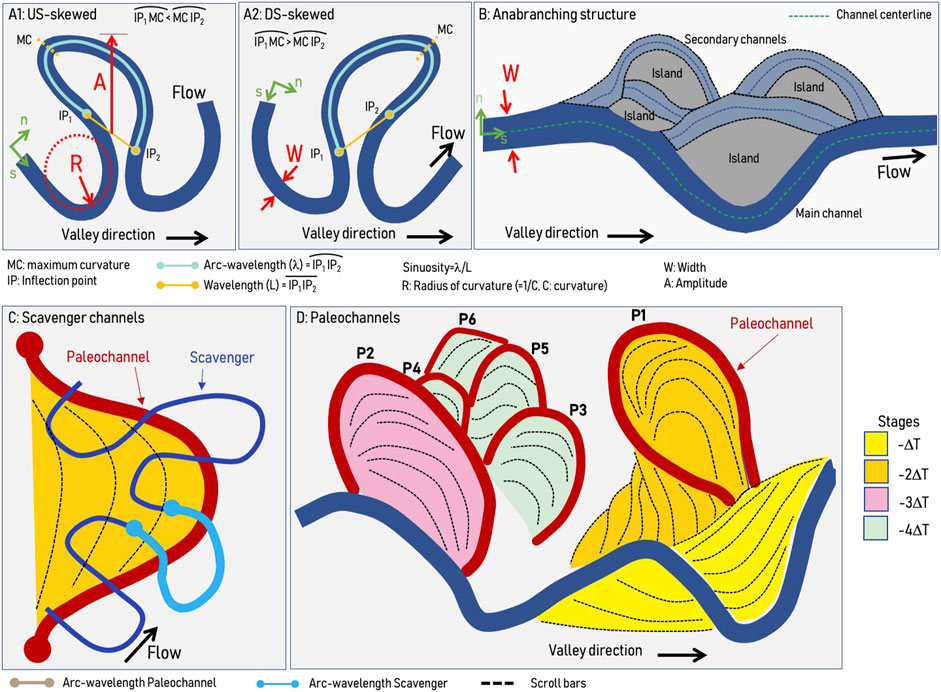
FIGURE 2. (A) meandering river (A1: US-skewed, A2: DS-skewed), (B) Anabranching structure, (C) Underfit-scavenger channels, (D) Paleochannels in different stages (ages).
This study covered satellite imagery processing every 5 years between 1985 and 2021 (the last time interval was 6 years from 2015 to 2021) due to the dynamism of meandering (Ucayali and Huallaga) and anabranching (Marañon) rivers (Salo et al., 1986; Frias et al., 2015; Abad et al., 2022a), reporting a total of 36 years of analysis. Landsat imagery was downloaded from the USGS Earth Explorer data portal (https://earthexplorer.usgs.gov/) considering a cloud percentage of less than 10% during the dry season in the area—from July to December. Landsat bands were then combined to determine the NDVI (Normalized Difference Vegetation Index) and later filtered to discretize “water” and “not water” areas (Frias et al., 2015; Marchetti et al., 2020). Using the polygonization tool, raster data were converted into vectors and removed clouds, disconnected streams, and ponds were to only determine the main river channels. For more details on imagery analysis, see the supplemental material.
After obtaining the channel GIS features (especially the channel centerline and curvature), planform metrics can be obtained. Catano et al. (2009), Gutierrez and Abad. (2014), and Gutierrez et al. (2014) developed the first studies to characterize river meandering dynamics using continuous wavelet transform (CWT). Later on, Ruben-Dominguez et al. (2021) developed the MStaT toolbox to extract river planform metrics (using CWT) and migration rates (from two centerlines at different time intervals). Rivers are composed of a wide spectrum of bends, thus, Vermeulen et al. (2016) also has used the CWT and described that river meandering planforms are multiscale, non-stationary features. By using the continuous wavelet transform and the curvature signal of the river centerline, Vermeulen et al. (2016) analyzed the wavelet spectrum in a hierarchically ordered tree. This approach aimed to describe how small-scale meanders are connected to large-scale meanders as in a train of meanders. As suggested by Vermeulen et al. (2016), in this study, the Gaussian wavelet of order 2 (Mexican-hat) was used.
3.2 Ancient river dynamics
River migration patterns can be identified by mapping the fluvial landforms on the terrain. Geomorphology is a key tool to interpret the temporal channel-floodplain changes and migrations, the soils mosaic and the morpho-hydrological patterns that control vegetational units in fluvial wetlands (Abad et al., 2013; Abad and Garcia, 2009b; Motta et al., 2012a; Motta et al., 2012b; Latrubesse and Suizu, 2022). These characteristics can be mapped from high-resolution Google Earth imagery and digital elevation models (DEM) (Ghinassi et al., 2014; Rojas et al., 2021). In this study, ancient planform architecture was determined using the methodology described by Lin et al. (2017) and Shan et al. (2018) to characterize past river dynamics and geomorphic features in meandering (Ucayali, Huallaga, Tapiche, Pacaya, Samiria, Yanayacu) and anabranching (Marañon) rivers.
River signatures were traced using both satellite and radar imagery. Satellite imagery consisted of a 30-m resolution DEM derived from the Aster Global Digital Elevation Model (ASTER GDEM) globe map data sets from the Peruvian Ministry of Environment and 90-m resolution data from the Shuttle Radar Topography Mission (SRTM) (Jarvis et al., 2008). In addition, radar imagery combined SAR mosaics from the Advanced Land Observing Satellite 2 (ALOS2) Phased Array L-band Synthetic Aperture Radar 2 (PALSAR-2) and the Uninhabited Aerial Vehicle Synthetic Aperture Radar (UAVSAR), of 12.50 m and 1.80 m resolution, respectively. SAR (Satellite Aperture Radar) imagery was acquired from the Advanced Land Observing Satellite 2 (ALOS2) Phased Array L-band Synthetic Aperture Radar 2 (PALSAR-2) mission available at the Alaska Satellite Facility (https://search.asf.alaska.edu/) while UAVSAR data were downloaded from the Jet Propulsion Laboratory at the California Institute of Technology (https://uavsar.jpl.nasa.gov/cgi-bin/data.pl). Radar mosaics have been previously processed by applying radiometric calibration and terrain correction to reveal surface characteristics.
Combining DEM, satellite, and radar imagery, paleochannels were reconstructed by tracing river signatures in erosion and deposition areas along streams. Ancient channels revealed river migration patterns and bend apex orientation observable on Google Earth based on the abandoned direction of the river (Shan et al., 2018). Depending on flow and sediment discharges, rivers tend to use the paleochannels structures usually formed by larger rivers, thus paleochannels dimensions are larger than those of underfit-scavenger rivers and try to develop their own planform configuration. Figure 2C shows a typical underfit-scavenger meandering channel being developed over paleochannels that were created by larger rivers. Likewise, Figure 2D shows paleochannels along a river including their scroll bars. Notice that paleochannels are classified into stages (depending on age), and depending on planform river dynamics, not all paleochannels are properly preserved.
4 Results
4.1 Modern and ancient river dynamics
Figure 3 shows the modern multitemporal analysis from 1985 to 2021 of large meandering (Huallaga, Ucayali, Puinahua) and anabranching (Marañon and Amazon) rivers surrounding the P-S wetland. Figures 3A, B show the Progreso and Santa Cruz, respectively, recent neck cutoffs along the Huallaga River (Valverde et al., 2019). The Santa Cruz cutoff occurred in 2018 producing an upstream erosional wave (Abad et al., 2012) that accelerated the Progresso cutoff in 2021. Figures 3G, I show two recent cutoffs along the Ucayali River (Abad et al., 2022a), unveiling a potential cutoff near Jenaro Herrera town (see Figure 3H).
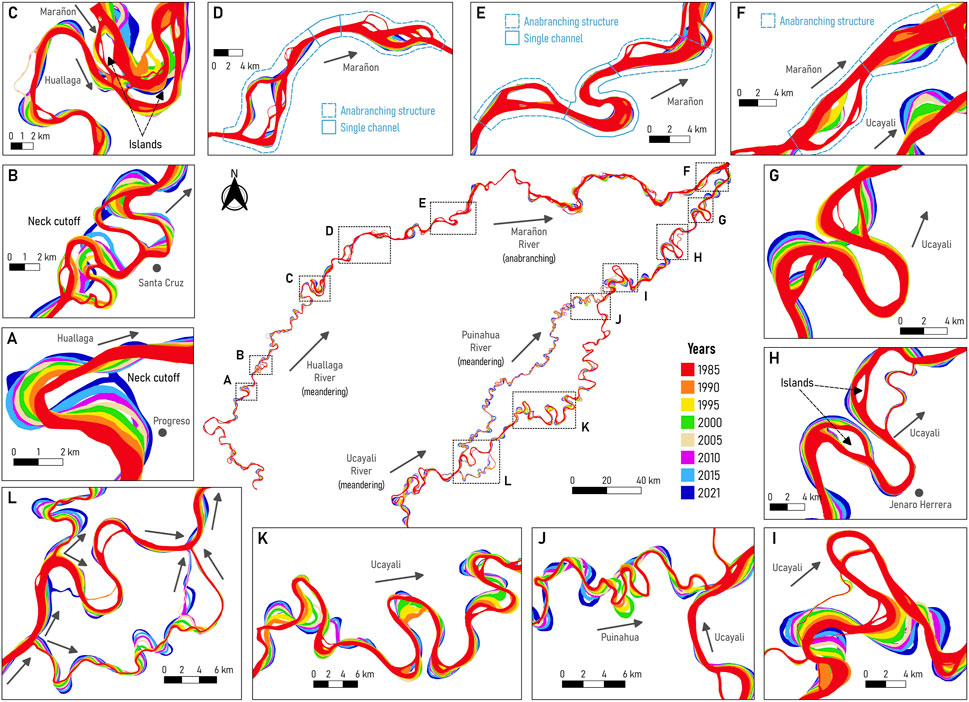
FIGURE 3. Modern River dynamics (from 1985 to 2021) in the P-S wetland. (A)] Huallaga River (near Progreso), (B) Huallaga River (near Santa Cruz), (C) Confluence of the Huallaga and Marañon Rivers, (D,E) Marañon River, (F) Confluence of the Marañon and Ucayali Rivers, (G–I) Lower Ucayali River, (J) Confluence of the Ucayali and Puinahua Rivers, (K) Ucayali River, (L) Bifurcation of the Ucayali and Puinahua Rivers.
Figures 3D, E show the planform configuration for the Marañon River, evidencing typical anabranching structures formed by islands and channels (main and secondary channels) which are most of the time connected with single channels. Indeed, Garcia et al. (2022) found a periodicity of 22.40 km for the occurrence of anabranching structures for the Peruvian Amazon River from Nauta to Santa Rosa (border with Colombia and Brazil). The anabranching structures have low migration rates compared to meandering channels (see Figure 3F), thus the confluence location of the Marañon and Ucayali Rivers (birthplace of the Amazon River) is dominated by the planform dynamics of the meandering Ucayali River (Garcia et al., 2022; Guerrero et al., 2022).
Abad et al. (2022a) described that bends along the lower Ucayali River transition from purely meandering to anabranching rivers, observing islands and multichannel structures. Figure 3K shows the planform dynamics of bends along the Ucayali River in the region where the Ucayali and Puinahua rivers share water flow. Figure 3L shows the bifurcation of the Ucayali River to form the Puinahua River and Figure 3J shows the confluence of the Puinahua and Ucayali Rivers. Notice that the bifurcation produces bends of varied sizes based on the trapping efficiency of water and sediment. In general, the planform migration rates of meandering rivers are higher than in anabranching rivers and cutoff occurrence happens in meandering rivers rather than in anabranching ones (Abad et al., 2013).
Figure 4 shows the reconstruction of paleochannels and oxbow lakes along the large meandering (Huallaga, Ucayali, Puinahua, and Tapiche) and anabranching (Marañon) rivers surrounding the P-S wetland, as well as those rivers (Samiria, Pacaya, and Yanayacu) located inside of the P-S wetland. The Huallaga, Ucayali, and Marañon rivers transport water and sediments originating in the Andes, the main source of sediments of the entire Amazon basin whereas the Tapiche River carries water and sediment from a watershed originated in the lowlands, indicating a lower sediment concentration than the Andean rivers. The Pacaya, Samiria, and Yanayacu Rivers have their origin in the P-S wetland, thus the water they conduct derives from multiple sources such as yearly flooding and springs from large rivers (Marañon, Ucayali, and Huallaga), and local rainfall (water table saturation-surface exposure, groundwater connectivity). These rivers present low sediment concentrations since they are not connected to the Andes region.
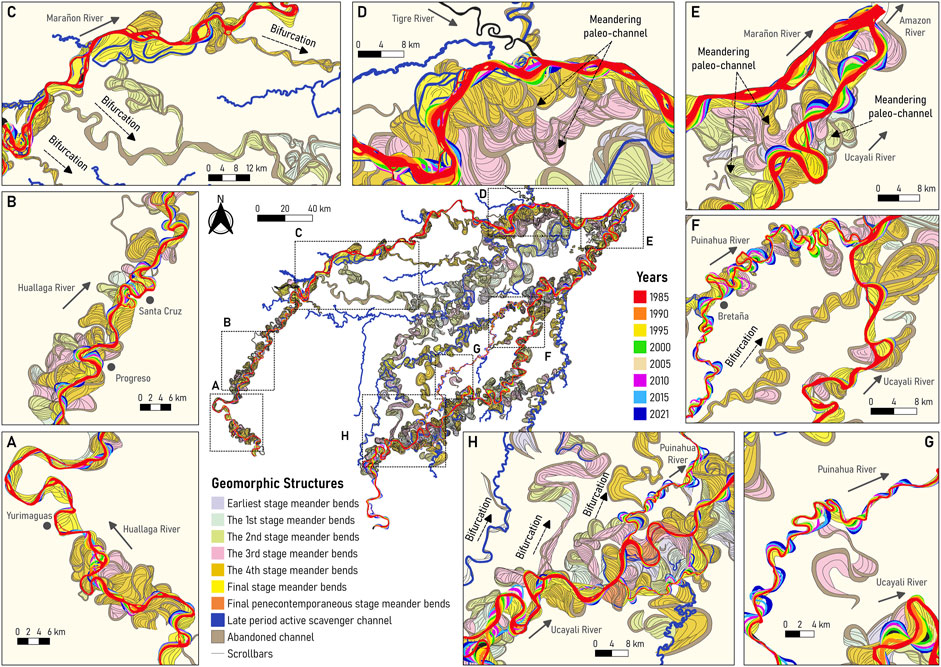
FIGURE 4. Ancient and modern river dynamics of the buffer zone in the P-S wetland. (A) Huallaga River near Yurimaguas city, (B) Huallaga River near Progreso and Santa Cruz cutoffs, (C) Birfurcated channels along the Marañon River, (D) Confluence of the Tigre and Marañon Rivers, (E) Confluence of the Marañon and Ucayali Rivers, (F) Confluence of the Puinahua and Ucayali Rivers, (G) Puinahua River, (H) Bifurcation of the Puinahua and Ucayali Rivers.
As discussed by Abad et al. (2013) and Marin-Diaz et al. (2020), planform dynamics show that Peruvian Amazon meandering rivers are more morphologically active than anabranching rivers. CITA (2021) described that lateral migration rates for meandering rivers are: 25.16 m/year (Huallaga), 50.47 m/year (Ucayali). Figures 4A, B illustrate paleochannels with comparable size as those of modern channels along the Huallaga River. Figure 4C shows several bifurcated paleochannels along the Marañon River that describe meander-type characteristics. Likewise, Figure 4D shows that the Marañon Rivers present high-sinuosity and high-amplitude paleochannels, contrary to those of modern dynamics. Depending on the occurrence of these bifurcated channels, the Marañon River has changed from purely anabranching―as today―to transition to a meandering configuration, thus presenting meander-type paleochannels. As described by Abad et al., 2013; Marin-Diaz et al. (2020), meandering rivers transition into anabranching rivers occur when water and sediment discharge are added (confluences), thus these river signatures suggested that the opposite could be also possible (bifurcations).
Figure 4E show the paleochannels in the confluence of the Marañon and Ucayali Rivers where meander-type paleochannels clearly dominated the floodplain whereas those paleochannels derived from the Ucayali River dominated the confluence location. Due to the geology and digital terrain model, the confluence valley is narrowed (see Figure 1B), thus allowing the Amazon River to shape (Guerrero et al., 2022).
Figure 4F shows the confluence of the Puinahua and Ucayali Rivers. As illustrated in Figure 4G, the Puinahua shows a mixture of paleochannels generated by the Puinahua itself together with paleochannels that were formed by the Ucayali River (large-size channels). The confluence between the Puinahua and Ucayali Rivers appears to have been placed in distinct locations depending on the bifurcated channels or the drainage system of the area. Figure 4H shows the bifurcated channels along the Ucayali River, describing that several rivers with similar characteristics as the Puinahua River have coexisted in the region. These paleochannels have similar patterns to those of the modern Ucayali River. In this study, the paleochannels were classified in different stages (Figure 4), describing what is older or younger, however, there is still a need for dating the sediments along the paleochannels and describing their age.
4.2 Underfit-scavenger rivers: Pacaya, Samiria, Yanayacu, Tapiche, and Sungaro
In Figure 5, underfit-scavenger rivers have used paleochannels to build up their planform dynamics. Figure 5A shows the Yanayacu River’s centerline that follows the Ucayali’s paleochannel except in regions where 1) connects different paleochannels, and 2) develops its own meanders, which are smaller size compared to the Ucayali’s paleochannel. Figures 5B, C show that Samiria’s planform configuration follows Ucayali’s paleochannel configuration but there is a region where significant reworking of the floodplain is observed. It suggests that the Samiria River produces its own meanders but still uses the paleochannels as its main alignment. Figure 5D shows that the Puinahua River is developing its own meanders slightly smaller than those of the Ucayali River but in certain regions, it uses the Ucayali’s paleochannels as the main alignment. As observed in Figure 3, the Puinahua modern migration has increased in the last years (from 1985 to 2021) and capturing more water and sediments; it suggests that the Puinahua River might become the new Ucayali River configuration in the upcoming years. Figures 5E, F show the Pacaya’s planform configuration and, similarly to the Samiria River, it follows the Ucayali’s paleochannel as the main alignment and develops smaller meanders. The Samiria, Yanayacu, and Pacaya rivers are underfit-scavenger meanders that are still developing their own size of meanders but most of the time they use the Ucayali’s paleochannel configuration. Figures 5G, H show that the Tapiche River has developed small-size meanders but clearly above the Ucayali’s paleochannel. This is a good example of how a underfit-scavenger river can modify significantly the paleochannel environment. Similar processes occur with the Sungaro River as shown in Figure 5I.
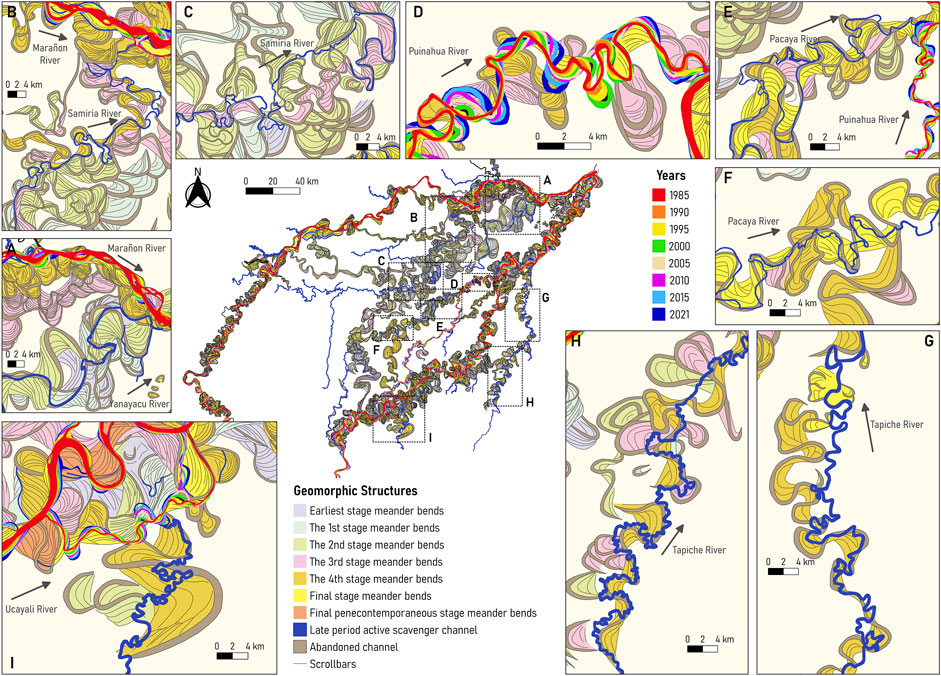
FIGURE 5. Free and underfit-scavenger river dynamics in the P-S wetland: (A)] Yanayacu River, (B,C) Samiria River, (D) Puinahua River, (E) confluence between the Pacaya and Puinahua Rivers, (F) Pacaya River, (G,H) Tapiche River, and (I) Sungaro River.
4.3 Morphometrics of free and underfit-scavenger rivers, and paleochannels
The goal is to analyze the planform behavior of the rivers that interact with large-scale paleochannels, thus the planform curvature for the Samiria (L = 1542W), Pacaya (L = 2192W), and Tapiche (L = 2225W) Rivers were extracted. Following Vermeulen et al. (2016)’s methodology, several regions with small-scale meanders are observed in Figure 6, those that are being reworked by the river itself (see Figure 5), that later connect to large-scale meanders, those of the size of the large-scale paleochannels from the ancient dynamics of the Ucayali River. The Samiria and Pacaya Rivers shape some smaller meanders on top of large-scale meanders (paleochannels from the Ucayali River), however for the case of the Tapiche River, the shaping of smaller meanders is more abundant, since the Tapiche River carries out more water and sediments from its own upstream watershed, in contrast to the Samiria and Pacaya Rivers that don´t have a clear watershed, they are interconnected, especially at high flows.
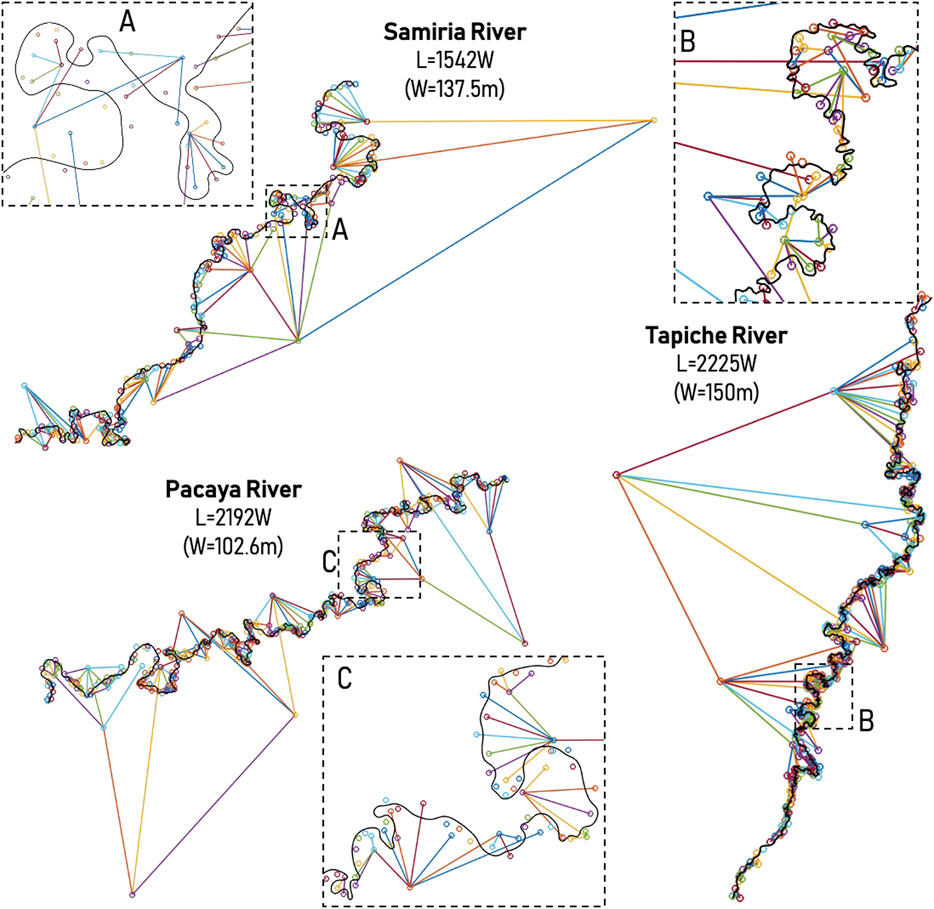
FIGURE 6. Hierarchically ordered in a tree using the methodology by Vermeulen et al. (2016). L is the longitudinal scale, and W is the channel width. (A–C) show examples of tree-based scales for the underfit-scavenger rivers Samiria, Tapiche and Pacaya, respectively.
By using the MStaT toolbox (Ruben-Dominguez et al., 2021), for the case of modern rivers, the skewness (see Figure 2) of meandering channels shows that more DS than US oriented bends are found along the Ucayali (59% vs. 15%), Puinahua (66% vs. 23%), Huallaga (46% vs. 29%), Tapiche (61% vs. 21%), Pacaya (56% vs. 24%) and Samiria (59% vs. 19%) Rivers. Along these rivers, a range between 11% and 26% are described as compound or symmetric bends. Besides, the averaged channel width for the modern rivers (using 2021 imagery) are: 602.44 m (Ucayali). 454.10 m (Puinahua), 370.71 m (Huallaga), 150.92 m (Tapiche), 102.57 m (Pacaya), 137.45 m (Samiria).
For the case of ancient rivers, paleochannels are usually reconstructed individually and not necessarily the alignment of the original river (just before the bend was abandoned) is accurate, thus, the assessment of US and DS bends becomes more challenging. Similarly, the averaged channel width and the arc-wavelength of the paleochannels are reduced from the original ones, since water does not flow constantly (temporal connectivity) and some portions are covered by vegetation.
By using the channel centerline of all rivers except the Marañon River (anabranching), the sinuosity and arc-wavelength are described in Figures 7A, B, respectively. The sinuosity for the modern bends (as those in Figure 3) shows that the highest percentage (between 30 and 50%) of bends is between 1.0 and 1.2 (being the Sinuosity[Samiria]> Sinuosity[Pacaya]> Sinuosity[Tapiche]). Similarly for the Ucayali, Puinahua, and Huallaga, most of the modern bends are between 1.0 and 1.2 sinuosity. Fewer bends reach 2.0 sinuosity and are rarely larger than 3.0. For the case of paleochannel’s sinuosity, for the case of the Pacaya and Samiria, more than 50% of the bends are in the range of 1.0–1.2 sinuosity, which does not happen to the Tapiche River. The Ucayali River also has close to 50% of the paleochannels around the 1.0 to 1.2 sinuosity, similar to the ones found near the Pacaya and Samiria Rivers. A decaying behavior of the sinuosity occurs for all rivers, similar to the modern bends; however, when the sinuosity is higher than 6.0 (40% of the paleochannels near the Pacaya River), there is evidence of high-amplitude bends that are preserved after cutoff occurred. A similar trend is also observed for the Ucayali, Puinahua, and Huallaga paleochannels, suggesting that paleochannel signature preserves sinuosities near cutoff events.
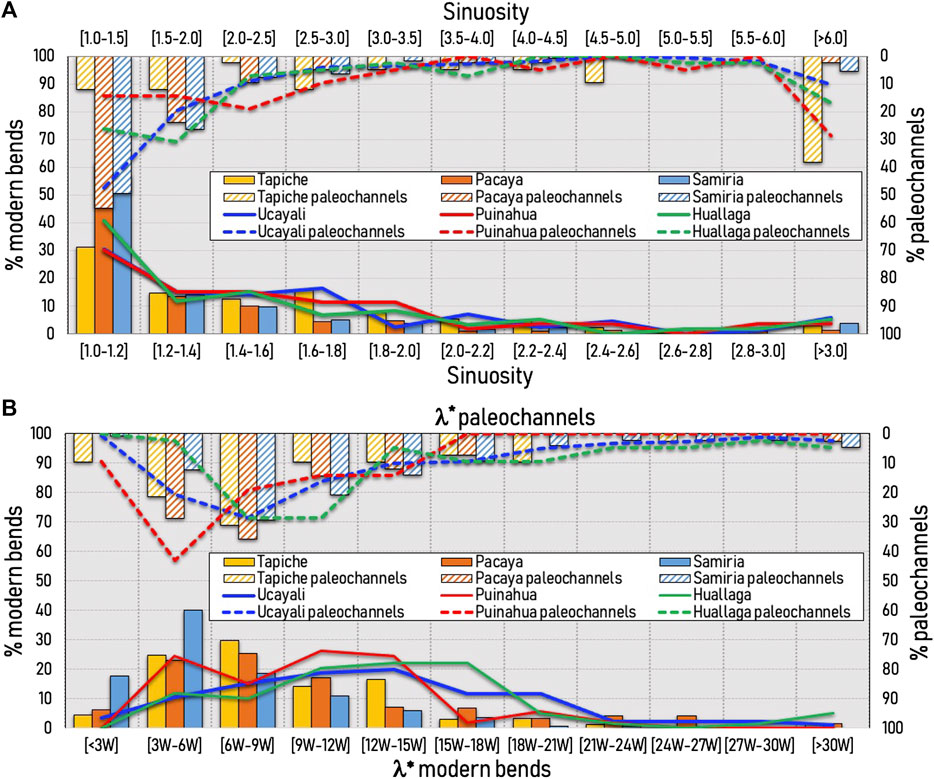
FIGURE 7. (A) Sinuosity and (B) arc-wavelength of the rivers in the P-S wetland (modern and paleochannels). The percentage plots are normalized using the total amount of bends for each river. The dimensionless arc-wavelength for the modern bends is obtained using the river width for each river; however, the dimensionless arc-wavelength for the paleochannels is obtained using the Ucayali’s width (except for the Huallaga River).
Figure 7B shows the normalized arc-wavelength (λ* = λ/W) of all rivers except the Marañon River. The λ* for the majority of modern bends of the Pacaya, Tapiche and Samiria Rivers are 3W–18W, 3W–15W, and 3W–15W, respectively. However, for the modern bends along the Ucayali, Puinahua, and Huallaga Rivers, the λ* are 3W–21W, 3W–18W, and 3W–21W, respectively; showing that the self-meandering rivers tend to have slightly larger meanders. The normalization of the arc-wavelength was performed for all paleochannels within the P-S wetland (except those from the Huallaga River) using the Ucayali’s current width since there is evidence that the Ucayali’s paleochannels are the main alignment for the development of the planform configuration for the Pacaya, Samiria, Yanayacu, Puinahua, and Tapiche Rivers. The peak of paleochannels for the Pacaya, Samiria and Tapiche rivers has moved towards having larger paleochannels, while for the case of the Ucayali and Huallaga Rivers the peak has moved towards having smaller paleochannels. Notice also that the river signatures shown in Figures 4, 5 does not fully reconstruct the entire paleochannel bend at cutoff (vegetation cover or partial or full loss of connectivity to the main river), thus a tendency for smaller bends was incorporated into the analysis. Moreover, more than 40% of paleochannels along the Puinahua River have λ* between three and six channel widths (similar peak behavior for the modern bends), showing that the Puinahua River developed paleochannels by itself that interact with paleochannels from the Ucayali River.
4.4 Relationship between modern and ancient river dynamics and fisheries
Figure 8 shows the overlap between the commercial fish extraction between 2015 and 2019 with the modern and ancient river dynamics in the P-S wetland. Notice that the fish extraction dataset corresponds to annual catch (Wildlife Conservation Society, 2022; Wildlife Conservation Society, 2020). Therefore, the following analysis does not provide conclusive evidence of a relationship between hydrology and fish biomass but indirect evidence that river dynamics impacts on fisheries of the PSNR.
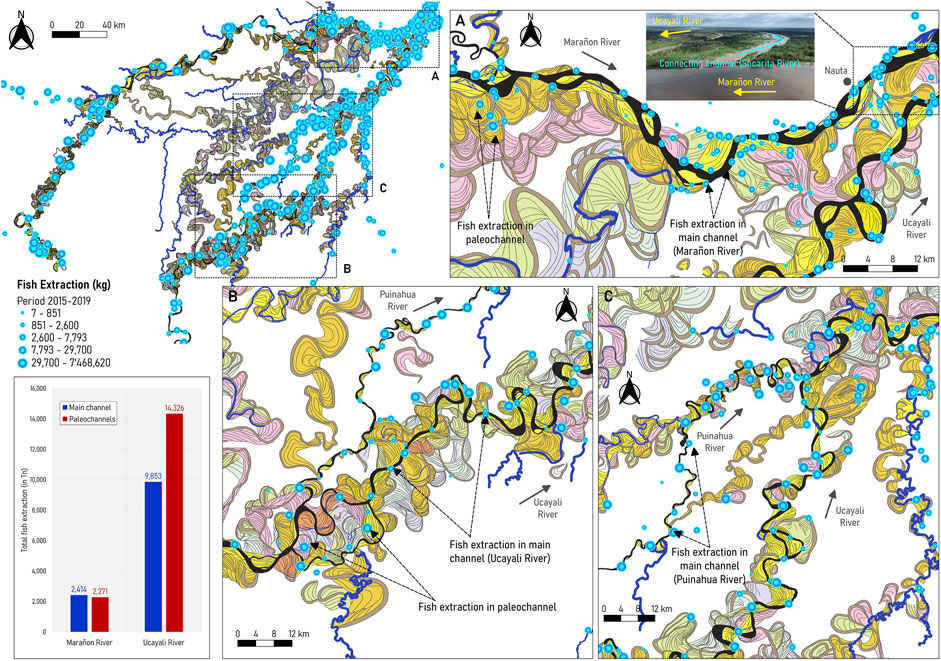
FIGURE 8. Modern and ancient river dynamics and fish production (photo courtesy of Senamhi-Loreto) (A) in the P-S wetland (reported by GOREL and WCS), (B) near the confluence of the Marañon and Ucayali Rivers, and (C) around the confluence of the Puinahua and Tapiche rivers with the Ucayali River. In (B), the picture shows the connecting channel (sacarita), a connecting channel that is well-observed during the peak of the high-flow season (around May 2022). Fisheries data provided by Wildlife Conservation Society, 2022.
As observed in Figure 8A, fishing occurs along the main channel and several paleochannels of the Marañon River, especially in the connecting channels (known as “sacaritas”) near Nauta city. These connecting channels become active and more visible during high flows (from March to June). Most of them were left by the Ucayali River dynamics, as indicated by Dumont. (1991). Along the Marañon River, fish extraction was similar in the main channel and paleochannels. On the other hand, in the Ucayali River, there are more zones for commercial fishing than in the Marañon River, located along the main channel (see Figures 8B, C). However, more fish are extracted from paleochannels than from the main channel (14,326 Tn in paleochannels compared to 9,853 Tn along the main channel of the Ucayali River).
Arantes et al. (2019) suggested that fish biomass was strongly associated with forest cover during the low-water season, suggesting that aquatic macrophyte cover plays a key role in maintaining functional richness and dispersion. Along the Marañon and Ucayali Rivers (Figure 9), the most representative and most abundant fish species include the black prochilodus or boquichico (Prochilodus nigricans), Pinecone Pleco or carachama (Pseudorinelepis genibarbis), llambina (Potamorhina altamazonica), tiger fish or fasaco (Hoplias malabaricus), among others. In particular, the Prochilodus nigricans is an important fishery species in the Andean-Amazon region, a detritus feeder that lives in floodplains and channels during the low-water season to later migrate to tributaries (McClain and Naiman, 2008). The Pseudorinelepis genibarbis is an omnivorous armored catfish that live in low-oxygen waters that can be found in floating meadows and oxbow lakes (Virgilio et al., 2020). As shown in Figure 9, fishing zones of Prochilodus nigricans include paleochannels within the Marañon and Ucayali floodplains; these areas are composed of palm swamps (Figure 10B) that contain detritus rich in amino acids with a greater nutritional value (Bowen, 1983). By contrast, Pseudorinelepis genibarbis is extracted from the riverbanks and ancient channels, corresponding to stagnated water zones.
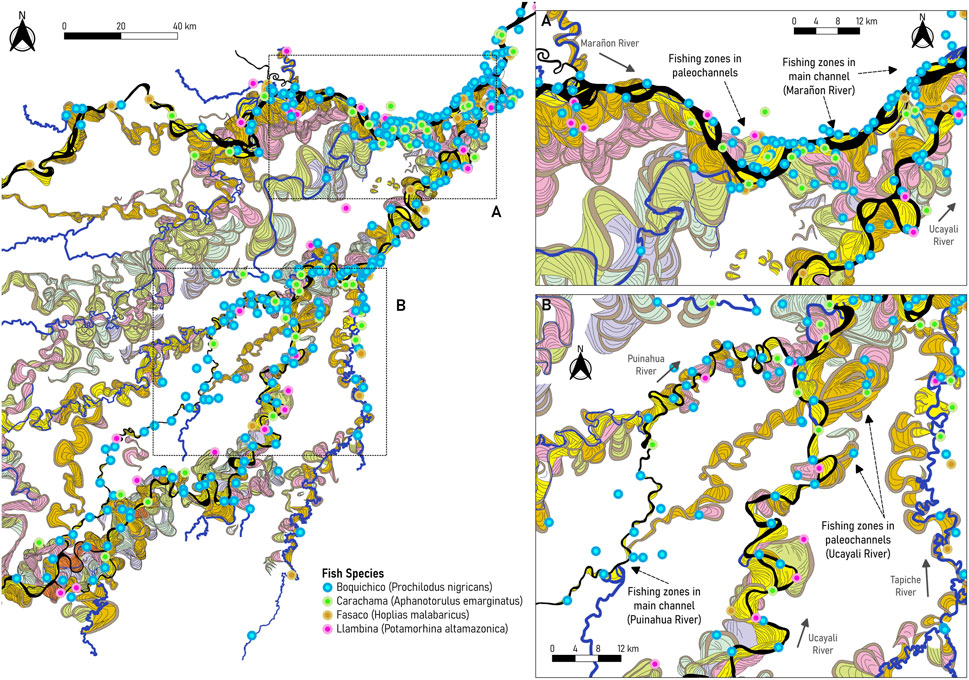
FIGURE 9. Most representative and abundant fish species for local commercial fishing in the PSNR (A) along the Marañon River, and (B) along the Ucayali and Puinahua rivers. Fisheries data provided by Wildlife Conservation Society, 2022.
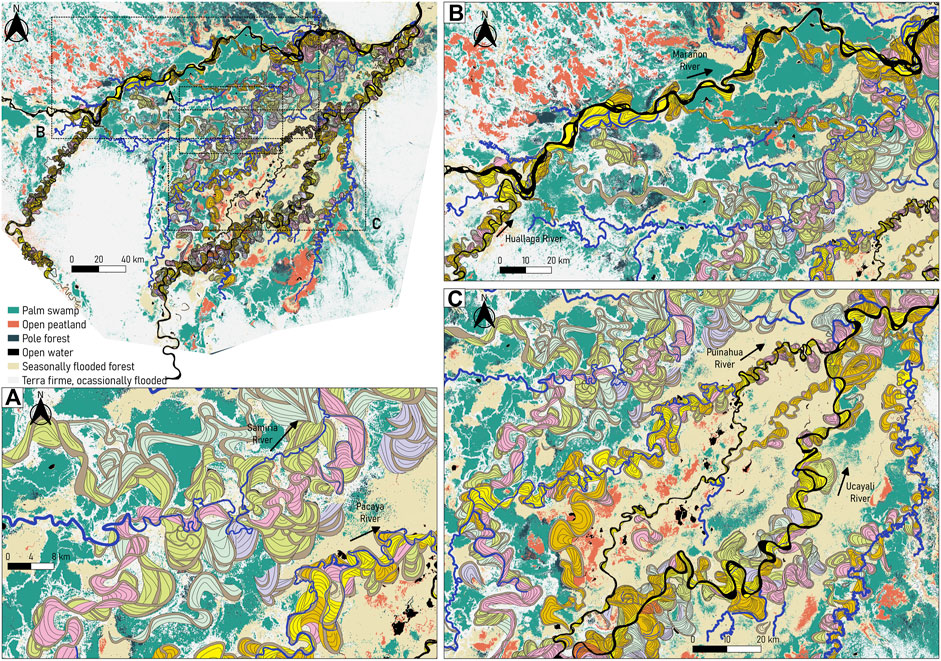
FIGURE 10. Modern and ancient river dynamics superposed to the vegetation cover map developed by Draper et al. (2014). (A) Section within the PSNR, showing the vegetation mosaic between the Samiria and Pacaya Rivers. (B) Section along the Marañon River surrounded by larger areas of palm swamps. (C) Section of the Ucayali River, covered by seasonally flooded forests and several areas of open peatlands. Land cover data provided by Draper et al. (2014).
In the last decade, trends indicated a decline in fish population and richness due to socio-economic and unsustainable practices, such as overharvesting and dams construction (Gerstner et al., 2006; Anderson et al., 2018; Kirkland et al., 2020), leading to the threatening of certain fish species, such as the paiche (Arapaima gigas) (Bodmer and Puertas, 2007) and the aruana (Osteoglossum bicirrhosum). Since tropical forests support fishery as the main source of protein and income for Indigenous communities (East et al., 2005), understanding river and floodplain connectivity is vital to improve the management of fisheries and aquatic life yield. Likewise, quantifying river planform dynamics in terms of flooding, sediment transport, and ecological processes will help understand the origin of species diversity and abundance.
5 Discussion
The Science Panel for the Amazon. (2021) described that the biodiversity of terrestrial and freshwater ecosystems is under the constant pressure from socioeconomic activities, such as deforestation for agricultural development and mining (Volckhausen, 2020), pollution (especially from constant oil spills; Sierra, 2020), climate change and the development of river-related infrastructure (dams, roads, waterways; Gonzales, 2019; Sierra, 2019), affecting indigenous lands and protected areas as well. Human-induced disturbances are generally associated with habitat degradation, loss of species, and, consequently, loss of ecosystem services. For instance, sedimentation (excessive deposition of fine particles) may cause impacts on community composition and species losses, thus affecting energy flow and trophic diversity (Osmundson et al., 2002; Burdon et al., 2013; Burdon et al., 2020). In the Amazon region, little is known about how ecosystem components (e.g., plant-animal interactions, habitat distribution) might respond to these pressures. The lack of adequate management strategies is affecting the management of not only aquatic habitats but also carbon-rich ecosystems and terrestrial biodiversity (Castello et al., 2013).
Previous studies indicates that fisheries productivity in the Amazon is linked to seasonal water level changes which conditions feeding and spawning opportunities (Junk et al., 1989; Isaac et al., 2016). Currently, population declines are driven by habitat fragmentation, overharvesting, barriers to migration, and climate change (Duponchelle et al., 2021). In general, Anderson et al. (2018), Gerstner et al. (2006) and Kirkland et al. (2020) have discussed how pressures (e.g., infrastructure) on rivers can affect aquatic diversity (fish richness and population; Wildlife Conservation Society, 2020). Thus, it is very relevant to incorporate the link between river geomorphology (from water to sediments to erosion/depositional patterns) and biodiversity, not only for baseline studies but for predicting future impacts, thus the science and information can be of support for better decision-making.
A major concern in the Amazon are the impacts produced by the existing dams and the potential impact of tens of planned dams. River disturbance by dams may affect sediment supplies river channel and floodplain dynamics and can decrease or even suppress ecological connectivity among populations of aquatic organisms and organisms that depend on flood seasonality (Finer and Jenkins, 2012; Flecker et al., 2022). Latrubesse et al. (2017) provided an analysis of the potential irreparable consequences for Amazon rivers that can be expected at different scales and sub-basins by more than 400 dam that exist already or are under consideration for construction, especially along the upper Marañón river (Flecker et al., 2022). The analysis to vulnerability by dams by using the Dam Environmental Vulnerability Index (DEVI) points that the Marañon and Ucayali are the most vulnerable Andean Rivers. Regarding flow regulation and decrease of sediment load, the Ucayali River, the most active river flowing through the P-S wetland, is the most sensitive Andean River to dam building (Latrubesse et al., 2017). Reductions of these factors by dam construction pose threats to wetland creation and maintenance. As discussed above, the anabranching Marañon River is less dynamic in terms of sediment regime and changing morphology but is critically threatened by a large number of planned and built dams in mountainous reaches of its main channel and it can decrease both the sediment supplies and inundation potential which maintain floodplain and the peripheral wetlands environments of PSNR. Furthermore, if sediment and water is reduced in an anabranching system, a transitional (from anabranching to meandering) condition could be triggered, therefore, promoting significant lateral migration of rivers (similar to the bifurcated channels in Figure 4C). The assessment of the potential impacts on the P-S wetlands by hydrophysical modifications of the Ucayali and Marañon by dams would need a priority. Both the Ucayali and Marañón rivers interact with the P-S wetland in terms of hydrology and geomorphology, proving the wetland with sediment and nutrient fluxes every year (hydrology dominated) and when there are bifurcations or significant planform modifications like the Puinahua River (geomorphology dominated), thus, the lateral connectivity of both systems is important for the sustainability of the biodiversity of the P-S wetland.
Likewise, this study provides indirect evidence that incorporating river dynamics is central to determine the distribution and to characterize the hydrophysical genesis and behavior of river wetlands and related peatland-type ecosystems. Figure 10 shows the overlap between the peat and non-peat-forming vegetation with the modern and ancient river dynamics in the P-S wetland unveiling vegetation patterns, composed of large areas of palm swamps. Palm swamps are nutrient-rich peatlands that resulted from a higher frequency of river avulsions and river dynamism (Draper et al., 2014), allowing oligotrophic conditions (low levels of nutrients) for certain aquatic species to spawn and feed (Lähteenoja and Page, 2011). Moreover, the current and past dynamics of the Ucayali River, evidenced by the complex network of paleochannels, support the development of early-successional vegetation.
As observed in Figure 10A, the recent low dynamism of the Marañon River (ancient and modern conditions) suggested appropriate conditions for pole forest and late-successional vegetation to develop, also allowing peat to accumulate and ombrotrophic condition (i.e., rainfall fed) to remain (Draper et al., 2014). On the other hand, in the bifurcation of the Ucayali River with the Puinahua River there are patches of open peatlands that are prone to frequent fluvial activity due to their high dynamics, as shown in Figures 10A, C. Large and dynamic rivers, such as the Ucayali River, then help maintain higher nutrient inputs to these areas, impeding to ombrotophic conditions and late-successional vegetation to develop (Dumont, 1991; Draper et al., 2014).
6 Conclusion
The hydrologic cycles in the Marañon and Ucayali Rivers are dominated by the precipitation patterns of the eastern Peruvian Mountains. Every year, these two rivers flood during the rainy season depositing nutrient-rich sediment into the flooded forests. Furthermore, the planform complex geomorphology between the Marañon and Ucayali rivers, showing numerous islands, oxbow lakes, and cutoffs within floodplains, has resulted in the creation of underfit-scavenger streams like Pacaya, Samiria and Tapiche rivers. In this research, modern and ancient signatures unveiled high river dynamics and shifting by the Ucayali River, evidencing frequent avulsions and erosional/depositional processes. The resultant environmental mosaic of the P-S wetland is the consequence of a palimpsest of relict geomorphological fluvial features that imprint spatial control to the hydrosedimentological routing and flooding processes in the wetlands. This investigation provides an integrative characterization of how fluvial shifting in the PSNR created suitable conditions and hydro-connectivity process to sustain both nutrient-poor and nutrient-rich environments, allowing different fish assemblages to spawn. The identification and characterization of present and paleo-fluvial landforms are then crucial for fishery management and carbon stock estimations.
During the high flow season, a considerable number of fluvial networks traverse the watershed between the Ucayali and Marañon rivers following ancient fluvial landforms (paleochannels). Annually, the flooded forests in the PSNR are enriched with nutrient-dense sediments generated by erosional processes upstream of the Marañon and Ucayali Rivers, which are later transported downstream and over the lowlands by the floods. Algae growth in the Amazon relies on the phosphorus and nitrogen conducted from the main rivers to the floodplains, and so the magnitude of the flood determines the number of algae produced and thus the number of fish that feed on these algae (Goulding, 1980). Additionally, these floods enable the lateral migration of fish and other aquatic life and the movement of nutrients from these rivers to one another or nearby streams. Therefore, the river dynamics associated with hydrogeomorphological events could favor the fragmentation, isolation, and dispersion of populations through changes in river courses (avulsions and captures phenomena) and disconnection and recapture of oxbow lakes from the main channel. The hydrogeomorphological events would favor allopatric speciation and then, during reconnections or course changes, it would lead to colonization of the sub-basins by neo-species.
Data availability statement
The raw data supporting the conclusion of this article will be made available by the authors, without undue reservation.
Author contributions
Conceptualization, JA; methods and materials, JA, TR, WR, and JS; formal analysis, TR, JA, and WR; data curation, TR, WR, and JS; interpretation and discussion, JA, TR, EL, and JS; writing—original draft preparation, TR and JA; review and editing, TR, JA, EL, and JS; visualization, TR, WR, and JS; supervision, JA; project administration, JA and TR; funding acquisition, JA. All authors have read and agreed to the published version of the manuscript.
Funding
This manuscript was started during JS visit to JA research group (based on visiting grant: National Natural Science Foundation of China, No. 41972098) and finalized based on RED YAKU’s seeding funding.
Acknowledgments
The authors thank the staff from the Peru Program of the Wildlife Conservation Society—WCS and Tim Baker (University of Leeds and IIAP) for providing the fisheries and vegetation cover map dataset, respectively. Thanks to the Peruvian institutions (Directorate of Hydrography and Navigation from the Peruvian Navy, the National Service of Natural Areas Protected by the State-SERNANP and the National Meteorology and Hydrology Service of Peru-SENAMHI) for providing insightful discussion related to P-S wetland. UAVSAR data courtesy NASA/JPL-Caltech. The authors also thank the reviewers and editors for their constructive comments on the manuscript.
Conflict of interest
The authors declare that the research was conducted in the absence of any commercial or financial relationships that could be construed as a potential conflict of interest.
Publisher’s note
All claims expressed in this article are solely those of the authors and do not necessarily represent those of their affiliated organizations, or those of the publisher, the editors and the reviewers. Any product that may be evaluated in this article, or claim that may be made by its manufacturer, is not guaranteed or endorsed by the publisher.
Supplementary material
The Supplementary Material for this article can be found online at: https://www.frontiersin.org/articles/10.3389/fenvs.2023.1082619/full#supplementary-material
References
Abad, J. D., and Garcia, M. H. (2009a). Experiments in a high-amplitude kinoshita meandering channel: 1. Implications of bend orientation on mean and turbulent flow structure. Water Resour. Res. 45, 2. doi:10.1029/2008wr007016
Abad, J. D., and Garcia, M. H. (2009b). Experiments in a high-amplitude kinoshita meandering channel: 2. Implications of bend orientation on bed morphodynamics. Water Resour. Res. 45, W02402. doi:10.1029/2008wr007017
Abad, J. D., Mendoza, A., Arceo, K., Torres, Z., Valverde, H., Medina, G., et al. (2022a). Planform dynamics and cut-off processes in the lower Ucayali River, Peruvian Amazon. Water 14 (19), 3059. doi:10.3390/w14193059
Abad, J. D., Motta, D., Guerrero, L., Paredes, M., Kuroiwa, J., and Garcia, M. H. (2022b). Hydrogeomorphology of asymmetric meandering channels: Experiments and field evidence. Water Resources Research.
Abad, J. D., Peralta, B., Paredes, J., Frias, C., Gutierrez, R., and Montoro, H. (2012). The meandering Ucayali River, a cyclic adaptation of cutoff and planform migration. San Jose, Costa Rica: River Flow.
Abad, J. D., Vizcarra, J., Paredes, J., Montoro, H., Frias, C., and Holguin, C. (2013). Morphodynamics of the upper peruvian amazonian rivers, implications into fluvial transportation EUT Edizioni Università di Trieste. Avaliable at: http://hdl.handle.net/10077/8822.
Albert, J. S., Carvalho, T. P., Petry, P., Holder, M. A., Maxime, E. L., Espino, J., et al. (2011). Aquatic biodiversity in the Amazon: Habitat specialization and geographic isolation promote species richness. Animals 1 (2), 205–241. doi:10.3390/ani1020205
Aldea-Guevara, M. I., Hargrove, J., and Austin, J. D. (2013). Diversity and geneflow in a migratory frugivorous fish: Implications for amazonian habitat connectivity. Conserv. Genet. 14 (5), 935–942. doi:10.1007/s10592-012-0442-y
Alsdorf, D. E. (2003). Water storage of the central Amazon floodplain measured with GIS and remote sensing imagery. Ann. Assoc. Am. Geogr. 93 (1), 55–66. doi:10.1111/1467-8306.93105
Anderson, E. P., Jenkins, C. N., Heilpern, S., Maldonado-Ocampo, J. A., Carvajal-Vallejos, F. M., Encalada, A. C., et al. (2018). Fragmentation of Andes-to-Amazon connectivity by hydropower dams. Sci. Adv. 4 (1), eaao1642. doi:10.1126/sciadv.aao1642
Arantes, C. C., Winemiller, K. O., Asher, A., Castello, L., Hess, L. L., Petrere, M., et al. (2019). Floodplain land cover affects biomass distribution of fish functional diversity in the Amazon River. Sci. Rep. 9 (1), 16684–16713. doi:10.1038/s41598-019-52243-0
Bodmer, R. E., and Puertas, P. E. (2007). “Impacts of displacement in the pacaya-samiria national Reserve, Peru,” in Protected areas and human displacement: A conservation perspective (Wildlife C, pp. 29–33). Editors E. Redford, and K. H. Fearn (New York, USA: Wildlife Conservation Society). Avaliable at: http://indiaenvironmentportal.org.in/files/Protected Areas and human displacement.pdf.
Bowen, S. H. (1983). Detritivory in neotropical fish communities. Environ. Biol. Fishes 9 (2), 137–144. doi:10.1007/BF00690858
Burdon, F. J., McIntosh, A. R., and Harding, J. S. (2013). Habitat loss drives threshold response of benthic invertebrate communities to deposited sediment in agricultural streams. Ecol. Appl. 23 (5), 1036–1047. doi:10.1890/12-1190.1
Burdon, F. J., McIntosh, A. R., and Harding, J. S. (2020). Mechanisms of trophic niche compression: Evidence from landscape disturbance. J. Animal Ecol. 89 (3), 730–744. doi:10.1111/1365-2656.13142
Castello, L., Mcgrath, D. G., Hess, L. L., Coe, M. T., Lefebvre, P. A., Petry, P., et al. (2013). The vulnerability of Amazon freshwater ecosystems. Conserv. Lett. 6 (4), 217–229. doi:10.1111/conl.12008
Catano, Y., Abad, J. D., and Garcia, M. H. (2009). Characterization of bedform morphology generated under combined flows and currents using wavelet analysis. Ocean. Eng. 36, 617–632. doi:10.1016/j.oceaneng.2009.01.014
CITA (2021). Guías metodológicas para el Desarrollo de la línea base física de los ríos en Andes-Amazonía. Centro de Investigación y Tecnología del Agua CITA-UTEC. Lima, Perú: Universidad de Ingeniería y Tecnología.
Couto, T. B., Zuanon, J., Olden, J. D., and Ferraz, G. (2017). Longitudinal variability in lateral hydrologic connectivity shapes fish occurrence in temporary floodplain ponds. Can. J. Fish. Aquatic Sci. 75 (2), 319–328. doi:10.1139/cjfas-2016-0388
da Silva-Caminha, S. A. F., D’Apolito, C., Jaramillo, C., Espinosa, B. S., and Rueda, M. (2020). Palynostratigraphy of the ramon and Solimões formations in the acre basin, Brazil. J. S. Am. Earth Sci. 103, 102720. doi:10.1016/j.jsames.2020.102720
Draper, F. C., Roucoux, K. H., Lawson, I. T., Mitchard, E. T. A., Honorio Coronado, E. N., Lähteenoja, O., et al. (2014). The distribution and amount of carbon in the largest peatland complex in Amazonia. Environ. Res. Lett. 9 (12), 124017. doi:10.1088/1748-9326/9/12/124017
Dumont, J. F. (1991). Fluvial shifting in the Ucamara Depression as related to the neotectonics of the Andean foreland Brazilian craton border (Peru). Geodynamique 6 (1), 9–20.
Dumont, J. F., and Garcia, F. (1991). Active subsidence controlled by basement structures in the Marañon Basin of northeastern Peru. IAHS Fourth Int. Symposium Land Subsidence 200, 343–350.
Dumont, J. F. (1992). Rasgos morfoestructurales de la llanura amazónica del perú: Efecto de la neotectónica sobre los cambios fluviales y la delimitación de las provincias morfológicas. Bull. l’Institut Français d’études Andin. 21 (3), 801–833. doi:10.3406/bifea.1992.1089
Duncan, W. P., and Fernandes, M. N. (2010). Physicochemical characterization of the white, black, and clearwater rivers of the Amazon Basin and its implications on the distribution of freshwater stingrays (Chondrichthyes, Potamotrygonidae). Pan-American J. Aquatic Sci. 5 (3), 454–464.
Duponchelle, F., Isaac, V. J., Rodrigues Da Costa Doria, C., Van Damme, P., Herrera-R, G., Anderson, E. P., et al. (2013). Conservation of migratory fishes in the Amazon basin. Aquatic Conservation: Marine and Freshwater Ecosystems 31 1087–1105. doi:10.1002/aqc.3550
East, T., Kümpel, N. F., Milner-Gulland, E. J., and Rowcliffe, J. M. (2005). Determinants of urban bushmeat consumption in Río Muni, Equatorial Guinea. Biol. Conserv. 126 (2), 206–215. doi:10.1016/j.biocon.2005.05.012
Ferreira, L. V., and Stohlgren, T. J. (1999). Effects of river level fluctuation on plant species richness, diversity, and distribution in a floodplain forest in Central Amazonia. Oecologia 120 (4), 582–587. doi:10.1007/s004420050893
Finer, M., and Jenkins, C. N. (2012). Proliferation of hydroelectric dams in the Andean Amazon and implications for Andes-Amazon connectivity. PLoS One 7 (4), e35126. doi:10.1371/journal.pone.0035126
Flecker, A. S., Shi, Q., Almeida, R. M., Angarita, H., Gomes-Selman, J. M., García-Villacorta, R., et al. (2022). Reducing adverse impacts of Amazon hydropower expansion. Science 375 (6582), 753–760. doi:10.1126/science.abj4017
Frias, C. E., Abad, J. D., Mendoza, A., Paredes, J., Ortals, C., and Montoro, H. (2015). Planform evolution of two anabranching structures in the Upper Peruvian Amazon River. Water Resour. Res., 51 (4), 2742–2759. doi:10.1002/2014WR015836
Garcia, A. P., Marin-Diaz, J., Escobar, C., Ortals, C., and Abad, J. D. (2022). The Peruvian upper Amazon River: A planform morphologic characterization of single and compound anabranching structures. Under review.
Gerstner, C. L., Ortega, H., Sanchez, H., and Graham, D. L. (2006). Effects of the freshwater aquarium trade on wild fish populations in differentially-fished areas of the Peruvian Amazon. J. Fish Biol. 68 (3), 862–875. doi:10.1111/j.0022-1112.2006.00978.x
Ghinassi, M., Nemec, W., Aldinucci, M., Nehyba, S., Özaksoy, V., and Fidolini, F. (2014). Plan-form evolution of ancient meandering rivers reconstructed from longitudinal outcrop sections. Sedimentology 61 (4), 952–977. doi:10.1111/sed.12081
Gonzales, J. (2019). Amazon infrastructure puts 68% of indigenous lands/protected areas at risk: Report. Mongabay: News & inspiration from Nature´s frontline. Avaliable at: https://news.mongabay.com/2019/06/amazon-infrastructure-puts-68-of-indigenous-lands-protected-areas-at-risk-report/.
Goulding, M. (1980). The fishes and the forest: Explorations in amazonian natural history. USA: Univ of California Press.
Guerrero, L., Abad, J., Ortals, C., Naito, K., Valverde, H., Estrada, Y., et al. (2022). The birthplace of the Amazon River, a confluence of anabranching and meandering rivers. Water Resources Research.
Gutierrez, R. R., Abad, J. D., Choi, M., and Montoro, H. (2014). Characterization of confluences in free meandering rivers of the Amazon basin. Geomorphology 220, 1–14. doi:10.1016/j.geomorph.2014.05.011
Gutierrez, R. R., and Abad, J. D. (2014). On the analysis of the medium term planform dynamics of meandering rivers. Water Resour. Res. 50, 3714–3733. doi:10.1002/2012WR013358
Hess, L. L., Melack, J. M., Affonso, A. G., Barbosa, C. C. F., Gastil-Buhl, M., and Novo, E. M. L. M. (2015). LBA-ECO LC-07 wetland extent, vegetation, and inundation: Lowland Amazon Basin. Oak Ridge, TN: Oak Ridge National Laboratory, Distributed Active Archive Center for Biogeochemical Dynamics.
Hoorn, C., Wesselingh, F. P., ter Steege, H., Bermudez, M. A., Mora, A., Sevink, J., et al. (2010). Amazonia through time: Andean uplift, climate change, landscape evolution, and biodiversity. Science 330 (6006), 927–931. doi:10.1126/science.1194585
Isaac, V. J., Castello, L., Santos, P. R. B., and Ruffino, M. L. (2016). Seasonal and interannual dynamics of river-floodplain multispecies fisheries in relation to flood pulses in the Lower Amazon. Fish. Res. 183, 352–359. doi:10.1016/j.fishres.2016.06.017
Jarvis, A., Reuter, H. I., Nelson, A., and Guevara, E. (2008). Hole-filled SRTM for the globe version 4, available from the CGIARCSI SRTM 90 m database. Avaliable at: http://srtm.csi.cgiar.org.
Jensen, K., McDonald, K., Podest, E., Rodriguez-Alvarez, N., Horna, V., and Steiner, N. (2018). Assessing L-Band GNSS-reflectometry and imaging radar for detecting sub-canopy inundation dynamics in a tropical wetlands complex. Remote Sens. 10 (9), 1431–1529. doi:10.3390/rs10091431
Junk, W. J., Bayley, P. B., and Sparks, R. (1989). The flood pulse concept in river-floodplain systems. Can. J. Fish. Aquatic Sci. 106 (1), 110–127.
Kalliola, R., Puhakka, M., and Danjoy, W. (1993a). Amazonia Peruana: Vegetacion Humeda Tropical en el Llano Subandino. Finland: Proyecto Amazonia, University of Tunku.
Kalliola, R., Puhakka, M., Salo, J., Linna, A., and Räsänen, M. (1993b). Mineral nutrients in fluvial sediments from the Peruvian Amazon. Catena 20 (3), 333–349. doi:10.1016/0341-8162(93)90009-E
Kalliola, R., Salo, J., Puhakka, M., Rajasilta, M., Hame, T., Neller, R. J., et al. (1992). Upper Amazon channel migration: Implications for vegetation perturbance and succession using bitemporal landsat MSS images. Naturwissenschaften 79, 75–79. doi:10.1007/BF01131806
Kirkland, M., Eisenberg, C., Bicerra, A., Bodmer, R. E., Mayor, P., and Axmacher, J. C. (2020). Sustainable wildlife extraction and the impacts of socio-economic change among the Kukama-Kukamilla people of the Pacaya-Samiria National Reserve, Peru. Oryx 54 (2), 260–269. doi:10.1017/S0030605317001922
Lähteenoja, O., and Page, S. (2011). High diversity of tropical peatland ecosystem types in the Pastaza-Marañón basin, Peruvian Amazonia. J. Geophys. Res. Biogeosciences 116 (G2), G02025. doi:10.1029/2010JG001508
Lähteenoja, O., Reátegui, Y. R., Räsänen, M., Torres, D. D. C., Oinonen, M., and Page, S. (2012). The large Amazonian peatland carbon sink in the subsiding Pastaza-Marañón foreland basin, Peru. Glob. Change Biol. 18 (1), 164–178. doi:10.1111/j.1365-2486.2011.02504.x
Latrubesse, E. M., Arima, E. Y., Dunne, T., Park, E., Baker, V. R., d’Horta, F. M., et al. (2017). Damming the rivers of the Amazon basin. Nature 546 (7658), 363–369. doi:10.1038/nature22333
Latrubesse, E. M., d'Horta, F. M., Ribas, C. C., Wittmann, F., Zuanon, J., Park, E., et al. (2021). Vulnerability of the biota in riverine and seasonally flooded habitats to damming of Amazonian rivers. Aquatic Conservation Mar. Freshw. Ecosyst. 31 (5), 1136–1149. doi:10.1002/aqc.3424
Latrubesse, E. M. (2015). Large rivers, megafans and other quaternary avulsive fluvial systems: A potential “who’s who” in the geological record. Earth-Science Rev. 146, 1–30. doi:10.1016/j.earscirev.2015.03.004
Latrubesse, E. M., and Suizu, T. M. (2022). “The geomorphology of river wetlands,” in Encyclopedia of inland waters. Editors T. Mehner, and K. Tockner (Oxford: Elsevier), pp33–50.
Lin, Z., Shan, J., and Chen, L. (2017). Geomorphology processes of channel planform migration on meandering rivers. Acta Geol. Sin. 91, 134–135. doi:10.1111/1755-6724.13223
Marchetti, Z., Latrubesse, E., Pereira, M., and Ramonell, C. (2013). Vegetation and its relationship with geomorphologic units in the Parana River floodplain, Argentina. J. S. Am. Earth Sci. 46, 122–136. doi:10.1016/j.jsames.2013.03.010
Marchetti, Z. Y., Villalba, A. B., Ramonell, C., Brumnich, F., and Pereira, M. S. (2020). Biogeomorphic succession in a fluvial-lacustrine delta of the Middle Paraná River (Argentina): Feedbacks between vegetation and morphodynamics. Sci. Total Environ. 739, 139799. doi:10.1016/j.scitotenv.2020.139799
Marin-Diaz, J., Flores, G., and Abad, J. D. (2020). Similarities and differences between meandering and anabranching rivers. AGU Fall Meeting Abstracts. San Francisco, United States: American Geophysical Union.
McClain, M. E., and Naiman, R. J. (2008). Andean influences on the biogeochemistry and ecology of the Amazon River. BioScience 58 (4), 325–338. doi:10.1641/B580408
Mendoza, A., Abad, J. D., Frias, C. E., Ortals, C. J., Paredes, J., Montoro, H., et al. (2016). Planform dynamics of the Iquitos anabranching structure in the Peruvian upper Amazon River. Earth Surf. Process. Landforms 41, 961–970. doi:10.1002/esp.3911
Montero, J. C., and Latrubesse, E. M. (2013). The igapo of the negro River in central Amazonia: Linking late-successional inundation forest with fluvial geomorphology. J. S. Am. Earth Sci. 46, 137–149. doi:10.1016/j.jsames.2013.05.009
Motta, D., Abad, J. D., Langendoen, E. J., and Garcia, M. H. (2012a). A simplified 2D model for meander migration with physically-based bank evolution. Geomorphology 163–164, 10–25. doi:10.1016/j.geomorph.2011.06.036
Motta, D., Abad, J. D., Langendoen, E. J., and Garcia, M. H. (2012b). The effects of floodplain soil heterogeneity on meander planform shape. Water Resour. Res. 48 (9), 2011WR011601. doi:10.1029/2011WR011601
Naiman, R. J., Decamps, H., and Pollock, M. (1993). The role of riparian corridors in maintaining regional biodiversity. Ecol. Appl. 3 (2), 209–212. doi:10.2307/1941822
Science Panel for the Amazon Nobre, C., Encalada, A., Anderson, E., Roca Alcazar, F. H., Bustamante, M., Mena, C., et al. (2021). Executive summary of the Amazon assessment report 2021. New York, USA: United Nations Sustainable Development Solutions Network.
Organizacion de Tratado de Cooperacion Amazonica (OTCA) (2009). Perspectivas del Medio Ambiente en la Amazonía. Avaliable at: https://sinia.minam.gob.pe/documentos/geo-amazonia-perspectivas-medio-ambiente-amazonia.
Osmundson, D. B., Ryel, R. J., Lamarra, V. L., and Pitlick, J. (2002). Flow-sediment-biota relations: Implications for river regulation effects on native fish abundance. Ecol. Appl. 12 (6), 1719–1739. doi:10.1890/1051-0761(2002)012[1719:FSBRIF]2.0.CO;2
Park, E., and Latrubesse, E. M. (2017). The hydro-geomorphologic complexity of the lower Amazon River floodplain and hydrological connectivity assessed by remote sensing and field control. Remote Sens. Environ. 198, 321–332. doi:10.1016/j.rse.2017.06.021
Peixoto, J. M. A., Nelson, B. W., and Wittmann, F. (2009). Spatial and temporal dynamics of river channel migration and vegetation in central Amazonian white-water floodplains by remote-sensing techniques. Remote Sens. Environ. 113 (10), 2258–2266. doi:10.1016/j.rse.2009.06.015
Puhakka, M., Kalliola, R., Rajasilta, M., and Salo, J. (1992). River types, site evolution and successional vegetation patterns in Peruvian Amazonia. J. Biogeogr. 19 (6), 651–665. doi:10.2307/2845707
Räsänen, M., Neller, R., Salo, J., and Jungner, H. (1992). Recent and ancient fluvial deposition systems in the Amazonian foreland basin, Peru. Geol. Mag. 129 (3), 293–306. doi:10.1017/S0016756800019233
Reis, V., Hermoso, V., Hamilton, S. K., Bunn, S. E., Fluet-Chouinard, E., Venables, B., et al. (2019). Characterizing seasonal dynamics of Amazonian wetlands for conservation and decision making. Aquatic Conservation Mar. Freshw. Ecosyst. 29 (7), 1073–1082. doi:10.1002/aqc.3051
Rojas, T. V., Bartl, K., and Abad, J. D. (2021). Assessment of the potential responses of ecosystem services to anthropogenic threats in the Eten wetland, Peru. Ecosyst. Health Sustain. 7 (1), 1942224. doi:10.1080/20964129.2021.1942224
Ruben-Dominguez, L. D., Naito, K., Gutierrez, R. R., Szupiany, R., and Abad, J. D. (2021). Meander statistics toolbox (MStaT): A toolbox for geometry characterization of bends in large meandering channels. SoftwareX 14, 100674. doi:10.1016/j.softx.2021.100674
Salo, J., Kalliola, R., Häkkinen, I., Mäkinen, Y., Niemelä, P., Puhakka, M., et al. (1986). River dynamics and the diversity of Amazon lowland forest. Nature 322 (6076), 254–258. doi:10.1038/322254a0
Salonen, M., Toivonen, T., Cohalan, J. M., and Coomes, O. T. (2012). Critical distances: Comparing measures of spatial accessibility in the riverine landscapes of Peruvian Amazonia. Appl. Geogr. 32 (2), 501–513. doi:10.1016/j.apgeog.2011.06.017
Shan, J. F., Lin, Z. P., Chen, L., Zhang, B., Fang, S. X., Yan, X., et al. (2018). Reconstruction of meandering paleo-channels using dense well data, Daqing Oil Field, Songliao Basin, China. Petroleum Sci. 15 (4), 722–743. doi:10.1007/s12182-018-0270-x
Sierra, Y. (2019). Jorge Abad: “Me preocupa que la Hidrovía amazónica se haga mal”. Mongabay: News & inspiration from Nature´s frontline. Avaliable at: https://es.mongabay.com/2019/05/peru-hidrovia-amazonica-jorge-abad/.
Sierra, Y. (2020). More than 470 oil spills in the Peruvian Amazon since 2000: Report. Mongabay: News & inspiration from Nature´s frontline. Avaliable at: https://news.mongabay.com/2020/10/more-than-470-oil-spills-in-the-peruvian-amazon-since-2000-report/.
Stevaux, J. C., Corradini, F. A., and Aquino, S. (2013). Connectivity processes and riparian vegetation of the upper Paraná River, Brazil. J. S. Am. Earth Sci. 46, 113–121. doi:10.1016/j.jsames.2011.12.007
Valverde, H., Estrada, Y., Naito, K., Guerrero, L., Canas, C., and Abad, J. D. (2019). Geomorphological characteristics of the Huallaga River. Peru: AGU Fall Meeting.
Vermeulen, B., Hoitink, A. J. F., Zolezzi, G., Abad, J. D., and Aalto, R. (2016). Multiscale structure of meanders. Geophys. Res. Lett. 43 (7), 3288–3297. doi:10.1002/2016GL068238
Virgilio, L. R., Silva, A. L. C., Saldanha, R. F., Suçuarana, M. da S., Fernandes, E. C., and Vieira, L. J. S. (2020). Fish fauna in oxbow lakes of the middle purus River in the neotropical region of the Amazon rainforest. Braz. J. Dev. 6 (8), 55545–55564. doi:10.34117/bjdv6n8-108
Volckhausen, T. (2020). As habitat degradation threatens Amazon species, one region offers hope. Mongabay: News & Inspiration from Nature´s frontline. Avaliable at: https://news.mongabay.com/2020/05/habitat-degradation-threatens-amazon-species-one-region-offers-hope-studies/.
Wildlife Conservation Society (WCS) (2020). Pesquerías en Loreto, amenazas emergentes y presiones prevalentes. Avaliable at: https://global.wcs.org.
Wildlife Conservation Society (WCS) (2022). Atlas de humedales y pesquerías en Loreto. Avaliable at: https://arcg.is/1OaanT.
Wilson, M. D., Bates, P., Alsdorf, D., Forsberg, B., Horritt, M., Melack, J., et al. (2007). Modeling large-scale inundation of Amazonian seasonally flooded wetlands. Geophys. Res. Lett. 34 (15), 4–9. doi:10.1029/2007GL030156
Wittman, F., Junk, W. J., and Piedade, M. T. (2006). The várzea forests in Amazonia: Flooding and the highly dynamic geomorphology interact with natural forest succession. For. Ecol. Manag. 196 (2-3), 199–212. doi:10.1016/j.foreco.2004.02.060
Keywords: wetlands, paleo-channels, Amazon basin, Pacaya-Samiria, meandering rivers, anabranching rivers, hydrologic connectivity, underfit-scavenger rivers
Citation: Rojas TV, Abad JD, Roque WR, Latrubesse EM and Shan J (2023) Free and underfit-scavenger river dynamics dominate the large Amazonian Pacaya-Samiria wetland structure. Front. Environ. Sci. 11:1082619. doi: 10.3389/fenvs.2023.1082619
Received: 28 October 2022; Accepted: 02 January 2023;
Published: 16 January 2023.
Edited by:
Omer Yetemen, Istanbul Technical University, TürkiyeReviewed by:
Richard George Pearson, James Cook University, AustraliaSteven Sandi, The University of Newcastle, Australia
Copyright © 2023 Rojas, Abad, Roque, Latrubesse and Shan. This is an open-access article distributed under the terms of the Creative Commons Attribution License (CC BY). The use, distribution or reproduction in other forums is permitted, provided the original author(s) and the copyright owner(s) are credited and that the original publication in this journal is cited, in accordance with accepted academic practice. No use, distribution or reproduction is permitted which does not comply with these terms.
*Correspondence: Jorge D. Abad, amFiYWRAcmVkeWFrdS5jb20=