- 1Kenya Marine and Fisheries Research Institute, Kisumu, Kenya
- 2Kenya Marine and Fisheries Research Institute, Kisii, Kenya
- 3Kenya Fisheries Service, Nairobi, Kenya
- 4Kenya Marine and Fisheries Research Institute, Mombasa, Kenya
- 5UK Centre for Ecology & Hydrology, Scotland, United Kingdom
The extent of flooding in vulnerable inland and lacustrine systems can demonstrate the coverage and the magnitude of such phenomenon for policy enhancement. This study examined the extent of flooding due to rising water levels in selected Afrotropical lakes to guide interventions that would sustain the livelihoods of communities affected. The years that were most prone to flooding (2010 and 2020) were used as a baseline in the extraction of changes in spatial extent and area of lacustrine shoreline, and rainfall and satellite altimetry data, using geospatial and remote sensing technologies. The extent of flooding was strongly but insignificantly related (R2 = 0.63; p = 0.07) to the sizes of the studied lakes and the amount of rainfall. Lakes with the smallest surface areas such as Baringo and Naivasha showed the greatest increase in flooding of 52.63% and 42.62%, respectively. Larger lakes such as Lakes Victoria (1.05%), Turkana (3.77%), and Tanganyika (0.07%) had the lowest increases in areal extent. Furthermore, the topography of the lakes studied further determined the residence time and the extent of flooding, such that lakes such as Edward (−0.09%) and Rukwa (−3.25%) receded during the period when other lakes were flooding. The information and data presented here provides the most up-to-date quantification of flooding to support adaptation strategies for inland lake systems and guide policy implementation.
1 Introduction
Water resources and wetland ecosystems, including rivers, lakes, swamps, floodplains, and man-made reservoirs, have been endowed to the world by various geological formations and climatic conditions (Delgado et al., 2002). Conversion to intensive irrigation agriculture, population growth, industrial and agricultural pollution, water diversion for drainage, and dam construction have all increased these geographical systems’ vulnerability to the effects of climate change, despite the benefits they provide (Junk, 2002; Benson, 2004). Tropical systems, including wetlands, are among the natural ecosystems with the highest rate of conversion, particularly in Africa (Nilsson et al., 2005). This is due, at least in part, to a lack of data and understanding of their various application values. Due to increased regulation, fragmentation, and other climate change-related scenarios, tropical geological formations are also losing value (Junk et al., 1989; Barbier, 1994). Inland lakes and their associated wetlands play important roles in aquatic biodiversity and ecosystem function, climate modulation, water and food provision for humans and other living organisms, flood regulation, water quality regulation and buffering, and groundwater recharge (Brasseur and Gallardo, 2018). The majority of these lakes are also UNESCO World Heritage Sites, Ramsar sites (Wetlands of International Importance), and Important Bird Areas (IBAs) due to their exceptional universal values (Cooke et al., 2016).
Non-etheless, the lack of data on the state of inland lakes limits their inclusion in major policy processes such as the SDGs (SDGs, Cooke et al., 2016). As a result, these systems do not benefit from associated management objectives, resulting in increased and persistent threats (Youn et al., 2014). To mitigate such unprecedented changes in geological forms, the Millennium Ecosystems Assessment (MEA) called for more research on measuring, modeling, and mapping the ecosystem services that they provide, as well as assessing changes in their delivery in relation to human wellbeing (Carpenter et al., 2006). Ecosystem services research has significantly developed overtime with applicability limitations, hence spatially explicit empirical research is urgently needed to address the insufficiency of information on patterns of ecosystem service provision (Nicholson et al., 2009; McDonough et al., 2017).
Inland lakes in the tropics are an obvious candidate for such research because they are an important component of marginalized communities that are increasingly threatened by natural calamities, land cover changes, human activities and climate change (Welcomme, 2008). The hydro-climatic anomalies resulting from population growth, environmental stressor and unsustainable anthropogenic activities greatly contribute as the causal factors of such threats (Papa et al., 2023). These lacustrine systems provide ecosystem services such as fisheries, transport, employment, cage culture development, freshwater provision, water purification and regulation, groundwater recharge and tourism, in addition to their cultural and spiritual values (Okely et al., 2010; Aura et al., 2020). As part of a collection of related flood-dependent services in complex socio-ecological landscapes, the study of these services captures the kinds of linkages and processes that should be a research priority, from biodiversity through the ecosystem services it produces and on to their contribution to human wellbeing in relation to existing risks and hazards (Hamerlynck et al., 2011).
Knowledge about flooding risks and hazards in relation to inland lakes play a critical role in driving both mitigation and adaptation strategies (Street and Grove, 1976). Water level changes of continental lakes are related to global and regional climate change, land cover changes, and human activities (Mercier et al., 2002). For example, information and data on induced flooding will provide the most recent knowledge from lake receding and retraction dymanics to support adaptation strategies for inland lake fisheries, water, health and other sectors (Trauth et al., 2003; Climate Services Partnership, 2015). The finding of such lacustrine research aims to serve user requirements that later help in developing our understanding of lake environments and related aquatic systems (Vaughan and Dessai, 2014). Apart from assisting in preparation for managing the effects of climate change such as lake level rises and flooding, one of the primary goals of quantifying the extent of flooding will be to provide current climatic knowledge and information that can be utilized to lessen the risk of climate-related disasters and increase welfare (WMO, 2019).
The African continent forms the major block of developing countries where, to date, weather forecast and information on flooding phenomena have not been available, making it difficult to deploy related interventions and services (WMO, 2012). Other potential barriers on the African continent are problems related to the lack of good quality information provided by the scientific community in relation to the needs of various users of aquatic environments (Brasseur and Gallardo, 2018; Papa et al., 2023).
Despite such barriers in the developing countries such as those in Africa, and in especially Eastern Africa, quantification of the extent of flooding and lake level rise could offer various benefits at community and individual levels, and to the environment. In this case, information on the extent of flooding might help vulnerable populations decide on specific crops, as well as the time of planting and fertilizer applications, to limit the impact of climate risk on agriculture (Vaughan and Dessai, 2014). Thus, using prior information acquired from climate services, disaster risk can be mitigated.
Issuance of warnings about the extent of flooding and the magnitude of threats can aid in adequate preparedness efforts, eventually saving lives (Dutton, 2002). Furthermore, data collection on extreme flooding events can aid in improving security and livelihoods (WMO, 2012). Moreover, the risks and extent of flooding damage can be used to raise awareness of the patterns and burdens of many water-related diseases, resulting in lower infection rates (Shafer, 2008). Since tourism activities have been known to occur at the shores of many lakes, knowing the extent of hazards due to flooding could support sustainable development of tourism and adaptation measures. This is due in part to the fact that historical flooding data can be useful for planning tourism infrastructure, locating new resorts, and designing architecture and landscapes (Scott et al., 2011). In general, the determination of the level of flooding in aquatic systems can increase resilience (Leal Filho, 2019).
Floods occurred in the African Great Lakes region in 2020, manifested by rising lake levels and bank overflows; this affected natural resources and livelihoods (Obando et al., 2016; BBC News, 2020). The effects of lake level changes have been studied widely (e.g., Gasse, 2000; Hulme et al., 2010; Hamerlynck et al., 2011; Kolding and van Zwieten, 2012; Jones and Jordan, 2013; Aura et al., 2020). These research found that water level changes influenced lake and fishery productivity, including the time and breadth of breeding, food availability, catchability, the extension of shallow productive inshore zones, and the placement of cage culture facilities.
Previous studies have evaluated the changes in lake water levels in the African Great Lakes region. Some of the studies include and not limited to extreme rainfall events and lake level changes (Odingo, 1962; Mörth, 1967; Birkett et al., 1999); inter-annual lake fluctuations (Mercier et al., 2002); and studies on inland surface waters from space (Guzinski et al., 2014; Papa et al., 2023), among others. However, there is a scarcity of information on the quantification of the extent of flooding on Afrotropical lakes using the years that are most prone to flooding as a baseline information on lake dynamics to guide intervention measures. In this regard, the water level in the African inland lakes rose unexpectedly in either 2010 and 2020. All of the lakes exceeded their boundaries from 2018 to 2021, with disastrous consequences such as shoreline infrastructure (ports and fish landing sites), homes, and farmland; but with lack of information-based spatial planning on the extent of flooding. The negative consequences were more pronounced in the developing world, particularly in Africa (BBC News, 2020). This highlighted the vulnerability of communities near these systems to the extent of flooding.
The lake level fluctuations caused by flooding are an important palaeohydrological study in response to climatically driven changes in the hydrological balance of a lake-catchment system on a spatiotemporal scale. The space and temporal dimensions will be critical in differentiating patterns of lake-level extent at the continental scale as a starting point for understanding the causes and quantifications of possible damage (Street and Grove, 1979; DeVogel et al., 2004) to support management interventions. This study was undertaken, therefore, to highlight the potential use of that information using East African lakes as a case study in the assessment of the vulnerability of such systems to the magnitude of ecological and infrastructural vulnerability. The aim of this study was to investigate the extent of flooding caused by rising water levels in the selected East African lakes in order to guide interventions to sustain the livelihoods of impacted communities.
2 Methods
2.1 Study area
The study was undertaken on the Kenyan (Lakes Victoria, Turkana, Baringo and Naivasha), Ugandan (Lakes Victoria, Edward, Albert, and Kyoga), Tanzanian (Lakes Victoria, Tanganyika, Rukwa and Eyasi) and Rwandan (Lake Kivu) major lakes (Figure 1). The selected lakes saw rises in water levels between 2010 and 2020, resulting in extensive flooding that impacted lakeside communities, particularly fishers, despite the fact that the population along the lakes’ coastlines relied nearly entirely on fishing and fish mongering (Vranken et al., 2022).
Lake Victoria is one of the world’s most distinctive bodies of water. With a water surface size of 169,858 km2, it is the world’s second biggest freshwater lake, with a drainage area of more than 193,000 km2 that spans five Eastern African countries (Twesigye et al., 2011). The lake’s fresh water drains into the Nile River and is replenished by major rivers including as the Mara, Nzoia, Yala, Sondu-Miriu, and Kuja (Masese et al., 2013). The lake is shared by Kenya, Uganda, and Tanzania. It serves around 30 million people and has a catchment population density of 170 people per square kilometer. The majority of the population relies on agriculture, livestock, and fishing for a living (Odada et al., 2004).
Lake Turkana is the largest inland lake (6,405 km2) in Kenya. The lake is located in a closed basin at 365 m above sea level, with mean and maximum depths of 31 and 115 m, respectively (Aura et al., 2020). It receives more than 90% of its yearly water input from the Omo River, which flows into the lake from Ethiopia. Because the lake is endorheic and has no surface outflow, its water budget is a balance of river intake and evaporation. As a result, the lake has become increasingly saline. Water level changes have a significant impact on the lake’s average yearly fish yield. Its fisheries are anticipated to generate 7,000 tonnes of fish per year, valued at USD 1.6 million, and to employ approximately 7,000 fishers and 6,500 fish traders and transporters (Long’ora et al., 2015).
Lake Baringo is a Ramsar site, and a Rift valley lake with a surface area of 130 km2 and a mean depth of 5.6 m. It varies greatly due to climatic changes. Except for suspected subsurface seepage, it has three river inlets and no apparent outflow (Aloo, 2002). The lake produces 263 tonnes of fish per year, with an ex-vessel worth of USD 250,000. (Aura et al., 2020).
Lake Naivasha’s surface area ranges between 120 and 150 km2 during the dry and wet seasons, respectively (Hickley et al., 2008), and its mean depth ranges between 4 m and 6 m. The lake is fed by two rivers, and its fisheries are influenced by changes in lake water level. The lake fishery produces 1,063 tons of fish per year with a farm-gate value of USD 1.40 million (Kitaka et al., 2002; Aura et al., 2020).
Lake Edward is one of Africa’s most productive inland water systems (Bassa et al., 2014), shared between Uganda and the Democratic Republic of the Congo (DRC) Decru et al. (2020) provides a full description of this system’s features. The lake supports fisheries with annual catches of 21,000 tonnes, as well as 22,000 fishers in Uganda and the Democratic Republic of the Congo (Lubala et al., 2018).
With only Lakes Victoria and Kyoga producing more fish, Lake Albert is an important contributor to Uganda’s economy. But like many other large inland water basins in East Africa, Lake Albert has been overfished (Eccles, 1976). Given its high salinity levels, various hydrological modelling scenarios, including the construction of a channel from it to the Coorong (Lake Albert Channel) have been proposed (Souter and Walters, 2006). Furthermore, the lake has experienced Nile perch mass mortalities in the past that have been attributed to the shock effect of an earthquake that exposed these intolerant fish to low oxygen levels (Eccles, 1976).
Lake Kyoga extends north of Lake Victoria from the Victoria Nile into a vast network of shallow open-water regions surrounded by papyrus swamps. The lake’s open water changes with the seasons and years, but its average extent is estimated to be around 2,700 km2, plus an additional 2,000 km2 of related marshes and small lakes (FAO, 1999). With a depth of about 5.7 m, this lake is second to Lake Victoria in terms of fish production and accounts for 35% of Uganda’s fish landings (Ogutu-Ohwayo, 1998).
Lake Tanganyika is a large Rift Valley Lake that is approximately 670 km long, 50 km broad, and 570 m deep. It features two primary basins, one in the north and one in the south, with maximum depths of roughly 1,320 and 1,470 m, respectively, divided by a 600 m sill (Naithani et al., 2002). The lake’s thermal stratification is highly defined, and its depth varies periodically above a permanent hypolimnion. Lake Tanganyika has long been recognized for its southern upwelling in direct response to its prevailing southeast winds during the dry season. Lake Tanganyika is the oldest of East Africa’s Great Lakes, with over 250 species of cichlid fishes. The majority are endemic species that have evolved rapidly and exhibit great variety in their shape, behavior, and ecology as a result of adaptive radiation into new habitats (Cocquyt and Vyverman, 2005). Cichlids are found from the lake’s shallow shores to its deep parts, with the assemblage of the cichlid community showing greater density and diversity in the shallow rocky regions where various cichlid species with similar diet niches coexist (Descy et al., 2005).
Lake Rukwa is a Rift Valley Lake in south-west Tanzania with an 88,000 km2 basin (Nicholson, 1999). The lake is bounded in the southeast by the Mbeya Range, in the west by the slopes of the Ufipa escarpment, and in the north-east by Mount Sange’s rocky cliffs and rolling hills, which rise 1,707 m above sea level (Baker and Baker, 2002). The presence of pools, as well as two separate north (big) and south (small) lake basins, indicate changes in the shape of the lake, which has recently become a single lake. The lake’s length is claimed to range from 135 m to 180 km, with an average width of 32 km (Lakepedia, 2016). It has an average depth of 3–5 m and a maximum depth of roughly 15 m in the south basin (World Lakes, 2016).
The seasonal and alkaline Lake Eyasi is the most southern of the big lakes in the Gregory Rift Valley; it is situated under a massive escarpment capped by the volcanic rocks of the Ngorongoro Volcanic Complex. The lake is quite saline (Bushozi et al., 2017). The lake basin is bordered by semi-perennial springs and river tributaries, although the Sibiti River at the southern end is the lake’s primary tributary. Notably, the lake may become entirely dry during the dry season, while its depth during the rainy season seldom reaches 1 m (Prendergast et al., 2007).
2.2 Determination of the extent of flooding
Several explanations have been proposed to explain the hydrological imbalances observed in the lakes that have drowned structures and infrastructure due to the displacement of humans, animals, and aquatic organisms (Aura et al., 2020). The hypothesis, in this case, is that higher wave height and increased rainfall are indicative of a larger lake extent during flooding. Figure 2 depicts a schematic illustration of the parameters used to determine the spatial extent of flooding and associated variables for the selected lakes. The schematic diagram illustrates the processes of sourcing the satellite data and information, processing the data, displaying the data and making comparisons to inform management decisions.
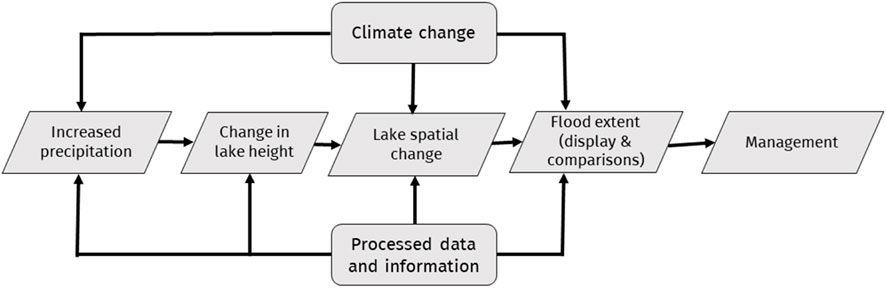
FIGURE 2. Systematic framework employed in the current study for determining flood extent for management and mitigation measures.
The geographical breadth and area of lacustrine shoreline variations were extracted using GIS and remote sensing techniques. The years when the studied lakes were most prone to increases in rainfall and flooding, and in which satellite altimetry data were available, were 2010 and 2020. These were used for comparisons. Multi-temporal satellite imagery from two different time windows within 2010 and 2020 was downloaded through the United States Geological Surveys (USGS) Earth Explorer website (EarthExplorer, 2022). The open-source satellite imagery used was from the USGS-NASA Landsat OLI sensor missions (Landsat Science, 2022). Use of EarthExplorer allowed the narrowing of the search to the required imagery by area, date range, and cloud cover (Elkafrawy et al., 2021).
All of the tiling grids containing the multispectral satellite data were downloaded according to the specific flight path and row that covered the studied lakes. Image classification was undertaken using the software QGIS 3.0 through the semi-automatic classification plugin (Tempa and Aryal, 2022). Utilising band set 1-7 of the downloaded Landsat imagery, the images were re-projected to the EPSG: 4,326, WGS 84 coordinate system, which is the global standard for latitude and longitude positions (Kelly, 2022). The re-projected images were merged and then converted to reflectance values and an atmospheric correction was applied (Kalluri et al., 2010). The new set of reflectance bands was loaded into QGIS, and a band combination of 5-6-4 (Near Infrared-Shortwave infrared 1-red) was chosen to create a virtual band-set that enabled water bodies to be distinguished from land and corresponded to the wavelength (µm, 0.63–1.66) (Jong et al., 2006).
Using existing literature for the exact area where the specific lakes were and a 1.0 km extent, a polygon was created around all of the lakes (Wu et al., 2019). Two polygons were drawn for each lake to represent the two different windows for 2010 and 2020. A field calculator function was used to obtain the difference in surface area (Zaki et al., 2021). The objective of this study was to analyze the fluctuation in lake spatial extent (size) owing to floods and to determine the link between lake spatial extent, lake level, and rainfall across the lake catchment region.
Meteorological parameters taken from NASA’s GMAO MERRA-2 assimilation model and GEOS 5.12.4 FP-IT were utilized to determine the rainfall amounts for each lake catchment. NASA’s Goddard Earth Observing System (GEOS) Data Assimilation System (MERRA-2) is an updated version of MERRA (Bosilovich et al., 2017). The GEOS 5.12.4 data are delivered daily and appended to the end of the MERRA-2 daily time series to create low-latency products that are typically ready for usage within two days of real-time (Hobeichi et al., 2020). Typically, the MERRA-2 data included in the resultant daily time series are updated every few months. Annual mean values representative of the rainfall over the lake catchment area were calculated for 2010 and 2020 and compared with the lake spatial extent and lake level.
The Global Reservoirs and Lake Monitor (G-REALM, https://ipad.fas.usda.gov/cropexplorer/global reservoir/) provided lake level (m) multi-year mean data. These were relative lake level changes estimated from TOPEX/POSEIDON (T/P), Jason-1 and Jason-2/OSTM satellite altimetry in comparison to a 9-year mean lake level determined from T/P altimeter measurements. The Jason-2 observations in near real time are taken from the currently available Interim GDR (IGDR). Wave heights for Lakes Baringo, Edward, Albert, and Eyasi were not provided by the website and hence their satellite altimeter readings were not used in the study. The study employed R version 3.5.0 (R Core team, 2020) for statistical analysis, with a significance threshold of p < 0.05.
3 Results and Discussion
The shores of inland lakes are centres of blue economy. They play a pivotal role in fishing, water supply, irrigation and agriculture, infrastructural development, public health, tourism, transport, and energy (Aura et al., 2022). However, it has been noted for such systems to experience several geophysical processes that may affect the lake level fluctuations. The factors considered negligible on affecting lake level rises whose amplitudes appear to be generally inferior to ten of centimetres, include temperature or composition alterations; surface pressure changes for such closed basins; wind-driven events; and tides (Birkett et al., 1999). Despite the fact that there were no known tectonic processes in the region inform of earthquakes to modify the morphology of such lakes (Mason et al., 1994), the water extent was specifically based on wave heights and precipitation levels as the causative factors to flooding scenarios (Aura et al., 2020).
However, it is important to note that the lake level fluctuations of various lakes in not straightforward since the level height is the critical measurement of such systems rather than their volume, hence the need to use the surface area in relation to the lake basin morphological characteristics (Mercier et al., 2002). Comparing precipitation and aerial extent for such lakes in the inter-tropical region with varying climatical characteristics in the study herein, further provided some impact of water levels at varying eco-regions. For example, lakes close to the equator (Victoria and Kyoga), Northern hemisphere and desert lakes (e.g., Turkana), and alteration between wet and dry seasons and southern-most border (e.g., Rukwa) experiences varying beginnings of lake level rise, rainfall intensity and the main rain season and peaks (Mercier et al., 2002) as factors related to the extent of vulnerability to damage effects. If the years 2010 and 2020 are foreshadowing of what is to come in terms of rising sea levels, there could be a clear baseline map of vulnerability for such systems and the need to implement mitigation and adaptation strategies for vulnerable inhabitants and ecosystems.
Figure 3 and Table 1 show the change in surface area of the studied lakes due to flooding in 2010 and 2020 as the years considered to have experienced increased flooding levels in inland lake systems (Aura et al., 2020). Despite having one of the smallest surface areas, Lakes Baringo (52.63%) and Naivasha (42.62%) showed the greatest increase in areal extent due to flooding. This agrees with Herrnegger et al. (2021) findings that Lake Baringo is susceptible to flooding from increasing water levels in the lake considering it is small in area and lies in a topographically flat basin. In 2010, the small endorheic Lakes Baringo and Naivasha had significant increase (28.8 km2 and 26.64 km2, respectively) in area from the rainfall experienced throughout that year (Obando et al., 2016), with disastrous effects causing displacement of human and wildlife settlement, destruction of properties, economic disruption, lake ecosystem and biodiversity.
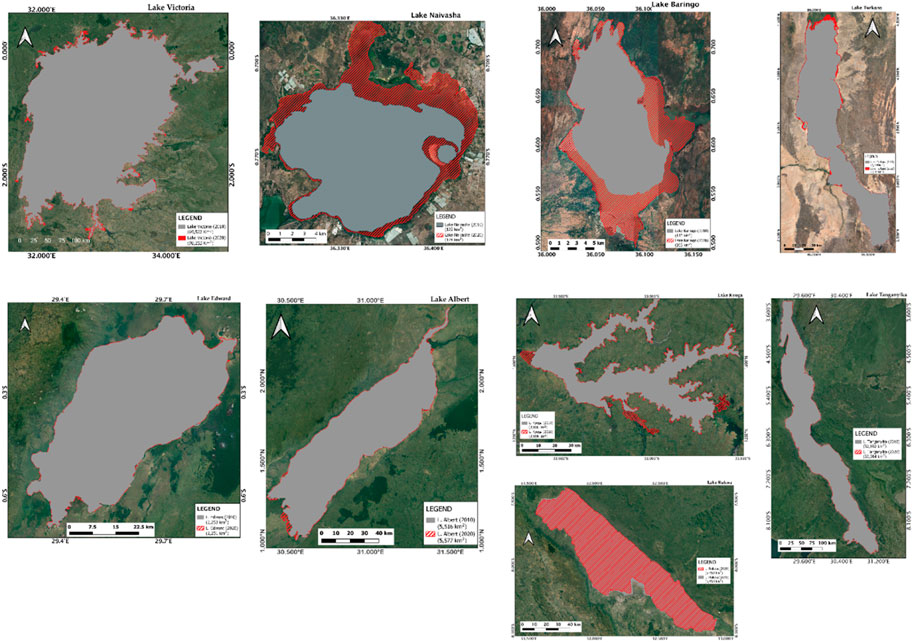
FIGURE 3. Changes in the areal extents of the selected African lakes due to flooding. The grey region indicates the lake’s shoreline in 2010 and the red shaded region is the shoreline in 2020.
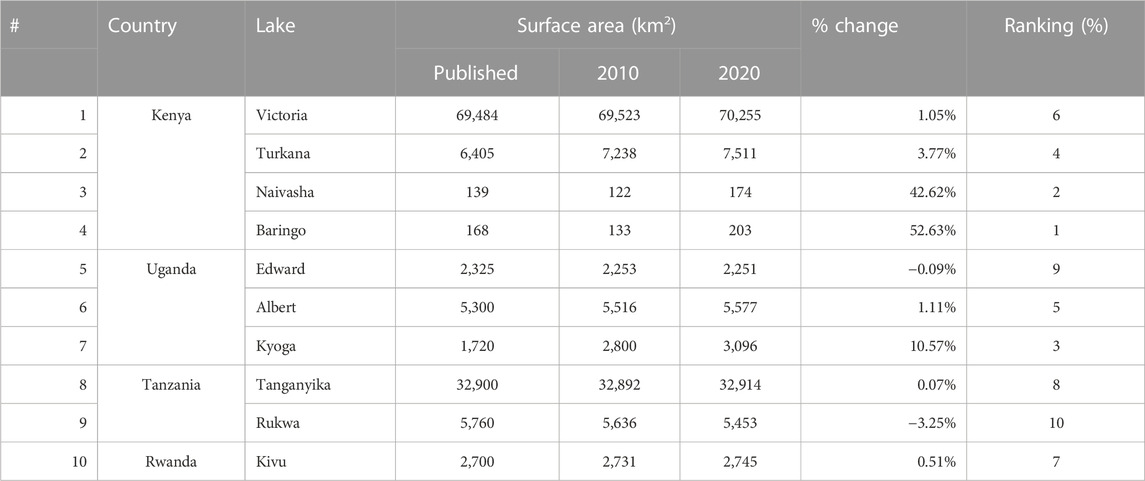
TABLE 1. Calculated areal extent (km2) and percentage change in surface area of the selected major African lakes due to flooding in the years 2010 and 2020.
The small lakes are found to be vulnerable to climatic anomalies (Herrnegger et al., 2021). Larger lakes, such as Victoria (1.05%), Turkana (3.77%), and Tanganyika (0.07%), recorded the lowest increases in areal extent. Because of their sizes, the larger lakes seemed to take longer to show considerable change in areal extent as compared to the small lakes that have immediate reactions to increased water levels from increased rainfall. This spatial dimension is especially useful because it allows us to detect patterns of lake level change at the continental scale and helps to understand the possible causes (Steffen et al., 2003). In this case, the extent of flooding was strongly but insignificantly related (R2 = 0.63; p = 0.07) to the sizes of the studied lakes and the amount of rainfall (Figures 4, 5). Therefore, the present study shows there is an influence of rainfall characteristics on the change of the lake’s shoreline. Generally, high rainfall areas seemed to have low percent change while low rainfall areas have high percentage change. This could be because of the size and the topographical nature of the lake. The flooding phenomenon was mainly attributed to increased precipitation levels in the years 2010 and 2020 compared to other years. If so, this would be a clear indication that lake level induced changes are likely to cause serious challenges to low-lying areas around the shoreline of lakes across the world. In this case, Lake Victoria was an exception perhaps due to other related factors such as land cover changes, human activities, and fluctuating climatic conditions around the lake. The is considered monomictic, meaning that it typically mixes from top to bottom once a year, usually between June and August climate change has made the timing of this mixing, precipitation patterns and lake level rise unpredictable, and it is happening more frequently and with greater intensity. The timing and location of these events are influenced by wind patterns and the lake’s shape (Nyamweya et al., 2020).
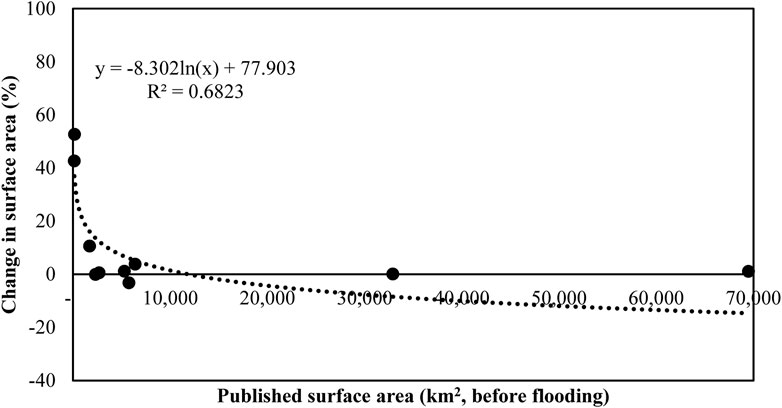
FIGURE 4. Relationship between change in areal extent (km2) due to flooding and surface area, and the percentage change in surface area for the years 2010 and 2020.
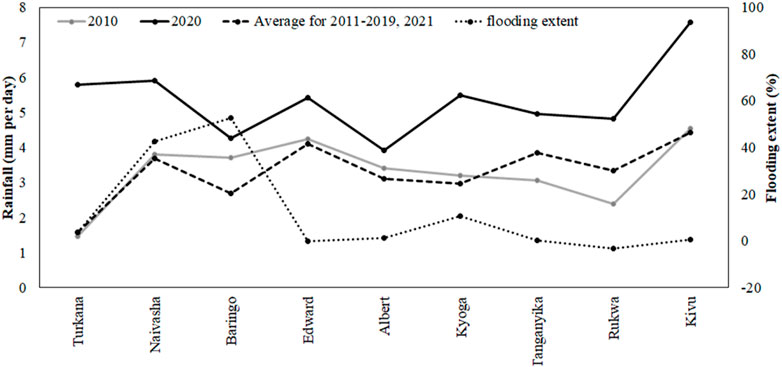
FIGURE 5. Amount of rainfall recorded in the years 2010 and 2020 compared to annual averages for other years and the aerial flooding extent of the studied lakes. Lake Victoria was exempted in the graph due to high precipitation differences of 1993 and 3,080 mm for 2010 and 2020, respectively.
Shoreline communities and their resources and infrastructures are the first to feel the effects of a shifting coastal climate and increasing sea levels (Tol et al., 2008). Individuals and organizations may take advantage of opportunities and avoid hazards by comprehending, preparing for, and adjusting to fluctuating precipitation patterns, which alter the lake’s surface area based on its size. To meet these needs, climate change communication and education play a crucial role in the dissemination of information, particularly in the translation of technical information to improve public comprehension and the incorporation of indigenous knowledge into climate change adaptation for policymakers (Khan et al., 2018).
The causative factors for the receding of Lakes Edward (−0.09%) and Rukwa (−3.25%) such as land cover change, human activities and climate changes, when other lakes were flooding could be further studied using citizen science and mapping of such factors. Furthermore, it is likely that wave heights are smaller and less prolonged in large-sized lakes than smaller lakes (Figure 6). Such easily accessible and timely socio-scientific information could help coastal communities not only to limit the economic and social advantage or damage of receding waters caused by climate-related risks, land cover changes and human activities, but also take advantage of opportunities provided by favourable conditions (Medri et al., 2012). Future studies could investigate the role of the increased hydrological connectivity provided by seasonal flooding for receding inland systems such as Lakes Edward and Rukwa in promoting fishery productivity and yield. This is because seasonal flooding has been known to provide accessibility to flood plain lagoons for some fish species such as Prochilodus lineatus (Agostinho and Zalewski, 1995).
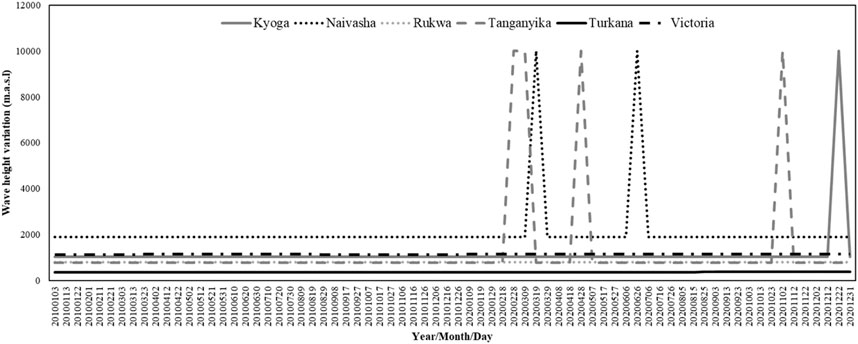
FIGURE 6. Smoothened wave height variations in selected lakes based on the availability of altimetry data.
Understanding water-level fluctuations based on varying wave heights due to increased flooding caused by increased precipitation and other lake characteristics such as surface area and depth of inland systems could play a crucial role in lacustrine dyamics. This is due to the fact that the region affected by floods influences the availability of space and habitat, the size of the photic zone, water chemistry, stratification, and mixing regimes. Falling lake levels in shallow lakes may prevent thermal stratification and raise salt concentrations, hence forcing a shift in lake pH (Harrison and Digerfeldt, 1993). Wave heights may also result into turbulence, leading to the erosion of exposed marginal areas to create varying levels of damage (DeVogel et al., 2004; Aura et al., 2022).
Information and statistics on lacustrine dynamics play a crucial role in directing mitigation and adaptation planning procedures. On the other hand, the challenges presented by flooding changes and the improvement of climate research have driven public and commercial organizations and specialized institutions to prioritize the creation and implementation of Global Climate Services (Jancloes et al., 2014). In this context, the extent of flooding will add to the climate services information in the definition of the creation, interpretation, dissemination, and use of climate knowledge and data for decision making and future planning. It provides the most current climate science research to enable adaptation plans for fisheries, agriculture, water, and other sectors (Climate Services Partnership, 2015).
The extent of flooding and its magnitude will emphasis on addressing customer needs, which contributes to the subsequent comprehension of climate services. One of the primary goals of climate services, in addition to assisting in preparing to manage the consequences of climate change, is to give up-to-date climate-related knowledge and information that may be utilized to minimize climate-related catastrophe risks and increase welfare (Vaughan and Dessai, 2014). By compiling baseline information and data on the areal extent of flooding as a case study, this research creates a benchmark for integrating climate services and climate research into sensitivity and quantification assessments, and into future planning. Therefore, the current study acts as a decision-support tool to guide possible mitigation measures not only for the studied lakes, but also highlights the need to undertake further studies on the continental inland systems to provide climate science information on the magnitude of changes in the areal extent of flooding for vulnerable communities, infrastructure and the critical ecosystem in such localities.
4 Conclusion and recommendations
The present study provides preliminary results on the quantification of rising water levels in Africa. The study showed that the areal extent of flooding strongly but insignificantly related to the sizes of the studied lakes and the amount of rainfall. The smaller lakes studied, such as Baringo and Naivasha, showed the greatest increase in areal extent due to flooding; more than 40% of their published surface areas. The larger lakes studied, such as Victoria, Turkana, and Tanganyika, had lower than 4% increases in area extent compared to their published surface area. Some of the smaller lakes, such as Edward and Rukwa, receded during the period when other lakes were flooding due to their topography and their water residence time. This study provides useful information and data that could support the development of a decision-support tool to determine possible changes in the areal extent of inland lakes that may undergo flooding, and the related risks and damages that it may cause, to inform adaptation strategies and guide policy and its implementation. However, future studies could focus on the extent of other causative factors such as land-cover changes, human activities and climate change simulations.
Data availability statement
The original contributions presented in the study are included in the article/supplementary material, further inquiries can be directed to the corresponding author.
Author contributions
CA—Data analysis and draft development; CN—Data sourcing and proof-reading; SM—Introduction development; ZO—GIS and mapping; WO—GIS and map development; MO—Introduction and methodology development; LM—Results and discussion development and proof-reading; JN—Proof-reading.
Funding
This work was supported by the Natural Environment Research Council as part of the NC-International programme (NE/X006247/1) delivering National Capability.
Acknowledgments
We acknowledge Kenya Marine and Fisheries Research Institute (KMFRI) in collaboration with United Kingdom Centre of Ecology & Hydrology for implementing the project.
Conflict of interest
The authors declare that the research was conducted in the absence of any commercial or financial relationships that could be construed as a potential conflict of interest.
Publisher’s note
All claims expressed in this article are solely those of the authors and do not necessarily represent those of their affiliated organizations, or those of the publisher, the editors and the reviewers. Any product that may be evaluated in this article, or claim that may be made by its manufacturer, is not guaranteed or endorsed by the publisher.
References
Agostinho, A. A., and Zalewski, M. (1995). The dependence of fish community structure and dynamics on floodplain and riparian ecotone zone in Parana River, Brazil. Hydrobiologia 303, 141–148. doi:10.1007/bf00034051
Aloo, P. A. (2002). “Effects of climate and human activities on the ecosystem of Lake Baringo,” in The East african great lakes: Limnology, paleolimnology and biodiversity. Advances in global research 12. Editors E. O. Odada, and D. O. Olago (Dordrecht: Kluwer Academic Publishers), 335–348.
Aura, M. C., Nyamweya, C., OdoliOwiti, H., Njiru, J. M., Outo, P., Waithaka, E., et al. (2020). Consequences of calamities and their management: The case of COVID-19 pandemic and flooding on inland capture fisheries in Kenya. J. Gt. Lakes. Res. 46, 1767–1775. doi:10.1016/j.jglr.2020.09.007
Aura, M. C., Roegner, A., Owiti, H., Birungi, D., Fiorella, K. J., Corman, J., et al. (2022). Mind the gaps for the best practices: Enhancing the management of Lake Victoria fisheries resources. Lakes Reservoirs Sci. Policy Manag. Sustain. Use 27 (3), e12411. doi:10.1111/lre.12411
Baker, N. E., and Baker, E. M. (2002). “Important bird areas in Tanzania,” in Wildlife conservation society of Tanzania Dar es Salaam, Tanzania.
Barbier, E. B. (1994). Valuing environmental functions: Tropical wetlands. Land Econ. 70, 155–173. doi:10.2307/3146319
Bassa, S., Mbabazi, D., Taabu-Munyaho, A., Nakiyende, H., and Balirwa, J. S. (2014). Can protected areas work in artisanal fisheries of Uganda? The case of lakes edward-george and kazinga channel. Uganda J. Agric. Sci. 15 (1), 27–39.
BBC News (2020). Rising water levels in Kenya's Great Rift Valley threaten jobs and wildlife. Available at: https://www.bbc.com/news/av/world-africa-53776774.
Benson, L. V., 2004. “Western lakes,”in The quaternary period in the United States development in quaternary science. J. Rose 185–204.
Birkett, C. M., Murtugudde, R., and Allan, J. A. (1999). Indian ocean climate event brings floods to East Africa's lakes and the sudd marsh. Geophys. Res. Lett. 26, 1031–1034. doi:10.1029/1999gl900165
Bosilovich, M. G., Robertson, F. R., Takacs, L., Molod, A., and Mocko, D. (2017). Atmospheric water balance and variability in the MERRA-2 reanalysis. J. Clim. Am. Meteorological Soc. 30 (4), 1177–1196. doi:10.1175/jcli-d-16-0338.1
Brasseur, G. P., and Gallardo, L. (2018). Climate services: Lessons learned and future prospects. Rev. Geophys 4, 79–89. doi:10.1002/2015ef000338
Bushozi, P. G. M., Luque, L., and Mabulla, A. Z. P. (2017). Geochronology and technological development: The microscopic and metric evidence from middle stone age (MSA) points at mumba rock shelter, northern Tanzania. Palaeoecol. Afr. 34, 183–206.
Carpenter, S. R., DeFries, R., Dietz, T., Mooney, H. A., Polasky, S., Reid, W. V., et al. (2006). Millennium ecosystem assessment: Research needs. Science 314, 257–258. doi:10.1126/science.1131946
Climate Services Partnership (2015). What are climate services? Climate services partnership. Available at: http://www.climate-services.org/about-us/what-are-climate-services/(Accessed May 9, 2022).
Cocquyt, C., and Vyverman, W. (2005). Phytoplankton in lake Tanganyika: A comparison of community composition and biomass off kigoma with previous studies 27 years ago. Gt. Lakes. Res. 31, 535–546. doi:10.1016/s0380-1330(05)70282-3
Cooke, S. J., Allison, E. H., Beard, T. D., Arlinghaus, R., Arthington, A. H., Bartley, D. M., et al. (2016). On the sustainability of inland fisheries: Finding a future for the forgotten. Ambio 45 (7), 753–764. doi:10.1007/s13280-016-0787-4
Decru, E., Vranken, N., Bragança, P. H., Snoeks, J., and Van Steenberge, M. (2020). Where ichthyofaunal provinces meet: The fish fauna of the Lake Edward system, East Africa. J. Fish Biol. 96 (5), 1186–1201. doi:10.1111/jfb.13992
Delgado, C., Rosegrant, M., Meijer, S., Wada, N., and Ahmed, M. (2002). “Fish as food: Projections to 2020,” in Paper presented in the biennial meeting of international Institute for fisheries economics and trade (IIFET). held on 19-23 August 2002. Wellington, New Zealand.
Descy, J.-P., Hardy, M. A., Stenuite, S., Pirlot, S., Leporcq, B., Kimirel, I., et al. (2005). Phytoplankton pigments and community composition in Lake Tanganyika. Freshw. Biol. 50, 668–684. doi:10.1111/j.1365-2427.2005.01358.x
DeVogel, S. B., Magee, J. W., Manley, W. F., and Miller, G. H. (2004). A GIS-based reconstruction of late Quaternary paleohydrology: Lake Eyre, arid central Australia.
Dutton, J. A. (2002). Opportunities and priorities in a new era for weather and climate services. Bull. Am. Meteorological Soc. 83, 1303–1312. doi:10.1175/1520-0477-83.9.1303
EarthExplorer (2022). EarthExplorer. Available at: https://earthexplorer.usgs.gov/(Accessed July 28, 2022).
Eccles, D. H. (1976). Mass mortalities of Lates (pisces, centropomidae) in lake Albert. J. Limnological Soc. South. Afr. 2 (1), 7–10. doi:10.1080/03779688.1976.9632913
Elkafrawy, S. B., Basheer, M. A., Mohamed, H. M., and Naguib, D. M. (2021). Applications of remote sensing and GIS techniques to evaluate the effectiveness of coastal structures along Burullus Headland-Eastern Nile Delta, Egypt. Egypt. J. Remote Sens. Space Sci. 24 (2), 247–254. doi:10.1016/j.ejrs.2020.01.002
FAO (1999). “FAO fisheries department country profile – Uganda,” in FID/CP/UGA rev. 1, december 1999 (Rome: FAO).
Gasse, F. (2000). Hydrological changes in the african tropics since the last glacial maximum. Quat. Sci. Rev. 19, 189–211. doi:10.1016/s0277-3791(99)00061-x
Guzinski, R., Kass, S., Huber, S., Bauer-Gottwein, P., Jensen, I. H., Naeimi, V., et al. (2014). Enabling the use of Earth observation data for integrated water resource management in Africa with the water observation and information system. Remote Sens. 6, 7819–7839. doi:10.3390/rs6087819
Hamerlynck, O., Duvail, S., Vandepitte, L., Kindinda, K., Nyingi, D. W., Paul, J.-L., et al. (2011). To connect or not to connect? Floods, fisheries and livelihoods in the lower rufiji floodplain lakes, Tanzania. Hydrological Sci. J. 56 (8), 1436–1451. doi:10.1080/02626667.2011.630002
Harrison, S. P., and Digerfeldt, G. (1993). European lakes as palaeohydrological and palaeoclimatic indicators. Quat. Sci. Rev. 12 (4), 233–248. doi:10.1016/0277-3791(93)90079-2
Herrnegger, M., Stecher, G., Schwatke, C., and Olang, L. (2021). Hydroclimatic analysis of rising water levels in the great Rift Valley lakes of Kenya. J. Hydrology Regional Stud. 36, 100857. doi:10.1016/j.ejrh.2021.100857
Hickley, P., Muchiri, M., Britton, R., and Boar, R. (2008). Economic gain versus ecological damage from the introduction of non-native freshwater fish: Case studies from Kenya∼!2008-10-24∼!2008-11-03∼!2008-12-04∼! Open Fish Sci. J. 1 (1), 36–46. doi:10.2174/1874401x00801010036
Hobeichi, S., Abramowitz, G., Contractor, S., and Evans, J. (2020). Evaluating precipitation datasets using surface water and energy budget closure. J. Hydrometeorol. 21 (5), 989–1009. doi:10.1175/jhm-d-19-0255.1
Hulme, M., Doherty, R., Ngara, T., New, M., and Lister, D. (2010). African climate change: 1900-2100. Clim. Res. 17, 145–168. doi:10.3354/cr017145
Jancloes, M., Thomson, M., Costa, M. M., Hewitt, C., Corvalan, C., Dinku, T., et al. (2014). Climate services to improve public health. Int. J. Environ. Res. Public Health 11, 4555–4559. doi:10.3390/ijerph110504555
Jones, R. T., and Jordan, J. T. (2013). LAKE LEVEL STUDIES | overview. Encycl. Quat. Sci., 483–498. doi:10.1016/b978-0-444-53643-3.00167-9
Jong, S. M., Meer, F. D., and Clevers, J. G. P. W. (2006). “Basics of remote sensing,” in Remote sensing image analysis: Including the spatial domain (Kluwer Academic Publishers), 1–15. doi:10.1007/1-4020-2560-2_1
Junk, W. J. (2002). Long-term environmental trends and the future of tropical wetlands. Environ. Conserv. 29, 414–435. doi:10.1017/s0376892902000310
Junk, W. J., Bayley, P. B., and Sparks, R. E. (1989). “The flood pulse concept in river-floodplain systems,” in Proceedings of the international rivers symposium. Editor D. P. Dodge (Honey Harbour, Ontario, Canada: Canadian Special Publication of Fisheries and Aquatic Sciences), 106, 110–127.
Kalluri, H. R., Prasad, S., and Bruce, L. M. (2010). Decision-level fusion of spectral reflectance and derivative information for Robust hyperspectral land cover classification. IEEE Trans. Geoscience Remote Sens. 48 (11), 4047–4058. doi:10.1109/TGRS.2010.2072787
Kelly, K. (2022). Geographic coordinate systems. Geogr. Inf. Sci. Technol. Body Knowl. 2022 (Q1). doi:10.22224/gistbok/2022.1.1
Khan, A. S., MacManus, K., Mills, J., Madajewicz, M., and Ramasubramanian, L. (2018). “Building resilience of urban ecosystems and communities to sea-level rise: Jamaica bay, New York city,” in Handbook of climate change resilience. Editor W. Leal Filho (Cham, Germany: Springer), 1–21.
Kitaka, N., Harper, D. M., and Mavuti, K. M. (2002). Phosphorus inputs to Lake Naivasha, Kenya, from its catchment and the trophic state of the lake. Hydrobiologia 488 (1-3), 73–80.
Kolding, J., and van Zwieten, P. A. M. (2012). Relative lake level fluctuations and their influence on productivity and resilience in tropical lakes and reservoirs. Fish. Res. 115-116, 99–109. doi:10.1016/j.fishres.2011.11.008
Lakepedia (2016). Lake Rukwa, Tanzania. Available at: www.lakepedia.com/lake/rukwa (Accessed May 26, 2022).
Landsat Science (2022). Landsat science. Available at: https://landsat.gsfc.nasa.gov/(Accessed July 28, 2022).
Long’ora, A. E., Abuom, P. O., Owiti, D. O., and Malala, J. O. (2015). Influence of artisanal fishing gears on physico-chemical parameters of Ferguson’s Gulf in Lake Turkana, Kenya. Sch. Acad. J. Biosci. 3 (12), 1005–1013.
Lubala, E.-B.-B., Mumbere, J. C., Masirika, J. M., and Micha, J. C. (2018). Production et impacts de la pêche dans la partie congolaise du Lac Edouard. Tropicultura 36 (3), 539–552.
Masese, F. O., Omukoto, J. O., and Nyakeya, K. (2013). Biomonitoring as a prerequisite for sustainable water resources: A review of current status, opportunities and challenges to scaling up in East Africa. Ecohydrol. Hydrobiology 13, 173–191. doi:10.1016/j.ecohyd.2013.06.004
Mason, I. M., Guzkowska, M. A. J., Rapley, C. G., and Street-Perrot, F. A. (1994). The response of lake levels and areas to climatic change. Clim. Change 27, 161–197. doi:10.1007/bf01093590
McDonough, K., Hutchinson, S., Moore, T., and Hutchinson, J. S. (2017). Analysis of publication trends in ecosystem services research. Ecosyst. Serv. 25, 82–88. doi:10.1016/j.ecoser.2017.03.022
Medri, S., de Guisasola, E. B., and Gualdi, S. (2012). Overview of the main international climate services. Italy: Centro Euro-Mediterraneo sui Cambiamenti Climatici, 109. Available at: https://papers.ssrn.com/sol3/papers.cfm?abstract_id=2194841 (Accessed September 24, 2022).
Mercier, F., Cazenave, A., and Maheu, C. (2002). Interannual lake level f luctuations (1993–1999) in Africa from topex/poseidon: Connections with ocean–atmosphere interactions over the Indian ocean. Glob. Plenary Changes 32, 141–163. doi:10.1016/s0921-8181(01)00139-4
Mörth, H. T. (1967). “Investigations into the meteorological aspects of the variations in the level of Lake Victoria,” in East African met. Dept. Memoirs, 4, 1–22.
Naithani, J., Deleersnijder, E., and Plisnier, P.-D. (2002). Origin of intraseasonal variability in lake Tanganyika. Geophys. Res. Lett. 29, 8-1–8-4. doi:10.1029/2002GL015843
Nicholson, E., Mace, G. M., Armsworth, P. R., Atkinson, G., Buckle, S., Clements, T., et al. (2009). Priority research areas for ecosystem services in a changing world. J. Appl. Ecol. 46 (6), 1139–1144. doi:10.1111/j.1365-2664.2009.01716.x
Nicholson, S. E. (1999). Historical and modern fluctuations of lakes Tanganyika and Rukwa. Clim. Change 41, 53–71. doi:10.1023/a:1005424619718
Nilsson, C., ReidyDynesius, M., and Revenga, C. (2005). Fragmentation and flow regulation of the world’s large river systems. Science 308, 405–408. doi:10.1126/science.1107887
Nyamweya, C. S., Natugonza, V., Taabu-munyaho, A., Aura, M. C., Njiru, M., Ongore, C., et al. (2020). A century of change: Induced revolution of Lake Victoria fisheries and ecology. Fish. Res. 230, 105564. doi:10.1016/j.fishres.2020.105564
Obando, J., Onywere, S., Shisanya, C., Ndubi, A., Masiga, D., Irura, Z., et al. (2016). “Impact of short-term flooding on livelihoods in the Kenya Rift Valley lakes,” in Geomorphology and society, 193–215. doi:10.1007/978-4-431-56000-5_12
Odada, E. C., Olago, D. O., Kulindwa, K., Ntiba, M., and Wandiga, S. (2004). Mitigation of environmental problems in lake Victoria, east Africa: Causal chain and policy options analyses. Ambio 33 (1- 2), 13–23. doi:10.1579/0044-7447-33.1.13
Ogutu-Ohwayo, R., 1998. The fishery of lakes Kyoga and kwania. In; R. Ogutu Ohwayo (Rapporteur general), proceedings of the stakeholders workshop on the fisheries of Lake Victoria and Kyoga. Jinja, Uganda: Fisheries Research Institute. 24-25th September 1998. 38–40.
Okely, P., Imberger, J., and Antenucci, J. P. (2010). Processes affecting horizontal mixing and dispersion in winam gulf, Lake Victoria. Limnol. Oceanogr. 55, 1865–1880. doi:10.4319/lo.2010.55.5.1865
Papa, F., Crétaux, J. F., Grippa, M., Robert, E., Trigg, M., Tshimanga, R. M., et al. (2023). Water resources in Africa under global change: Monitoring surface waters from space. Surv. Geophys. 44, 43–93. doi:10.1007/s10712-022-09700-9
Prendergast, M., Luque, L., Domínguez-Rodrigo, M., Diez-Martín, F., Mabulla, A., and Barba, R. (2007). New excavations at Mumba rockshelter, Tanzania. J. Afr. Archaeol. 5 (2), 217–243. doi:10.3213/1612-1651-10093
R Core Team (2020). R: A language and environment for statistical computing. Vienna, Austria: R Foundation for Statistical Computing. Available at: https://www.R-project.org/.
Scott, D., Lemieux, C., and Malone, L. (2011). Climate services to support sustainable tourism and adaptation to climate change. Clim. Res. 47, 111–122. doi:10.3354/cr00952
Shafer, M. A. (2008). Climate literacy and a national climate service. Phys. Geogr. 29 (6), 561–574. doi:10.2747/0272-3646.29.6.561
Souter, N., and Walters, A. (2006). Proposed Lake Albert Channel – ecological assessment. Technical note tn2006/05. Adelaide, South Australia: Department of Water, Land and Biodiversity Conservation, 28.
Steffen, W., Sanderson, A., Tyson, P. D., Jager, J., Matson, P. A., Moore, B., et al. (2003). Global change and the Earth system: A planet under pressure. The IGBP series. Springer.
Street, F. A., and Grove, A. T. (1976). Environmental and climatic implications of Late Quaternary lake-level fluctuations in Africa. Nature 261 (5559), 385–390.
Street, F. A., and Grove, A. T. (1979). Global maps of lake-level fluctuations since 30,000 yr BP. Quaternary Research 12 (1), 83–118.
Tempa, K., and Aryal, K. R. (2022). Semi-automatic classification for rapid delineation of the geohazard-prone areas using Sentinel-2 satellite imagery. SN Applied Sciences 4 (5). doi:10.1007/s42452-022-05028-6
Tol, R. S. J., Klein, R. J. T., and Nicholls, R. J. (2008). Towards successful adaptation to sea-level rise along Europe’s coasts. J. Coast. Res. 24 (2), 432–442. doi:10.2112/07a-0016.1
Trauth, M. H., Deino, A. L., and Bergner, A. G. N. (2003). East African climate change and orbital forcing during the last 175 kyr BP. Earth Planet. Sci. Lett. 206 (3-4), 297–313.
Twesigye, C. K., Onywere, S. M., Getenga, Z. M., Mwakalila, S. S., and Nakiranda, J. K. (2011). The impact of land use activities on vegetation cover and water quality in the Lake Victoria watershed. Open Enviornmental J. 4, 66–77. doi:10.2174/1874829501104010066
Vaughan, C., and Dessai, S. (2014). Climate services for society: Origins, institutional arrangements, and design elements for an evaluation framework. Wiley Interdiscip. Revis. Clim. Change 5, 587–603. doi:10.1002/wcc.290
Vranken, N., Van Steeberge, M., Heylen, A., Decru, E., and Snoeks, J. (2022). From a pair to a dozen: The piscivorous species of Haplochromis (cichlidae) from the lake edward system. Eur. J. Taxonomy 815, 1–94. doi:10.5852/ejt.2022.815.1749
Welcomme, R. (2008). World prospects for floodplain fisheries. Ecohydrol. Hydrobiology 8, 169–182. doi:10.2478/v10104-009-0013-0
World Lakes (2016). Lake profile Rukwa. Available at: www.worldlakes.org/lakedetails.asp?lakeid=8579 (Accessed February 26, 2022).
World Meteorological Organization (WMO) (2012). Guidelines on frameworks for climate services at the national level. Geneva: World Meteorological Organization. Available at: https://gfcs.wmo.int/sites/default/files/events/Regional%20Workshop%20on%20Climate%20Services%20at%20the%20National%20Level%20for%20the%20LDCs%20in%20Asia/GuidetoClimateServicesattheNationalLevelFinalOctober2012.pdf (Accessed May 9, 2022).
World Meteorological Organization (WMO) (2019). What is the global framework for climate services and what will it accomplish? gfcs. Available at: https://gfcs.wmo.int/node/219. Accessed 9 May 2022.
Wu, H., Chen, J., Xu, J., Zeng, G., Sang, L., Liu, Q., et al. (2019). Effects of dam construction on biodiversity: A review. J. Clean. Prod. 221, 480–489. doi:10.1016/j.jclepro.2019.03.001
Youn, S.-J., Taylor, W. W., Lynch, A. J., Cowx, I. G., Douglas Beard, T., Bartley, D., et al. (2014). Inland capture fishery contributions to global food security and threats to their future. Glob. Food Secur. 3 (3–4), 142–148. doi:10.1016/j.gfs.2014.09.005
Keywords: flooding, rising water levels, wetland, conservation, great lakes
Citation: Aura CM, Nyamweya C, Musa S, Ogari Z, Owoko W, Osore M, May L and Njiru JM (2023) The quantification of the extent of flooding on selected major Afrotropical lakes to guide management implications. Front. Environ. Sci. 11:1062289. doi: 10.3389/fenvs.2023.1062289
Received: 05 October 2022; Accepted: 15 March 2023;
Published: 23 March 2023.
Edited by:
Jianghua Wu, Memorial University of Newfoundland, CanadaReviewed by:
Polina Lemenkova, Université libre de Bruxelles, BelgiumYanhu He, Guangdong University of Technology, China
Juraj Parajka, Vienna University of Technology, Austria
Copyright © 2023 Aura, Nyamweya, Musa, Ogari, Owoko, Osore, May and Njiru. This is an open-access article distributed under the terms of the Creative Commons Attribution License (CC BY). The use, distribution or reproduction in other forums is permitted, provided the original author(s) and the copyright owner(s) are credited and that the original publication in this journal is cited, in accordance with accepted academic practice. No use, distribution or reproduction is permitted which does not comply with these terms.
*Correspondence: Christopher Mulanda Aura, auramulanda@yahoo.com, aura.mulanda@gmail.com