- Departamento de Estudios del Agua y de la Energía, Universidad de Guadalajara, Guadalajara, Mexico
Carbon-based electrodes have been extensively used as cathodes for the electro-generation of H₂O₂, which is used in the production of the hydroxyl radical •OH to mineralize organic compounds in several types of wastewater. Carbon cloth has been also used in combination with activated carbon for the combined adsorption and oxidation of persistent organic compounds present in tequila vinasse wastewater. Whereas most of the works regarding the H₂O₂ electro-generation involve relatively complex processes to enhance the catalytic activity or the electrochemically active area of these electrodes, calcination by itself represents a simple and low-cost option to enhance these cathodic and anodic functions, especially in the fabrication of large area electrodes that could be needed to treat the large amount of tequila vinasse wastewater that is produced daily. In this work, the effect of calcination at 300°C and 600°C of carbon clothes in the oxidation current and H₂O₂ production at different potentials in H₂SO₄ was studied. Oxidation current increased 700% upon calcination at 600°C, compared to no calcination. H₂O₂ was produced only in a narrow range of polarizations, whereas calcination at 600 °C increased the generation rate from 7.1 ± 0.3 to 17.8 ± 0.4 mg L⁻1 h⁻1.
1 Introduction
Tequila is an alcoholic beverage with appellation from middle west region in Mexico (SAGARPA, 2017). In 2021, a total of 527 million liters of Tequila were produced (Consejo Regulador del Tequila, 2021). For each liter of tequila, between 10 and 12 L of vinasse wastewater are produced (López-López et al., 2010). Due to its corrosive nature and high content of total suspended and dissolved solids, discharge of wastewater from tequila vinasse into water bodies could represent a major environmental problem.
Although the contents of wastewater that is discharged into water bodies are regulated in Mexico since 1996, only a fraction of this wastewater receives the proper treatment before being discharged (López-López et al., 2010). Recently it has been estimated that 69% ± 14% of the vinasse wastewater in Jalisco, the state of Mexico where most of tequila is produced, receive full treatment and is discharged into water bodies or used for irrigation, 25% ± 5% receives incomplete treatment and is discharged into the soil or used for irrigation, and 4% ± 1% is treated by an external company (Zurita et al., 2022). The main reason for the discharge without treatment could be the economic limitation of distilleries to achieve treatment technologies (Díaz-Vázquez et al., 2021).
The direct discharge of vinasse wastewater into water bodies or soil represent a serious threat to these ecosystems, since they have high temperature (70°C or more), acidic pH (3.0–4.5) and a large amount of total suspended (2,000–8,000 mg L-1) and dissolved (23,000–42,000 mg L-1) solids, modifying the color, pH and conductivity of water and adding putrescible organic compounds (Prajapati and Chaudhari, 2015; Castillo-Monroy et al., 2020). Additionally to these physicochemical properties, Biochemical Oxygen Demand (BOD5) and Chemical Oxygen Demand (COD) of tequila vinasse wastewater have received special attention, since water with a high BOD or COD load decreases the dissolved oxygen concentration, affecting the aquatic life. Between 1996 and 2021, Mexican regulation allowed a maximum of 150 mg L-1 of BOD5, whereas COD was not regulated (SEMARNAT, 1996). Distilleries with a conventional wastewater treatment consisting of a combination of biological and physicochemical steps such as coagulation, flotation, aerobic and/or anaerobic digestion, etc., were able to comply with this regulation. More than 100 volatile compounds have been identified in tequila vinasse, which are mainly removed by the anaerobic digestion process (Rodriguez Arreola et al., 2020). However, tequila vinasse wastewater has a small content of persistent organic pollutants, which remain after conventional treatment and maintain the COD values between 300 and 1,000 mg L-1 (Castillo-Monroy et al., 2020). These compounds correspond mainly to phenolic species (Colin et al., 2016).
In 2022, a limit of 150 mg L-1 COD value was published in Mexican regulation (SEMARNAT, 2022). This new COD limit encouraged distilleries to adopt tertiary treatment approaches capable of reaching regulatory discharge standards. Although physicochemical treatments such as adsorption have been implemented to remove the persistent pollutants and lower the COD, these methods imply the formation of solid waste. Processes that aim to oxidize at least partially these persistent pollutants have been recently explored, such as Advanced Oxidation Processes (AOPs) (Ferral-Pérez et al., 2016; Castillo-Monroy et al., 2020; Rodriguez Arreola et al., 2020).
Among AOPs, the Fenton reagent constitutes a popular approach (Fenton, 1894). Briefly, the Fenton mixture consists of H2O2 and Fe2+ in acidic medium that react to produce the hydroxyl radical (•OH), as stated in Eq. 1:
The Fenton process presents cost, stability and safety issues regarding the acquisition and handling of H2O2 reagent. In this context, electrochemically assisted AOPs (E-AOPs) present a solution to these problems by a double approach: Firstly, they allow the direct electro-oxidation of the persistent pollutants on the anode surface. Secondly, the E-AOP effect can be enhanced by the production of the •OH species in the electrode/solution interphase or in the bulk of the solution, eliminating some safety risks of H2O2 transportation and handling and reducing the reagents cost (Oturan and Brillas, 2007; Fang et al., 2017; Moreira et al., 2017). Enhanced electro-oxidation of vinasse wastewater has been studied by Castillo-Monroy et al. (2020). However, the effect of anodic removal of pollutants and electro-Fenton process could not be studied separately since the complex composition of vinasse wastewater hinders the determination of •OH formation.
H2O2 can be produced by electrochemical reduction of O2 in the cathode as stated in Eq. 2:
The formation of H2O2 in carbonaceous materials such as vitreous carbon, graphite and carbon felt, sponge, and cloth has been extensively studied (Pérez et al., 2017). More recently, H2O2 production rates of 1,387, 30 and 1,035 mg l-1 h-1 have been achieved by using respectively modified carbon cloth and carbon black as electrodes (Pérez et al., 2017; Zárate-Guzmán et al., 2019; Zhang et al., 2019). These works regard the modification of carbon-based materials to improve the catalytic activity or the electroactive area of the electrode and therefore the H2O2 generation rate.
Calcination of carbonaceous materials has shown to introduce oxygen functional groups in carbon cloth and carbon black, enhancing desired functionality (Gu et al., 2018; Zhang et al., 2019). Although some of the mentioned works include calcination of carbon-based electrodes as a step of the modification process, only the work of Zhang et al. (2019) regards the calcination temperature effect. Taking into consideration the large amounts of vinasse produced in tequila industry, calcination could represent a simple and low-cost modification process that facilitates the scaling up of electrode size for E-AOP-based wastewater treatment processes. In this regard, this work aims to study the effect of calcination per se in the electro-generation rate of H2O2 by using carbon clothes as electrodes, and to analyze the possibility to extend these results to other carbonaceous materials.
2 Materials and methods
2.1 Materials
Calcination of carbon clothes (Grupo ROOE) was developed at 300 or 600°C in a simple furnace. H2SO4, FeSO4•7H2O, FeCl2•4H2O (Sigma-Aldrich), dry air 99% (Grupo Infra, S.A. de C.V.) were used as received. Conventionally treated tequila vinasse wastewater was collected from the effluent at the exit of the treatment plant of a tequila distillery from Tequila, Jalisco, Mexico, and stored at 2°C.
2.2 Fenton reaction
Conventionally treated tequila vinasse wastewater (170 mg L-1 COD) was mixed with H2O2 in excess and enough FeSO4•7H2O or FeCl2•4H2O to produce •OH that stoichiometrically would remove the mentioned COD.
2.3 Cyclic voltammetry
A three-electrode-cell was built by using a carbon cloth with an exposed area of 3.63 cm2 as working electrode, a Pt mesh of 5 cm × 5 cm as counter electrode and a Ag|AgCl wire as reference electrode. This wire is considered a pseudo-reference electrode since it is directly immersed in the solution (Inzelt, 2013). A PalmSens4 potentiostat was used to control the cell potential. An air stone (Pawfly) connected to a dry air tank was used to bubble dry air in the cell when necessary.
2.4 H2O2 generation
Six carbon clothes with a total area of 15 cm2 were immersed and used as electrodes in 110 mL of 0.100 M H2SO4. The potential/current was controlled with a 42AH0477 Newark power supply. Dry air was bubbled from the bottom of the cell. Figure 1 shows a schematic diagram of the electrochemical cell. Polarization between 0.6 and 3.6 V was applied for about 12 min in order to generate H2O2 in the cathode.
2.5 H2O2 determination
Samples of 3 mL from the solution in the H2O2 generation cell were taken and mixed with 10 µL TiOSO4 solution in order to form the Ti•H2O2 complex (Ribeiro et al., 2009). The mixture absorbance at 405 nm was measured by means of a V-770 UV-Vis spectrometer (JASCO) in order to determine the complex concentration with a previously developed calibration curve by using QtiPlot® software.
2.6 COD determination
Tequila vinasse wastewater was sampled and put in digestion vials (Hach) for 2 h in a digestor (Hanna Instruments). The COD of digested samples was measured with a DR900 colorimeter (Hach) (Oxygen Demand, Chemical USEPA 1 Reactor Digestion Method 2 Method 8000 Test preparation Instrument-specific information, 2021).
3 Results
3.1 Fenton reagent
Fenton reagent has shown previously its potential to oxidize persistent organic compounds in vinasse wastewater (Castillo-Monroy et al., 2020). However, in the industry it is known that the persistent compounds concentration for vinasse from different sources or even from the same source and different batch process could vary. In this regard, the oxidation experiment had to be repeated for the specific vinasse wastewater sample collected for this work. Tequila vinasse wastewater with COD0 = 170 ± 7 mg L−1 was mixed with H2O2 with or without FeSO4 or FeCl2 salts to compare the COD removal with or without the expected generation of •OH. The final CODs in mg l-1 of different mixtures were: H2O2: greater than 1,500; H2O2 + FeSO4: 29 ± 11; H2O2 + FeCl3: 293 ± 3.
3.2 H2O2 generation
Figure 2 shows a cyclic voltammograms at 50 mV s-1 of a carbon cloth used as working electrode with exposed area of 3.63 cm2. An increment of about 0.6 mA cm-2 is observed in the reduction peak at −0.6 V vs. Ag|AgCl as dry air is bubbled, indicating that an oxygen-consuming reduction reaction occurred. A control experiment by bubbling N2 instead of dry air was performed to ensure that the increase in the current was due to the presence of oxygen in the electrolyte, and not to the electrolyte agitation by bubbling (not shown).
Figure 3 shows cyclic voltammograms at 50 mV s-1 of carbon clothes previously calcinated at different temperatures, immersed in 0.020 M H2SO4 with exposed area of 3.63 cm2 and a 5 cm × 5 cm Pt mesh as counter electrode. Solid lines and marks represent respectively no bubbling and bubbling air during the experiment. Although greater oxidation and reduction currents were observed as the calcination temperature increased, the increase in reduction current due to air bubbling at −0.6 V vs. Ag|AgCl was not proportional to the current for each calcination temperature.
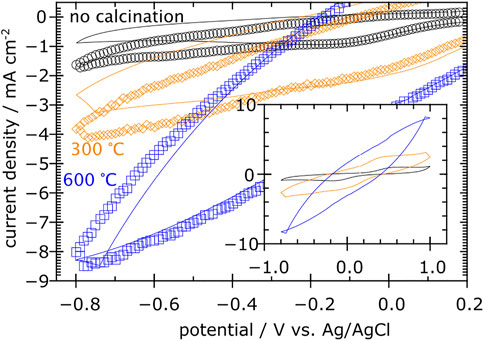
FIGURE 3. Cyclic voltammograms of carbon clothes calcinated at different temperatures and immersed in 0.020 M H2SO4.
The H2O2 production as a function of the polarization for carbon clothes calcinated at different temperatures and used as electrodes for the electrochemical cell in section 3.4 is shown in Figure 4. For all calcination temperatures, H2O2 is formed only above certain potential that ranges from 2.4 to 2.8 V. Maximum generation rates of 7.1 ± 0.3, 14.3 ± 0.2 and 17.8 ± 0.4 mg l h-1 were achieved by using clothes without calcinating and calcinated at 300°C and 600°C respectively. At polarizations above the maximum H2O2 generation potential for each calcination temperature, the generation decreased.
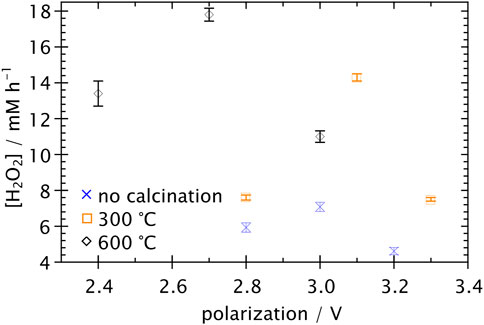
FIGURE 4. H2O2 formation rate as a function of potential using carbon clothes calcinated at different temperatures.
4 Discussion
4.1 Fenton reagent
The Fenton reaction was attempted to be performed with excess of H2O2 and Fe2+ salts. The [H2O2]/[Fe2+] ratio was high, although optimal concentrations to maximize •OH generation were not used (Bouafia-Chergui et al., 2010). Given the final COD values, apparently only FeSO4 removed COD with an efficiency of 83%. It should be noted that since Fe3+/Fe2+ concentrations were below the solubility limit of all Fe salts, thereby no acid was added to lower the pH and prevent precipitation. This achieved COD removal is lower than the 90% at high pH reported previously by Castillo-Monroy et al. (2020). However, the purpose of the experiment was to ensure that Fenton reagent could remove the COD of the vinasse wastewater collected for this work.
H2O2 added to vinasse wastewater possibly did not react with the persistent organic compounds, and therefore reduced Cr6+ in the vial during digestion, leading to an apparently higher COD than the original vinasse wastewater sample (Kawanishi et al., 1986). H2O2 mixed with FeCl2 presented an apparently higher COD than vinasse wastewater possibly due to the excess of H2O2, which could promote the regeneration of Fe2+ according to Eqs 3, 4, therefore consuming the Cr6+ of digestion vials to oxidate Fe2+ to Fe3+ (Bouafia-Chergui et al., 2010).
4.2 H2O2 generation
The increase in reduction current due to air bubbling at −0.6 V vs. Ag|AgCl in Figure 2 could correspond to oxygen-consuming reactions, although clearly only a fraction corresponds to H2O2 formation as set in Eq. 2. From Figure 2, we can infer that applying potentials below −0.6 V vs. Ag|AgCl could diminish the H2O2 production due to the presence of side reactions such as (5) and (6), or even worse, H2O2 reduction reactions, such as (7), as reported previously (Brillas and Casado, 2002; Luo et al., 2015).
From Figure 3, the cathodic current at −0.6 V vs. Ag|AgCl increased with the calcination temperature, from 1 to 7 mA cm-2 at no calcination and calcination at 600°C respectively. This result agreed with the one from Zhang et al. (2019), where reduction current increased from 8.1 to 117.6 mA with calcination. Additionally, the inset showed that both reduction and oxidation current increased with calcination. This result suggests that calcination has the potential to enhance the electro-oxidation of persistent organic compounds in carbon-based anodes. A constant reduction current increase of about 0.6 mA cm-2 as dry air is bubbled, compared to the experiment without bubbling, regardless of the calcination temperature, could be observed. This suggests that the enhancement of H2O2 electro-generation in the cathode could be significatively lower than the electro-oxidation enhancement.
Figure 4 showed the behaviour of H2O2 generation rate with applied polarization for electrodes without calcination and calcinated at 300°C and 600°C in the electrochemical cell described in section 3.4. The minimum polarization value for H2O2 formation (2.4–2.8 V) differed substantially from the −0.6 V potential needed for observing oxygen-consuming reaction in cyclic voltammetry. This can be explained since H2O2 generation experimental setup corresponded to a two-electrode cell, without reference electrode and using the carbon clothes as cathode and anode, instead of using a Pt mesh with greater area as counter-electrode. Therefore, higher overpotentials were needed for reduction reactions to occur in anode-cathode cells used in industry than three-electrode cells used typically in the laboratory. H2O2 production reached a maximum and then diminished at higher polarizations, suggesting that it is mainly produced only at a small range of potentials, as concluded previously by Zárate-Guzmán et al. (2019). A possible explanation is the fact that H2O2 reduction presented in Eq. 7 occurs slowly in carbon-based materials, however, high overpotentials could increase the reaction kinetics and therefore reduce H2O2 in the cathode as it is formed according to Eq. 2 (Luo et al., 2015). Additional experiments at potential differences greater than 3.6 V for 12 min were performed after electro-generating H2O2 in carbon clothes at 3.0 V for 12 min. The H2O2 concentration decreased totally after the experiments at higher potential differences.
Furthermore, Figure 4 showed that the H2O2 formation rate increased with calcination temperature. This result has been previously explained by considering that calcination introduces carbon oxidized surface groups such as C-O-C, C-OH, C=O and O=C-OH, enhancing the oxygen reduction reaction in the electrode surface and increasing the specific surface area (Gu et al., 2018; Zhang et al., 2019). The maximum H2O2 production rate was achieved by applying a polarization of 2.7 V using carbon clothes calcinated a 600°C. This rate of 17.8 ± 0.4 mg l-1 h-1 is still below to the 31 mg l-1 h-1 rate achieved in carbon felt by Zárate-Guzmán et al. (2019), and far below the 1,051 and 1,387 mg l-1 h-1 rates obtained respectively in black carbon by Zhang et al. (2019) and in carbon cloth modified with carbon black by Pérez et al. (2017). This result can be explained by the small specific area of carbon cloth compared to those of carbon felt and carbon black. However, these results showed that calcination by itself without further electrode modification increased the H2O2 generation rate, opening the possibility to enhance the oxidation of persistent compounds in tequila vinasse wastewater by a simple method, which is easier to scale up in electrode size than other surface modification techniques. Aditionally, these results open the possibility to study the enhancement of H2O2 production by further calcination in other modification processes such as the one proposed by Zárate-Guzmán et al. (2019), or to study the effect of calcination at higher temperatures than 300°C in the modification process proposed by Pérez et al. (2017). Naturally, degradation of materials different to carbon in modified electrodes by calcination must be taken into account to avoid negative effects (Zhao et al., 2021).
Data availability statement
The raw data supporting the conclusion of this article will be made available by the authors, without undue reservation.
Author contributions
EM and AE-V contributed to conception and design of the study. RC-D-T and MH-R performed the experiments. LL, EM, and AE-V wrote sections of the manuscript. All authors listed have made a substantial, direct, and intellectual contribution to the work and approved it for publication.
Funding
The authors acknowledge Secretaría de Educación Pública (SEP, Mexico) for funding this research work through grant FCA-23212-2020, and Universidad de Guadalajara (Mexico) for funding the open access publication fee. Scholarships for RC-D-T. and LL from Consejo Nacional de Ciencia y Tecnología (CONACYT, Mexico) are also fully acknowledged.
Conflict of interest
The authors declare that the research was conducted in the absence of any commercial or financial relationships that could be construed as a potential conflict of interest.
Publisher’s note
All claims expressed in this article are solely those of the authors and do not necessarily represent those of their affiliated organizations, or those of the publisher, the editors and the reviewers. Any product that may be evaluated in this article, or claim that may be made by its manufacturer, is not guaranteed or endorsed by the publisher.
References
Bouafia-Chergui, S., Oturan, N., Khalaf, H., and Oturan, M. A. (2010). Parametric study on the effect of the ratios [H2O 2]/[Fe3+] and [H2O2]/[substrate] on the photo-Fenton degradation of cationic azo dye Basic Blue 41. J. Environ. Sci. Health A Tox Hazard Subst. Environ. Eng. 45, 622–629. doi:10.1080/10934521003595746
Brillas, E., and Casado, J. (2002). Aniline degradation by Electro-Fenton® and peroxi-coagulation processes using a flow reactor for wastewater treatment. Chemosphere 47, 241–248. doi:10.1016/S0045-6535(01)00221-1
Castillo-Monroy, J., Godínez, L. A., Robles, I., and Estrada-Vargas, A. (2020). Study of a coupled adsorption/electro-oxidation process as a tertiary treatment for tequila industry wastewater. Environ. Sci. Pollut. Res. 28, 23699–23706. doi:10.1007/s11356-020-11031-4
Consejo Regulador del Tequila (2021). Informacion estadistica. Available at: https://www.crt.org.mx/EstadisticasCRTweb/.
Díaz-Vázquez, D., Carrillo-Nieves, D., Orozco-Nunnelly, D. A., Senés-Guerrero, C., and Gradilla-Hernández, M. S. (2021). An integrated approach for the assessment of environmental sustainability in agro-industrial waste management practices: The case of the tequila industry. Front. Environ. Sci. 9, 25. doi:10.3389/fenvs.2021.682093
Fang, C., Megharaj, M., and Naidu, R. (2017). Electrochemical Advanced Oxidation Processes (EAOP) to degrade per- and polyfluoroalkyl substances (PFASs). J. Adv. Oxid. Technol. 20, 0014. doi:10.1515/jaots-2017-0014
Fenton, H. J. H. (1894). LXXIII.—oxidation of tartaric acid in presence of iron. J. Chem. Soc. Trans. 65, 899–910. doi:10.1039/CT8946500899
Ferral-Pérez, H., Torres Bustillos, L. G., Méndez, H., Rodríguez-Santillan, J. L., and Chairez, I. (2016). Sequential treatment of tequila industry vinasses by biopolymer-based coagulation/flocculation and catalytic ozonation. Ozone Sci. Eng. 38, 279–290. doi:10.1080/01919512.2016.1158635
Gu, Y. J., Wen, W., and Wu, J. M. (2018). Simple air calcination affords commercial carbon cloth with high areal specific capacitance for symmetrical supercapacitors. J. Mater Chem. A Mater 6, 21078–21086. doi:10.1039/C8TA07561A
Kawanishi, S., Inoue, S., and Sano, S. (1986). Mechanism of DNA cleavage induced by sodium chromate (VI) in the presence of hydrogen peroxide. J. Biol. Chem. 261, 5952–5958. doi:10.1016/s0021-9258(17)38476-4
López-López, A., Davila-Vazquez, G., León-Becerril, E., Villegas-García, E., and Gallardo-Valdez, J. (2010). Tequila vinasses: Generation and full scale treatment processes. Rev. Environ. Sci. Biotechnol. 9, 109–116. doi:10.1007/s11157-010-9204-9
Luo, H., Li, C., Wu, C., Zheng, W., and Dong, X. (2015). Electrochemical degradation of phenol by in situ electro-generated and electro-activated hydrogen peroxide using an improved gas diffusion cathode. Electrochim Acta 186, 486–493. doi:10.1016/j.electacta.2015.10.194
Moreira, F. C., Boaventura, R. A. R., Brillas, E., and Vilar, V. J. P. (2017). Electrochemical advanced oxidation processes: A review on their application to synthetic and real wastewaters. Appl. Catal. B 202, 217–261. doi:10.1016/j.apcatb.2016.08.037
Oturan, M. A., and Brillas, E. (2007). Electrochemical advanced oxidation processes (EAOPs) for environmental applications. Port. Electrochimica Acta 25, 1–18. doi:10.4152/pea.200701001
Oxygen Demand, Chemical USEPA 1 Reactor Digestion Method 2 Method 8000 Test preparation Instrument-specific information (2021). Available at: https://latam.hach.com/asset-get.download.jsa?id=7639983816 (Accessed March 30, 2023)
Pérez, J. F., Sáez, C., Llanos, J., Cañizares, P., López, C., and Rodrigo, M. A. (2017). Improving the efficiency of carbon cloth for the electrogeneration of H2O2: Role of polytetrafluoroethylene and carbon black loading. Ind. Eng. Chem. Res. 56, 12588–12595. doi:10.1021/acs.iecr.7b02563
Ribeiro, J. P. N., Segundo, M. A., Reis, S., and Lima, J. L. F. C. (2009). Spectrophotometric FIA methods for determination of hydrogen peroxide: Application to evaluation of scavenging capacity. Talanta 79, 1169–1176. doi:10.1016/j.talanta.2009.02.039
Rodriguez Arreola, A., Sanchez Tizapa, M., Zurita, F., Morán-Lázaro, J. P., Castañeda Valderrama, R., Rodríguez-López, J. L., et al. (2020). Treatment of tequila vinasse and elimination of phenol by coagulation–flocculation process coupled with heterogeneous photocatalysis using titanium dioxide nanoparticles. Environ. Technol. (United Kingdom) 41, 1023–1033. doi:10.1080/09593330.2018.1518994
SAGARPA (2017). Planeación agrícola nacional 2017-2030, AGAVE TEQUILERO Y MEZCALERO. 1st ed. Ciudad de México: SAGARPA.
SEMARNAT (1996). Norma oficial mexicana NOM-001-SEMARNAT-1996. México: Diario Oficial de la Federación.
SEMARNAT (2022). Norma oficial mexicana NOM-001-SEMARNAT-2021. México: Diario Oficial de la Federación.
Zárate-Guzmán, A. I., González-Gutiérrez, L. V., Godínez, L. A., Medel-Reyes, A., Carrasco-Marín, F., and Romero-Cano, L. A. (2019). Towards understanding of heterogeneous Fenton reaction using carbon-Fe catalysts coupled to in-situ H2O2 electro-generation as clean technology for wastewater treatment. Chemosphere 224, 698–706. doi:10.1016/j.chemosphere.2019.02.101
Zhang, H., Li, Y., Zhao, Y., Li, G., and Zhang, F. (2019). Carbon black oxidized by air calcination for enhanced H2O2 generation and effective organics degradation. ACS Appl. Mater Interfaces 11, 27846–27853. doi:10.1021/acsami.9b07765
Zhao, Q., Li, N., Liao, C., Tian, L., An, J., and Wang, X. (2021). The UV/H2O2 process based on H2O2 in-situ generation for water disinfection. J. Hazard. Mater. Lett. 2, 100020. doi:10.1016/j.hazl.2021.100020
Keywords: Tequila vinasse wastewater, tertiary treatment, electro-oxidation, hydrogen peroxide generation, Fenton reagent
Citation: Covarrubias-Del-Toro R, Huerta-Rocha M, Lezama L, García EXM and Estrada-Vargas A (2023) Hydrogen peroxide formation in carbon clothes for enhancement of an electro-oxidation tertiary treatment for tequila vinasse wastewater. Front. Environ. Sci. 11:1059259. doi: 10.3389/fenvs.2023.1059259
Received: 01 October 2022; Accepted: 10 May 2023;
Published: 23 May 2023.
Edited by:
Maria Soledad Peresin, Auburn University, United StatesReviewed by:
Yufei Nan, Auburn University, United StatesLuis Godinez, Autonomous University of Queretaro, Mexico
Copyright © 2023 Covarrubias-Del-Toro, Huerta-Rocha, Lezama, García and Estrada-Vargas. This is an open-access article distributed under the terms of the Creative Commons Attribution License (CC BY). The use, distribution or reproduction in other forums is permitted, provided the original author(s) and the copyright owner(s) are credited and that the original publication in this journal is cited, in accordance with accepted academic practice. No use, distribution or reproduction is permitted which does not comply with these terms.
*Correspondence: Arturo Estrada-Vargas, YXJ0dXJvLmV2YXJnYXNAYWNhZGVtaWNvcy51ZGcubXg=
†These authors have contributed equally to this work and share first authorship