Corrigendum: Constructing futures, enhancing solutions: Stakeholder-driven scenario development and system modeling for climate-change challenges
- 1University of Idaho, Moscow, ID, United States
- 2Environmental Science, Policy, and Sustainability, Southern Oregon University, Ashland, OR, United States
Finding effective and practical solutions to climate change challenges in food-energy-water systems requires the integration of experts in local/regional social and biophysical systems, and these are commonly local community members. In the Magic Valley, Idaho we investigated the tensions between water used for energy and to irrigate cropland for food production, as well as, strategies for protecting water quantity and quality. Incorporating stakeholders with long-standing expertise allows the development of solutions to these challenges that are locally and regionally practical and consistent with the values of the social system into which they are incorporated. We describe a stakeholder-driven process used in a case study in the Magic Valley that incorporated local experts to develop plausible future scenarios, identify drivers of change, vet impact and hydrological modeling and map areas of change. The process described allowed stakeholders to envision alternative futures in their region, leading to development of enhanced context and place-based solutions and an anticipated time line for adoption of those solutions. The solutions developed by the stakeholders have been applied across many geographic areas. The described process can also be applied across a broad range of geographic levels. Most importantly, stakeholders should be involved in anticipating solutions and solution timing to the differing challenges posed by each scenario.
Introduction
In the absence of planning for plausible future change, communities and regions are unlikely to be prepared to meet future challenges, particularly when future scenarios are developed over time-periods longer than the one-to five-year time frame in which planning usually takes place (Parkison, 2021). Effective planning requires a number of steps, including setting the limits of imagined futures (finding scenarios between the worst and best imagined futures), setting spatial and time boundaries, identifying the issues that most concern the stakeholders building the scenarios, and defining the uncertainties with which stakeholders will be confronted when planning for the future (Cronan et al., 2022b). Most importantly, stakeholders should be involved in anticipating solutions and solution timing to the differing challenges posed by each scenario. Much research has been devoted to participatory scenario planning (PSP) which commonly incorporates most or all of the steps described above and sometimes involves modeling (e.g., (Kok, 2009; Palomo et al., 2011; Oteros-Rozas et al., 2015; Flynn et al., 2018; Planque et al., 2019).
Taking the process beyond PSP, conceptual, representational, and impact modeling can be used (e.g., Walz et al., 2007; Volkery et al., 2008; Nol et al., 2012; Plieninger et al., 2013; Reed et al., 2013; Hassenforder et al., 2015; Kok et al., 2015; McBride et al., 2017; Kebede et al., 2018; Reinhardt et al., 2018; DasGupta et al., 2019; Izydorczyk et al., 2019; Kabaya et al., 2019; Xexakis and Trutnevyte, 2019; Hagemann et al., 2020; Sanjuan-Delmás et al., 2021) to simulate population change, planting of different crops and increases or decreases in agricultural land, changes to temperature and precipitation and timing of precipitation (climate), as well as hydrologic change to ground and surface water. Modeling can help stakeholders visualize and understand the time scales and geographic extent of likely changes for their region as well as to identify the primary drivers and impacts of projected changes (Cronan et al., 2022a). Mapping potential changes predicted by the models can help stakeholders visualize the geographic extent of changes (Cronan et al., 2022a). All of these tools combined are key to helping stakeholders fully understand and visualize plausible futures for their region, the temporal and geographic scales of the change, how they might adapt to varied changes described in each future scenario and identify potential solutions to challenges explored in each scenario.
Stakeholder-developed solutions are not a common practice in food, energy, water systems (FEWS) research. Meta-analysis of 217 FEWS related papers published world-wide indicated that only 45 involved people other than a research team in their project (Kliskey et al., 2021). Of those, 11 projects involved community members in identifying solutions to local problems (Kliskey et al., 2021). Most of the 217 papers proposed solutions, but it is unclear how many, if any, of them were adopted.
Solutions envisioned by stakeholders are more likely to be regionally and contextually appropriate because stakeholders are aware of values and attitudes that would make solutions easier or more difficult to adopt, accepted by their communities (Buchecker et al., 2013) and more likely to be implemented (Luz, 2000) because stakeholders can advocate for implementation. Involvement of stakeholders reduces community perception that scientists are dictating solutions to communities without their input (Huxham et al., 2000; Ansell and Gash, 2008; Ansell, 2012; Emerson et al., 2012; Jones and White, 2022; Kliskey et al., In review).
Long residence in a region allows stakeholders to visualize how other parts of a FEWS will respond when there is a change to one part of that system. They are able to conceptually integrate drivers and impacts of change into effective solutions. Adding timing of solutions allows stakeholders to envision future impacts of adopting a solution earlier or later in a scenario’s time horizon. All exercises lead to a better understanding of possible futures, possible solutions, and different future trajectories given differing timing of solution adoption and adoption of different solutions.
Solutions to FEWS problems are necessarily context and place-based (Kliskey et al., In review). The objectives of this research were to co-produce solutions and strategies for reducing use, and increasing reuse, of water, nutrients, and energy in the linked dairy and feed crop sectors of Idaho’s agricultural industry in the Magic Valley (MV). The solutions generated were specific to this research question and to the Magic Valley. Non-etheless, all of the solutions developed in this project have been adopted in other geographic regions (Table 1). Not surprisingly, solutions were selected to address stressors presented in each scenario, for example, scenarios depicting water stress elicited solutions to conserve water, and scenarios with high population growth elicited solutions to accommodate more people.
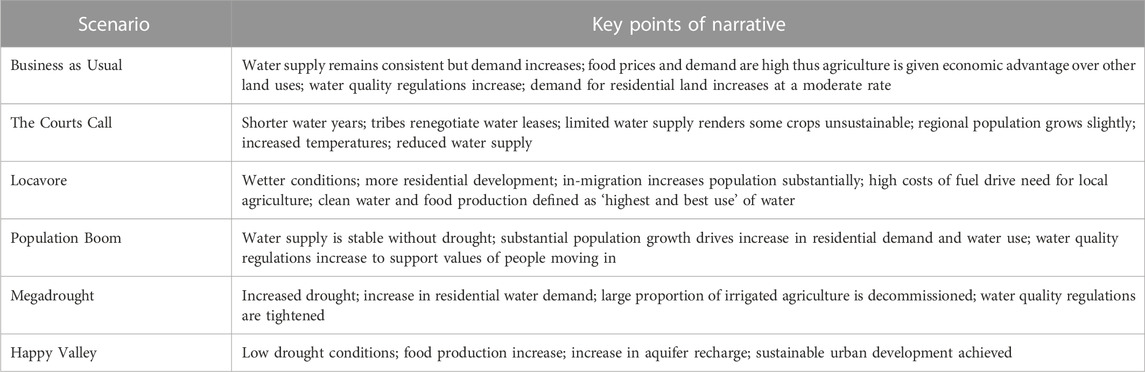
TABLE 1. Summary narratives for the six scenarios co-developed with the stakeholder advisory group for the Magic Valley, Idaho (based on Cronan et al., 2022b).
In a given situation, many factors will impact which scenarios are developed and which solutions are applied in each scenario. Those factors include the issues that stakeholders and researchers chose to address, the composition of the stakeholder group, and individual stakeholder’s roles in the community. For example, in our project, a canal company executive improved our hydrologic modeling because of his in-depth understanding of the water system and of water models. Our rural planning stakeholder consistently provided a more progressive viewpoint and advocated for different solutions, which were sometimes incorporated by the group. We present our process and explain the solutions in depth as an example of how this approach can lead to more appropriate and acceptable local and regional solutions.
Study site
The Magic Valley, Idaho, United States of America (Figure 1) was the focus of our research. It is situated along the Snake River in Southern Idaho. It receives approximately 250 mm precipitation annually, making it a semi-arid environment. Much of the Upper Snake River Basin (USRB) is underlain by the East Snake River Plain aquifer, a highly transmissive aquifer illustrated by the increase in aquifer head during periods that flood irrigation was used and a current decline due to more efficient irrigation techniques (Zuidema et al., 2020). The population of the valley is approximately 186,000; the City of Twin Falls (population 50,000) is the major urban center. Agricultural production, notably dairy and crop production, is the primary economic engine of the area and is a significant contributor to Idaho’s agricultural economy. In 2013, Magic Valley farm gate receipts represented 47% of Idaho’s total farm gate receipts (Hines et al., 2013).
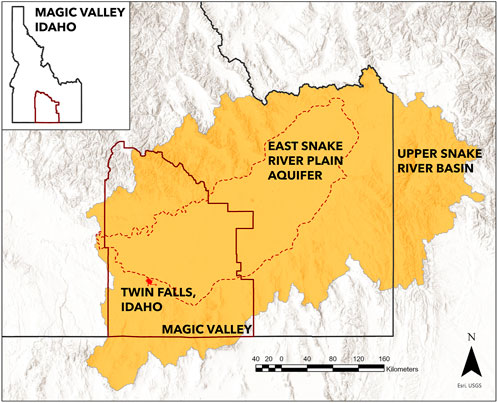
FIGURE 1. Location map of the Upper Snake River Basin and the Magic Valley (outlined in maroon), Idaho, United States of America.
Water, energy, and food components
Climatologically, Idaho has experienced a long-term but variable warming of about 0.8°C over the last century (Abatzoglou et al., 2014), which has increased evapotranspiration and thus reduced the amount of water available in the system (Kliskey et al., 2019). Reduced spring snowpack, especially in lower-elevation watersheds, has contributed to a one-to 2-week advancement in the center of timing of runoff of snowmelt, decreases in annual streamflow, and annual minimum streamflow have been attributed to changes in precipitation (Kliskey et al., 2019).
Although about 70% of Idaho’s energy comes from out of state (U.S. Energy Information Administration - EIA - Independent Statistics and Analysis, 2022), in 2021 hydroelectric power supplied 51% of Idaho’s in-state generation and that percentage has decreased over time because of lower river flows (U.S. Energy Information Administration - EIA - Independent Statistics and Analysis, 2022). As population and demand for electricity generation has grown, so has tension between use of water for agriculture and for power generation. In 1984, an agreement was entered into between the State of Idaho and Idaho Power that established Idaho Power rights to in-stream flow for power generation, known as the Swan Falls Agreement (Strong and Orr, 2016).
Dairy farming has expanded exponentially in Idaho. Most of this growth in dairy has occurred in the MV in which approximately 73% of dairy cows in Idaho are located (Idaho Dairymen’s Association, 2019). In 1997 USDA reported about 265,000 dairy cows in all of Idaho (USDA National Agricultural Statistics Service, 1997a), 580,000 head in 2008, the 4th largest in the U.S. (Brown, 2012), and an estimated 732,000 cows (Idaho State Department of Agriculture, 2020) in 2018/2019 with a ranking of 3rd in the nation in dairy cow inventory (Leytem et al., 2013). The increased number of cows has caused nutrient management issues in the MV (Hristov et al., 2006; Leytem et al., 2021).
Agriculture in the region has responded to the growth of dairy. In 1997 total hectares of forage crops (including alfalfa) in the MV was 116,243 and corn silage was 35,423 (USDA National Agricultural Statistics Service, 1997b). From 2014 to 2018 the average hectares of cropland dedicated to alfalfa alone (not other forages) was 147,709 and 84,438 to corn silage (USDA National Agricultural Statistics Service). Idaho is first in the nation in production of alfalfa hay; in addition to their traditionally grown potatoes and barley production (Idaho Crops–Idaho State Department of Agriculture, N.D.). Other major crops include sugarbeets and wheat (Idaho Crops–Idaho State Department of Agriculture, N.D.). Forage crops, particularly corn silage and alfalfa, use more water than other crops that were more prevalent in the past, such as dry beans, and this has increased the competition, in short water years, between water for energy production and water for agriculture. This served as the background for stakeholder development of solutions to potential futures in the MV.
Methods
We adopted and developed a deliberative, participatory co-production process (Meadow et al., 2015; Kliskey et al., 2021; Kliskey et al., in review) with a stakeholder advisory group (SAG) for MV1. The goal of the process was to iteratively co-develop a conceptualization of the MV FEWS, to construct plausible alternative futures, model those futures, and identify potential solutions to key challenges identified in the alternative futures. The process followed seven steps:
1. Stakeholder advisory group development: During the early phase of the project a SAG was recruited as an indicative group of stakeholder via a snowball technique (Kliskey et al., in review).
2. Key issues: The SAG identified top issues and decisions facing FEWS in the MV over a 30-year time horizon (2020–2050) (Villamor et al., 2020). This time frame was selected as one in which uncertainties would not be so large as to significantly hinder scenario development.
3. Critical uncertainties: The SAG then listed and prioritized critical uncertainties that would affect the issues and decisions they had listed (Cronan et al., 2022b).
4. Iterate scenarios: From those issues, decisions and critical uncertainties the research team built draft plausible future scenario narratives (Cronan et al., 2022b) that explored the variation around the issues, decisions and critical uncertainties (Table 2).
5. Solutions suite per each scenario: Over the course of the following 2 years, those six scenarios were co-developed with the SAG who named, critiqued, refined and improved each scenario and identified solutions to address issues raised by each scenario Figure 2.
6. Iterate scenarios into futures: The scenario narratives were then used to develop scenario representations (also known as alternative futures) by integrating hydrological model outputs, projections of population change, and climate change predictions scaled to the region and tuned to each scenario. This also included geo-planning to map and graphically represent changes in the region (e.g., crops, population growth, housing development).
7. Iterate timing of solutions into scenarios and alternative futures: Timing of solutions was varied in the models to determine impacts of solution implementation at different times. These changes were iterated with and critiqued by the SAG.
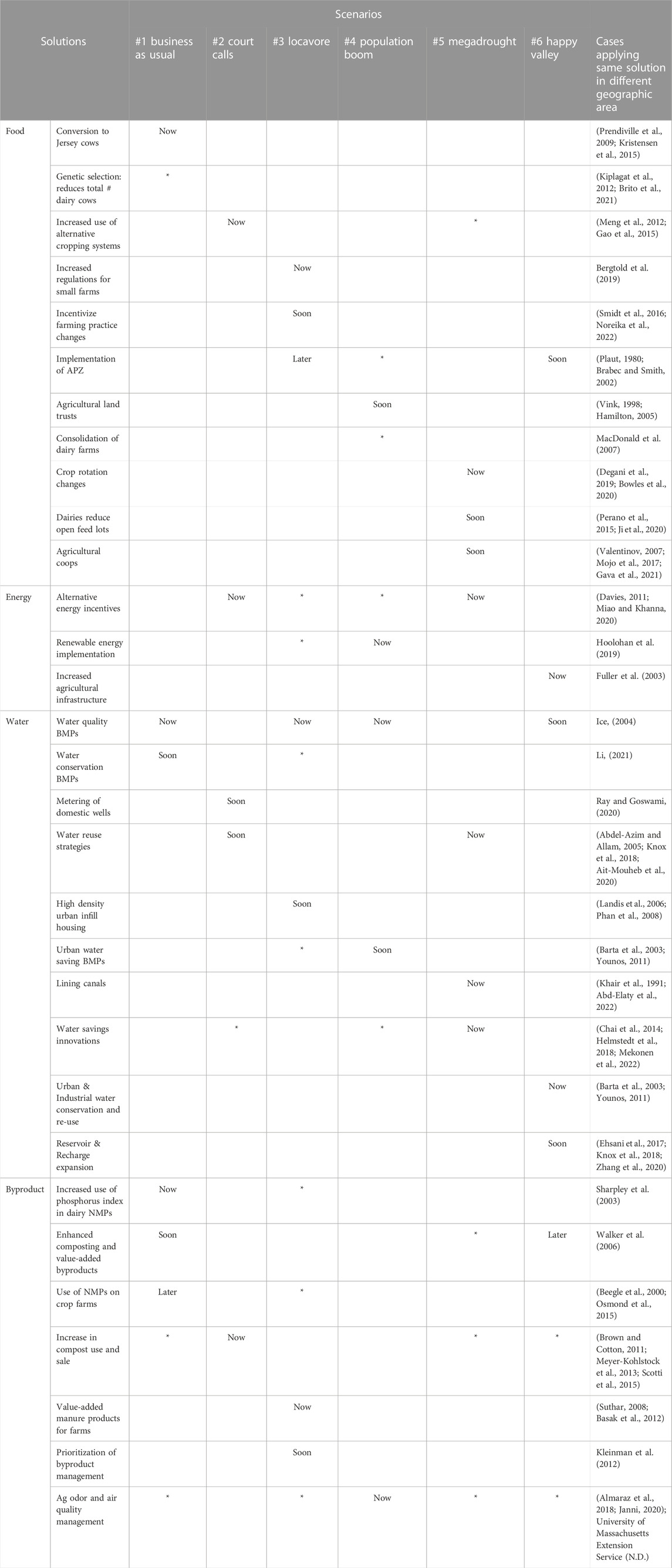
TABLE 2. Co-developed food-energy-water solutions and timing of solutions per scenario for the Magic Valley, Idaho (Key to timing: Now = 2020–2029; Soon = 2030–2039; Later = 2040–2050; * timing not specified).
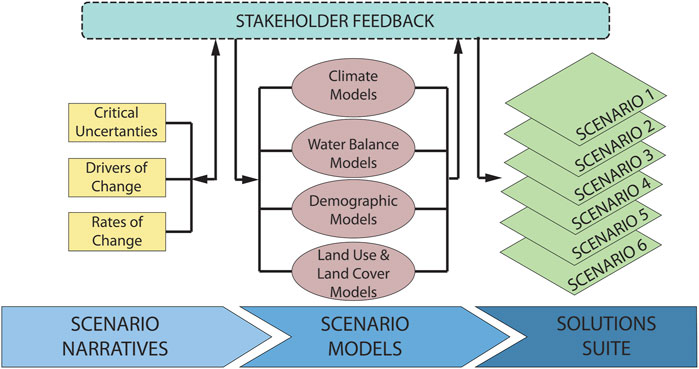
FIGURE 2. Graphic representation of changes in available water and population change and likely solutions and timing of solutions for Happy Valley scenario. Drivers include population change (in beige) and changes in average water availability (blue histograms). “Model agreement” refers to the average of multiple climate model runs, “model uncertainty” indicates the variability between those model runs, and “model with solutions” indicates the water available once the identified solutions are implemented (see Cronan et al., 2022a for more information).
Stakeholder Advisory Group Development
With the help of our Cooperative Extension Service stakeholder, a rural economic development stakeholder, and a lifelong agricultural stakeholder. We identified other key stakeholders representing interests related to our objectives of developing methods and strategies for reducing use, and increasing reuse, of water, nutrients, and energy in the linked dairy and feed crop sectors of Idaho’s agricultural industry in the Magic Valley (MV). Our stakeholders including a canal company executive, municipal water engineers, a food processor manager, farmer/retired Idaho Power executive, rural planning NGO member, farmer/retired food processor executive, Native American representatives, dairy industry advocates, an organic, and a commercial farmer with a total of 12 members. These regional experts formed our SAG.
Workshops involving the SAG were held twice a year, with email communication and report distribution between meetings. A total of seven workshops were held. Not all stakeholders were able to attend all meetings and we scheduled virtual meetings to follow up with those stakeholders. Our Native American rights holders provided their input primarily through telephone and email contact. A complete description of the challenges and successes of the process of engaging the stakeholders and co-producing knowledge is set out in Kliskey et al. In Process. Stakeholders were offered reimbursement for their travel costs, as well as an honorarium, but only three of our stakeholders accepted the offer. The other stakeholders were happy to participate without compensation. We also ended our in-person workshops with a complimentary dinner at a local restaurant for the entire team (researchers and stakeholders).
Process of solution development
Solution development followed aspects of both participatory scenario processes and transdisciplinary research (Kliskey et al. In Process; Meadow et al., 2015), reflecting aspects of both consultative and collaborative types of engagement and the participatory integrated assessment as described by Meadow et al. (2015). We describe our process in detail below. Solutions were introduced and continuously critiqued and modified by the SAG starting at the May 2019 (fourth) workshop and continuing through the April 2021 meeting (seventh workshop).
Initial identification and expansion of suite of solutions
As future scenarios were modified by the SAG they often informally discussed potential solutions to problems presented by the different scenarios and researchers compiled a list of those solutions from meeting notes. Researchers were organized into teams based on expertise and supplemented the list of solutions compiled from SAG comments. As a few examples, the hydrological team brainstormed hydrological solutions, for example, increased dam capacity, building more dams, lining of irrigation canals and increased aquifer recharge during months that irrigation was not taking place. The water policy team suggested changing Idaho regulations restricting the timing of irrigation where canals would contribute to aquifer recharge, metering of domestic wells, incentives for xeriscaping, and for reducing water use. The water quality team recommended increased composting of manures, incentives to distribute manure and slurry from holding ponds greater distances, use of cover crops, and incentives for best management practices (BMPs) for water quality.
A nutrient and waste reduction team identified a set of technological and best management practices (BMP) solutions focused on sustainability with on farm operations and animal behavior and health. The team compiled solutions that are already in use, others that are ready for use but not yet adopted in the area, and others that are in their initial stage of adoption or application and could be available within the time-horizon of the study. Sources of material to evaluate the applicability of solutions to this study included conversations with SAG members and local academics from the University of Idaho Extension, USDA Agricultural Research Service (ARS), and USDA-NRCS. Internet and bibliographic searches were also conducted to identify other potential solutions.
A total of 119 solutions were generated (see Supplemental Materials). A solutions team that included a core group of researchers and our extension service researcher/SAG member met and assigned likely solutions to each scenario. The solutions team undertook this to reduce the amount of time and effort for the SAG. Also, some stakeholders did not have the expertise to propose solutions across all sets of issues and uncertainties.
Iterating solutions with stakeholders
Draft scenarios with a limited set of solutions targeted to each scenario were introduced to the SAG in May of 2019. The primary focus of this workshop was narrative development where we asked stakeholders to check the internal consistency of each scenario. For example, would population increase in a megadrought as had been the past trend in this region? Would water quality regulations be relaxed if there was more water? The SAG applied their expert knowledge of the interacting FEW and waste systems to ensure each narrative included logical and plausible interacting issues and potential solutions. Although the SAG rejected a number of solutions proposed by the research team, and added others that they believed were more likely to be adopted, solutions were not the main focus of that particular workshop.
The second iteration (in November 2019) focused on identifying drivers of change in each scenario, selecting appropriate solutions for each scenario, and again assuring that scenario assumptions were consistent and logical. The SAG applied their expert analysis of whether a solution was practical, potential drawbacks of the solution, likelihood of adoption, and potential timing of adoption depending on the conditions of each scenario. In this iteration, viable solutions were the focus of the discussion. Specifying solutions and clarifying the meaning of solutions (e.g., specifying which BMPs, clarifying by-product management strategies) was the focus of the meeting. Changes in population, dairy cow numbers, residential and industrial development, applicable to each scenario were also discussed and refined. The focus of the workshop was on solutions; several were eliminated (e.g., adoption of biodigesters except by the largest dairies) and more were added, including land trusts to prevent agricultural land from being developed, and establishment of agricultural and dairy coops to reduce costs of by-product management, and to reduce costs of storage and transportation of both agricultural products and by-products.
At the conclusion of the workshop, solutions that impacted land use and land cover as well as those that impacted hydrology were incorporated into water balance and demographic models to determine likely consequences of solution adoption and what the impact of earlier or later adoption might be. Using the demographic model and stakeholder input, we modeled where population increase was likely to occur, and where residential and commercial/industrial development might occur if protections for agriculture were, or were not, in place. In most of the scenarios, stakeholders commented that our population predictions were either too high or too low. Our hydrologic model helped stakeholders understand when the Swan Falls Agreement might be violated if given solutions were adopted earlier or later, and differences in water use of different selections of crops.
The interplay between water balance modeling and stakeholder input occurred at multiple steps of the engagement process. Interested stakeholders engaged with our water balance modeling research team to discuss parameters and potential outputs for the model. All stakeholders gave input that inspired modifications to the inputs to the hydrologic model. As one example, stakeholders commented that the “Megadrought” scenario did not adequately represent the severity of a potential drought which motivated the team to model conditions of the Dust Bowl. The joint stakeholder-researcher team proposed three products from the WBM; model agreement, model uncertainty, and model with solutions. Model agreement (Figures 3, 4) indicates the average water that would be available under multiple climate model runs, similar to how global circulation models are presented for future climates. Model uncertainty (Figures 3, 4) represents the variability between those multiple model runs, which was primarily used by the modeling team, although it was also presented to and discussed with the SAG. Model with solutions indicates the water that would be available once the identified solutions were implemented (see Cronan et al., 2022a for more information). The change in water availability became a critical element of our stakeholder engagement because it a) validated stakeholder feedback in the modeling process, b) provided a check of model sensitivity to the proposed solutions and c) provided tangible examples of how effective the proposed solutions might be on conserving water. This metric was examined extensively by the SAG, across each of the six scenarios, and in some cases led to a revision of model parameters or scenario elements.
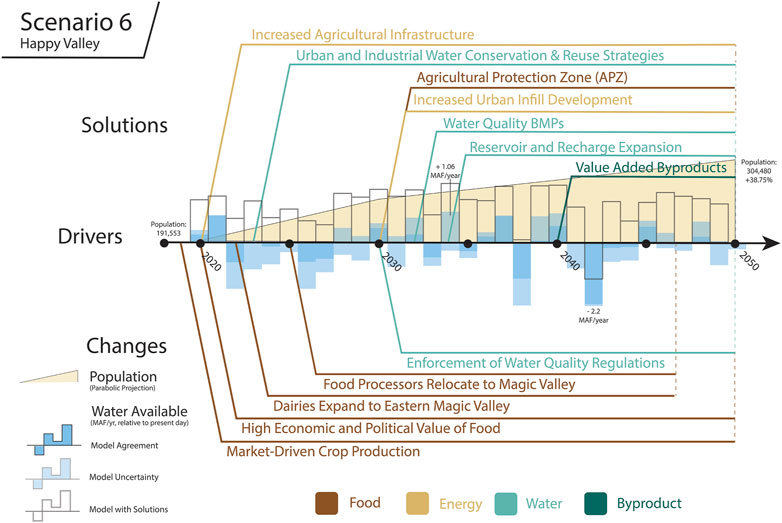
FIGURE 3. Graphic representation of changes in available water and population, likely solutions and timing of solutions for Megadrought scenario. Drivers include population change (in beige) and changes in average water availability (blue histograms). “Model agreement” refers to the average of multiple climate model runs, “model uncertainty” indicates the variability between those model runs, and “model with solutions” indicates the water available once the identified solutions are implemented (see Cronan et al., 2022b for more information).
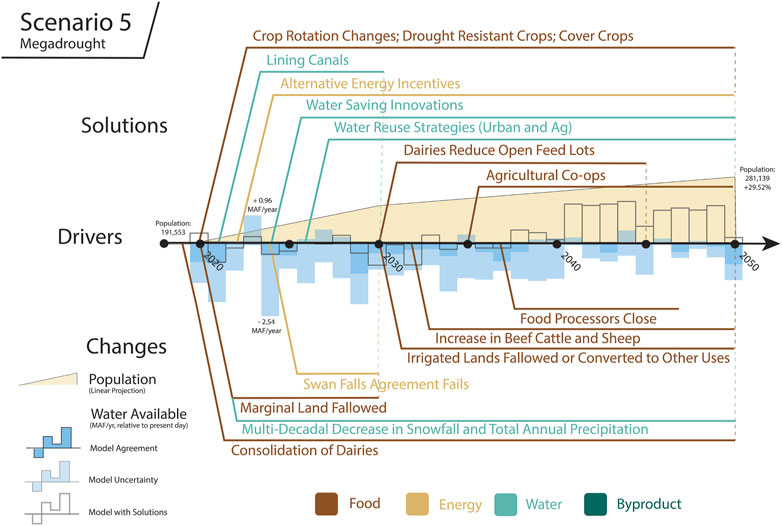
FIGURE 4. Representation of the solution development process from scenario narratives, to coupled biophysical modeling, to development of solutions and modeling the impacts of solutions. “Model agreement” refers to the average of multiple climate model runs, “model uncertainty“ indicates the variability between those model runs, and “model with solutions” indicates the water available once the identified solutions are implemented (see Cronan et al., 2022b for more information). For more details on the coupling of models, see Cronan et al., 2022b. For more on the coproduction process see Kliskey et al. (In review).
The solutions suite incorporated into the models, and model outputs were presented to the SAG, revised based on their input, and iterated again with the SAG during the sixth and seventh SAG workshops in 2020. The seventh workshop focused primarily on fine-tuning the timing of solution adoption with additional discussion of the consequences of either early or late adoption (Figure 4).
Results
Co-developed food-energy-water solutions
Of the six scenarios co-developed with the stakeholders, the best agricultural future envisioned was labeled “Happy Valley” and the worst was named “Megadrought”. We use these two scenarios as book-end illustrations of the results of the stakeholder-driven process described above, and to highlight the interactions among stressors. Solutions developed in these two scenarios provide an illustration of the wide range of possible futures and solutions to issues presented in those futures (Table 1). Not surprisingly, fewer solutions were needed in the Happy Valley scenario as compared to the Megadrought scenario. Three other scenarios envisioned futures with less extreme (favorable or unfavorable) climate and social conditions than Happy Valley and Megadrought, while the first scenario, Business as Usual, anticipated continuation of current trends and provided a baseline to which the other scenarios could be compared.
Modeled changes and co-developed solutions for the Happy Valley scenario
The Happy Valley scenario anticipated low drought conditions, an increase in both food production and in aquifer recharge. The scenario posited that sustainable urban development would be achieved (urban infill and increased urban population density), relieving pressure to convert agricultural land to residential and commercial property. Stakeholders expressed both hope and skepticism that this scenario was realistic - they commented that in this ideal scenario, not many solutions were needed to adapt to the future. Since agricultural production would be high, stakeholders noted that increased infrastructure to store and transport agricultural products would be needed as a solution early in time - 2020 rather than 2025 (Table 1; Figure 4). Stakeholders agreed that an agricultural protection zone was a realistic, or even necessary solution to create this circumstance, and commented that since people would be motivated to move to the area, a solution to population expansion should be added (Table 1; Figure 4). They suggested urban infill would need to be adopted to protect agricultural land. Stakeholders disagreed with researcher assumptions that water quality BMPs might be relaxed in support of higher agricultural production, stating that they would increase or remain unchanged. Stakeholders agreed that reservoir capacity would be increased, and added that aquifer recharge was likely to increase as well. Industrial and dairy byproducts and wastes were anticipated to be converted to value-added products (Figure 4).
Modeled changes and co-developed solutions for the Megadrought scenario
In contrast, the Megadrought scenario anticipated an extended drought and hydrologic conditions similar to those experienced during the Dust Bowl were modeled over the 30-year time frame. Changes included a multi-decadal reduction in snow fall and precipitation, high energy costs because hydroelectric power would not be expected to meet demand, farmers would be forced to fallow land and agricultural land would be sold for other uses. Stakeholders anticipated that the first solution would be modification of crop rotations, adoption of both drought resistant varieties and crops that require less water, and use of cover crops (Table 1; Figure 2). Within a year or two of that response, stakeholders anticipated that irrigation canals would be lined and covered to increase the amount of water reaching crops. Stakeholders acknowledged that this solution would have the effect of reducing recharge of the aquifer and would exacerbate the tensions between holders of surface water and holders of groundwater rights. Additional solutions included adoption of alternative energy incentives in response to the decreased availability of hydropower; water saving innovations would include industrial, urban, and agricultural water reuse strategies; and dairies would reduce open feedlots in favor of cross-ventilated barns in an effort to address reduced milk production due to heat stress (Table 1; Figure 2). Approximately 15 years into this scenario stakeholders anticipated that agricultural co-ops would become more common to support surviving agriculture.
Discussion
Solutions selected by the SAG were directly relevant to the stressors posited in each of the scenarios. In scenarios where water scarcity was an issue, water saving solutions were selected and when population pressures were a stressor, solutions to accommodating more people were selected. Solutions proposed in each scenario were the same or similar when stressors aligned (Table 4). For example, implementation of agricultural protection zones was proposed as a solution in four of the scenarios and was motivated by pressures to convert agricultural land to residential or commercial. Installation of anaerobic digesters, water quality BMPs and increase in compost use and sale were proposed in four scenarios in response to water quality stressors. Other solutions were unique to the scenario in which they were proposed. Lining canals, as one example, was envisioned to occur only in the most extreme and long-term drought conditions, and high-density urban infill was only proposed in one scenario as a response to dual stressors of increased population and increased importance of agricultural production. Forty-one solutions were selected by the SAG and of those, 18 were common to one or more other scenarios and 22 were unique to one scenario.
Since solutions are responsive to stressors, all of the solutions selected by the SAG have been applied or proposed as solutions to similar stressors in different geographic areas (Table 1). In fact, of the total 39 solutions listed, all have been applied in other geographic areas; none are unique to the MV region.
The variety of scenarios provided stakeholders with an opportunity to think about solutions across a wide range of potential futures (Table 1). Including visualization tools, such as mapping where population would increase and where agricultural land might be converted to other uses, fallowed, or transitioned to other crops enhanced the ability of stakeholders to anticipate the location of likely changes. The full process of scenario development, modeling, and using GIS to map change enhanced visualization of regional climate change and impacts, and allowed the SAG to develop solutions that were context- and place-based. Stakeholders anticipated economic pressures, likelihood of adoption and whether the solution would be accepted in the community when evaluating solutions and determining when they might occur. One example of their analysis was their rejection of the addition of any new reservoirs because of the expense and resistance, but acceptance of increasing the capacity of existing reservoirs as a more realistic alternative. An additional example was that they did not agree that biodigesters would ever be adopted by any but the largest dairies because of past economic failures of this technology in smaller dairies in the MV - contrary to the wide-spread adoption of small-scale anerobic digestors in Western Europe (De Dobbelaere et al., 2015).
Solutions proposed by a SAG will likely vary based on the roles each member plays in the community as well as their personal values. In this project, the SAG was weighted toward traditional agricultural and dairy practices. One of our SAG members consistently advocated for more progressive solutions to stressors, but few of those suggestions were incorporated into scenarios by the SAG. If the SAG had been weighted as more progressive leaning, solutions proposed would likely have differed.
The co-production process applied in this study strengthens the connections between scenario narratives, as stories, and representation and impact models (Kok et al., 2015), and enhances the effectiveness of scenario development via the co-design and co-development process (McBride et al., 2017). The outcomes from the approach also demonstrate the expansion of climate, hydrological, and land use projections for environmental planning (Kliskey et al., 2019) through the identification of plausible solutions and the relative timing of those solutions.
Visualizations of the scenarios and solutions are available as a digital atlas for policymakers and for the general public (CRC - Center for Resilient Communities, 2020) and the research team and stakeholders reached out to legislators and others with information about the project and access to the digital atlas as a tool for decision-making. Also, one of our stakeholders was a water manager who could discuss and distribute results to other water managers.
Conclusion
We have described a step-wise process we used to co-produce solutions for the Magic Valley, Idaho that can be used by others as a template for producing solutions in their region. Our process illustrated to stakeholders the impact on water and land-use of implementing solutions at different time periods. Tailoring solutions to a suite of plausible future scenarios allows stakeholders of a region to envision a range of possible future changes to their region and to plan solutions for each of the potential futures. In this paper, these solutions were modeled to determine whether there were potential unintended consequences of adopting solutions and advantages or disadvantages to adopting solutions at different times. The scenarios were key to focusing stakeholders on potential changes in the future and from those, stakeholders envisioned how they could adapt to a range of different climate futures. The visualization of futures was enhanced by hydrological modeling to illustrate changes in surface and ground water availability, population modeling, and mapping of the likely location of changes in residential expansion, industrial development, and in agricultural expansion or contraction and types of crops grown. Solutions were tied to impacts and drivers of change in each scenario. Proactively envisaging solutions allowed the SAG to develop possible means of adapting to a range of scenarios, enhancing their ability to respond to future stressors.
Throughout the process of developing solutions, the modeling outcomes, scenarios and solutions were discussed with stakeholders at our workshops and critiques and recommendations by stakeholders were incorporated into changes in models, scenarios and solutions. Maintaining agricultural livelihoods, water quantity and water quality were of high concern to stakeholders in the Magic Valley. The Swan Falls Agreement was the indicator of water quantity. Violation of the agreement could lead to cascading effects that would have serious consequences to agriculture in the region and avoidance of long-term violation was a primary concern for the stakeholders. Although the solutions that were developed in the paper are context specific (e.g., only large dairy producers will adopt biodigesters to process dairy by-product), selected solutions have been adopted in other regions around the world. Non-etheless, different regions will have place-specific (and stakeholder perspective-specific) needs that create unique solutions to emerging FEWS problems.
Identification of solutions rarely results in implementation of those solutions. As we have experienced, informing politicians and other policymakers of a suite of solutions and the consequences of delaying adoption of solutions also does not necessarily lead to implementation. Future research focus will include engaging stakeholders, politicians and policymakers in identification and co-development of strategies and plans for implementation of solutions. It is also important to carefully consider stakeholders that are invited to be part of the co-development process. These decisions should be made at the inception of a project. The suite of solutions identified by stakeholders are heavily dependent on their world-views and life experiences and this should be taken into account when selecting stakeholders. A different set of stakeholders would likely result in a different mix and/or prioritization of the suite of solutions developed to address FEWS challenges in the region, but this diversity of stakeholders priorities is critical to finding creative solutions.
Data availability statement
The original contributions presented in the study are included in the article, in the Supplementary Material, and at https://the-infews-atlasmagic-valley-idaho-idaho.hub.arcgis.com/pages/Results. Further inquiries can be directed to the corresponding author.
Ethics statement
The studies involving human participants were reviewed and approved by University of Idaho Institutional Review Board. The patients/participants provided their written informed consent to participate in this study.
Author contributions
Conceptualization, AK, ET, and PW; methodology, AK, ET, DC, and PW; formal analysis, DC, PW, AK, JW, and MD; writing preparation, PW, AK, and JW, writing—review and editing, JT, DC, PW, MD, and AK; visualization, AK; supervision, DC, AK, MD, and PW; project administration, AK, DC, and PW; funding acquisition, AK; All authors have read and agreed to the published version of the manuscript.
Funding
This research was funded by the National Science Foundation: grant number SES-1639524 through the Innovations at the Nexus of Food, Energy, and Water Systems program; grant number OIA-1757324 through the NSF Idaho ESPSCoR Program, and; BCS-1856059 through the EngageINFEWS Research Coordination Network. Publication of this article was partially funded by the University of Idaho Open Access Publishing Fund.
Conflict of interest
Author JW is employed by Glanbia Nutritionals.
The remaining authors declare that the research was conducted in the absence of any commercial or financial relationships that could be construed as a potential conflict of interest.
Publisher’s note
All claims expressed in this article are solely those of the authors and do not necessarily represent those of their affiliated organizations, or those of the publisher, the editors and the reviewers. Any product that may be evaluated in this article, or claim that may be made by its manufacturer, is not guaranteed or endorsed by the publisher.
Supplementary material
The Supplementary Material for this article can be found online at: https://www.frontiersin.org/articles/10.3389/fenvs.2023.1055547/full#supplementary-material
Footnotes
1Neither surveys nor questionnaires were used in this paper.
References
Abatzoglou, J. T., Rupp, D. E., and Mote, P. W. (2014). Seasonal climate variability and change in the Pacific Northwest of the United States. J. Clim. 27, 2125–2142. doi:10.1175/JCLI-D-13-00218.1
Abd-Elaty, I., Pugliese, L., Bali, K. M., Grismer, M. E., and Eltarabily, M. G. (2022). Modelling the impact of lining and covering irrigation canals on underlying groundwater stores in the Nile Delta, Egypt. Hydrol. Process. 36, e14466. doi:10.1002/hyp.14466
Abdel-Azim, R., and Allam, M. N. (2005). “Agricultural drainage water reuse in Egypt: Strategic issues and mitigation measures,” in Non-conventional water use: WASAMED project. Editors A. Hamdy, F. El Gamal, N. Lamaddalena, C. Bogliotti, and R. Guelloubi (Bari: CIHEAM/EU DG Research), 105–117. Available at: http://om.ciheam.org/om/pdf/b53/00800755.pdf.
Ait-Mouheb, N., Mayaux, P.-L., Mateo-Sagasta, J., Hartani, T., and Molle, B. (2020). “Chapter 5 - water reuse: A resource for mediterranean agriculture,” in Water resources in the mediterranean region. Editors M. Zribi, L. Brocca, Y. Tramblay, and F. Molle (Elsevier), 107–136. doi:10.1016/B978-0-12-818086-0.00005-4
Almaraz, M., Bai, E., Wang, C., Trousdell, J., Conley, S., Faloona, I., et al. (2018). Agriculture is a major source of NO x pollution in California. Sci. Adv. 4 (1), eaao3477. doi:10.1126/sciadv.aao3477
Ansell, C. (2012). “Collaborative governance,”. The oxford handbook of governance. Editor D. Levi-Faur (Oxford University Press), 0. doi:10.1093/oxfordhb/9780199560530.013.0035
Ansell, C., and Gash, A. (2008). Collaborative governance in theory and practice. J. Public Adm. Res. Theory 18, 543–571. doi:10.1093/jopart/mum032
Barta, R., Ward, R., Wasksom, R. M., Reagan, M., and Smith, D. (2003). Stretching urban water supplies in Colorado: Strategies for landscape water conservation. Fort Collins, Colorado: Colorado Water Resources Research Institute. Available at: https://mountainscholar.org/handle/10217/661 (Accessed September 22, 2022).
Basak, B. B., Biswas, D. R., and Rattan, R. K. (2012). Comparative effectiveness of value-added manures on crop productivity, soil mineral nitrogen and soil carbon pools under maize-wheat cropping system in an Inceptisol. J. Indian Soc. Soil Sci. 60 (4), 288–298.
Beegle, D. B., Carton, O. T., and Bailey, J. S. (2000). Nutrient management planning: Justification, theory, practice. J. Environ. Qual. 29, 72–79. doi:10.2134/jeq2000.00472425002900010009x
Bergtold, J. S., Ramsey, S., Maddy, L., and Williams, J. R. (2019). A review of economic considerations for cover crops as a conservation practice. Renew. Agric. Food Syst. 34, 62–76. doi:10.1017/S1742170517000278
Bowles, T. M., Mooshammer, M., Socolar, Y., Calderón, F., Cavigelli, M. A., Culman, S. W., et al. (2020). Long-term evidence shows that crop-rotation diversification increases agricultural resilience to adverse growing conditions in North America. One Earth 2 (3), 284–293. doi:10.1016/j.oneear.2020.02.007
Brabec, E., and Smith, C. (2002). Agricultural land fragmentation: The spatial effects of three land protection strategies in the eastern United States. Landsc. Urban Plan. 58, 255–268. doi:10.1016/S0169-2046(01)00225-0
Brito, L. F., Bédère, N., Douhard, F., Oliveira, H. R., Arnal, M., Peñagaricano, F., et al. (2021). Review: Genetic selection of high-yielding dairy cattle toward sustainable farming systems in a rapidly changing world. Animal 15 (1), 100292. doi:10.1016/j.animal.2021.100292
Brown, S., and Cotton, M. (2011). Changes in soil properties and carbon content following compost application: Results of on-farm sampling. Compost Sci. Util. 19, 87–96. doi:10.1080/1065657X.2011.10736983
Brown, S. (2012). The competitive position of the Idaho dairy industry. Agricultural markets and policy (AMAP) group, division of applied social sciences, college of agriculture, food and natural resources (CAFNR). Columbia, Missouri: University of Missouri. Available at: https://amap.missouri.edu/wp-content/uploads/2018/05/idahodairy2012.pdf.
Buchecker, M., Salvini, G., Di Baldassarre, G., Semenzin, E., Maidl, E., and Marcomini, A. (2013). The role of risk perception in making flood risk management more effective. Nat. Hazards Earth Syst. Sci. 13, 3013–3030. doi:10.5194/nhess-13-3013-2013
Chai, Q., Gan, Y., Turner, N. C., Zhang, R. Z., Yang, C., Niu, Y., et al. (2014). Water-saving innovations in Chinese agriculture. Adv. Agron. 126, 149–201. doi:10.1016/B978-0-12-800132-5.00002-X
CRC (Center for Resilient Communities) (2020). Magic Valley futures atlas for innovations at the Nexus of food, energy. Moscow, ID, USA: University of Idaho. URL: https://the-infews-atlasmagic-valley-idaho-alternative-futures-uidaho.hub.arcgis.com/pages/Results.
Cronan, D., Trammell, E. J., and Kliskey, A. (2022a). Images to evoke decision-making: Building compelling representations for stakeholder-driven futures. Sustainability 14, 2980. doi:10.3390/su14052980
Cronan, D., Trammell, E. J., Kliskey, A., Williams, P., and Alessa, L. (2022b). Socio-ecological futures: Embedded solutions for stakeholder-driven alternative futures. Sustainability 14, 3732. doi:10.3390/su14073732
DasGupta, R., Hashimoto, S., Okuro, T., and Basu, M. (2019). Scenario-based land change modelling in the Indian Sundarban delta: An exploratory analysis of plausible alternative regional futures. Sustain. Sci. 14, 221–240. doi:10.1007/s11625-018-0642-6
Davies, L. L. (2011). Incentivizing renewable energy deployment: Renewable portfolio standards and feed-in tariffs. KLRI J. Law Legislation 1.
De Dobbelaere, A., De Keulenaere, B., De Mey, J., Lebuf, V., Meers, E., Ryckaert, B., et al. (2015). Small-scale anaerobic digestion: Case studies in western Europe. Rumbeke, Belgium: Inagro.
Degani, E., Leigh, S. G., Barber, H. M., Jones, H. E., Lukac, M., Sutton, P., et al. (2019). Crop rotations in a climate change scenario: Short-term effects of crop diversity on resilience and ecosystem service provision under drought. Agric. Ecosyst. Environ. 285, 106625. doi:10.1016/j.agee.2019.106625
Ehsani, N., Vörösmarty, C. J., Fekete, B. M., and Stakhiv, E. Z. (2017). Reservoir operations under climate change: Storage capacity options to mitigate risk. J. Hydrology 555, 435–446. doi:10.1016/j.jhydrol.2017.09.008
Emerson, K., Nabatchi, T., and Balogh, S. (2012). An integrative framework for collaborative governance. J. Public Adm. Res. Theory 22, 1–29. doi:10.1093/jopart/mur011
Flynn, M., Ford, J. D., Pearce, T., and Harper, S. L. (2018). Participatory scenario planning and climate change impacts, adaptation and vulnerability research in the Arctic. Environ. Sci. Policy 79, 45–53. doi:10.1016/j.envsci.2017.10.012
Fuller, S., Yu, T.-H., Fellin, L., Lalor, A., and Krajewski, R. (2003). Transportation developments in South America and their effect on international agricultural competitiveness. Transp. Res. Rec. 1820, 62–68. doi:10.3141/1820-08
Gao, B., Ju, X., Meng, Q., Cui, Z., Christie, P., Chen, X., et al. (2015). The impact of alternative cropping systems on global warming potential, grain yield and groundwater use. Agric. Ecosyst. Environ. 203, 46–54. doi:10.1016/j.agee.2015.01.020
Gava, O., Ardakani, Z., Delalić, A., Azzi, N., and Bartolini, F. (2021). Agricultural cooperatives contributing to the alleviation of rural poverty. The case of Konjic (Bosnia and Herzegovina). J. Rural Stud. 82, 328–339. doi:10.1016/j.jrurstud.2021.01.034
Hagemann, N., van der Zanden, E. H., Willaarts, B. A., Holzkämper, A., Volk, M., Rutz, C., et al. (2020). Bringing the sharing-sparing debate down to the ground—lessons learnt for participatory scenario development. Land Use Policy 91, 104262. doi:10.1016/j.landusepol.2019.104262
Hamilton, L. B. (2005). Agricultural land trusts: Preserving small farm heritage. Winnipeg, Manitoba, Canada: Canadian Centre Policy Alternatives.
Hassenforder, E., Smajgl, A., and Ward, J. (2015). Towards understanding participatory processes: Framework, application and results. J. Environ. Manag. 157, 84–95. doi:10.1016/j.jenvman.2015.04.012
Helmstedt, K. J., Stokes-Draut, J. R., Larsen, A. E., and Potts, M. D. (2018). Innovating at the food, water, and energy interface. J. Environ. Manag. 209, 17–22. doi:10.1016/j.jenvman.2017.12.026
Hines, S., Packham, J., Wilmore, C., and Taylore, G. (2013). Contribution of agribusiness to the Magic Valley economy, 2013. Moscow, Idaho: University of Idaho Extension. Available at: https://www.extension.uidaho.edu/publishing/html/BUL916-Contribution-of-Agribusiness-to-the-Magic-Valley-Economy.aspx?title=Search&category1=Search&category2=NULL.
Hoolohan, C., Soutar, I., Suckling, J., Druckman, A., Larkin, A., and McLachlan, C. (2019). Stepping-up innovations in the water–energy–food nexus: A case study of anaerobic digestion in the UK. Geogr. J. 185, 391–405. doi:10.1111/geoj.12259
Hristov, A. N., Hazen, W., and Ellsworth, J. W. (2006). Efficiency of use of imported nitrogen, phosphorus, and potassium and potential for reducing phosphorus imports on Idaho dairy farms. J. Dairy Sci. 89, 3702–3712. doi:10.3168/jds.S0022-0302(06)72411-0
Huxham, C., Vangen, S., Huxham, C., and Eden, C. (2000). The challenge of collaborative governance. Public Manag. Int. J. Res. Theory 2, 337–358. doi:10.1080/14719030000000021
Ice, G. (2004). History of innovative best management practice development and its role in addressing water quality limited waterbodies. J. Environ. Eng. 130, 684–689. doi:10.1061/(asce)0733-9372(2004)130:6(684)
Idaho Crops Idaho crops – Idaho state department of agriculture (N.D.). Available at: https://agri.idaho.gov/main/about/about-idaho-agriculture/idaho-crops/ (Accessed February 4, 2020).
Idaho Dairymen’s Association (2019). 2019 industry profile. Available at: https://static1.squarespace.com/static/5980acf9197aea5e857a81eb/t/5d35ff9c7a7496000119757f/1563819956244/2019+Industry+Profile_WEB.pdf (Accessed March 3, 2020).
Izydorczyk, K., Piniewski, M., Krauze, K., Courseau, L., Czyż, P., Giełczewski, M., et al. (2019). The ecohydrological approach, SWAT modelling, and multi-stakeholder engagement – a system solution to diffuse pollution in the Pilica Basin, Poland. J. Environ. Manag. 248, 109329. doi:10.1016/j.jenvman.2019.109329
Janni, K. (2020). Reflections on odor management for animal feeding operations. Atmosphere 11, 453. doi:10.3390/atmos11050453
Ji, B., Banhazi, T., Perano, K., Ghahramani, A., Bowtell, L., Wang, C., et al. (2020). A review of measuring, assessing and mitigating heat stress in dairy cattle. Biosyst. Eng. 199, 4–26. doi:10.1016/j.biosystemseng.2020.07.009
Jones, J. L., and White, D. D. (2022). Understanding barriers to collaborative governance for the food-energy-water nexus: The case of Phoenix, Arizona. Environ. Sci. Policy 127, 111–119. doi:10.1016/j.envsci.2021.10.025
Kabaya, K., Hashimoto, S., Fukuyo, N., Uetake, T., and Takeuchi, K. (2019). Investigating future ecosystem services through participatory scenario building and spatial ecological–economic modelling. Sustain. Sci. 14, 77–88. doi:10.1007/s11625-018-0590-1
Kebede, A. S., Nicholls, R. J., Allan, A., Arto, I., Cazcarro, I., Fernandes, J. A., et al. (2018). Applying the global RCP–SSP–SPA scenario framework at sub-national scale: A multi-scale and participatory scenario approach. Sci. Total Environ. 635, 659–672. doi:10.1016/j.scitotenv.2018.03.368
Khair, A., Nalluri, C., and Kilkenny, W. M. (1991). Soil-cement tiles for lining irrigation canals. Irrig. Drain. Syst. 5, 151–163. doi:10.1007/BF01140519
Kiplagat, S. K., Limo, M. K., and Kosgey, I. S. (2012). Genetic improvement of livestock for milk production. London, UK: IntechOpen. doi:10.5772/50761
Kleinman, P., Blunk, K. S., Bryant, R., Saporito, L., Beegle, D., Czymmek, K., et al. (2012). Managing manure for sustainable livestock production in the Chesapeake Bay Watershed. J. Soil Water Conservation 67, 54A–61A. doi:10.2489/jswc.67.2.54A
Kliskey, A., Abatzoglou, J., Alessa, L., Kolden, C., Hoekema, D., Moore, B., et al. (2019). Planning for Idaho’s waterscapes: A review of historical drivers and outlook for the next 50 years. Environ. Sci. Policy 94, 191–201. doi:10.1016/j.envsci.2019.01.009
Kliskey, A., Williams, P., Griffith, D. L., Dale, V. H., Schelly, C., Marshall, A. M., et al. (2021). Thinking big and thinking small: A conceptual framework for best practices in community and stakeholder engagement in food, energy, and water systems. Sustainability 13, 2160. doi:10.3390/su13042160
Kliskey, A., Williams, P., Trammell, J., Cronan, D., Griffith, D., Alessa, L., et al. Building trust, building futures: Knowledge co-production as relationship, design, and process in transdisciplinary science. Front. Environ. Sci. (In review).
Knox, J. W., Haro-Monteagudo, D., Hess, T. M., and Morris, J. (2018). Identifying trade-offs and reconciling competing demands for water: Integrating agriculture into a robust decision-making framework. Earth’s Future 6, 1457–1470. doi:10.1002/2017EF000741
Kok, K., Bärlund, I., Flörke, M., Holman, I., Gramberger, M., Sendzimir, J., et al. (2015). European participatory scenario development: Strengthening the link between stories and models. Clim. Change 128, 187–200. doi:10.1007/s10584-014-1143-y
Kok, K. (2009). The potential of fuzzy cognitive maps for semi-quantitative scenario development, with an example from Brazil. Glob. Environ. Change 19, 122–133. doi:10.1016/j.gloenvcha.2008.08.003
Kristensen, T., Jensen, C., Østergaard, S., Weisbjerg, M. R., Aaes, O., and Nielsen, N. I. (2015). Feeding, production, and efficiency of Holstein-Friesian, Jersey, and mixed-breed lactating dairy cows in commercial Danish herds. J. Dairy Sci. 98, 263–274. doi:10.3168/jds.2014-8532
Landis, J. D., Hood, H., Li, G., Rogers, T., and Warren, C. (2006). The future of infill housing in California: Opportunities, potential, and feasibility. Hous. Policy Debate 17, 681–725. doi:10.1080/10511482.2006.9521587
Leytem, A. B., Dungan, R. S., Bjorneberg, D. L., and Koehn, A. C. (2013). Greenhouse gas and ammonia emissions from an open-freestall dairy in Southern Idaho. J. Environ. Qual. 42, 10–20. doi:10.2134/jeq2012.0106
Leytem, A. B., Williams, P., Zuidema, S., Martinez, A., Chong, Y. L., Vincent, A., et al. (2021). Cycling phosphorus and nitrogen through cropping systems in an intensive dairy production region. Agronomy 11, 1005. doi:10.3390/agronomy11051005
Li, S. (2021). Landscape integrated soil and water conservation (LISWC) system for sloping landscapes in atlantic Canada. Agriculture 11, 427. doi:10.3390/agriculture11050427
Luz, F. (2000). Participatory landscape ecology – a basis for acceptance and implementation. Landsc. Urban Plan. 50, 157–166. doi:10.1016/S0169-2046(00)00087-6
MacDonald, J. M., McBride, W. D., O'Donoghue, E., Nehring, R. F., Sandretto, C., and Mosheim, R. (2007). Profits, costs, and the changing structure of dairy farming. USDA-ERS Economic Research. Report No. 47. Accessed September 1, 2007. doi:10.2139/ssrn.1084458
McBride, M. F., Lambert, K. F., Huff, E. S., Theoharides, K. A., Field, P., and Thompson, J. R. (2017). Increasing the effectiveness of participatory scenario development through codesign. Ecol. Soc. 22, art16. art16. doi:10.5751/ES-09386-220316
Meadow, A. M., Ferguson, D. B., Guido, Z., Horangic, A., Owen, G., and Wall, T. (2015). Moving toward the deliberate coproduction of climate science knowledge. Weather, Clim. Soc. 7 (2), 179–191. doi:10.1175/WCAS-D-14-00050.1
Mekonen, B. M., Moges, M. F., and Gelagl, D. B. (2022). Innovative irrigation water-saving strategies to improve water and yield productivity of onions. Int. J. Res. Agric. Sci. 9 (1), 37–2022.
Meng, Q., Sun, Q., Chen, X., Cui, Z., Yue, S., Zhang, F., et al. (2012). Alternative cropping systems for sustainable water and nitrogen use in the North China Plain. Agric. Ecosyst. Environ. 146 (1), 93–102. doi:10.1016/j.agee.2011.10.015
Meyer-Kohlstock, D., Hädrich, G., Bidlingmaier, W., and Kraft, E. (2013). The value of composting in Germany – economy, ecology, and legislation. Waste Manag. 33, 536–539. doi:10.1016/j.wasman.2012.08.020
Miao, R., and Khanna, M. (2020). Harnessing advances in agricultural technologies to optimize resource utilization in the food-energy-water nexus. Annu. Rev. Resour. Econ. 12, 65–85. doi:10.1146/annurev-resource-110319-115428
Mojo, D., Degefa, T., and Fischer, C. (2017). The development of agricultural cooperatives in Ethiopia: History and a framework for future trajectory. Ethiop. J. Soc. Sci. Humanit. 13, 49–77. doi:10.4314/ejossah.v13i1.3
Nol, L., Verburg, P. H., and Moors, E. J. (2012). Trends in future N2O emissions due to land use change. J. Environ. Manag. 94, 78–90. doi:10.1016/j.jenvman.2011.06.053
Noreika, N., Li, T., Winterova, J., Krasa, J., and Dostal, T. (2022). The effects of agricultural conservation practices on the small water cycle: From the farm-to the management-scale. Land 11, 683. doi:10.3390/land11050683
Osmond, D. L., Hoag, D. L. K., Luloff, A. E., Meals, D. W., and Neas, K. (2015). Farmers’ use of nutrient management: Lessons from watershed case studies. J. Environ. Qual. 44, 382–390. doi:10.2134/jeq2014.02.0091
Oteros-Rozas, E., Martín-López, B., Daw, T. M., Bohensky, E. L., Butler, J. R. A., Hill, R., et al. (2015). Participatory scenario planning in place-based social-ecological research: Insights and experiences from 23 case studies. Ecol. Soc. 20, art32. art32. doi:10.5751/ES-07985-200432
Palomo, I., Martín-López, B., López-Santiago, C., and Montes, C. (2011). Participatory scenario planning for protected areas management under the ecosystem services framework: The doñana social-ecological system in southwestern Spain. Ecol. Soc. 16, art23. art23. doi:10.5751/ES-03862-160123
Parkison, J. (2021). Government financial planning in a time of uncertainty. Journal of Accountancy. April/May, 34–37. Available at: https://www.journalofaccountancy.com/issues/2021/may/government-financial-planning.html.
Perano, K. M., Usack, J. G., Angenent, L. T., and Gebremedhin, K. G. (2015). Production and physiological responses of heat-stressed lactating dairy cattle to conductive cooling. J. Dairy Sci. 98, 5252–5261. doi:10.3168/jds.2014-8784
Phan, T., Peterson, J., and Chandra, S. (2008). Urban infill: Extent and implications in the city of monash. People Place 16, 23–31. doi:10.3316/informit.536194734920252
Planque, B., Mullon, C., Arneberg, P., Eide, A., Fromentin, J. M., Heymans, J. J., et al. (2019). A participatory scenario method to explore the future of marine social-ecological systems. Fish Fish. 20 (3), 434–451. doi:10.1111/faf.12356
Plaut, T. R. (1980). Urban expansion and the loss of farmland in the United States: Implications for the future. Am. J. Agric. Econ. 62, 537–542. doi:10.2307/1240211
Plieninger, T., Bieling, C., Ohnesorge, B., Schaich, H., Schleyer, C., and Wolff, F. (2013). Exploring futures of ecosystem services in cultural landscapes through participatory scenario development in the Swabian Alb, Germany. Ecol. Soc. 18, art39. art39. doi:10.5751/ES-05802-180339
Prendiville, R., Pierce, K. M., and Buckley, F. (2009). An evaluation of production efficiencies among lactating Holstein-Friesian, Jersey, and Jersey×Holstein-Friesian cows at pasture. J. Dairy Sci. 92, 6176–6185. doi:10.3168/jds.2009-2292
Ray, A., and Goswami, S. (2020). “IoT and cloud computing based smart water metering system,” in Processing of the 2020 International Conference on Power Electronics & IoT Applications in Renewable Energy and its Control (PARC), Mathura, India, February 2020 (IEEE), 308–313. doi:10.1109/PARC49193.2020.236616
Reed, M., Kenter, J., Bonn, A., Broad, K., Burt, T., Fazey, I., et al. (2013). Participatory scenario development for environmental management: A methodological framework illustrated with experience from the UK uplands. J. Environ. Manag. 128, 345–362. doi:10.1016/j.jenvman.2013.05.016
Reinhardt, J., Liersch, S., Abdeladhim, M. A., Diallo, M., Dickens, C., Fournet, S., et al. (2018). Systematic evaluation of scenario assessments supporting sustainable integrated natural resources management: Evidence from four case studies in africa. Ecol. Soc. 23, art5. art5. doi:10.5751/ES-09728-230105
Sanjuan-Delmás, D., Taelman, S. E., Arlati, A., Obersteg, A., Vér, C., Óvári, Á., et al. (2021). Sustainability assessment of organic waste management in three EU Cities: Analysing stakeholder-based solutions. Waste Manag. 132, 44–55. doi:10.1016/j.wasman.2021.07.013
Scotti, R., D'ascoli, R., Gonzalez Caceres, M., Bonanomi, G., Sultana, S., Cozzolino, L., et al. (2015). Combined use of compost and wood scraps to increase carbon stock and improve soil quality in intensive farming systems: Large C:N ratio amendment to improve soil quality. Eur. J. Soil Sci. 66, 463–475. doi:10.1111/ejss.12248
Sharpley, A. N., Weld, J. L., Beegle, D. B., Kleinman, P. J., Gburek, W. J., Moore, P. A., et al. (2003). Development of phosphorus indices for nutrient management planning strategies in the United States. J. Soil Water Conservation 58 (3), 137–152.
Smidt, S. J., Haacker, E. M., Kendall, A. D., Deines, J. M., Pei, L., Cotterman, K. A., et al. (2016). Complex water management in modern agriculture: Trends in the water-energy-food nexus over the High Plains Aquifer. Sci. Total Environ. 566, 988–1001. doi:10.1016/j.scitotenv.2016.05.127
Strong, C. J., and Orr, M. C. (2016). Understanding the 1984 swan falls settlement. Ida. Law Rev. 52, 223.
Suthar, S. (2008). Bioconversion of post harvest crop residues and cattle shed manure into value-added products using earthworm Eudrilus eugeniae Kinberg. Ecol. Eng. 32, 206–214. doi:10.1016/j.ecoleng.2007.11.002
USDA National Agricultural Statistics Service CropScape - NASS CDL program. Available at: https://nassgeodata.gmu.edu/CropScape/ (Accessed February 8, 2020).
USDA National Agricultural Statistics Service (1997a). Milk cow herd size by inventory and sales: 1997. Available at: https://agcensus.library.cornell.edu/wp-content/uploads/1997-Idaho-CHAPTER_1_State_Data-1599-Table-29.pdf (Accessed March 12, 2020).
USDA National Agricultural Statistics Service (1997b). Selected crops harvested: 1997 and 1992. Available at: https://agcensus.library.cornell.edu/wp-content/uploads/1997-Idaho-CHAPTER_2_County_Data-1600-Table-13.pdf (Accessed March 27, 2020).
U.S. Energy Information Administration - EIA - Independent Statistics and Analysis (2022). U.S. Energy information administration - EIA - independent Statistics and analysis. Available at: https://www.eia.gov/state/analysis.php?sid=ID (Accessed April 1, 2022).
Valentinov, V. (2007). Why are cooperatives important in agriculture? An organizational economics perspective. J. Institutional Econ. 3, 55–69. doi:10.1017/S1744137406000555
Villamor, G., Griffith, D., Kliskey, A., and Alessa, L. (2020). Contrasting stakeholder and scientist conceptual models of food-energy-water systems: A case-study in Magic Valley, southern Idaho. Socio-Environmental Syst. Model. 2, 16312. doi:10.18174/sesmo.2020a16312
Vink, E. (1998). Land trusts conserve California farmland. Calif. Agric. 52, 27–31. doi:10.3733/ca.v052n03p27
Volkery, A., Ribeiro, T., Henrichs, T., and Hoogeveen, Y. (2008). Your vision or my model? Lessons from participatory land use scenario development on a European scale. Syst. Pract. Action Res. 21 (6), 459–477. doi:10.1007/s11213-008-9104-x
Walker, P., Williams, D., and Waliczek, T. M. (2006). An analysis of the horticulture industry as a potential value-added market for compost. Compost Sci. Util. 14, 23–31. doi:10.1080/1065657X.2006.10702259
Walz, A., Lardelli, C., Behrendt, H., Grêt-Regamey, A., Lundström, C., Kytzia, S., et al. (2007). Participatory scenario analysis for integrated regional modelling. Landsc. urban Plan. 81 (1-2), 114–131. doi:10.1016/j.landurbplan.2006.11.001
Xexakis, G., and Trutnevyte, E. (2019). Are interactive web-tools for environmental scenario visualization worth the effort? An experimental study on the Swiss electricity supply scenarios 2035. Environ. Model. Softw. 119, 124–134. doi:10.1016/j.envsoft.2019.05.014
Younos, T. (2011). Paradigm shift: Holistic approach for water management in urban environments. Front. Earth Sci. 5, 421–427. doi:10.1007/s11707-011-0209-7
Zhang, H., Xu, Y., and Kanyerere, T. (2020). A review of the managed aquifer recharge: Historical development, current situation and perspectives. Phys. Chem. Earth, Parts A/B/C 118–119, 102887. doi:10.1016/j.pce.2020.102887
Keywords: stakeholder coproduction, participatory scenario planning, modeling, mapping, context-and place-based solutions
Citation: Williams P, Kliskey AA, Cronan D, Trammell EJ, de Haro-Martí ME and Wilson J (2023) Constructing futures, enhancing solutions: Stakeholder-driven scenario development and system modeling for climate-change challenges. Front. Environ. Sci. 11:1055547. doi: 10.3389/fenvs.2023.1055547
Received: 27 September 2022; Accepted: 02 January 2023;
Published: 18 January 2023.
Edited by:
Charles Vörösmarty, CUNY Advanced Science Research Center, United StatesReviewed by:
Hatim M. E. Geli, New Mexico State University, United StatesThomas Webler, Social and Environmental Research Institute (SERI), United States
Copyright © 2023 Williams, Kliskey, Cronan, Trammell, de Haro-Martí and Wilson. This is an open-access article distributed under the terms of the Creative Commons Attribution License (CC BY). The use, distribution or reproduction in other forums is permitted, provided the original author(s) and the copyright owner(s) are credited and that the original publication in this journal is cited, in accordance with accepted academic practice. No use, distribution or reproduction is permitted which does not comply with these terms.
*Correspondence: Paula Williams, cGF1bGF3aWxsaWFtc0B1aWRhaG8uZWR1