Corrigendum: Building trust, building futures: Knowledge as co-production as relationship, design, and process in transdisciplinary science
- 1Center for Resilient Communities, University of Idaho, Moscow, ID, United States
- 2Sustainability, Environmental Science, Southern Oregon University, Ashland, OR, United States
- 3Water Environment Research Center, University of New Hampshire, Durham, NH, United States
- 4University of Idaho Extension, Gooding, ID, United States
- 5Crossroads Concepts and Consulting, Boise, ID, United States
Understanding and modeling the trajectories of change in broad level interactions in food-energy-water systems is incomplete when it is undertaken by researchers in isolation from those who live and work in the systems. For models and outcomes to have validity they need to be subjected to sustained development and iteration with stakeholders. This requires a paradigm shift in our thinking of stakeholder engagement from viewing such engagement as an isolated activity or part of the data collection methods to thinking of engagement as a process of knowledge generation. That process hinges on building relationships and building trust, and also sustaining these as long-term relationships through multiple elements of research design and execution. Using the case-study of a mid-size river basin we demonstrate a co-production of knowledge process for food-energy-water systems. The findings highlight the multiple and different ways in which knowledge co-production can be transacted in food-energy-water systems while also generating solutions to the use and re-use of water, energy, and nutrients at the landscape level.
1 Introduction
The transformation of landscapes in the Anthropocene (Alessa and Chapin, 2008) involves the complex interactions of society’s major food, energy, and water systems (FEWS). Understanding and modeling the trajectories of change in FEWS is imperative for sustainable futures on Earth that balance food production, water availability, and energy needs. Advances in mapping, modeling, and visualization have all contributed to our knowledge and representation of these broad scale systems and how they potentially interact. However, researchers alone cannot produce the knowledge necessary to develop solutions to the vexing problems of sustaining food, energy, and water systems in concert, this requires consideration of the context in which these FEWS interplay with people and communities. As we illustrate in the case study below, community expertise is needed to allow researchers to reassess invalid assumptions and misconceptions about local system interactions and effective solutions (Polk, 2015; Howarth and Monasterolo, 2017; Djenontin and Meadow, 2018). In turn, stakeholder misconceptions can also be revised based on information provided by researchers (Polk, 2015; Howarth and Monasterolo, 2017; Djenontin and Meadow, 2018). The process necessary to navigate this complex interaction among community/stakeholders and researchers in FEWS is the domain of co-production of knowledge.
Co-production of knowledge refers to collaborative approaches that encapsulate socially relevant and scientifically reliable knowledge that is germane to real world solutions (Polk, 2015; Howarth and Monasterolo, 2017; Djenontin and Meadow, 2018). Co-production of knowledge can be viewed within a broader suite of stakeholder engagement efforts. Stakeholder engagement has been characterized as bottom-up, or stakeholder-initiated, versus top-down, or decision-maker initiated (Fraser et al., 2006). Stakeholder engagement can also be distinguished according to the purposes and outcomes that drive engagement and knowledge exchange ranging from communication (one-way), to consultation, to deliberation, to co-production (two-way) (Rowe and Frewer, 2004;Reed, 2008; Reed et al., 2013). Co-production of knowledge can therefore be either researcher-initiated or stakeholder-initiated but must be a two-way deliberative approach between stakeholders and researchers.
Common processes for engaging stakeholders that are similar to those in this case study include participatory stakeholder engagement (PSP) which sometimes involves modeling to simulate scenarios (e.g., Walz et al., 2007; Volkery et al., 2008; Nol et al., 2012; Plieninger et al., 2013; Reed et al., 2013; Hassenforder et al., 2015; Kok et al., 2015; McBride et al., 2017; Kebede et al., 2018; Reinhardt et al., 2018; DasGupta et al., 2019; Izydorczyk et al., 2019; Kabaya et al., 2019; Xexakis and Trutnevyte, 2019; Hagemann et al., 2020; Sanjuan-Delmás et al., 2021)] and sometimes does not (e.g., Kok, 2009; Palomo et al., 2011; Oteros-Rozas et al., 2015; Flynn et al., 2018; Planque et al., 2019). A similar approach is story and simulation (SAS) which also may or may not include modeling and mapping (De Stefano et al., 2017; Elsawah et al., 2020). If publications are representative of practice, SAS has been used much less frequently than PSP and it is rarely iterated with stakeholders over 4–5 years, or used within the FEWS framework.
Stakeholder engagement in FEWS has been categorized according to the type of participation based on the form and function that is pursued (White, 1996; Ghodsvali et al., 2019). The Ghodsvali et al. framework categorizes community and stakeholder engagement as nominal, instrumental, representative or transformative (Ghodsvali et al., 2019), with co-production of knowledge spanning instrumental, representational, and transformative participation for stakeholders.
The International Association for Public Participation (IAP2) has adopted a similar framework with levels of involvement spanning inform, consult, involve, collaborate and empower (Nabatchi, 2012; Brown and Chin, 2013; Bammer, 2019). Table 1 describes the definitions of each type of engagement as defined by Ghodsvali et al. (2019) and by the IAP2.
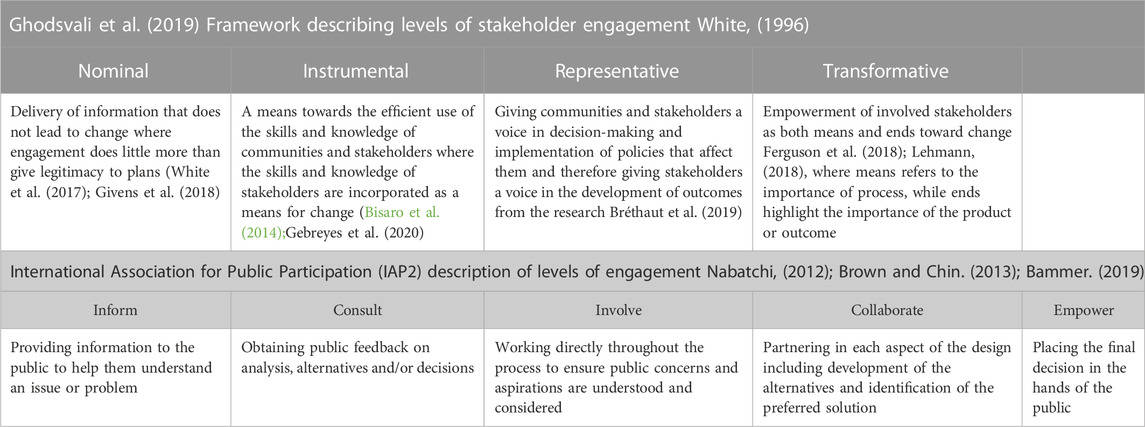
TABLE 1. Range of stakeholder engagement based on the form and function of a project or study using the Ghodsvali et al. (2019) framework and the IAP2 framework. Types of engagement that fit co-production of knowledge are shown in grey.
Stakeholder engagement generally, and co-production of knowledge specifically, utilizes a wide range of methods of interaction with stakeholders, including: interviews (White et al., 2017), questionnaire surveys (Bielicki et al., 2019), focus group discussions (Hoolohan et al., 2018b), participatory mapping (Reilly et al., 2018), structured workshops (Yan and Roggema, 2019), gaming exercises (Mochizuki et al., 2018), and participatory scenario development (Johnson and Karlberg, 2017) and story and simulation (De Stefano et al., 2017; Elsawah et al., 2020). Each method can support different contributions to the understanding of the FEWS nexus (Ghodsvali et al., 2019) and collectively a multi-method approach can support transdisciplinary co-production of knowledge.
An organized, consistent, and (ideally) representative group of stakeholders that can co-design and co-generate knowledge in a sustained manner, that is a stakeholder advisory group (SAG), has the potential to provide multi-method engagement in FEWS research resulting in co-produced knowledge and validated models. Stakeholder advisory groups, research advisory councils, and citizen advisory committees have been used since the 1940s in the Cooperative Extension Service (Kelsey and Hearne, 1949; Franz et al., 2015; Garst and McCawley, 2015), and from the 1970s to support planning studies (Ertet, 1979; Lafon et al., 2004), environmental policy development (Lynn and Busenberg, 1995), forest management (McGurk et al., 2006; Hunt and McFarlane, 2007; Robson and Rosenthal, 2014), phosphorus management (Iwaniec et al., 2016), coastal risk management (Creed et al., 2018), and more recently in FEWS research (Bielicki et al., 2019). The scope of advisory groups has typically been nominal engagement (e.g., (Ertet, 1979; Lynn and Busenberg, 1995; McGurk et al., 2006; Bielicki et al., 2019), or instrumental engagement (e.g., (Lafon et al., 2004; McGurk et al., 2006; Hunt and McFarlane, 2007; Robson and Rosenthal, 2014), with few advisory groups that are set up to provide representative engagement (McGurk et al., 2006; Creed et al., 2018) or transformative engagement (Iwaniec et al., 2016). Most studies that involve advisory groups primarily use interviews or surveys to elicit engagement while few studies adopt multi-method engagement. In some circumstances, nominal or instrumental engagement with communities and stakeholders may be appropriate, depending upon the objectives of the research. If research objectives include empowering communities and stakeholders to envision futures of their communities so that they may institute change or implement solutions, using a multi-methods approach to enrich understanding and enable visualization of potential futures is a necessary level of engagement (Koua et al., 2006; Walker et al., 2008; Jones, 2011; Cronan et al., 2022).
In the context of FEW systems and the FEWS nexus the use of co-production processes have supported qualitative assessments of stakeholder perspectives on water-limited southwestern US urban FEWS systems via focus groups (White et al., 2017); climate shocks on the FEW nexus at the national scale in the United Kingdom, including hazard shocks for FEWS, FEWS infrastructure mitigation, FEWS insurance and finance issues, and governance and FEWS shocks (Howarth & Monasterolo, 2017); documentation of stakeholder knowledge of climate change and food security issues at the FEWS nexus (Hoolohan et al., 2018a); measurement of stakeholder attitudes, perceptions, and values of FEW management issues for corn belt agriculture, fossil fuel and renewable energy productions, and ecological impacts in the US Great Lakes region (Bielicki et al., 2019); to support envisioning, experimenting, and learning purposes toward understanding sustainable development with respect to FEW systems and issues (Ghodsvali et al., 2019), and; to facilitate knowledge exchange between research and non-research actors toward identifying solutions to FEW crises in African cities under rapid population growth (Sesan et al., 2022).
This paper explores the development and application of a SAG as a means for supporting a multi-method process for the co-production of knowledge in FEWS research and addresses the research question: What are the features of a SAG engagement process that can support the co-production of knowledge for developing models and solutions to FEWS issues? This question is addressed using the case-study of agricultural production in the Magic Valley, Idaho, United States (Figure 1).
2 Methods for knowledge Co-production
This study was part of a food-energy-water systems project that was focused on developing integrated solutions to reducing water use, increasing nutrient reuse, and reducing energy at the watershed scale across an agricultural landscape dominated by dairy production (Villamor et al., 2020). The study took place in the Magic Valley, Idaho, United States (Figure 1), which is situated in the Snake River Plain within the Columbia River Basin in Southern Idaho. It is a semi-arid environment receiving approximately 250 mm precipitation annually. The Magic Valley comprises six counties in the Upper Snake River Basin (USRB) between American Falls and Glenns Ferry, much of which is underlain by the East Snake River Plain aquifer, a sizable basalt aquifer. The Magic Valley supports a population of approximately 186,000 people in numerous farming communities, the City of Twin Falls (50,000 inhabitants) is the major urban center. The area is dominated by agricultural production, notably dairy and crop production, and is a significant contributor to Idaho’s agricultural economy, in 2013, Magic Valley farm gate receipts accounted for 47% of Idaho’s total farm gate receipts (Hines et al., 2013).
2.1 The stakeholder advisory group
A preliminary SAG was established during the development of the research proposal to co-develop research questions and guide the formation of the proposal. The 3 members of the preliminary group formed the initial SAG which was expanded to 12 members, using a snowball sampling technique (Hines et al., 2013), once the project was funded. SAG members were chosen for their experience living and working in the Magic Valley, their knowledge in a key sector relevant to FEWS, diversity, and ability to work in a group setting with multiple perspectives. A research team member with 30 years of experience working in Idaho’s agricultural sector, including in the Magic Valley, and a local researcher from the Extension Service with 12 years of service in the area were instrumental in identifying and recruiting advisory group members. The identification of which organizations were significant was determined both from the researchers’ understanding of the system and SAG members’ conceptualization of the FEWS (Villamor et al., 2020). To ensure representation from key organizations that were not included as part of snowball sampling, targeted recruitment was used for food processors, water governance organizations, canal companies, municipal water engineers, and dairy industry groups.
Dialog, collaboration, and co-development of knowledge with the SAG was facilitated using an ongoing, iterative Delphi approach (Alessa et al., 2018) modified to provide in-person discussions throughout the four-year project. This was organized as one-day workshops lasting approximately seven hours every six months from May 2017—May 2020 held in Twin Falls, Idaho. Each workshop started with a brief re-cap of progress to date, stakeholder suggestions for refining the process, revisitation and adjustment (if needed) of assumptions were solicited in each workshop. Upon presentation of new work, stakeholder critique was again sought. Following each workshop a draft set of notes, action items and graphics were sent to SAG members for verification, addition of any critical items missed in the notes, and approval of the material. Each workshop built upon the previous workshop and on some occasions an intermediate virtual meeting was held between workshops if the SAG and research team deemed it appropriate. In some situations, questionnaire surveys, interviews, or focus groups were used to generate specific information or discussions.
Early discussions with the SAG were purposefully wide-ranging with few boundaries or direction and covered topics such as the potential for goat farming vis-a-vis dairy cattle for sustaining the local dairy industry; how cooperatives among smaller family farms could support cost-effective technological solutions such as anaerobic digesters, and; the role of robotic dairy operations in providing greater production efficiencies. This process allowed stakeholders to direct discussions to topics they found most relevant as well as to build trust that researchers would listen and incorporate stakeholder input into products. Gradually, discussions became more focused and tied to an overarching process (Figure 2). The co-development process was multi-faceted and iterative (Figure 2) leading to: development of a conceptual model of the actors, resources, issues and dynamics; identification of critical uncertainties (Shearer et al., 2006; Trammell et al., 2021) and underlying future trajectories; establishment of a set of alternative futures (scenarios); validation and improvement of hydrological, demographic, and agent-based models; identification of solutions for reducing water use, reducing or reusing nutrients, or reducing energy consumption, and; evaluation of mapping and visualization of data and outcomes for FEWS in the Magic Valley. Each of these outcomes were interrelated with one outcome providing an input to another process leading to another outcome through an iterative process (Figure 2) where the SAG input, contributions, and validation was central to the process. This process reflects recent transdisciplinary research design processes (Hoolohan et al., 2018a) by deeply integrating stakeholder knowledge with insights from multiple disciplines. In addition to the schedule of core SAG workshops, stakeholder engagement was supplemented with other approaches including representative questionnaire surveys, focus group discussions with specific constituents, site visits, and individual interviews, to expand on the knowledge and outcomes from the SAG workshops.
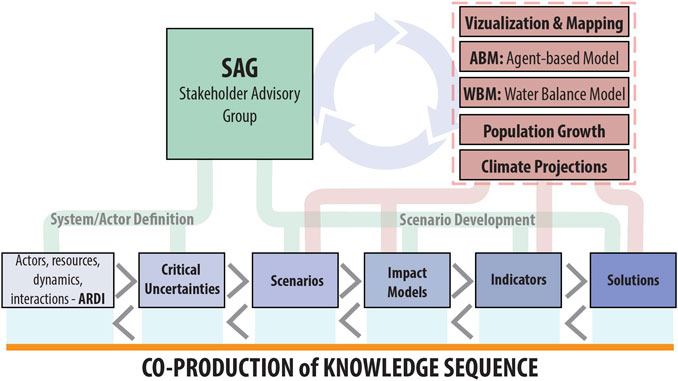
FIGURE 2. Conceptual diagram of stakeholder advisory group role in the process of co-production of knowledge in food-energy-water research.
2.2 Co-production processes
A series of 12 sequential workshops were run with the SAG between May 2017 and April 2021 (Table 2). A broad range of engagement methods were used for co-producing knowledge with the SAG (Figure 2; Table 2). Some engagement methods were iterated over several workshops (e.g., Scenario Narrative development, geospatial scenario mapping, FEWS solutions), and prior workshop outcomes based on one engagement method were frequently discussed in a subsequent workshop to provide context and to establish a basis for a different engagement method. The research purpose of co-production with the SAG for the Magic Valley was to frame a conceptual model of the food-energy-water system as a social-ecological system (SES), identify the pertinent FEWS stakeholder network, generate a set of alternative futures or scenarios for FEWS, develop representation models of the alternative futures, generate descriptive narratives of each scenario, validate and vet impact model outcomes for each scenario, and identify and assess potential integrated solutions to reducing and reusing water, nutrients, and energy for each scenario at the landscape level. The purposes that SAG members had for participating varied depending on each member’s perspective and values. However, common goals for all SAG members included envisioning strategies to preserve agricultural land from development as much as possible, maintain agricultural production in the region, retain as much water in the region as possible, allow market forces to dictate standards and practices rather than government intervention, and avoid long-term violations of the Swan Falls Agreement. The majority of SAG members were keenly aware of the pressures facing the FEW system, the magnitude of those pressures, and that they would be unable to maintain the status quo–for example, with respect to waste management and water quality. Through the end of 2019 workshops were held in person in Twin Falls, Idaho, the urban hub of Magic Valley (Table 2). During 2020 and 2021 workshops were held virtually due to COVID travel restrictions and social distancing (Table 2). Virtual meetings benefited from the relationships that had been developed in person in the preceding workshops. The sequence and timing of workshops characterizes how the utilization of a co-production approach shaped the trajectory of the research–based on the number of workshops that a single activity or phase was interested at and for any single workshop that combination of activities and research phases that were integrated.
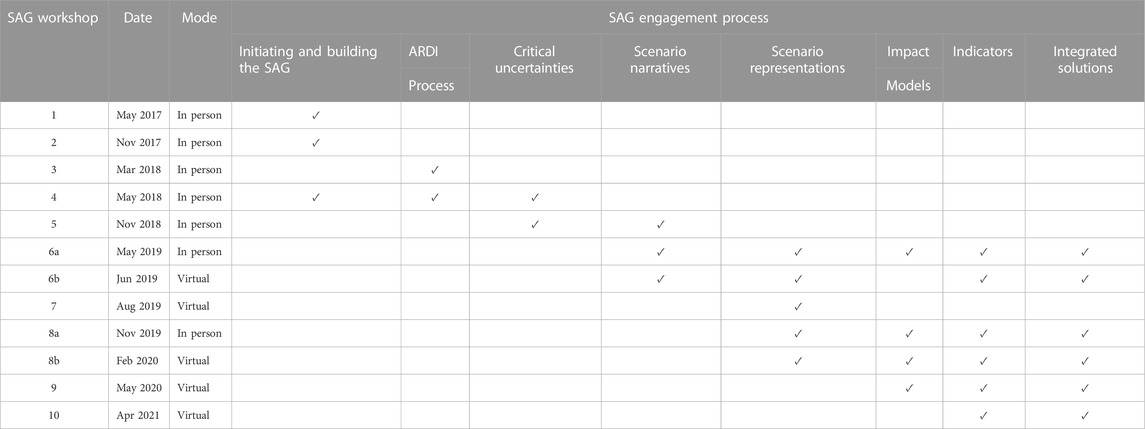
TABLE 2. Stakeholder Advisory Group (SAG) workshop series showing each element of the engagement and co-production process. When workshop participants were not able to attend in person and a virtual workshop was scheduled at a later date to follow up with those who were unable to attend, we have designated the in-person workshop as “a" and the virtual workshop as “b”.
2.2.1 Actors-Resources-Dynamics-Interactions Process
The SAG participated in workshops #3 and #4 for the purpose of co-producing a conceptual model of the FEWS in the Magic Valley. This utilized the ARDI process (Etienne et al., 2011) to identify key direct and indirect actors, identify critical resources relevant to the actors, map the main processes that drive resource dynamics, and construct the interactions among the actors, resources, and dynamics (Villamor et al., 2020). Actors were described as entities who have demonstrated significant influence within the Magic Valley with respect to FEWS. Research team members facilitated open discussion sessions with the SAG to elicit and then work through collective responses on FEWS actors in the Magic Valley, water and nutrient resources, FEWS dynamics, and interactions among these actors and resources. This included ranking each of these elements individually, computing the group ranking, and then engaging in an open discussion to generate a consensus on the final ranking for each element of the ARDI sequence.
The ARDI process produced a ranked list of important actors (organizations, entities, and decision-making bodies) who played major roles in managing and deciding use of surface- and groundwater, and of nutrient and byproduct management. Through this process the SAG also ranked the importance of the main dynamics and processes affecting the state of, and creating change in, water availability and use and nutrient management in the Magic Valley. The outcome of ARDI was co-production of a conceptual model of the FEWS in Magic Valley (Villamor et al., 2020) that incorporated local stakeholder knowledge. Stakeholders focused on snowpack and water impoundment as primary drivers of water availability while researchers had focused on aquifer levels as key drivers - both surface water and aquifer levels are in fact closely linked in the Eastern Snake River Plain Aquifer and USRB (Villamor et al., 2020). The divergence in focus between the two groups, especially early in our interactions, illustrates the process of co-production of knowledge through communication between stakeholders and researchers. Stakeholders identified socio-political and economic dynamics as important drivers of decision-making and system changes whereas researchers focused on biophysical and mechanistic processes. Both of these knowledge bases were synthesized into a single conceptual model that formed the initial representation of the Magic Valley FEWS.
2.2.2 Critical Uncertainties
In workshop #4 stakeholders brainstormed the question: “What are the most critical things that we cannot control?” (Trammell et al., 2021). These were called critical uncertainties and were defined as any factor that would profoundly impact the Magic Valley in the future and was hard to predict. Stakeholders were then asked to rank the critical uncertainties in terms of importance. Twenty-nine points were allocated to each stakeholder to distribute among the uncertainties as they wished. They could allocate all 29 points to one uncertainty or one point to 29 uncertainties, or any combination of points to any number of uncertainties. Scientists often struggle with communicating likelihood of occurrence (confidence intervals) to stakeholders (Spiegelhalter, 2017). This technique elucidated stakeholders’ assessment of uncertainties in terms of importance to the region as well as likelihood of occurrence (Shearer et al., 2006; Trammell et al., 2018).
These discussions were initiated and directed entirely by the SAG with clarification and follow-up questions from the research team. The SAG ranked the top five critical uncertainties as: 1. Will there be sufficient water supply for demand? (31 points), 2. Will water quality regulations change? (21 points) 3. Will allocation of [water] resources affect growth? (20 points), 4. Will highest and best use of water be the driver? (16 points) and 5. Will agriculture continue to be used as a national security tool? (10 points). The uncertainties were then linked to actors in the Magic Valley by how much either an increase or decrease in the uncertainty would inhibit or facilitate each actor’s objectives (Trammell et al., 2021). In this meeting, SAG members identified additional key agents in the system that had not previously been identified, including canal companies, irrigation districts and Native American tribes as direct rather than indirect actors (e.g., Figure 2). The research team represented these linkages as an impact matrix. The draft impact matrix was revisited at the beginning of workshop #5 to check and refine the critical uncertainties.
2.2.3 Scenario Development
The actors, uncertainties, and impacts identified by the SAG in the earlier workshops yielded six draft plausible scenario narratives or storylines for the Magic Valley for 2020–2050 that were documented by the research team, presented to the SAG at workshop #5 and refined at workshops #6a and #6b in the series. The scenarios were developed using the geodesign framework (Steinitz, 2012) which iterates possible futures with stakeholders and asks the question “How do we get from the present state of this geographical study area to the best possible future?” coupled with projecting impacts across the Magic Valley. Each scenario varied two or three of the uncertainties, issues and decisions identified by the SAG to allow them to explore implications of changes in those factors without varying so many factors that imagining the scenario would be overwhelming. The scenarios have been described in detail (Cronan et al., 2022) and are summarized in Table 3. See Figure 3 below illustrating one example, the megadrought scenario co-produced with the SAG.
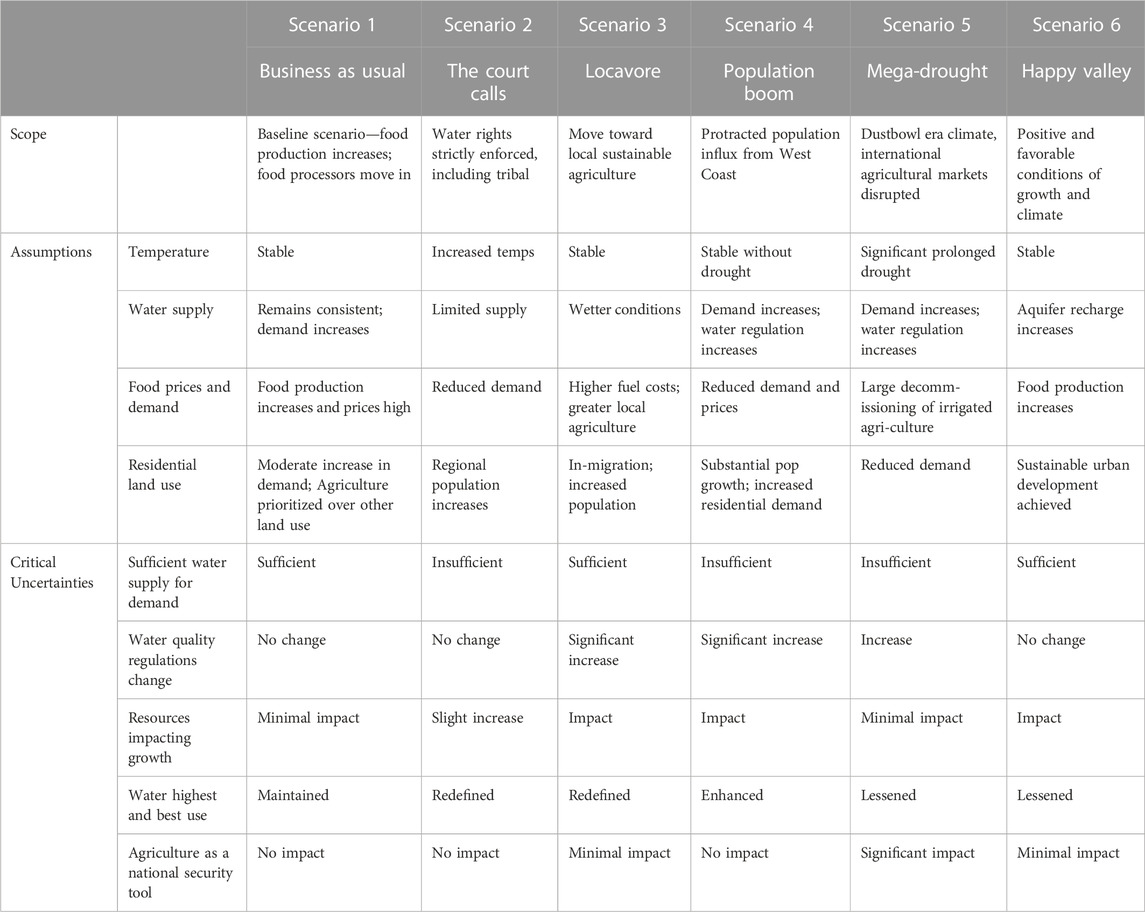
TABLE 3. Summary of scope, assumptions, and uncertainties for Magic Valley stakeholder-derived scenarios and storylines (Cronan et al., 2022).
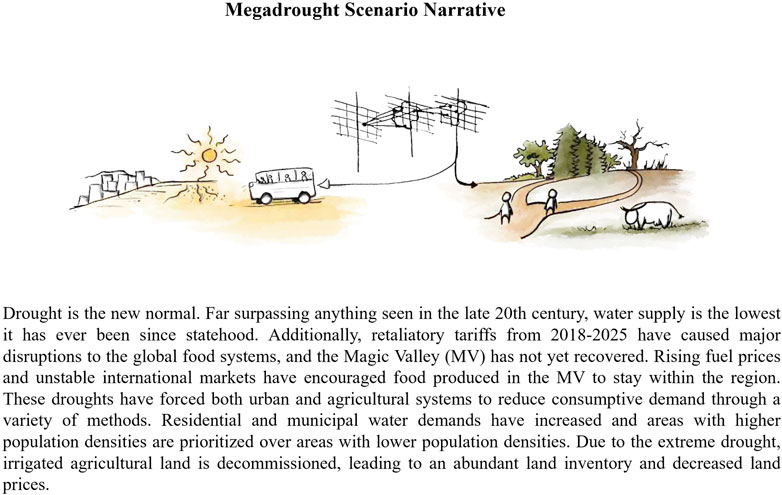
FIGURE 3. Example of stakeholder co-produced scenario narrative for food-energy-water systems in Magic Valley, ID; Megadrought scenario.
The scenario narratives were evaluated, critiqued, refined and co-produced by the SAG with the research team. The co-production process requires listening to input from the SAG and incorporating comments into models and scenario planning. Examples of SAG input into our assumptions, data and projections described below illustrate the respectful interactions, flexibility in approach and humility needed for co-production of knowledge. For instance, our SAG commented that our projections of growth in dairy cows was too high. They pointed out that many counties in the Magic Valley had begun restricting Concentrated Animal Feeding Operations (CAFOs) because residents had complained about smells and due to concerns over water quality. The research team and SAG settled that growth would be limited to an additional 75,000 to 100,000 cows likely located in the eastern part of the region. Without SAG insight and input, the research team would have likely over-estimated the number of dairy cows that could be supported in the future.
The research team also speculated that in a scenario in which food production became more valuable and water was available, water quality standards could be loosened. Our SAG strongly disagreed and pointed out that although standards imposed by federal or state environmental agencies might not be as stringently enforced, health departments would assure that nitrogen (as nitrates) standards would not be exceeded in drinking water. SAG members stated that they could not envision water quality standards being loosened and it was likely that they would become stricter. The SAG commented that previous assumptions about phosphorus being stationary and not a threat are being questioned. They predicted there would be increased use of a phosphorus index to manage nutrient (both nitrogen and phosphorus) levels. Without SAG input, one scenario would have unrealistically relaxed water quality standards.
The research team also anticipated an increase in agricultural acreage should water availability increase. The SAG strenuously disagreed with that situation as well. Pointing out that water in this system has been over-allocated, and current practices had resulted in a decrease in ground-water levels, they stated that additional water, if any, would go to recharge the aquifer first and to storage in reservoirs. Since water rights are tied to land, and there are no additional water rights, there would be no agricultural land added, although land with junior water rights would be planted more often and there would be less land kept fallow if water availability increased. There was also a rich discussion of the relative roles of the water laws of “first in time, first in right” and of “beneficial use.” The SAG further elucidated that current movement toward conjunctive management of ground and surface water was impacting water allocation among junior and senior water rights holders. The research teams’ allocation of water to various users was more accurate and reflected potential future changes more effectively with input from the SAG.
3 Scenario simulation, feedback, and refinement
Multiple computational impact models were developed or adapted to the Magic Valley region by the research team by incorporating both research conducted by the researchers and critiques of the validity and applicability to the region by the SAG. The models represented hydrological processes and water availability (a water balance model or WBM); a land use/landcover change model incorporating crop cover; crop decisions influencing land use and land use change and water use (WBM and land use model); climate projections, and; human population growth and density projections. Combining quantitative modeling, which incorporates a probabilistic approach to predict a likely outcome, with future scenario development, that allow stakeholders to explore novel changes that cannot be fully predicted or anticipated, enriches navigation of uncertainties by stakeholders (Yung et al., 2019).
3.1 Hydrologic modeling
The WBM is a distributed hydrological model incorporating surface runoff, groundwater pools, soil layer, river network, evapotranspiration, and representations of human interventions on the hydrologic cycle (e.g., dams, irrigation, and domestic water use) that was used to characterize the water balance, water availability, and water use (Zuidema et al., 2020). The WBM was modified and applied by the hydrologic modeling team and then preliminary model outputs were presented to, and discussed with, the SAG during model validation and verification. SAG input on model outcomes under each scenario were used to iteratively modify and improve the WBM runs. One of the key lessons relating to the hydrologic model from the SAG was the importance of the Swan Falls Agreement that requires 5,900 cfs water flow at the Swan Falls gauge during months that irrigation is taking place. The SAG informed the team that the agreement was violated regularly during the summer, but usually not for long periods of time. If violations occur over longer periods of time or more frequently, the SAG predicted that agriculture in the Magic Valley would be significantly negatively impacted. The WBM was modified to anticipate future violations of the agreement. The SAG learned from the research team that a significant increase in population (six million people, a ×30 increase) would only increase water use by one percent over current consumption for both urban and agriculture; most had assumed that population increase would consume a much larger portion of available water.
3.2 Climate modeling
Existing climate models were used to build climate futures for the Magic Valley. Stakeholders identified climate futures as critical to understanding each of the Magic Valley scenarios. Climate futures, that is the climate model outcome for a scenario, were selected by the research team to reflect each scenario narrative from a suite of general circulation models (GCMs) and greenhouse gas concentration trajectories (RCPs) that were downscaled (Abatzoglou and Brown, 2012; Rupp et al., 2013; Jiang et al., 2018; Alder and Hostetler, 2019). Climate futures were selected by examining hydrological change over the USRB between 2021 and 2050 and late 20th century (1971–2000) (Gergel et al., 2017). Climate futures were presented to, and discussed with, the SAG. The veracity of annual precipitation and runoff in each climate future was assessed by the SAG. All scenarios except Megadrought (scenario five) used RCP 4.5 utilizing different models that related best to the social and physical conditions described in the scenarios, scenario five utilized a synthetic climate model that simulated Dust Bowl conditions. Each climate future was then represented as an input to the WBM to determine the resulting hydrologic change under each scenario. The SAG discussed how water retention in reservoirs and groundwater recharge might be impacted by changes in precipitation, both in terms of less snow and more rain and in terms of changes in timing of precipitation. These were discussed, at length, as they relate to regulations restricting irrigation to summer months, and what it might mean for delivery systems.
3.3 Agent-based modeling
An ABM was developed to examine the implications of dairy feed-crop rotation on the total energy budget at the landscape level in Magic Valley. The ABM represented manure application, land use cover, total nitrogen and phosphorus, and crop yields. Agent decisions were incorporated in the ABM for changing the composition of the dairy cow feed ration requirement, application of manure to cropland, and the relative nutrient sensitivity. ABM model outputs were used to determine the relative energy efficiency at the landscape level of different crop rotation combinations, and whether a combination of manure reinvestment (as fertilizer) and crop rotation provides greater energy efficiency than conventional agriculture. The ABM was parameterized from the ARDI workshop with the SAG and a questionnaire survey on nutrient management in dairy farming administered to dairy farmers in the Upper Snake River Basin. The preliminary ABM was presented to the SAG.
3.4 Population growth and density
Population growth and density were primarily modeled using past population trends and Dasymetric Mapping (Sleeter and Gould, 2007). A basic linear regression trend was presented to the stakeholders for initial feedback and the assessment of plausibility. Then, two variants of the linear trend were presented: geometric and parabolic growth models. In all cases, the population was projected to increase. On several of the scenarios, SAG members commented that we had not projected population accurately and suggested that we not use linear trends, and recommended percentages of increase or decrease appropriate to the scenario. For the Business As Usual scenario, they commented that our population increase based on a linear projection was not high enough. For our megadrought scenario they commented that there would be a population decline after about 5 years (departure from linear), and for our scenario in which technology companies moved into the Magic Valley, there was a rich discussion about whether technology industries or agriculture would employ more people, and the resulting increase or decrease in population. Once population projections were agreed upon, Dasymetric mapping (Jiang et al., 2018) was used to portray population density and distribution specific to each scenario, using the National Land Cover Database and census tract data as the source of current population densities.
3.5 Indicators
A set of indicators were developed for comparing the outcomes for each scenario over the 2020–2050 time horizon across the Magic Valley landscape (Boyko et al., 2012). Indicators were derived in part from the outputs and outcomes of each of the impact models and in part by ensuring metrics were included for each of the food, energy, and water systems. An initial set of indicators were proposed by the research team and discussed with the SAG from which a modified set of indicators were produced that incorporated suggestions and ideas of the SAG members (Table 4). The indicators were revisited and revised following the sixth and seventh stakeholder workshop (Tables 2 and 4). The final set of indicators presented for each scenario included: 1) total human population, 2) total dairy cow count, 3) total urban area, 4) total agriculture in production, 5) total agriculture supporting dairies, 6) total agriculture converted to urban, and 7) water availability. Landuse and landcover maps and interactive dashboards were used to allow stakeholders to map locations of change in the indicators and in impact model revisions (Center for Resilient Communities, 2020).
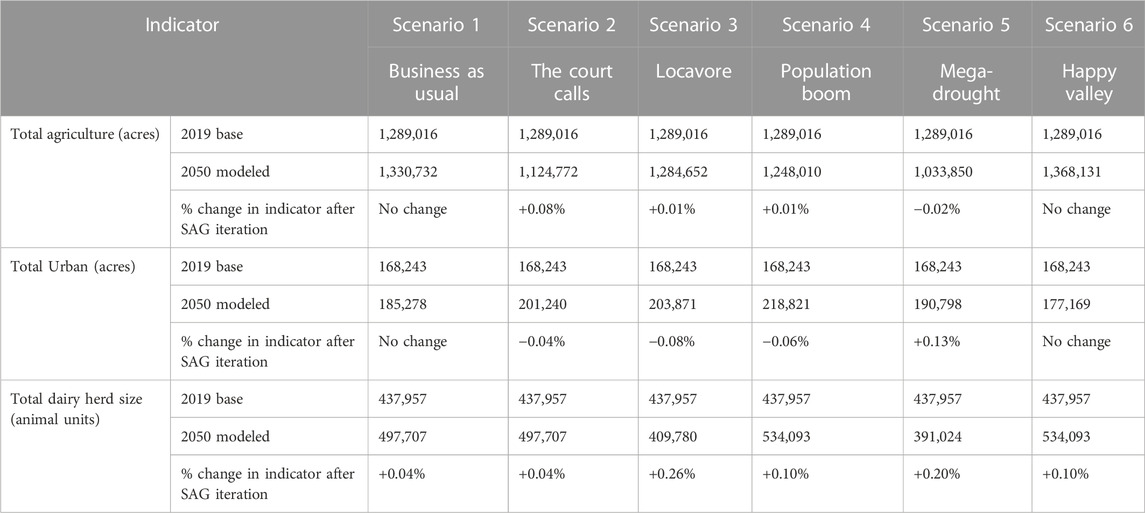
TABLE 4. Comparison of select indicators for Magic Valley scenarios showing indicator values following initial land use landcover and water balance model runs through 2050 and refined indicator values following completion of stakeholder input and iterations.
3.6 Solutions
Because each scenario incorporated different issues the decisions, uncertainties, and solutions were tailored to each scenario and included strategies to reduce water use, reduce and/or reuse nutrients, and reduce energy use over the 2020–2050 scenario time-horizon at the landscape scale. The initial suite of integrated solutions was proposed by the research team based on discussions with stakeholders during the second through fourth SAG workshops as well as brainstorming with a SAG member who is an Extension Service employee. A draft suite of solutions was formally presented to the SAG during the fifth SAG workshop and feedback was sought and documented. Input was provided by the SAG on the practicality, timing, likely adoption, and drawbacks for each proposed solution as well as which solutions best fit each scenario narrative. A revised solution suite was presented to the SAG during the sixth workshop. A further round of revisions was made to solutions related to each scenario and those were presented to stakeholders at the seventh SAG workshop. At that workshop, the timing of each solution in each scenario was fine-tuned (Figure 4). In general, the SAG agreed that stricter water quality regulations would be imposed, that best management practices (structural interventions and policies) would be implemented in crop and dairy farming as well as industrial, municipal and residential land use (Williams et al., 2023). The SAG felt that increased composting of animal wastes would occur quickly. They struggled with when and whether biodigesters would be used because dairy farmers had negative prior experiences with them, and were reluctant to adopt this technology, which they commented had only been successfully implemented by the largest dairies. Spatial land use and land cover changes were also modeled as solutions (e.g. replacement of high water demand crops for less water-intensive crop types in multiple scenarios).
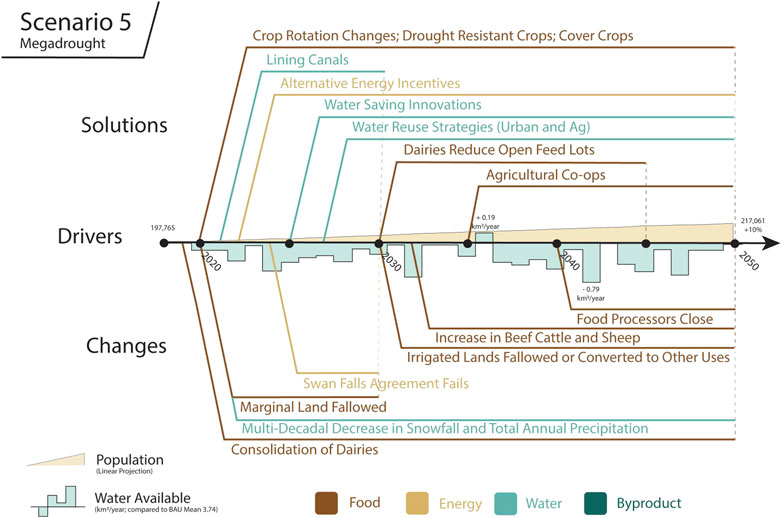
FIGURE 4. Example of integrated set of co-produced visualizations portraying drivers of change, impact models, and indicators for a FEWS scenario in Magic Valley, ID; Megadrought scenario.
3.7 Visualization and mapping
An integrated set of visualizations were developed by the research team portraying drivers of change, impact models, and indicators for each scenario (Cronan et al., 2022). Initially, spatial results and implications were drawn on paper maps by SAG members. Hand drawn-graphics and maps assisted in the SAG with coalescing the scenarios in the early phases of iterating scenarios. While hand-drawn graphics were not essential they did help to align some of the thinking among SAG members and researchers with disparate backgrounds and also aided the communication process. More advanced geospatial representations were then developed for each scenario to highlight changes on crop cover and other attributes. Visualizations of the scenarios were shown to the SAG at workshops #6a and #6b and SAG members preferred the integrated solutions schematic shown below (Figure 4). ESRI GeoPlanner, ESRI Storymaps, and ESRI ArcHub (Ward and Chapman, 2008; Center for Resilient Communities, 2020; Kliskey et al., 2021) were all used as part of the toolkit for delivering geospatial representations of, and outcomes for, scenarios. The visualizations were modified based on SAG input and incorporated into material presented to the SAG at workshops #6 and #7. The most popular visualization incorporated a timeline showing water levels and violations of the Swan Falls Agreement, with solutions overlay as they occurred across the timeline (Figure 4 below; Cronan et al., 2022). All visualizations were delivered through an editable platform which made revisions possible during the workshops.
4 Stakeholder engagement as relationship, design, and process
In our project, the research team learned much about the FEWS system in the Magic Valley region from stakeholders. The in-depth understanding of the SAG regarding interactions among food, energy and water systems and social system constraints (laws, policies, attitudes and values for example) as well as the solutions most likely to be adopted and the timing of adoption (Williams et al., 2023) enabled the research team to better align the impact models with stakeholder knowledge of the system and develop a set of scenarios to guide decision-making for the future of the Magic Valley.
4.1 Engagement as relationship
The application of a SAG in this instance represents the co-production of knowledge as a set of relationships among stakeholders, rights holders, communities, and researchers. Consistent with best practices for stakeholder engagement (Kliskey et al., 2021) this instance of a SAG process emphasizes a set of relationships and their contribution to a culture of respect and trust, co-ownership of the process, and co-production of knowledge and outcomes. Most importantly, relationship was built in our project through listening, respecting local knowledge of the SAG and incorporating their data into our products and outcomes.
The multi-method and iterative engagement approach (Figure 2; Table 2) speaks to the collaborative nature of all involved, it underlies the importance of having stakeholders who are willing to challenge the scientists, and these examples can be viewed as a “qualitative scientific method” where the researchers state a hypothesis (what they believe about the system), the “data” (assessments) from the SAG rejected the hypothesis, and the researchers changed their conceptual model in response, and then re-presented the updated model to the SAG for updated “data” as further input. This was particularly evident in the iterative co-production of scenarios (Cronan et al., 2022) and of solutions (Williams et al., 2023) to FEWS challenges with the SAG.
The process, and the relationships, were not always perfect. Although the research team sought to build a representational SAG, members could be more accurately characterized as being indicative of the region. We were not successful in recruiting members of some groups, aquaculture representatives as one example, whose insights we felt would be important. Recruiting allies of particular groups to a SAG may motivate participation by those groups (Ward and Chapman, 2008; Conallin et al., 2017). Another effective strategy might be to approach one or more influential key representatives and ask for referrals. Initially our outreach to the Idaho Dairymen’s Association was unsuccessful, but we initiated a conversation with that group and discovered that language in our research literature had offended them. After a face-to-face meeting in which we listened to their concerns and agreed to modify our language going forward, we were successful in obtaining their representation on our SAG. We were also unaware until over a year into the project that migrant workers and beef cattle producers were key stakeholders in the region, and should have been involved with the SAG. Ongoing participation of SAG members was also a challenge. A few key members consistently attended workshops and responded to emails, others attended when able, and a few, including our Indigenous members and an organic dairy farmer, stopped attending meetings early in the process (although the Indigenous members continued to provide critiques of our process by email). There are tradeoffs to consider if SAG membership is initially expanded to a number beyond optimal size in anticipation of attrition, as well as to recruiting new members mid-project who will need to be brought up to speed. For longer term projects recruiting new members mid-project could be an intentional strategy to provide new expertise as a project evolves.
In developing models and iterating scenarios with stakeholders, there were sometimes differences of perspective between stakeholder suggestions and researcher assumptions and knowledge. Several examples of those were discussed above and included challenges by stakeholders to researcher modeling of increases in the number of dairy cows and their geographic placement, as well as an initial lack of appreciation by the research team of the importance of the Swan Falls Agreement on minimum permissible river flows. A particular point of tension was discussion of changing water quality standards. The research team proposed in a scenario assuming that the Magic Valley became an area essential to national agricultural production, that water quality standards might be relaxed. Stakeholders did not want to consider any reductions of water quality laws, and seemed to be most uncomfortable with any discussion around it. Also, there was a clear difference in what SAG members understood versus the modeled/projected numbers the research team provided for increasing agricultural area if water became more available. There was, perhaps, reluctance by the research team to immediately address that, and it took several iterations and presentations of outcomes before the research team got it right and SAG members felt comfortable with the outcomes. In the case of significant water reduction, researchers assumed that agricultural land would be decommissioned, but stakeholders disagreed and indicated that land would be fallowed, not decommissioned.
When we asked meeting attendees to evaluate the engagement process, several participants observed that two members who consistently attended meetings were openly disdainful of some opinions expressed by others. They felt that the tension created by those members, in addition to the required time commitment, were probably the key factors in members’ ability and willingness to continue to attend. In future engagements, we will institute a rule about respectful disagreement at the beginning of the process with consistent reminders throughout. Some researchers have also addressed this problem by allocating stakeholders to separate groups when it is felt that interaction among them would not be successful (Conallin et al., 2017; Trammell et al., 2021). Our group has also discussed, in response to the critique about time commitments, whether we could have enhanced participation by breaking our meetings into smaller units of time (our SAG meetings were scheduled to last 6 h) held every 3 or 4 months rather than every 6 months as we did during the project. Our process provided a number of valuable lessons on how to improve the co-production process in the future.
The longitudinal engagement with community members by both university cooperative extension researchers and non-extension researchers was a particularly beneficial aspect of stakeholder engagement viewed as relationship building. Noteworthy from this experience in the Magic Valley case-study was leveraging the longitudinal engagement with community members that the University of Idaho Cooperative Extension provided. The development of the SAG in the early part of the project benefited from the research team members with decades of experience in the locale and who possessed strong social network connectivity. The long-standing relationships with both the land owners, dairy and farm workers, and non-extension scientists not only ensured the appropriate stakeholders were involved, but provided another level of relationship between the research team and the SAG. Stakeholders also contributed to relationship building through pre-existing relationships and networks with other SAG members.
Another set of relationships worth noting were those among the research team, primarily university faculty, staff, and graduate students, and the tensions in balancing the demands of the engagement and co-production goals of the project with the traditional career demands of academic institutions. Senior faculty on the team were able to mentor and advocate for early-career faculty to ameliorate possible impacts from the additional time that stakeholder engagement and co-production efforts demand. By actively including early-career faculty and graduate students in the engagement process it was possible for these team members to gain the necessary intellectual ownership/leadership of their contributions, including research papers. Nevertheless, the significant time that needs to be invested in co-produced science and lengthy start-up, execution, and sustaining of stakeholder engagement continues to be a critical issue.
4.2 Engagement as design
The importance of carefully designed stakeholder engagement efforts for producing effective outcomes in environmental management has been well stated (Reed et al., 2013; Eaton et al., 2021). This is correctly termed as engagement by design. Notably, transdisciplinary research design for supporting stakeholder engagement in FEWS has been more widely adopted (Carpenter et al., 2015; Johnson and Karlberg, 2017; Hoolohan et al., 2018a) and encourages well-thought out and designed approaches to stakeholder engagement. A different way to look at the role of design in stakeholder engagement based on the experience with SAG engagement in the Magic Valley case-study is that co-production of knowledge with stakeholders can elicit design thinking and design outcomes, leading to effective solutions to FEWS challenges. Design thinking is the iterative process of understanding the user, questioning the assumptions, and redefining problems in an attempt to generate alternative strategies and solutions (Brown, 2009; Cross, 2011; Brown and Martin, 2015). The lack of “involved” and stakeholder-driven research tasks and projects continues to create barriers between research and implementation (Lenzholzer et al., 2013), however engagement as design (otherwise known as projective design), has the capacity to demonstrate processes of what might occur requiring an abductive approach rather than inductive (LaGro, 1999; Deming and Swaffield, 2011). The entire co-production process (Figure 2) is in essence engagement as design where scientific methods (e.g., impact models, geospatial analyses) are leveraged by creative thinking between researchers and local stakeholders, and in this project specifically as geodesign thinking, to co-produce solutions to FEWS challenges. Design thinking is explicit in the development of scenario narratives (Table 2: SAG Workshops 5, 6a, and 6b), in the identification of integrated solutions (Table 2: SAG Workshops 6a, 6b, 8a, 8b, and 9), and in development of geospatial land use models representing each scenario as alternative futures. So rather than engagement by design (which is important) we highlight the value of engagement as design.
4.3 Engagement as process
The SAG development and engagement in Magic Valley FEWS highlights co-production of knowledge that entails ongoing, sustained, iterative, and sequential engagement (Figure 2; Table 2) that is as much about the co-production and engagement process as it is about the end products. In this case co-production of knowledge consisted of a series of interconnected engagement processes (Figure 2) that spanned a spectrum of engagement types (Table 5). The ARDI, critical uncertainties, and impact model representations were representational or collaborative engagement; the scenario generation and representations, and the design of solutions were transformative or empowered engagement; while visualization is nominal or consultative engagement. Rather than a single method of engagement this approach to using a SAG demonstrates a complex set of engagement processes that serve different purposes and collectively contribute to the co-production of knowledge.
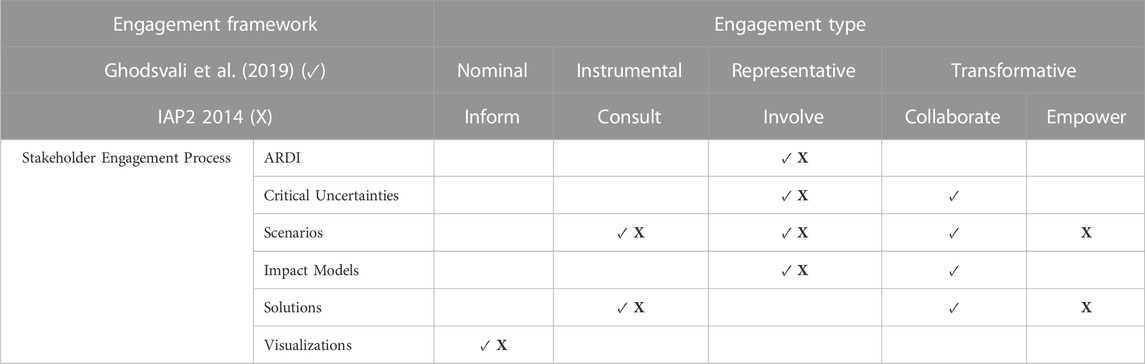
TABLE 5. Type or level of engagement (White, 1996; Bammer, 2019; Ghodsvali et al., 2019) for each process involving the stakeholder advisory group for the Magic Valley FEWS project.
A focus on stakeholder engagement as a process also highlights the importance of flexibility in the design and implementation of the SAG as an approach for engagement in FEWS. The highly iterative nature of the stakeholder engagement processes developed in the Magic Valley case-study (Figure 2; Table 3) and its complex set of engagement approaches (Table 2) provide opportunities for flexibility, adaptation, and learning. In transdisciplinary frameworks these notions of flexibility and learning have been expressed in the form of learning loops (Kliskey et al., 2017; Bammer, 2019; Steger et al., 2021) and in natural resource management as adaptive management (Allen and Gunderson, 2011). For the Magic Valley SAG engagement process the solutions to FEWS challenges and the iterative development of scenarios were the most transformative processes with potential for empowering stakeholders (Table 5). For example, the derivation of scenarios by the SAG members over 15 months via 6 workshops (Table 2) develops a sense of ownership in the scenarios. The sense of ownership by the SAG is demonstrated by the stakeholder-driven solutions that were generated by the group (Williams et al., 2023) although it is premature to determine the extent of implementation of the integrated solutions set. Anecdotally, two of the SAG members commented to the authors that following the summer of 2021 in Magic Valley, during which residents witnessed historic low winter snowpack, low seasonal rainfall, and record low river levels insufficient to fully support irrigation, that the conditions of the Megadrought scenario seemed apparent and that several of the co-generated solutions (e.g., fallowing some agricultural land, converting some feed crop pastures to more drought resistant crops) were being considered. Some SAG members have also agreed to continue as SAG members on new and related research proposals suggesting buy-in to the process.
By situating SAG engagement as an ongoing and iterative process it becomes possible to instill considerable flexibility in stakeholder engagement and in the methodological approaches leading to more acceptable outcomes. This includes flexibility regarding who shows up for which meetings and how that shapes the interpersonal dynamics, and what tools/resources will be most effective. For example, when the trained hydrologist from a canal company was in attendance at meetings we needed to be very quantitative in our discussions and presentation of results. In addition, flexibility in where the conversation will go, being willing to be wrong, and allowing stakeholders to be wrong in support of the evolving narrative of understanding in the process taken as a whole. It is important to respond to the stakeholder interests and provide due diligence in bringing their ideas into the scientific work. The role of co-production processes is increasingly recognized as essential to sustainability initiatives (Schneider et al., 2021) and in this case-study the co-production processes mirror recent iterative and collaborative principles for knowledge co-production (Norström et al., 2020; Kliskey et al., 2021).
In the context of FEWS research and practice co-production processes are both beneficial and challenging at the same time due to the need to work across multiple systems, scales, jurisdictions, and issues. This complexity necessitates multi-faceted, diverse, and flexible stakeholders and engagement approaches.
5 Conclusion
The understanding, representation, and modeling of FEWS trajectories can be enhanced by adopting a process of knowledge co-production with stakeholders, rights holders, and community members. A SAG provides a valuable mechanism for supporting multi-faceted and iterative processes for knowledge co-production. In the Magic Valley FEWS case-study the SAG provided an indicative set of stakeholders of the FEW system, and from the locale, rather than a representative sample of residents. The SAG by engaging as relationship, as design, and as process highlights the co-production and co-ownership of the engagement process, co-production of knowledge, and co-production of outcomes that reflect best practices for stakeholder engagement in FEWS (Bammer, 2019; Norström et al., 2020; Kliskey et al., 2021). This paper expands on best practices in each of the three modes of co-production. For co-production as relationship this translates to spending time to build trust with stakeholders, and the importance of fostering a culture of respect. For co-production as design best practices include the purposeful iteration for understanding the stakeholder, questioning the fundamental assumptions, and redefining problems. And for co-production as process best practices include: iterative engagement, sequential engagement, use of a set of processes for engagement that serve multiple purposes for knowledge co-production, and accommodating flexibility, adaptation, and learning throughout the process. SAG co-production as a process rather than an activity places a premium on building trust and longer-term relationships than social survey approaches and one-off meetings typically involve. This is especially key for new and innovative work (i.e., coupling WBM with climate and land use for the Magic Valley). Without that longer term engagement, stakeholders would likely have been skeptical about those models, and would not have been confident enough to co-produce solutions. Because of the iterative and participatory nature of the project, stakeholders exhibited ownership and stewardship with the project. The researchers utilized various methods of engagement and visualization to evoke a sense of affinity with the scenarios and the process utilized. Similar future studies have the capacity to elicit stakeholder buy-in as well as trust leading to transformative engagement (Ghodsvali et al., 2019) that empowers the local community (Bammer, 2019) rather than simply informing or consulting with stakeholders.
Data availability statement
The datasets presented in this study can be found in online repositories. The names of the repository/repositories and accession number(s) can be found below: CRC (Center for Resilient Communities, 2020) Magic Valley Futures Atlas for Innovations at the Nexus of Food, Energy: URL: https://the-infews-atlas-magic-valley-idaho-alternative-futures-uidaho.hub.arcgis.com/pages/Results.
Ethics statement
The studies involving human participants were reviewed and approved by University of Idaho Institutional Review Board. The patients/participants provided their written informed consent to participate in this study.
Author contributions
Conceptualization, LA and AK; methodology, AK, DG, ET, and DC; formal analysis, AK, ET, DC, and PW; writing—original draft preparation, AK, PW, and D G; writing—review and editing, ET, DC, RL, MM, JO-I, LA, PW, and AK; visualization, AK and DC; supervision, AK, DC, and PW; project administration, AK; funding acquisition, LA, AK, and RL; All authors have read and agreed to the published version of the manuscript.
Funding
This research was funded by the National Science Foundation: Grant number SES-1639524 through the Innovations at the Nexus of Food, Energy, and Water Systems program; grant number OIA-1757324 through the NSF Idaho EPSCoR Program, and; BCS-1856059 through the EngageINFEWS Research Coordination Network. Publication of this article was partially funded by the University of Idaho Open Access Publishing Fund.
Conflict of interest
Author JO-I is employed by Crossroads Concepts and Consulting.
The remaining authors declare that the research was conducted in the absence of any commercial or financial relationships that could be construed as a potential conflict of interest.
Publisher’s note
All claims expressed in this article are solely those of the authors and do not necessarily represent those of their affiliated organizations, or those of the publisher, the editors and the reviewers. Any product that may be evaluated in this article, or claim that may be made by its manufacturer, is not guaranteed or endorsed by the publisher.
References
Abatzoglou, J. T., and Brown, T. J. (2012). A comparison of statistical downscaling methods suited for wildfire applications. Int. J. Climatol. 32, 772–780. doi:10.1002/joc.2312
Alder, J. R., and Hostetler, S. W. (2019). The dependence of hydroclimate projections in snow-dominated regions of the western United States on the choice of statistically downscaled climate data. Water Resour. Res. 55, 2279–2300. doi:10.1029/2018WR023458
Alessa, L., and Chapin, F. S. (2008). Anthropogenic biomes: A key contribution to earth-system science. Trends Ecol. Evol. 23, 529–531. doi:10.1016/j.tree.2008.07.002
Alessa, L., Moon, S., Griffith, D., and Kliskey, A. (2018). Operator driven policy: Deriving action from data using the quadrant enabled Delphi (QED) method. Homeland Security Affairs 14, 6. Available at: https://www.hsdl.org/?abstract&did=.
Allen, C. R., and Gunderson, L. H. (2011). Pathology and failure in the design and implementation of adaptive management. J. Environ. Manag. 92, 1379–1384. doi:10.1016/j.jenvman.2010.10.063
Bammer, G. (2019). Key issues in co-creation with stakeholders when research problems are complex. Evid. Policy A J. Res. Debate Pract. 15, 423–435. doi:10.1332/174426419X15532579188099
Bielicki, J. M., Beetstra, M. A., Kast, J. B., Wang, Y., and Tang, S. (2019). Stakeholder perspectives on sustainability in the food-energy-water nexus. Front. Environ. Sci. 7, 7. doi:10.3389/fenvs.2019.00007
Boyko, C. T., Gaterell, M. R., Barber, A. R. G., Brown, J., Bryson, J. R., Butler, D., et al. (2012). Benchmarking sustainability in cities: The role of indicators and future scenarios. Glob. Environ. Change 22, 245–254. doi:10.1016/j.gloenvcha.2011.10.004
Bréthaut, C., Gallagher, L., Dalton, J., and Allouche, J. (2019). Power dynamics and integration in the water-energy-food nexus: Learning lessons for transdisciplinary research in Cambodia. Environmental Science and Policy 94, 153–162. doi:10.1016/j.envsci.2019.01.010
Brown, G., and Chin, S. Y. W. (2013). Assessing the effectiveness of public participation in neighbourhood planning. Plan. Pract. Res. 28, 563–588. doi:10.1080/02697459.2013.820037
Brown, T. (2009). Change by design: How design thinking transforms organizations and inspires innovation. New York: HarperBusiness.
Brown, T., and Martin, R. (2015). How to use design thinking to make great things actually happen. Harv. Bus. Rev. 5, 1–10.
Carpenter, S. R., Booth, E. G., Gillon, S., Kucharik, C. J., Loheide, S., Mase, A. S., et al. (2015). Plausible futures of a social-ecological system: Yahara watershed. E&S 20, art10. doi:10.5751/ES-07433-200210
Center for Resilient Communities (2020). Magic Valley Innovations at the nexus of food, energy, and water systems futures Atlas. Moscow, ID, USA: University of Idaho. Available at: https://the-infews-atlas-magic-valley-idaho-alternative-futures-uidaho.hub.arcgis.com/pages/Results.
Conallin, J. C., Dickens, C., Hearne, D., and Allan, C. (2017). “Chapter 7 - stakeholder engagement in environmental water management,” in Water for the environment. Editors A. C. Horne, J. A. Webb, M. J. Stewardson, B. Richter, and M. Acreman (Academic Press), 129–150. doi:10.1016/B978-0-12-803907-6.00007-3
Creed, R., Baily, B., Potts, J., Bray, M., and Austin, R. (2018). Moving towards sustainable coasts: A critical evaluation of a stakeholder engagement group in successfully delivering the mechanism of adaptive management. Mar. Policy 90, 184–193. doi:10.1016/j.marpol.2017.12.009
Cronan, D., Trammell, E. J., KliskeyWilliams, A. P., and Alessa, L. (2022). Images to evoke decision-making: Building compelling representations for stakeholder-driven futures. Sustainability 14, 2980. doi:10.3390/su14052980
DasGupta, R., Hashimoto, S., Okuro, T., and Basu, M. (2019). Scenario-based land change modelling in the Indian sundarban delta: An exploratory analysis of plausible alternative regional futures. Sustain Sci. 14, 221–240. doi:10.1007/s11625-018-0642-6
De Stefano, L., Hernández-Mora, N., Iglesias, A., and Sánchez, B. (2017). Defining adaptation measures collaboratively: A participatory approach in the doñana socio-ecological system, Spain. J. Environ. Manag. 195, 46–55. doi:10.1016/j.jenvman.2016.10.042
Deming, M. E., and Swaffield, S. (2011). Landscape architectural research: Inquiry, strategy, design. Hoboken, NJ: John Wiley and Sons.
Djenontin, I. N. S., and Meadow, A. M. (2018). The art of co-production of knowledge in environmental sciences and management: Lessons from international practice. Environ. Manag. 61, 885–903. doi:10.1007/s00267-018-1028-3
Eaton, W. M., Brasier, K. J., Burbach, M. E., Whitmer, W., Engle, E. W., Burnham, M., et al. (2021). A conceptual framework for social, behavioral, and environmental change through stakeholder engagement in water resource management. Soc. Nat. Resour. 34, 1111–1132. doi:10.1080/08941920.2021.1936717
Elsawah, S., Hamilton, S. H., Jakeman, A. J., Rothman, D., Schweizer, V., Trutnevyte, E., et al. (2020). Scenario processes for socio-environmental systems analysis of futures: A review of recent efforts and a salient research agenda for supporting decision making. Sci. Total Environ. 729, 138393. doi:10.1016/j.scitotenv.2020.138393
Ertet, M. O. (1979). The role of citizen advisory groups in water resources Planning1. JAWRA J. Am. Water Resour. Assoc. 15, 1515–1523. doi:10.1111/j.1752-1688.1979.tb01166.x
Etienne, M., Du Toit, D. R., and Pollard, S. (2011). Ardi: A Co-construction method for participatory modeling in natural resources management. Ecol. Soc. 16, 1–14. Available at: https://www.jstor.org/stable/26268857 (Accessed January 12, 2021).
Ferguson, L., Chan, S., and Santelmann, M. V. (2018). Transdisciplinary Research in Water Sustainability: What’s in It for an Engaged Researcher-Stakeholder Community?. Water Alternatives 11, 18.
Flynn, M., Ford, J. D., Pearce, T., and Harper, S. L. (2018). Participatory scenario planning and climate change impacts, adaptation and vulnerability research in the Arctic. Environ. Sci. Policy 79, 45–53. doi:10.1016/j.envsci.2017.10.012
Franz, N., Garst, B., and Gagnon, R. (2015). The cooperative extension program development model: Adapting to a changing context. J. Hum. Sci. Ext. 3, 3–12. doi:10.54718/cwez3223
Fraser, E. D. G., Dougill, A. J., Mabee, W. E., Reed, M., and McAlpine, P. (2006). Bottom up and top down: Analysis of participatory processes for sustainability indicator identification as a pathway to community empowerment and sustainable environmental management. J. Environ. Manag. 78, 114–127. doi:10.1016/j.jenvman.2005.04.009
Garst, B. A., and McCawley, P. F. (2015). Solving problems, ensuring relevance, and facilitating change: The evolution of needs assessment within cooperative extension. J. Hum. Sci. Ext. 3, 684. Available at: https://www.jhseonline.com/article/view/684 (Accessed July 29, 2021).
Gebreyes, M., Bazzana, D., Simonetto, A., Müller-Mahn, D., Zaitchik, B., and Gilioli, G. (2020). Local Perceptions of Water-Energy-Food Security: Livelihood Consequences of Dam Construction in Ethiopia. Sustainability 12, 2161. doi:10.3390/su12062161
Gergel, D. R., Nijssen, B., Abatzoglou, J. T., Lettenmaier, D. P., and Stumbaugh, M. R. (2017). Effects of climate change on snowpack and fire potential in the Western USA. Clim. Change 141, 287–299. doi:10.1007/s10584-017-1899-y
Ghodsvali, M., Krishnamurthy, S., and de Vries, B. (2019). Review of transdisciplinary approaches to food-water-energy nexus: A guide towards sustainable development. Environ. Sci. Policy 101, 266–278. doi:10.1016/j.envsci.2019.09.003
Givens, J. E., Padowski, J., Guzman, C. D., Malek, K., Witinok-Huber, R., Cosens, B., et al. (2018). Incorporating Social System Dynamics in the Columbia River Basin: Food-Energy-Water Resilience and Sustainability Modeling in the Yakima River Basin. Front. Environ. Sci 6. doi:10.3389/fenvs.2018.00104
Hagemann, N., van der Zanden, E. H., Willaarts, B. A., Holzkämper, A., Volk, M., Rutz, C., et al. (2020). Bringing the sharing-sparing debate down to the ground—lessons learnt for participatory scenario development. Land Use Policy 91, 104262. doi:10.1016/j.landusepol.2019.104262
Hassenforder, E., Smajgl, A., and Ward, J. (2015). Towards understanding participatory processes: Framework, application and results. J. Environ. Manag. 157, 84–95. doi:10.1016/j.jenvman.2015.04.012
Hines, S., Packham, J., Wilmore, C., and Taylore, G. (2013). Contribution of agribusiness to the Magic Valley economy, 2013. Available at: https://www.extension.uidaho.edu/publishing/html/BUL916-Contribution-of-Agribusiness-to-the-Magic-Valley-Economy.aspx?title=Search&category1=Search&category2=NULL.
Hoolohan, C., Larkin, A., McLachlan, C., Falconer, R., Soutar, I., Suckling, J., et al. (2018a). Engaging stakeholders in research to address water–energy–food (WEF) nexus challenges. Sustain Sci. 13, 1415–1426. doi:10.1007/s11625-018-0552-7
Hoolohan, C., McLachlan, C., and Mander, S. (2018b). Food related routines and energy policy: A focus group study examining potential for change in the United Kingdom. Energy Res. Soc. Sci. 39, 93–102. doi:10.1016/j.erss.2017.10.050
Howarth, C., and Monasterolo, I. (2017). Opportunities for knowledge co-production across the energy-food-water nexus: Making interdisciplinary approaches work for better climate decision making. Environ. Sci. Policy 75, 103–110. doi:10.1016/j.envsci.2017.05.019
Hunt, L. M., and McFarlane, B. L. (2007). Understanding self-evaluations of effectiveness by forestry advisory committee members: A case of ontario’s local citizens committee members. J. Environ. Manag. 83, 105–114. doi:10.1016/j.jenvman.2006.02.007
Iwaniec, D. M., Metson, G. S., and Cordell, D. (2016). P-FUTURES: Towards urban food and water security through collaborative design and impact. Curr. Opin. Environ. Sustain. 20, 1–7. doi:10.1016/j.cosust.2016.03.001
Izydorczyk, K., Piniewski, M., Krauze, K., Courseau, L., Czyż, P., Giełczewski, M., et al. (2019). The ecohydrological approach, SWAT modelling, and multi-stakeholder engagement – a system solution to diffuse pollution in the Pilica basin, Poland. J. Environ. Manag. 248, 109329. doi:10.1016/j.jenvman.2019.109329
Jiang, Y., Kim, J. B., Still, C. J., Kerns, B. K., Kline, J. D., and Cunningham, P. G. (2018). Inter-comparison of multiple statistically downscaled climate datasets for the Pacific Northwest, USA. Sci. Data 5, 180016. doi:10.1038/sdata.2018.16
Johnson, O. W., and Karlberg, L. (2017). Co-Exploring the water-energy-food nexus: Facilitating dialogue through participatory scenario building. Front. Environ. Sci. 5, 24. doi:10.3389/fenvs.2017.00024
Jones, P. (2011). Developing and applying interactive visual tools to enhance stakeholder engagement in accessibility planning for mobility disadvantaged groups. Res. Transp. Bus. Manag. 2, 29–41. doi:10.1016/j.rtbm.2011.08.001
Kabaya, K., Hashimoto, S., Fukuyo, N., Uetake, T., and Takeuchi, K. (2019). Investigating future ecosystem services through participatory scenario building and spatial ecological–economic modelling. Sustain Sci. 14, 77–88. doi:10.1007/s11625-018-0590-1
Kebede, A. S., Nicholls, R. J., Allan, A., Arto, I., Cazcarro, I., Fernandes, J. A., et al. (2018). Applying the global RCP–SSP–SPA scenario framework at sub-national scale: A multi-scale and participatory scenario approach. Sci. Total Environ. 635, 659–672. doi:10.1016/j.scitotenv.2018.03.368
Kelsey, L. D., and Hearne, C. C. (1949). Cooperative extension work [by] lincoln david kelsey, with the collaboration of cannon chiles Hearne. Ithaca, NY: Comstock Pub Associates.
Kliskey, A., Alessa, L., Wandersee, S., Williams, P., Trammell, J., Powell, J., et al. (2017). A science of integration: Frameworks, processes, and products in a place-based, integrative study. Sustain Sci. 12, 293–303. doi:10.1007/s11625-016-0391-3
Kliskey, A., Williams, P., Griffith, D. L., Dale, V. H., Schelly, C., Marshall, A. M., et al. (2021). Thinking big and thinking small: A conceptual framework for best practices in community and stakeholder engagement in food, energy, and water systems. Sustainability 13, 2160. doi:10.3390/su13042160
Kok, K., Bärlund, I., Flörke, M., Holman, I., Gramberger, M., Sendzimir, J., et al. (2015). European participatory scenario development: Strengthening the link between stories and models. Clim. Change 128, 187–200. doi:10.1007/s10584-014-1143-y
Kok, K. (2009). The potential of Fuzzy Cognitive Maps for semi-quantitative scenario development, with an example from Brazil. Glob. Environ. Change 19, 122–133. doi:10.1016/j.gloenvcha.2008.08.003
Koua, E. L., Maceachren, A., and Kraak, M. -J. (2006). Evaluating the usability of visualization methods in an exploratory geovisualization environment. Int. J. Geogr. Inf. Sci. 20, 425–448. doi:10.1080/13658810600607550
Lafon, N. W., McMullin, S. L., Steffen, D. E., and Schulman, R. S. (2004). Improving stakeholder knowledge and agency image through collaborative planning. Wildl. Soc. Bull. 32, 220–231. doi:10.2193/0091-7648(2004)32[220:ISKAAI]2.0.CO;2
LaGro, J. A. (1999). Research capacity: A matter of semantics? Landsc. Jrnl 18, 179–186. doi:10.3368/lj.18.2.179
Lehmann, S. (2018). Implementing the Urban Nexus approach for improved resource-efficiency of developing cities in Southeast-Asia. City, Culture and Society 13, 46–56. doi:10.1016/j.ccs.2017.10.003
Lenzholzer, S., Duchhart, I., and Koh, J. (2013). ‘Research through designing’ in landscape architecture. Landsc. Urban Plan. 113, 120–127. doi:10.1016/j.landurbplan.2013.02.003
Lynn, F. M., and Busenberg, G. J. (1995). Citizen advisory committees and environmental policy: What we know, what’s left to discover. Risk Anal. 15, 147–162. doi:10.1111/j.1539-6924.1995.tb00309.x
McBride, M. F., Lambert, K. F., Huff, E. S., Theoharides, K. A., Field, P., and Thompson, J. R. (2017). Increasing the effectiveness of participatory scenario development through codesign. E&S 22, art16. doi:10.5751/ES-09386-220316
McGurk, B., Sinclair, A. J., and Diduck, A. (2006). An assessment of stakeholder advisory committees in forest management: Case studies from manitoba, Canada. Soc. Nat. Resour. 19, 809–826. doi:10.1080/08941920600835569
Mochizuki, J., Magnuszewski, P., and Linnerooth-Bayer, J. (2018). “Games for aiding stakeholder deliberation on nexus policy issues,” in Managing water, soil and waste resources to achieve sustainable development goals: Monitoring and implementation of integrated resources management. Editors S. Hülsmann, and R. Ardakanian (Cham: Springer International Publishing), 93–124. doi:10.1007/978-3-319-75163-4_5
Nabatchi, T. (2012). Putting the “public” back in public values research: Designing participation to identify and respond to values. Public Adm. Rev. 72, 699–708. doi:10.1111/j.1540-6210.2012.02544.x
Nol, L., Verburg, P. H., and Moors, E. J. (2012). Trends in future N2O emissions due to land use change. J. Environ. Manag. 94, 78–90. doi:10.1016/j.jenvman.2011.06.053
Norström, A. V., Cvitanovic, C., Löf, M. F., West, S., Wyborn, C., Balvanera, P., et al. (2020). Principles for knowledge co-production in sustainability research. Nat. Sustain. 3, 182–190. doi:10.1038/s41893-019-0448-2
Oteros-Rozas, E., Martín-López, B., Daw, T. M., Bohensky, E. L., Butler, J. R. A., Hill, R., et al. (2015). Participatory scenario planning in place-based social-ecological research: Insights and experiences from 23 case studies. E&S 20, art32. doi:10.5751/ES-07985-200432
Palomo, I., Martín-López, B., López-Santiago, C., and Montes, C. (2011). Participatory scenario planning for protected areas management under the ecosystem services framework: The doñana social-ecological system in southwestern Spain. E&S 16, art23. doi:10.5751/ES-03862-160123
Planque, B., Mullon, C., Arneberg, P., Eide, A., Fromentin, J., Heymans, J. J., et al. (2019). A participatory scenario method to explore the future of marine social-ecological systems. Fish. Fish. 20, 434–451. doi:10.1111/faf.12356
Plieninger, T., Bieling, C., Ohnesorge, B., Schaich, H., Schleyer, C., and Wolff, F. (2013). Exploring futures of ecosystem services in cultural landscapes through participatory scenario development in the swabian alb, Germany. E&S 18, art39. doi:10.5751/ES-05802-180339
Polk, M. (2015). Transdisciplinary co-production: Designing and testing a transdisciplinary research framework for societal problem solving. Futures 65, 110–122. doi:10.1016/j.futures.2014.11.001
Reed, M. S. (2008). Stakeholder participation for environmental management: A literature review. Biological Conservation 141, 2417–2431. doi:10.1016/j.biocon.2008.07.014
Reed, M. S., Kenter, J., Bonn, A., Broad, K., Burt, T. P., Fazey, I. R., et al. (2013). Participatory scenario development for environmental management: A methodological framework illustrated with experience from the UK uplands. J. Environ. Manag. 128, 345–362. doi:10.1016/j.jenvman.2013.05.016
Reilly, K., Adamowski, J., and John, K. (2018). Participatory mapping of ecosystem services to understand stakeholders’ perceptions of the future of the Mactaquac Dam, Canada. Ecosyst. Serv. 30, 107–123. doi:10.1016/j.ecoser.2018.01.002
Reinhardt, J., Liersch, S., Abdeladhim, M. A., Diallo, M., Dickens, C., Fournet, S., et al. (2018). Systematic evaluation of scenario assessments supporting sustainable integrated natural resources management: Evidence from four case studies in africa. E&S 23, art5. doi:10.5751/ES-09728-230105
Robson, M., and Rosenthal, J. (2014). Evaluating the effectiveness of stakeholder advisory committee participation in forest management planning in Ontario, Canada. For. Chron. 90, 361–370. doi:10.5558/tfc2014-070
Rupp, D. E., Abatzoglou, J. T., Hegewisch, K. C., and Mote, P. W. (2013). Evaluation of CMIP5 20th century climate simulations for the Pacific Northwest USA. J. Geophys. Res. Atmos. 118 (10), 10884–10906. doi:10.1002/jgrd.50843
Sanjuan-Delmás, D., Taelman, S. E., Arlati, A., Obersteg, A., Vér, C., Óvári, Á., et al. (2021). Sustainability assessment of organic waste management in three EU Cities: Analysing stakeholder-based solutions. Waste Manag. 132, 44–55. doi:10.1016/j.wasman.2021.07.013
Schneider, F., Tribaldos, T., Adler, C., Biggs, R., (Oonsie),, , de Bremond, A., et al. (2021). Co-Production of knowledge and sustainability transformations: A strategic compass for global research networks. Curr. Opin. Environ. Sustain. 49, 127–142. doi:10.1016/j.cosust.2021.04.007
Sesan, T., Sanfo, S., Sikhwivhilu, K., Dakyaga, F., Aziz, F., Yirenya-Tawiah, D., et al. (2022). Mediating knowledge Co-production for inclusive governance and delivery of food, water and energy services in african cities. Urban Forum 33 (3), 281–307. doi:10.1007/s12132-021-09440-w
Shearer, A. W., Mouat, D. A., Bassett, S. D., Binford, M. W., Johnson, C. W., and Saarinen, J. A. (2006). Examining development-related uncertainties for environmental management: Strategic planning scenarios in Southern California. Landsc. Urban Plan. 77, 359–381. doi:10.1016/j.landurbplan.2005.04.005
Sleeter, R., and Gould, M. (2007). Geographic information system software to remodel population data using dasymetric mapping methods. Available at: https://pubs.usgs.gov/tm/tm11c2/(Accessed July 17, 2021).
Spiegelhalter, D. (2017). Risk and uncertainty communication. Annu. Rev. Stat. Appl. 4, 31–60. doi:10.1146/annurev-statistics-010814-020148
Steger, C., Klein, J. A., Reid, R. S., Lavorel, S., Tucker, C., Hopping, K. A., et al. (2021). Science with society: Evidence-based guidance for best practices in environmental transdisciplinary work. Glob. Environ. Change 68, 102240. doi:10.1016/j.gloenvcha.2021.102240
Steinitz, C. (2012)A framework for geodesign: Changing geography by design. Available at: https://library.wur.nl/WebQuery/titel/2147477 (accessed on August 29, 2019).
Trammell, E. J., Thomas, J. S., Mouat, D., Korbulic, Q., and Bassett, S. (2018). Developing alternative land-use scenarios to facilitate natural resource management across jurisdictional boundaries. J. Environ. Plan. Manag. 61, 64–85. doi:10.1080/09640568.2017.1289901
Trammell, J., Krupa, M., Williams, P., and Kliskey, A. (2021). Using comprehensive scenarios to identify social–ecological threats to salmon in the kenai river watershed, Alaska. Sustainability 13, 5490. doi:10.3390/su13105490
Villamor, G. B., Griffith, D. L., Kliskey, A., and Alessa, L. (2020). Contrasting stakeholder and scientist conceptual models of food-energy-water systems: A case study in Magic Valley. South. Ida. 2, 16312. doi:10.18174/sesmo.2020a16312
Volkery, A., Ribeiro, T., Henrichs, T., and Hoogeveen, Y. (2008). Your vision or my model? Lessons from participatory land use scenario development on a European scale. Syst. Pract. Action Res. 21, 459–477. doi:10.1007/s11213-008-9104-x
Walker, D. H. T., Bourne, L. M., and Shelley, A. (2008). Influence, stakeholder mapping and visualization. Constr. Manag. Econ. 26, 645–658. doi:10.1080/01446190701882390
Walz, A., Lardelli, C., Behrendt, H., Grêt-Regamey, A., Lundström, C., Kytzia, S., et al. (2007). Participatory scenario analysis for integrated regional modelling. Landsc. Urban Plan. 81, 114–131. doi:10.1016/j.landurbplan.2006.11.001
Ward, S., and Chapman, C. (2008). Stakeholders and uncertainty management in projects. Constr. Manag. Econ. 26, 563–577. doi:10.1080/01446190801998708
White, D., Jones, J., Maciejewski, R., Aggarwal, R., and Mascaro, G. (2017). Stakeholder analysis for the food-energy-water nexus in phoenix, Arizona: Implications for nexus governance. Sustainability 9, 2204. doi:10.3390/su9122204
White, S. C. (1996). Depoliticising development: The uses and abuses of participation. Dev. Pract. 6, 6–15. doi:10.1080/0961452961000157564
Williams, P., Kliskey, A., Cronan, D., Trammell, J., de Haro-Marti, M., and Wilson, J. (2023). Constructing futures, enhancing solutions: Stakeholder-driven scenario development and system modeling for climate-change challenges. Front. Environ. Sci. 11, 1055547. doi:10.3389/fenvs.2023.1055547
Xexakis, G., and Trutnevyte, E. (2019). Are interactive web-tools for environmental scenario visualization worth the effort? An experimental study on the Swiss electricity supply scenarios 2035. Environ. Model. Softw. 119, 124–134. doi:10.1016/j.envsoft.2019.05.014
Yan, W., and Roggema, R. (2019). Developing a design-led approach for the food-energy-water nexus in cities. Urban Plan. 4, 123–138. doi:10.17645/up.v4i1.1739
Yung, L., Louder, E., Gallagher, L. A., Jones, K., and Wyborn, C. (2019). How methods for navigating uncertainty connect science and policy at the water-energy-food nexus. Front. Environ. Sci. 7, 37. doi:10.3389/fenvs.2019.00037
Keywords: engagement design, engagement process, food-energy-water systems, impact model, knowledge coproduction, representation model, stakeholder advisory group, stakeholder engagement
Citation: Kliskey A“, Williams P, Trammell EJ, Cronan D, Griffith D, Alessa L, Lammers R, Haro-Martí MEd and Oxarango-Ingram J (2023) Building trust, building futures: Knowledge co-production as relationship, design, and process in transdisciplinary science. Front. Environ. Sci. 11:1007105. doi: 10.3389/fenvs.2023.1007105
Received: 29 July 2022; Accepted: 24 January 2023;
Published: 06 February 2023.
Edited by:
Jeffrey M. Bielicki, The Ohio State University, United StatesReviewed by:
Saba Siddiki, Syracuse University, United StatesDouglas Jackson-Smith, The Ohio State University, United States
Copyright © 2023 Kliskey, Williams, Trammell, Cronan, Griffith, Alessa, Lammers, Haro-Martí and Oxarango-Ingram. This is an open-access article distributed under the terms of the Creative Commons Attribution License (CC BY). The use, distribution or reproduction in other forums is permitted, provided the original author(s) and the copyright owner(s) are credited and that the original publication in this journal is cited, in accordance with accepted academic practice. No use, distribution or reproduction is permitted which does not comply with these terms.
*Correspondence: Andrew “Anaru” Kliskey, YWtsaXNrZXlAdWlkYWhvLmVkdQ==