- Department of Biology and Program in Environmental Studies and Sciences, Rhodes College, Memphis, TN, United States
Microplastics are ubiquitous in aquatic ecosystems globally, with tropical freshwater systems underrepresented in the literature. The ubiquity of microplastics may affect the feeding, growth, reproduction, and survival of organisms in aquatic systems; however, the data on the potential effects of microplastics on aquatic organisms is tentative. In the current study, I conducted a meta-analysis using published data to examine the impacts of microplastic exposure on functional traits (i.e., feeding, growth, reproduction, survival) of fish and aquatic invertebrates. The data revealed that while there were within-taxa negative effects on traits such as reproduction and growth some effect sizes were low, suggesting that the exposure to microplastics may vary across taxa. Globally, negative effects on growth, reproduction, and even survival were evident in some taxa (e.g., bivalves, crustaceans). Considering feeding habits, negative effects of microplastic were more pronounced in bacterivores, omnivores, predators, and filter feeders compared to shredders. In tropical freshwater systems, microplastics had no significant effects on the feeding, growth, reproduction, and survival of aquatic organisms. It is worth noting that organisms that are passive feeders (e.g., bivalves) may be particularly susceptible to microplastic pollution, which in turn may have long-lasting effects on the stability of lacustrine and lotic food webs. Because microplastics may impart more chronic effects than acute effects, future works must include understudied regions of the world (e.g., freshwater systems) and must emphasize the subtle role that microplastics may play on the physiology and behavior of organisms in the long term.
Introduction
Microplastic ubiquity in aquatic environments is widely recognized (Gregory, 1978; Brahney et al., 2020), with much of the data suggesting that microplastics are the most abundant man-made pollutant on Earth (Geyer et al., 2017; Lavers and Bond, 2017). Microplastics originate from the breakdown of large plastic material via prolonged exposure to ultra-violet light and/or physical scuffing (Andrady, 2011). Other sources of microplastics include textile manufacturing industries (Dris et al., 2017; Gasperi et al., 2018), personal care products (e.g., toothpaste; Biginagwa et al., 2016), tire wear plastics and road-wear-associated microplastics (Vogelsang et al., 2018; Rødland et al., 2022), artificial turfs (e.g., playgrounds; van Kleunen et al., 2020), street litter (Haave and Henriksen, 2022), paint (Gaylarde et al., 2021), and wastewater from washing machines (Napper and Thompson, 2016). The ubiquity of microplastics in aquatic systems has been recognized for over four decades, with the effects of microplastics recognized among scientists and non-science audiences. With the effect of microplastics recognized, many researchers and citizens have emphasized the need for studies documenting the effect of microplastics on aquatic animals. To this end, it is not surprising that studies examining the effect of microplastics on aquatic animals have burgeoned in the last two decades (Lusher et al., 2017; Bucci et al., 2020).
Aquatic organisms may consume microplastics via the active or passive ingestion of microplastics attached to algae or sediment, which is a source of food for other animals (Desforges et al., 2015; Tongo and Erhunmwunse, 2022). Because microplastics can persist in the body of organisms for long periods, they can potentially be passed to higher trophic levels (predator species) such as fish, birds, and humans (reviewed comprehensively by Carbery et al., 2018). Microplastics can also be incorporated into an organism’s tissues via the gills and gut walls (Watts et al., 2016). To date, several researchers have demonstrated the effects of microplastics on different organisms such as fish (e.g., Tongo and Erhunmwunse, 2022) and aquatic invertebrates (Paul-Pont et al., 2016). Specifically, microplastic exposure has been associated with several detrimental physiological effects, including decreased food consumption in annelid worms (Arenicola marina; Besseling et al., 2013), decreased growth in freshwater amphipods (Gammarus fossarum; Straub et al., 2017), depletion of energy reserves in African catfish (Clarias gariepinus; Karami et al., 2016), negative impacts on the fecundity of oyster (Crassostrea gigas; Sussarellu et al., 2016) and death in amphipods (Hyalella azteca; Au et al., 2015). Additionally, microplastics can adsorb organic pollutants (e.g., pesticides, polychlorinated biphenyls; PCBs), which subsequently have detrimental health effects such as endocrine disruption and death (Bakir et al., 2014; Horn et al., 2020). While microplastics can adversely affect organisms, some research has shown that microplastics do not affect aquatic organisms (e.g., Green et al., 2016). These data show that conclusions about the effects of microplastics on aquatic organisms may not be consistent across studies. For instance, ingestion of microplastics had not effect on mortality of zooplankton (Daphnia magna; Ogonowski et al., 2016) and African sharptooth catfish (Clarias gariepinus; Tongo and Erhunmwunse, 2022). Similarly, microplastic ingestion had no effect on the New Zealand mud snails (Potamopyrgus antipodarum; Imhof and Laforsch, 2016). Elsewhere, ingestion of microplastics caused death in brine shrimp (Artemia franciscana; Eom et al., 2020) and Zebra fish (Danio rerio; Dinani et al., 2021). It would seem that some organisms may be resilient to stresses induced by microplastic exposure (Watts et al., 2016) and more importantly microplastic effects may be taxon specific (Yıldız et al., 2022). For example, microplastics do not affect growth, behavior and metabolism of some amphipods (Gammarus pulex; Weber et al., 2018) whereas the same plastics decrease reproduction and growth in other groups of amphipods (Hyalella azteca; Au et al., 2015). In Japanese rice fish (Oryzias latipes), ingestion of fish causes stress and necrosis of single cells, while in other fishes it causes lipid accumulation and oxidative stress (Rochman et al., 2013; Lu et al., 2016). The divergent results on the potential impact of microplastic pollution in aquatic systems and the factors that drive those impacts remain to be extensively addressed.
In aquatic ecosystems, several abiotic factors may influence the effects of microplastics to aquatic consumers, including temperature, pH, ultraviolet radiation, and the presence of other environmental contaminants (Oliveira et al., 2013; Fonte et al., 2016; Cao et al., 2021; Ahechti et al., 2022). Among these factors, temperature is of special relevance because it can influence many biological and ecological processes. Moreover, aquatic communities are under increasing pressure from global warming, with projected increases of at least 1.5°C by the end of the century (IPCC, 2022). The survival of water fleas (Daphnia magna) under the presence of microplastics has been noted to decrease sharply with water temperature increases from 18 to 26°C (Jaikumar et al., 2018), indicating that these two stressors acted synergistically when they were combined. The underlying mechanisms of the synergistic effects of temperature and microplastics, may be because higher temperatures increase metabolic rates in organisms, which subsequently increases individual feeding rates and alters consumer-prey interactions (Brown et al., 2004; Rall et al., 2012). In spite of the potential interactions between temperature and microplastics, there is lack of research about the interactive effects of warming and microplastics on metabolic rates (but see Kratina et al., 2019; Serra et al., 2020). This uncertainty about the potential for water temperature to modify the impact of microplastics requires some attention for ecologists to fully understand the current and future risks of microplastic exposure to aquatic fauna.
Microplastic size and length of exposure to microplastics dictate the potential effects of microplastic on aquatic fauna’s functional traits (Kögel et al., 2020). For instance, large microplastics can often block guts in aquatic organisms, which often decreases feeding and resource availability as has been documented in zooplankton (Cole et al., 2013). Moreover, microplastics can induce immune and strong inflammatory responses in invertebrates (von Moos et al., 2012; Avio et al., 2015; Ribeiro et al., 2017), which may alter organisms response to disease and parasites and potentially cause organisms to divert energy from other physiological processes (Greven et al., 2016). The concentration and length of microplastic exposure are key factors behind effects of microplastic on aquatic organisms. Daphnia magna a non-selective feeder that plays key roles in freshwater food chains and is a food source for many aquatic organisms (Hiltunen et al., 2017), is significantly affected after acute exposure to microplastic concentrations ranging between 75 and 150 mg/L (Mattsson et al., 2017). Similarly, after exposure periods of 24 h, Daphnia magna can become immobilized with reduced egg hatching rates and embryo development. Elsewhere, studies have shown that prolonged exposure to plastic particles ranging in size (20 nm to 5 μm) and long-term exposures (21 or 103 days of exposure) show reduced reproduction in crustaceans after exposure to polystyrene particles (Besseling et al., 2014; Kelpsiene et al., 2020).
While there is growing evidence that microplastics have effects on both freshwater and marine ecosystems there is a general paucity of studies from freshwater systems and those in tropical ecosystems (Wagner et al., 2014; Eerkes-Medrano et al., 2015; Scherer et al., 2018). The paucity of studies in freshwater systems and the tropics could be an artefact of biases towards marine research, the underfunding of research in inland waters (Maasri et al., 2022), and the general low research capacity in some of parts of the tropics (Marincola and Kariuki, 2020; Mutapi, 2021). The effects of microplastic on aquatic animals in the tropics are probably more pronounced considering many tropics, as in the whole world, are threatened by land use changes and anthropogenic global climate change (Ramírez et al., 2015; Vitule et al., 2017). In addition, to the threats of global warming and habitat degradation, tropical aquatic ecosystems are under increasing pressure from rapid population and economic growth and with that come the escalating problem of plastic and demand and waste disposal (Scherer et al., 2018; Donoso and Rios-Touma, 2020; Horton and Barnes, 2020; Álvarez-Lopeztello et al., 2021). To understand the full extent of the impacts of microplastics on aquatic animals there is need to include all regions including scanty data from freshwater systems and the aquatic systems in the tropics as a whole (Sarijan et al., 2021; Yardy et al., 2022).
Here I synthesize studies examining microplastics’ effects on aquatic fauna using a meta-analysis. I compiled data from empirical studies to test the following hypotheses: 1) microplastic exposure will negatively affect key functional traits (i.e, feeding, growth, reproduction, survival) with effects more pronounced in some taxa (e.g., filter feeders or omnivores), because some taxa are passive feeders and do not select the foods they eat 2) the effect on functional traits will be directly related to temperature, size of plastics and length of exposure. In addressing these hypotheses, I also considered how these findings may relate to tropical freshwater systems (where experiments and mesocosm studies are scanty). In addition, some research needs for freshwater aquatic systems with an emphasis on understudied tropical systems are also considered.
Methods
Literature search
Literature searches were conducted using the free software “Publish or Perish” (Harzing, 2007) to collect articles that could be suitable for data extraction. The search was aimed at collecting articles examining the effects of microplastics on aquatic organisms (fish and other aquatic invertebrates). To search for potential articles the following Boolean phrase was used: [(“plastic” OR “microplastic*” OR “micro plastic*” OR “micro-plastic*”) AND (“function*” OR “response*” OR “measure*” OR “rate*” OR “Feeding*” OR “Growth*” OR “Reproduction” OR “Survival*”) AND (“laborator*” OR “experiment*” OR “treatment*” OR “manipulat*”) AND (“benth*” OR “animals*” OR “taxa” OR “invertebrate” OR “fish”)]. The results of the search were further refined by choosing the range of publication years from 1900—2021. These publications years were selected to cover the wide range of studies that could potentially be included in the meta-analysis. The literature search yielded 958 results. During the screening, all extracted articles were checked for consistency with inclusion criteria following steps recommended by the Preferred Reporting Items for Systematic Reviews and Meta-Analysis (PRISMA; See Supplementary Table S1 in supplementary materials; Moher et al., 2009). Initially, screening of articles was based on titles, then abstracts, and finally full text screenings of all remaining articles. Using a Population, Intervention, Control, and Outcome framework (PICO; Huang et al., 2006), papers from that used empirical approaches (experiments or mesocosms) to quantify the effects of microplastics on feeding, survival, reproduction, and growth of aquatic organisms were selected for the meta-analyses (Population). During the scoping exercise, only the following studies were included: 1) studies that actually measured at least one response of organisms to microplastics with adequate replication (Intervention) 2) studies that measured faunal effects (Outcome), and included a control group with no exposure to microplastics (Control) 3) studies that reported mean, samples sizes and associated variances for controls and treatments. The reason for only including the aforementioned studies is that it would be easier to eliminate the effects of other factors that could affect the physiology of aquatic organisms. After considering the above criteria, 72 studies were included in the final analyses (see Supplementary for all data included in the final meta-analyses).
Data extraction
After literature sources were compiled, data were extracted from text, tables, or graphs. If the data were not reported in numbers, they were extracted from published diagrams using PlotDigitizer Version 3 (http://plotdigitizer.sourceforge.net/; Rohatgi, 2021). Data that presented the central tendency (mean or median if no mean was presented) in response to microplastic exposure were also extracted. If the study was an experimental manipulation, the data from the treatments containing the manipulation and the control were extracted. If a study tested multiple treatments (e.g., different concentrations of microplastics), all the data contained in that paper were extracted. If the authors described multiple experiments for a given response that were independent of each other, e.g., conducted experiments over two discrete periods, or if the authors reported results of a pilot study as well as the main study, those were included in the final analyses.
The literature search yielded studies with a range of response variables. Only studies including response variables most strongly related to feeding, growth, reproduction, and survival (see Table 1 for descriptions) and grouped different specific response variables into representative general categories were included. Those four responses were selected as they are the commonly measured variables in experiments and mesocosms. Some authors reported behavior but these were not always recorded in all studies, as such behavior could not be included as a measure. For each study, the following were documented: 1) the focal species, 2) the commonly designated functional feeding group of the focal species simplified into broad categories such as predator, omnivore, filter feeder 3) the response variable measured (feeding, growth, reproduction, survival), 4) abiotic and physical conditions of the experiment including microplastic type, size, temperature and length of exposure, and 5) the ecosystem type (freshwater versus marine) food web 6) whether the organism being tested was from the tropics or not.
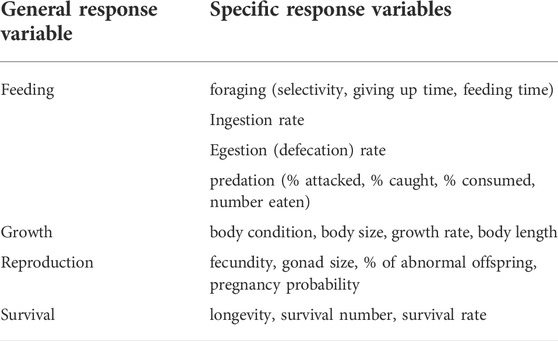
TABLE 1. Summary of the general categories of organism responses to microplastic exposure, and the specific description of response variables that comprise them.
Data analyses
To account for the differences in variables and reporting of results across all studies considered, effect sizes were measured using a Hedges’g statistic. The Hedges’g statistic is a standardized mean difference (SMD) between control and treatment that accounts for the heterogeneity in variables reported by dividing the SMD by the pooled standard deviation (Hedges, 1981; Buck et al., 2022). Because the sign of Hedges’g tells the direction of the effect, a negative value of Hedges’ g indicates that microplastics have a higher effect on impairing the specific trait considered. Hedges’g values of less than 0.2 represent small effects, values between 0.2 and 0.5 represent moderate effects, with values greater than 0.5 suggesting large effects (Borenstein et al., 2010).
All statistical models were performed using the “rma.mv” function of the metafor package in R. The “rma.mv” function uses a Wald-type test to determine statistical difference among tested groups (Viechtbauer, 2010; Saldaña-Vázquez and Munguía-Rosas, 2013). Mixed effects models were performed that included the study identification number (i.e., the ID of the study as reported in my dataset) and the functional traits (feeding, growth, reproduction, survival) as random effects to account for heterogeneity (Viechtbauer, 2007) and non-independence of results (Salerno et al., 2021). Effect sizes for the models including categorical fixed factors were considered to be statistically significant if their 95% confidence interval (CI) did not overlap with zero and if their alpha value (p) values were less than 0.05.
To examine the effects of temperature, and microplastic size a meta-regression was performed using the ‘lmer’ function from the lme4 library to perform mixed-effect regression analyses (Bates and Maechler, 2009).
All statistical analyses and graphing plotting were performed in R software (R Core Team, 2022, version 4.1.2, Vienna, Austria).
Results
Microplastic exposure to key functional traits (hypothesis 1)
Broadly, results revealed that the effect of microplastic exposure varies by taxonomic groupings (Figure 1). Nematode growth, reproduction, and survival were all moderately reduced by exposure to microplastics. Contrariwise, feeding had no effect on the feeding of nematodes. Rotifer exposure to microplastics largely affected their feeding, growth and survival, but exposure to microplastics, had a moderately positive effect on reproduction in rotifers. Annelid feeding, growth, and survival were not affected by exposure to microplastics. Exposure to microplastic had a large positive effect on the feeding and growth of aquatic insects while having no effect on reproduction and survival of aquatic insects. Microplastics negatively affected gastropod growth while it conversely had positive effects on gastropod survival. In gastropods, microplastics had no effect on reproduction. Crustacean and bivalve growth and reproduction were all moderately reduced when exposed to microplastics. Similarly, crustacean and bivalve exposure to microplastics reduced feeding. Exposure to microplastics did not affect survival of crustaceans. Fish growth and reproduction were largely negatively affected and marginally affected by exposure to microplastics, respectively. Exposure to microplastics had a marginal negative effect on feeding in fishes. Overall, microplastic exposure did not affect survival in most groups, it actually had positive effects on fishes and gastropods (Figure 1).
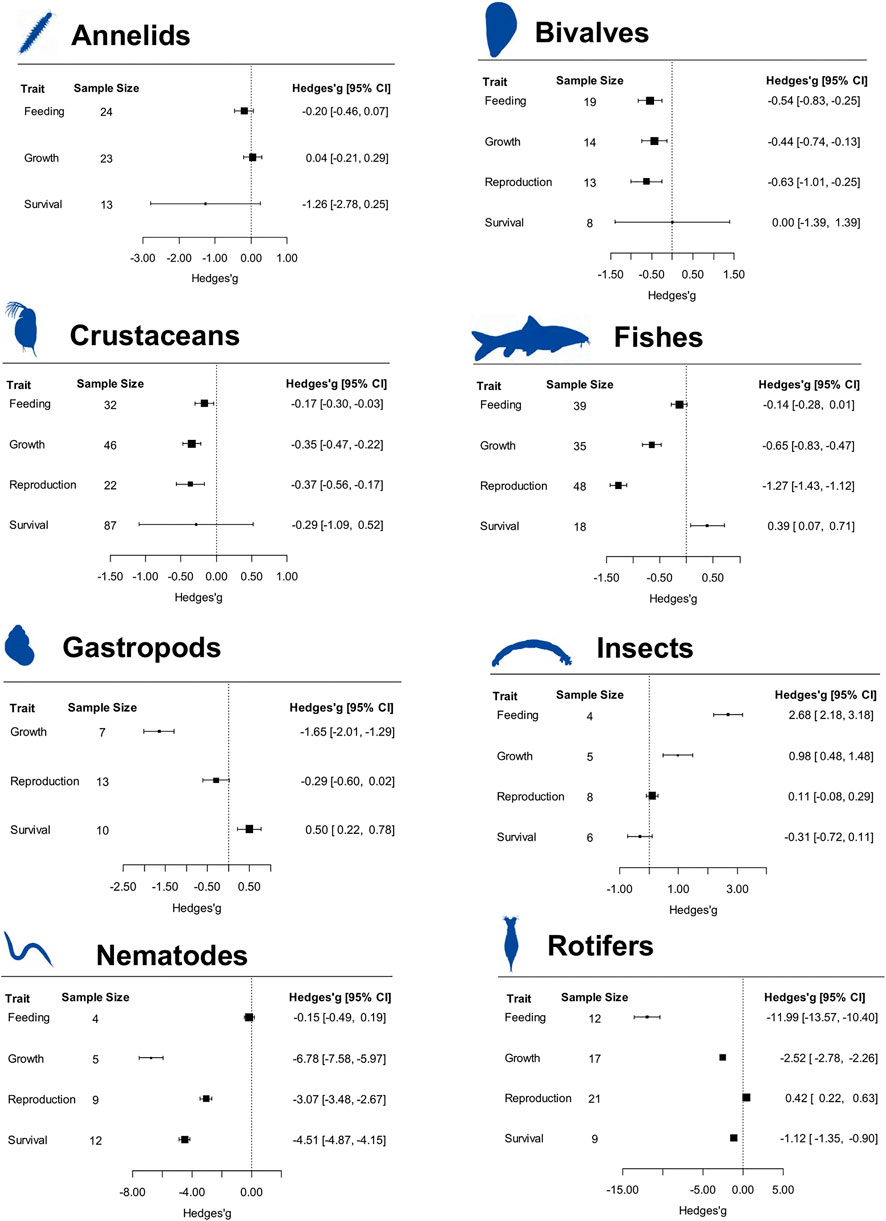
FIGURE 1. Forest plot of effect sizes of response categories based on taxonomic groupings. Analyses were conducted using a mixed-effects model. Black boxes denote the hedges’g value and horizontal lines denote the 95% confidence intervals (CI) for each hedges’g value. Hedges’g values of less than 0.2 represent small effects, values between 0.2 and 0.5 represent moderate effects, with values greater than 0.5 suggesting large effects. The relative size of the black boxes shows the relative effect size of the studies included in each analysis.
Among feeding modes, bacterivores (e.g., nematodes; Hedges’g = -2.71 ± 0.10), Omnivores (Hedges’g = -0.64 ± 0.04), and predators (many fishes; Hedges’g = -0.81 ± 0.12) were negatively affected to a larger extent than filter feeders (Hedges’g = -0.36 ± 0.05). Shredders were marginally affected by microplastics (Table 1). Grazers and deposit feeders were not affected by microplastics (Table 2).
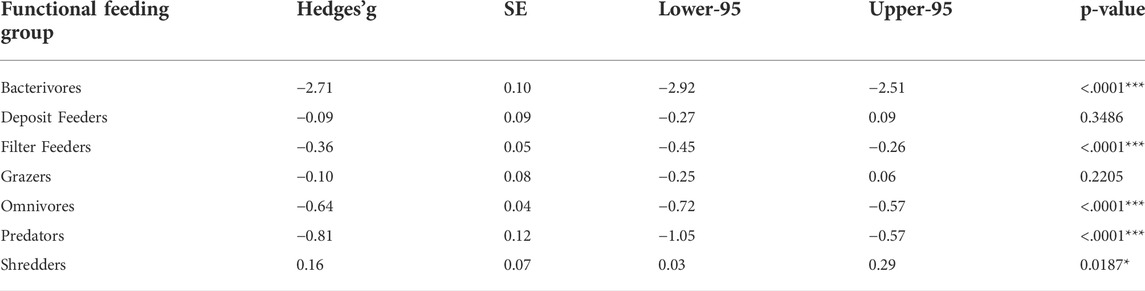
TABLE 2 . Effect sizes of microplastic responses of aquatic organisms based on their feeding modes included in the meta-analysis. Hedges’g values and associated p-values are also reported. Lower-95 and Upper -95 represent credibility intervals. Asterisks significant effects for each species *p < 0.05, **p < 0.01, ***p < 0.001. Hedges’g values of less than 0.2 represent small effects, values between 0.2 and 0.5 represent moderate effects, with values greater than 0.5 suggesting large effects.
In tropical freshwater systems (Figure 2B), exposure to microplastics had no effect on growth, survival, and feeding. Strikingly, microplastics had large positive effect on reproduction and feeding in tropical aquatic systems.
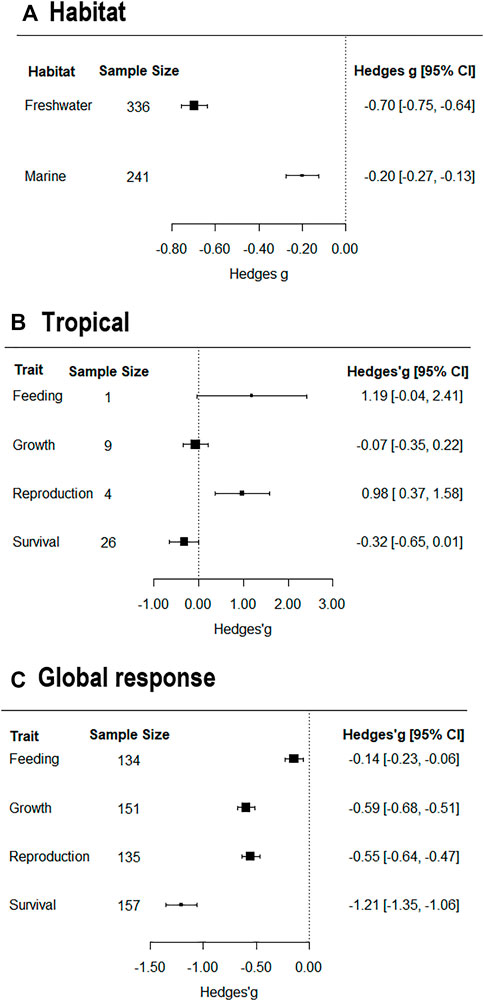
FIGURE 2. Forest plot of effect sizes by (A) Habitat (B) Tropical freshwater systems functional traits (C) Global response traits. Analyses were conducted using a mixed-effects model. Black boxes denote the hedges’g value and horizontal lines denote the 95% confidence intervals (CI) for each hedges’g value. Hedges’g values of less than 0.2 represent small effects, values between 0.2 and 0.5 represent moderate effects, with values greater than 0.5 suggesting large effects. The relative size of the black boxes shows the relative effect size of the studies included in each analysis.
Summarizing data across all groups (Figure 2C), shows that exposure to microplastics had a significantly negative effect on the feeding, growth, reproduction, and survival of organisms.
Effect of temperature, microplastic size, and length of exposure (hypothesis 2)
In general, temperature, microplastic size, and length of exposure had no effect on different taxonomic groups with a few exceptions (Table 3). Specifically, temperature was positively related to responses in annelids but negatively affected fish traits. Days of microplastic exposure only influenced rotifers (positive relationship) and annelids. Microplastic size only had effects on annelids.
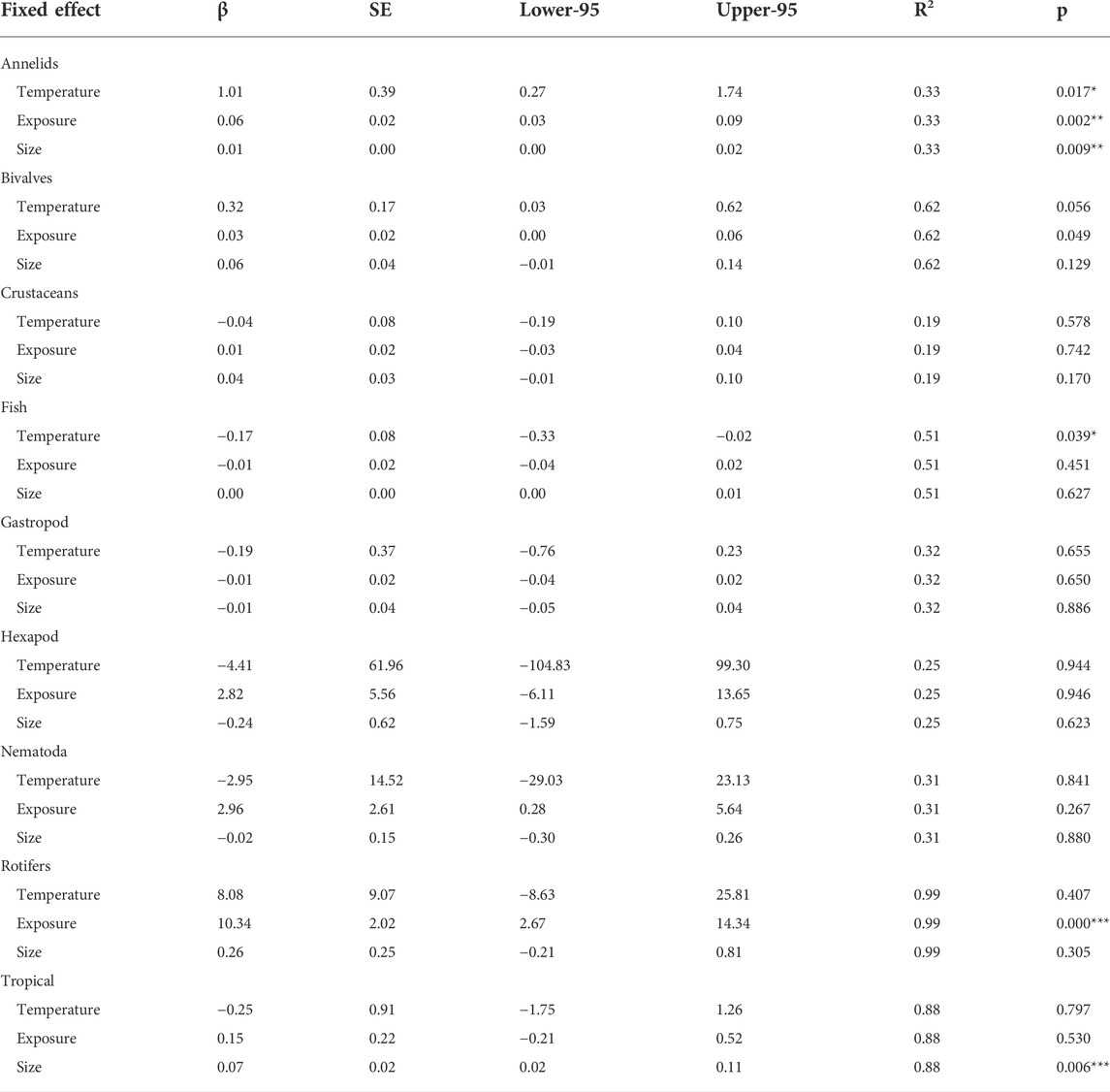
TABLE 3. Meta-regression analyses between effect size (dependent variable = Hedges’ g of microplastic response) and three independent variables extracted from the literature (temperature in oC, maximum exposure in days, microplastic size in µm). Beta (β) represents the slope of the best fit line. SE represent the standard error of β. Lower-95 and Upper -95 represent credibility intervals. R2 denotes the proportion of the variance explained by the regression model. p represents statistical significance. Asterisks significant effects for each species *p < 0.05, **p < 0.01, ***p < 0.001.
Species specific and habitat effect of microplastics
The effect of microplastics varied by species (Table 4). The extracted data, which covers 47 species shows significant negative effects on 23 of the species considered. The effects of microplastics ranged from moderate to large effects in all organisms considered.
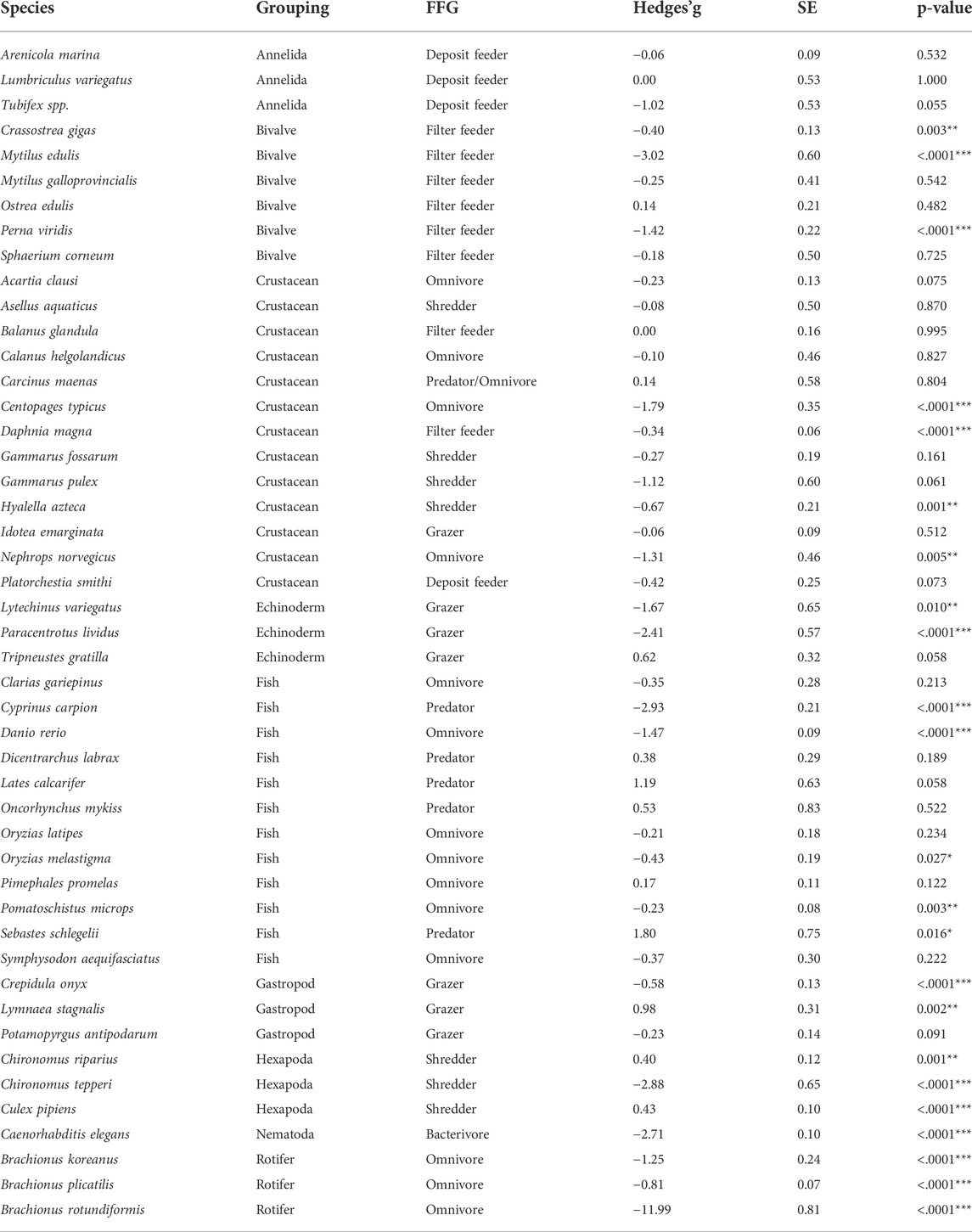
TABLE 4. Effect sizes of microplastic responses for each species included in the meta-analysis. Hedges’g values and associated p-values are also reported. Asterisks significant effects for each species *p < 0.05, **p < 0.01, ***p < 0.001. FFG denotes the functional feeding group. Hedges’g values of less than 0.2 represent small effects, values between 0.2 and 0.5 represent moderate effects, with values greater than 0.5 suggesting large effects.
Considering habitat effects, microplastics have a significant negative effect on organisms in both freshwater and marine systems (Figure 2A). Considering the effect size by habitat reveals that the effects of microplastics are greater in freshwater systems than marine systems.
Discussion
Microplastics are ubiquitous and questions remain on the full extent to which they are harmful to organisms (Galloway and Lewis, 2016; Issac and Kandasubramanian, 2021). Here, a global perspective of the potential effects of microplastics was explored using a plethora of data from different aquatic systems. This study revealed substantially negative effects of microplastics on growth, reproduction, survival and feeding regardless of habitat or species as documented in other studies (e.g., Yin et al., 2018; Salerno et al., 2021). One key finding from this work is that the effect of microplastics varies with taxa, and may negatively affect shredders and filter feeders; with the largest negative effects evident in omnivores, predators and bacterivores (hypothesis 1 partially supported). Congruent to previous research (e.g., Au et al., 2015; Foley et al., 2018; Salerno et al., 2021), the results demonstrate significant impacts of exposure to microplastics on functional traits, and highlight that temperature, microplastics size and length of exposure may not have detrimental effects on aquatic organisms (hypothesis 2 not supported).
Nematode growth, reproduction and survival was negatively affected by exposure to microplastics. The effects of microplastic on nematodes may be related to their linear digestive systems, and damage to the pharynx (and associated glands) that produce lubricants and digestive enzymes. Studies on nematode anatomy and physiology have shown that the consumption of microplastics causes the accumulation of microplastic in the intestine, damage to small intestine lining, oxidative stress, and increases in calcium levels; which subsequently leads to decreases in reproduction, survival and growth (Jeong et al., 2016; Lei et al., 2018). Researchers have shown that microplastic exposure to nematodes causes the inhibition of Glutathione S-transferase 4 (GST-4), an enzyme that plays roles in the detoxification and excretion of contaminants from cells (Kahn et al., 2008; Lei et al., 2018). The aforementioned factors may explain the negative effects of microplastics on nematodes.
Rotifer feeding was negatively affected by microplastics. Microplastics in rotifers interfere with normal food ingestion, and in addition, the particles function as a non-food item, providing no energy resource. Thus, microplastics effectively occupy space in the digestive tract, decreasing the available space for algal food. A similar study on zooplankton (Daphnia magna) determined that chronic exposure to microplastics led to reduction in fitness (number of offspring), because of inactivation of several digestive enzymes, which ultimately interfered with the animal’s nutrient supply (Trotter et al., 2021). The uniqueness of the reproduction systems in rotifers may explain why Rotifera reproduction actually increased. Considering that rotifers considered here are monogononts (they essentially reproduce by parthenogenesis) that can produce diploid eggs by mitosis (amictic), haploid eggs (mictic) by meiosis, haploid and diploid eggs simultaneously (amphoteric) or resting eggs (diapause eggs) when conditions are unfavourable (Stelzer, 2005), it is not surprising that their microplastics did not cause negative affect reproduction. The null effects of microplastic on rotifers, could be because rotifers exposed to microplastics may not obtain enough energy resources, thus leading to a significant reduction in reproduction (Van Cauwenberghe et al., 2015; Sun et al., 2019). The weakened overall performances of rotifers may inevitably be the mechanism that causes them to change in reproductive strategies.
Microplastics did not affect annelid feeding, growth, and survival. These finding are consistent with the results obtained by other researchers. For instance, Green et al. (2016) used annelids as model organisms (Arenicola marina) and demonstrated that microplastics (polyethylene and polylactic acid) had no effect on survivorship, biomass and behavior, although respiration rate was affected to an extent. It was expected that microplastics would have a significant effect on annelids considering the amount of microplastics consumed by annelids is higher than most herbivores, as suggested by other researchers (e.g., Parker et al., 2022). However, as has been demonstrated by many authors, it would seem that microplastics have no immediate effects on annelids because annelids process microplastics in similar ways as inorganic particles (e.g., sand grains). The digestive tract of most annelids can process inorganic material ingested along with organic particles (Jumars et al., 2015). For example, in some species of annelids (e.g., Saccocirrus and Arenicola marina) their straight digestive tracts (with cilia; Pechenik, 2014) prevent hindrances or blockages by microplastics and other indigestible particles. Additionally, the ability to quickly regenerate damaged tissues (Kostyuchenko and Kozin, 2021), may increase their resilience to physical injuries caused by the ingestion of these particles (Besseling et al., 2013). However, it must be noted, there may be a long-term trade-off of ingesting microplastics, which may affect the individual fitness of annelids. For instance, ingesting plastic may cause annelids to expend energy capturing, ingesting and expelling a particle that offers no nutritional value.
Exposure of microplastics did not affect reproduction and survival of aquatic insects as documented by many authors (Ziajahromi et al., 2018; Khosrovyan et al., 2020). Researchers have hypothesized that aquatic larvae may have an effective feeding strategy to avoid non-food material (Khosrovyan and Kahru, 2020). This hypothesis is tenable considering that some aquatic insects have been shown to preferentially select for high quality food rich in long-chain polyunsaturated fatty acids (Guo et al., 2018).
In concordance with Hämer et al. (2014), microplastic exposure did not have deleterious effects on gastropod reproduction and survival. However, the decreased growth in gastropods exposed to microplastics could be compensatory effect due to food depletion by an increased abundance of microplastic particles in the food and a subsequent reduction of energy reserves. Such effects have already been demonstrated in sediment feeding annelids (Wright et al., 2013a).
Exposure to microplastics had negative effects on feeding, growth, and reproduction of bivalves. Bivalves as filter feeders, use their gill cilia to drive water through their mantle cavity to obtain food (McElwain and Bullard, 2014). Ingestion of microplastics probably affects feeding by substantially decreasing water flow through blocking bivalve openings. These blockages potentially limit the uptake of food as well as gas exchange in bivalves. To date several works have demonstrated that mussels adjust the opening width of their valves to the amount of food particles in the water column and to the quality of the suspended particulate matter. An increase in the abundance of microplastics, which can potentially harm the animals by injuring epithelia or by causing blockages, leads to a rapid reduction of valve openings (Wright et al., 2013b; Rist et al., 2016). A reduction in feeding and of oxygen uptake by suspended inorganic particles ultimately results in reductions in energy availability and metabolism, which ultimately reduces growth. Because microplastics microplastic are absorbed into gill filaments and the gill septum of bivalves this may cause problems in the reproduction of bivalves (McElwain and Bullard, 2014). For instance, many bivalves (freshwater unionids) incubate their larvae during part of their development in “marsupials” formed by the septa within the gill’s filaments, therefore microplastic particles could impair reproduction by damaging gill filaments and gill septa (Pechenik, 2014).
Crustaceans (mostly zooplankton) and bivalves (many of which are filter feeders) were susceptible to microplastic exposure and their feeding, growth and reproduction were negatively affected (Figure 1). The effects of microplastic on feeding in filtering organism are reasonable considering that filter feeders do not choose their food and obtain food by filtering large volumes of water and usually display the highest quantities of microplastics in their digestive tracts (Sfriso et al., 2020). Considering crustaceans and bivalves are key to many aquatic food webs effects on the feeding and growth may affect consumers at higher trophic levels. Considering bivalves and crustaceans make up the bulk of seafood consumed by humans (Guillen et al., 2019; Hu and Chan, 2020) there is potential that these microplastics may be passed to humans (Smith et al., 2018), however this is beyond the scope of this work.
Fish, the only vertebrate included in this study, were negatively affected by microplastics (Figure 1). As vertebrates the effect of microplastics on fish may occur due to the buildup of ingested microplastics in their digestive tract, which ultimately leads to low caloric intake and malnutrition (Yin et al., 2018). Decreases in caloric intake are ultimately associated with decreases in growth and reproduction in fishes (Watts et al., 2016). Additionally, microplastics may block gills that may affect oxygen consumption and the movement of ions in and out of fishes. These blockages may affect the osmoregulation and respiration rates in fishes, which in turn may affect behavior and fitness as a whole (Li et al., 2018). For instance, fish exposed to microplastics have been shown to swim slower and catch fewer prey items than their conspecifics not exposed to plastics (Huang et al., 2022). In addition, fish that are exposed to microplastics have elevated levels of Gamma-Aminobutyric Acid (GABA), an inhibitory neurotransmitter that reduces excitability, in their brains. These high levels of GABA caused slower reaction times, and reduced ability to swim and catch prey (Huang et al., 2022).
Congruent to finding by Parolini et al. (2020), grazers and deposit-feeders were not significantly affected by microplastics. With regards to grazers many of them use their ‘teeth’ to scrap, grasp and tear the food from the benthos (Contreras and Castilla, 1987). Subsequently, many grazers will form boluses (Bonasoro and Carnevali, 1994) that may prevent fragmented and irregular plastics from damaging tissues of the digestive tracts. With regards to deposit feeders, that obtain their food on the surface and subsurface layers of sediment may not be affected by microplastics because many of deposit feeders have mucus-lined proboscis and tentacles that only sticks to small particles while not attaching to larger particles may reduce the effects of microplastics of deposit feeders (Lopez and Levinton, 1987; Wright et al., 2013b). Similarly, many deposit-feeders are able to rapidly egest materials that are not nutritionally beneficial (Lopez and Levinton, 1987; Pechenik, 2014).
Broadly, I found that microplastics had no effect on survival for most aquatic organisms. This may suggest that some organisms are able to withstand exposure to microplastics. A potential explanation for the none effects of microplastics observed may be related to gut morphology of some animals considered. For instance, detritivorous shredders, amphipods are evolutionary adapted to process non-digestible food components. The chitinous peritrophic membrane in crustaceans is secreted in the midgut where it encloses the food to protect the digestive system against particle-induced injuries (Pechenik, 2014). While there is no way from the studies analysed here to determine whether organisms selectively avoid microplastics, there is evidence in the literature that organism may potentially avoid microplastics when food is plenteous. For instance, sea urchins (Tripneustes gratilla) selected algae (their preferred food) over polyethylene microplastics when food was abundant (Kaposi et al., 2014). Elsewhere, marine copepods (Temora longicornis) selectively avoided 80% of microplastics after ‘tasting’ them with their mouth parts, almost indicative of taste discrimination between plastic and algae in zooplankton (Xu et al., 2022).
I found significant differences in effect sizes between freshwater and marine systems, with freshwater systems showing more negative effects (Figure 2B). The data reveal the ubiquity of microplastic in both systems and that the microplastic effects vary by habitat and may be more pronounced in freshwater systems than marine systems (sensu Scherer et al., 2018). These findings also support the assertion that aquatic organisms may be affected by microplastics globally, as evidenced by the number of studies that document microplastic occurrence globally. Currently, there are few studies that have documented the transfer of microplastics to higher trophic levels (but see (Nelms et al., 2018; Costa et al., 2020). The effects of microplastic transfer to higher trophic levels remains an opportunity for future research.
Tropical freshwater revealed that microplastic have no effect on feeding, growth and survival of organisms. These findings are mostly congruent with studies on microplastics in freshwater biota that occur in the tropics (summarized in Table 5). Studies on the potential adverse effects caused by microplastic exposure are scarce for freshwater compared to marine ecosystems. Some of our conclusions on freshwater systems and also the freshwater in the tropics may be limited by the few available studies. The few studies in freshwater organisms focus on water fleas (Daphnia magna; Ogonowski et al., 2016), amphipods (Hyalella Azteca and Gammarus pulex; Au et al., 2015; Weber et al., 2018), New Zealand mud snail (Potamopyrgus antipodarum; Imhof and Laforsch, 2016) as well as the African Catfish (Tongo and Erhunmwunse, 2022). Null effects in tropical freshwater systems may be related to crustacean gut morphology (as discussed above) and studies conducted over noticeably short microplastic exposures (1–28 days with most studies carried over 2 days). More studies assessing the acute and chronic effect of microplastic on freshwater organism are therefore warranted. This is particularly tenable considering that a study by Gallitelli et al. (2021) showed that microplastic over time can cause subtle changes in behavior in freshwater insects. Particularly, case building caddisflies (Odontocerum albicorne) constructed cases using microplastic polymers instead of natural items when presented with microplastics. Moreover, when presented with natural substrates and microplastics, mayflies (Ephemera Danica) were observed to burrow into microplastic substrates rather than natural ones. These preferences for microplastics versus natural substrates may not harm individual animals but may affect the animals that feed on some of these organisms. Indeed, insect larvae appear to not necessarily perceive microplastics as a direct stressor especially considering that freshwater insect feeding and growth was positively affected by microplastic exposure, but microplastic exposure had no effects on reproduction and survival (Figure 1). Further studies ought to be conducted to understand the chronic effects of microplastics on insects. Such, investigations should also focus on behavior (e.g., drift behavior) in invertebrates exposed to microplastics as these are the chronic effects that are common in some organisms exposed to microplastics (Issac and Kandasubramanian, 2021).
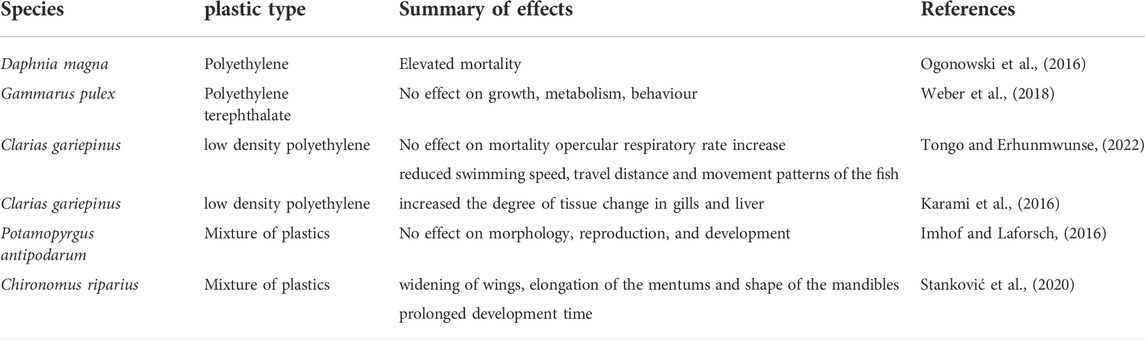
TABLE 5. Select studies documenting the effect of microplastics in freshwater systems. Only species that occur in the tropics are documented here.
Microplastic size, duration of exposure to microplastic, and temperature had no effects on the effect of microplastic exposure on fish and invertebrates. I would have expected the aforementioned factors to have significant effects on organism response to microplastics. Considering, climate change is already causing increased temperatures, extreme weather events, including tropical storms, which can redistribute microplastics between terrestrial, freshwater and marine environments (Chen et al., 2021), the effects of temperature of organisms may become more evident in the future. For instance, microplastic are known to increase in seawater and sediments after a severe storm (Wang et al., 2019). As such, stronger winds, rainfall, and sea level rises are likely to release plastics trapped in sediments. Researchers have demonstrated that flooding in rivers worsens riverine plastic pollution, with flood risk areas often becoming sites with high plastic accumulation during flooding events (Roebroek et al., 2021). Elsewhere, temperature rises (from 20 to 25°C), increased the microplastic induced mortality (from 8 to 33%). Authors should consider conducting controlled experiments across varying temperature regimes. Concerning the effect of microplastic sizes, it may be expedient for authors to not just report microplastic size but to report the weight (or volume) of microplastic particles used, because size alone does not actually consider the density of microplastics. Concerning duration, where most studies only occurred for 2 days it is very plausible that the exposure time was short in most studies. As such, it may be useful for authors to focus on more chronic effects (long term studies) as opposed to the acute (short-term) effects of microplastic exposure.
Here I focused on four main responses assessed out of the copious number of response recorded in many studies in the literature that include gene expression (Mazurais et al., 2015) and physical malformations (Pedà et al., 2016). The exposure to microplastics may have more indirect or subtle effects. For instance, microplastic ingestions may alter the community structure of microbiota in fishes (Zhang et al., 2021), and even humans (Tamargo et al., 2022). Since gut microbiota are shaped by diet: they have coevolved with its host to create a symbiotic relationship, whereby the gut community receives favorable conditions, whilst the host benefits from access to essential nutrients. As such, if the host’s diet is impaired by microplastics, the host will suffer from malnutrition, but its gut symbionts will also be afflicted by a lack of nutrients and substrate necessary to feed the community, potentially leading to shifts in gut community structure. Consequently, symbiotic bacteria may not be able to provide the same functions, especially considering that microbial gene expression is also linked to host diet (sensu Friberg et al., 2019). In addition, microplastics may have effects other than the four I considered. Specifically, microplastics may act as endocrine disruptors by releasing chemicals that mimic or antagonize sex hormones such estrogen (Yang et al., 2011). These chemicals may subsequently negatively impact reproduction (e.g., change in functions of reproductive organs, change in sex specific behaviors) and respiratory functions (Hu et al., 2020). Examining the aforementioned data in a way similar to the current study may be challenging, as quantification of these responses are sometimes qualitative (presence or absence of fluorescence) or not always reported in forms that can be easily extracted.
Additional considerations and conclusions
Here the meta-analysis considered studies from 1900 to 2021, and as such the results presented here may change with future perturbations and microplastic production. Considering the world experienced a global pandemic (Covid 19; Liu et al., 2020) that affected millions of people globally (Simonsen and Viboud, 2021). The use of plastic-based personal protection equipment has risen dramatically since the COVID-19 pandemic (De-la-Torre and Aragaw, 2021). Surgical masks are made from various polymer materials (e.g., polyethylene, polyester, etc.). The use of plastic-based personal protection devices (such as N95 masks) during pandemic increases plastic waste, which may end up in aquatic systems. The constant use of plastic made protection devices will continue to increase and may potentially change the interpretation of my results in the future owing to potential deposition of microplastic into aquatic systems (De-la-Torre and Aragaw, 2021). A pandemic (Liu et al., 2020), anthropogenic climate change (Fawzy et al., 2020) and an increasing human population (Sadigov, 2022) will all change the potential effects of microplastics on aquatic biota.
Comparing the distribution of studies in the literature -where tropics and the African continent underrepresented- there is a need for more controlled studies examining the effects of microplastics on fauna in the tropics and the African continent. Some of the immediate needs for tropical aquatic systems include and are not limited to long-term multiple species studies that examine effects of microplastic, the potential of microplastic transfer to higher trophic levels and how microplastics can alter the ethology of organisms. Similarly, standardization of concentrations and exposure conditions are needed from several aquatic systems to allow for more comparable data. Specifically, methods for reporting microplastic concentrations varied considerable among studies, impeding the analysis of microplastic concentration as moderator variable. Given that many authors report the concentration microplastics as number of microplastic pieces per unit volume (e.g., de Sá et al., 2015; Blarer and Burkhardt-Holm, 2016), while others report weight of microplastic per unit volume (e.g., Fonte et al., 2016), and many others report percent plastic by weight of sediment and/or food (e.g., Hämer et al., 2014). While standardization of how concentrations are reported may be difficult across many academic fields in microplastic research, researchers should think about reporting both the size and weight of microplastics as density per unit volume (detailed in Phuong et al., 2016). Additionally, authors should consider studies from various freshwater systems with varying limnological characteristics (e.g., stormwater wetlands, natural lakes, streams and rivers with varying flowing regimes).
Overall, the findings here demonstrate that the effects of microplastics vary by species and feeding mode. The differences may be mediated depend on the taxa as well as the anatomy and physiology of the organism in question. Additionally, microplastics coupled with contaminates that adhere to them may pose an additional threat to freshwater and terrestrial biota (Colabuono et al., 2010; Tanaka et al., 2013; Carbery et al., 2018). Further, research is required on how different species are affected in the field, where they encounter different microplastic types of varied sizes and shapes throughout their lifetime. There is a need to consider other physiological effects such as those presented here especially when considering the nexus between plastics ecosystem structure and function. Addressing questions on the potential threat of microplastics for freshwater environments is crucial for policy makers to develop appropriate and informed measures to address microplastics contamination in freshwater systems.
Data availability statement
The original contributions presented in the study are included in the article/Supplementary Material, further inquiries can be directed to the corresponding author.
Author contributions
The author confirms being the sole contributor of this work and has approved it for publication.
Funding
This study was funded from start-up funds provided to SM by Rhodes College. The APC was funded by the Rhodes College Department of Biology.
Acknowledgments
I am grateful for all the authors whose hard work made these data available for this study. I am also grateful for the assistance of Gabriele M. Dagan who helped with data extraction.
Conflict of interest
The author declares that the research was conducted in the absence of any commercial or financial relationships that could be construed as a potential conflict of interest.
Publisher’s note
All claims expressed in this article are solely those of the authors and do not necessarily represent those of their affiliated organizations, or those of the publisher, the editors and the reviewers. Any product that may be evaluated in this article, or claim that may be made by its manufacturer, is not guaranteed or endorsed by the publisher.
Supplementary material
The Supplementary Material for this article can be found online at: https://www.frontiersin.org/articles/10.3389/fenvs.2022.999349/full#supplementary-material
References
Ahechti, M., Benomar, M., El Alami, M., and Mendiguchía, C. (2022). Metal adsorption by microplastics in aquatic environments under controlled conditions: Exposure time, pH, and salinity. Int. J. Environ. Anal. Chem. 102, 1118–1125. doi:10.1080/03067319.2020.1733546
Álvarez-Lopeztello, J., Robles, C., and del Castillo, R. F. (2021). Microplastic pollution in neotropical rainforest, savanna, pine plantations, and pasture soils in lowland areas of Oaxaca, Mexico: Preliminary results. Ecol. Indic. 121, 107084. doi:10.1016/j.ecolind.2020.107084
Andrady, A. L. (2011). Microplastics in the marine environment. Mar. Pollut. Bull. 62, 1596–1605. doi:10.1016/j.marpolbul.2011.05.030
Au, S. Y., Bruce, T. F., Bridges, W. C., and Klaine, S. J. (2015). Responses of Hyalella azteca to acute and chronic microplastic exposures: Effects of Microplastic Exposure on Hyalella azteca. Environ. Toxicol. Chem. 34, 2564–2572. doi:10.1002/etc.3093
Avio, C. G., Gorbi, S., Milan, M., Benedetti, M., Fattorini, D., d’Errico, G., et al. (2015). Pollutants bioavailability and toxicological risk from microplastics to marine mussels. Environ. Pollut. 198, 211–222. doi:10.1016/j.envpol.2014.12.021
Bakir, A., Rowland, S. J., and Thompson, R. C. (2014). Enhanced desorption of persistent organic pollutants from microplastics under simulated physiological conditions. Environ. Pollut. 185, 16–23. doi:10.1016/j.envpol.2013.10.007
Besseling, E., Wang, B., Lürling, M., and Koelmans, A. A. (2014). Nanoplastic affects growth of S. obliquus and reproduction of D. magna. Environ. Sci. Technol. 48, 12336–12343. doi:10.1021/es503001d
Besseling, E., Wegner, A., Foekema, E. M., van den Heuvel-Greve, M. J., and Koelmans, A. A. (2013). Effects of microplastic on fitness and PCB bioaccumulation by the Lugworm Arenicola marina (L.). Environ. Sci. Technol. 47, 593–600. doi:10.1021/es302763x
Biginagwa, F. J., Mayoma, B. S., Shashoua, Y., Syberg, K., and Khan, F. R. (2016). First evidence of microplastics in the african great lakes: Recovery from lake victoria nile perch and nile tilapia. J. Gt. Lakes. Res. 42, 146–149. doi:10.1016/j.jglr.2015.10.012
Blarer, P., and Burkhardt-Holm, P. (2016). Microplastics affect assimilation efficiency in the freshwater amphipod Gammarus fossarum. Environ. Sci. Pollut. Res. 23, 23522–23532. doi:10.1007/s11356-016-7584-2
Bonasoro, F., and Carnevali, M. D. C. (1994). Atypical chordoid structures in the aristotle’s lantern of regular echinoids. Acta Zool. 75, 89–100. doi:10.1111/j.1463-6395.1994.tb01114.x
Borenstein, M., Hedges, L. V., Higgins, J. P. T., and Rothstein, H. R. (2010). A basic introduction to fixed-effect and random-effects models for meta-analysis. Res. Synth. Methods 1, 97–111. doi:10.1002/jrsm.12
Brahney, J., Hallerud, M., Heim, E., Hahnenberger, M., and Sukumaran, S. (2020). Plastic rain in protected areas of the United States. Science 368, 1257–1260. doi:10.1126/science.aaz5819
Brown, J. H., Gillooly, J. F., Allen, A. P., Savage, V. M., and West, G. B. (2004). Toward a metabolic theory of ecology. Ecology 85, 1771–1789. doi:10.1890/03-9000
Bucci, K., Tulio, M., and Rochman, C. M. (2020). What is known and unknown about the effects of plastic pollution: A meta-analysis and systematic review. Ecol. Appl. 30, e02044. doi:10.1002/eap.2044
Buck, R. J., Fieberg, J., and Larkin, D. J. (2022). The use of weighted averages of Hedges’ d in meta-analysis: Is it worth it? Methods Ecol. Evol. 13, 1093–1105. doi:10.1111/2041-210X.13818
Cao, Y., Zhao, M., Ma, X., Song, Y., Zuo, S., Li, H., et al. (2021). A critical review on the interactions of microplastics with heavy metals: Mechanism and their combined effect on organisms and humans. Sci. Total Environ. 788, 147620. doi:10.1016/j.scitotenv.2021.147620
Carbery, M., O’Connor, W., and Palanisami, T. (2018). Trophic transfer of microplastics and mixed contaminants in the marine food web and implications for human health. Environ. Int. 115, 400–409. doi:10.1016/j.envint.2018.03.007
Chen, L., Li, J., Tang, Y., Wang, S., Lu, X., Cheng, Z., et al. (2021). Typhoon-induced turbulence redistributed microplastics in coastal areas and reformed plastisphere community. Water Res. 204, 117580. doi:10.1016/j.watres.2021.117580
Colabuono, F. I., Taniguchi, S., and Montone, R. C. (2010). Polychlorinated biphenyls and organochlorine pesticides in plastics ingested by seabirds. Mar. Pollut. Bull. 60, 630–634. doi:10.1016/j.marpolbul.2010.01.018
Cole, M., Lindeque, P., Fileman, E., Halsband, C., Goodhead, R., Moger, J., et al. (2013). Microplastic ingestion by zooplankton. Environ. Sci. Technol. 47, 6646–6655. doi:10.1021/es400663f
Contreras, S., and Castilla, J. (1987). Feeding behavior and morphological adaptations in two sympatric sea urchin species in central Chile. Mar. Ecol. Prog. Ser. 38, 217–224. doi:10.3354/meps038217
Costa, E., Piazza, V., Lavorano, S., Faimali, M., Garaventa, F., and Gambardella, C. (2020). Trophic transfer of microplastics from copepods to jellyfish in the marine environment. Front. Environ. Sci. 8, 571732. doi:10.3389/fenvs.2020.571732
de Sá, L. C., Luís, L. G., and Guilhermino, L. (2015). Effects of microplastics on juveniles of the common goby (Pomatoschistus microps): Confusion with prey, reduction of the predatory performance and efficiency, and possible influence of developmental conditions. Environ. Pollut. 196, 359–362. doi:10.1016/j.envpol.2014.10.026
De-la-Torre, G. E., and Aragaw, T. A. (2021). What we need to know about PPE associated with the COVID-19 pandemic in the marine environment. Mar. Pollut. Bull. 163, 111879. doi:10.1016/j.marpolbul.2020.111879
Desforges, J.-P. W., Galbraith, M., and Ross, P. S. (2015). Ingestion of microplastics by zooplankton in the northeast pacific ocean. Arch. Environ. Contam. Toxicol. 69, 320–330. doi:10.1007/s00244-015-0172-5
Dinani, F. S. H., Baradaran, A., and Ebrahimpour, K. (2021). Acute toxic effects of polyurethane microplastics on adult Zebra fish (Danio rerio). Int. J. Environ. Health Eng. 10, 9. doi:10.4103/ijehe.ijehe_12_21
Donoso, J. M., and Rios-Touma, B. (2020). Microplastics in tropical andean rivers: A perspective from a highly populated Ecuadorian basin without wastewater treatment. Heliyon 6, e04302. doi:10.1016/j.heliyon.2020.e04302
Dris, R., Gasperi, J., Mirande, C., Mandin, C., Guerrouache, M., Langlois, V., et al. (2017). A first overview of textile fibers, including microplastics, in indoor and outdoor environments. Environ. Pollut. 221, 453–458. doi:10.1016/j.envpol.2016.12.013
Eerkes-Medrano, D., Thompson, R. C., and Aldridge, D. C. (2015). Microplastics in freshwater systems: A review of the emerging threats, identification of knowledge gaps and prioritisation of research needs. Water Res. 75, 63–82. doi:10.1016/j.watres.2015.02.012
Eom, H.-J., Nam, S.-E., and Rhee, J.-S. (2020). Polystyrene microplastics induce mortality through acute cell stress and inhibition of cholinergic activity in a brine shrimp. Mol. Cell. Toxicol. 16, 233–243. doi:10.1007/s13273-020-00088-4
Fawzy, S., Osman, A. I., Doran, J., and Rooney, D. W. (2020). Strategies for mitigation of climate change: A review. Environ. Chem. Lett. 18, 2069–2094. doi:10.1007/s10311-020-01059-w
Foley, C. J., Feiner, Z. S., Malinich, T. D., and Höök, T. O. (2018). A meta-analysis of the effects of exposure to microplastics on fish and aquatic invertebrates. Sci. Total Environ. 631–632, 550–559. doi:10.1016/j.scitotenv.2018.03.046
Fonte, E., Ferreira, P., and Guilhermino, L. (2016). Temperature rise and microplastics interact with the toxicity of the antibiotic cefalexin to juveniles of the common goby ( Pomatoschistus microps ): Post-exposure predatory behaviour, acetylcholinesterase activity and lipid peroxidation. Aquat. Toxicol. 180, 173–185. doi:10.1016/j.aquatox.2016.09.015
Friberg, I. M., Taylor, J. D., and Jackson, J. A. (2019). Diet in the driving seat: Natural diet-immunity-microbiome interactions in wild fish. Front. Immunol. 10, 243. doi:10.3389/fimmu.2019.00243
Gallitelli, L., Cera, A., Cesarini, G., Pietrelli, L., and Scalici, M. (2021). Preliminary indoor evidences of microplastic effects on freshwater benthic macroinvertebrates. Sci. Rep. 11, 720. doi:10.1038/s41598-020-80606-5
Galloway, T. S., and Lewis, C. N. (2016). Marine microplastics spell big problems for future generations. Proc. Natl. Acad. Sci. U. S. A. 113, 2331–2333. doi:10.1073/pnas.1600715113
Gasperi, J., Wright, S. L., Dris, R., Collard, F., Mandin, C., Guerrouache, M., et al. (2018). Microplastics in air: Are we breathing it in? Curr. Opin. Environ. Sci. Health 1, 1–5. doi:10.1016/j.coesh.2017.10.002
Gaylarde, C. C., Neto, J. A. B., and da Fonseca, E. M. (2021). Paint fragments as polluting microplastics: A brief review. Mar. Pollut. Bull. 162, 111847. doi:10.1016/j.marpolbul.2020.111847
Geyer, R., Jambeck, J. R., and Law, K. L. (2017). Production, use, and fate of all plastics ever made. Sci. Adv. 3, e1700782. doi:10.1126/sciadv.1700782
Green, D. S., Boots, B., Sigwart, J., Jiang, S., and Rocha, C. (2016). Effects of conventional and biodegradable microplastics on a marine ecosystem engineer (Arenicola marina) and sediment nutrient cycling. Environ. Pollut. 208, 426–434. doi:10.1016/j.envpol.2015.10.010
Gregory, M. R. (1978). Accumulation and distribution of virgin plastic granules on New Zealand beaches. N. Z. J. Mar. Freshw. Res. 12, 399–414. doi:10.1080/00288330.1978.9515768
Greven, A.-C., Merk, T., Karagöz, F., Mohr, K., Klapper, M., Jovanović, B., et al. (2016). Polycarbonate and polystyrene nanoplastic particles act as stressors to the innate immune system of fathead minnow (Pimephales promelas). Environ. Toxicol. Chem. 35, 3093–3100. doi:10.1002/etc.3501
Guillen, J., Natale, F., Carvalho, N., Casey, J., Hofherr, J., Druon, J.-N., et al. (2019). Global seafood consumption footprint. Ambio 48, 111–122. doi:10.1007/s13280-018-1060-9
Guo, F., Bunn, S. E., Brett, M. T., Fry, B., Hager, H., Ouyang, X., et al. (2018). Feeding strategies for the acquisition of high-quality food sources in stream macroinvertebrates: Collecting, integrating, and mixed feeding. Limnol. Oceanogr. 63, 1964–1978. doi:10.1002/lno.10818
Haave, M., and Henriksen, T. (2022). “Sources and fate of microplastics in urban systems,” in Handbook of microplastics in the environment. Editors T. Rocha-Santos, M. F. Costa, and C. Mouneyrac (Cham: Springer International Publishing), 849–875. doi:10.1007/978-3-030-39041-9_44
Hämer, J., Gutow, L., Köhler, A., and Saborowski, R. (2014). Fate of microplastics in the marine Isopod Idotea emarginata. Environ. Sci. Technol. 48, 13451–13458. doi:10.1021/es501385y
Harzing, A. W. (2007). Publish or perish on macOS. Available at: available from: https://harzing.com/resources/publish-or-perish.
Hedges, L. V. (1981). Distribution theory for glass’s estimator of effect size and related estimators. J. Educ. Statistics 6, 107–128. doi:10.2307/1164588
Hiltunen, M., Honkanen, M., Taipale, S., Strandberg, U., and Kankaala, P. (2017). Trophic upgrading via the microbial food web may link terrestrial dissolved organic matter to Daphnia. J. Plankton Res. 39, 861–869. doi:10.1093/plankt/fbx050
Horn, D. A., Granek, E. F., and Steele, C. L. (2020). Effects of environmentally relevant concentrations of microplastic fibers on Pacific mole crab ( Emerita analoga ) mortality and reproduction. Limnol. Oceanogr. Lett. 5, 74–83. doi:10.1002/lol2.10137
Horton, A. A., and Barnes, D. K. A. (2020). Microplastic pollution in a rapidly changing world: Implications for remote and vulnerable marine ecosystems. Sci. Total Environ. 738, 140349. doi:10.1016/j.scitotenv.2020.140349
Hu, L., Chernick, M., Lewis, A. M., Ferguson, P. L., and Hinton, D. E. (2020). Chronic microfiber exposure in adult Japanese medaka (Oryzias latipes). PLOS ONE 15, e0229962. doi:10.1371/journal.pone.0229962
Hu, X. F., and Chan, H. M. (2020). Seafood consumption and its contribution to nutrients intake among Canadians in 2004 and 2015. Nutrients 13, 77. doi:10.3390/nu13010077
Huang, X., Lin, J., and Demner-Fushman, D. (2006). Evaluation of PICO as a knowledge representation for clinical questions. AMIA Annu. Symp. Proc. 2006, 359–363.
Huang, J.-N., Wen, B., Xu, L., Ma, H.-C., Li, X.-X., Gao, J.-Z., et al. (2022). Micro/nano-plastics cause neurobehavioral toxicity in discus fish (Symphysodon aequifasciatus): Insight from brain-gut-microbiota axis. J. Hazard. Mat. 421, 126830. doi:10.1016/j.jhazmat.2021.126830
Imhof, H. K., and Laforsch, C. (2016). Hazardous or not – are adult and juvenile individuals of Potamopyrgus antipodarum affected by non-buoyant microplastic particles? Environ. Pollut. 218, 383–391. doi:10.1016/j.envpol.2016.07.017
IPCC (2022). “Summary for policymakers,” in Climate change and land: An IPCC special report on climate change, desertification, land degradation, sustainable land management, food security, and greenhouse gas fluxes in terrestrial ecosystems. Editors P. R. Shukla, J. Skea, E. Calvo Buendia, V. Masson-Delmotte, H.- O. Pörtner, D. C. Robertset al. Available at: https://www.ipcc.ch/srccl/(Accessed February 19, 2021).
Issac, M. N., and Kandasubramanian, B. (2021). Effect of microplastics in water and aquatic systems. Environ. Sci. Pollut. Res. 28, 19544–19562. doi:10.1007/s11356-021-13184-2
Jaikumar, G., Baas, J., Brun, N. R., Vijver, M. G., and Bosker, T. (2018). Acute sensitivity of three Cladoceran species to different types of microplastics in combination with thermal stress. Environ. Pollut. 239, 733–740. doi:10.1016/j.envpol.2018.04.069
Jeong, C.-B., Won, E.-J., Kang, H.-M., Lee, M.-C., Hwang, D.-S., Hwang, U.-K., et al. (2016). Microplastic size-dependent toxicity, oxidative stress induction, and p-JNK and p-p38 activation in the monogonont rotifer ( Brachionus koreanus ). Environ. Sci. Technol. 50, 8849–8857. doi:10.1021/acs.est.6b01441
Jumars, P. A., Dorgan, K. M., and Lindsay, S. M. (2015). Diet of worms emended: An update of polychaete feeding guilds. Annu. Rev. Mar. Sci. 7, 497–520. doi:10.1146/annurev-marine-010814-020007
Kahn, N. W., Rea, S. L., Moyle, S., Kell, A., and Johnson, T. E. (2008). Proteasomal dysfunction activates the transcription factor SKN-1 and produces a selective oxidative-stress response in Caenorhabditis elegans. Biochem. J. 409, 205–213. doi:10.1042/BJ20070521
Kaposi, K. L., Mos, B., Kelaher, B. P., and Dworjanyn, S. A. (2014). Ingestion of microplastic has limited impact on a marine Larva. Environ. Sci. Technol. 48, 1638–1645. doi:10.1021/es404295e
Karami, A., Romano, N., Galloway, T., and Hamzah, H. (2016). Virgin microplastics cause toxicity and modulate the impacts of phenanthrene on biomarker responses in African catfish (Clarias gariepinus). Environ. Res. 151, 58–70. doi:10.1016/j.envres.2016.07.024
Kelpsiene, E., Torstensson, O., Ekvall, M. T., Hansson, L.-A., and Cedervall, T. (2020). Long-term exposure to nanoplastics reduces life-time in Daphnia magna. Sci. Rep. 10, 5979. doi:10.1038/s41598-020-63028-1
Khosrovyan, A., Gabrielyan, B., and Kahru, A. (2020). Ingestion and effects of virgin polyamide microplastics on Chironomus riparius adult larvae and adult zebrafish Danio rerio. Chemosphere 259, 127456. doi:10.1016/j.chemosphere.2020.127456
Khosrovyan, A., and Kahru, A. (2020). Evaluation of the hazard of irregularly-shaped co-polyamide microplastics on the freshwater non-biting midge Chironomus riparius through its life cycle. Chemosphere 244, 125487. doi:10.1016/j.chemosphere.2019.125487
Kögel, T., Bjorøy, Ø., Toto, B., Bienfait, A. M., and Sanden, M. (2020). Micro- and nanoplastic toxicity on aquatic life: Determining factors. Sci. Total Environ. 709, 136050. doi:10.1016/j.scitotenv.2019.136050
Kostyuchenko, R. P., and Kozin, V. V. (2021). Comparative aspects of annelid regeneration: Towards understanding the mechanisms of regeneration. Genes 12, 1148. doi:10.3390/genes12081148
Kratina, P., Watts, T. J., Green, D. S., Kordas, R. L., and O’Gorman, E. J. (2019). Interactive effects of warming and microplastics on metabolism but not feeding rates of a key freshwater detritivore. Environ. Pollut. 255, 113259. doi:10.1016/j.envpol.2019.113259
Lavers, J. L., and Bond, A. L. (2017). Exceptional and rapid accumulation of anthropogenic debris on one of the world’s most remote and pristine islands. Proc. Natl. Acad. Sci. U. S. A. 114, 6052–6055. doi:10.1073/pnas.1619818114
Lei, L., Wu, S., Lu, S., Liu, M., Song, Y., Fu, Z., et al. (2018). Microplastic particles cause intestinal damage and other adverse effects in zebrafish Danio rerio and nematode Caenorhabditis elegans. Sci. Total Environ. 619-620, 1–8. doi:10.1016/j.scitotenv.2017.11.103
Li, M., Wang, X., Qi, C., Li, E., Du, Z., Qin, J. G., et al. (2018). Metabolic response of Nile tilapia (Oreochromis niloticus) to acute and chronic hypoxia stress. Aquaculture 495, 187–195. doi:10.1016/j.aquaculture.2018.05.031
Liu, Y.-C., Kuo, R.-L., and Shih, S.-R. (2020). COVID-19: The first documented coronavirus pandemic in history. Biomed. J. 43, 328–333. doi:10.1016/j.bj.2020.04.007
Lopez, G. R., and Levinton, J. S. (1987). Ecology of deposit-feeding animals in marine sediments. Q. Rev. Biol. 62, 235–260. doi:10.1086/415511
Lu, Y., Zhang, Y., Deng, Y., Jiang, W., Zhao, Y., Geng, J., et al. (2016). Uptake and accumulation of polystyrene microplastics in zebrafish (Danio rerio) and toxic effects in liver. Environ. Sci. Technol. 50, 4054–4060. doi:10.1021/acs.est.6b00183
Lusher, A. L., Welden, N. A., Sobral, P., and Cole, M. (2017). Sampling, isolating and identifying microplastics ingested by fish and invertebrates. Anal. Methods 9, 1346–1360. doi:10.1039/C6AY02415G
Maasri, A., Jähnig, S. C., Adamescu, M. C., Adrian, R., Baigun, C., Baird, D. J., et al. (2022). A global agenda for advancing freshwater biodiversity research. Ecol. Lett. 25, 255–263. doi:10.1111/ele.13931
Marincola, E., and Kariuki, T. (2020). Quality research in africa and why it is important. ACS Omega 5, 24155–24157. doi:10.1021/acsomega.0c04327
Mattsson, K., Johnson, E. V., Malmendal, A., Linse, S., Hansson, L.-A., and Cedervall, T. (2017). Brain damage and behavioural disorders in fish induced by plastic nanoparticles delivered through the food chain. Sci. Rep. 7, 11452. doi:10.1038/s41598-017-10813-0
Mazurais, D., Ernande, B., Quazuguel, P., Severe, A., Huelvan, C., Madec, L., et al. (2015). Evaluation of the impact of polyethylene microbeads ingestion in European sea bass (Dicentrarchus labrax) larvae. Mar. Environ. Res. 112, 78–85. doi:10.1016/j.marenvres.2015.09.009
McElwain, A., and Bullard, S. A. (2014). Histological atlas of freshwater mussels (Bivalvia, unionidae): Villosa nebulosa (ambleminae: Lampsilini), fusconaia cerina (ambleminae: Pleurobemini) and strophitus connasaugaensis (unioninae: Anodontini). Malacologia 57, 99–239. doi:10.4002/040.057.0104
Moher, D., Liberati, A., Tetzlaff, J., and Altman, D. G. (2009). Preferred reporting items for systematic reviews and meta-analyses: The PRISMA statement. BMJ 339, b2535. doi:10.1136/bmj.b2535
Mutapi, F. (2021). Africa needs to speed up research excellence: here’s how. Conversat. Available at: http://theconversation.com/africa-needs-to-speed-up-research-excellence-heres-how-169552 (Accessed August 31, 2022).
Napper, I. E., and Thompson, R. C. (2016). Release of synthetic microplastic plastic fibres from domestic washing machines: Effects of fabric type and washing conditions. Mar. Pollut. Bull. 112, 39–45. doi:10.1016/j.marpolbul.2016.09.025
Nelms, S. E., Galloway, T. S., Godley, B. J., Jarvis, D. S., and Lindeque, P. K. (2018). Investigating microplastic trophic transfer in marine top predators. Environ. Pollut. 238, 999–1007. doi:10.1016/j.envpol.2018.02.016
Ogonowski, M., Schür, C., Jarsén, Å., and Gorokhova, E. (2016). The effects of natural and anthropogenic Microparticles on individual fitness in Daphnia magna. PLOS ONE 11, e0155063. doi:10.1371/journal.pone.0155063
Oliveira, M., Ribeiro, A., Hylland, K., and Guilhermino, L. (2013). Single and combined effects of microplastics and pyrene on juveniles (0+ group) of the common goby Pomatoschistus microps (Teleostei, Gobiidae). Ecol. Indic. 34, 641–647. doi:10.1016/j.ecolind.2013.06.019
Parker, B., Britton, J. R., Pabortsava, K., Barrow, M., Green, I. D., Dominguez Almela, V., et al. (2022). Distinct microplastic patterns in the sediment and biota of an urban stream. Sci. Total Environ. 838, 156477. doi:10.1016/j.scitotenv.2022.156477
Parolini, M., Ferrario, C., De Felice, B., Gazzotti, S., Bonasoro, F., Candia Carnevali, M. D., et al. (2020). Interactive effects between sinking polyethylene terephthalate (PET) microplastics deriving from water bottles and a benthic grazer. J. Hazard. Mat. 398, 122848. doi:10.1016/j.jhazmat.2020.122848
Paul-Pont, I., Lacroix, C., González Fernández, C., Hégaret, H., Lambert, C., Le Goïc, N., et al. (2016). Exposure of marine mussels Mytilus spp. to polystyrene microplastics: Toxicity and influence on fluoranthene bioaccumulation. Environ. Pollut. 216, 724–737. doi:10.1016/j.envpol.2016.06.039
Pedà, C., Caccamo, L., Fossi, M. C., Gai, F., Andaloro, F., Genovese, L., et al. (2016). Intestinal alterations in European sea bass Dicentrarchus labrax (Linnaeus, 1758) exposed to microplastics: Preliminary results. Environ. Pollut. 212, 251–256. doi:10.1016/j.envpol.2016.01.083
Phuong, N. N., Zalouk-Vergnoux, A., Poirier, L., Kamari, A., Châtel, A., Mouneyrac, C., et al. (2016). Is there any consistency between the microplastics found in the field and those used in laboratory experiments? Environ. Pollut. 211, 111–123. doi:10.1016/j.envpol.2015.12.035
R Core Team (2022). A language and environment for statistical computing. Vienna, Austria: R Foundation for Statistical Computing.
Rall, B. C., Brose, U., Hartvig, M., Kalinkat, G., Schwarzmüller, F., Vucic-Pestic, O., et al. (2012). Universal temperature and body-mass scaling of feeding rates. Phil. Trans. R. Soc. B 367, 2923–2934. doi:10.1098/rstb.2012.0242
Ramírez, A., Ardón, M., Douglas, M., and Graça, M. A. S. (2015). Tropical freshwater sciences: An overview of ongoing tropical research. Freshw. Sci. 34, 606–608. doi:10.1086/681257
Ribeiro, F., Garcia, A. R., Pereira, B. P., Fonseca, M., Mestre, N. C., Fonseca, T. G., et al. (2017). Microplastics effects in Scrobicularia plana. Mar. Pollut. Bull. 122, 379–391. doi:10.1016/j.marpolbul.2017.06.078
Rist, S. E., Assidqi, K., Zamani, N. P., Appel, D., Perschke, M., Huhn, M., et al. (2016). Suspended micro-sized PVC particles impair the performance and decrease survival in the Asian green mussel Perna viridis. Mar. Pollut. Bull. 111, 213–220. doi:10.1016/j.marpolbul.2016.07.006
Rochman, C. M., Hoh, E., Kurobe, T., and Teh, S. J. (2013). Ingested plastic transfers hazardous chemicals to fish and induces hepatic stress. Sci. Rep. 3, 3263. doi:10.1038/srep03263
Rødland, E. S., Lind, O. C., Reid, M., Heier, L. S., Skogsberg, E., Snilsberg, B., et al. (2022). Characterization of tire and road wear microplastic particle contamination in a road tunnel: From surface to release. J. Hazard. Mat. 435, 129032. doi:10.1016/j.jhazmat.2022.129032
Roebroek, C. T. J., Harrigan, S., Emmerik, T. H. M. van, Baugh, C., Eilander, D., Prudhomme, C., et al. (2021). Plastic in global rivers: Are floods making it worse? Environ. Res. Lett. 16, 025003. doi:10.1088/1748-9326/abd5df
Rohatgi, A. (2021). Plotdigitizer: Version 3. Available at: https://automeris.io/WebPlotDigitizer.
Sadigov, R. (2022). Rapid growth of the world population and its socioeconomic results. Sci. World J. 2022, 1–8. doi:10.1155/2022/8110229
Saldaña-Vázquez, R. A., and Munguía-Rosas, M. A. (2013). Lunar phobia in bats and its ecological correlates: A meta-analysis. Mamm. Biol. 78, 216–219. doi:10.1016/j.mambio.2012.08.004
Salerno, M., Berlino, M., Mangano, M. C., and Sarà, G. (2021). Microplastics and the functional traits of fishes: A global meta‐analysis. Glob. Change Biol. 27, 2645–2655. doi:10.1111/gcb.15570
Sarijan, S., Azman, S., Said, M. I. M., and Jamal, M. H. (2021). Microplastics in freshwater ecosystems: A recent review of occurrence, analysis, potential impacts, and research needs. Environ. Sci. Pollut. Res. 28, 1341–1356. doi:10.1007/s11356-020-11171-7
Scherer, C., Weber, A., Lambert, S., and Wagner, M. (2018). “Interactions of microplastics with freshwater biota,” in Freshwater microplastics : Emerging environmental contaminants? The handbook of environmental chemistry. Editors M. Wagner, and S. Lambert (Cham: Springer International Publishing), 153–180. doi:10.1007/978-3-319-61615-5_8
Serra, T., Barcelona, A., Pous, N., Salvadó, V., and Colomer, J. (2020). Synergistic effects of water temperature, microplastics and ammonium as second and third order stressors on Daphnia magna. Environ. Pollut. 267, 115439. doi:10.1016/j.envpol.2020.115439
Sfriso, A. A., Tomio, Y., Rosso, B., Gambaro, A., Sfriso, A., Corami, F., et al. (2020). Microplastic accumulation in benthic invertebrates in terra nova bay (ross sea, Antarctica). Environ. Int. 137, 105587. doi:10.1016/j.envint.2020.105587
Simonsen, L., and Viboud, C. (2021). A comprehensive look at the COVID-19 pandemic death toll. eLife 10, e71974. doi:10.7554/eLife.71974
Smith, M., Love, D. C., Rochman, C. M., and Neff, R. A. (2018). Microplastics in seafood and the implications for human health. Curr. Environ. Health Rep. 5, 375–386. doi:10.1007/s40572-018-0206-z
Stanković, J., Milošević, D., Savić-Zdraković, D., Yalçın, G., Yildiz, D., Beklioğlu, M., et al. (2020). Exposure to a microplastic mixture is altering the life traits and is causing deformities in the non-biting midge Chironomus riparius Meigen (1804). Environ. Pollut. 262, 114248. doi:10.1016/j.envpol.2020.114248
Stelzer, C.-P. (2005). Evolution of rotifer life histories. Hydrobiologia 546, 335–346. doi:10.1007/s10750-005-4243-x
Straub, S., Hirsch, P. E., and Burkhardt-Holm, P. (2017). Biodegradable and petroleum-based microplastics do not differ in their ingestion and excretion but in their biological effects in a freshwater invertebrate Gammarus fossarum. Int. J. Environ. Res. Public Health 14, E774. doi:10.3390/ijerph14070774
Sun, Y., Xu, W., Gu, Q., Chen, Y., Zhou, Q., Zhang, L., et al. (2019). Small-sized microplastics negatively affect rotifers: Changes in the key life-history traits and rotifer–phaeocystis population Dynamics. Environ. Sci. Technol. 53, 9241–9251. doi:10.1021/acs.est.9b02893
Sussarellu, R., Suquet, M., Thomas, Y., Lambert, C., Fabioux, C., Pernet, M. E. J., et al. (2016). Oyster reproduction is affected by exposure to polystyrene microplastics. Proc. Natl. Acad. Sci. U. S. A. 113, 2430–2435. doi:10.1073/pnas.1519019113
Tamargo, A., Molinero, N., Reinosa, J. J., Alcolea-Rodriguez, V., Portela, R., Bañares, M. A., et al. (2022). PET microplastics affect human gut microbiota communities during simulated gastrointestinal digestion, first evidence of plausible polymer biodegradation during human digestion. Sci. Rep. 12, 528. doi:10.1038/s41598-021-04489-w
Tanaka, K., Takada, H., Yamashita, R., Mizukawa, K., Fukuwaka, M., and Watanuki, Y. (2013). Accumulation of plastic-derived chemicals in tissues of seabirds ingesting marine plastics. Mar. Pollut. Bull. 69, 219–222. doi:10.1016/j.marpolbul.2012.12.010
Tongo, I., and Erhunmwunse, N. O. (2022). Effects of ingestion of polyethylene microplastics on survival rate, opercular respiration rate and swimming performance of African catfish (Clarias gariepinus). J. Hazard. Mat. 423, 127237. doi:10.1016/j.jhazmat.2021.127237
Trotter, B., Wilde, M. V., Brehm, J., Dafni, E., Aliu, A., Arnold, G. J., et al. (2021). Long-term exposure of Daphnia magna to polystyrene microplastic (PS-MP) leads to alterations of the proteome, morphology and life-history. Sci. Total Environ. 795, 148822. doi:10.1016/j.scitotenv.2021.148822
Van Cauwenberghe, L., Devriese, L., Galgani, F., Robbens, J., and Janssen, C. R. (2015). Microplastics in sediments: A review of techniques, occurrence and effects. Mar. Environ. Res. 111, 5–17. doi:10.1016/j.marenvres.2015.06.007
van Kleunen, M., Brumer, A., Gutbrod, L., and Zhang, Z. (2020). A microplastic used as infill material in artificial sport turfs reduces plant growth. PLANTS PEOPLE PLANET 2, 157–166. doi:10.1002/ppp3.10071
Viechtbauer, W. (2007). Accounting for heterogeneity via random-effects models and moderator analyses in meta-analysis. Zeitschrift fur Psychol./J. Psychol. 215, 104–121. doi:10.1027/0044-3409.215.2.104
Viechtbauer, W. (2010). Conducting meta-analyses in R with the metafor package. J. Stat. Softw. 36, 1–48. doi:10.18637/jss.v036.i03
Vitule, J. R. S., Agostinho, A. A., Azevedo-Santos, V. M., Daga, V. S., Darwall, W. R. T., Fitzgerald, D. B., et al. (2017). We need better understanding about functional diversity and vulnerability of tropical freshwater fishes. Biodivers. Conserv. 26, 757–762. doi:10.1007/s10531-016-1258-8
Vogelsang, C., Lusher, A., Dadkhah, M., Sundvor, I., Umar, M., Ranneklev, S., et al. (2018). Microplastics in road dust - characteristics, pathways and measures. Norway: Norwegian Institute for Water Research.
von Moos, N., Burkhardt-Holm, P., and Köhler, A. (2012). Uptake and effects of microplastics on cells and tissue of the blue mussel Mytilus edulis L. After an experimental exposure. Environ. Sci. Technol. 46, 11327–11335. doi:10.1021/es302332w
Wagner, M., Scherer, C., Alvarez-Muñoz, D., Brennholt, N., Bourrain, X., Buchinger, S., et al. (2014). Microplastics in freshwater ecosystems: What we know and what we need to know. Environ. Sci. Eur. 26, 12. doi:10.1186/s12302-014-0012-7
Wang, J., Li, Y., Lu, L., Zheng, M., Zhang, X., Tian, H., et al. (2019). Polystyrene microplastics cause tissue damages, sex-specific reproductive disruption and transgenerational effects in marine medaka (Oryzias melastigma). Environ. Pollut. 254, 113024. doi:10.1016/j.envpol.2019.113024
Watts, A. J. R., Urbina, M. A., Goodhead, R., Moger, J., Lewis, C., and Galloway, T. S. (2016). Effect of microplastic on the gills of the Shore crab Carcinus maenas. Environ. Sci. Technol. 50, 5364–5369. doi:10.1021/acs.est.6b01187
Weber, A., Scherer, C., Brennholt, N., Reifferscheid, G., and Wagner, M. (2018). PET microplastics do not negatively affect the survival, development, metabolism and feeding activity of the freshwater invertebrate Gammarus pulex. Environ. Pollut. 234, 181–189. doi:10.1016/j.envpol.2017.11.014
Wright, S. L., Rowe, D., Thompson, R. C., and Galloway, T. S. (2013a). Microplastic ingestion decreases energy reserves in marine worms. Curr. Biol. 23, R1031–R1033. doi:10.1016/j.cub.2013.10.068
Wright, S. L., Thompson, R. C., and Galloway, T. S. (2013b). The physical impacts of microplastics on marine organisms: A review. Environ. Pollut. 178, 483–492. doi:10.1016/j.envpol.2013.02.031
Xu, J., Rodríguez-Torres, R., Rist, S., Nielsen, T. G., Hartmann, N. B., Brun, P., et al. (2022). Unpalatable plastic: Efficient taste discrimination of microplastics in planktonic copepods. Environ. Sci. Technol. 56, 6455–6465. doi:10.1021/acs.est.2c00322
Yang, C. Z., Yaniger, S. I., Jordan, V. C., Klein, D. J., and Bittner, G. D. (2011). Most plastic products release estrogenic chemicals: A potential health problem that can Be solved. Environ. Health Perspect. 119, 989–996. doi:10.1289/ehp.1003220
Yardy, L. D., Al-Jaibachi, R., and Callaghan, A. (2022). “Chapter 9 - microplastics in freshwater ecosystems with special reference to tropical systems: Detection, impact, and management,” in Emerging freshwater pollutants. Editors T. Dalu, and N. T. Tavengwa (Elsevier), 151–169. doi:10.1016/B978-0-12-822850-0.00017-X
Yıldız, D., Yalçın, G., Jovanović, B., Boukal, D. S., Vebrová, L., Riha, D., et al. (2022). Effects of a microplastic mixture differ across trophic levels and taxa in a freshwater food web: In situ mesocosm experiment. Sci. Total Environ. 836, 155407. doi:10.1016/j.scitotenv.2022.155407
Yin, L., Chen, B., Xia, B., Shi, X., and Qu, K. (2018). Polystyrene microplastics alter the behavior, energy reserve and nutritional composition of marine jacopever (Sebastes schlegelii). J. Hazard. Mat. 360, 97–105. doi:10.1016/j.jhazmat.2018.07.110
Zhang, X., Wen, K., Ding, D., Liu, J., Lei, Z., Chen, X., et al. (2021). Size-dependent adverse effects of microplastics on intestinal microbiota and metabolic homeostasis in the marine medaka (Oryzias melastigma). Environ. Int. 151, 106452. doi:10.1016/j.envint.2021.106452
Keywords: review, plastics, pollution, climate, tropics, food webs, freshwater, marine
Citation: Moyo S (2022) An enigma: A meta-analysis reveals the effect of ubiquitous microplastics on different taxa in aquatic systems. Front. Environ. Sci. 10:999349. doi: 10.3389/fenvs.2022.999349
Received: 20 July 2022; Accepted: 07 September 2022;
Published: 19 September 2022.
Edited by:
Unique Ndubuisi Keke, Federal University of Technology Minna, NigeriaReviewed by:
Kathryn Hassell, RMIT University, AustraliaMarta Baccaro, Wageningen University and Research, Netherlands
Copyright © 2022 Moyo. This is an open-access article distributed under the terms of the Creative Commons Attribution License (CC BY). The use, distribution or reproduction in other forums is permitted, provided the original author(s) and the copyright owner(s) are credited and that the original publication in this journal is cited, in accordance with accepted academic practice. No use, distribution or reproduction is permitted which does not comply with these terms.
*Correspondence: Sydney Moyo, bW95b3NAcmhvZGVzLmVkdQ==