- 1State Key Laboratory of Nuclear Resources and Environment, East China University of Technology, Nanchang, China
- 2School of Chemistry, Biological and Materials Science, East China University of Technology, Nanchang, China
In this study, nine soils at different depths in a decommissioned uranium tailing pond in Jiangxi Province are selected as the research objects to study their mineralogical properties and reveal the leaching law of radioactive element uranium under natural rainfall conditions. The research results are as follows: 1) The distribution characteristics of uranium are affected by the mineral composition, microscopic morphology and physical and chemical properties of uranium tailings. 2) In static leaching experiments, acidic solutions, small particles, and high solid-to-liquid ratios all promote the release of uranium. 3) In the dynamic leaching experiment, the lower the pH of simulated rainfall, the more uranium is released. According to Fick theory, the migration mechanism of uranium is mainly surface dissolution, and the release of uranium is related to the content of migratory uranium.
1 Introduction
Energy is a resource that human beings depend on for survival and an indispensable and powerful guarantee for social development. Traditional fossil energy cannot be regenerated, and it also causes huge pollution to the environment. Nuclear energy is an efficient, economical and clean type of energy, which plays an important role in improving the energy system and reducing environmental pollution (Lin and Zhu 2019; Azam et al., 2022). Uranium is an important fuel for nuclear power and a necessary strategic resource for national development (Li et al., 2021; Simionescu et al., 2022). The continuous development of the nuclear industry has greatly increased the demand for uranium, and the amount of uranium mining has also increased (Rweyemamu and Kim 2020). Uranium tailing are waste residues produced in the process of extracting and processing uranium from uranium ore in beneficiation plants. Due to its large scale, large quantity, and mostly open-air accumulation, it is one of the key radioactive pollution sources (Nixon et al., 2018). Uranium waste rock and tailing slag will gradually release radioactive metal elements such as uranium and thorium under atmospheric erosion and rain wash (Tawfic et al., 2019), and pollutants will continue to migrate into water and soil along with the leachate (Akortia et al., 2021), which is harmful to human health and ecosystems (Liu et al., 2020). The early uranium mines in my country generally completed decommissioning treatment, while the early uranium mining area treatment and restoration methods (Chopra et al., 2013; Jha et al., 2016) were relatively simple. For tailing ponds, waste rock plants, etc., only soil surface covering was often used. Intercept ditches and drainage ditches and other municipal waste removal and diversion, in order to reduce the release rate of harmful elements. These measures cannot fundamentally guarantee that decommissioned uranium mines will no longer have a negative impact on the environment (Holanda and Johnson 2020), and the concentration of heavy metal ions in mine wastewater cannot immediately return to acceptable standards. The quality of the seepage water generally takes decades, hundreds or even thousands of years to recover, causing long-term adverse effects on the ecological environment (Mkandawire 2013; De Carvalho Filho et al., 2017).
The release of radioactive elements is a complex process controlled by many factors. The migration modes and reaction mechanisms of uranium in different media and environmental systems are very different (Aba et al., 2021; Yin et al., 2021a). Hydrodynamic conditions, physicochemical properties of solid medium, chemical composition of liquid phase, pH, and particle size all have varying degrees of influence on the release process (Yan and Luo 2015; Yang et al., 2019). Uranium tailing are mainly composed of minerals, organic matter and water, which is a multiphase coexisting system, and the various components are interrelated (Bone et al., 2020). The environmental behavior and migration ability of radioactive elements depend on their occurrence form (He et al., 2021), and the physical and chemical properties of uranium tailing play a decisive role in the occurrence form of uranium (Ouyang et al., 2019). Particle size affects the specific surface area, porosity, and permeability of tailing, and indirectly affects the diffusion and migration behavior in tailing (Yin et al., 2020). Especially the extremely fine particles in tailing have large specific surface area and strong adsorption capacity for uranium, which can become the carrier of pollutants, and continuously migrate downward with the flow of water (Liu et al., 2017b). The solubility and occurrence form of uranium, thorium and other nuclides in the medium are affected by pH (Zuo et al., 2017). Under acidic conditions (Wang et al., 2021), uranium usually exists in the free form of UO22+, which has good solubility and mobility. In neutral and alkaline solutions, uranyl ions chelate with carbonate to form uranyl carbonate complexes, the main forms are UO2(CO3)22- and UO2(CO3)34- (Atta et al., 2010).
The researchers (Gorshenina et al., 2020) simulated the migration experiments of heavy metal elements in soil-plant systems, passing the solution into the soil column for regular irrigation, and found that most of the uranium would be bound to the components in the rhizosphere soil as non-exchangeable compounds. Some scholars (Patra et al., 2011) collected the tailing after smelting uranium and copper mines, and selected several leaching solvents to conduct long-term semi-dynamic leaching experiments. Through a series of analytical methods to explore the effect of weathering on the stability of granite waste rock piles and uranium migration, it was found that corrosion and weathering can affect the rock structure and permeability, and part of the uranium is released from the easily altered granite minerals. Other secondary minerals combine and transform into a new uranium-containing phase, and the stable uranyl phosphate will inhibit the mobility of uranium and limit the spatial migration of uranium (Boekhout et al., 2015). The GMS software (Wang et al., 2022) simulated the migration of U in the groundwater system, analyzed the effect of rainfall on the uranium migration rate, and predicted the treatment effect of the tailing pond groundwater system on U.
In order to accurately evaluate the impact of radioactive uranium on the surrounding environment, this paper focuses on the physical and chemical properties of uranium in soil samples from decommissioned uranium tailing. This aspect simulates the release and migration of uranium in soil during rainfall. This paper studies the pollution risks in decommissioned uranium tailing ponds, and the conclusions of the research have certain practical significance for pollution prevention and control in mining areas.
2 Experiments and method
2.1 Overview of the research area
The research area was selected from a decommissioned uranium tailing reservoir in Jiang xi Province, China, and the research object was taken from the soil of the tailing reservoir. This uranium ore is rich in mineral resources and is currently the largest volcanic rock-type uranium deposited in China. The annual rainfall is about 1,550–2000 mm, and the total evaporation is about 1,100–1,600 mm. The rainy season is mainly concentrated in April-June. During the rainy season, the rainfall is strictly higher than the national average monthly rainfall. A large amount of slag and radioactive waste are piled up in the tailing reservoir in the research area.
2.2 Sample collection and processing
According to the environmental characteristics of the research area, the tailing reservoir area above the tailing dam is selected as the sampling area. Using the principles of randomness and equivalence, 9 sampling points are selected, numbered 1–9 (Figure 1).
During the sampling process, the weeds on the surface of the sampling point were removed first, and the column tube (height: 50 cm, inner diameter: 43 mm, wall thickness: 1 mm) was placed vertically at the sampling position. The column tube is continuously pushed down with the help of a pressure plate. When the desired depth is reached, the column tube is slowly drawn out, so that the original tailings pillar is successfully collected (Figure 2). Divide the sample into four layers: 0–10 cm, 10–20 cm, 20–30 cm and 30–40 cm. Lay the sample on the enamel tray, remove debris, dry it naturally for 2 weeks, and grind the sample into a different particle size according to different experimental requirements.
2.3 Attribute Research
2.3.1 Characterization methods
In order to explore the physical properties of the samples, some experimental instruments are used as shown in the table (Table 1).
Sample grinding (below 74 μm), the treated powder is used in X-ray diffraction analysis (XRD) for mineral composition analysis and X-ray fluorescence spectroscopy analysis (XRF) to determine the main chemical components of uranium tailing. The microscopic appearance of the sample was observed by scanning electron microscope (SEM) of the dried tailing particles, and the microscopic composition of the substance was analyzed by X-ray energy spectrometer (EDS).
2.3.2 pH
The tailing sample of different depths was weighed at 10.0 g (accurate to 0.01 g), 25 ml deionized water (solid-liquid ratio 1:2.5) was added to the middle, stirred by the magnetic agitator for 1 min, and determined by a pH meter after static for 30 min.
2.3.3 Particle size analysis
Samples with different mass and depths are weighed, completely dried and screened (after screening, the particle sizes are 0.9, 0.3–0.9, 0.2–0.3, 0.15–0.2, 0.098–0.015, <0.098 mm, respectively), weigh the sample weight of each particle size range, and calculate the mass percentage of tailing in each particle size range.
2.3.4 Dissolution of uranium
In order to determine the total amount of uranium in uranium tailings samples, the samples were digested by the traditional wet digestion method. The specific steps are as follows (Chen et al., 2021): Put the 0.5000 g sample (<74 μm) after grinding at different depths into the polytetrafluoroethylene beaker, add the existing 10 ml of royal water, and heat it on the 200°C electric heating plate until viscous. After removing the beaker and cooling to room temperature, add 3 ml HNO3, 2 ml HF and 1 ml HClO4 and heat until the white smoke perchlorate dissipates. Remove the beaker and add 3 ml HNO3, heat and dissolve the residue until the solution is clarified and remove it. Wash the digestion cup with deionized water for three times, shake it to volume, and then filter it into the solution to be measured.
2.3.5 Uranium-existing morphology extraction
To explore the different occurrence states of uranium in uranium tailing, the improved BCR extraction method is used to determine (Chen et al., 2019). The BCR method is a more mature morphological extraction method proposed by the European Community Bureau of Reference (Frentiu et al., 2009; Lavelle et al., 2015). It divides heavy metals into four forms in the environment, namely, acid extractable state, reducing state, oxidizable and residue states. The specific BCR extraction steps are as follows (Sylvia et al., 2017):
(1) Acid extractable state: 1.000 g of sample after dry grinding (particle size of 200 mesh) is put into the centrifugal tube, 40 ml HOAc (0.1 mol/L) is added to it, the water bath shaker (22°C) oscillates continuously for 16 h, and centrifugal 10 min (8000 r/min). After the centrifugal liquid is removed to the capacity bottle, 30 ml deionized water is added to the centrifugal tube and then centrifugal. When the serum is poured into the capacity bottle, add 1 ml of concentrated nitric acid, dilute it with water to the scale, and shake well. Residue is used for the next experiment.
(2) Reducible state: add 40 ml NH4OH·HCl to the residue of the previous step, shake and centrifugate, and the step is consistent with (1). The third step of the residue state is carried out.
(3) Oxidable state: add 10 ml H2O2 to the residue, add 10 ml H2O2 after 1 h, heat it in the constant temperature bath (85°C) to liquid steam, add 50 ml NH4Ac(1 mol/L) and shake the water bath thermostatic shaker (22°C) for 16 h. The next steps are the same as (1). The residue is carried out in the fourth step experiment.
(4) Add 10 ml of royal water to the residue, and heat and digest it on the electric heating plate after 12 h. The digestion steps are the same as the wet digestion steps.
2.3.6 Uranium content determination
Uranium ion concentration is determined by inductively coupled plasma atomic emission spectrometer (ICP-OES) and inductively coupled plasma mass spectrometer (ICP-MS). ICP-OES mainly determines the digested solution, and ICP-MS mainly determines trace ion concentrations in leachate.
2.4 Static leaching experiment
The water environment plays a certain role in the leaching and migration of uranium ions, and the characteristics of the soil itself also affects the leaching effect of uranium. In order to analyze the leaching characteristics of uranium ions, this study takes pH, solid-liquid ratio and tailing particle size of rainwater as independent variables to simulate rainfall to conduct leaching experiments on uranium tailing.
2.4.1 Materials and reagents
According to the distribution of sampling points, two samples of uranium tailings at depths of 0–10 cm and 30–40 cm in the No. 6 sample column were selected and air-dried after pretreatment. The two tailings were screened into three tailings samples with different particle size ranges (>0.3 mm, 0.15–0.3 mm, < 0.15 mm).
According to the investigation, the study area is sulfuric acid rainfall. The main acid-causing substances in the rainwater are SO42- and NO3−. The soaking solution was prepared with H2SO4, HNO3 and deionized water. First, concentrated H2SO4 and concentrated HNO3 with a volume ratio of 4∶1 were mixed, and then mixed acid drops were added to deionized water to prepare simulated rainwater with pH 4.0, 5.0 and 6.0, respectively.
2.4.2 Experimental method
At room temperature, two groups of uranium tailings samples with different depths were subjected to leaching experiments with a period of 15 days. Tailings sample (5 g) was accurately weighed into a 250 ml beaker, and a certain volume of simulated rainwater was added as the soaking solution. Different pH, solid-liquid ratio and particle size were set in each experimental group to study the leaching characteristics of U in tailings. When soaking, seal the mouth of the beaker with plastic wrap to reduce evaporation.
(1) Under the condition of 1:20 solid-liquid ratio, the simulated rainwater was soaked with different pH (pH = 4.0, 5.0, 6.0).
(2) According to the solid-liquid ratio of 1:10, 1:20 and 1:40 respectively, the experimental groups were prepared with rainwater with pH = 5.
(3) Select 0–10 cm, 30–40 cm deep tailing samples, and sieve the tailing into three tailing samples with different particle size ranges (>0.3 mm, 0.15–0.3 mm, <0.15 mm), The immersion solution was prepared with rainwater with pH = 5 and solid-liquid ratio of 1:20.
After soaking for 1, 3, 6, 9, 12, and 15 days, the pH of the supernatant in the beaker was measured by a pH meter. The supernatant (5 ml) was taken from each beaker, filtered through a needle filter, and transferred to a 50-ml volumetric flask. A drop of concentrated nitric acid was added, and then deionized water was added to the flask. The content of uranium in supernatant was determined by ICP-OES and ICP-MS. At the end of the experiment, some tailings samples with pH = 5.0 were taken to simulate rainwater immersion, dried and ground for morphological extraction experiment.
2.5 Dynamic leaching experiment
Three leaching columns (column height 500 mm, inner diameter 430 mm) are selected, 2 cm of coarse quartz sand (filter layer) was loaded at the upper and lower ends of the leaching column, and the middle part is filled with tailing samples (0–10 cm, 10–20 cm, 20–30 cm, and 30–40 cm) are filled sequentially from deep to shallow (length is about 420 mm), and each leaching column is connected to a peristaltic pump (controlling the speed of leaching) (Figure 3). At room temperature, 100 ml of simulated rainwater with different pH (pH = 4, 5, 6) is leached every day. The experimental period is 30 days, with a total of 3,000 mm of rainfall. The permeate collected at the bottom of each tailing column was put into a centrifuge tube, and then the permeate was passed through a syringe filter with a pore size of 0.22 μm, and the concentration of uranium ions in the filtrate is determined by ICP-MS.
3 Results and Discussion
3.1 Sample property analysis
3.1.1 Characterization analysis
The study analyzes the mineral composition of uranium tailing at different depths in the profile by XRD (Figure 4). The mineral composition of tailing at different depths is roughly the same. The minerals corresponding to these diffraction peaks are mainly quartz, albite, and a small amount of clay minerals such as kaolin and illite. Among them, quartz and albite usually exist in the form of component components, mainly derived from primary or associated minerals of uranium ore.
The chemical composition of uranium tailing is related to the mineralization process. The chemical composition of the uranium tailing was tested by XRF (Table 2). It can be seen from the table that the main chemical components of uranium tailing are SiO2, Al2O3, followed by K2O, CaO, Fe2O3, and all contain a small amount of uranium and thorium. According to the XRF test results of tailing at different depths, it is found that the uranium content in the surface layer is significantly higher than that in the lower layer tailing, indicating that uranium has a surface enrichment trend in the tailing accumulation area. The content of uranium in tailing samples ranges from 265 mg/kg to 412 mg/kg, which is much higher than the average content of uranium in general surface rocks and soils. Once this part of uranium is released, it will cause great harm to the surrounding environment.
The surface morphology of tailing samples is observed by scanning electron microscope, and EDS data is obtained by combining with energy dispersive spectrometer (Figure 5; Table 3). In the SEM images of the two tailing samples, it is found that the surface of the tailing samples is relatively rough, dominate by lumps and particles, and show more holes at high resolution. The uranium tailing samples are mainly composed of O, Al, Si and K elements, which are consistent with the analysis results of XRF. The U element is detected in the EDS scan at 1) but not at 2), indicating that the distribution of U in the tailing is very uneven. The uranium content in tailing is very low, and most of them are wrapped in minerals or attached to the surface of other minerals in the form of dispersed adsorption.
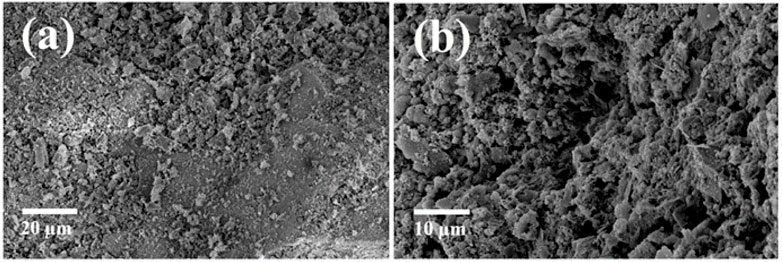
FIGURE 5. SEM image of some uranium tailings samples: (A) Uranium is present there; (B) Uranium is not present there.
3.1.2 pH analysis
According to the data in the table (Table 4), it can be found that the pH of tailing is about 8.32 on average, and the pH difference of tailing at different depths is not large. In the previous uranium beneficiation and hydrometallurgy process, the mine wastewater and tailing slag after acid leaching are neutralized by quicklime and then discharged into the tailing pond, so the tailing in the accumulation area is alkaline. Other heavy metal compounds such as uranium will co-precipitate with aluminum hydroxide, calcium hydroxide, and iron hydroxide in an alkaline environment, which will reduce the dissolution rate of uranium. However, long-term acid rain and atmospheric erosion may change the pH of tailing, which is also a key point that needs to be continuously monitored during the treatment of decommissioned uranium mining areas.
3.1.3 Particle size distribution
After being screened by different screens, the proportions of uranium tailings in different particle size ranges (Figure 6). Combining with the chart, it can be seen that the particle size distribution of uranium tailing is wide, mostly concentrated in the range of 0.3–0.9 mm, and the proportion of tailing with particle size larger than 0.2 mm is about 80%. Uranium tailing are mainly composed of coarse sand grains with large porosity and good gas permeability and permeability. These pores provide convenient channels for the diffusion and migration of harmful metals such as uranium and thorium.
3.1.4 Uranium characteristic analysis
In order to study the longitudinal distribution characteristics of uranium in tailing, tailing column samples from three sampling points are selected, and samples at four depths of 5 cm, 15 cm, 25 cm, and 35 cm are collected from top to bottom for digestion. The total amount of uranium is determined experimentally. It can be seen from the distribution figure that the content of uranium in the surface tailing is relatively high, mainly in the range of 400 mg/kg-600 mg/kg (Figure 7). In general, the uranium content gradually decreases with the increase of the depth of the tailing, and the largest decrease is in the range of 10–20 cm. The difference in the uranium content in the deep tailing is small, indicating that the tailing has a certain adsorption and absorption capacity for uranium. The filtering ability can effectively slow down the migration of uranium. However, compared with the U in the crust (3 mg/kg), the total amount of U in the uranium tailing samples far exceeds the background value in the crust.
It can be seen from the uranium speciation distribution map of uranium tailing in the study area that the relative mass proportion of U in each occurrence form is (Figure 8): residual uranium (40.11%) > acid-extractable uranium (36.61%) >available oxidized uranium (21.35%) >reducible uranium (1.93%). Elements in the residual state are relatively stable, they are generally fixed or encapsulated in the lattice of other minerals, migrate or deposit together with carrier minerals, and are less harmful to the environment. The elements in the acid-extractable state have more active physical and chemical properties and are easily released by the influence of the external environment. Among them, the proportion of residual uranium is the highest, indicating that most of the uranium in the tailing is stable in the lattice of silicate minerals and is not easy to be released. Acid-extractable uranium includes uranium adsorbed on the surface of tailing such as clay and humus, and uranium combined with carbonate and then co-precipitated. This part of uranium has strong dissolving ability and migration ability and is the focus of pollution prevention and control.
3.2 Leaching Characteristics of U from Tailings by Simulated Rainfall
This chapter uses simulated rainfall to conduct experimental research on leaching of uranium tailing, explores the effects of simulated rainwater pH, solid-liquid ratio and tailing particle size on the leaching effect of tailing, and deeply analyzes the leaching characteristics of uranium, and try to put forward an effective scheme for tailing pollution control (Shi et al., 2021).
Simulated rainwater with different pH affects the leaching content of uranium in uranium tailing. It can be seen from the figure that the variation trends of uranium release under different pH conditions in the tailing samples at 0–10 cm (Figure 9A) and 30–40 cm (Figure 9B) are generally consistent. In the initial stage of immersion, the leaching concentration of uranium increases with the decrease of pH of the soaking solution. In the leaching experiment with pH = 4.0, the leaching concentration of uranium is slightly higher than that of the other two groups. The higher the pH, the less uranium is released. After leaching for 3 days, the concentrations of uranium ions in the leaching solutions of the three groups gradually approach, and the leaching rate of uranium becomes slower than before. In the later stage of leaching, the leaching amount of uranium in the experimental group with pH = 4.0 increases to a greater extent compared with the other two groups, indicating that the more acidic solution promotes the release of U from the uranium tailing.
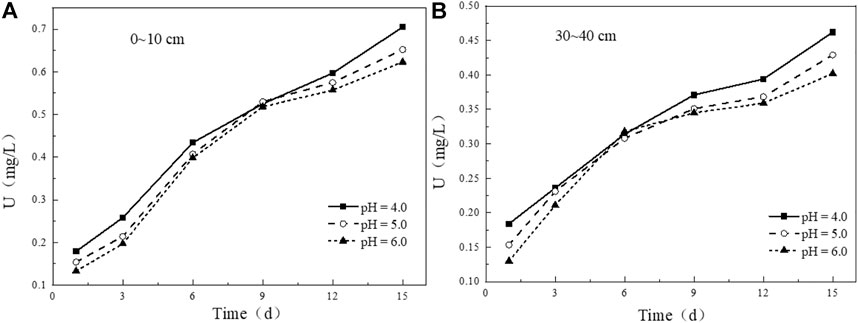
FIGURE 9. Variation of uranium leaching concentration under different initial pH conditions: (A) 0–10 cm; (B) 30–40 cm.
By comparing the leaching curves of uranium in the two tailing samples at 0–10 cm and 30–40 cm with time (Figure 10), it is found that under the same pH conditions, in the initial stage of leaching, the leaching amount of uranium in the shallow tailing is very close to that in the deep tailing, and the uranium released at this stage may be easily dissolved uranium on the surface of the tailing. However, as the leaching time increases, the uranium release in the two tailing samples begins to differ significantly. After 6–days of leaching, the leaching amount of uranium in the shallow tailing is 25%–42% higher than that in the deep tailing, indicating that the uranium in the deep tailing requires stronger substances to be extracted. The uranium of the migration component is more than that in the deep tailing, which is more harmful to the environment. The focus of pollution control is in the shallow tailing.
The solid-liquid ratio also plays a key role in the leaching process of uranium in tailing. Under the condition of solid-liquid ratio of 1:10, the leaching concentration of uranium in the tailing in the two tailing has a substantial increase in the initial stage of leaching, while the growth rate of uranium concentration in the leaching solution of the other two groups of solid-liquid ratios is relatively slow. With the prolongation of soaking time, the concentration of uranium in soaking solution decreased with the increase of solid-liquid ratio (Figure 11). After 15 days of leaching, the cumulative leaching amount of uranium in uranium tailing increases linearly with the increase of solid-liquid ratio (Figure 12), which is completely opposite to the change of leaching concentration. The reason for this result may be due to the smaller contact area of tailing and water in less solution, the mineral particles in tailing cannot be fully dissolved, the viscosity of tailing/water system will also increase slowly, and the amount of uranium leaching is also reduced.
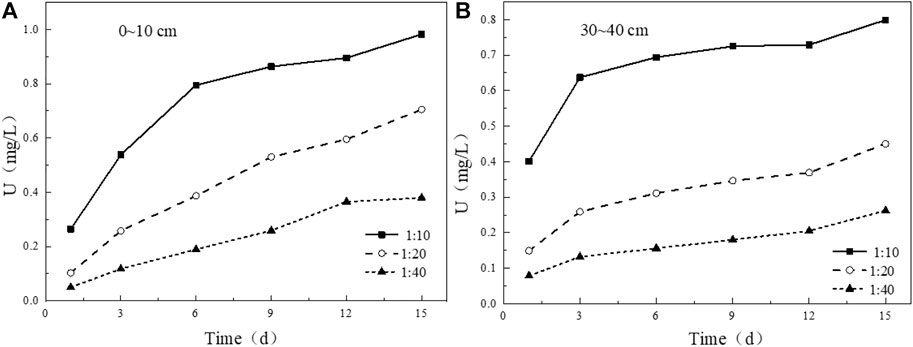
FIGURE 11. Variation of uranium leaching concentration under different solid-liquid ratio conditions: (A) 0–10 cm; (B) 30–40 cm.
Tailing particle size is an important factor in determining the porosity and permeability of tailing. The proportion of clay minerals in tailing particles with small particle size will increase, which is also one of the important factors affecting the release and migration process of uranium. For the tailing samples with a depth of 0–10 cm (Figure 13A), the leaching characteristics of uranium are that the smaller the particle size is, the greater the leaching amount will be. If the particle size of the tailing is reduced, the specific surface area will be increased, and the contact area between the tailing and the solution will increase accordingly, thereby increasing the leaching rate (Liu et al., 2017a). For the 30–40 cm tailing samples (Figure 13B), the leaching amount of uranium in the tailing with particle size <0.15 mm is significantly higher than that of the other two particle size groups, and the uranium-bearing minerals attached to the surface of the fine particles dissolves faster. However, the leaching rate of uranium in the tailing with a particle size >0.3 mm begins to be higher than that of the experimental group with a particle size of 0.15–0.3 mm, and the leaching rate in the later period begins to slow down again, indicating that in addition to the tailing particle size, there are also mineral substances. Other factors such as composition or internal structure affect the leaching of uranium from uranium tailing.
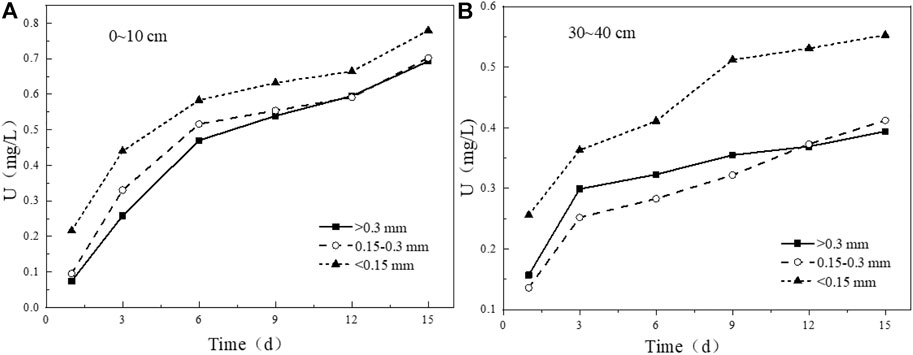
FIGURE 13. Variation of uranium leaching concentration under different particle size conditions. (A) 0–10 cm; (B) 30–40 cm.
Four forms of uranium in the uranium tailing before and after the leaching experiment are measured by the improved BCR step-by-step extraction method. Comparing the data of the two groups of tailing samples before and after leaching in the shallow and deep layers (Table 5; Figure 14), it is found that the extractable uranium has decreased to a certain extent after immersion, and the reducible uranium has no obvious change, indicating that the uranium in the immersion solution is mainly in the immersion process. It comes from the acid-soluble part of the tailing surface. Both oxidizable uranium and residual uranium have a small increase, which may be because the pH of the soaking solution continues to rise, some heavy metal ions will be hydrolyzed to form hydroxides, and uranium and Fe-OH, Al-OH and other inorganic colloids will occur. The complexation reaction produces a precipitate, which is converted into a new bound state.
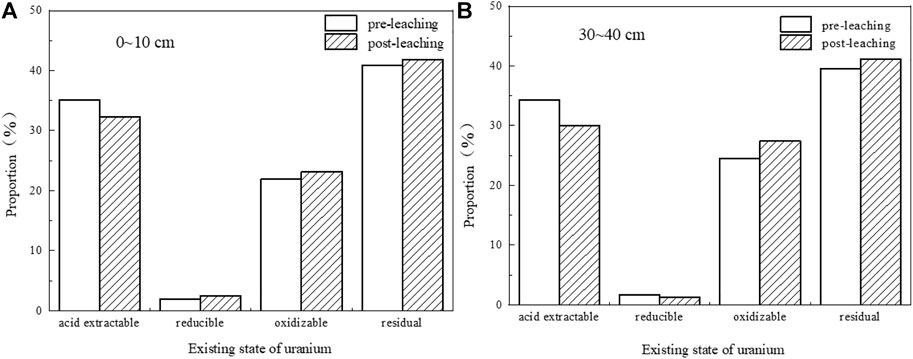
FIGURE 14. Speciation distribution of uranium in tailings before and after leaching. (A) 0–10 cm; (B) 30–40 cm.
3.3 Longitudinal Migration of U in Tailings under Simulated Rainfall
Previous studies have shown that acid rain can promote the activation of metals in tailing systems (Saber et al., 2019), and the complexation and adsorption reactions of some metals are affected by pH. This chapter focuses on exploring the change of uranium concentration under different rainfall conditions, and combines relevant mathematical models to find the release mechanism of uranium, in order to provide theoretical reference for the subsequent radioactive pollution control and restoration of decommissioned uranium tailing ponds.
This leaching experiment is to simulate three kinds of natural rainwater with different pH. The pH of the simulated rainwater is 4.0, 5.0, and 6.0, respectively, to analyze the release law in the uranium tailing column under rainfall conditions (Figure 15). A large amount of U is released during the interaction between uranium tailing and water under simulated rainfall conditions of different pH. The variation law of uranium concentration in the leachate of the three experimental groups is roughly the same, and the basic performance is from the quickly releasing phase to the slowly release phase, and finally reaches a stable state. The pH of the leachate has a certain influence on the release process of uranium. In the initial stage of the experiment, At the initial stage of the experiment, the simulated rainwater with pH of 4.0 leaches the most uranium, and the uranium content in the other two groups of leachate would be less, indicating that the increase of pH would slow down the release of uranium, and the uranium in tailing is easier to leach from the rainwater with lower pH. With the increase of the cumulative leaching amount, the effect of pH gradually decreases, probably because the silicate minerals and CaCO3 in the uranium tailing play a buffering role, and the acidification effect of rainwater on the tailing is short-term. According to the data in the figure, the amount of rainwater infiltrated into the tailing column changes sharply in the range of 0–600 ml, which belongs to the rapid release period of uranium. During the 30-day leaching process, the highest concentration of uranyl ions in the leachate is 1.349 mg·L−1, which is much higher than the discharge standard of mine wastewater (50 μg·L−1). The uranium eluted in the early stage mainly comes from the water-soluble and acid-soluble uranium on the surface of the tailing. This part of the uranium is easy to migrate in the environment and enter the solution. After leaching 400 ml of rainwater, the uranium concentration in the leachate turns to decrease, and the decreasing range also increases sharply. After 800 ml of continuous leaching, the uranium concentration in the leachate is maintained at a very low concentration and begins to stabilize. At this time, the existing form of uranium in the tailing is mostly insoluble substances, and the surface of the tailing is covered by an aged oxide film, resulting in a new surface, which improves the adsorption capacity of the tailing (Chang and Zhou 2017) and inhibits the mobility of uranium.
Under the dynamic leaching conditions of simulated rainfall, the release and migration behavior of U in uranium tailing will be affected by multiple reaction mechanisms simultaneously (Yin et al., 2019). In order to find out the dissolution and release mechanism of uranium in tailing during leaching, Fick diffusion theory (Yin et al., 2021b) can be combined to analyze the main control means of uranium migration through the logarithm of cumulative outflow flux and the kinetic model of time logarithm. The formula for calculating the cumulative release flux (B) is as follows:
In the above formulas: Bt is the release flux of the element at a certain time point t, mg/m2; t is the dissolution time, h; B is the sum of the released fluxes before the time point t; Dt is the mass concentration of the eluting element at a certain time point t, mg/L; V is the volume of the effluent, L; S is the geometric surface area of the sample in contact with the effluent, m2.
The release process of elements is mainly controlled by three mechanisms: 1) When the slope of the straight line is less than 0.35, it is surface scouring, that is, the soluble substances on the mineral surface are quickly washed down; 2) when the slope of the straight line is in the range of 0.35–0.65, the element is diffusion transport through the mineral pore space; 3) When the slope of the straight line is greater than 0.65, the leaching process is controlled by the dissolution of minerals and the contacting water phase, and both the fast and slow stages of dissolution actually lead to the release of dissolved substances, but does not lead to material depletion.
According to the linear fitting curve data (Figure 16; Table 6), when the pH of the simulated rainwater is 4.0, 5.0, and 6.0, the slopes of the linear fitting curves of uranium are all greater than 0.65, indicating that the release and migration of uranium in the long-term leaching process are mainly affected by dissolution factors. The uranium-bearing minerals adsorbed on the surface of the tailing particles in the acidic solution will dissolve, and then enter the leachate for longitudinal migration. In the tailing environment in the actual study area, with the extension of the open-air stacking time and the accumulation of rainfall, the total amount of uranium dissolved and released in the tailing will also increase.
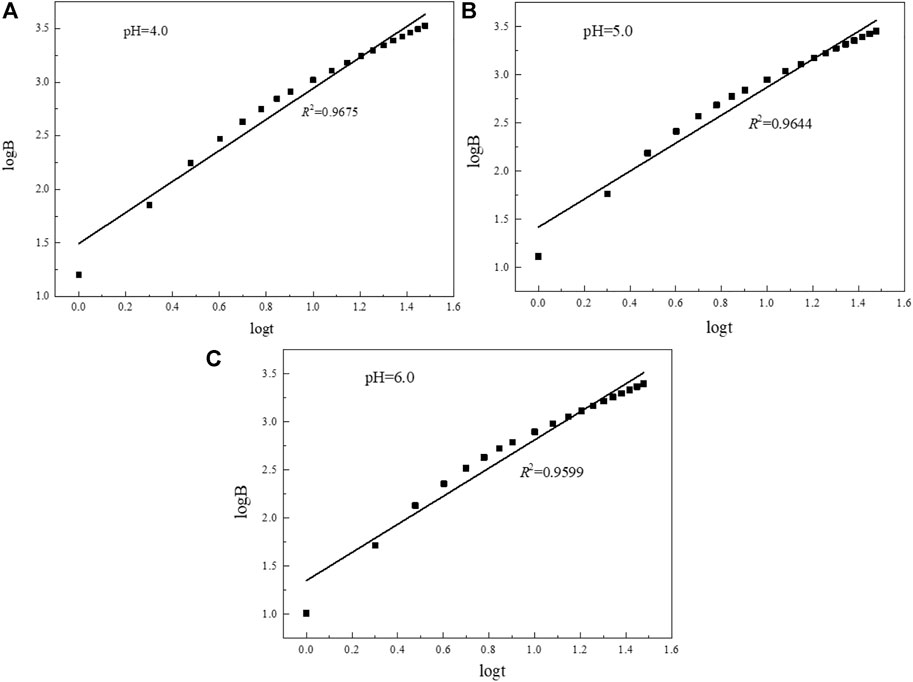
FIGURE 16. Linear fitting curves of uranium in tailings under different pH rainfall conditions. (A) pH=4.0; (B) pH=5.0; (C) pH=6.0.
4 Conclusion
This paper preliminarily studies the physical and chemical properties of a decommissioned uranium tailing pond and the distribution and occurrence of uranium. On this basis, indoor simulation experiments are carried out to further explore the release and migration rules of uranium in tailing and the effect of tailing on uranium. Adsorption-desorption. A series of conclusions are drawn as follows:
(1) The uranium tailing are mainly silicate minerals. The overall tailing is alkaline, with an average pH of 8.32, which may be the effect of mixing with quicklime to neutralize before discharge. The alkaline environment can greatly reduce the dissolution rate of uranium. The particle size distribution of tailing is wide, mainly coarse sand particles, mostly concentrated in the range of 0.3–0.9 mm, with large porosity and permeability. The tailing digestion experiment found that the total amount of U in the uranium tailing samples was in the range of 200–600 mg/kg, which was much higher than the uranium content in the crust. The enrichment effect of uranium in the surface tailings is obvious, mostly in the range of 400 mg/kg-600 mg/kg, and the uranium content in the deep tailings is quite different. The relative mass proportion of U in each occurrence form is: residual uranium (40.11%) > acid-extractable uranium (36.61%) > oxidizable uranium (21.35%) > reducible uranium (1.93%). About 40% of the uranium in the tailings exists in the residual state, but the content of the transportable state is also relatively high.
(2) In the static leaching process of simulated rainfall, the leaching amount of uranium with pH 4.0 of simulated rainwater is higher than that of the other two groups, indicating that acidic solution is conducive to the release of uranium from tailings. The leaching rate of uranium in tailings with particle size (<0.15 mm) is the fastest, indicating that small silicate minerals are more soluble. The cumulative leaching amount of U increases with the increase of the solid-liquid ratio, and the environmental water content of tailings increases or the soaking time increases, which will promote the release of uranium. At the end of the experiment, the pH of the immersion solution was maintained at about 8.0, and the oxidizable uranium at different depths increased from 21.93% to 24.56% and 23.21%–27.49%, respectively. The leaching amount of uranium in the shallow tailings is 25%–42% higher than that in the deep tailings, indicating that there is more active uranium in the shallow tailings than that in the deep tailings, which is more harmful to the environment.
(3) The effects of different acid rainfall on the release of uranium from tailings were discussed in the simulated acid rain dynamic leaching experiment. At the beginning of the experiment, the uranium concentration peaked at a percolation of 400 ml, and the release rate and amount of uranium gradually increased as the pH of the simulated rainwater decreased. With the increase of leaching volume, the release process of uranium gradually flattens out, and the uranium content stays at a low value in the middle and late stage. In the input process of external rainfall, iron, aluminum and sodium plasma will be released from the tailings to jointly regulate the pH of the system and reduce the mobility and migration of uranium. According to Fick diffusion theory, the release of uranium from tailings in the process of rainfall leaching is mainly controlled by surface dissolution, and the uranium in leachate mainly comes from the water-soluble part attached to the surface of tailings particles.
Therefore, the purpose of this study is to systematically explore the physical and chemical properties of uranium tailing ponds, the distribution characteristics of uranium in tailing ponds, the state of occurrence, and the law of migration and transformation, which will help in-depth analysis of uranium in tailing. It can predict the dynamic migration trend of radioactive elements in the uranium tailing reservoir in the natural environment, provide a theoretical basis for establishing an effective uranium tailing pollution prevention and control system, and promote the sustainable development of uranium resources.
Data availability statement
The original contributions presented in the study are included in the article/Supplementary Material, further inquiries can be directed to the corresponding author.
Author contributions
SX: Ideas, investigation, methodology, data curation and writing original draft. ZW: Performing the experiments and data/evidence collection. JO: Provision of study materials and reagents. LZ: Provision of study materials and reagents. ZL*: Supervision, writing-review and editing, funding acquisition and project administration.
Funding
This work was supported by the National Natural Science Fund Program (11875105, 21866006); General Project of Jiangxi Province Key Research and Development Program (20203BBFL63070, 20192BBG70062).
Conflict of interest
The authors declare that the research was conducted in the absence of any commercial or financial relationships that could be construed as a potential conflict of interest.
Publisher’s note
All claims expressed in this article are solely those of the authors and do not necessarily represent those of their affiliated organizations, or those of the publisher, the editors and the reviewers. Any product that may be evaluated in this article, or claim that may be made by its manufacturer, is not guaranteed or endorsed by the publisher.
References
Aba, A., Al-Boloushi, O., Ismaeel, A., and Al-Tamimi, S. (2021). Migration behavior of radiostrontium and radiocesium in arid-region soil. Chemosphere 281, 130953. doi:10.1016/j.chemosphere.2021.130953
Akortia, E., Glover, E. T., Nyarku, M., Dawood, A. M. A., Essel, P., Sarfo, E. O., et al. (2021). Geological interactions and radio-chemical risks of primordial radionuclides 40K, 226Ra, and 232Th in soil and groundwater from potential radioactive waste disposal site in Ghana. J. Radioanal. Nucl. Chem. 328, 577–589. doi:10.1007/s10967-021-07675-2
Atta, A. M., Abdel-Rahman, A. A. H., El Aassy, I. E., Ahmed, F. Y., and Hamza, M. F. (2010). Adsorption properties of uranium (VI) ions on reactive crosslinked acrylamidoxime and acrylic acid copolymer resins. J. Dispersion Sci. Technol. 32, 84–94. doi:10.1080/00377990903543053
Azam, A., Rafiq, M., Shafique, M., and Yuan, J. (2022). Mitigating carbon emissions in China: The role of clean energy, technological innovation, and political-institutional quality. Front. Environ. Sci. 10, 814439. doi:10.3389/fenvs.2022.814439
Boekhout, F., Gérard, M., Kanzari, A., Michel, A., Déjeant, A., Galoisy, L., et al. (2015). Uranium migration and retention during weathering of a granitic waste rock pile. Appl. Geochem. 58, 123–135. doi:10.1016/j.apgeochem.2015.02.012
Bone, S. E., Cliff, J., Weaver, K., Takacs, C. J., Roycroft, S., Fendorf, S., et al. (2020). Complexation by organic matter controls uranium mobility in anoxic sediments. Environ. Sci. Technol. 54, 1493–1502. doi:10.1021/acs.est.9b04741
Chang, Z., and Zhou, S. (2017). Study on immobilization and migration of nuclide u in superficial soil of uranium tailings pond. IOP Conf. Ser. Earth Environ. Sci. 64, 012021. doi:10.1088/1755-1315/64/1/012021
Chen, H., Chen, Z., Chen, Z., Chen, H., and Ou, X. (2021). Migration of rare earth elements in the topsoil of abandoned mines under rainfall leaching. J. Radioanal. Nucl. Chem. 328, 1189–1198. doi:10.1007/s10967-021-07741-9
Chen, L., Wang, K-P., and Yang, J-Y. (2019). Evaluate the potential bioavailability of vanadium in soil and vanadium titano-magnetite tailing in A mining area using BCR sequential and single extraction: A case study in panzhihua, China. Soil Sediment Contam. Int. J. 29, 232–245. doi:10.1080/15320383.2019.1702623
Chopra, M., Rastogi, R., Kumar, A. V., Sunny, F., and Nair, R. N. (2013). Response surface method coupled with first-order reliability method based methodology for groundwater flow and contaminant transport model for the uranium tailings pond site. Environ. Model. Assess. (Dordr). 18, 439–450. doi:10.1007/s10666-012-9352-0
De Carvalho Filho, C. A., Moreira, R. M., Branco, O. E. A., Dutra, P. H., Dos Santos, E. A., Moura, I. F. S., et al. (2017). Combined hydrochemical, isotopic, and multivariate statistics techniques to assess the effects of discharges from A uranium mine on water quality in neighboring streams. Environ. Earth Sci. 76, 830. doi:10.1007/s12665-017-7165-9
Frentiu, T., Ponta, M., Levei, E., and Cordos, E. (2009). Study of partitioning and dynamics of metals in contaminated soil using modified four-step BCR sequential extraction procedure. Chem. Pap. 63, 239–248. doi:10.2478/s11696-008-0102-3
Gorshenina, E., Baitelova, A., Rakhimova, N., Bykova, L., and Savchenkova, E. (2020). Monitoring of the migratory ability of heavy metals in the soil-plant system. Biointerface Res. Appl. Chem. 11, 10351–10357.
He, W., Zhang, X., Wu, X., Li, M., Zhang, J., Peng, Y., et al. (2021). Effects of ageing on the occurrence form of uranium in vertical soil layers near an uranium tailing reservoir. J. Radioanal. Nucl. Chem. 327, 847–856. doi:10.1007/s10967-020-07552-4
Holanda, R., and Johnson, D. B. (2020). Removal of zinc from circum-neutral pH mine-impacted waters using a novel “hybrid” low pH sulfidogenic bioreactor. Front. Environ. Sci. 8, 22. doi:10.3389/fenvs.2020.00022
Jha, V. N., Tripathi, R. M., Sethy, N. K., and Sahoo, S. K. (2016). Uptake of uranium by aquatic plants growing in fresh water ecosystem around uranium mill tailings pond at Jaduguda, India. Sci. Total Environ. 539, 175–184. doi:10.1016/j.scitotenv.2015.08.120
Lavelle, K. B., Miller, J. L., Hanson, S. K., Connick, W. B., Spitz, H. B., Glover, S. E., et al. (2015). Measurements of plutonium, 237Np, and 137Cs in the BCR 482 lichen reference material. J. Radioanal. Nucl. Chem. 307, 2085–2090. doi:10.1007/s10967-015-4497-3
Li, C., Yan, X., Liu, B., Yang, Z., and Zhou, L. (2021). Potential impacts of fukushima nuclear leakage on China’s carbon neutrality—An investigation on nuclear power avoidance and regional heterogeneity. Front. Environ. Sci. 9, 764941. doi:10.3389/fenvs.2021.764941
Lin, B., and Zhu, J. (2019). The role of renewable energy technological innovation on climate change: Empirical evidence from China. Sci. Total Environ. 659, 1505–1512. doi:10.1016/j.scitotenv.2018.12.449
Liu, B., Peng, T., and Sun, H. (2017a). Leaching behavior of U, Mn, Sr, and Pb from different particle-size fractions of uranium mill tailings. Environ. Sci. Pollut. Res. 24, 15804–15815. doi:10.1007/s11356-017-8921-9
Liu, B., Peng, T., Sun, H., and Yue, H. (2017b). Release behavior of uranium in uranium mill tailings under environmental conditions. J. Environ. Radioact. 171, 160–168. doi:10.1016/j.jenvrad.2017.02.016
Liu, Y., Zhou, W., Liu, H., Wei, Q., Gao, B., and Chen, G. (2020). Spatial variability and radiation assessment of the radionuclides in soils and sediments around a uranium tailings reservoir, south of China. J. Radioanal. Nucl. Chem. 324, 33–42. doi:10.1007/s10967-020-07077-w
Mkandawire, M. (2013). Biogeochemical behaviour and bioremediation of uranium in waters of abandoned mines. Environ. Sci. Pollut. Res. 20, 7740–7767. doi:10.1007/s11356-013-1486-3
Nixon, S. L., van Dongen, B. E., Boothman, C., Small, J. S., and Lloyd, J. R. (2018). Additives in plasticised polyvinyl chloride fuel microbial nitrate reduction at high pH: Implications for nuclear waste disposal. Front. Environ. Sci. 6, 97. doi:10.3389/fenvs.2018.00097
Ouyang, J., Liu, Z., Ye, T., and Zhang, L. (2019). Uranium pollution status and speciation analysis in the farmland-rice system around a uranium tailings mine in southeastern China. J. Radioanal. Nucl. Chem. 322, 1011–1022. doi:10.1007/s10967-019-06783-4
Patra, A. C., Sumesh, C. G., Mohapatra, S., Sahoo, S. K., Tripathi, R. M., and Puranik, V. D. (2011). Long-term leaching of uranium from different waste matrices. J. Environ. Manag. 92, 919–925. doi:10.1016/j.jenvman.2010.10.046
Rweyemamu, M., and Kim, J. (2020). Potential environmental hazard to the public from the operation of uranium mining and milling facility. Radiat. Prot. Dosim. 192, 75–88. doi:10.1093/rpd/ncaa195
Saber, A. N., Somjunyakul, P., Ok, J., and Watanabe, H. (2019). Rainfall-runoff simulation of radioactive cesium transport by using a small-scale portable rainfall simulator. Water Air Soil Pollut. 230, 226–315. doi:10.1007/s11270-019-4268-9
Shi, P., Zhang, Y., Sun, Q., and Ta, X. (2021). Eluviation and leaching of elements from broken fly-ash-based porous geopolymer. Mater. (Basel) 14, 6884. doi:10.3390/ma14226884
Simionescu, M., Schneider, N., and Gavurova, B. (2022). Decarbonized energies and the wealth of three European nations: A comparative nexus study using granger and toda-yamamoto approaches. Front. Environ. Sci. 9, 817982. doi:10.3389/fenvs.2021.817982
Sylvia, G., Nikita, T. T., Michael, J. K., Fanyana, M. M., Sekomeng, J. M., and Vusumzi, E. P. (2017). Quantification of Cd, Cu, Pb and Zn from sewage sludge by modified-BCR and ultrasound assisted-modified BCR sequential extraction methods. Afr. J. Pure Appl. Chem. 11, 9–18. doi:10.5897/ajpac2016.0712
Tawfic, A. F., Mira, H. I., and Omar, A. M. (2019). Chemical alteration processes for uranium migration and their significance on uranium isotopic ratio changes. Int. J. Environ. Anal. Chem. 101, 1–13. doi:10.1080/03067319.2019.1702172
Wang, J., Yin, M., Liu, J., Shen, C. C., Yu, T. L., Li, H. C., et al. (2021). Geochemical and U-Th isotopic insights on uranium enrichment in reservoir sediments. J. Hazard. Mater. 414, 125466. doi:10.1016/j.jhazmat.2021.125466
Wang, L., Cheng, J., Bao, C., Wang, Y., Jiang, Q., Pan, Y., et al. (2022). Simulation of nuclide migration in a middle- and low-level radioactive waste repository based on GMS. J. Radioanal. Nucl. Chem. 331, 2159–2167. doi:10.1007/s10967-022-08260-x
Yan, X., and Luo, X. (2015). Radionuclides distribution, properties, and microbial diversity of soils in uranium mill tailings from southeastern China. J. Environ. Radioact. 139, 85–90. doi:10.1016/j.jenvrad.2014.09.019
Yang, S., Zhang, X., Wu, X., Li, M., Zhang, L., Peng, Y., et al. (2019). Understanding the solid phase chemical fractionation of uranium in soil profile near a hydrometallurgical factory. Chemosphere 236, 124392. doi:10.1016/j.chemosphere.2019.124392
Yin, M., Sun, J., Chen, Y., Wang, J., Shang, J., Belshaw, N., et al. (2019). Mechanism of uranium release from uranium mill tailings under long-term exposure to simulated acid rain: Geochemical evidence and environmental implication. Environ. Pollut. 244, 174–181. doi:10.1016/j.envpol.2018.10.018
Yin, M., Sun, J., He, H., Liu, J., Zhong, Q., Zeng, Q., et al. (2021a). Uranium re-adsorption on uranium mill tailings and environmental implications. J. Hazard. Mater. 416, 126153. doi:10.1016/j.jhazmat.2021.126153
Yin, M., Tsang, D. C. W., Sun, J., Wang, J., Shang, J., Fang, F., et al. (2020). Critical insight and indication on particle size effects towards uranium release from uranium mill tailings: Geochemical and mineralogical aspects. Chemosphere 250, 126315. doi:10.1016/j.chemosphere.2020.126315
Yin, M., Zhou, Y., Tsang, D. C. W., Beiyuan, J., Song, L., She, J., et al. (2021b). Emergent thallium exposure from uranium mill tailings. J. Hazard. Mater. 407, 124402. doi:10.1016/j.jhazmat.2020.124402
Keywords: uranium, uranium tailing, simulated rainfall, leaching characteristics, release mechanism
Citation: Xue S, Wang Z, Ouyang J, Zhou L and Liu Z (2022) Release behavior of uranium from a uranium tailing in Jiangxi Province. Front. Environ. Sci. 10:995533. doi: 10.3389/fenvs.2022.995533
Received: 16 July 2022; Accepted: 21 September 2022;
Published: 12 October 2022.
Edited by:
Oladele Ogunseitan, University of California, Irvine, United StatesReviewed by:
Baogang Zhang, China University of Geosciences, ChinaQinhe Pan, MOFs, Sensors, Adsorption, China
Xiaoyan Wu, University of South China, China
Copyright © 2022 Xue, Wang, Ouyang, Zhou and Liu. This is an open-access article distributed under the terms of the Creative Commons Attribution License (CC BY). The use, distribution or reproduction in other forums is permitted, provided the original author(s) and the copyright owner(s) are credited and that the original publication in this journal is cited, in accordance with accepted academic practice. No use, distribution or reproduction is permitted which does not comply with these terms.
*Correspondence: Zhirong Liu, emhybGl1QGVjaXQuY24=