- 1Department of Soil and Environmental Sciences, The University of Agriculture, Peshawar, Pakistan
- 2Department of Agronomy, The University of Agriculture, Peshawar, Pakistan
- 3Department of Horticulture, Ondokuz Mayis University, Samsun, Turkey
- 4Department of Crop and Soil Sciences, University of Georgia, Athens, Georgia
- 5Department of Agriculture, Bacha Khan University, Charsadda Khyber Pakhtunkhwa, Pakistan
Soil salinity is one of the significant abiotic threats to crops that deteriorates crop yields, and the world’s increasing population faces serious food problems due to abiotic threats. It is one of the major abiotic problems affecting more than 30% of irrigated land across the globe. The concentrations of various salts, such as NaCl, KCl, Na2SO4, and Na2CO3, cause saline stress; however, NaCl is the most abundant salt in the soil. Salinity could affect seed germination due to osmotic potential or due to specific toxic ion effects, and it decreases germination percentage and increases germination time, and high salt stress is responsible for delaying seed germination. Therefore, it is necessary to alleviate the negative impact of soil salinity during seedling growth periods, so the growth of crops in salt-affected soil will be much more enhanced. Seed priming is the utmost effective technique that could mitigate the harmful impact of soil salinity. This methodology not only minimizes the salinity tolerance but also strengthens the defense system of crops. In this technique, the hydration level within the seeds is controlled by applying pre-sowing treatments, allowing specific pre-germinative metabolic processes to occur and preventing radical emergence. Seed priming also decreases the seed germination time and improves antioxidant enzyme activities, stopping or minimizing reactive oxygen species’ adverse effects. It enhances the seedling performance with rapid and homogenous germination and vigorous and dynamic growth of the seedling, achieving a physiological situation leading to quick and enhanced emergence and germination of various crops. This review covers the mechanisms of seed priming, salinity tolerance, seed priming crosstalk with salinity tolerance, and seed priming techniques that induce biochemical, physiological, and morphological mechanisms in saline stress. Further research needs to be performed on advanced seed priming methods such as priming with nanoparticles and seed priming with physical agents (UV radiation, X-rays, gamma rays, and microwaves) to minimize the negative impact of salinity stress on different crops under different harsh environmental conditions.
Introduction
Extreme temperature, drought, heavy metal toxicity, and soil salinity are the primary damaging abiotic plant stresses. A harsh environmental condition applies a considerable amount of stress on plants’ existence in which salinity is the principal prevailing abiotic stress. This sharply hinders the growth of the plant and developmental processes and brings a substantial annual loss in the yield (Mbarki et al., 2018; Safdar et al., 2019). The impact of salinity stress is more dominant in coastal, arid, and semi-arid areas of the world (Shalaby and El-Messairy, 2018). Saline soils contain various dissolved salts, including NaCl, MgSO4, Na2SO4, KCl, MgCl2, CaSO4, and Na2CO3. These salts’ presence contributes to salt stress; however, NaCl, among other salts, is the most predominant salt and focuses on salinity studies. Accumulation of higher salts occurs in the upper top 10 cm layer of the soil than in lower soil layers (Esechie, 1995; Al-Saady et al., 2012). Seeds of cultivated plants are generally sown in the top layer of the soil. Salinity is becoming more widespread due to less sustainable irrigation practices, less effective salinity reclamation methods, and bringing marginal area for crop production (Kang et al., 2014; Kopittke et al., 2019). The saline soils exceed 800 million hectares worldwide, which is nearly about 6 percent of the world’s entire land area (Munns and Tester 2008; FAO, 2011). According to the FAO (2011), water-logging and interrelated salinity have adversely influenced 60–80 million hectares of soil. Soil deteriorated by salinity is not less than 10 mha of agricultural soil annually worldwide, and land degradation due to salinity is a very significant problem worldwide, which has negatively affected land productivity (Qadir et al., 2014; Hossain et al., 2020). At the end of the 21st century, more than 50% of agricultural land will be lost due to soil salinity problems (FAO, 2011).
Soil salinity influences plants’ growth at all developmental periods, but it varies from one growth stage to another. Seed germination is a critical growth stage that could be negatively affected by high salinity. The survival of plants during germination and emergence is considered the most important sign of tolerance to salt. Therefore, most crop species are salt sensitive at the early stages of growth, comprising seedling and germination growth stages (Wang and Han, 2009). Restricted water uptake results from biochemical, physiological, and structural changes in a germinating seed and is the ultimate reason for poor seed germination (M. A. Ashraf and Ashraf, 2012; M. Ashraf and Foolad, 2005). Adaptation of plants to a saline environment during growth stages (germination and early seedling growth) is critical for stand establishment. The germination initiation was delayed in susceptible plant seeds due to salinity stress (E. A. Ibrahim, 2016). Soil salinity stress is also responsible for seedling establishment and slow germination of seeds (Q. Ali et al., 2017; Jisha et al., 2013). Additionally, in later stages after stand establishment, the Na+ toxic concentration in leaves encourages a decline in photosynthesis and premature leaf senescence by limiting carbon assimilation (Hussain S. et al., 2018; Saddiq et al., 2019). A high salt concentration reduces percentage germination and extends germination time (Sucre and Suárez, 2011; Ansari et al., 2019). The amount and percentage of germination of germinating seeds fluctuate at a specific time among cultivars and species. Läuchli and Grattan, 2007 reported a comprehensive relationship between time and percentage of germination after water addition at various salt levels. Salinity could affect plant growth and seed germination by creating osmotic stress or specific ion effects. This results in stomatal closure and imbibition of several biochemical and physiological paths, which include uptake of nutrients (like K+ and Ca+2) and CO2 assimilation (Safdar et al., 2019; H. Alam et al., 2021b; Fu et al., 2020; Parihar et al., 2015). Soil salinity stress causes a reduction in the early growth rate of crops. The reductions in plant growth due to greater salinity result from specific ion effect (high Na+ and Cl− ions) and osmotic effect, which induce a water deficit situation on critical biochemical processes (Munns and Tester, 2008; Hasanuzzaman et al., 2013; Marriboina et al., 2017). The inhibition of plant growth due to salinity stress in soil water is because of two reasons. First, it creates an osmotic effect, and second, it causes a salt-specific ion effect. These two effects of saline stress give rise to a two-stage growth response to salt (Munns, 1993, 2005) (Figure 1).
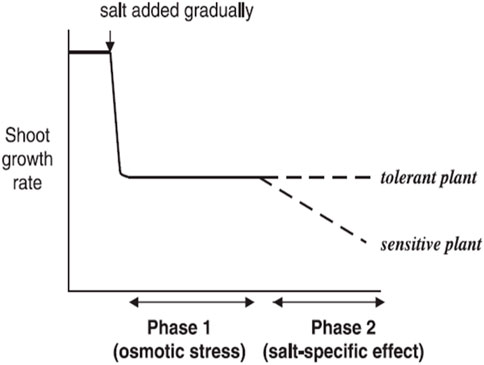
FIGURE 1. Salinity induces a two-phase growth performance in different salt-sensitive plants (Munns, 2005).
Salinity tolerance
Salinity tolerance can be defined as crops’ ability to grow well and complete their life cycle in high saline soil. Crops are generally classified into two groups (halophytes and glycophytes) based on their tolerance to salts. Halophytes are those plants that can grow well in a saline environment and tolerate a high level of salt stress, while glycophytes are highly salt-sensitive plants that cannot tolerate a high concentration of salt stress (Ellouzi et al., 2011; Hapani and Marjadi, 2015) (Figure 2). There is a great difference between species and even cultivars based on their ability to tolerate salinity stress (Izadi et al., 2014).
Seed priming crosstalk with salinity tolerance
Rapid germination of seeds and stand establishment are essential aspects that influence crop production in stressful environments. Therefore, it is vital to improve cultivated crop tolerance to high salinity stress. Different strategies are used to improve the salinity tolerance of other cultivated crops; one of the best approaches is seed priming, which has been evaluated as an active method to alleviate salinity stress (Munns and Gilliham, 2015; Banerjee and Roychoudhury, 2018; Farooq et al., 2019). This methodology minimizes the abiotic stress tolerance; moreover, it strengthens the protection system of crops. In this technique, the hydration level within the seeds is controlled by applying pre-sowing treatments, allowing specific pre-germinative metabolic processes to occur and preventing radical emergence (M. A. Ashraf and Ashraf, 2012; M. Ashraf and Foolad, 2005; Lutts et al., 2016; D. Singh and Kumar, 2021). Such a phenomenon is termed as enhanced stimulation defense mechanisms of a plant (Anderson et al., 2017; Yang et al., 2022). Seed priming decreases the time duration between planting and the emergence of a seed. It induces physiological modification within the target seeds, which consume natural and synthetic compounds within their pre-germination period. It is a beneficial technique for modifying seed quality through specific germinative metabolic processes, which involve osmotic adjustments, membrane reorganization and restructure, and retarding of electrolyte seepage (Mansour et al., 2019; Srivastava et al., 2021). It also helps in DNA replication responses, antioxidant function, more ATP availability, and encouraged protein biosynthesis, restoring cellular bio-membranes, and improving antioxidant enzymes (Chen and Arora, 2013; Hussain et al., 2015; Hussain et al., 2019; Mansour et al., 2019; Sen and Puthur, 2020). Anaytullah 2007 stated that the seed priming process strengthens the biochemical status of plants by inducing the activity of hydrolyses (e.g., amylase) and levels of sugars in germination whereas the enzyme (nitrate reductase) and nitrogen content in seedlings under normal conditions concerning unprimed seeds. Moreover, the seed priming role has been well known in improving germination and growth of various crops under various abiotic stresses (Hussain M. I. et al., 2018; Jisha et al., 2013). The antioxidant enzyme defense system enhanced in primed seeds, which has improved levels of superoxide dismutase (SOD), catalase (CAT), glutathione reductase (GR), peroxidase (POD), and ascorbic acid and regulates stress proteins, i.e., aquaporin, dehydrins (DHNs), and late embryogenesis abundant proteins (Blokhina et al., 2003; Chen and Arora, 2013; Zhu et al., 2017). Seed priming enhances the performance of seeds with rapid and homogenous germination and vigorous growth of seedlings, producing favorable physiological conditions which lead to faster and healthier germination of various crops (Abdel-Moneim Khafagy, 2017; A. Khan et al., 2017; Mondo et al., 2016; Patel et al., 2017; Yücel and Heybet, 2016).
Mechanism of seed priming
A method before sowing involves partly hydration of seeds for a specific period in a particular environment followed by drying; this phenomenon is usually termed as seed priming. Seed priming is an essential and effective strategy that provides a vital defense mechanism and abiotic stress tolerance. Seed priming allows the physiochemical processes (metabolic activities) before germination in treated seeds, keeping the optimum hydration level before sowing and restricting radicle emergence (Ahmad et al., 2021; do Espirito Santo Pereira et al., 2021; Hussain et al., 2019; E. A. Ibrahim, 2016; Hussain et al., 2015; Lutts et al., 2016; Sheteiwy et al., 2021). The priming process permits processes required for germination before germination. Seed priming in water contributes to metabolic changes essential for germination like breaking seed dormancy, activation of enzymes, and water imbibition (Ajouri et al., 2004; Dagar et al., 2021; Islam et al., 2021; Rehman et al., 2021; Bhardwaj et al., 2022). Germination requires moisture; therefore, seed priming provides moisture suitable for germination, which is just less than the actual moisture necessary for germination, however, ideal for metabolic processes involved in germination (Khan M. I. et al., 2021; Cao et al., 2022; Dagar et al., 2022; Khan et al., 2022).
Additionally, seed hydration activates germination in three phases: lag phase, imbibition, and radicle protrusion over the testa (E. A. Ibrahim, 2016). The water supply is controlled to seed in seed priming to make the seed moisture at a level needed for actual germination. This level is sufficient to initiate various physiological processes linked with the early phase of germination; however, the seed transition is avoided toward complete germination (M. Ashraf and Foolad, 2005; Jisha et al., 2013; Nawaz et al., 2013; Paparella et al., 2015). The initial growth stages, in which phase III is avoided, whereas the lag and hydration phases, also known as phase II and phase I, are achieved by primed seeds (Moreno et al., 2018; E. A.-A. Ibrahim, 2019). Before completing germination, the reserves (nutrients and moisture) in primed seeds are actually converted that efficiently nourish the newly formed embryo. When suitable environmental conditions are achieved, the primed seeds transfer to the next (third) phase of germination, i.e., protrusion of the radicle (Rajjou et al., 2012; E. A. Ibrahim, 2016; Kubala et al., 2015a) (Figure 3).
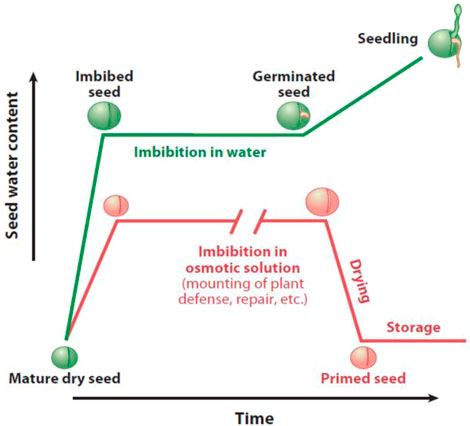
FIGURE 3. Graphical demonstration of the water uptake inside the seed in the seed priming process and standard germination (Rajjou et al., 2012).
Before seeds dry, the treated seeds are rinsed and dried to the optimum level essential for seed storage. Seeds are dried after priming to attain a vital benefit of storing seeds and having a beneficial effect of maintaining quality and avoiding the worsening of seeds (Di Girolamo and Barbanti, 2012; Bhanuprakash, 2015; Pawar and Laware, 2018; Sano et al., 2020). Seed vigor and viability are affected by seed drying once the radicle emergence initiates; however, this can be managed in phase III without any loss by gaining starter moisture (E. A. Ibrahim, 2016; Rajjou et al., 2012). The treated or primed seed possesses two vital qualities of dryness (desiccation tolerance) and durability (longevity), for which these treated seeds can be stored up to sowing (M. A. Ashraf and Ashraf, 2012; M. Ashraf and Foolad, 2005). Durability or longevity is very closely associated with storage and dehydration procedures. Rapid dehydration might vary the soluble carbohydrate content, and the desiccation tolerance and slow dehydration of these treated seeds increase the seed’s durability (Khalaki et al., 2021). Primed seeds are useful to be stored, but their life span compared to non-primed seeds is less; therefore, an optimal storage condition is highly recommended to avoid any seed damage during the storage period.
Priming is not a simple emulation of the early imbibition germination phase. It is responsible for activating the stress-responsive system by imposing adequate stress on seeds, which confers a cross-tolerance on seeds when it opens to future stresses (Bhanuprakash and Yogeesha, 2016). In the early germination phase, metabolic activities took place in primed seeds such as RNA and protein production, structural (membrane fortification in imbibition time), and genetic repair and antioxidant mechanisms. These processes are responsible for seedling growth and good germination (Saddiq et al., 2019; Li et al., 2021, 2022).
Seed priming techniques
Seed priming could be conducted by using conventional and advanced methods as shown in Figure 4. Conventional methods include halo-priming (seeds soaked in salt solutions), hydro-priming, osmopriming, solid matrix priming, and bio-stimulators (ASA and SA) (Abdel-Moneim Khafagy, 2017; A. Khan et al., 2017; A. Z. Khan and Muhammad, 2017; Mondo et al., 2016; Ruttanaruangboworn et al., 2017). Advanced methods include seed priming with nanoparticles and seed priming through physical agents (UV radiation, X-rays, and microwaves). Halo-priming includes soaking of seeds in brine solutions (KCl and NaCl) up to concentrations of 50–200 mM (Afzal et al., 2008; Shabala and Munns, 2012; El-Sanatawy et al., 2021; M. Kumar et al., 2016; Sahab et al., 2020). Again, seed treatment’s critical role before sowing with different salt concentrations is to encourage salt tolerance against salinity in emerging seedlings (Basra et al., 2005). The published literature review has indicated that halo-priming with CaCl2 and KCl mitigates salinity stress by regulating seedling growth, phenolic and proline concentration, and photosynthetic activity (A. Khan et al., 2019a, Khan A. et al., 2021; M. Kumar et al., 2016).
Table 1 presents that seed priming with CaCl2 has increased shoot fresh and dry weight, root fresh and dry weight, and root length, except for root and shoot Na+ concentration in wheat varieties (Khan M. N. et al., 2019). Different priming techniques improved the height of the plant, chlorophylls a and b, leaf area, total soluble protein and phenolics, glycine betaine, proline, K+ content, osmotic potential, cell membrane stability, relative water content, grain yield, and harvest index while decreasing leaf MDA and Na+ content under each level of imposed salinity in barley (Tabassum et al., 2018). Mehboob et al. (2018) concluded that different priming techniques followed by redrying and surface drying were the most effective in enhancing seedling emergence and maize crop growth. Soil salinity deleteriously affected seed germination, seedling potency, plant establishment, and yield of crops. Ali et al. (2017) investigated that seed priming with sodium nitroprusside effectively decreased the negative impact of saline stress-induced oxidative stress and played an essential role due to NO in the enhancement of seed vigor, germination, and initial formation of seedlings. They concluded that seed priming with sodium nitroprusside regulated the antioxidative defense mechanisms, encouraged salt tolerance, and resulted in high biomass and grain yield. The seeds of maize primed with CaCl2 and NaCl took less time for germination than unprimed seeds, and the impact of priming was significant in early growth, seed germination, number of branches, number of cobs, and seed yield (Gebreegziabher and Qufa, 2017).
Al-Tamimi et al. (2016) reported that CaCl2 priming mitigated salt stress in all varieties by enhancing chlorophyll content and growth, stabilizing cell membranes, encouraging nitrate reductase activity, and hindering ROS activities. Seeds primed with KCl and CaCl2 has increased germination, antioxidant enzyme activities (CAT, POD, and APX), enhanced proline accumulation, and reduced the accumulation of H2O2 in spring wheat varieties. The method of priming with glycine betaine, vitamin B12, sodium nitroprusside, jasmonate, CaCl2, and KCl) improves the activity of POX, APX, and SOD and decreases MDA and H2O2 levels in crops under salinity problems (Sadeghi and Robati, 2015; Keshavarz and Moghadam, 2017). The seed priming with ZNPs, glycine betaine, jasmonate, and 3°C chilling enhances APX, POX, and SOD activity, which is related to enhanced nutrient contents of Ca+2, K+, Zn, and Mg+2 in salt-stressed crops (Alasvandyari et al., 2017; M Iqbal and Ashraf, 2007a). Through inorganic salts, the priming process enhances antioxidant enzyme activities in the germination of seeds and regulates organic substance utilization in various portions of an embryo. Additionally, seed priming with ZnSO4 has improved POD, CAT, and SOD activities as compared with hydropriming (Aboutalebian and Nazari, 2017). Such actions also mitigated stress induced by drought by enhancing the antioxidants in N. sativa seedlings (Fallah et al., 2018). Azooz (2009) indicated that the priming of faba bean seeds with SA improved stress tolerance by enhancing POX, APX, CAT, and GR activities. Through seed priming with polyamines, the saline stress tolerance improved rice seedlings by reducing oxidative damage and altering osmolytes, antioxidants, and the photosynthetic system. It also boosted antioxidant enzyme activation and reduced levels of H2O2 and MDA (Paul and Roychoudhury, 2017).
A novel mechanism for seed priming modulates molecular, physiologic and biochemical variations
Seed priming enhances rapid and healthy seed growth and vigorous seedling production under saline stress or normal situations. This is due to the priming stimulation of molecular, physiological, and biochemical changes. These alterations consist of cell enlargement and cell division, upsurge in the production of protein, antioxidant enzyme defense system, and stress receptive proteins (e.g., LEA proteins, dehydrins, and heat shock proteins), epigenetic changes, changes in hormone biosynthesis genes, weaken endosperm and its cap, variations in transcriptome and proteome (Jisha et al., 2013; Alam A. et al., 2021; Ahmad et al., 2021). Abdelhamid et al. (2019) proposed a systematic diagram (Figure 5) in which they explained that seed priming improved redox network, primary and secondary metabolism, osmoregulation, photosynthesis and stomatal conductance, photosynthetic rate and dry matter production, enhanced salinity stress tolerance, and finally plant yield. Additionally, seed priming creates minor stress during the drying of the seed-stage, which suppresses radicle protrusion but later permits seeds to struggle with stress treatment (M. Ashraf and Foolad, 2005; Chen and Arora, 2013; Zulfiqar, 2021). Seed priming induces stress and also improves ROS production, while in small amounts, it functions in signaling networks.
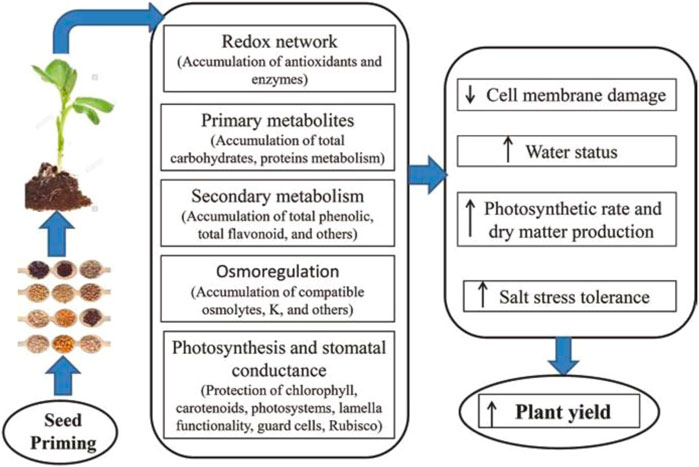
FIGURE 5. Schematic diagram showing the impact of seed priming on physical, chemical, and biological processes (Abdelhamid et al., 2019).
A series of biochemical, cellular, physiological, systemic, and molecular changes is triggered by priming, and this increases plant growth in abiotic stress settings (Varier et al., 2010; Gupta and Huang, 2014; El-Sanatawy et al., 2021; Hussain et al., 2022).
The influence of seed priming on molecular variations
Seed priming enhances the uniformity and germination rate through metabolic restoration during osmotic adjustment, imbibition, and stimulation of pre-germinative metabolic developments in seeds. These comprise an impact on DNA processing, initial reserve mobilization, and reparation mechanisms during duplication (E. A. Ibrahim, 2016; Läuchli and Grattan, 2007; Lutts et al., 2016). However, priming also encourages sprouting activities, i.e., reserve mobilization, energy metabolism, respiration, promoting the enlargement of embryo cells, and endosperm weakening (Lal et al., 2018; Sher et al., 2019; Xu et al., 2021).
By repairing DNA pathways, controlling degrading enzymes, catalase, and antioxidant enzymes, producing nucleic acids and proteins, and accumulating sterols and phospholipids, seed priming improves seed germination (Afzal et al., 2013; Kubala et al., 2015a; Paparella et al., 2015). Furthermore, the appropriate development of seedlings from primed seeds might be due to abiotic stress-responsive proteins, increased plasma membrane fluidity, cell division, and elongation (E. A. Ibrahim, 2016). Similarly, the seed priming process encourages specific defense system mechanisms associated with abiotic stress responses. It enhances the heat shock proteins (HSPs), the addition of defensive proteins, and late embryogenesis abundant and stimulates the membrane efflux pumps (Chen and Arora, 2013; Shalaby and El-Messairy, 2018). It also enhances the protein production potential and post-translational modifications, which encourages abiotic stress tolerance (Kubala et al., 2015).
The seed priming process induces the cross-tolerance manifestation in germinating seeds due to enhanced stress tolerance (Chen and Arora, 2013). The method of seed priming that creates abiotic stress tolerance is likely obtained by two separate approaches. During the first approach, priming encourages the pre-germination metabolism processes such as elongation of embryo cells, improvement in the energy metabolic rate, initial mobilization of reserve food in the seed, and weakening of the endosperm (Chen and Arora, 2013; Becerra-Vázquez et al., 2020; Tania et al., 2020), which is responsible for the transformation of inactive seeds to the state of germination and in turn increases germination. Seed priming executes stresses upon seeds due to which radicle protrusion is suppressed but encourages enzyme activation, support stress responses, osmotic adjustment, and cross-tolerance to abiotic stresses in the second approach. These tolerance stress approaches make a “priming memory” in seeds (primed seeds) that could be employed upon the release of stress and facilitate additional stress tolerance (Chen and Arora, 2013; Hussain et al., 2016; E. A. Ibrahim, 2016; Johnson and Puthur, 2021; Marthandan et al., 2020; Tania et al., 2020).
The boosted germination conducted by the seed priming process is linked to the abundance of the protein involved in the tolerance of oxidative stress, removal of dormancy blocks, membrane repair, targeted proteolysis and post-translation processing capacity, development of immature embryos, modification of embryo tissues, and improvement of pre-germination metabolism (Kubala et al., 2015b; Chen et al., 2021). The priming process-induced molecular responses include expression of the gene to produce new RNAs and proteins and the DNA repair mechanism (Varier et al., 2010). The cycle of DNA repair must be maintained at sufficient rates inside the embryo to promote germination (Wojtyla et al., 2016; Sisodia et al., 2018; Mansour et al., 2019; Rhaman et al., 2021; Baig et al., 2022). During imbibition, the triggered DNA repair processes reactivate the cell cycle of embryonic cells and pass through DNA replication that improves germination output, but faulty DNA repair significantly causes cell death (Balestrazzi et al., 2011a; Balestrazzi et al., 2011). Moreover, seed priming activates DNA repair, which is the most important process, and regulates the potential priming advantages (Rajjou et al., 2012; Wojtyla et al., 2016; Rao et al., 2019). Seed priming can enhance germination through the regulation of specific genes (germination-related genes, plant growth-promoting genes, and antioxidants) (Sadeghi and Robati, 2015; Wojtyla et al., 2016; Panuccio et al., 2018).
The effects of seed priming on physiological variations
The mechanism in which plants maintain a sufficient amount of water for growth and germination by collecting compatible solutes is called the osmotic adjustment (Farhoudi, 2011; Farhoudi et al., 2011; Mansour and Ali, 2017). Seed priming encourages vital physiological mechanisms such as osmotic adjustment and ion homeostasis (M. Ashraf and Foolad, 2005; Sheteiwy et al., 2021; Zhang et al., 2019). Furthermore, osmolytes such as soluble sugars, proline, sugar alcohols, glycine betaine, organic acids, and trehalose play an important role in seed germination after seed priming (Abdel Latef and Tran, 2016; M. Ashraf and Foolad, 2005; Jiang et al., 2022). Under stress conditions, these osmolytes play an essential role in defensive and signaling functions (Iqbal and Ashraf, 2007b; Mansour and Ali, 2017; Chen et al., 2022), and such agents formed in primed seeds enhance germination and increase tolerance to subsequent stress environments (Jiménez-Arias et al., 2015; Tan et al., 2022). The addition of these solutes, as well as inorganic ions, reduces the solute potential of the cells, resulting in a potential gradient of water, and thereafter enables water to be absorbed from the adjacent environment, which results in fast seed germination and stimulates metabolic processes before germination (M. Ashraf and Foolad, 2005; E. A. Ibrahim, 2016; Mansour and Ali, 2017). Flowers et al. (2010) stated that excessive toxic ion omission is a physiologically plant adaptive trait to get saline tolerance. Primed seeds encourage salinity tolerance in plants which is credited to increasing osmotic modification and ionic adjustment maintenance with the accumulation of K+ and Ca+2 (Farhoudi, 2011). Primed seeds reduce the toxic accumulation of Na+ and Cl− while increasing K+ and Ca+2 and K+/Na+ ratios in seedling growth under saline stress conditions (Akram et al., 2010; M. A. Ashraf and Ashraf, 2012; M. Ashraf and Foolad, 2005). Moreover, seed priming strengthens the physiological base (germination) under the high stress conditions, thereby enhancing the growth of plants.
The dynamic uptake of inorganic ions starts the process of osmoregulation in crops. The process of seed priming mitigates the deleterious effects of saline stress on seed germination and healthy development of seedlings that enhances the addition of Ca+2 and K+ and decreases Na+ and Cl− accumulation in emerging seedlings (Afzal et al., 2008; Hussain M. I. et al., 2018; Iqbal et al., 2006), causing maximum uptake of water with less osmotic potential (M. Ashraf and Foolad, 2005; M Iqbal and Ashraf, 2007a). The role of K+ is significant in enzyme activation, in balancing membrane and turgor potential, and in osmotic regulation of cells (Chérel, 2004; Qin et al., 2022). Similarly, calcium also performs vital roles in cell division and elongation, sustains the integrity of the cell wall, adjusts nutrient uptake through the membrane, increases water uptake in crops, and mitigates the deleterious impact of sodium throughout crop growth (Chérel, 2004; Damalas et al., 2019; Mansour et al., 2019).
The effects of seed priming on biochemical variations
Biochemical variations include the enzymatic activities and antioxidant defense induction through seed priming under a high saline stress scenario. The enzymes involved in cell metabolism, such as esterase, acid phosphatase, and glyoxysome enzymes, as well as those involved in the consumption of accumulated reserves (isocitrate lyase and amylases) perform better after the seeds have been primed (Lee and Kim, 2000; Varier et al., 2010; Di Girolamo and Barbanti, 2012). Furthermore, priming enhances antioxidant enzyme activities and addition of non-enzymatic antioxidants (e.g., soluble sugars, GSH, proline, and ASA) (M. Ali et al., 2019; R Farhoudi, 2012; Farhoudi et al., 2011; Fu et al., 2020; Nawaz et al., 2013; Sedghi et al., 2010). These enhancements can further strengthen the defense mechanism by reducing ROS production (e.g., H2O2 and O2) and improving the ROS scavenging during the germination of seeds (Sedghi et al., 2010; Paparella et al., 2015; Banerjee and Roychoudhury, 2018). Seed priming develops the metabolic activities during germination inside the seeds required for breaking the dormancy of seeds, activation of enzymes, protein synthesis and nucleic acids (such as DNA and RNA), transcription and translation, repair mechanisms, germination metabolism, DNA duplication, antioxidant enzyme activities, enhancing the content of ATP and quantity of mitochondria, repairing destroyed seed parts, and reducing leakage of metabolites. Generally, priming motivates sequences of biochemical, metabolic, and physiological changes that are associated with improvement of healthy growth and germination performance and enhance tolerance of abiotic stresses (E. A. Ibrahim, 2016; Tanou et al., 2012; Gao et al., 2021). Compared to normal imbibition, the advantages of seed priming comprise the upregulation of genes and proteins involved in cell elongation and division, translation, mobilization of the reserve, modification of the cell wall, transcription, water transport, and stress oxidative response, as well as membrane and DNA repair (Farooq et al., 2020; Kubala et al., 2015a).
The antioxidant system is the most critical protection system in germinating primed seeds to regulate the addition of reactive oxygen species under abiotic stress (Gupta and Huang, 2014; Paparella et al., 2015; Banerjee and Roychoudhury, 2018). Seed priming encourages the activities of antioxidant enzymes, for e.g., SOD, GR, POX, and CAT, and increases nonenzymatic antioxidant levels (i.e., ascorbic acid). These activities are responsible for hindering reactive oxygen species and seed protection by reduction of hydrogen peroxide and superoxide production (Biosci et al., 2019; E. A. Ibrahim, 2016; Paparella et al., 2015; Sedghi et al., 2010). Furthermore, it encourages the addition of photoprotective pigments (anthocyanin) that increase ROS scavenging and crop protection (Banerjee and Roychoudhury, 2016, 2018; Wang et al., 2022). The addition of MDA decreases with seed priming, which increases with abiotic stress, and is further used as an oxidative stress measure. This decline is related to improved membrane repair of antioxidant enzyme inductive responses during the seed priming process (Bhanuprakash, 2015; Banerjee and Roychoudhury, 2018; Hossain et al., 2020; Zulfiqar, 2021).
Conclusion
From the literature, it is concluded that the process of seed priming with various priming materials could mitigate salinity stress through the development of physiological, biochemical, and morphological mechanisms in germinating seeds, which enhance saline tolerance of different crop seeds to ameliorate salinity. Seed priming enhances antioxidant enzyme activities (APX, POD, SOD, and POX), which helps in the scavenging of ROS such as hydroxyl radicals (-OH) H2O2, and superoxide (O2−), resulting in better seedling growth and germination. Furthermore, seed priming enhances crops’ overall yield by reducing germination time, enhancing germination percentage, germination index, seedling growth, total water content, and photosynthesis activity, and improving saline tolerance of crops under saline stress conditions. Additionally, seed priming also reduces MDA accumulation, which is related to inductive responses of antioxidant enzymes and better membrane repair. The application of seed priming decreases the toxic ion effect by reducing the Na+ and Cl− uptake and increasing the K+ and Ca+2 accumulation in developing seedlings. Furthermore, seed priming also encourages photoprotective pigments (anthocyanin) that increase ROS scavenging and crop protection. Various kinds of priming agents have varying properties and effects on different crops.
Future prospects
• Different crop capabilities need to be evaluated under varying saline and environmental conditions.
• Different seed priming chemicals need further exploration for enhanced seed germination and growth and their evaluation on crop production under saline-stressed environments.
• Different priming methods, including osmopriming, bio-priming, hydro-priming, halo-priming, and hormonal priming, with various priming agents in different proportions need to be studied on different crops under different salinity conditions.
• Rapid seed growth and crop production need to be the utmost duty of scientists to achieve food security goals for humanity in the near future under different adverse environmental conditions.
• Advanced seed priming methods such as priming with nanoparticles and seed priming with physical agents (UV radiation, X-rays, gamma rays, and microwaves) need to be used in order to minimize the salinity stress and finally increase the overall yield of different crops.
Author contributions
Manuscript writing MOK, Supervision and conceptualization MOK, MI, Rewriting and Editing AM, SN, MKK, Proofreading and reviewing IU, MaA. All authors have read and approved this manuscript.
Acknowledgments
The authors express their gratitude to the editorial board and reviewer for their efforts for suggesting and reviewing this work. The authors also appreciate the editor for his cooperation during the review process.
Conflict of interest
The authors declare that the research was conducted in the absence of any commercial or financial relationships that could be construed as a potential conflict of interest.
Publisher’s note
All claims expressed in this article are solely those of the authors and do not necessarily represent those of their affiliated organizations, or those of the publisher, the editors, and the reviewers. Any product that may be evaluated in this article, or claim that may be made by its manufacturer, is not guaranteed or endorsed by the publisher.
Abbreviations
AA, ascorbic acid; APX, ascorbate peroxidase; DHNs, dehydrins; GR, glutathione reductase; ABA, abscisic acid; CAT, catalase; HSPs, heat shock proteins; LEA, late embryogenic abundance; MDA, malondialdehyde; POD, peroxidase; POX, peroxidase reductase; ROS, reactive oxygen species; SOD, superoxide dismutase.
References
Abdel Latef, A. A., and Tran, L.-S. P. (2016). Impacts of priming with silicon on the growth and tolerance of maize plants to alkaline stress. Front. Plant Sci. 7, 243. doi:10.3389/fpls.2016.00243
Abdel-Moneim Khafagy, M. (2017). Effect of pre-treatment of barley grain on germination and seedling growth under drought stress. Advances in Applied Sci. 2 (3), 33. doi:10.11648/j.aas.20170203.12
Abdelhamid, M. T., El-masry, R. R., Darwish, D. S., Abdalla, M. M. F., Oba, S., Ragab, R., et al. (2019). “Priming and pretreatment of seeds and seedlings,” in Priming and pretreatment of seeds and seedlings. doi:10.1007/978-981-13-8625-1
Aboutalebian, M. A., and Nazari, S. (2017). Seedling emergence and activity of some antioxidant enzymes of canola (Brassica napus) can be increased by seed priming. J. Agric. Sci. 155 (10), 1541–1552. doi:10.1017/s0021859617000661
Afzal, I., Basra, S. M. A., Cheema, M. A., Farooq, M., Jafar, M. Z., Shahid, M., et al. (2013). Seed priming: A shotgun approach for alleviation of salt stress in wheat. Int. J. Agric. Biol. 15 (6), 1199–1203.
Afzal, I., Rauf, S., Basra, S. M. A., and Murtaza, G. (2008). Halopriming improves vigor, metabolism of reserves and ionic contents in wheat seedlings under salt stress. Plant Soil Environ. 54 (9), 382–388. doi:10.17221/408-pse
Ahmad, F., Kamal, A., Singh, A., Ashfaque, F., Alamri, S., Siddiqui, M. H., et al. (2021). Seed priming with gibberellic acid induces high salinity tolerance in Pisum sativum through antioxidants, secondary metabolites and up‐regulation of antiporter genes. Plant Biol. (Stuttg). 23, 113–121. doi:10.1111/plb.13187
Ajouri, A., Asgedom, H., and Becker, M. (2004). Seed priming enhances germination and seedling growth of barley under conditions of P and Zn deficiency. Z. Pflanzenernahr. Bodenk. 167 (5), 630–636. doi:10.1002/jpln.200420425
Akram, M., Ashraf, M. Y., Ahmad, R., Waraich, E. A., Iqbal, J., and Mohsan, M. (2010). Screening for salt tolerance in maize (Zea mays L.) hybrids at an early seedling stage. Pak. J. Bot. 42 (1), 141–154.
Al-Saady, N. A., Khan, A. J., Rajesh, L., and Esechie, H. A. (2012). Effect of salt stress on germination, proline metabolism and chlorophyll content of fenugreek (Trigonella foenum gracium L.). J. Plant Sci. 7 (5), 176–185. doi:10.3923/jps.2012.176.185
Al-Tamimi, N., Brien, C., Oakey, H., Berger, B., Saade, S., Ho, Y. S., et al. (2016). Salinity tolerance loci revealed in rice using high-throughput non-invasive phenotyping. Nat. Commun. 7 (1), 1–11. doi:10.1038/ncomms13342
Alam, A., Ullah, H., Cha-um, S., Tisarum, R., and Datta, A. (2021a). Effect of seed priming with potassium nitrate on growth, fruit yield, quality and water productivity of cantaloupe under water-deficit stress. Sci. Hortic. 288, 110354. doi:10.1016/j.scienta.2021.110354
Alam, H., Khattak, J. Z. K., Ksiksi, T. S., Saleem, M. H., Fahad, S., Sohail, H., et al. (2021b). Negative impact of long‐term exposure of salinity and drought stress on native Tetraena mandavillei L. Physiol. Plant. 172 (2), 1336–1351. doi:10.1111/ppl.13273
Alasvandyari, F., Mahdavi, B., and Hosseini, S. M. (2017). Glycine betaine affects the antioxidant system and ion accumulation and reduces salinity-induced damage in safflower seedlings. Arch. Biol. Sci. 69 (1), 139–147. doi:10.2298/abs160216089a
Ali, M., Hayat, S., Ahmad, H., Ghani, M. I., Amin, B., Atif, M. J., et al. (2019). Priming of Solanum melongena L. seeds enhances germination, alters antioxidant enzymes, modulates ROS, and improves early seedling growth: Indicating aqueous garlic extract as seed-priming bio-stimulant for eggplant production. Applied Sci. 9 (11), 2203. doi:10.3390/app9112203
Ali, Q., Daud, M. K., Haider, M. Z., Ali, S., Rizwan, M., Aslam, N., et al. (2017). Seed priming by sodium nitroprusside improves salt tolerance in wheat (Triticum aestivum L.) by enhancing physiological and biochemical parameters. Plant Physiology Biochem. 119, 50–58. doi:10.1016/j.plaphy.2017.08.010
Anaytullah, Bose B. (2007). Nitrate-hardened seeds increase germination, amylase activity and proline content in wheat seedlings at low temperature. Physiol. Mol. Biol. Plants 13, 199–207.
Anderson, A. J., McLean, J. E., Jacobson, A. R., and Britt, D. W. (2017). CuO and ZnO nanoparticles modify interkingdom cell signaling processes relevant to crop production. J. Agric. Food Chem. 66 (26), 6513–6524. doi:10.1021/acs.jafc.7b01302
Ansari, F. A., Ahmad, I., and Pichtel, J. (2019). Growth stimulation and alleviation of salinity stress to wheat by the biofilm forming Bacillus pumilus strain FAB10. Applied Soil Ecol. 143, 45–54. doi:10.1016/j.apsoil.2019.05.023
Ashraf, M. A., and Ashraf, M. (2012). Salt-induced variation in some potential physiochemical attributes of two genetically diverse spring wheat (Triticum aestivum L.) cultivars: Photosynthesis and photosystem II efficiency. Pak J. Bot. 44 (1), 53–64.
Ashraf, M., and Foolad, M. R. (2005). Pre-sowing seed treatment-A shotgun approach to improve germination, plant growth, and crop yield under saline and non-saline conditions. Advances in Agron. 88 (05), 223–271. doi:10.1016/S0065-2113(05)88006-X
Azooz, M. M. (2009). Salt stress mitigation by seed priming with salicylic acid in two faba bean genotypes differing in salt tolerance. Int. J. Agric. Biol. 11 (4), 343–350.
Baig, I. A., Irfan, M., Salam, M. A., and Işik, C. (2022). Addressing the effect of meteorological factors and agricultural subsidy on agricultural productivity in India: A roadmap towards environmental sustainability.
Balestrazzi, A., Confalonieri, M., Macovei, A., and Carbonera, D. (2011). Seed imbibition in medicago truncatula gaertn: expression profiles of DNA repair genes in relation to PEG-mediated stress. J. Plant Physiology 168 (7), 706–713. doi:10.1016/j.jplph.2010.10.008
Balestrazzi, A., Confalonieri, M., Macovei, A., Donà, M., and Carbonera, D. (2011a). Genotoxic stress and DNA repair in plants: emerging functions and tools for improving crop productivity. Plant Cell Rep. 30 (3), 287–295. doi:10.1007/s00299-010-0975-9
Banerjee, A., and Roychoudhury, A. (2016). Group II late embryogenesis abundant (LEA) proteins: structural and functional aspects in plant abiotic stress. Plant Growth Regul. 79 (1), 1–17. doi:10.1007/s10725-015-0113-3
Banerjee, A., and Roychoudhury, A. (2018). Seed priming technology in the amelioration of salinity stress in plants. Advances in Seed Priming, 81–93. doi:10.1007/978-981-13-0032-5_5
Basra, S. M. A., Farooq, M., Tabassam, R., and Ahmad, N. (2005). Physiological and biochemical aspects of pre-sowing seed treatments in fine rice (Oryza sativa L.). Seed Sci. Technol. 33 (3), 623–628. doi:10.15258/sst.2005.33.3.09
Becerra-Vázquez, Á. G., Coates, R., Sánchez-Nieto, S., Reyes-Chilpa, R., and Orozco-Segovia, A. (2020). Effects of seed priming on germination and seedling growth of desiccation-sensitive seeds from Mexican tropical rainforest. J. Plant Res. 133 (6), 855–872. doi:10.1007/s10265-020-01220-0
Bhanuprakash, K. (2015). “Seed biology and technology,” in Plant biology and biotechnology (Springer), 469–497.
Bhanuprakash, K., and Yogeesha, H. S. (2016). Seed priming for abiotic stress tolerance: an overview. Abiotic Stress Physiology Hortic. Crops, 103–117. doi:10.1007/978-81-322-2725-0_6
Bhardwaj, A., Dagar, V., Khan, M. O., Aggarwal, A., Alvarado, R., Kumar, M., et al. (2022). Smart IoT and machine learning-based framework for water quality assessment and device component monitoring. Environ. Sci. Pollut. Res. Int. 29 (30), 46018–46036. doi:10.1007/s11356-022-19014-3
Biosci, I. J., Khan, M. O., Khan, M. J., Khan, M. A., Shafi, M., Anwar, S., et al. (2019). Wheat yield as affected by sources of sulfur and its time of application. 6655, 37–50.
Blokhina, O., Virolainen, E., and Fagerstedt, K. V. (2003). Antioxidants, oxidative damage and oxygen deprivation stress: a review. Ann. Bot. 91 (2), 179–194. doi:10.1093/aob/mcf118
Cao, H., Khan, M. K., Rehman, A., Dagar, V., Oryani, B., and Tanveer, A. (2022). Impact of globalization, institutional quality, economic growth, electricity and renewable energy consumption on Carbon Dioxide Emission in OECD countries. Environ. Sci. Pollut. Res. Int. 29 (16), 24191–24202. doi:10.1007/s11356-021-17076-3
Chen, F., Aqeel, M., Maqsood, M. F., Khalid, N., Irshad, M. K., Ibrahim, M., et al. (2022). Mitigation of lead toxicity in Vigna radiata genotypes by silver nanoparticles. Environ. Pollut. 308, 119606. doi:10.1016/j.envpol.2022.119606
Chen, K., and Arora, R. (2013). Priming memory invokes seed stress-tolerance. Environ. Exp. Bot. 94, 33–45. doi:10.1016/j.envexpbot.2012.03.005
Chen, X., Quan, Q., Zhang, K., and Wei, J. (2021). Spatiotemporal characteristics and attribution of dry/wet conditions in the Weihe River Basin within a typical monsoon transition zone of East Asia over the recent 547 years. Environ. Model. Softw. 143, 105116. doi:10.1016/j.envsoft.2021.105116
Chérel, I. (2004). Regulation of K+ channel activities in plants: from physiological to molecular aspects. J. Exp. Bot. 55 (396), 337–351. doi:10.1093/jxb/erh028
Dagar, V., Khan, M. K., Alvarado, R., Rehman, A., Irfan, M., Adekoya, O. B., et al. (2022). Impact of renewable energy consumption, financial development and natural resources on environmental degradation in OECD countries with dynamic panel data. Environ. Sci. Pollut. Res. Int. 29, 18202–18212. doi:10.1007/s11356-021-16861-4
Dagar, V., Khan, M. K., Alvarado, R., Usman, V., Dagar, V., Zakari, A., Rehman, A., et al. (2021). Variations in technical efficiency of farmers with distinct land size across agro-climatic zones: Evidence from India. J. Clean Prod. 315, 128109. doi:10.1016/j.jclepro.2021.128109
Damalas, C. A., Koutroubas, S. D., and Fotiadis, S. (2019). Hydro-priming effects on seed germination and field performance of faba bean in spring sowing. Agriculture 9 (9), 201. doi:10.3390/agriculture9090201
Di Girolamo, G., and Barbanti, L. (2012). Treatment conditions and biochemical processes influencing seed priming effectiveness. Ital. J. Agron. 7 (2), e25. doi:10.4081/ija.2012.e25
do Espirito Santo Pereira, A., Caixeta Oliveira, H., Fernandes Fraceto, L., and Santaella, C. (2021). Nanotechnology potential in seed priming for sustainable agriculture. Nanomaterials 11 (2), 267. doi:10.3390/nano11020267
El-Sanatawy, A. M., Ash-Shormillesy, S. M. A. I., Qabil, N., Awad, M. F., and Mansour, E. (2021). Seed Halo-priming improves seedling Vigor, grain yield, and Water use efficiency of maize under Varying irrigation regimes. Water 13 (15), 2115. doi:10.3390/w13152115
Ellouzi, H., Ben Hamed, K., Cela, J., Munné‐Bosch, S., and Abdelly, C. (2011). Early effects of salt stress on the physiological and oxidative status of Cakile maritima (halophyte) and Arabidopsis thaliana (glycophyte). Physiol. Plant. 142 (2), 128–143. doi:10.1111/j.1399-3054.2011.01450.x
Esechie, H. A. (1995). Partitioning of chloride ion in the germinating seed of two forage legumes under varied salinity and temperature regimes. Commun. in Soil Sci. Plant Analysis 26 (19–20), 3357–3370. doi:10.1080/00103629509369532
Fallah, S., Malekzadeh, S., and Pessarakli, M. (2018). Seed priming improves seedling emergence and reduces oxidative stress in Nigella sativa under soil moisture stress. J. Plant Nutr. 41 (1), 29–40. doi:10.1080/01904167.2017.1381719
FAO (2011). The state of the world’s land and water resources for food and agriculture (SOLAW) -Managing systems at risk. London.
Farhoudi, R. (2011). Effect of salt stress on physiological and morphological parameters of rapeseed cultivars. Advances in Environ. Biol., 2501–2509.
Farhoudi, R., Saeedipour, S., and Mohammadreza, D. (2011). The effect of NaCl seed priming on salt tolerance, antioxidant enzyme activity, proline and carbohydrate accumulation of Muskmelon (Cucumis melo L.) under saline condition. Afr. J. Agric. Res. 6 (6), 1363–1370.
Farhoudi, R. (2012). Evaluation effect of KNO3 seed priming on seedling growth and cell membrane damage of sunflower (Heliantus annuus) under salt stress. American-Eurasian J. Agric. Environ. Sci. 12 (3), 384–388.
Farooq, M., Hussain, M., Habib, M. M., Khan, M. S., Ahmad, I., Farooq, S., et al. (2020). Influence of seed priming techniques on grain yield and economic returns of bread wheat planted at different spacings. Crop Pasture Sci. 71 (8), 725–738. doi:10.1071/cp20065
Farooq, M., Usman, M., Nadeem, F., Rehman, H. U., Wahid, A., Basra, S. M. A., et al. (2019). Seed priming in field crops: Potential benefits, adoption and challenges. Crop Pasture Sci. 70 (9), 731–771. doi:10.1071/CP18604
Flowers, T. J., Galal, H. K., and Bromham, L. (2010). Evolution of halophytes: Multiple origins of salt tolerance in land plants. Funct. Plant Biol. 37 (7), 604–612. doi:10.1071/fp09269
Fu, Y., Zhang, Z., Liu, J., Chen, M., Pan, R., Hu, W., et al. (2020). Seed priming with spermidine and trehalose enhances chilling tolerance of rice via different mechanisms. J. Plant Growth Regul. 39 (2), 669–679. doi:10.1007/s00344-019-10009-y
Gao, C., Hao, M., Chen, J., and Gu, C. (2021). Simulation and design of joint distribution of rainfall and tide level in Wuchengxiyu Region, China. Urban Clim. 40, 101005. doi:10.1016/j.uclim.2021.101005
Gebreegziabher, B. G., and Qufa, C. A. (2017). Plant physiological stimulation by seeds salt priming in maize (Zea mays): Prospect for salt tolerance. Afr. J. Biotechnol. 16 (5), 209–223. doi:10.5897/ajb2016.15819
Gupta, B., and Huang, B. (2014). Mechanism of salinity tolerance in plants: Physiological, biochemical, and molecular characterization. Int. J. Genomics, 1–18. doi:10.1155/2014/701596
Hapani, P., and Marjadi, D. (2015). Salt tolerance and biochemical responses as a stress indicator in plants to salinity: a review. CIBTech J. Biotechnol. ISSN.
Hasanuzzaman, M., Nahar, K., and Fujita, M. (2013). “Plant response to salt stress and role of exogenous protectants to mitigate salt-induced damages,” in Ecophysiology and responses of plants under salt stress (Springer), 25–87.
Hossain, A., Krupnik, T. J., Timsina, J., Mahboob, M. G., Chaki, A. K., Farooq, M., et al. (2020). “Agricultural land degradation: Processes and problems undermining future food security,” in Environment, climate, plant and vegetation growth (Springer), 17–61.
Hussain, I., Rehman, A., and Işık, C. (2022). Using an asymmetrical technique to assess the impacts of CO2 emissions on agricultural fruits in Pakistan. Environ. Sci. Pollut. Res. 29 (13), 19378–19389. doi:10.1007/s11356-021-16835-6
Hussain, M. I., Al-Dakheel, A. J., and Reigosa, M. J. (2018b). Genotypic differences in agro-physiological, biochemical and isotopic responses to salinity stress in quinoa (Chenopodium quinoa Willd.) plants: Prospects for salinity tolerance and yield stability. Plant Physiology Biochem. 129, 411–420. doi:10.1016/j.plaphy.2018.06.023
Hussain, S., Hussain, S., Khaliq, A., Ali, S., and Khan, I. (2019). “Physiological, biochemical, and molecular aspects of seed priming,” in Priming and pretreatment of seeds and seedlings (Springer), 43–62.
Hussain, S., Khaliq, A., Tanveer, M., Matloob, A., and Hussain, H. A. (2018). Aspirin priming circumvents the salinity-induced effects on wheat emergence and seedling growth by regulating starch metabolism and antioxidant enzyme activities. Acta Physiol. Plant. 40 (4), 68–12. doi:10.1007/s11738-018-2644-5
Hussain, S., Khan, F., Cao, W., Wu, L., and Geng, M. (2016). Seed priming alters the production and detoxification of reactive oxygen intermediates in rice seedlings grown under sub-optimal temperature and nutrient supply. Front. Plant Sci. 7, 439. doi:10.3389/fpls.2016.00439
Hussain, S., Zheng, M., Khan, F., Khaliq, A., Fahad, S., Peng, S., et al. (2015). Benefits of rice seed priming are offset permanently by prolonged storage and the storage conditions. Sci. Rep. 5, 8101. doi:10.1038/srep08101
Ibrahim, E. A.-A. (2019). “Fundamental processes involved in seed priming,” in Priming and pretreatment of seeds and seedlings (Springer), 63–115.
Ibrahim, E. A. (2016). Seed priming to alleviate salinity stress in germinating seeds. J. Plant Physiology 192, 38–46. doi:10.1016/j.jplph.2015.12.011
Iqbal, M., and Ashraf, M. (2007a). Seed preconditioning modulates growth, ionic relations, and photosynthetic capacity in adult plants of hexaploid wheat under salt stress. J. Plant Nutr. 30 (3), 381–396. doi:10.1080/01904160601171330
Iqbal, M., Ashraf, M., Jamil, A., and ur-Rehman, S. (2006). Does seed priming induce changes in the levels of some endogenous plant hormones in hexaploid wheat plants under salt stress? J. Integr. Plant Biol. 48 (2), 181–189. doi:10.1111/j.1744-7909.2006.00181.x
Iqbal, M., and Ashraf, M. (2007b). Seed treatment with auxins modulates growth and ion partitioning in salt‐stressed wheat plants. J. Integr. Plant Biol. 49 (7), 1003–1015. doi:10.1111/j.1672-9072.2007.00488.x
Iqbal, M., and Ashraf, M. (2013). Gibberellic acid mediated induction of salt tolerance in wheat plants: Growth, ionic partitioning, photosynthesis, yield and hormonal homeostasis. Environ. Exp. Bot. 86, 76–85. doi:10.1016/j.envexpbot.2010.06.002
Islam, F., Yasmeen, T., Ali, S., Ali, B., Farooq, M. A., and Gill, R. A. (2015). Priming-induced antioxidative responses in two wheat cultivars under saline stress. Acta Physiol. Plant. 37 (8), 1–12. doi:10.1007/s11738-015-1897-5
Islam, M., Khan, M. K., Tareque, M., Jehan, N., and Dagar, V. (2021). Impact of globalization, foreign direct investment, and energy consumption on CO2 emissions in Bangladesh: Does institutional quality matter? Environ. Sci. Pollut. Res. Int. 28 (35), 48851–48871. doi:10.1007/s11356-021-13441-4
Izadi, M. H., Rabbani, J., Emam, Y., Pessarakli, M., and Tahmasebi, A. (2014). Effects of salinity stress on physiological performance of Various Wheat and barley cultivars. J. Plant Nutr. 37 (4), 520–531. doi:10.1080/01904167.2013.867980
Jafar, M. Z., Farooq, M., Cheema, M. A., Afzal, I., Basra, S. M. A., Wahid, M. A., et al. (2012). Improving the performance of wheat by seed priming under saline conditions. J. Agron. Crop Sci. 198 (1), 38–45. doi:10.1111/j.1439-037X.2011.00485.x
Jiang, G., Alvarado, R., Murshed, M., Tillaguango, B., Toledo, E., Méndez, P., et al. (2022). Effect of agricultural employment and export diversification index on environmental pollution: building the agenda towards sustainability. Sustainability 14 (2), 677. doi:10.3390/su14020677
Jiménez-Arias, D., Borges, A. A., Luis, J. C., Valdés, F., Sandalio, L. M., and Pérez, J. A. (2015). Priming effect of menadione sodium bisulphite against salinity stress in Arabidopsis involves epigenetic changes in genes controlling proline metabolism. Environ. Exp. Bot. 120, 23–30. doi:10.1016/j.envexpbot.2015.07.003
Jisha, K. C., Vijayakumari, K., and Puthur, J. T. (2013). Seed priming for abiotic stress tolerance: An overview. Acta Physiol. Plant. 35 (5), 1381–1396. doi:10.1007/s11738-012-1186-5
Johnson, R., and Puthur, J. T. (2021). Seed priming as a cost effective technique for developing plants with cross tolerance to salinity stress. Plant Physiology Biochem. 162, 247–257. doi:10.1016/j.plaphy.2021.02.034
Kang, S.-M., Khan, A. L., Waqas, M., You, Y.-H., Kim, J.-H., Kim, J.-G., et al. (2014). Plant growth-promoting rhizobacteria reduce adverse effects of salinity and osmotic stress by regulating phytohormones and antioxidants in Cucumis sativus. J. Plant Interact. 9 (1), 673–682. doi:10.1080/17429145.2014.894587
Keshavarz, H., and Moghadam, R. S. G. (2017). Seed priming with cobalamin (vitamin B12) provides significant protection against salinity stress in the common bean. Rhizosphere 3, 143–149. doi:10.1016/j.rhisph.2017.04.010
Khalaki, M. A., Moameri, M., Lajayer, B. A., and Astatkie, T. (2021). Influence of nano-priming on seed germination and plant growth of forage and medicinal plants. Plant Growth Regul. 93 (1), 13–28. doi:10.1007/s10725-020-00670-9
Khan, A., Shafi, M., Bakht, J., and Anwar, S. (2017). Effect of salinity and seed priming on growth characters of Wheat Varieties. Sarhad J. Agric. 33 (3). doi:10.17582/journal.sja/2017/33.3.435.446
Khan, A., Shafi, M., Bakht, J., Anwar, S., and Khan, M. O. (2021). Effect of salinity (NaCl) and seed priming (CaCl2) on biochemical parameters and biological yield of wheat. Pak. J. Bot. 53 (3), 779–789. doi:10.30848/PJB2021-3(12
Khan, A., Shafi, M., Bakht, J., Khan, M. O., and Anwar, S. (2019a). Response of wheat varieties to salinity stresses as ameliorated by seed priming. Pak. J. Bot. 51 (6), 1969–1978. doi:10.30848/PJB2019-6(42
Khan, A. Z., and Muhammad, A. (2017). Influence of pre-sowing seed treatments on germination properties and seedling vigor of wheat influence of PRE-SOWING seed treatments on introduction wheat ( triticumaestivum L .) is an important food crop of Pakistan and mostly cultivated in rainfed area. April.
Khan, M. I., Kamran Khan, M., Dagar, V., Oryani, B., Akbar, S. S., and Salem, S. (2021). Testing environmental Kuznets curve in the USA: what role institutional quality, globalization, energy consumption, financial development, and remittances can play? New evidence from dynamic ARDL simulations approach. Front. Environ. Sci. 9, 789715. doi:10.3389/fenvs.2021.789715
Khan, M. K., Babar, S. F., Oryani, B., Dagar, V., Rehman, A., Zakari, A., et al. (2022). Role of financial development, environmental-related technologies, research and development, energy intensity, natural resource depletion, and temperature in sustainable environment in Canada. Environ. Sci. Pollut. Res. Int. 29 (1), 622–638. doi:10.1007/s11356-021-15421-0
Khan, M. N., Zhang, J., Luo, T., Liu, J., Rizwan, M., Fahad, S., et al. (2019b). Seed priming with melatonin coping drought stress in rapeseed by regulating reactive oxygen species detoxification: Antioxidant defense system, osmotic adjustment, stomatal traits and chloroplast ultrastructure perseveration. Industrial Crops Prod. 140, 111597. doi:10.1016/j.indcrop.2019.111597
Kopittke, P. M., Menzies, N. W., Wang, P., McKenna, B. A., and Lombi, E. (2019). Soil and the intensification of agriculture for global food security. Environ. Int. 132, 105078. doi:10.1016/j.envint.2019.105078
Kubala, S., Garnczarska, M., Wojtyla, Ł., Clippe, A., Kosmala, A., Żmieńko, A., et al. (2015a). Deciphering priming-induced improvement of rapeseed (Brassica napus L.) germination through an integrated transcriptomic and proteomic approach. Plant Sci. 231, 94–113. doi:10.1016/j.plantsci.2014.11.008
Kubala, S., Wojtyla, Ł., Quinet, M., Lechowska, K., Lutts, S., and Garnczarska, M. (2015b). Enhanced expression of the proline synthesis gene P5CSA in relation to seed osmopriming improvement of Brassica napus germination under salinity stress. J. Plant Physiology 183, 1–12. doi:10.1016/j.jplph.2015.04.009
Kumar, M., Pant, B., Mondal, S., and Bose, B. (2016). Hydro and halo priming: influenced germination responses in wheat var-HUW-468 under heavy metal stress. Acta Physiol. Plant. 38 (9), 217–7. doi:10.1007/s11738-016-2226-3
Lal, S. K., Kumar, S., Sheri, V., Mehta, S., Varakumar, P., Ram, B., et al. (2018). “Seed priming: an emerging technology to impart abiotic stress tolerance in crop plants,” in Advances in seed priming (Springer), 41–50.
Läuchli, A., and Grattan, S. R. (2007). “Plant growth and development under salinity stress,” in Advances in molecular breeding toward drought and salt tolerant crops (Springer), 1–32.
Lee, S.-S., and Kim, J.-H. (2000). Total sugars, $\alpha $-amylase activity, and germination after priming of normal and aged rice seeds. Korean J. Crop Sci. 45 (2), 108–111.
Li, J., Charles, L. S., Yang, Z., Du, G., and Fu, S. (2022). Differential mechanisms drive species loss under artificial shade and fertilization in the alpine meadow of the Tibetan plateau. Front. Plant Sci. 13, 832473. doi:10.3389/fpls.2022.832473
Li, W., Shi, Y., Zhu, D., Wang, W., Liu, H., Li, J., et al. (2021). Fine root biomass and morphology in a temperate forest are influenced more by the nitrogen treatment approach than the rate. Ecol. Indic. 130, 108031. doi:10.1016/j.ecolind.2021.108031
Lutts, S., Benincasa, P., Wojtyla, L., Kubala, S., Pace, R., Lechowska, K., et al. (2016). Seed priming: new comprehensive approaches for an old empirical technique. New Challenges in Seed Biology-Basic Transl. Res. Driv. Seed Technol. 1–46.
Mansour, M. M. F., and Ali, E. F. (2017). Evaluation of proline functions in saline conditions. Phytochemistry 140, 52–68. doi:10.1016/j.phytochem.2017.04.016
Mansour, M. M. F., Ali, E. F., and Salama, K. H. A. (2019). “Does seed priming play a role in regulating reactive oxygen species under saline conditions?” in Reactive oxygen, nitrogen and sulfur species in plants: Production, metabolism, signaling and defense mechanisms 1, 437–488. doi:10.1002/9781119468677.ch18
Marriboina, S., Sengupta, D., Kumar, S., and Reddy, A. R. (2017). Physiological and molecular insights into the high salinity tolerance of Pongamia pinnata (L.) pierre, a potential biofuel tree species. Plant Sci. 258, 102–111. doi:10.1016/j.plantsci.2017.02.008
Marthandan, V., Geetha, R., Kumutha, K., Renganathan, V. G., Karthikeyan, A., and Ramalingam, J. (2020). Seed priming: a feasible strategy to enhance drought tolerance in crop plants. Int. J. Mol. Sci. 21 (21), 8258. doi:10.3390/ijms21218258
Mbarki, S., Sytar, O., Cerda, A., Zivcak, M., Rastogi, A., He, X., et al. (2018). Strategies to mitigate the salt stress effects on photosynthetic apparatus and productivity of crop plants. Salin. Responses Toler. in Plants 1, 85–136.
Mehboob, N., Minhas, W. A., Nawaz, A., Shahzad, M., Ahmad, F., and Hussain, M. (2018). Surface drying after seed priming improves the stand establishment and productivity of maize than seed re-drying. Int. J. Agric. Biol. 20, 1283–1288.
Mondo, V. H. V., Nascente, A. S., Neves, P., Taillebois, J. E., and Oliveira, F. H. S. (2016). “Seed hydropriming in upland rice improves germination and seed vigor and has no effects on crop cycle and grain yield,” in Embrapa Arroz e Feijão-Artigo Em Periódico Indexado (ALICE).
Moreno, C., Seal, C. E., and Papenbrock, J. (2018). Seed priming improves germination in saline conditions for Chenopodium quinoa and Amaranthus caudatus. J. Agron. Crop Sci. 204 (1), 40–48. doi:10.1111/jac.12242
Munns, R. (2005). Genes and salt tolerance: bringing them together. New Phytol. 167 (3), 645–663. doi:10.1111/j.1469-8137.2005.01487.x
Munns, R., and Gilliham, M. (2015). Salinity tolerance of crops–what is the cost? New Phytol. 208 (3), 668–673. doi:10.1111/nph.13519
Munns, R. (1993). Physiological processes limiting plant growth in saline soils: some dogmas and hypotheses. Plant Cell Environ. 16 (1), 15–24. doi:10.1111/j.1365-3040.1993.tb00840.x
Munns, R., and Tester, M. (2008). Mechanisms of salinity tolerance. Annu. Rev. Plant Biol. 59, 651–681. doi:10.1146/annurev.arplant.59.032607.092911
Nawaz, J., Hussain, M., Jabbar, A., Nadeem, G. A., Sajid, M., Subtain, M., et al. (2013). Seed priming A technique. Int. J. Agric. Crop Sci. 6 (20), 1373–1381.
Panuccio, M. R., Chaabani, S., Roula, R., and Muscolo, A. (2018). Bio-priming mitigates detrimental effects of salinity on maize improving antioxidant defense and preserving photosynthetic efficiency. Plant Physiology Biochem. 132, 465–474. doi:10.1016/j.plaphy.2018.09.033
Paparella, S., Araújo, S. S., Rossi, G., Wijayasinghe, M., Carbonera, D., and Balestrazzi, A. (2015). Seed priming: state of the art and new perspectives. Plant Cell Rep. 34 (8), 1281–1293. doi:10.1007/s00299-015-1784-y
Parihar, P., Singh, S., Singh, R., Singh, V. P., and Prasad, S. M. (2015). Effect of salinity stress on plants and its tolerance strategies: a review. Environ. Sci. Pollut. Res. 22 (6), 4056–4075. doi:10.1007/s11356-014-3739-1
Patel, R., Pandya, K., Jasari, R. T., and Brahmbhatt, N. (2017). Effect of hydropriming and biopriming on seed germination of Brinjal and Tomato seed. Res. J. Agric. For. Sci. 5 (6), 1–4.
Paul, S., and Roychoudhury, A. (2016). Seed priming with spermine ameliorates salinity stress in the germinated seedlings of two rice cultivars differing in their level of salt tolerance. Trop. Plant Res. 3 (3), 616–633. doi:10.22271/tpr.2016.v3.i3.082
Paul, S., and Roychoudhury, A. (2017). Seed priming with spermine and spermidine regulates the expression of diverse groups of abiotic stress-responsive genes during salinity stress in the seedlings of indica rice varieties. Plant gene. 11, 124–132. doi:10.1016/j.plgene.2017.04.004
Pawar, V. A., and Laware, S. L. (2018). Seed priming a critical review. Int. J. Sci. Res. Biol. Sci. 5, 94–101. doi:10.26438/ijsrbs/v5i5.94101
Qadir, M., Quillérou, E., Nangia, V., Murtaza, G., Singh, M., Thomas, R. J., et al. (2014). Economics of salt‐induced land degradation and restoration. Nat. Resour. Forum 38 (4), 282–295. doi:10.1111/1477-8947.12054
Qin, Y., Xi, B., Sun, X., Zhang, H., Xu, C., and Wu, B. (2022). Methane emission reduction and biological characteristics of landfill cover soil amended With hydrophobic biochar. Front. Bioeng. Biotechnol. 10, 905466. doi:10.3389/fbioe.2022.905466
Rajjou, L., Duval, M., Gallardo, K., Catusse, J., Bally, J., Job, C., et al. (2012). Seed germination and vigor. Annu. Rev. Plant Biol. 63, 507–533. doi:10.1146/annurev-arplant-042811-105550
Rao, M. J., Hussain, S., Anjum, M. A., Saqib, M., Ahmad, R., Khalid, M. F., et al. (2019). “Effect of seed priming on seed dormancy and vigor,” in Priming and pretreatment of seeds and seedlings (Springer), 135–145.
Rehman, A., Radulescu, M., Ma, H., Dagar, V., Hussain, I., and Khan, M. K. (2021). The impact of globalization, energy use, and trade on ecological footprint in Pakistan: does environmental sustainability exist?. Energies 14 (17), 5234. doi:10.3390/en14175234
Rhaman, M. S., Imran, S., Rauf, F., Khatun, M., Baskin, C. C., Murata, Y., et al. (2021). Seed priming with phytohormones: An effective approach for the mitigation of abiotic stress. Plants 10 (1), 37. doi:10.3390/plants10010037
Ruttanaruangboworn, A., Chanprasert, W., Tobunluepop, P., and Onwimol, D. (2017). Effect of seed priming with different concentrations of potassium nitrate on the pattern of seed imbibition and germination of rice (Oryza sativa L). J. Integr. Agric. 16 (3), 605–613. doi:10.1016/S2095-3119(16)61441-7
Saddiq, M. S., Iqbal, S., Afzal, I., Ibrahim, A. M. H., Bakhtavar, M. A., Hafeez, M. B., et al. (2019). Mitigation of salinity stress in wheat (Triticum aestivum L.) seedlings through physiological seed enhancements. J. Plant Nutr. 42 (10), 1192–1204. doi:10.1080/01904167.2019.1609509
Sadeghi, H., and Robati, Z. (2015). Response of Cichorium intybus L. to eight seed priming methods under osmotic stress conditions. Biocatal. Agric. Biotechnol. 4 (4), 443–448. doi:10.1016/j.bcab.2015.08.003
Safdar, H., Amin, A., Shafiq, Y., Ali, A., and Yasin, R. (2019). Abbas shoukat, maqsood ul hussan, Muhammad ishtiaq sarwar. A review: Impact of salinity on plant growth. Nat. Sci. 17 (1), 34–40. doi:10.7537/marsnsj170119.06
Sahab, S., Suhani, I., Srivastava, V., Chauhan, P. S., Singh, R. P., and Prasad, V. (2020). Potential risk assessment of soil salinity to agroecosystem sustainability: Current status and management strategies. Science of The Total Environment, 144164.
Sano, N., Rajjou, L., and North, H. M. (2020). Lost in translation: Physiological roles of stored mRNAs in seed germination. Plants 9 (3), 347. doi:10.3390/plants9030347
Sedghi, M., Nemati, A., and Esmaielpour, B. (2010). Effect of seed priming on germination and seedling growth of two medicinal plants under salinity. Emir. J. Food Agric. 22 (2), 130–139. doi:10.9755/ejfa.v22i2.4900
Sen, A., and Puthur, J. T. (2020). “Seed priming-induced physiochemical and molecular events in plants coupled to abiotic stress tolerance: An overview,” in Priming-mediated stress and cross-stress tolerance in crop plants (Elsevier). doi:10.1016/B978-0-12-817892-8.00018-0
Shabala, S., and Munns, R. (2012). Salinity stress: Physiological constraints and adaptive mechanisms. Plant Stress Physiol., 59–93. doi:10.1079/9781845939953.0059
Shalaby, O. A. E.-S., and El-Messairy, M. M. (2018). Humic acid and boron treatment to mitigate salt stress on the melon plant. Acta Agric. Slov. 111 (2), 349–356. doi:10.14720/aas.2018.111.2.10
Sher, A., Sarwar, T., Nawaz, A., Ijaz, M., Sattar, A., and Ahmad, S. (2019). “Methods of seed priming,” in Priming and pretreatment of seeds and seedlings (Springer), 1–10.
Sheteiwy, M. S., Shao, H., Qi, W., Daly, P., Sharma, A., Shaghaleh, H., et al. (2021). Seed priming and foliar application with jasmonic acid enhance salinity stress tolerance of soybean ( Glycine max L. ) seedlings. J. Sci. Food Agric. 101 (5), 2027–2041. doi:10.1002/jsfa.10822
Singh, D., and Kumar, A. (2021). Multivariate screening approach indicated adaptive tolerance to salt stress in the seedlings of an agroforestry tree, Eucalyptus tereticornis Sm. Plant Cell Tissue Organ Cult. 145 (3), 545–560. doi:10.1007/s11240-021-02025-2
Sisodia, A., Padhi, M., Pal, A. K., Barman, K., and Singh, A. K. (2018). “Seed priming on germination, growth and flowering in flowers and ornamental trees,” in Advances in seed priming (Springer), 263–288.
Srivastava, A. K., Suresh Kumar, J., and Suprasanna, P. (2021). Seed ‘primeomics’ plants memorize their germination under stress. Biol. Rev. Camb. Philos. Soc. 96 (5), 1723–1743. doi:10.1111/brv.12722
Sucre, B., and Suárez, N. (2011). Effect of salinity and PEG-induced water stress on water status, gas exchange, solute accumulation, and leaf growth in Ipomoea pes-caprae. Environ. Exp. Bot. 70 (2–3), 192–203. doi:10.1016/j.envexpbot.2010.09.004
Tabassum, T., Farooq, M., Ahmad, R., Zohaib, A., Wahid, A., and Shahid, M. (2018). Terminal drought and seed priming improves drought tolerance in wheat. Physiol. Mol. Biol. Plants 24 (5), 845–856. doi:10.1007/s12298-018-0547-y
Tan, Z., Zhu, H., He, X., Xi, B., Tian, Y., Sun, X., et al. (2022). Effect of ventilation quantity on electron transfer capacity and spectral characteristics of humic substances during sludge composting. Environ. Sci. Pollut. Res. Int. Epub ahead of print. PMID: 35589896. doi:10.1007/s11356-022-20808-8
Tania, S. S., Rhaman, M. S., and Hossain, M. M. (2020). Hydro-priming and halo-priming improve seed germination, yield and yield contributing characters of okra (Abelmoschus esculentus L). Trop. Plant Res. 7, 86–93. doi:10.22271/tpr.2020.v7.i1.012
Tanou, G., Fotopoulos, V., and Molassiotis, A. (2012). Priming against environmental challenges and proteomics in plants: update and agricultural perspectives. Front. Plant Sci. 3, 216. doi:10.3389/fpls.2012.00216
Tsegay, B. A., and Andargie, M. (2018). Seed priming with gibberellic acid (ga 3) alleviates salinity induced inhibition of germination and seedling growth of Zea mays L., pisum sativum Var. abyssinicum A. Braun and lathyrus sativus L. J. Crop Sci. Biotechnol. 21 (3), 261–267. doi:10.1007/s12892-018-0043-0
Varier, A., Vari, A. K., and Dadlani, M. (2010). The subcellular basis of seed priming. Curr. Sci. 99 (4), 450–456.
Wang, Q., Sha, H., Cao, S., Zhao, B., Wang, G., and Zheng, P. (2022). Tourmaline enhanced methane yield via regulating microbial metabolic balance during anaerobic co-digestion of corn stover and cow manure. Bioresour. Technol. 359, 127470. doi:10.1016/j.biortech.2022.127470
Wang, X. shan, and Han, J. guo (2009). Changes of proline content, activity, and active isoforms of antioxidative enzymes in two alfalfa cultivars under salt stress. Agric. Sci. in China 8 (4), 431–440. doi:10.1016/S1671-2927(08)60229-1
Wojtyla, Ł., Lechowska, K., Kubala, S., and Garnczarska, M. (2016). Molecular processes induced in primed seeds—increasing the potential to stabilize crop yields under drought conditions. J. Plant Physiology 203, 116–126. doi:10.1016/j.jplph.2016.04.008
Xu, J., Lan, W., Ren, C., Zhou, X., Wang, S., and Yuan, J. (2021). Modeling of coupled transfer of water, heat and solute in saline loess considering sodium sulfate crystallization. Cold regions Sci. Technol. 189, 103335. doi:10.1016/j.coldregions.2021.103335
Yang, Y., Dou, Y., Wang, B., Wang, Y., Liang, C., An, S., et al. (2022). Increasing contribution of microbial residues to soil organic carbon in grassland restoration chronosequence. Soil Biol. Biochem. 170, 108688. doi:10.1016/j.soilbio.2022.108688
Younesi, O., and Moradi, A. (2014). Effect of different priming methods on germination and seedling establishment of two medicinal plants under salt stress conditions.
Yücel, N. C., and Heybet, E. H. (2016). Salicylic acid and calcium treatments improves wheat vigor, lipids and phenolics under high salinity. Acta Chim. Slov. 63 (4), 738–746. doi:10.17344/acsi.2016.2449
Zhang, K., Wang, S., Bao, H., and Zhao, X. (2019). Characteristics and influencing factors of rainfall-induced landslide and debris flow hazards in Shaanxi Province, China. Nat. Hazards Earth Syst. Sci. 19 (1), 93–105. doi:10.5194/nhess-19-93-2019
Zhu, Z., Chen, Y., Shi, G., and Zhang, X. (2017). Selenium delays tomato fruit ripening by inhibiting ethylene biosynthesis and enhancing the antioxidant defense system. Food Chem. 219, 179–184. doi:10.1016/j.foodchem.2016.09.138
Keywords: soil salinity, seed priming, abiotic stress, antioxidant enzymes, ROS
Citation: Khan MO, Irfan M, Muhammad A, Ullah I, Nawaz S, Khalil MK and Ahmad M (2022) A practical and economical strategy to mitigate salinity stress through seed priming. Front. Environ. Sci. 10:991977. doi: 10.3389/fenvs.2022.991977
Received: 12 July 2022; Accepted: 16 August 2022;
Published: 05 October 2022.
Edited by:
Cem Işık, Anadolu University, TurkeyReviewed by:
Sumit Dagar, Delhi Technological University, IndiaMuhammad Daniyal Junaid, Ömer Halisdemir University, Turkey
Copyright © 2022 Khan, Irfan, Muhammad, Ullah, Nawaz, Khalil and Ahmad. This is an open-access article distributed under the terms of the Creative Commons Attribution License (CC BY). The use, distribution or reproduction in other forums is permitted, provided the original author(s) and the copyright owner(s) are credited and that the original publication in this journal is cited, in accordance with accepted academic practice. No use, distribution or reproduction is permitted which does not comply with these terms.
*Correspondence: Muhammad Owais Khan, owaisk@aup.edu.pk