- 1Faculty of Environment and Natural Resources, Chair of Wildlife Ecology and Management, University of Freiburg, Freiburg, Germany
- 2Trafikverket, Malmö, Sweden
- 3Department of Animal Ecology, University of Potsdam, Potsdam, Germany
- 4Department of Biology, Lund University, Lund, Sweden
- 5Department of Ecology, Grimsö Wildlife Research Station, Swedish University of Agricultural Sciences, Riddarhyttan, Sweden
- 6EnviroPlanning AB, Gothenburg, Sweden
Wildlife-vehicle collisions (WVC) cost millions of euros each year in loss of life and damages. Wildlife crossing structures and fencing are commonly implemented mitigation strategies to reduce WVC and increase landscape connectivity for wildlife. Typically, crossing structures are over- or under-pass structures that allow animals to safely cross the road, while separating them from the road and traffic. An alternative strategy could be an at-grade fauna passage coupled with a Roadside Animal Detection System (RADS). At-grade fauna passages are designated locations where a gap in fencing allows animals to cross over the road, while RADS alert drivers of animals at the upcoming passage, so that they can adjust their driving behaviour accordingly and avoid collisions. In this pilot study, we investigated the use of one at-grade fauna passage by roe deer (Capreolus capreolus), red deer (Cervus elaphus) and wild boar (Sus scrofa) in southern Sweden, and compared changes in the number of WVCs before, during and after the construction of the passage. We collected a total of 326 wildlife crossings from 722 individuals over 1 year of monitoring (24 January 2020–24 January 2021). We found that crossing events tended to be dominated by the time animals spent in the roadside verge from which they approached the at-grade fauna passage, particularly for roe deer that spent a lot of time browsing in the roadside verge during dusk. We also found that animals spent longer in the passage if vehicles were present. In our 1 year of surveys, we only recorded three accidents, and when comparing the annual collision statistics before, during, and after construction of the at-grade fauna passage, we demonstrated an overall reduction in collisions by 66%. While our pilot only evaluates a single site, it does provide promising preliminary results that suggest that at-grade fauna passages can help in efforts to reduce collisions, while maintaining connectivity over medium-sized roads for large ungulates.
1 Introduction
Wildlife vehicle collisions (WVCs) are one of the most prominent, and detrimental impacts of roads on wildlife (Bruinderink and Hazebroek, 1996; van der Ree et al., 2011; Seiler and Bhardwaj, 2020). Wildlife crossing structures (i.e., over- and under-passes) and fencing are commonly implemented mitigation strategies to reduce WVCs while providing safe crossing opportunities for wildlife (Jaeger and Fahrig, 2004; Smith et al., 2015; van der Ree et al., 2015). Due to costs and technical restrictions, installation of fencing and crossing structures tends to be prioritized where they would benefit traffic-safety the most, i.e., along high traffic, high speed, primary roads (Huijser and McGowen, 2003; van der Ree et al., 2015). This often leaves secondary roads unmitigated, despite the fact that WVCs occur most frequently on roads with mid-range traffic volume and posted speed limits (Seiler, 2005). Thus, it is necessary to develop and implement mitigation strategies that reduce the impacts of roads on wildlife but are less costly than traditional crossing, particularly for implementation on secondary road networks.
At-grade fauna passages, paired with roadside animal detection systems (RADS), may be an alternative solution to traditional fauna passages (Huijser et al., 2015; Gagnon et al., 2019). At-grade fauna passages with RADS consist of three components: 1) The passage, which is an opening along a fenced roadway. The fencing is used to guide animals to the passage, and across the road at a specific, designated location while reducing access of the individuals to the rest of the road (up to the fencing limits); 2) A detection system, which detects animals as they approach the fence opening; and 3) An alerting system, which alerts drivers of the presence of animals, usually through variable traffic signs. While static signs become less effective over time (Bond and Jones, 2013), variable and temporary sign have been shown to decrease WVC occurrences by at least 50% along non-fenced roads (Sullivan et al., 2004; Huijser et al., 2009). When paired with an at-grade fauna passage, signs can reduce WVCs by up to 80% (Kistler, 1998; Romer et al., 2003). The main difference between at-grade fauna passages and traditional crossing structures is that at-grade fauna passages do not physically separate where animal cross from the flow of traffic. Thus, the success of at-grade fauna passage depends on how the focal species approach and cross the road.
Recently, it has become increasingly popular to investigate the response of wildlife to roads and oncoming vehicles (e.g., Jacobson et al., 2016; Jasińska et al., 2019; Bhardwaj et al., 2022; Breiger et al., 2022; Lunn et al., 2022). While a lot of literature supports the connection between WVCs and road characteristics (e.g., Clevenger et al., 2003; Jaarsma et al., 2007; Barrientos et al., 2009; Chyn et al., 2021), or driver behaviour (e.g., Litvaitis and Tash, 2008; Collinson et al., 2019), the way wildlife respond to traffic and cross roads has been identified as another key component of WVCs (Lima et al., 2015; Jacobson et al., 2016; Breiger et al., 2022). Wildlife that do not detect or avoid vehicles (i.e., ‘nonresponders’) and those that pause or slow down when a vehicle is approaching (‘pausers’), tend to spend the most time on roads, and thus have the greatest potential to be involved in WVCs (Jacobson et al., 2016). Investigating how much time the animals spend in at-grade fauna passages, either in the roadside verge or on the road, can help improve the design of the structure and warning system, and provide insights into how effective they may be to reducing WVCs.
Ungulates are the most commonly studied taxa in regards to WVCs (Bhardwaj et al., in press). This is largely due to the fact that ungulate-vehicle collisions often entail high risks to human safety, and the damages are often reported to police and insurance companies, thus the data is abundantly available (Conover et al., 1995; Langbein et al., 2011; Hill et al., 2021; Bhardwaj et al., in press). Approximate 60% of the total number of police reported traffic accidents in Sweden involved ungulates (Seiler and Folkesson, 2006). Between 2012 and 2019, roe deer (Capreolus capreolus) was the most common species involved in WVCs, with an average of 40 940 (sd = 5689) police reported accidents per year, followed by moose (Alces alces) with in average 5 640 (sd = 407) WVC/year and wild boar (Sus scrofa) with 5 150 (sd = 1534) WVC/year (Nationella viltolycksrådet, 2022). Red deer (Cervus elaphus) are less abundant throughout Sweden, however, they are still involved in an average 374 (sd = 66) WVC/year, particularly in the southern parts of Sweden (Nationella viltolycksrådet, 2022). At-grade fauna passages paired with RADS have successfully reduced the rate of ungulate-vehicle collisions in North America (Gordon et al., 2004; Huijser et al., 2003; 2015; Gagnon et al., 2019), however they are not as widely implemented in Sweden, and their effectiveness, particularly along secondary roads, remains to be investigated.
In this study, we evaluated the crossing behaviour of roe deer, red deer, and wild boar at one at-grade fauna passage paired with RADS. Using video footage, we explored how groups of each species approached and crossed the road. Since we were interested in the interplay between how wildlife cross roads, or respond to traffic, and the risk of potential collision, we explored: the success of a group to cross the road, the time it took for them to cross, and the average number of WVC before, during and after construction of the at-grade fauna passage. We predicted that: 1) Species that travel in larger groups would have lower crossing success, as they would take longer to cross and groups may get separated; 2) The presence of vehicles would reduce crossing success, and increase the time animals spent in the at-grade fauna passage; 3) Daily and seasonal use of the at-grade fauna passage would correspond to the ecology of these species, in that they would be used most during dawn and dusk, and through spring and summer and autumn when these species are most active; and 4) WVCs would be lower after the at-grade fauna passage was constructed. The results of this pilot can be used to evaluate whether wild ungulates use at-grade fauna passages and to lay the foundation for a larger study at multiple sites.
2 Materials and methods
2.1 Study site
We conducted this study at one at-grade fauna passage along Road 108 in Skåne County, south-east of Malmö in southern Sweden (Figure 1). This region is predominated by agricultural fields, scattered forests, and large urban/suburban developments. The region supports large densities of ungulates, namely roe deer, red deer, wild boar, and fallow deer (Dama dama). The density of roads, in combination with the scattered patches of natural habitat have resulted in numerous incidents of WVCs, and this region is among the densest for WVCs in Sweden (Nordström, 2014).
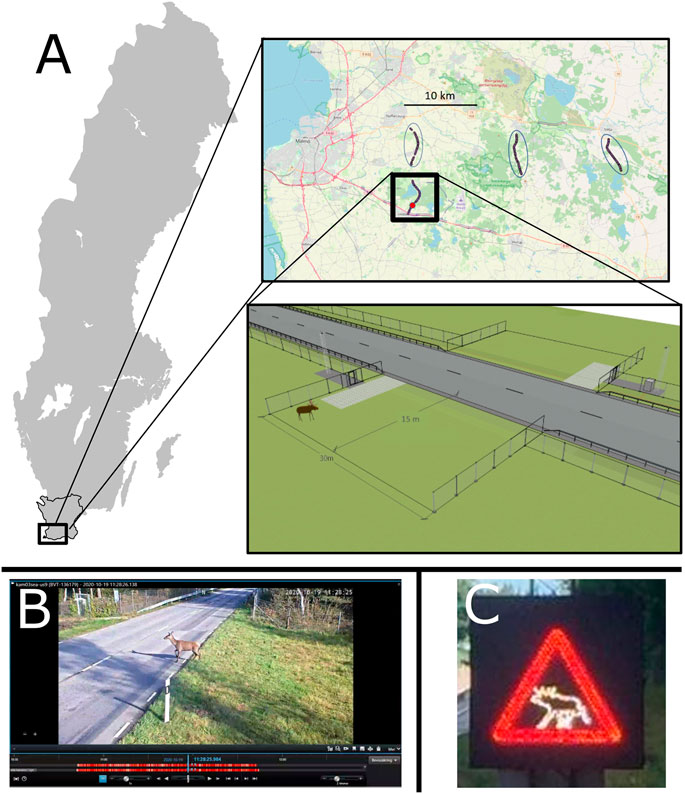
FIGURE 1. (A) Top: Location of the at-grade fauna passage in southern Sweden, in Skåne County. Road 108 is shown then black box, and the exact location of the fauna passage is shown by a red dot. The three unmitigated reference road sections are shown in blue ovals (Basemap: OpenStreetMaps). The schematic below the localized map shows a technical drawing of the at-grade fauna passage, with a 30 m wide opening in the fence with the roadside verge detection zones on each side of the road, where the animals are detected by the video surveillance system before crossing the road. (B) Bottom left: An example of a video as viewed from the Milestone XProtect video management software. (C) Bottom right: An example of warning sign A19-1, displayed on the variable message sign to warn drivers of an animal within the at-grade fauna passage.
Road 108 is 7.5 m wide, runs in a north-south direction and has one lane for traffic in each direction. The posted speed limit is 80 km/h, and the annual average daily traffic volume (AADT) is 6000 vehicles/day, of which approximately 600 vehicles/day are transport trucks (Nordström, 2014). In 2019, a 5.2 km section of Road 108 was upgraded, which included introducing various strategies to reduce the occurrence of WVCs along this segment of the road. These strategies included wildlife fencing, jump-outs and cattle guards at private roads that intersect Road 108, in addition to the at-grade fauna passage and RADS structure which is the focus of this study. Prior to the upgrade, there was a poorly-maintained private fence on one-side of the road, and, on average, 30 WVC/year involving wild ungulates (Nordström, 2014). Now, Road 108 is fenced on both sides along the entire length of the 5.2 km upgrade, except for at the at-grade fauna passage, where there is a 30 m wide opening in the fence (Figure 1). At the opening, fencing extends 15 m perpendicularly from the road into the roadside verge, to create two well-defined approaching areas where animals are detected as they enter the fauna passage (further referred to as the “roadside verge”). On Road 108, approximately 100–130 m before the at-grade fauna passage, there is a 1 × 1 m variable message sign, mounted on a 2 m high pole, directed towards oncoming traffic to warn drivers of wildlife within the at-grade fauna passage (Figure 1).
The at-grade fauna passage and RADS is monitored and activated using the Swedish Transport Administration’s technical platform (Trafikverket, 2021), which includes thermal imaging cameras, control systems and external communication for function control. Three heat cameras (Axis, Q1941-E), two radars (Axis, D2050-VE) and two Infrared-sensitive Pan-Tilt-Zoom (PTZ) cameras with external Infrared-lamps (Axis, Q6155-E PTZ R2) are mounted on two 5 m high masts, which survey the road and both roadside verges on each side of the at-grade fauna passage. One mast is located in the north-western corner of the at-grade fauna passage, and consists of one heat camera, one radar, and one PTZ camera with an Infrared-lamp. The second mast is in the south-eastern corner of the at-grade fauna passage, and has two heat cameras, one radar and one PTZ camera with an Infrared-lamp. Video footage from the at-grade fauna passage is stored on a technical platform that uses Milestone with XProtect video management software (Milestone Systems A/S; https://www.milestonesys.com/video-technology/platform/xprotect/) (Figure 1). The pilot site cost approximately 300 000 Euro and 6 months to plan and build.
The cameras are continuously recording the site. The heat and radar cameras detect wildlife on the roadside verges, and the PTZ camera can rotate, zoom in and follow animal movements across the whole at-grade fauna passage, from one roadside verge across the road and into the second roadside verge. At each animal detection, the variable message signs are automatically activated, and a conventional “warning of moose” graphic sign (A19-1, Transportstyrelsen, 2007) is displayed and remains active until 60 s after the last animal is detected at the at-grade fauna passage (Figure 1). The software stores video sequences of animals that use the at-grade fauna passage.
2.2 Data collection and analysis
We analysed every animal detection captured between 24 January 2020 and 24 January 2021. The camera angles and technical setup allowed us to follow each individual as it approached, crossed and exited the at-grade fauna passage. Since the videos were continuous, with no time restrictions, we could observe wildlife for the full duration that they were within the detection area of the cameras. We defined videos as “events” that could have included one or more individuals of conspecifics within the at-grade fauna passage. Events finished once all of the detected individuals were no longer within the at-grade fauna passage.
For each event, we summarized the behaviour of individuals/groups into crossing success and time spent in the passage in total, in the roadside verge or on the road. We assumed that if individuals were detected in the roadside verge (i.e., within the detection area of the milestone system), they had the intention to cross. Thus, if individuals only spent time in the roadside verge and did not cross, this was recorded as an unsuccessful crossing. A crossing was only considered successful if all individuals of the group crossed the road (i.e., without turning back, or being involved in a collision).
In events with more than one individual detected (i.e., a group), we summarized the behaviour of the group rather than treating each individual independently. Thus, the duration of time spent in the passage or crossing the road spans from the time the first individual is detected until the last individual completes the behaviour (e.g., from the time that the first individual steps on the road until the last individual steps off the road). The at-grade fauna passage was used by many species, for example, fox (Vulpes vulpes), and fallow deer. However, our analysis focuses on the most frequently observed species: roe deer, red deer, and wild boar. Finally, we also documented how many vehicles passed the at-grade fauna passage during each event.
Crossing activity and the risk of WVC could change depending on the time of day, and the season. We recorded the time of day that the events occurred in, and categorized this into dawn (morning twilight to sunrise), day (sunrise to sunset), dusk (sunset to evening twilight) and night (evening twilight to morning twilight). The hours of the day and duration of each time period changed throughout the year with changes in sunrise and sunset time. To account for changes in animal behaviour throughout the year, we also recorded four meteorological seasons: autumn (September–November), winter (December–February), spring (March–May) and summer (June–August).
We explored crossing success as a binomial regression, in relation to the presence of a vehicle during the event, the group size, and the time of day. To explore the time ungulates spent at the at-grade fauna passage we explored three separate measurements of time, recorded in seconds: total time of an event, the time the individual/group spent on the roadside verge, and the time the individual/group spent on the roads. For each time measure, we used gamma regressions (with a log link) to explore the influence of vehicle presence, crossing success, group size, time of day and season on the time spent. In each model, the reference time of day was dawn, and the reference season was autumn. We explored these relationships separately for roe deer, red deer and wild boar. All analyses were performed using R (v.3.5.3, R Core Team, 2019), using the ‘glm’ function, in the ‘stats’ package.
To determine if mitigation along the focal segment of Road 108 reduced the number of WVC, we undertook posthoc analyses, comparing the number of WVC at the focal road section, to three unfenced roads within the same road network (Figure 1). The three reference sections were similar to our focal road segment: 5.2 km in length, had similar traffic volume and posted speed limits. We explored WVC data from 1 January 2015 to 30 December 2021, covering 1307 days before, 424 days during, and 822 days after the focal road segment was upgraded and the at-grade fauna passage was constructed. At the focal road segment, we collected WVC data for the entire 5.2 km upgraded extent, as well as 300 m to the north of the fence-end. The 100 m furthest from the fence-end had a lower posted speed limit of 60 km/h as the road approached a roundabout. In the southern part, Road 108 intersected Highway 65 (Figure 1), and so the fencing was connected to the existing highway fencing.
Since roe deer, red deer and wild boar populations in this region are long-established, stable and controlled through hunting, we assumed that populations densities and spatial extent are relatively similar across this time period, and thus an equal opportunity for collisions exists (with the exception of natural fluctuations). Further, since this is a long human-populated and developed part of Sweden, we assumed that the landscape surrounding the roads did not change significantly enough to change the likelihood of wildlife to occur around the roads, over the duration that we recorded WVCs.
3 Results
Through the Milestone System at the at-grade fauna passage, we collected a total of 332 events with 737 individual animals over the one-year sampling period. We analysed 326 of these events, of which 91 included roe deer (nindividuals = 186), 99 included red deer (nindividuals = 105) and 136 included wild boar (nindividuals = 431). A total of 608 individual animals crossed through the at-grade fauna passage in 248 of the 326 events (Table 1). Roe deer were only active at the at-grade fauna passage from April to November. Red deer activity was highest from April to December, and wild boar were active throughout the year, but less so from January to May. On average, roe deer tend to spend the most time in the at-grade fauna passage (7 min, range: 0–55 min) and wild boar tended to spend the least amount of time (2.5 min, range 0–62 min). Red deer spent 5 min on average (range: 0–33 min).
Generally, all individuals in a group responded the same way; either the whole group crossed or the whole group were repelled. In 62% of roe deer events (56/91), 77% of red deer events (76/99), and 84% of wild boar events (114/136), the whole group crossed the road. In only one roe deer event, five red deer events and three wild boar events did the groups get split up. In two of the three events where the whole group of wild boars did not cross, the individual that did not cross were involved in an accident. In the remaining events (34 roe deer events, 18 red deer events and 19 wild boar events), no members of the group crossed the road.
3.1 Crossing success and time spent at the at-grade fauna passage
Roe deer events included either one or two individuals (Table 1). Roe deer crossing success was lower in events with two individuals than in events with one individual. However, group size did not have any influence on the time roe deer spent at the at-grade fauna passage, in the roadside verge or on the road (Figure 2; Table 2). Roe deer events tended to be longest during dawn, compared to other times of the day (dawn: 555 ± 699 s [34, 2113] (mean ± SD [range]); day: 164 ± 477 s [14, 3255]; dusk: 162 ± 262 s [19, 908]; night: 1244 ± 1224 s [18, 3322]); Figure 2 and Table 2). The time roe deer spent at the at-grade fauna passage tended to be dominated by time spent in the roadside verge, and the longer roe deer spent at the at-grade fauna passage, the less likely they were to cross the road (roadside verge: 347 ± 769 s [0, 3322]; road: 18 ± 58 s [0, 482]; Figures 2, 3 and Table 2). Roe deer spent most of the time at the at-grade fauna passage during autumn, compared to spring and summer (autumn: 1837 ± 1041 s [225, 3322]; spring: 193 ± 563 s [14, 3255]; summer: 249 ± 458 s [14, 2113]. Roe deer were not present at the fauna passage during winter; Figure 2 and Table 2). Again, this mainly reflects the time roe deer spent in the roadside verge. However, this is also true, while more uncertain, of the time roe deer spend on the road (Figures 2, 3; Table 2). When vehicles were present, roe deer spent more time in the roadside verge but had lower crossing success, regardless of time of day (Figures 2, 3; Table 2).
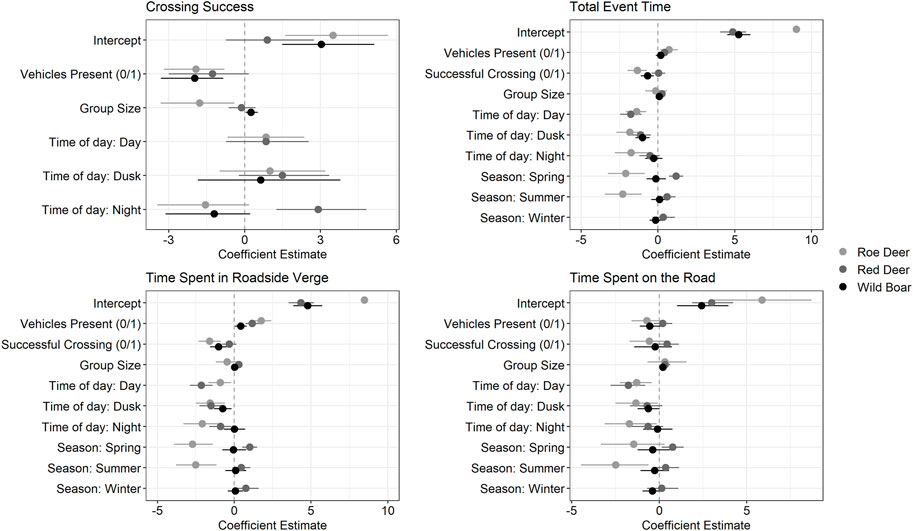
FIGURE 2. Coefficient plot of the four generalized linear models used in this study, to investigate crossing success, the time for a total event, the time animals spent in the roadside verge, and the time spent on the road. The points represent the beta estimates, and the error bars show the 95% confidence intervals. Those estimates that overlap 0 are deemed non-significant. Roe deer models are presented in light grey, red deer in dark grey, and wild boar in black. The reference categories were: Time of day: Dawn, and Season: autumn. Full model outputs are available in Table 2.
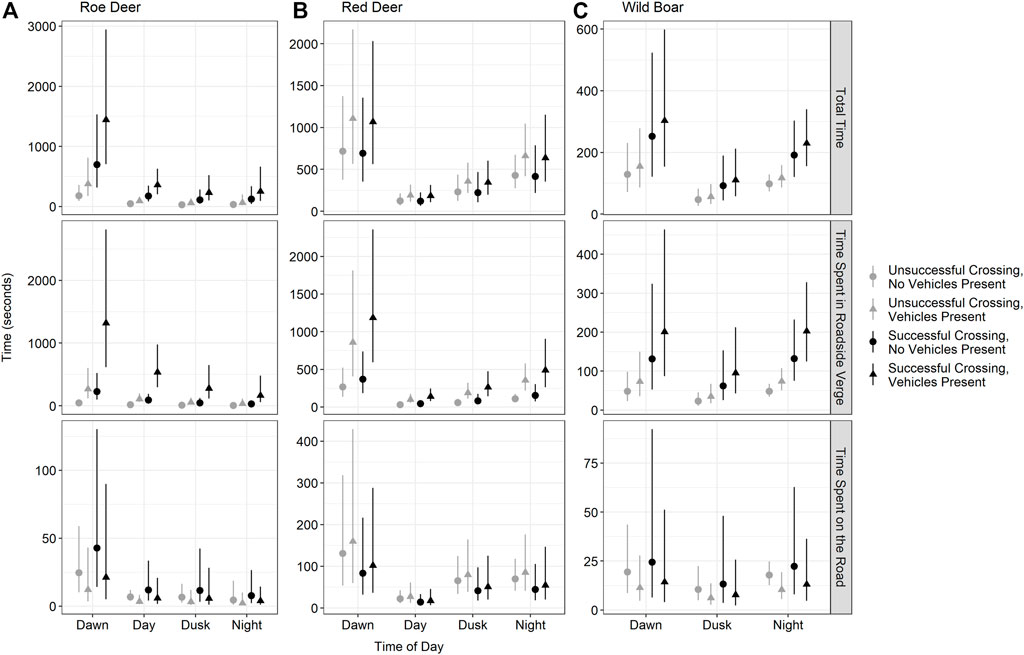
FIGURE 3. Predicted amount of time, in seconds, roe deer [left-(A)], red deer [middle-(B)] and wild boar [right-(C)] spent in the at-grade fauna passage overall (total time, top), in the roadside verge (middle) and on the road (bottom). Grey points show events with unsuccessful crossings, while black points show events with successful crossing. Circles indicate events while no vehicles are present, and triangles are events where vehicles are present. Error bars are 95% confidence intervals. Note: the y-axes differ to facilitate visualization.
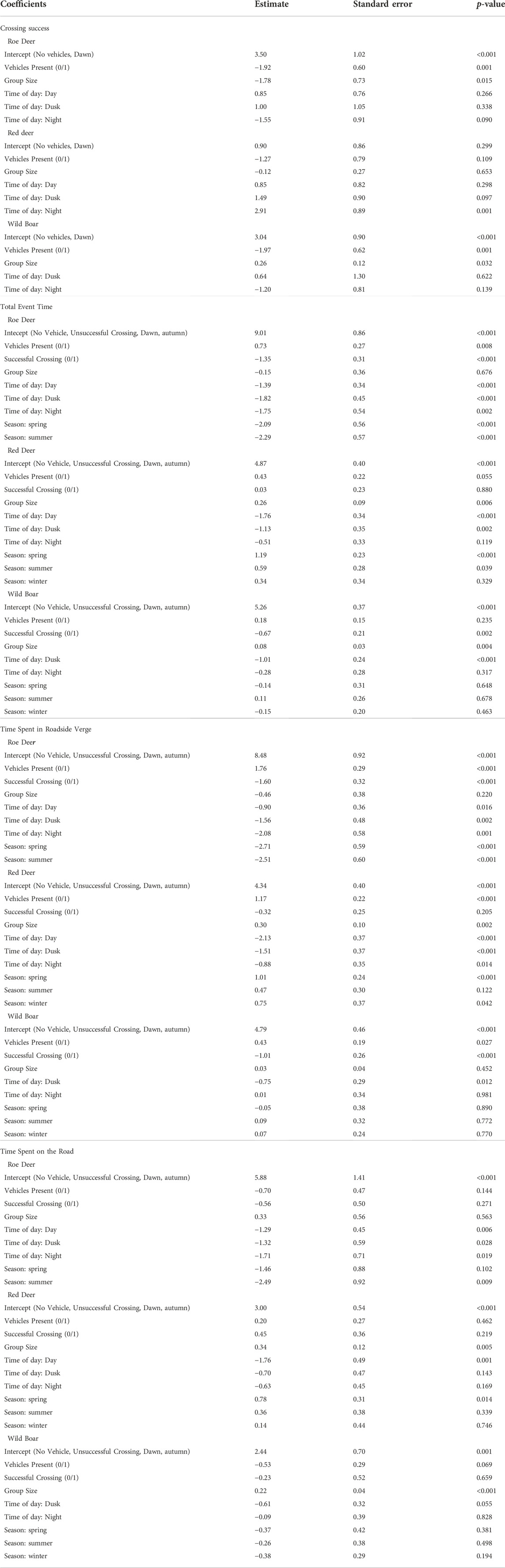
TABLE 2. Model outputs for roe deer, red deer and wild boar. Crossing success was estimated using a binomial regression, in relation to the presence of a vehicle during the event, the group size, and the time of day. A crossing was only considered successful if all individuals of the group crossed the road (i.e., without turning back, or being involved in a collision). Total time of an event, the time the individual/group spent on the roadside verge, and the time the individual/group spent on the roads were estimated using gamma regressions (with a log link) to explore the influence of vehicle presence, crossing success, group size, time of day and season on the time spent. In each model, the reference time of day was dawn, and the reference season was autumn. Roe deer, red deer and wild boar were modelled separately.
Red deer had higher crossing success at night, compared to other times of the day, (Figure 2; Table 2). Like roe deer, red deer events were dominated by time spent in the roadside verge, particularly during dawn (roadside verge (average throughout day): 173 ± 272 s [12, 1453]; road (average throughout day): 43 ± 67 s [0, 492]; dawn (total event time): 607 ± 605 s [37, 1966]; day (total): 110 ± 112 s [20, 404]; dusk (total): 272 ± 244 s [17, 978]; night (total): 311 ± 357 s [22, 1439]; Figures 2, 3 and Table 2). During night and dawn, red deer spent less time on the road than during the day, which is also reflected in the measure of total time spent in the at-grade fauna passage (Figure 2; Table 2). Red deer events were longer during spring compared to other times of the year (autumn: 151 ± 162 s [22, 831]; spring: 449 ± 493 s [20, 1966]; summer: 225 ± 228 s [17, 712]; winter: 306 ± 361 s [68, 1198] Figure 2 and Table 2). While group size did not influence the crossing success of red deer, as groups got bigger, events got longer (Figure 2; Table 2). The time red deer took to cross the at-grade fauna passage did not differ significantly between successful and unsuccessful crossings. However, the presence of vehicles did increase the time red deer spent in the roadside verge and in the at-grade fauna passage overall (Figures 2, 3; Table 2).
While wild boar spent, on average, less time at the at-grade fauna passage that roe deer and red deer, their behaviour was often similar. Wild boar spent the majority of the total event time in the roadside verge, and at events where they spent more time in the roadside verge, they were less successful at crossing the road (roadside verge: 76 ± 94 s [6, 588]; road: 14 ± 27 s [0, 259]; Figures 2, 3 and Table 2). Vehicle presence was also negatively associated with wild boar crossing success, but positively associated with the time they spent in the roadside verge (Figures 2, 3; Table 2). Crossing success, and total time spent at the at-grade fauna passage increased with increasing group size (Figure 2; Table 2). There were only nine events of wild boar during the day, so we incorporated these events into dawn if they were before 12:00 (n = 7) and dusk if there were after 12:00 (n = 2). Wild boar crossing success did not differ throughout the day. However, events during dusk were shorter than events during night and dawn (dawn: 162 ± 177 s [32, 810]; dusk: 65 ± 42 s [15, 226]; night: 105 ± 106 s [15, 588]; Figures 2, 3 and Table 2). Finally, there was no difference in the amount of time wild boar spent at the at-grade fauna passage throughout the year (autumn: 135 ± 115 s [15, 588]; spring: 112 ± 136 s [29, 445]; summer: 120 ± 138 s [20, 810]; winter: 100 ± 93 s [17, 481]; Figure 2 and Table 2).
3.2 Wildlife-vehicle collisions
Along the section of Road 108 where the at-grade fauna passage was constructed, there was a 66% decrease in WVCs, compared to before mitigation (from 0.078 WVC/day to 0.027 WVC/day; Figures 1, 2, 3, 4 and Table 3). Comparatively, there was relative increase in WVCs at three unmitigated but otherwise similar road segments (reference 1: 0.047 WVC/day to 0.052 WVC/day; reference 2: 0.130 WVC/day to 0.141 WVC/day; reference 3: 0.142 WVC/day to 0.139 WVC/day; Figure 4 and Table 3). During the year of data collection for this study, we captured three WVC at the at-grade fauna passage. All of the accidents involved wild boar, resulting in an individual accident rate of 0.5% of the crossing animals (Ncrossing wild boar = 608 from 248 events).
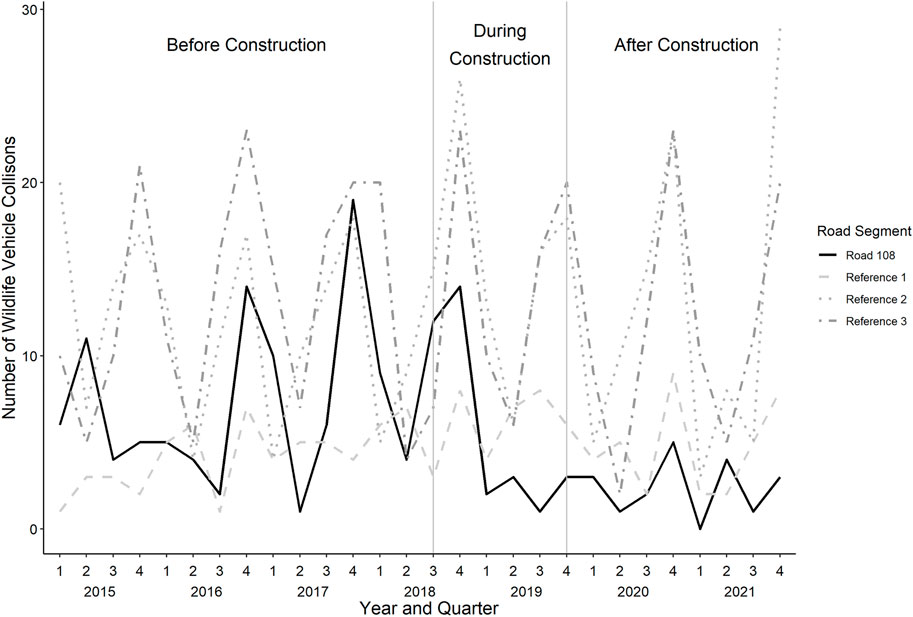
FIGURE 4. The number of wildlife vehicle collisions, summarized per quarter (i.e., Q1: January–March; Q2: April–June; Q3: July–September; Q4: October–December), from 2015–2021. The solid black line represents the road segment corresponding to the focal at-grade fauna passage. The dashed grey lines represent the three unmitigated reference sites that are similar in length, traffic volume and posted speed limit.

TABLE 3. Average number of WVC per annual quarter (i.e., Q1: January–March; Q2: April–June; Q3: July–September; Q4: October–December), before, during and after the construction of the focal at-grade fauna passage. WVC are summarized for the focal road segment, Road 108, as well as three unmitigated reference road segments that are similar in size, traffic volume and posted speed limit.
4 Discussion
4.1 Ungulate use of at-grade fauna passage with RADS
In this study, we used 1 year of high-quality, detailed video footage to investigate the use of an at-grade fauna passage by roe deer, red deer and wild boar in southern Sweden. Generally, groups of all three species responded the same way–either the whole group crossed or the whole group remained on one side of the road. We expected group size to have a strong impact on how long events at the at-grade fauna passage lasted, with the expectation that larger groups would take longer to cross the road. However, we did not see any clear influence of group size on the time to cross. Rather, contrary to our expectations, in larger groups, wild boar were more likely to cross the road than in smaller groups. Roe deer tended to have lower crossing success with increased group size, but since the maximum number of individuals recorded was two, this may just be an artefact of the sampling method. Further, the roadside verge appeared to be an attractive browsing resource for roe deer, and individuals spent a lot of time there without attempting to cross the road.
Since there were very few events where the whole group did not cross the road, we suggest that the “decision” to cross was made before individuals entered the field of view of the detection system. This is also evident by the fact that when groups crossed, they spent little time on the road. We also found that roe deer, red deer and wild boar spent longer in the roadside verge when a vehicle was present than when there were no vehicles present, which also resulted in longer events overall. However, vehicle presence did not affect the amount of time that roe deer, red deer or wild boar spent on the road. The relationship between total time spent in at the at-grade fauna passage and the number of vehicles is circular–when individuals spend up to an hour in the roadside verge, they have a greater chance of a car passing them during that time. Thus, it is not possible to disentangle if they spent more time in the roadside verge because of vehicles or if the vehicle count simply increases as time goes on. To derive these answers, it may be important to investigate the landscape-level effect that roads have on wildlife, and the decision-making processes, and behaviour of individuals as they approach or cross the road (Neumann et al., 2012; Meisingset et al., 2013; Kämmerle et al., 2017). Furthermore, understanding the composition of the landscape around the road may provide insights into how valuable the roadside verge is as a resource, and if that would motivate individuals to spend more time near a road without the intent to cross. Finally, investigation of the way wildlife approach a road could also provide insights to the larger question of landscape-connectivity. In the current set up of our data collection, we are only able to observe the response of wildlife that are already within 15 m of the road, however if the road is having a filtering effect on wildlife, and few individuals are approaching the road, that impact may occur further away from the road. Thus, observing the behaviour of wildlife further away from the road, as they approach the road, would provide further insights into the behavioural response of wildlife to roads.
We recorded more crossing events over dusk and night compared to during the day, and this may be because of a combination of species activity patterns, and reduced traffic volume/vehicle presence (Ensing et al., 2014; Ikeda et al., 2016; Gagnon et al., 2019; Knufinke et al., 2019; Mysłajek et al., 2020; Elfström, 2021; Mayer et al., 2021). Roe deer spent less time at the at-grade fauna passage during spring and summer compared to autumn. In the autumn, other foliage and food resources may be limited, and so roe deer may be spending more time browsing in the roadside verge. Red deer, on the other hand, tended to spend more time during the spring and summer than during the autumn. These results corroborate previous findings, that show activity level and frequency of animal road visits also often varies by season, with most activity during summer and autumn (Mata et al., 2009; Grace et al., 2017; Knufinke et al., 2019; Mysłajek et al., 2020; Mayer et al., 2021). One potential reason for this increase can be mating displays. For example, ungulates often have an increased road crossing activity during the rutting period (Neumann and Ericsson, 2018; Mayer et al., 2021). Alternatively, increased human disturbance, e.g., during the hunting season typically in autumn, can also result in increased animal movement and displacement, and thus more road crossing behaviour (Mayer et al., 2021). Wild boar showed little variability in use of the at-grade fauna passage throughout the year, and this is also similar to the findings from other studies (Elfström, 2021; Brieger et al., 2022).
4.2 Collisions
The focal road segment was one of the densest for WVCs in southern Sweden, prior to the installation of fencing in 2019 (Nordström, 2014). Through the road mitigations, including jump-outs and cattle guards in addition to fencing and the at-grade fauna passage, overall WVCs have reduced by 66% along this road. This is in line with other studies that demonstrate that fencing in addition to safe crossing opportunities can reduce WVCs from between 50%–66% (Clevenger et al., 2001; Olsson, 2007; Sullivan et al., 2004; Strein et al., 2008; Huijser et al., 2009). While it was not the direct aim of the present study, future studies should compare the effectiveness of at-grade fauna passages to under- and overpass crossing structures, in order to determine how well they perform by comparison. These studies should also expand the focus away from ungulates and identify if at-grade crossings are used by other wildlife. If they are similarly effective, and feasible (according to traffic safety, road class, speed, and AADT), the reduced costs may warrant installation of at-grade fauna passage frequently within the road network. These investigations would contribute significantly to guidelines as to when at-grade fauna passages are appropriate strategies to mitigate the impacts of roads on wildlife.
In the present study, we did not demonstrate any “fence-end effects”, such as an increased frequency of WVCs at the end of the mitigated road section (Huijser et al., 2016). At the individual level, three out of 608 (0.5%) crossing animals were involved in a WVC at the at-grade fauna passage. Previous studies have reported a quite high variation of WVCs within the at-grade fauna passage, for example, 0.26% (Strein, 2010), 0.4% (Elfström, 2022) or 5.2% (Kastdalen, 1996) of crossing animals involved in WVC. But with an individual rate of 0.5% per year, the accumulated risk during the 4 years this at-grade fauna passage was operational would amount to a 2% risk of a WVC. Thus, while this study is limited in replication, we feel confident to suggest that at-grade fauna passage may be useful to reducing WVCs. Future studies should expand this work and incorporate more sites in order to develop more definitive answers.
The impact of traffic on ungulates depends highly on the speed and traffic volume on the road, and the landscapes through which the roads cross. Roads with an AADT ranging from 500 to 10 000 have been shown to deter animals during peak traffic hours (Alexander et al., 2005; Coe et al., 2015; Riginos et al., 2018; Gagnon et al., 2019; Kušta et al., 2017). Proximity to riparian habitat, on the other hand, can make high AADT-levels tolerable for ungulates (Gagnon et al., 2007). Roads with these aforementioned AADT could possibly be crossed by animals during the night when traffic volume is low (Gagnon et al., 2019; Kušta et al., 2017). The time between vehicles is also an important factor in the success of crossing. For example, mule deer (Odocoileus hemionus) were less likely to cross if the time between vehicles was shorter than 30. Only when there was 60 s or more between vehicles could mule deer cross the road safely (Riginos et al., 2018). Followers within a group of animals may accept a shorter distance between vehicles than leading animals or single individuals (Riginos et al., 2018). This may be problematic due to an increased risk of accidents. Given the influence traffic flow and speed have on the ability of ungulates to cross roads, these factors may also influence how often ungulates use an at-grade fauna passage. Thus, it is important to know what species are the target users of the at-grade fauna passage, and for future studies to investigate the effectiveness of at-grade fauna passage on roads with different traffic volumes and speeds.
4.3 Technical design considerations
One of the main limitations with at-grade fauna passage is that, by design, they provide access for animals to the roadway. If this is not carefully controlled, it is possible that wildlife will walk along the road and get trapped between the fencing, effectively rendering the fauna passage useless. In 12 of the 326 events we recorded over the year (3.7% of total events), animals walked around the fence terminus in the at-grade fauna passage, adjacent to the roadway, thus entering the fenced corridor. On all of those occasions these animals returned to the at-grade fauna passage without incident. This behaviour was primarily observed in roe deer (9 out of 12 events), that were browsing in the roadside verge, and then continued to do so in the road edge. It is important to consider vegetation in the design of the at-grade fauna passages to avoid such problems. Adding cattle guards to the edges of the crossing area may help to reduce ungulate access to the roadway, but may not necessarily work for all wildlife. Further, reducing the amount of roadside vegetation entirely may reduce browsing opportunities, thus reducing the amount of time individuals spend near the road. However, such actions should be considered on a site-by-site basis, since it can also come at a cost for other fauna, and may simply reduce how attractive the at-grade fauna passage is to wildlife to begin with. Further investigation into different vegetation and guarding schemes would help to improve the design of at-grade fauna passages.
In this study, we observed animal response to vehicles but could not observe driver reactions to wildlife or the warning signs. Detailed information of vehicle speed and time between vehicles related to the activation of RADS are missing in our database. In approximately 70% of the occasions when animals were within the at-grade fauna passage (in the road verges or on the road), there was at least one vehicle passing along the road through the fauna passage. Thus, it is imperative that drivers react to the warning sign and slowdown in order to limit the risk of WVC and allow animals pass successfully. Cars travelling at slower speeds are more likely to avoid WVC, particularly if drivers reduce their speed to 73 km/h or lower (Gunther et al., 1998; Muurinen and Ristola, 1999; Gordon et al., 2004; Sharafsaleh et al., 2012; Grace et al., 2017; Druta and Alden, 2019; Gagnon et al., 2019; Riginos et al., 2019). However, the difficulty is understanding and controlling driving behaviour (Collinson et al., 2019; Riginos et al., 2022). While drivers tend to slow down more during the night than during the day (Murrinen and Ristola, 1998; Sharafsaleh et al., 2012), some studies have shown habituation effects of drivers to signs (e.g., Sullivan et al., 2004), while others demonstrate high reactiveness throughout their entire study period (e.g., Sharafsaleh et al., 2012). To what extent a reduction of speed has on traffic safety and wildlife protection near an at-grade fauna passage remains to be determined. In addition to activation of a moose warning sign, we recommend that signs also suggest a slower speed. Furthermore, we suggest at-grade fauna passage should be coupled with speed cameras to help enforce speed reductions. Future studies should address this issue and incorporate it in the study design (see: Grace et al., 2017; Huijser et al., 2009), with traffic counters and vehicle speed monitors on the road before, within and after the at-grade fauna passage.
4.4 Recommendations and conclusion
In this study, we demonstrate the effectiveness of one at-grade fauna passage with RADS to provide safe crossing opportunities for wildlife, while, together with other measures, reducing the risk of WVCs. Typically, mitigation of WVCs are viewed from a traffic-safety perspective, rather than the wildlife perspective. As such, roads with medium to low AADT tend not to be fenced or otherwise mitigated. However, it is also apparent that WVCs are more frequent along medium and small roads. Different strategies will serve different purposes, and at-grade fauna passages can be included as another tool in the toolbox to reduce WVCs and improve landscape connectivity. On the landscape level, connectivity may be better benefited from several small crossing opportunities along the road network, rather than few, large, concentrated opportunities (Helldin 2022). Over- and underpasses will likely serve their purposes, and benefit those species that avoid roads and traffic, by providing a crossing opportunity that is separated from the flow of traffic. In areas where over- and underpasses are not possible to construct, at-grade fauna passages may provide a cost-efficient solution to improving the permeability of road networks for wildlife. The feasibility of this suggestion warrants investigation, the ultimate goal of reducing WVCs and maintain landscape connectivity for wildlife will likely be achieved through a combination of different mitigation measures. While our pilot only evaluates a single site, it does provide promising preliminary results that suggest that at-grade fauna passages can help in efforts to reduce collisions, while maintaining connectivity over medium-sized roads for large ungulates.
Data availability statement
The raw data supporting the conclusion of this article will be made available by the authors, without undue reservation.
Author contributions
MB: Statistical analysis–lead, writing–lead, editing–lead. FE: Data collection–equal contribution, statistical analysis–equal contribution, editing–equal contribution. IH: Data collection–equal contribution, statistical analysis–equal contribution, editing–equal contribution. AS: Conceptualization and design–contribution; Editing–contribution. EH: Data collection–equal contribution, statistical analysis–equal contribution, editing–equal contribution. ME: Conceptualization and design–contribution; Data collection–equal contribution, editing–equal contribution; writing–contribution. MO: Conceptualization and design–lead; Data collection–lead, writing–contribution, editing–equal contribution.
Funding
The study was conducted within the TRIEKOL research project financed by The Swedish Transport Administration.
Acknowledgments
We thank Jan-Olof Helldin for project coordination and for comments on the manuscript. We thank Mattias Blomberg and Johanna Holmin at the Transport Administration for practical assistance to access films of wildlife produced by the heat cameras. Finally, we thank the three reviewers to the valuable feedback they provided to help improve the quality of our manuscript.
Conflict of interest
The authors declare that the research was conducted in the absence of any commercial or financial relationships that could be construed as a potential conflict of interest.
Publisher’s note
All claims expressed in this article are solely those of the authors and do not necessarily represent those of their affiliated organizations, or those of the publisher, the editors and the reviewers. Any product that may be evaluated in this article, or claim that may be made by its manufacturer, is not guaranteed or endorsed by the publisher.
References
Alexander, S. M., Water, N. M., and Paquet, P. C. (2005). Traffic volume and highway permeability for a mammalian community in the Canadian Rocky Mountains. Can. Geogr. Ogr. Can. 49 (4), 321–331. doi:10.1111/j.0008-3658.2005.00099.x
Barrientos, R., and Bolonio, L. (2009). The presence of rabbits adjacent to roads increases polecat road mortality. Biodivers. Conserv. 18 (2), 405–418. doi:10.1007/s10531-008-9499-9
Bhardwaj, M., Teixara, F. Z., and Collinson, W. “The effects of roads on mammals,” in Road ecology: Synthesis and perspective. Editors M. D’Amico, R. Barrientos, and F. Ascensão (Springer Nature). Berlin, Germany New York City, USA (In Press).
Bhardwaj, M., Olsson, M., Håkansson, E., Söderström, P., and Seiler, A. (2022). Ungulates and trains –Factors influencing flight responses and detectability. J. Environ. Manag. 313, 114992. doi:10.1016/j.jenvman.2022.114992
Bond, A. R., and Jones, D. N. (2013). Wildlife warning signs: Public assessment of components, placement and designs to optimise driver response. Animals 3 (4), 1142–1161. doi:10.3390/ani3041142
Brieger, F., Kämmerle, J. L., Hagen, R., and Suchant, R. (2022). Behavioural reactions to oncoming vehicles as a crucial aspect of wildlife-vehicle collision risk in three common wildlife species. Accid. Analysis Prev. 168, 106564. doi:10.1016/j.aap.2021.106564
Bruinderink, G., and Hazebroek, E. (1996). Ungulate traffic collisions in Europe. Conserv. Biol. 4 (10), 1059–1067. doi:10.1046/j.1523-1739.1996.10041059.x
Chyn, K., Lin, T. E., Wilkinson, D. P., Tracy, J. L., Lawing, A. M., and Fitzgerald, L. A. (2021). Fine-scale roadkill risk models: Understanding the intersection of wildlife and roads. Biodivers. Conserv. 30 (1), 139–164. doi:10.1007/s10531-020-02083-6
Clevenger, A. P., Chruszcz, B., and Gunson, K. E. (2003). Spatial patterns and factors influencing small vertebrate fauna road-kill aggregations. Biol. Conserv. 109 (1), 15–26. doi:10.1016/s0006-3207(02)00127-1
Clevenger, A. P., Chruszcz, B., and Gunson, K. (2001). Highway mitigation fencing reduces WVC. Wildl. Soc. Bull. 29 (2), 646–653.
Coe, P. K., Nielson, R. M., Jackson, D. H., Cupples, J. B., Seidel, N. E., Johnson, B. K., et al. (2015). Identifying migration corridors of mule deer threatened by highway development. Wildl. Soc. Bull. 39 (2), 256–267. doi:10.1002/wsb.544
Collinson, W. J., Marneweck, C., and Davies-Mostert, H. T. (2019). Protecting the protected: Reducing wildlife roadkill in protected areas. Anim. Conserv. 22 (4), 396–403. doi:10.1111/acv.12481
Conover, M. R., Pitt, W. C., Kessler, K. K., Dubow, T. J., and Sanborn, W. A. (1995). Review of human injuries, illnesses, and economic losses caused by wildlife in the United States. Wildl. Soc. Bull. 23 (3), 407–414.
Druta, C., and Alden, A. S. (2019). Implementation and evaluation of a buried cable roadside animal detection system and deer warning sign (FHWA/VCTIR 19-R28). Virginia Department of Transportation.,Charlottesville, VA, United States.
Elfström, M. (2022). Miljöuppföljning - barriäreffekter för däggdjur vid planpassage för fauna och efter faunastängsling utmed väg 42 förbi Lagmansered under året 2020. Trafikverket i samverkan med EnviroPlanning AB. (in Swedish).
Elfström, M. (2021). Miljöuppföljning planpassage för fauna över väg 108 vid Sjödiken. Förekomst och fördelning av större däggdjur vid planpassage för fauna 2019-2020 (TRV 2021:109). Trafikverket i samverkan med EnviroPlanning AB. Borlänge: Trafikverkets publikationer. (in Swedish).
Ensing, E. P., Ciuti, S., de Wijs, F. A. L. M., Lentferink, D. H., ten Hoedt, A., Boyce, M. S., et al. (2014). GPS based daily activity patterns in European Red Deer and North American Elk (Cervus elaphus): Indication of a weak circadian clock in ungulates. PLOS ONE 9 (9), e106997. doi:10.1371/journal.pone.0106997
Gagnon, J. W., Dodd, N. K., Sprague, S. C., Ogren, K. S., Loberger, C. D., and Schweinsburg, R. E. (2019). Animal-activated highway crosswalk: Long term impact on elk-vehicle collisions, vehicle speeds, and motorist braking response. Hum. Dimensions Wildl. 24 (2), 132–147. doi:10.1080/10871209.2019.1551586
Gagnon, J. W., Theimer, T. C., Dodd, N. L., Boe, S., and Schweinsburg, R. E. (2007). Traffic volume alters elk distribution and highway crossings in Arizona. J. Wildl. Manage. 71 (7), 2318–2323. doi:10.2193/2006-224
Gordon, K. M., McKinstry, M. C., and Anderson, S. H. (2004). Motorist response to a deer sensing warning system. Wildl. Soc. Bull. 32 (2), 565–573. doi:10.2193/0091-7648(2004)32[565:mrtadw]2.0.co;2
Grace, M. K., Smith, D. J., and Noss, R. S. (2017). Reducing the threat of wildlife-vehicle collisions during peak tourism periods using a Roadside Animal Detection System. Accid. Analysis Prev. 109, 55–61. doi:10.1016/j.aap.2017.10.003
Gunther, K. A., Biel, M. J., and Robison, H. L. (February 1998).Factors influencing the frequency of road-killed wildlife in Yellowstone National Park Proceedings of the international conference on wildlife ecology and transportation. Fort Meyers, FL.
Helldin, J. O. (2022). Are several small wildlife crossing structures better than a single large? Arguments from the perspective of large wildlife conservation. Nat. Conserv. 47, 197–213. doi:10.3897/natureconservation.47.67979
Hill, J. E., DeVault, T. L., and Belant, J. L. (2021). A review of ecological factors promoting road use by mammals. Mamm. Rev. 51, 214–227. doi:10.1111/mam.12222
Huijser, M. P., and McGowen, P. (March 2003). “Overview of animal detection and animal warning systems in North America and Europe,” in Proceedings of the international conference on ecology and transportation, lake placid. Editor C. L. Irwin (New York, USA: North Carolina State University), 368–382.
Huijser, M. P., Fairbank, E. R., Camel-Means, W., Graham, J., Watson, V., Basting, P., et al. (2016). Effectiveness of short sections of wildlife fencing and crossing structures along highways in reducing wildlife–vehicle collisions and providing safe crossing opportunities for large mammals. Biol. Conserv. 197, 61–68. doi:10.1016/j.biocon.2016.02.002
Huijser, M. P., Holland, T. D., Kociolek, A. V., Barkdoll, A. M., and Schwalm, J. D. (2009). Animal – vehicle crash mitigation using advanced technology. Phase II: System effectiveness and system acceptance. FHWA-OR-TPF-09-14. Bozeman, USA: Western Transportation Institute – Montana State University.
Huijser, M. P., Mosler-Berger, C., Olsson, M., and Strein, M. (2015). “Wildlife warning signs and animal detection systems aimed at reducing WVC,” in The handbook of road ecology. Editors R. van der Ree, D. J. Smith, and C. Grilo (John Wiley & Sons),Hoboken, New Jersey 198–212.
Ikeda, T., Uchida, K., Matsuura, Y., Takahashi, H., Yoshida, T., Kaji, K., et al. (2016). Seasonal and diel activity patterns of eight sympatric mammals in northern Japan revealed by an intensive camera-trap survey. PLOS ONE 10, e0163602. doi:10.1371/journal.pone.0163602
Jaarsma, C. F., van Langevelde, F., Baveco, J. M., van Eupen, M., and Arisz, J. (2007). Model for rural transportation planning considering simulating mobility and traffic kills in the badger Meles meles. Ecol. Inf. 2 (2), 73–82. doi:10.1016/j.ecoinf.2007.04.004
Jacobson, S. L., Bliss-Ketchum, L. L., de Rivera, C. E., and Smith, W. P. (2016). A behavior-based framework for assessing barrier effects to wildlife from vehicle traffic volume. Ecosphere 7 (4), e01345. doi:10.1002/ecs2.1345
Jaeger, J. A. G., and Fahrig, L. (2004). Effects of road fencing on population persistence. Conserv. Biol. 18 (6), 1651–1657. doi:10.1111/j.1523-1739.2004.00304.x
Jasińska, K. D., Żmihorski, M., Krauze-Gryz, D., Kotowska, D., Werka, J., Piotrowska, D., et al. (2019). Linking habitat composition, local population densities and traffic characteristics to spatial patterns of ungulate-train collisions. J. Appl. Ecol 56 (12), 2630–2640.
Kämmerle, J. L., Brieger, F., Kröschel, M., Hagen, R., Storch, I., and Suchant, R. (2017). Temporal patterns in road crossing behaviour in roe deer (Capreolus capreolus) at sites with wildlife warning reflectors. PLOS ONE 12 (9), 01847611–e184818. doi:10.1371/journal.pone.0184761
Kastdalen, L. (1996). Romerikselgen og gardermoutbyggingen - hovedrapport fra elgprosjektet på Øvre Romerike. Fylkesmannen i Oslo og Akershus. miljøvernavdelingen. Oslo: Fylkesmannen i Oslo og Akershus.
Kistler, R. (1998). Wissenschaftliche begleitung der wildwarnanlagen Calstrom WWA-12-S. July 1995–November 1997. Schlussbericht. Switzerland: Infodienst Wildbiologie & Oekologie, Zürich.
Knufinke, J. F., Helldin, J. O., Bhardwaj, M., and Olsson, M. (2019). Temporal patterns of humans and ungulates at bridges. Uppsla: The Swedish.
Kušta, T., Keken, Z., Ježek, M., Holá, M., and Šmíd, P. (2017). The effect of traffic intensity and animal activity on probability of ungulate-vehicle collisions in the Czech Republic. Safety Science, 91, 105–113. doi:10.1016/j.ssci.2016.08.002
Langbein, J., Putman, R., Pokorny, B., Putman, R., Apollonio, M., and Andersen, R. (2011). “Traffic collisions involving deer and other ungulates in Europe and available measures for mitigation,” in Ungulate management in europe: Problems and practices. Editors R. Putman, M. Apollonio, and R. Andersen (Cambridge: Cambridge University Press), 215–259.
Lima, S. L., Blackwell, B. F., DeVault, T. L., and Fernández-Juricic, E. (2015). Animal reactions to oncoming vehicles: A conceptual review. Biol. Rev. 90 (1), 60–76. doi:10.1111/brv.12093
Litvaitis, J. A., and Tash, J. P. (2008). An approach toward understanding wildlife-vehicle collisions. Environ. Manag. 42, 688–697. doi:10.1007/s00267-008-9108-4
Lunn, R. B., Blackwell, B. F., DeVault, T. L., and Fernández-Juricic, E. (2022). Can we use antipredator behavior theory to predict wildlife responses to high-speed vehicles? PLOS ONE 17 (5), e0267774. doi:10.1371/journal.pone.0267774
Mata, C., Hervás, I., Herranz, J., Malo, J. E., and Suárez, F. (2009). Seasonal changes in wildlife use of motorway crossing structures and their implication for monitoring programmes. Transp. Res. Part D Transp. Environ. 14 (7), 447–452. doi:10.1016/j.trd.2009.05.001
Mayer, M., Nielsen, J. C., Elmeros, M., and Sunde, P. (2021). Understanding spatio-temporal patterns of deer-vehicle collisions to improve roadkill mitigation. J. Environ. Manag. 295, 113148. doi:10.1016/j.jenvman.2021.113148
Meisingset, E. L., Loe, L. E., Brekkum, Ø., Van Moorter, B., and Mysterud, A. (2013). Red deer habitat selection and movements in relation to roads. J. Wildl. Manage. 77 (1), 181–191. doi:10.1002/jwmg.469
Muurinen, I., and Ristola, T. (1999). Elk accidents can be reduced by using transport telematics. Finncontact 7 (1), 7–8.
Mysłajek, R. W., Olkowska, E., Wronka-Tomulewicz, M., and Nowak, S. (2020). Mammal use of wildlife crossing structures along a new motorway in an area recently recolonized by wolves. Eur. J. Wildl. Res. 66 (79), 79. doi:10.1007/s10344-020-01412-y
Nationella viltolycksrådet, (2022). Official statistics on wildlife-vehicle collisions in Sweden. www.viltolycka.se.
Neumann, W., Ericsson, G., Dettki, H., Bunnefeld, N., Keuler, N. S., Helmers, D. P., et al. (2012). Difference in spatiotemporal patterns of wildlife road-crossings and wildlife-vehicle collisions. Biol. Conserv. 145 (1), 70–78. doi:10.1016/j.biocon.2011.10.011
Neumann, W., and Ericsson, G. (2018). Influence of hunting on movements of moose near roads. J. Wildl. Manage. 82 (5), 918–928. doi:10.1002/jwmg.21448
Nordström, A. (2014). Förenklad åtgärdsvalstudie väg 108, faunapassage vid Bökeberg. Trafikverket. Prestudy Proj. (in Swedish).
Olsson, M. (2007). The use of highway crossings to maintain landscape connectivity for moose and roe deer. Dissertation, Karlstad University studies. 16.
Riginos, C., Fairbank, E., Hansen, E., Kolek, J., and Huijser, M. (2019). Effectiveness of night-time speed limit Reduction in reducing WVC (FHWA-WY-1904F).Cheyenne Wyoming Department of Transportation.
Riginos, C., Fairbank, E., Hansen, E., Kolek, J., and Huijser, M. P. (2022). Reduced speed limit is ineffective for mitigating the effects of roads on ungulates. Conserv. Sci. Pract. 4, e618. doi:10.1111/csp2.618
Riginos, C., Smith, C., Fairbank, E., Hansen, E., and Hallsten, P. (2018). Traffic Thresholds in deer road-crossing behavior (WY-1807F).Cheyenne Wyoming Department of Transportation.
Romer, J., Mosler, C., and Kistler, R. (November 2003). Preventing vehicle–WVCs. The animal detection system CALSTROM. Poster presentation infra eco network europe (IENE) conference. Habitat fragmentation due to transport infrastructure & presentation to the COST 341 action. Brussels, Belgium. November 13–15,2003.
Seiler, A., and Bhardwaj, M. (2020). “Wildlife and traffic: An inevitable but not unsolvable problem?,” in Problematic wildlife II. Editors F. Angelici, and L. Rossi (Cham: Springer), 171–190.
Seiler A. (2005). Predicting locations of moose-vehicle collisions in Sweden. J. Appl. Ecol 42, 371–382. doi:10.1111/j.1365-2664.2005.01013.x
Seiler, A.,, and Folkeson, L., (Editors) (2006). Cost 341 – habitat fragmentation due to transportation infrastructure: National state-of-the-art report Sweden (Linköping, Sweden: VTI).
Sharafsaleh, M., Huijser, M., Nowakowski, C., Greenwood, M. C., Hayden, L., Felder, J., et al. (2012). California PATH research report.Evaluation of an animal warning system effectiveness phase two – final report (UCB-ITS-PRR-2012-12) California, Berkeley, Institute of Transportation Studies, University of California Berkeley.
Smith, D. J., van der Ree, R., and Rosell, C. (2015). “Wildlife crossing Structures: An effective strategy to restore or maintain wildlife connectivity across roads,” in The handbook of road ecology. Editors R. van der Ree, D. J. Smith, and C. Grilo (John Wiley & Sons),New York, United States 172–183.
Strein, M., Burghardt, F., Haas, F., and Suchant, R. (2008). Pilotprojekt Elektronische Wildwarnanlage B292 bei Aglasterhausen. Report to Innenministeriums Baden-Württemberg.Zum Monitoring nach zwei Betriebsjahren im Auftrag des Innenministeriums Baden-W{\"u}rttemberg Projekt BWPLUS L,7525003.
Sullivan, T. L., Williams, A. F., Messmer, T. A., Hellinga, L. A., and Kyrychenko, S. Y. (2004). Effectiveness of temporary warning signs in reducing deer-vehicle collisions during mule deer migrations. Wildl. Soc. Bull. 32 (3), 907–915. doi:10.2193/0091-7648(2004)032[0907:eotwsi]2.0.co;2
Trafikverket, (2021). Viltvarningssystem vid faunapassage, version 2. Borlénge: Trafikverkets publikationer Swedish, 2021–2112.-07.
van der Ree, R., Gagnon, J. W., and Smith, D. J. (2015). “Fencing: A valuable tool for reducing wildlife–vehicle collisions and funnelling fauna to crossing structures,” in The handbook of road ecology. Editors R. van der Ree, D. J. Smith, and C. Grilo (John Wiley & Sons),New York, United States 159–171.
Keywords: at-grade fauna passage, animal behavior, road ecology, traffic, AADT, RADS
Citation: Bhardwaj M, Erixon F, Holmberg I, Seiler A, Håkansson E, Elfström M and Olsson M (2022) Ungulate use of an at-grade fauna passage and roadside animal detection system: A pilot study from Southern Sweden. Front. Environ. Sci. 10:991551. doi: 10.3389/fenvs.2022.991551
Received: 11 July 2022; Accepted: 21 November 2022;
Published: 06 December 2022.
Edited by:
Francesco Maria Angelici, National Center for Wildlife, Riyadh, Saudi ArabiaReviewed by:
Emmanuel Serrano Ferron, Universitat Autònoma de Barcelona, SpainTom Langen, Clarkson University, United States
Copyright © 2022 Bhardwaj, Erixon, Holmberg, Seiler, Håkansson, Elfström and Olsson. This is an open-access article distributed under the terms of the Creative Commons Attribution License (CC BY). The use, distribution or reproduction in other forums is permitted, provided the original author(s) and the copyright owner(s) are credited and that the original publication in this journal is cited, in accordance with accepted academic practice. No use, distribution or reproduction is permitted which does not comply with these terms.
*Correspondence: Manisha Bhardwaj, manisha.bhardwaj@live.ca