- 1Department of Ecoscience, Aarhus University, Roskilde, Denmark
- 2Arctic Research Centre, Aarhus University, Aarhus, Denmark
- 3Conservation of Arctic Flora and Fauna (CAFF), Secretariat, Akureyri, Iceland
- 4Icelandic Institute of Natural History, Garðabær, Iceland
- 5Polar Knowledge Canada, Canadian High Arctic Research Station, Cambridge Bay, NU, Canada
Extreme weather events are increasing in frequency and intensity across the Arctic, one of the planet’s most rapidly warming regions. Studies from southern latitudes have revealed that the ecological impacts of extreme events on living organisms can be severe and long-lasting, yet data and evidence from within the terrestrial Arctic biome appear underrepresented. By synthesizing a total of 48 research articles, published over the past 25 years, we highlight the occurrence of a wide variety of extreme events throughout the Arctic, with multiple and divergent impacts on local biota. Extreme event impacts were quantified using a myriad of approaches ranging from circumpolar modelling to fine-scale experimental studies. We also identified a research bias towards the quantification of impacts related to a few extreme event types in the same geographic location (e.g. rain-on-snow events in Svalbard). Moreover, research investigating extreme event impacts on the ecology of arthropods and especially freshwater biota were scant, highlighting important knowledge gaps. While current data allow for hypotheses development, many uncertainties about the long-term consequences of extreme events to Arctic ecosystems remain. To advance extreme event research in the terrestrial Arctic biome, we suggest that future studies i) objectively define what is extreme in terms of events and ecological impacts using long-term monitoring data, ii) move beyond single-impact studies and single spatial scales of observation by taking advantage of pan-Arctic science-based monitoring networks and iii) consider predictive and mechanistic modelling to estimate ecosystem-level impacts and recovery.
Introduction
There is ample scientific evidence revealing the destructive impacts from long-term changes in mean climatic conditions on biodiversity and ecosystem functioning (Sintayehu, 2018; Turner et al., 2020; Weiskopf et al., 2020). However, in addition to gradual changes in global climate, there is also an increase in the intensity, frequency and duration of extreme events that are directly or indirectly related to weather and climate. Extreme events are considered rare within their statistical reference distributions at a particular time or place (IPCC, 2012); from here on: “extreme events”. Some well-known examples are transient but severe precipitation events (Kirchmeier-Young and Zhang, 2020), heatwaves (Perkins-Kirkpatrick and Lewis, 2020) and wildfires (Boer et al., 2020). The impact of extreme events on ecosystems can be severe and long-lasting (Harris et al., 2018), with profound implications for species conservation and environmental management (Maxwell et al., 2019). Documenting, quantifying, and understanding the impacts of extreme events on species and their natural environment has become a prominent research focus, demonstrated by the sharp increase in the number of published research papers over the last 5 decades (Figure 1A) and numerous systematic reviews on the topic (Ummenhofer and Meehl, 2017; McPhillips et al., 2018; Aoki et al., 2022).
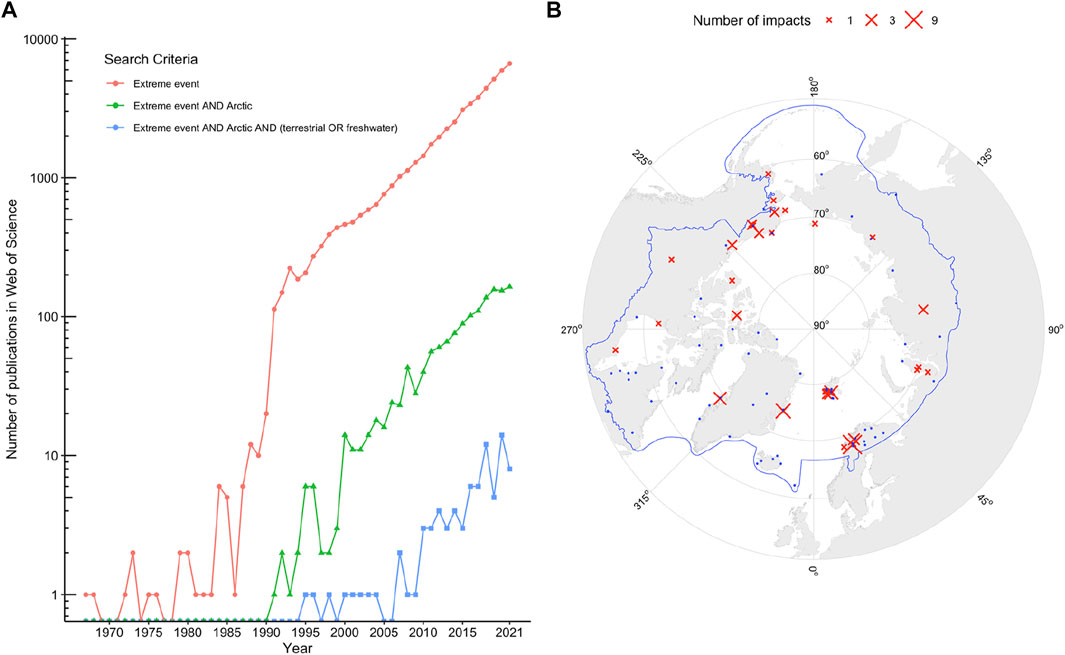
FIGURE 1. Panel a shows trends in annual number of published research articles dealing with extreme events across the entire globe (in SALMON), extreme event studies in the Arctic (in GREEN) and extreme event studies in the Arctic considering only terrestrial and freshwater studies (in BLUE). Trends are based on a literature search through the Institute for Scientific Information (ISI) Web of Science database (June 2022) using the search criteria provided in the figure legend. Note that the y-axis of the graph is on a logarithmic scale. Panel b shows the location (indicated with a red ×) of the 48 published studies included in our mini-review that were concerned with impacts of extreme events on terrestrial and freshwater biota in the Arctic. The size of the study location indicator is scaled relative to the total number of impacts quantified (n = 69). The Arctic boundary as defined by the Conservation of Arctic Flora and Fauna (CAFF) group is shown as a blue line. Blue dots on the map show the location of research stations connected to the International Network for Terrestrial Research and Monitoring in the Arctic (INTERACT).
Nowhere else is climate change more apparent than in the Arctic (Post et al., 2019; IPCC, 2021). Because of the long-term and gradual increase in surface air temperatures, sea ice loss, and permafrost thaw associated with Arctic amplification (Previdi et al., 2021), the Arctic biophysical system is now experiencing unprecedented change, with implications for regions far beyond the Arctic (Box et al., 2019). Occurrences of extreme events across the region have also increased considerably over the past decades (Landrum and Holland, 2020; Walsh et al., 2020), specifically in terms of precipitation (Bintanja, 2018) and temperature (Dobricic et al., 2020) anomalies. In some areas, such extremes are leading to a marked increase in frequency of icing (Tyler, 2010) and rain-on-snow events during winter (Putkonen et al., 2009) with negative ecological and socioeconomic consequences (Hansen et al., 2014; Forbes et al., 2016). As elsewhere in the world, the intensity and duration of wildfires are also increasing and pose a serious risk to the carbon-storage capacity of the Arctic ecosystem (Hu et al., 2015).
The potential of extreme events to influence the Arctic and its biodiversity is substantial and documenting these impacts is a research priority (Post et al., 2009b; McCrystall et al., 2021). However, the number of published papers dealing with extreme event impacts in the Arctic biome represents only a small fraction of the entire research output on this topic and, moreover, the number of studies on terrestrial and freshwater biota appear especially limited (Figure 1A). To fill this knowledge gap, we synthesized the scientific literature dealing with extreme events in the Arctic and their ecological impacts on terrestrial and freshwater biota. We are particularly interested in providing an overview of the different types of extreme events studied, the ecological impacts detected, the focal species or taxonomic groups studied, the methods employed, and whether or not impact recovery was quantified. We finish the mini-review with a number of recommendations aimed to facilitate the detection, evaluation, and documentation of extreme event impacts on terrestrial and freshwater species within the Arctic biome.
Extreme event research in the terrestrial arctic: A mini-review
Based on a literature search through the Institute for Scientific Information (ISI) Web of Science database and using the search criteria “Extreme event” AND “Arctic” AND (“terrestrial” OR “freshwater”), we found 48 published studies that investigated ecological impacts of extreme events on terrestrial and freshwater biota in the Arctic (Figure 1B). An overview of the published articles and data extracted can be found in Supplementary Table S1.
Our literature search detected research from sites that were scattered across the Arctic region (Figure 1B), yet the majority of studies were conducted in mainland Scandinavia (n = 16), Svalbard (n = 14), Alaska (n = 13), and Greenland (n = 11) and relatively few extreme event impact studies took place in Russia (n = 7) and Canada (n = 7). No published studies were found from Iceland, Finland and the Faroe Islands. Geographic bias in research output is a known limitation within ecological literature originating from temperate and tropical systems (Culumber et al., 2019). Such bias may lead to important gaps in scientific knowledge with implications for environmental management, conservation and policy. While it has been argued that studies of extreme events in northern regions are somewhat opportunistic and uncoordinated (Walsh et al., 2020), geographic bias in extreme event research in the Arctic has never been formally tested. A likely explanation for the clustered research output identified here is that logistical constraints associated with working in remote and often inaccessible areas in the Arctic hinders the design and implementation of coordinated and geographically balanced research (Metcalfe et al., 2018).
Ten different types of extreme events in the Arctic were reported in the literature (Figure 2) with most of the effort dedicated to quantifying potential impacts of rain-on-snow events on terrestrial biota (n = 19). Extreme summer cooling and rain events, pest outbreaks and landslides were reported least (Figure 2). In addition, the vast majority of studies reported on ecological responses following a single extreme event (n = 44) and only four studies quantified impacts of multiple extreme events. Although extreme events are by definition rare and as such single-event studies should be most common, the occurrence of multiple extreme events as well as the documentation of their impacts on species and ecosystems elsewhere in the world are increasing (Bailey and van de Pol, 2016). While the current data and knowledge gained through single-event studies in the Arctic can contribute to future hypothesis development and more dedicated experimental designs, there seems to be a need for a greater focus on multi-event studies as these are expected to become more common under continuing global warming (Raymond et al., 2020).
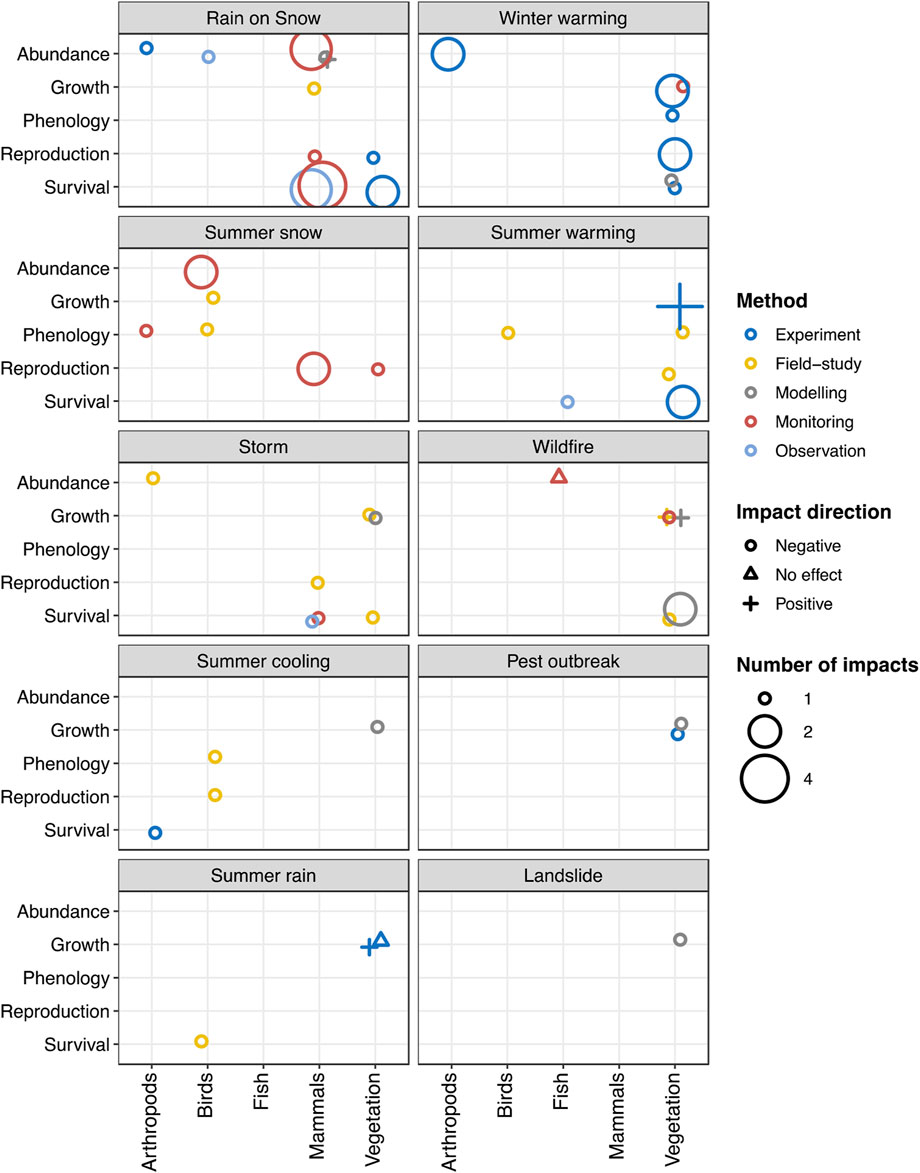
FIGURE 2. Categorization of the ecological impacts (n = 69) of extreme events on biota that were observed in terrestrial and freshwater Arctic ecosystems as documented in 48 studies published between 1995 and 2021. The shapes of the symbols indicate the direction of the ecological impact detected. The colours of the symbols reflect the method used to quantify the impact, and the size of the symbols are scaled relative to the number of studies that detected the impact.
To synthesize ecological impacts of extreme events on Arctic biota (Figure 2), we relied on five categories as used in the structure of the Circumpolar Biodiversity Monitoring Programme (CBMP), which was developed specifically to monitor and report on trends in Arctic biodiversity entities (Christensen et al., 2012; Culp et al., 2012). Potential ecological impacts of extreme events were most often quantified on vegetation (n = 33) followed by mammals (n = 19), birds (n = 9), arthropods (n = 6) and lastly on freshwater fish (n = 2). Hence, the total number of ecological impacts included in our mini-review (n = 69) was larger than the number of published studies (Figure 1 and Figure 2, Supplementary Table S1). Studying climate change effects on arthropods in the Arctic is a known research gap (Høye, 2020), but the reasons for the few published research articles of extreme event impacts on freshwater biota in the Arctic are unclear, especially given the large body of work done on extreme event impacts in aquatic systems elsewhere in the world (Aoki et al., 2022). It is possible that freshwater systems in the Arctic are generally studied less compared to terrestrial systems or that extreme events are less common in Arctic freshwater systems. Alternatively, studies on extreme events in freshwater systems may be reported using different definitions and terminology than those used in our literature search criteria. Whatever the reason, multiple and intimate linkages exist between terrestrial and aquatic ecosystems (Gratton et al., 2008; Wrona et al., 2016), and a more integrated approach to quantify extreme event effects on catchments in the future seems desirable, at least in the Arctic.
Ecological responses to extreme events by terrestrial and freshwater biota in the Arctic were predominantly negative (n = 61), with six of the ecological impacts being positive and only two publications found no effect (Figure 2). Positive responses were mostly restricted to increased growth of Arctic vegetation following wildfires (Frost et al., 2020) or experimental summer warming (Marchand et al., 2005). Interestingly, simulating extreme rain events during summer also increased growth of the shrub vegetation in north east Siberia but the same experimental treatment in northern Sweden showed no effect on the same vegetation community (Keuper et al., 2012). It remains unclear why extreme events can have such divergent impacts on the same species or conversely why ecological impacts are often species- or group-specific (i.e. idiosyncratic) and what the involved mechanisms are. Such understanding is required to improve our predictive capacity on future change so as to determine whether extreme event-induced ecological impacts are important determinants of long-term population trends or ecosystem changes (van de Pol et al., 2017).
Quantification of extreme event impacts on Arctic biota were most often obtained through experimental studies (n = 20), followed by empirical monitoring (n = 18), field-studies (n = 15), modelling (n = 10) and opportunistic observation (n = 6). The size of the impacted area varied between the different methods employed (Supplementary Figure S1) with experiments covering smaller areas than dedicated field-studies, long-term monitoring, opportunistic observation data, and empirical-based modelling approaches. Impact recovery (i.e. post extreme event) on Arctic biota was assessed in 42% of the cases (n = 29) while in the remaining 58% this was not considered or done (n = 40). Impact recovery was most often quantified using experimental (14% of all cases) or long-term monitoring studies (10% of cases).
Overall, this mini-review highlights that a wide variety of extreme events do occur throughout the Arctic and dedicated effort has been undertaken over the past 25 years to document and quantify associated ecological impacts on local biota. It is certainly possible that this mini-review underestimates the work being done on extreme events in the Arctic as we did not, for example, consider “grey” literature. While this makes our synthesis necessarily myopic, the rationale was to provide a concise and transparent overview of extreme event impacts in the Arctic based on research that is easily accessible and interpretable by the entire scientific community. In doing so, we identified various knowledge gaps and outstanding questions related to extreme event effects. As climate change will continue to put pressure on Arctic biodiversity (Post et al., 2009a; Box et al., 2019), improved and coordinated efforts to study and monitor potential impacts and recoveries over extended temporal and geographic ranges are critical. Below we provide a number of recommendations that will hopefully benefit the design and effectiveness of future research and monitoring efforts on extreme event impacts in the Arctic biome.
Define what is considered extreme in terms of event and ecological impact
There is a general lack of coherence and scientific agreement in what constitutes and defines an extreme event and whether or not an ecological impact is even required (van de Pol et al., 2017; McPhillips et al., 2018; Latimer and Zuckerberg, 2019). In our mini-review, we considered both qualitative and quantitative definitions of extreme events as well as “no effect” studies, but many of the examined papers were opaque in their definition or qualification of the extremeness of the event and any associated impact. Lack of clear definitions of “extreme” makes it difficult to formally attribute ecological impacts or responses to anthropogenic climate change and, moreover, limits our ability to generalize ecological responses from site-specific events and comparison of findings through time and space (i.e. meta-analyses). Therefore, we support previous pleas that clear definitions of what constitutes extreme are needed (Smith, 2011; Aoki et al., 2022). Because extreme events and their impacts are research foci across disciplines (Broska et al., 2020) more precise definitions of extreme events will improve the application of ecological research within a multidisciplinary research context. We do acknowledge, however, that adopting a universal definition of extreme is difficult under contemporary climate and environmental change. This may be especially applicable to research taking place in the Arctic where climate change is occurring at such a pace that baseline estimates and values on weather and climatic conditions are rapidly changing. This means that what may be quantified as an anomaly or extreme event now, may no longer be so in the (near) future (Landrum and Holland, 2020). Fitting examples of this issue are rain-on-snow events and tundra wildfires. Although such events were rare and considered extreme in the past (and still are in some areas), they appear to become routine (e.g. annual) occurrence in some areas of the Arctic and could thus be considered the new normal. Nonetheless, consistent definitions, quantification and clear communication of extreme events can only enhance the understanding and management of potential impacts. We urge future studies in the Arctic to objectively and quantitatively define the extremeness of the event (i.e. conditions observed are at the xth percentile of their statistical reference distributions at a particular time or place) but also from the perspective of the response of the biota under investigation by comparing the impacts to baseline variability in the system. Clearly the formulation of such definitions relies on long-term data of environmental conditions as being collected in some science-based monitoring programs such as the International Tundra Experiment (ITEX: Henry and Molau, 1997), the Long Term Ecological Research Network (LTER: Knapp et al., 2012) and the Circumpolar Biodiversity Monitoring Program (CBMP: Christensen et al., 2020). Increased collaboration among such long-term and science-based monitoring programmes across the Arctic would greatly benefit our understanding of extreme event impacts on the Arctic environment and biota as it facilitates collection of critical data on the spatiotemporal variation in the occurrences of extreme events and their ecological responses.
Go beyond single-event-impact studies and spatial scales of observation
As we move further into the Anthropocene with extreme climatic events impacting biodiversity and peoples livelihood (Hansen et al., 2014; Forbes et al., 2016), there is a strong scientific and socioeconomic need to go beyond single-event and single-impact studies. We found only three studies that quantified multiple impacts of an extreme event across local biota (Supplementary Table S1). However, given that food webs in the Arctic are highly interconnected (Schmidt et al., 2017), impacts on one trophic level may easily cascade onto others, with potential ecosystem-wide consequences. Drawing (statistical) inference on the ecological impacts of extreme climatic events, and identifying the mechanisms involved, relies on replication and control across a wide range and number of events and impacts (Altwegg et al., 2017). Again, long-term and science-based monitoring programmes provide the temporal resolution and data needed to start quantifying patterns and drivers of natural variability in biodiversity across scales. Such programmes will also increase chances of capturing effects of multiple extreme events in an area or multiple impacts of single events, and finally they allow for quantification of potential cascading consequences. Crucial in this respect is experimental replication over time and space so as to increase the likelihood of collecting data from areas that did experience the extreme events (impact) and sites that did not (control) but also before and after an extreme event (Elmendorf et al., 2012). We recommend that such experimental setups within a sound hypothesis testing framework are implemented more widely across the Arctic, which would be possible by taking advantage of an already existing network of research stations under the umbrella of the International Network for Terrestrial Research and Monitoring in the Arctic (INTERACT, 2015). One clear advantage of designing and implementing a coordinated research structure on extreme event impacts using INTERACT is that it will likely reduce geographic bias in research output identified in our mini-review as connected research stations are present in some of the areas that are currently understudied (Figure 1B). Moreover, a pan-Arctic, long-term monitoring and science-based network will facilitate the application of a myriad of data collection methods that can transcend difficulties with spatial and temporal upscaling of results (Ummenhofer and Meehl, 2017). We acknowledge that it is not always possible to design long-term experiments or use a combination of research methods that captures all possible impacts or extreme events, and most often study designs need to be tailored to specific research questions. However, to achieve a more holistic understanding of extreme event impacts the goal should be to use a combination of research methods as much as possible and where baseline data from long-term science-based monitoring programmes are complemented with insights from field studies, opportunistic observations, remote sensing, experimental data, and models. Long-term experiments or dedicated field studies are especially valuable as they can identify potential lag effects, feedbacks and interactions of extreme event impacts and to assess system recovery (Harris et al., 2020). Implementing multiple study designs into pan-Arctic research networks will offer great potential to further improve our understanding of extreme event impacts that can occur across multiple spatial and temporal scales.
Consider predictive modelling and ecosystem-level impacts
Empirical data and evidence on the impacts of extreme events on biota are often geographically and taxonomically idiosyncratic (Bailey and van de Pol, 2016; Metcalfe et al., 2018; Landrum and Holland, 2020). We also found that the direction of extreme event impacts in the Arctic sometimes differed within and between biota and across extreme events (Figure 2). Although this highlights that the predictability of local responses to extreme events is low, and more empirical data is clearly required to better understand the mechanisms and processes involved, we echo the view that predictive modelling efforts are a useful addition to the toolkit required to tackle some of the current knowledge gaps (Sillmann et al., 2017; Boult and Evans, 2021). Empirical data derived from long-term, science-based monitoring programmes combined with e.g., remote sensing products greatly facilitates the development of hindcasting and forecasting models to assess previous and future conditions over large geographic areas and temporal scales. While modelling entire ecosystems is a demanding task, statistical models that can incorporate multiple data streams derived from research-based long-term monitoring programmes to assess ecosystem dynamics and state changes are already available (McClintock et al., 2020). We see particular value for what are called next-generation Individual Based Models, which can be tailored specifically to study and assess how ecosystem function and services respond to changes in e.g. land use and climate (Grimm et al., 2017). Such process-based simulation models are constructed using pattern-oriented modelling approaches based on data that can be directly provided by long-term monitoring programmes and experimental work embedded within. Doing so makes these models structurally realistic and increases predictive power when applied under changing climatic conditions (Stillman et al., 2015). As we collect more empirical data and gain better understanding of the processes and drivers underlying extreme events and their impacts on Arctic biota, these models can be used to iteratively evaluate and progress current evidence and knowledge.
Author contributions
FvB, TB, TC, and NS conceived the study. FvB coordinated the literature search and all authors contributed to the search and review of articles. FvB drafted the manuscript and all authors critically revised the manuscript, gave approval for publication, and agree to be accountable for all aspects of the work.
Acknowledgments
We thank the International Network for Terrestrial Research and Monitoring in the Arctic (INTERACT)-III for financial support (GA n. 871120). We also wish to thank Knud Falk and Willem Goedkoop for their constructive feedback on a previous version of this manuscript.
Conflict of interest
The authors declare that the research was conducted in the absence of any commercial or financial relationships that could be construed as a potential conflict of interest.
Publisher’s note
All claims expressed in this article are solely those of the authors and do not necessarily represent those of their affiliated organizations, or those of the publisher, the editors and the reviewers. Any product that may be evaluated in this article, or claim that may be made by its manufacturer, is not guaranteed or endorsed by the publisher.
Supplementary material
The Supplementary Material for this article can be found online at: https://www.frontiersin.org/articles/10.3389/fenvs.2022.983637/full#supplementary-material
References
Altwegg, R., Visser, V., Bailey, L. D., and Erni, B. (2017). Learning from single extreme events. Phil. Trans. R. Soc. B 372, 20160141. doi:10.1098/rstb.2016.0141
Aoki, L. R., Brisbin, M. M., Hounshell, A. G., Kincaid, D. W., Larson, E. I., Sansom, B. J., et al. (2022). Preparing aquatic research for an extreme future: Call for improved definitions and responsive, multidisciplinary approaches. Bioscience 72, 508–520. doi:10.1093/BIOSCI/BIAC020
Bailey, L. D., and van de Pol, M. (2016). Tackling extremes: Challenges for ecological and evolutionary research on extreme climatic events. J. Anim. Ecol. 85, 85–96. doi:10.1111/1365-2656.12451
Bintanja, R. (2018). The impact of Arctic warming on increased rainfall. Sci. Rep. 8, 16001–16006. doi:10.1038/s41598-018-34450-3
Boer, M. M., Resco de Dios, V., and Bradstock, R. A. (2020). Unprecedented burn area of Australian mega forest fires. Nat. Clim. Chang. 10, 171–172. doi:10.1038/s41558-020-0716-1
Boult, V. L., and Evans, L. C. (2021). Mechanisms matter: Predicting the ecological impacts of global change. Glob. Chang. Biol. 27, 1689–1691. doi:10.1111/GCB.15527
Box, J. E., Colgan, W. T., Christensen, T. R., Schmidt, N. M., Lund, M., Parmentier, F. J. W., et al. (2019). Key indicators of arctic climate change: 1971-2017. Environ. Res. Lett. 14, 045010. doi:10.1088/1748-9326/aafc1b
Broska, L. H., Poganietz, W. R., and Vögele, S. (2020). Extreme events defined—a conceptual discussion applying a complex systems approach. Futures 115, 102490. doi:10.1016/J.FUTURES.2019.102490
Christensen, T., Barry, T., Taylor, J. J., Doyle, M., Aronsson, M., Braa, J., et al. (2020). Developing a circumpolar programme for the monitoring of Arctic terrestrial biodiversity. Ambio 49, 655–665. doi:10.1007/s13280-019-01311-w
Christensen, T., Payne, J., Doyle, M., Ibarguchi, G., Taylor, J., Schmidt, N. M., et al. (2012). The arctic terrestrial biodiversity monitoring plan. Akureyri, Iceland: CAFF.
Culp, J., Goedkoop, W., Lento, J., Christoffersen, K., Frenzel, S., Guobergsson, G., et al. (2012). Arctic freshwater biodiversity monitoring plan. Akureyri, Iceland: Caff. Available at: https://www.caff.is/freshwater/freshwater-monitoring-publications/196-arctic-freshwater-biodiversity-monitoring-plan (Accessed March 25, 2021).
Culumber, Z. W., Anaya-Rojas, J. M., Booker, W. W., Hooks, A. P., Lange, E. C., Pluer, B., et al. (2019). Widespread biases in ecological and evolutionary studies. Bioscience 69, 631–640. doi:10.1093/BIOSCI/BIZ063
Dobricic, S., Russo, S., Pozzoli, L., Wilson, J., and Vignati, E. (2020). Increasing occurrence of heat waves in the terrestrial Arctic. Environ. Res. Lett. 15, 024022. doi:10.1088/1748-9326/ab6398
Elmendorf, S. C., Henry, G. H. R., Hollister, R. D., Björk, R. G., Bjorkman, A. D., Callaghan, T. V., et al. (2012). Global assessment of experimental climate warming on tundra vegetation: Heterogeneity over space and time. Ecol. Lett. 15, 164–175. doi:10.1111/j.1461-0248.2011.01716.x
Forbes, B. C., Kumpula, T., Meschtyb, N., Laptander, R., Macias-Fauria, M., Zetterberg, P., et al. (2016). Sea ice, rain-on-snow and tundra reindeer nomadism in Arctic Russia. Biol. Lett. 12, 20160466. doi:10.1098/rsbl.2016.0466
Frost, G. V., Loehman, R. A., Saperstein, L. B., Macander, M. J., Nelson, P. R., Paradis, D. P., et al. (2020). Multi-decadal patterns of vegetation succession after tundra fire on the Yukon-Kuskokwim Delta, Alaska. Environ. Res. Lett. 15, 025003. doi:10.1088/1748-9326/ab5f49
Gratton, C., Donaldson, J., and Zanden, M. J. V. (2008). Ecosystem linkages between lakes and the surrounding terrestrial landscape in northeast Iceland. Ecosystems 11, 764–774. doi:10.1007/s10021-008-9158-8
Grimm, V., Ayllón, D., and Railsback, S. F. (2017). Next-generation individual-based models integrate biodiversity and ecosystems: Yes we can, and yes we must. Ecosystems 20, 229–236. doi:10.1007/s10021-016-0071-2
Hansen, B. B., Isaksen, K., Benestad, R. E., Kohler, J., Pedersen, Å., Loe, L. E., et al. (2014). Warmer and wetter winters: Characteristics and implications of an extreme weather event in the High Arctic. Environ. Res. Lett. 9, 114021–114031. doi:10.1088/1748-9326/9/11/114021
Harris, R. M. B., Beaumont, L. J., Vance, T. R., Tozer, C. R., Remenyi, T. A., Perkins-Kirkpatrick, S. E., et al. (2018). Biological responses to the press and pulse of climate trends and extreme events. Nat. Clim. Chang. 8, 579–587. doi:10.1038/s41558-018-0187-9
Harris, R. M. B., Loeffler, F., Rumm, A., Fischer, C., Horchler, P., Scholz, M., et al. (2020). Biological responses to extreme weather events are detectable but difficult to formally attribute to anthropogenic climate change. Sci. Rep. 10, 14067. doi:10.1038/s41598-020-70901-6
Henry, G. H. R., and Molau, U. (1997). Tundra plants and climate change: The international tundra experiment (ITEX). Glob. Chang. Biol. 3, 1–9. doi:10.1111/J.1365-2486.1997.GCB132.X
Høye, T. T. (2020). Arthropods and climate change – arctic challenges and opportunities. Curr. Opin. Insect Sci. 41, 40–45. doi:10.1016/j.cois.2020.06.002
Hu, F. S., Higuera, P. E., Duffy, P., Chipman, M. L., Rocha, A. V., Young, A. M., et al. (2015). Arctic tundra fires: Natural variability and responses to climate change. Front. Ecol. Environ. 13, 369–377. doi:10.1890/150063
INTERACT (2015). Research and monitoring international network for terrestrial research and monitoring in the arctic. Brussels: European Commision.
IPCC (2021). Climate change 2021: The physical science basis. Contribution of working group I to the sixth assessment report of the intergovernmental Panel on climate change. Cambridge, UK: Cambridge University Press.
Keuper, F., Parmentier, F. J. W., Blok, D., Van Bodegom, P. M., Dorrepaal, E., Van Hal, J. R., et al. (2012). Tundra in the rain: Differential vegetation responses to three years of experimentally doubled summer precipitation in siberian shrub and Swedish bog tundra. Ambio 41, 269–280. doi:10.1007/s13280-012-0305-2
Kirchmeier-Young, M. C., and Zhang, X. (2020). Human influence has intensified extreme precipitation in North America. Proc. Natl. Acad. Sci. U. S. A. 117, 13308–13313. doi:10.1073/pnas.1921628117
Knapp, A. K., Smith, M. D., Hobbie, S. E., Collins, S. L., Fahey, T. J., Hansen, G. J. A., et al. (2012). Past, present, and future roles of long-term experiments in the LTER network. Bioscience 62, 377–389. doi:10.1525/BIO.2012.62.4.9
Landrum, L., and Holland, M. M. (2020). Extremes become routine in an emerging new Arctic. Nat. Clim. Chang. 10, 1108–1115. doi:10.1038/s41558-020-0892-z
Latimer, C. E., and Zuckerberg, B. (2019). How extreme is extreme? Demographic approaches inform the occurrence and ecological relevance of extreme events. Ecol. Monogr. 89, e01385. doi:10.1002/ecm.1385
Marchand, F., Mertens, S., Kockelbergh, F., Beyens, L., and Nijs, I. (2005). Performance of High Arctic tundra plants improved during but deteriorated after exposure to a simulated extreme temperature event. Glob. Chang. Biol. 11, 2078–2089. doi:10.1111/j.1365-2486.2005.01046.x
Maxwell, S. L., Butt, N., Maron, M., McAlpine, C. A., Chapman, S., Ullmann, A., et al. (2019). Conservation implications of ecological responses to extreme weather and climate events. Divers. Distrib. 25, 613–625. doi:10.1111/ddi.12878
McClintock, B. T., Langrock, R., Gimenez, O., Cam, E., Borchers, D. L., Glennie, R., et al. (2020). Uncovering ecological state dynamics with hidden Markov models. Ecol. Lett. 23, 1878–1903. doi:10.1111/ele.13610
McCrystall, M. R., Stroeve, J., Serreze, M., Forbes, B. C., and Screen, J. A. (2021). New climate models reveal faster and larger increases in Arctic precipitation than previously projected. Nat. Commun. 121 (12), 6765. doi:10.1038/s41467-021-27031-y
McPhillips, L. E., Chang, H., Chester, M. V., Depietri, Y., Friedman, E., Grimm, N. B., et al. (2018). Defining extreme events: A cross-disciplinary review. Earth's. Future 6, 441–455. doi:10.1002/2017EF000686
Metcalfe, D. B., G Hermans, T. D., Ahlstrand, J., Becker, M., Berggren, M., Björk, R. G., et al. (2018). Patchy field sampling biases understanding of climate change impacts across the Arctic. Nat. Ecol. Evol. 2, 1443–1448. doi:10.1038/s41559-018-0612-5
IPCC (2012). “Climate change: New dimensions in disaster risk, exposure, vulnerability, and resilience,” in Managing the risks of extreme events and disasters to advance climate change adaptation. Editors P. M. M. C. B. Field, V. Barros, T. F. Stocker, D. Qin, D. J. Dokkenet al. (Cambridge, UK, and New York, NY, USA): Cambridge University Press), 22–64.
Perkins-Kirkpatrick, S. E., and Lewis, S. C. (2020). Increasing trends in regional heatwaves. Nat. Commun. 11, 3357–3358. doi:10.1038/s41467-020-16970-7
Post, E., Alley, R. B., Christensen, T. R., Macias-Fauria, M., Forbes, B. C., Gooseff, M. N., et al. (2019). The polar regions in a 2°C warmer world. Sci. Adv. 5, eaaw9883. doi:10.1126/sciadv.aaw9883
Post, E., Brodie, J., Hebblewhite, M., Anders, A. D., Maier, J. A. K., and Wilmers, C. C. (2009a). Global population dynamics and hot spots of response to climate change. Bioscience 59, 489–497. doi:10.1525/bio.2009.59.6.7
Post, E., Forchhammer, M. C., Bret-Harte, M. S., Callaghan, T. V., Christensen, T. R., Elberling, B., et al. (2009b). Ecological dynamics across the arctic associated with recent climate change. Science 325, 1355–1358. doi:10.1126/science.1173113
Previdi, M., Smith, K. L., and Polvani, L. M. (2021). Arctic amplification of climate change: A review of underlying mechanisms. Environ. Res. Lett. 16, 093003. doi:10.1088/1748-9326/AC1C29
Putkonen, J., Grenfell, T. C., Rennert, K., Bitz, C., Jacobson, P., and Russell, D. (2009). Rain on snow: Little understood killer in the north. Eos Trans. AGU. 90, 221–222. doi:10.1029/2009EO260002
Raymond, C., Horton, R. M., Zscheischler, J., Martius, O., AghaKouchak, A., Balch, J., et al. (2020). Understanding and managing connected extreme events. Nat. Clim. Chang. 107 (10), 611–621. doi:10.1038/s41558-020-0790-4
Schmidt, N. M., Hardwick, B., Gilg, O., Høye, T. T., Krogh, P. H., Meltofte, H., et al. (2017). Interaction webs in arctic ecosystems: Determinants of arctic change? Ambio 46, 12–25. doi:10.1007/s13280-016-0862-x
Sillmann, J., Thorarinsdottir, T., Keenlyside, N., Schaller, N., Alexander, L. V., Hegerl, G., et al. (2017). Understanding, modeling and predicting weather and climate extremes: Challenges and opportunities. Weather Clim. Extrem. 18, 65–74. doi:10.1016/j.wace.2017.10.003
Sintayehu, D. W. (2018). Impact of climate change on biodiversity and associated key ecosystem services in africa: A systematic review. Ecosyst. Health Sustain. 4, 225–239. doi:10.1080/20964129.2018.1530054
Smith, M. D. (2011). An ecological perspective on extreme climatic events: A synthetic definition and framework to guide future research. J. Ecol. 99, 656–663. doi:10.1111/j.1365-2745.2011.01798.x
Stillman, R. A., Railsback, S. F., Giske, J., Berger, U., and Grimm, V. (2015). Making predictions in a changing world: The benefits of individual-based ecology. Bioscience 65, 140–150. doi:10.1093/biosci/biu192
Turner, M. G., Calder, W. J., Cumming, G. S., Hughes, T. P., Jentsch, A., LaDeau, S. L., et al. (2020). Climate change, ecosystems and abrupt change: Science priorities. Phil. Trans. R. Soc. B 375, 20190105. doi:10.1098/rstb.2019.0105
Tyler, N. J. C. (2010). Climate, snow, ice, crashes, and declines in populations of reindeer and caribou (Rangifer tarandus L.). Ecol. Monogr. 80, 197–219. doi:10.1890/09-1070.1
Ummenhofer, C. C., and Meehl, G. A. (2017). Extreme weather and climate events with ecological relevance: A review. Phil. Trans. R. Soc. B 372, 20160135. doi:10.1098/rstb.2016.0135
van de Pol, M., Jenouvrier, S., Cornelissen, J. H. C., and Visser, M. E. (2017). Behavioural, ecological and evolutionary responses to extreme climatic events: Challenges and directions. Phil. Trans. R. Soc. B 372, 20160134. doi:10.1098/rstb.2016.0134
Walsh, J. E., Ballinger, T. J., Euskirchen, E. S., Hanna, E., Mård, J., Overland, J. E., et al. (2020). Extreme weather and climate events in northern areas: A review. Earth. Sci. Rev. 209, 103324. doi:10.1016/j.earscirev.2020.103324
Weiskopf, S. R., Rubenstein, M. A., Crozier, L. G., Gaichas, S., Griffis, R., Halofsky, J. E., et al. (2020). Climate change effects on biodiversity, ecosystems, ecosystem services, and natural resource management in the United States. Sci. Total Environ. 733, 137782. doi:10.1016/j.scitotenv.2020.137782
Keywords: arctic environment, climate change, environmental monitoring, extreme event, terrestrial
Citation: van Beest FM, Barry T, Christensen T, Heiðmarsson S, McLennan D and Schmidt NM (2022) Extreme event impacts on terrestrial and freshwater biota in the arctic: A synthesis of knowledge and opportunities. Front. Environ. Sci. 10:983637. doi: 10.3389/fenvs.2022.983637
Received: 01 July 2022; Accepted: 29 August 2022;
Published: 13 September 2022.
Edited by:
Qiang Zhang, Beijing Normal University, ChinaReviewed by:
Li Wen, Industry and Environment, AustraliaCopyright © 2022 van Beest, Barry, Christensen, Heiðmarsson, McLennan and Schmidt. This is an open-access article distributed under the terms of the Creative Commons Attribution License (CC BY). The use, distribution or reproduction in other forums is permitted, provided the original author(s) and the copyright owner(s) are credited and that the original publication in this journal is cited, in accordance with accepted academic practice. No use, distribution or reproduction is permitted which does not comply with these terms.
*Correspondence: Floris M. van Beest, flbe@ecos.au.dk
†ORCID: Floris M. van Beest, orcid.org/0000-0002-5701-4927; Tom Barry, orcid.org/0000-0002-0633-3602; Tom Christensen, orcid.org/0000-0002-8125-6459; Starri Heiðmarsson, orcid.org/0000-0003-0329-6882; Niels M. Schmidt, orcid.org/0000-0002-4166-6218