- 1Institute of Hydrogeology and Environmental Geology, Chinese Academy of Geological Sciences, Shijiazhuang, Hebei, China
- 2Key Laboratory of Groundwater Contamination and Remediation of Hebei Province and China Geological Survey, Shijiazhuang, Hebei, China
- 3New Energy Geological Team of Hebei Coalfield Geological Bureau, Xingtai, Hebei, China
Wheat–maize rotation is one of the most popular systems and successful intensification cropping systems in Northern China, while soils in some of this area are contaminated by cadmium (Cd). However, few studies have performed experiments on the reduction of Cd accumulation in the wheat–maize rotation system. In this study, wheat- and maize-derived biochars are applied to the Cd-contaminated soil to reduce the Cd accumulation in the wheat and maize plants. The results showed that soil biochar applications can significantly decrease DTPA-extracted Cd concentrations by 12.7–26.0% and 13.1–20.5% by wheat- and maize-derived biochars, respectively. Sequential extractions showed that biochar applications significantly reduced the Cd mobility and bioavailability in soils and changed the exchangeable and carbonate-bound fractions of Cd to organic material-bound and residual fractions. The biochar applications increased the plant growth, yield, and quality of both wheat and maize, especially a significant increase in high dosages. The biochar applications also improved the antioxidant enzyme activities and reduced the reduction of MDA in both wheat and maize roots. The Cd concentrations in wheat grains were reduced by 38.1–91.5% and 65.9–80%, and maize grain Cd concentrations were reduced by 20.9–54.2% and 30.8–44% by wheat- and maize-derived biochar applications, respectively, and the Cd concentrations in the root, stem, and leaf were also significantly reduced. The wheat-derived biochar was more effective on the Cd reduction in soil bioavailable fractions and Cd accumulation in crop plants.
Introduction
Anthropogenic activities (e.g., non-ferrous/ferrous mining and smelting, biosolids, and manures, pesticides, and fertilizers) have released large amounts of heavy metals to the earth’s surface environment (e.g., pedosphere) over the past decades (Proshad et al., 2018; Jafarabadi et al., 2020). One of the widely concerned metals cadmium (Cd) is known to be widespread in the environment and toxic to humans and ecosystems (Bashir et al., 2020; Cui et al., 2020; Huang et al., 2021). Compared to most of the heavy metals, Cd shows relatively high mobility and toxicity, and crops can readily take up Cd from soils and transfer it into food chains, threatening human health (Du et al., 2020; Zhang et al., 2021). More than 50% of the world’s population subsists on wheat and maize as staple food (Nuss and Tanumihardjo, 2010; Pang et al., 2020). However, agricultural soils and crops contaminated with Cd are becoming a global concern, especially in eastern Asia. In China, Cd ranked first among all the pollutants with the sample points of 7% exceeding the tolerance limit (GB 15618-1995) (Wang et al., 2015). The serious soil pollution resulted in wheat samples collected from fields with Cd contamination exceeding the national food threshold (0.1 mg kg−1, GB 2762-2017) (Qu et al., 2020; Zhuang et al., 2021).
Exposure to Cd can lead to many chronic diseases, such as respiratory cancer, early atherosclerosis, and kidney damage (Fatima et al., 2019; Du et al., 2020). Reduction of staple Cd accumulation is the most effective way to reduce Cd exposure risk, as staple food is the dominant pathway of the daily Cd intake and becomes the major source of Cd exposure (Li et al., 2021; Zhuang et al., 2023). Many strategies have been applied to reduce wheat and corn Cd accumulation in Cd-contaminated soils, but most of them have a high technical barrier or environmental hazard (Wang L. et al., 2021; Cao et al., 2021). Biochar is pyrolyzed by thermal decomposition of raw biomass at a wide range of temperatures under the condition of hypoxia (Thengane and Bandyopadhyay, 2020; Siddique et al., 2021). Biochar is widely studied and applied to heavy-metal-contaminated soils as it has a large amount of microporous structure, high specific surface area, and organic functional groups, which can potentially regulate the soil pH and structure and decrease the mobilization of soil heavy metals via adsorption and precipitation (Cui et al., 2021). Additionally, the raw biomass and temperature during pyrolysis can also influence the performance of heavy metal immobilization, and their immobilization mechanisms also varied from each other (Inyang et al., 2016).
Recent studies showed that applications of biochar to soils could effectively immobilize Cd in soils and reduce Cd accumulation in crops, such as rice, rice–rape–corn rotation, wheat, and lettuce (Abbas et al., 2017; Zheng et al., 2017; Qu et al., 2020; Zong et al., 2021). Generally, a large number of studies are available for lab incubation experiments and rice Cd accumulation experiments where biochar can effectively immobilize and reduce Cd accumulation in rice from contaminated acid soils (Zheng et al., 2017; Bashir et al., 2020; Cui et al., 2021). Wheat–maize rotation is one of the most popular systems and successful intensification cropping systems in Northern China, which has produced about 45 and 30% of the national wheat and maize, respectively (Kamaruzzaman et al., 2021). Additionally, soil, maize, and wheat Cd contaminations have been reported in these areas (Zhuang et al., 2021; Zhuang et al., 2023); however, few studies have performed experiments on the reduction of Cd concentrations in the wheat–maize rotation system. In the current study, the goals of the pot experiment in the greenhouse were: 1) to evaluate the ability of wheat/maize-straw biochar on the reduction of the Cd bioaccumulation from soils under the wheat–maize rotation system; and 2) to explore the biochar impact on the soil Cd chemical fractions and the translocation of soil bioavailable Cd to the wheat and maize plants.
Materials and methods
Soil and biochar preparation
Pot soil samples were collected from the plow layer of a wheat–maize rotation field in the sewage irrigated area of Xiaohe, Shijiazhuang City, China, which is alkaline sandy loam. The polluted soils were collected using a stainless steel shovel at various locations and then pooled, ground thoroughly, and removed plant residue and scree. The collected soils were dried in the greenhouse and then grounded to less than 2 mm. Other basic properties of the soil like pH, soil organic matter (SOC), and cation exchange capacity (CEC) were measured as 7.9, 1.47%, and 23.2 cmol kg−1, respectively; total N and alkali-hydrolyzed N concentrations were 880 and 84.8 mg kg−1, respectively; total P and available P concentrations were 22.4 and 1.47 mg kg−1, respectively. Because the experiment soils were located downstream of the industrial zone, the mean concentration was 1.45 ± 0.13 mg kg−1. The soil was polluted by Cd based on the soil environment quality risk control standard (GB 15618–2018, pH > 7.5, 0.6 mg kg−1).
Wheat and maize straws were washed with tap water to remove impurities, air-dried to constant weight, and then cut into particle sizes (<1 cm). The straw pieces were pyrolyzed in a ceramic crucible at 500°C in a muffle furnace for 3 h blowing with N2 (0.5 dm3 min−1). Then, the biochar was cooled down, sifted through a 50-mesh sieve, and stored in a clean bag for the pot experiment.
Experimental design
The experiment was conducted in the greenhouse including three treatments at two levels (low dosage (L) and high dosage (H)): 1) control treatment (CK), 2) wheat stalk biochar (WB-L and WB-H), and 3) maize straw biochar (MB-L and MB-H). Totally, five treatments were performed with environmental triplicate. Initially, 10 kg of the contaminated soil was placed in each ceramic pot, and the prepared biochar was added at 1 and 2% levels as low dosage (L) and high dosage (H), respectively. After 2 weeks, the biochar thoroughly mixed and balanced with the soil, and fifteen wheat seeds were sown in. During the seedling stage, wheat seedlings were thinned to five per pot. Then, the wheat plants were harvested at the mature stage. Sequentially, two maize seedlings per pot were planted in the same soils. The nutrition solution containing N, P, and K was also applied at the seeding stage of the two crops.
Soil and plant sample collection and analysis
During the filling stage, the wheat and maize roots were collected to measure the activities of the antioxidant enzymes and malondialdehyde (MDA). The activities of superoxide dismutase (SOD), axial tomography (CAT), peroxidase (POD), and MDA were determined spectrophotometrically, which were detailed in the previous study (Wu et al., 2020). Briefly, MDA activities were determined using 0.1% thiobarbituric acid. Antioxidant enzyme (SOD, POD, CAT, and APX) activities were determined spectrophotometrically. Fresh wheat root was ground into small pieces in liquid nitrogen for the determination of antioxidant enzyme activities. Phosphate buffer (0.05 M) with a pH of 7.8 was used to standardize the samples and centrifuged at 4 °C for 10 min (12,000 r). The supernatants were transferred for further analysis.
In the mature stages, whole wheat and maize plants were collected and separated into root, stem, leaf, and grains in the laboratory. All the tissues were cleaned with tap water, rinsed with Milli-Q water, and dried in the oven at 90°C for 0.5 h and then at 60°C to constant weight. Each pot soil was mixed thoroughly and about 500 g was collected after wheat and maize harvest. All the samples were milled through 100-mesh using a coffee grinder. For Cd measurement, the plant samples were digested by an acid mixture of HNO3: HClO4 (v: v = 5: 1) in a conical flask and kept overnight. On the following day, the conical flasks were placed on an electric hot plate at 80°C for 2 h, gradually increased to 120°C for 2 h, and then kept at 160°C. The soil samples were digested with an acid mixture of HNO3, HClO4, and HF. Bioavailable Cd was extracted with 0.005 M DTPA at pH 7.3 and shaken for 2 h. The sequential extraction was conducted based on the approach performed by Tessier’s method (Tessier et al., 1980). Five fractions of Cd were chemically extracted, i.e., an exchangeable fraction (EX), a carbonate-bound fraction (CB), Fe-Mn oxide-bound (OX), organic material-bound (OM), and a residual fraction (RE). The digested and extracted solutions were filtered through a 0.45-μM membrane into centrifuge tubes. The Cd concentrations were measured using ICP-MS (NexION 2000, Perkin Elmer, United States). Quality assurance and quality control during the measurement were conducted with triplicate, blanks, matrix spikes, and standard materials (GBW10020 (GSB−11) and GBW07427 (GSS-13)). The recoveries of Cd in soil and plant standard materials were in the range of 89–103% and 96–108%, respectively. The soil and biochar properties were measured according to the previous study by Zong et al. (2021).
Data analysis
The translocation factor (TF) and bioconcentration factors (BCFs) of wheat and maize are calculated using the following equations (Zhou et al., 2020):
where m and n are the plant upper and lower parts and Csoil is the Cd concentrations in the soil.
Statistical significance was determined by one-way ANOVA using SPSS 22.0, and all variations in means were significant at the p = 0.05 level (two-tailed). All figures were plotted by Origin 2021 software.
Results
Wheat and maize yield
The biochar application significantly increased soil pH after the harvest of wheat. The variations in soil pH were relatively proportionable to the dosage of biochar. Soil pH significantly increased by 3.65–4.6% for wheat-biochar treatments and by 3.9–5.3% for maize-biochar treatments after wheat harvest compared to CK, respectively. After maize harvest, we observed similar results. Soil organic carbon was also notably enhanced by the biochar applications during the wheat–maize rotation system, especially at high biochar applications, indicating that biochar applications have potential effects on the soil improvement of its low fertility.
Compared to the control treatment, the biochar applications increased plant growth, yield, and quality of both wheat and maize (Figure 1). For example, WB-H and MB-H significantly increased the grain yield and grain number per spike for both wheat and maize (p < 0.05). The plant height and 100-grain weight of wheat was also significantly increased by the high dosage of biochar implications (p < 0.05), but not for maize (p > 0.05).
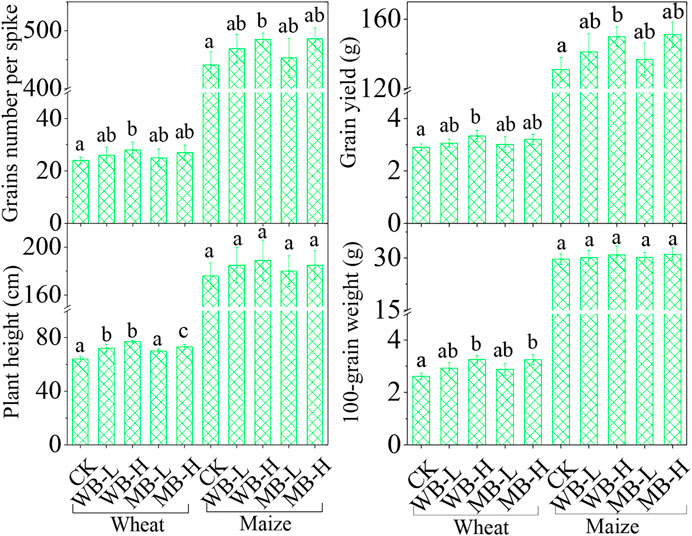
FIGURE 1. Effect of biochar on grain number per spike, grain yield, plant height, and 100-grain weight of wheat and maize.
Soil cadmium mobility
The results of DTPA-extracted Cd concentrations in the soils of wheat and maize are shown in Figure 2A. In the CK treatment, DTPA-extracted Cd concentrations were 0.47 and 0.48 mg kg− 1 in the soils after wheat and maize harvest, respectively. Biochar addition significantly reduced the DTPA-extracted Cd fraction at both low and high dosage treatments (p < 0.05). The DTPA-extracted Cd concentrations significantly decreased to 0.37–0.42 and 0.39–0.41 mg kg−1 in the treatments of WB-H and MB-H in wheat–maize rotation soils, respectively.
The results showed that the DTPA-extracted Cd concentrations were reduced by 15.6, 26.0, 17.3, and 20.5% with WB-L, WB-H, MB-L, and MB-H treatments, respectively, in wheat-harvested soils. The DTPA-extracted Cd concentrations were reduced by 12.7, 21.7, 13.1, and 16.5% in treatments of WB-L, WB-H, MB-L, and MB-H of maize-harvested soils. It followed that soil DTPA-extracted Cd was more reduced but not significantly in the high dosage treatment than that in the low dosage treatment (p > 0.05). Additionally, more DTPA-extracted Cd was reduced compared to that in MB-treated soils during the wheat–maize rotation. These results suggest that biochar applications can significantly decrease the DTP-extracted Cd concentrations in soils compared to the CK treatment.
Soil cadmium chemical fractions
Sequential extractions were conducted to explore the biochar application impact on Cd fractions in wheat–maize rotation soils (Figure 2B). In CK, Cd was mainly in RE, CB, OM, and EX and the lowest in the OX. The results display that high dosage of biochar applications was more effective than the lower dosage in the reduction of the fractions of EX and CB and increasing OM and RE fractions of Cd. In the wheat growing season, the EX fractions decreased considerably to 9.3 and 10.3% at the low dosage and 6.3 and 8.2% at the high dosage of biochar applications compared to that of 13.0% in the control treatment. CB fractions of Cd were also reduced with biochar applications, while the OX fractions had no significant reduction. In the maize growing season, the EX Cd decreased considerably to 9.7 and 9.9% at the low dosage and 7.6 and 8.0% at the high dosage of biochar applications compared to that of 11.6% in the control treatment. CB fractions of Cd were also reduced with biochar applications, while the OX fractions had no significant reduction. The OM and RE fractions significantly increased to 19.2–25.9% and 49.9–56.0% compared to those of 17.7 and 46.3% in the control treatment. Therefore, biochar applications changed EX and CB fractions of Cd to OM and RE fractions. These results indicate that the soil Cd bioavailability was reduced by biochar applications, which would reduce the Cd uptake risks by plants. Sequential extractions suggested that biochar applications significantly influence the distributions of soil Cd fractions.
Plant activities of the antioxidant enzymes and malondialdehyde
To explore the effects of wheat and maize biochar on the antioxidant system in Cd-stressed wheat and maize, MDA, CAT, SOD, and POD activities in the wheat and maize roots after biochar applications were measured and shown in Figure 3. For wheat, the MDA activities were significantly reduced with high dosage of both WB and MB applications by 28.0 and 20.1% (p < 0.05), respectively, and for maize, high dosages also significantly decreased the MDA activities by 31.3 and 27.6% (p < 0.05); however, there were no significant differences between the low dosage of biochar applications compared to control treatments of both wheat and maize (p > 0.05). The high dosages of both wheat- and maize biochar (WB-H and MB-H) significantly increased the CAT and POD activities in wheat compared to the control treatment by 15.6–18.2% and 12.5–15.7% (p < 0.05) and also significantly increased these activities in maize by 18.5–19.0% and 23.4–24.6% (p < 0.05), respectively. However, the low dosage had no effects on the CAT and POD activities in both wheat and maize (p > 0.05). Additionally, both wheat and maize biochar at low and high dosages can also significantly increase the SOD activities compared to control treatments for both wheat and maize, particularly at the high dosages that can increase the averaged 168 and 36.8% of SOD activities in wheat and maize, respectively. Our results indicate that biochar applications can improve the antioxidant enzyme activities and reduce MDA in both wheat and maize roots.
Cadmium concentrations in wheat and maize
The effects of biochar on the Cd accumulations in wheat and maize are shown in Figure 4. In CK, wheat grain Cd concentration was 0.21 mg kg−1, which was about 1.1 times higher than the safe limit value of the National Food Safety Standard (0.1 mg kg−1) (GB 2762, 2017), but the Cd concentration in the maize grain (0.091 mg kg−1) was under the limit. Both wheat and maize roots depicted the highest Cd concentration (1.12 and 0.98 mg kg−1) among other plant parts, displaying the following order: root > stem > leaf > grain. The biochar applications can significantly decrease all the Cd concentrations in wheat and maize tissues at both levels of biochar dosages (p < 0.05). For example, the Cd concentrations in wheat grains were reduced by 38.1–91.5% and 65.9–80.0% by WB and MB applications, and maize grain Cd concentrations were reduced by 20.9–54.2% and 30.8–44% by WB and MB applications. For roots, both biochars significantly decreased the Cd concentrations by 24.1–62.4% in wheat and 23.5–42.9% in maize. Leaf Cd concentrations were also significantly reduced by 32.3–71.4% and 17.4–36.8% for wheat and maize, respectively, and stem Cd concentrations were reduced by 32.1–80.6% and 15.6–34.2%, respectively.
Discussion
Mechanisms of soil cadmium immobilization by biochar
Biochar applications can effectively decrease the bioavailability and immobilize the Cd in contaminated soil in the wheat–maize rotation system. The important phenomenon reducing bioavailability and mobility of soil Cd after biochar applications is the availability of cations (Ca2+, Mg2+, K+, and Zn2+) and nutrients (nitrogen, silicon, and phosphorus). Some studies showed that the biochar applications bring large amounts of nutrients into contaminated soils (Kim et al., 2015; Xia et al., 2021; Gong et al., 2022). Additionally, high specific surface area and presence of negative charges decrease the bioavailability by the electrostatic interaction, increase the presence of organic functional groups, and form the sulfhydryl and phosphate precipitation (Cui et al., 2021; Yang et al., 2021; Gong et al., 2022).
In general, soil pH plays a role in heavy-metal speciation, mobility, and bioavailability in polluted soils because most of the metals are available in soils with acidic pH values. Biochar is normally alkaline, and our results confirmed that biochar applications can significantly increase soil pH with increasing application rates (Table 1). This enhancement of pH neutralized the free-state H+ and thus in turn increased the hydrolysis of Cd, increased its immobilization by soil and the biochar in soils, and accelerated the transformation of exchangeable fractions to the organic-bound and residual Cd fractions (Gong et al., 2022). This phenomenon was also observed in this study that showed biochar applications had significant effects on the transformation of EX and CB Cd fractions to OM and RE Cd fractions (Figure 2). The enhancement of pH can also strengthen Cd complexation, reducing the desorption of Cd2+ from soils (Xiang et al., 2021). Furthermore, decreased hydrogen ions can reduce the production of Cd solubility products and thus reduce the Cd bioavailability.
Large specific surface area, high micro-porous structure, and abundant large porosity distribution of biochar promote soil to contain the biochar in soil pores. The large specific surface area and high micro-porous structure also contained high electronegativity that can promote the electrostatic adsorption of positively charged ions (Inyang et al., 2016; Guo et al., 2020). The adsorption strength is dependent on the negatively charged groups on the biochar surface, that is, increasingly negative with the increase of pH values (He et al., 2019). Soil pH increased by biochar applications would also facilitate the electrostatic adsorption of Cd in this study. Additionally, in general, biochar contains high CEC and can release large amounts of cations, such as Ca2+, Mg2+, K+, and Zn2+, which can promote the cationic exchange with soil solution heavy metals on the biochar surface (Xu et al., 2016). Thus, the biochars can increase their adsorption capability for heavy metals, for e.g., Cd, Pb, and Cu (Li et al., 2017; Wu et al., 2021). A previous study by Lei et al. (2019) found that the animal-derived biochars contained about 27% higher Ca than plant-derived biochar, so ion exchanges were regarded as the dominant pathway of animal-derived biochars during the adsorption of heavy-metal ions.
The surface functional groups of biochars can also immobilize metal ions through ion exchange processes and surface complexation, particularly for biochars with relatively low mineral concentrations (Uchimiya et al., 2010; He et al., 2019). Previous studies suggested that crop straw-made biochars mainly immobilize heavy metals through the surface-complexation formation (Jiang and Xu, 2013; Lahori et al., 2017). The functional groups, for e.g., phenolic –OH, –COOH, –C=O–, and –C=N, largely exist in biochars, providing binding sites to form complexes, which increase the adsorption and immobilization for Cd (Uchimiya, 2014; Zong et al., 2021). Soil heavy metals adsorbed by biochar functional groups are regarded as the key mechanism in heavy-metal immobilization (Sun et al., 2021). Additionally, iron and manganese oxides and inorganic ions such as Si, Se, S, and Cl contained in biochar can complex with heavy metals, (e.g., Cd2+), reducing the mobility and bioavailability in soils (Lahori et al., 2017). A previous study suggested that the main reason of soil DTPA-extractable Cu reduction was from the complexation of functional groups with Cu in the soils (Wang Y. et al., 2021). Furthermore, precipitation between the mineral elements in biochar and soil heavy metals was considered another mechanism of Cd immobilization of heavy metals, especially with biochar contained with mineral elements (e.g., P, S, and Se). For instance, the biochar produced by impregnation of P with the pyrolysis of bamboo can enhance the Cd absorption capacity by 10 times, and the solution Cd removal efficiency increased by 85% (Zhang et al., 2018).
Effects of biochars on the cadmium reduction in plants
Our results suggested that the soil applications of wheat- and maize-derived biochars can improve both wheat and maize growth and yield (Figure 1). Reduced grain yield, grain number per spike, plant height, and 100-grain weight of wheat and corn would result from the toxic effects induced by Cd, as previous studies found that Cd is toxic to plants, causing nutrient loss, reduction of antioxidant enzyme activities, and damage to photosynthesis in plants (Anwar et al., 2019; Wu et al., 2020; Zhou et al., 2020; Liu et al., 2021; Zhou et al., 2021; Li et al., 2022). It is also reported that Cd can also produce oxidative stress via high binding capacity for oxygen, nitrogen, and sulfur atoms, which resulted in reactive oxygen species (ROS) accumulation (Anwar et al., 2019). In this study, wheat and maize roots had the highest Cd concentrations in the control treatments, whereas wheat- and maize-derived biochar applications decreased their levels, as shown in Figure 3. The increased ROS is from higher Cd concentration and toxicity in wheat and maize roots in the control treatment as oxidative stress induced by Cd was well elucidated in wheat (Kaya et al., 2020), maize (Alharby et al., 2021), and other plants (Dabral et al., 2019; Gao et al., 2020). MDA contents reduced by biochar applications would be due to the reduction of Cd concentrations in wheat and maize tissues (Figures 3, 4). Previous studies also found that biochar applications can not only decrease oxidative stress in other plant species cultivated in Cd-contaminated soils but also reduce the oxidative stress by other heavy metals (Hafeez et al., 2019; Yue et al., 2019; Qu et al., 2020). Furthermore, biochar applications can also decrease the oxidative stress of wheat and maize in this study (Figure 3) and some other plants under the stresses of heavy metals in previous studies (Abbas et al., 2017; Hafeez et al., 2019; Alharby et al., 2021). For example, rice-straw biochar application elevated SOD, CAT, and POD by 18, 958, and 27%, respectively, in Brassica napus L. planted in the Cd-contaminated soils (Lei et al., 2022). Our results suggested that the enhancement of the enzyme activities resulted from decreased Cd concentrations in wheat and maize tissues and increased the plant tolerance to soil Cd stresses, thus improving the plant growth (Figures 1, 4).
The effects of biochar applications on TFs and BAFs in wheat and maize are shown in Table 2. Biochar applications significantly reduced BAFs, particularly in both wheat- and maize-derived biochar at high application rates. Additionally, the biochar applications also significantly decrease TFs from root to stems and grains (TFS/R and TFG/R) and also increase Cd in stem translocation to leaves in the high dosage treatments of wheat. More Cd transported to the leaf from the stem would be due to the wheat node allocation of Cd in the stem as previous studies suggest that node can allocate heavy metals and nutrient ions to grains and leaves (Feng et al., 2021). Additionally, the biochar applications would enhance the photosynthetic physiology (Shahbaz et al., 2019), which can also promote Cd in the stem transporting to the leaf. Our results agree with other findings that high dosages of biochars can decrease the Cd transport from roots to shoots (Zong et al., 2021). The Cd translocation from underground tissues to aboveground tissues is regarded as one of the most important factors in determining the accumulations of heavy metals in plants (Wang et al., 2020). In this study, regression analysis showed that the Cd concentrations in wheat and maize tissues (root, stem, leaf, and grain) were significantly correlated with bioavailable Cd (DTPA-extracted and exchangeable Cd) (Table 3), which indicates that the soil Cd bioavailability is directly contributed to the Cd accumulation in these crops. Therefore, reduction of bioavailable Cd concentrations in the soils is the most effective approach to reduce the soil Cd migration to crops and control food safety. Zong et al. (2021) also found that crop straw-derived biochar induced the Cd reduction in the rice–rape–corn rotation system that was notably correlated with the decreased DTPA extracted in soils. Additionally, high cation (e.g., Ca2+, Mg2+, K+, and Zn2+) concentration in biochar would be another reason for the reduction of Cd accumulation in wheat and maize tissues. These cautions can complete with Cd during the root Cd uptake and thus reduce the Cd uptake by crop roots (Xu et al., 2016).

TABLE 3. Regression relationship between DTPA-extracted and exchangeable Cd in soils and Cd concentrations in wheat and maize tissues.
Conclusion
Wheat straw- and maize straw-derived biochar applications to the Cd-contaminated soils can significantly decrease DTPA-extracted Cd concentrations by 12.7–26.0% and 13.1–20.5%, respectively, and WB applications can reduce more DTPA-extracted Cd in soils than MB applications during the wheat–maize rotation. Sequential extractions suggested that biochar applications can decrease the Cd bioavailability in biochar-amended soils and changed the EX and CB fractions of Cd to OM and RE fractions. For wheat and maize, the biochar applications increased the plant growth, yield, and quality of both wheat and maize, especially a significant increase in high dosages can also improve the antioxidant enzyme activities and reduce the reduction of MDA in both wheat and maize roots. The Cd concentrations in the wheat grains were reduced by 38.1–91.5% and 65.9–80% under WB and MB applications, and maize grain Cd concentrations were reduced by 20.9–54.2% and 30.8–44.0% under WB and MB applications, respectively. Cd concentrations in the root, stem, and leaf are also significantly reduced under WB and MB applications.
Although biochar applications can significantly reduce the Cd accumulation in wheat and maize grains, more effective approaches are necessary to qualify relevant transporters and provide useful information for breeding in the future. Additionally, mitigation strategies are in development, and short-term and long-term solutions with cost-effectiveness are needed to cope with the current Cd-contaminated paddy fields and Cd accumulation in rice grains. Moreover, long-term field trials are highly desirable to examine the residual effects of biochar amendments on different soil types and environmental conditions.
Data availability statement
The original contributions presented in the study are included in the article/Supplementary Material; further inquiries can be directed to the corresponding author.
Author contributions
YD: collected and analyzed samples, organized data, and wrote the manuscript; CW: designed experiments and wrote and revised the manuscript; MZ: collected and analyzed samples; XT: designed experiments; GW: collected samples.
Funding
This research was supported by the National Key R and D Program of China (2019YFC1805300).
Conflict of interest
Author MZ was employed by the company New Energy Geological Team of Hebei Coalfield Geological Bureau.
The remaining authors declare that the research was conducted in the absence of any commercial or financial relationships that could be construed as a potential conflict of interest.
Publisher’s note
All claims expressed in this article are solely those of the authors and do not necessarily represent those of their affiliated organizations, or those of the publisher, the editors, and the reviewers. Any product that may be evaluated in this article, or claim that may be made by its manufacturer, is not guaranteed or endorsed by the publisher.
References
Abbas, T., Rizwan, M., Ali, S., Zia-ur-Rehman, M., Qayyum, M. F., Abbas, F., et al. (2017). Effect of biochar on cadmium bioavailability and uptake in wheat (Triticum aestivum L.) grown in a soil with aged contamination. Ecotoxicol. Environ. Saf. 140, 37–47. doi:10.1016/j.ecoenv.2017.02.028
Alharby, H. F., Al-Zahrani, H. S., Hakeem, K. R., Alsamadany, H., Desoky, E.-S. M., Rady, M. M., et al. (2021). Silymarin-enriched biostimulant foliar application minimizes the toxicity of cadmium in maize by suppressing oxidative stress and elevating antioxidant gene expression. Biomolecules 11 (3), 465. doi:10.3390/biom11030465
Anwar, S., Khan, S., Hussain, I., Bashir, R., and Fahad, S. (2019). Chelators induced uptake of cadmium and modulation of water relation, antioxidants, and photosynthetic traits of maize. Environ. Sci. Pollut. Res. 26 (17), 17577–17590. doi:10.1007/s11356-019-05170-6
Bashir, S., Hussain, Q., Jun, Z., Qingling, F., Houben, D., and Hongqing, H. (2020). Efficiency of KOH-modified rice straw-derived biochar for reducing cadmium mobility, bioaccessibility and bioavailability risk index in red soil. Pedosphere 30 (6), 874–882. doi:10.1016/s1002-0160(20)60043-1
Cao, X., Gao, X., Zeng, X., Ma, Y., Gao, Y., Baeyens, W., et al. (2021). Seeking for an optimal strategy to avoid arsenic and cadmium over-accumulation in crops: Soil management vs cultivar selection in a case study with maize. Chemosphere 272, 129891. doi:10.1016/j.chemosphere.2021.129891
Cui, H., Li, H., Zhang, S., Yi, Q., Zhou, J., Fang, G., et al. (2020). Bioavailability and mobility of copper and cadmium in polluted soil after phytostabilization using different plants aided by limestone. Chemosphere 242, 125252. doi:10.1016/j.chemosphere.2019.125252
Cui, H., Wang, Q., Zhang, X., Zhang, S., Zhou, J., Zhou, D., et al. (2021). Aging reduces the bioavailability of copper and cadmium in soil immobilized by biochars with various concentrations of endogenous metals. Sci. Total Environ. 797, 149136. doi:10.1016/j.scitotenv.2021.149136
Dabral, S., Yashaswee, Varma, A., Choudhary, D. K., Bahuguna, R. N., and Nath, M. (2019). Biopriming with Piriformospora indica ameliorates cadmium stress in rice by lowering oxidative stress and cell death in root cells. Ecotoxicol. Environ. Saf. 186, 109741. doi:10.1016/j.ecoenv.2019.109741
Du, B., Zhou, J., Lu, B., Zhang, C., Li, D., Zhou, J., et al. (2020). Environmental and human health risks from cadmium exposure near an active lead-zinc mine and a copper smelter, China. Sci. Total Environ. 720, 137585. doi:10.1016/j.scitotenv.2020.137585
Fatima, G., Raza, A. M., Hadi, N., Nigam, N., and Mahdi, A. A. (2019). Cadmium in human diseases: it’s more than just a mere metal. Indian J. Clin. biochem. 34 (4), 371–378. doi:10.1007/s12291-019-00839-8
Feng, J., Shen, R. F., and Shao, J. F. (2021). Transport of cadmium from soil to grain in cereal crops: A review. Pedosphere 31 (1), 3–10. doi:10.1016/s1002-0160(20)60015-7
Gao, M., Chang, X., Yang, Y., and Song, Z. (2020). Foliar graphene oxide treatment increases photosynthetic capacity and reduces oxidative stress in cadmium-stressed lettuce. Plant Physiology Biochem. 154, 287–294. doi:10.1016/j.plaphy.2020.06.021
Gong, H., Zhao, L., Rui, X., Hu, J., and Zhu, N. (2022). A review of pristine and modified biochar immobilizing typical heavy metals in soil: Applications and challenges. J. Hazard. Mater. 432, 128668. doi:10.1016/j.jhazmat.2022.128668
Guo, M., Song, W., and Tian, J. (2020). Biochar-facilitated soil remediation: Mechanisms and efficacy variations. Front. Environ. Sci. 183, 521512. doi:10.3389/fenvs.2020.521512
Hafeez, F., Rizwan, M., Saqib, M., Yasmeen, T., Ali, S., Abbas, T., et al. (2019). Residual effect of biochar on growth, antioxidant defence and cadmium (cd) accumulation in rice in a cd contaminated saline soil. Pak. J. Agric. Sci. 56 (1), 197–204. doi:10.21162/pakjas/19.7546
He, L., Zhong, H., Liu, G., Dai, Z., Brookes, P. C., Xu, J., et al. (2019). Remediation of heavy metal contaminated soils by biochar: Mechanisms, potential risks and applications in China. Environ. Pollut. 252, 846–855. doi:10.1016/j.envpol.2019.05.151
Huang, X., Yu, H., Zhao, X., Guo, X., Ye, Y., and Xu, Z. (2021). Spatial variation in cadmium and mercury and factors influencing their potential ecological risks in farmland soil in poyang lake plain, China. Front. Environ. Sci. 9, 641497. doi:10.3389/fenvs.2021.641497
Inyang, M. I., Gao, B., Yao, Y., Xue, Y., Zimmerman, A., Mosa, A., et al. (2016). A review of biochar as a low-cost adsorbent for aqueous heavy metal removal. Crit. Rev. Environ. Sci. Technol. 46 (4), 406–433. doi:10.1080/10643389.2015.1096880
Jafarabadi, A. R., Mitra, S., Raudonytė-Svirbutavičienė, E., and Bakhtiari, A. R. (2020). Large-scale evaluation of deposition, bioavailability and ecological risks of the potentially toxic metals in the sediment cores of the hotspot coral reef ecosystems (Persian Gulf, Iran). J. Hazard. Mater. 400, 122988. doi:10.1016/j.jhazmat.2020.122988
Jiang, J., and Xu, R.-k. (2013). Application of crop straw derived biochars to Cu (II) contaminated ultisol: Evaluating role of alkali and organic functional groups in Cu (II) immobilization. Bioresour. Technol. 133, 537–545. doi:10.1016/j.biortech.2013.01.161
Kamaruzzaman, M., Wang, Z., Wu, M., Yang, L., Han, Y., Li, G., et al. (2021). Promotion of tomato growth by the volatiles produced by the hypovirulent strain QT5-19 of the plant gray mold fungus Botrytis cinerea. Microbiol. Res. 247, 126731. doi:10.1016/j.micres.2021.126731
Kaya, C., Ashraf, M., Alyemeni, M. N., and Ahmad, P. (2020). Responses of nitric oxide and hydrogen sulfide in regulating oxidative defence system in wheat plants grown under cadmium stress. Physiol. Plant. 168 (2), 345–360. doi:10.1111/ppl.13012
Kim, H.-S., Kim, K.-R., Kim, H.-J., Yoon, J.-H., Yang, J. E., Ok, Y. S., et al. (2015). Effect of biochar on heavy metal immobilization and uptake by lettuce (Lactuca sativa L.) in agricultural soil. Environ. Earth Sci. 74 (2), 1249–1259. doi:10.1007/s12665-015-4116-1
Lahori, A. H., Zhang, Z., Guo, Z., Li, R., Mahar, A., Awasthi, M. K., et al. (2017). Beneficial effects of tobacco biochar combined with mineral additives on (im) mobilization and (bio) availability of Pb, Cd, Cu and Zn from Pb/Zn smelter contaminated soils. Ecotoxicol. Environ. Saf. 145, 528–538. doi:10.1016/j.ecoenv.2017.07.071
Lei, M., Li, Z., Zhang, B., Wang, X., Tie, B., Ayaz, T., et al. (2022). Mechanisms of stress alleviation after lime and biochar applications for Brassica napus L. In cadmium-contaminated soil. Adsorpt. Sci. Technol. 2022, 4195119. doi:10.1155/2022/4195119
Lei, S., Shi, Y., Qiu, Y., Che, L., and Xue, C. (2019). Performance and mechanisms of emerging animal-derived biochars for immobilization of heavy metals. Sci. Total Environ. 646, 1281–1289. doi:10.1016/j.scitotenv.2018.07.374
Li, H., Dong, X., da Silva, E. B., de Oliveira, L. M., Chen, Y., and Ma, L. Q. (2017). Mechanisms of metal sorption by biochars: Biochar characteristics and modifications. Chemosphere 178, 466–478. doi:10.1016/j.chemosphere.2017.03.072
Li, W., He, X., Zhang, J., and Liu, K. (2022). Determination of soil cadmium threshold for potato. Front. Environ. Sci. 10, 808362. doi:10.3389/fenvs.2022.808362
Li, Y., Chen, W., Yang, Y., Wang, T., and Dai, Y. (2021). Quantifying source-specific intake risks of wheat cadmium by associating source contributions of soil cadmium with human health risk. Ecotoxicol. Environ. Saf. 228, 112982. doi:10.1016/j.ecoenv.2021.112982
Liu, K., Liu, H., Zhou, X., Chen, Z., and Wang, X. (2021). Prediction of cadmium transfer from soil to potato in karst soils, China. Front. Environ. Sci. 9, 684887. doi:10.3389/fenvs.2021.684887
Nuss, E. T., and Tanumihardjo, S. A. (2010). Maize: A paramount staple crop in the context of global nutrition. Compr. Rev. food Sci. food Saf. 9 (4), 417–436. doi:10.1111/j.1541-4337.2010.00117.x
Pang, Y., Liu, C., Wang, D., Amand, P. S., Bernardo, A., Li, W., et al. (2020). High-resolution genome-wide association study identifies genomic regions and candidate genes for important agronomic traits in wheat. Mol. Plant 13 (9), 1311–1327. doi:10.1016/j.molp.2020.07.008
Proshad, R., Kormoker, T., Mursheed, N., Islam, M. M., Bhuyan, M. I., Islam, M. S., et al. (2018). Heavy metal toxicity in agricultural soil due to rapid industrialization in Bangladesh: A review. Int. J. Adv. Geosciences 6 (1), 83. doi:10.14419/ijag.v6i1.9174
Qu, M., Chen, J., Huang, B., and Zhao, Y. (2020). Exploring the spatially varying relationships between cadmium accumulations and the main influential factors in the rice-wheat rotation system in a large-scale area. Sci. Total Environ. 736, 139565. doi:10.1016/j.scitotenv.2020.139565
Shahbaz, A. K., Ramzani, P. M. A., Saeed, R., Turan, V., Iqbal, M., Lewińska, K., et al. (2019). Effects of biochar and zeolite soil amendments with foliar proline spray on nickel immobilization, nutritional quality and nickel concentrations in wheat. Ecotoxicol. Environ. Saf. 173, 182–191. doi:10.1016/j.ecoenv.2019.02.025
Siddique, A. B., Rahman, M. M., Islam, M. R., Mondal, D., and Naidu, R. (2021). Response of iron and cadmium on yield and yield components of rice and translocation in grain: Health risk estimation. Front. Environ. Sci. 9, 716770. doi:10.3389/fenvs.2021.716770
Sun, T., Xu, Y., Sun, Y., Wang, L., Liang, X., Jia, H., et al. (2021). Crayfish shell biochar for the mitigation of Pb contaminated water and soil: Characteristics, mechanisms, and applications. Environ. Pollut. 271, 116308. doi:10.1016/j.envpol.2020.116308
Tessier, A., Campbell, P., and Bisson, M. (1980). Trace metal speciation in the yamaska and st. Francois rivers (quebec). Can. J. Earth Sci. 17 (1), 90–105. doi:10.1139/e80-008
Thengane, S. K., and Bandyopadhyay, S. (2020). Biochar mines: Panacea to climate change and energy crisis? Clean. Technol. Environ. Policy 22 (1), 5–10. doi:10.1007/s10098-019-01790-1
Uchimiya, M. (2014). Influence of pH, ionic strength, and multidentate ligand on the interaction of CdII with biochars. ACS Sustain. Chem. Eng. 2 (8), 2019–2027. doi:10.1021/sc5002269
Uchimiya, M., Lima, I. M., Thomas Klasson, K., Chang, S., Wartelle, L. H., and Rodgers, J. E. (2010). Immobilization of heavy metal ions (CuII, CdII, NiII, and PbII) by broiler litter-derived biochars in water and soil. J. Agric. Food Chem. 58 (9), 5538–5544. doi:10.1021/jf9044217
Wang, L., Cui, X., Cheng, H., Chen, F., Wang, J., Zhao, X., et al. (2015). A review of soil cadmium contamination in China including a health risk assessment. Environ. Sci. Pollut. Res. 22 (21), 16441–16452. doi:10.1007/s11356-015-5273-1
Wang, L., Zhang, Q., Liao, X., Li, X., Zheng, S., Zhao, F., et al. (2021a). Phytoexclusion of heavy metals using low heavy metal accumulating cultivars: A green technology. J. Hazard. Mater. 413, 125427. doi:10.1016/j.jhazmat.2021.125427
Wang, Y., Zhang, K., Lu, L., Xiao, X., and Chen, B. (2020). Novel insights into effects of silicon-rich biochar (Sichar) amendment on cadmium uptake, translocation and accumulation in rice plants. Environ. Pollut. 265, 114772. doi:10.1016/j.envpol.2020.114772
Wang, Y., Zheng, K., Zhan, W., Huang, L., Liu, Y., Li, T., et al. (2021b). Highly effective stabilization of Cd and Cu in two different soils and improvement of soil properties by multiple-modified biochar. Ecotoxicol. Environ. Saf. 207, 111294. doi:10.1016/j.ecoenv.2020.111294
Wu, C., Dun, Y., Zhang, Z., Li, M., and Wu, G. (2020). Foliar application of selenium and zinc to alleviate wheat (Triticum aestivum L.) cadmium toxicity and uptake from cadmium-contaminated soil. Ecotoxicol. Environ. Saf. 190, 110091. doi:10.1016/j.ecoenv.2019.110091
Wu, J., Wang, T., Wang, J., Zhang, Y., and Pan, W.-P. (2021). A novel modified method for the efficient removal of Pb and Cd from wastewater by biochar: Enhanced the ion exchange and precipitation capacity. Sci. Total Environ. 754, 142150. doi:10.1016/j.scitotenv.2020.142150
Xia, Y., Li, Y., Sun, Y., Miao, W., and Liu, Z. (2021). Co-pyrolysis of corn stover with industrial coal ash for in situ efficient remediation of heavy metals in multi-polluted soil. Environ. Pollut. 289, 117840. doi:10.1016/j.envpol.2021.117840
Xiang, J., Lin, Q., Yao, X., and Yin, G. (2021). Removal of Cd from aqueous solution by chitosan coated MgO-biochar and its in-situ remediation of Cd-contaminated soil. Environ. Res. 195, 110650. doi:10.1016/j.envres.2020.110650
Xu, P., Sun, C.-X., Ye, X.-Z., Xiao, W.-D., Zhang, Q., Wang, Q., et al. (2016). The effect of biochar and crop straws on heavy metal bioavailability and plant accumulation in a Cd and Pb polluted soil. Ecotoxicol. Environ. Saf. 132, 94–100. doi:10.1016/j.ecoenv.2016.05.031
Yang, T., Xu, Y., Huang, Q., Sun, Y., Liang, X., Wang, L., et al. (2021). An efficient biochar synthesized by iron-zinc modified corn straw for simultaneously immobilization Cd in acidic and alkaline soils. Environ. Pollut. 291, 118129. doi:10.1016/j.envpol.2021.118129
Yue, L., Lian, F., Han, Y., Bao, Q., Wang, Z., Xing, B., et al. (2019). The effect of biochar nanoparticles on rice plant growth and the uptake of heavy metals: Implications for agronomic benefits and potential risk. Sci. Total Environ. 656, 9–18. doi:10.1016/j.scitotenv.2018.11.364
Zhang, S., Xu, Y., Wu, M., Mao, X., Yao, Y., Shen, Q., et al. (2021). Geogenic enrichment of potentially toxic metals in agricultural soils derived from black shale in northwest Zhejiang, China: Pathways to and risks from associated crops. Ecotoxicol. Environ. Saf. 215, 112102. doi:10.1016/j.ecoenv.2021.112102
Zhang, S., Zhang, H., Cai, J., Zhang, X., Zhang, J., Shao, J., et al. (2018). Evaluation and prediction of cadmium removal from aqueous solution by phosphate-modified activated bamboo biochar. Energy fuels. 32 (4), 4469–4477. doi:10.1021/acs.energyfuels.7b03159
Zheng, R., Sun, G., Li, C., Reid, B. J., Xie, Z., Zhang, B., et al. (2017). Mitigating cadmium accumulation in greenhouse lettuce production using biochar. Environ. Sci. Pollut. Res. 24 (7), 6532–6542. doi:10.1007/s11356-016-8282-9
Zhou, J., Zhang, C., Du, B., Cui, H., Fan, X., Zhou, D., et al. (2020). Effects of zinc application on cadmium (Cd) accumulation and plant growth through modulation of the antioxidant system and translocation of Cd in low- and high-Cd wheat cultivars. Environ. Pollut. 265, 115045. doi:10.1016/j.envpol.2020.115045
Zhou, J., Zhang, C., Du, B., Cui, H., Fan, X., Zhou, D., et al. (2021). Soil and foliar applications of silicon and selenium effects on cadmium accumulation and plant growth by modulation of antioxidant system and Cd translocation: Comparison of soft vs. durum wheat varieties. J. Hazard. Mater. 402, 123546. doi:10.1016/j.jhazmat.2020.123546
Zhuang, Z., Niño-Savala, A. G., Mi, Z.-d., Wan, Y.-n., Su, D.-c., Li, H.-f., et al. (2021). Cadmium accumulation in wheat and maize grains from China: Interaction of soil properties, novel enrichment models and soil thresholds. Environ. Pollut. 275, 116623. doi:10.1016/j.envpol.2021.116623
Zhuang, Z., Wang, Q., Huang, S., NiñoSavala, A. G., Wan, Y., Li, H., et al. (2023). Source-specific risk assessment for cadmium in wheat and maize: Towards an enrichment model for China. J. Environ. Sci. 125, 723–734. doi:10.1016/j.jes.2022.02.024
Zong, Y., Xiao, Q., Malik, Z., Su, Y., Wang, Y., Lu, S., et al. (2021). Crop straw-derived biochar alleviated cadmium and copper phytotoxicity by reducing bioavailability and accumulation in a field experiment of rice-rape-corn rotation system. Chemosphere 280, 130830. doi:10.1016/j.chemosphere.2021.130830
Keywords: DTPA-extracted Cd, sequential extractions, antioxidant enzyme, yield, translocation factor
Citation: Dun Y, Wu C, Zhou M, Tian X and Wu G (2022) Wheat straw- and maize straw-derived biochar effects on the soil cadmium fractions and bioaccumulation in the wheat–maize rotation system. Front. Environ. Sci. 10:980893. doi: 10.3389/fenvs.2022.980893
Received: 29 June 2022; Accepted: 13 July 2022;
Published: 26 August 2022.
Edited by:
Jun Zhou, University of Massachusetts Lowell, United StatesReviewed by:
Hailong Liu, Yangzhou University, ChinaBuyun Du, University of Massachusetts Lowell, United States
Copyright © 2022 Dun, Wu, Zhou, Tian and Wu. This is an open-access article distributed under the terms of the Creative Commons Attribution License (CC BY). The use, distribution or reproduction in other forums is permitted, provided the original author(s) and the copyright owner(s) are credited and that the original publication in this journal is cited, in accordance with accepted academic practice. No use, distribution or reproduction is permitted which does not comply with these terms.
*Correspondence: Chao Wu, ZWtpbnBhdWwxMDA0QDE2My5jb20=