- 1Department of Applied Chemistry, College of Sciences, China Agricultural University, Beijing, China
- 2Institute for the Control of Agrochemicals, Ministry of Agriculture and Rural Affairs of the People’s Republic of China, Beijing, China
- 3Engineering Research Center of Plant Growth Regulator, Ministry of Education & College of Agronomy and Biotechnology, China Agricultural University, Beijing, China
In aquatic ecosystems, pesticide residues meanly present as mixtures of varying complexity, which may lead to compound pollution of organisms. Therefore, it is of great practical importance to evaluate the combined toxic effects of pesticides. In this study, the mixture acute toxicities of fenvalerate with tebuconazole on zebrafish larvae and adults were investigated. The effects of mixture toxicity on swimming behavior of larvae were also analyzed. The mixture acute toxicity of the systems showed antagonistic effects on adult zebrafish when the mixing ratios of fenvalerate and tebuconazole were equal, but the other two ratios of the mixes both showed synergistic effects. The strongest synergistic effect was observed when fenvalerate was mixed with tebuconazole in the ratio of 8:2. The swimming behavior of zebrafish larvae was significantly inhibited after exposure to single or mixed systems for 2 days. However, significant up-regulation of behavioral indicators in zebrafish larvae was observed at the beginning of exposure in the mixture exposure groups. The results showed that fenvalerate and tebuconazole had the risk of synergistic toxic effect on zebrafish. It is recommended that the simultaneous use of both pesticides in agricultural activities near water bodies should be scientifically controlled to avoid adverse effects on aquatic organisms.
1 Introduction
In modern agricultural production, pesticides are inevitably used to prevent, control, and mitigate the invasion of diseases, weeds, and pests. The use of pesticides has largely improved the yield and quality of crops to meet the needs of a growing world population (Arias-Estevez et al., 2008). After the application of pesticides, they enter the aquatic ecosystem through various pathways and present a mixture of different substances. As a result, organisms are exposed to this system and suffer from mixed toxicity effects (Zhang J. et al., 2017). Mixture effects are generally classified into three types: 1) independent effects, which refer to the sum of the individual effects of each compound acting alone, without affecting each other; 2) additive effects, which refer to the accumulation of toxic effects of all toxic substances; 3) interactions, which also include synergistic and antagonistic effects, respectively, which refer to the mixture toxic effects of the system with an intensity much greater and less than the sum of their individual effects. The combined toxicity of pesticide mixtures has received increasing attention for the past several decades. However, the studies are more focused on insecticide-to-insecticide or fungicide-to-fungicide, with relatively few studies on mixture toxicity of insecticides and fungicides reported (Maloney et al., 2018; Pham et al., 2018; Wang et al., 2018).
Pyrethroids are a class of synthetic broad-spectrum insecticides with high activity and better insecticidal efficacy than organochlorines, organophosphates, and carbamates (Yoo et al., 2016). They are also highly selective and less toxic to non-target organisms such as birds, earthworms, and mammals, and have been widely used in modern agriculture and outdoor/indoor pest control (Vijverberg and Vandenbercken, 1990; Yang et al., 2016). It once occupied more than 30% of the world market and has become one of the top three pesticides in the world (Barr et al., 2010; Xiao et al., 2012). Fenvalerate is a second generation pyrethroid insecticide with neurotoxicity associated with the opening and closing of Na+ channels (Tripathi and Verma, 2006). It is mainly used to control pests on cotton, vegetables and fruit trees. As systemic highly effective fungicides, triazoles are characterized by high systemic absorption, long residual period and broad spectrum. Among them, tebuconazole is widely used worldwide as a seed treatment and foliar spray with three major roles: protection, treatment, and eradication (Hua, 2013).
Fenvalerate and tebuconazole have been detected in the water environment in the ng/L to low µg/L range. The residue level of tebuconazole has been detected at 0.010–0.115 μg/L in US Streams (Battaglin et al., 2010; Bradley et al., 2017), and approximately 0.001–1.071 μg/L in surfacewater in São Paulo State (Montagner et al., 2018). Fenvalerate concentrations were significantly higher in industrial wastewater (0.034–0.349 μg/L) compared with surfacewater (0.017–0.021 μg/L) (Ge et al., 2010). Notably, there is considerable overlap in the occurrence and distribution of fenvalerate and tebuconazole in the applicable crops. They have been detected simultaneously in the same water environment (fenvalerate 0.033 μg/L, tebuconazole 0.021 μg/L) (Allinson et al., 2015), drawing considerable attention to their possible impact on aquatic organisms. Some studies have indicated the potential mechanisms of fenvalerate and tebuconazole to aquatic organisms. Zhang et al. reported that fenvalerate may cause developmental toxicity to zebrafish larvae by disrupting endocrine signaling at environmentally relevant concentrations (Zhang Q. et al., 2017). After exposure to a sublethal dose of fenvalerate at 50 μg/L, notable signs of apoptosis were found in the brain of zebrafish larvae, with the oxidative-DNA repair system, as well as neurogenesis impaired (Gu et al., 2010). Moreover, zebrafish exposed to 100 μg/L fenvalerate for 120 h exhibited decreased swimming activity accompanied by significantly elevated oxidative stress (Han et al., 2017). Tebuconazole (1, 2, and 4 mg/L) were reported to disrupt thyroid endocrine system and alter gene transcription in the hypothalamic-pituitary-thyroid axis in zebrafish larvae (Yu et al., 2013). In addition, a previous study found that tebuconazole (4 mg/L) could modulate the cholinergic system by altering AChE activity and thus reduce the locomotion of zebrafish larvae (Altenhofen et al., 2017). Therefore, it is necessary to study the combined toxic effects of fenvalerate and tebuconazole on aquatic organisms.
Fish are one of the most important aquatic vertebrates and play a crucial role in aquatic food webs through top-down and bottom-up regulation of nutrient and energy flows. They are susceptible to intoxication by pollutions in the aquatic environment, such as pesticides, heavy metals, and antibiotics, which can be rapidly absorbed through the skin and gills (Rubinstein, 2006). Therefore, fish are an indispensable component for testing the combined toxicity of the aquatic environment and are often used as biological indicators for assessing water pollution (Lammer et al., 2009; Lana et al., 2010). Behavior assay is considered a valuable method in ecological and toxicological studies. The behavioral indicators can reflect anxiety, alarm response, stress, and neurotoxic effects on aquatic organisms, creating important connections between biochemical and ecological processes (Barcellos et al., 2007; Egan et al., 2009; Shuman-Goodier and Propper, 2016). Therefore, behavior assay is of increasing interest to evaluate the effects of pesticides on aquatic organisms (Zala and Penn, 2004; Bossus et al., 2014; Kohler et al., 2018). For example, several studies have examined behavior in zebrafish, and the behavioral endpoints (travel time, move distance, speed, etc) under pesticide exposure (Seguret et al., 2016; Altenhofen et al., 2017; van den Bos et al., 2017). In this study, the individual and mixture toxicity effects of fenvalerate and tebuconazole on zebrafish were investigated in terms of acute toxicity and behavioral changes using zebrafish larvae and adults as test subjects. The purpose is to assess the potential risk of its mixture on the aquatic environment.
2 Materials and methods
2.1 Chemicals and reagents
Fenvalerate (CAS-No.51630–58-1; purity ≥ 93%, the remaining 7% constituents are optical isomers as follows: (R)-α-cyano-3-phenoxybenzyl-(S)-2-(4-chlorophenyl)-3-methylbutyrate (5%), (R)-α-cyano-3-phenoxybenzyl-(R)-2-(4-chlorophenyl)-3-methylbutyrate (1%), (S)-α-cyano-3-phenoxybenzyl-(R)-2-(4-chlorophenyl)-3-methylbutyrate (1%)) and tebuconazole (CAS-No.107534–96-3; purity ≥ 95%, the remaining 5% constituent is 3-((4H-1,2,4-triazol-4-yl)methyl)-1-(4-chlorophenyl)-4,4-dimethylpentan-3-ol)) provided by the College of Science, China Agricultural University were selected as test reagents. The toxicity of all constituents were predicted by the Toxicity Estimation Software Tool Version 5.1 provided by Environmental Protection Agency and the results showed that their acute toxicity values to fish were the same or lower than the main components. Stock solutions of 10,000 mg/L fenvalerate and tebuconazole were prepared in acetone (analytical grade) containing 5% Tween-80 for further dilution.
2.2 Experimental animals
The parental and adult zebrafish of wild-type AB-strain were obtained from Gao Feng Aquarium (Beijing, China). Parental zebrafish for egg production were cultured in a flow-through feeding equipment (Esen Corp., China), and adult zebrafish were first domesticated in reconstituted water (containing 0.33 mM CaCl2, 0.33 mM MgSO4, 5 mM NaCl, and 0.17 mM KCl) (Standardization, 1996) for more than 14 days before the test. Fish were fed twice a day with commercial fish food (Tetramin) at a level of approximately 1% of their body weight per day (Sancho et al., 2010) and 1/3 volume of the water was replaced every 24 h. Feeding water conditions were as follows: aeration and dechlorination treatment, pH (7.5 ± 0.5), water hardness (calculated as CaCO3) of 250 ± 25 mg/L, dissolved oxygen in the water at more than 80% of the air saturation value, water temperature (26.0 ± 1.0°C), and 14 h light/10 h dark photoperiod. The experiment was approved by the guidelines of the Institutional Animal Care and Use Committee (IACUC) of China Agricultural University.
2.3 Acute toxicity of individual and mixture pesticides to zebrafish adults
The tests were designed according to international standards for acute toxicity tests in fish and OECD-203 (Standards Press of China, 2012; OECD, 2019). Test fish were healthy adult zebrafish (3.0–4.0 cm) of different sex and have normal appearance. The reported environmentally relevant concentration of fenvalerate and tebuconazole are comparable (Allinson et al., 2015), while actual residues may vary, so we measured different ratios (Fenvalerate:Tebuconazole = 8:2, 5:5, and 2:8) to investigate the combined effect. Exposure tests were performed in 5 L beakers. Each beaker contained 4 L of pesticide solution (Table 1). Reconstituted water was used to prepare all diluted exposure solutions, which also served as blank control. Solvent control was arranged containing 0.06% acetone and 0.0003‰ Tween 80 as that in the test solutions with the highest concentrations of tebuconazole. Exposure concentrations were chosen based on OECD-203 (OECD, 2019) and preliminary experiments. There were three replicates of each treatment and 10 adult fish in each replicate. All test solutions were changed every 24 h. Mortality was recorded at 96 h. When no obvious movement was observed by lightly touching the fish tail with a glass rod, the fish were judged to be killed and the dead fish were removed promptly.
2.4 Acute toxicity of individual and mixture pesticides to zebrafish larvae
Embryos were obtained from the healthy parental zebrafish with a ratio of 1:2 (female to male) in the spawning box (Esen Corp., China). Spawning was induced in the morning when the light was turned on, and eggs were collected 30 min later and rinsed with reconstituted water. Fertilized and normally developed eggs were selected at approximately 2 h post-fertilization (hpf) using a dissecting microscope.
The embryos (n = 10) were exposed to a 50 ml beaker containing 30 ml solution (Table 2). Each treatment contains three replicates. Similar to 2.2, a blank control and solvent control were set up. During the exposure period, the larvae were put into a constant temperature incubator with a light/dark ratio of 14 h: 10 h at 28°C. 3/4 volume of solution was replaced every 24 h. The mortality was recorded at 96 h after exposure (Giari et al., 2012).
2.5 Evaluation of mixed toxicity
The additive index (AI) method was proposed by Marking in 1977 to evaluate the toxic effects of mixed systems. It is defined based on the LC50 or EC50 with 95% confidence interval (Marking, 1977). Its calculation formula is as follows.
S < 1.0, AI = (1⁄S) − 1; S ≥ 1.0, AI = −S + 1.0.
S represents the total biological activity, A, B and C represent the compounds in the mixture, i represents the LC50 of the individual pesticide, and m represents the LC50 of every pesticide in the mixture.
When AI < 0, the mixture toxic effect of the mixture is antagonistic, when AI = 0, it represents additive effect, when AI > 0, it is synergistic effect, and the larger the AI value, the stronger the synergistic effect.
2.6 Behavioral experiment of zebrafish larvae
Healthy zebrafish larvae were randomly selected after 3 d of hatching and placed in the solution. Two individual agents and binary mixture systems (fenvalerate:tebuconazole = 8:2) were selected for behavioral tests on zebrafish larvae based on the results of acute toxicity tests. The concentrations of all treatment groups were set at 3.00 × 10–5, 3.00 × 10–4 and 3.00 × 10–3 mg/L. Simultaneously, 3.00 × 10–5 mg/L was close to the detected concentrations in surfacewater in Australia (Allinson et al., 2015). Stock solutions of 1, 10, 100 mg/L fenvalerate and tebuconazole were prepared in acetone containing 5% Tween-80 for further dilution. A blank control was set up and the solvent control was arranged containing 0.003% acetone and 0.0015‰ Tween 80 as that in the diluted test solutions with the highest concentration (3.00 × 10–3 mg/L). Exposure tests were conducted in 500 ml beakers, each containing 300 ml solution as one replicate (containing 100 larvae). Three replicates were set for every group. Tests were performed at 28°C under a photoperiod of light/dark = 14 h/10 h. 3/4 volume of the solution was changed every 24 h and dead fish were removed promptly. At the beginning of exposure and 2 d, 20 normal larvae were randomly selected from each replicate. The larvae were raised under the same light conditions as the behavioral assay. Zebrafish swimming behavior was measured and analyzed using a brightness adjustable white light luminous plate (BGdz103,143-7B4) and the LoliTrack Animal Behavior Analysis System (IDSUI-3240CP-C-GL). The movement of the larvae was observed and photographed within 15 min using uEye Cockpit software. Monitoring indicators include average velocity, average acceleration, activity time and swimming distance of zebrafish larvae.
2.7 Statistical analysis
Statistical analysis was performed via Excel 2016 and SPSS 20.0. Significant differences were evaluated using Dunnett’s one-way analysis of variance (ANOVA). LC50 values were compared using the method by Sprague and Fogels 1976) to test for differences in toxic effects between adults zebrafish and larvae (Sprague and Fogels).
3 Results
3.1 Acute lethal test results of single and mixture systems on zebrafish
As shown in Table 3, the acute toxicity of fenvalerate to adult zebrafish was higher than that of tebuconazole. The AI of mixture systems of fenvalerate and tebuconazole in different ratios were calculated using the equations. The mixture acute toxicity of the systems showed antagonistic effects on adult zebrafish when the mixing ratios of fenvalerate and tebuconazole were equal, but the other two ratios of the mixes both showed synergistic effects. The strongest synergistic effect was observed when fenvalerate was mixed with tebuconazole in the ratio of 8:2 (Table 3).
Similar to the results of adult fish, the acute toxicity of fenvalerate to zebrafish larvae is higher than tebuconazole (Table 3). According to the toxicity results of adult fish, the mixture system with the strongest synergistic effect (fenvalerate: tebuconazole = 8:2) was selected to evaluate the combined toxic effects of fenvalerate and tebuconazole on larvae. The results also showed a synergistic effect (Table 3). In addition, after performing the method by Sprague and Fogels, the significant differences were found in the LC50 values between adults and larvae, with adult zebrafish exhibiting lower LC50 values to individual and mixed pesticides.
3.2 Effects of different systems on the larvae behavior
There was no mortality in the larvae during exposure. In addition, no significant differences were found in all tested swimming behavior indicators between the blank control and solvent control treatments. The average velocity, average acceleration, active time and swimming distance of zebrafish larvae were significantly inhibited after 2 d of exposure to fenvalerate or tebuconazole (Figures 1–8). Different from individual exposure, the swimming behavior of larvae was significantly up-regulated at the beginning of mixture solutions exposure (fenvalerate:tebuconazole = 8:2). When the mixture exposure was prolonged to 2 d, all behavioral indicators decreased significantly (Figures 9–12).
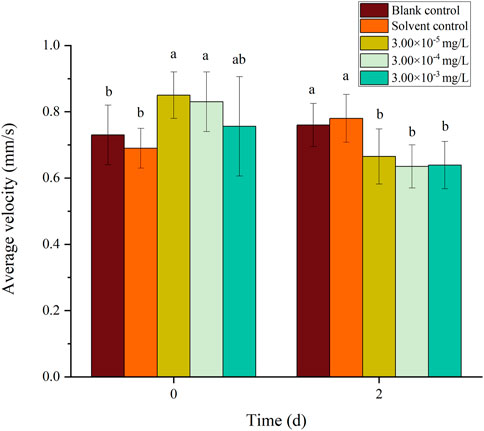
FIGURE 9. Effects of the mixture systems of fenvalerate and tebuconazole on the average velocity of zebrafish larvae locomotion.
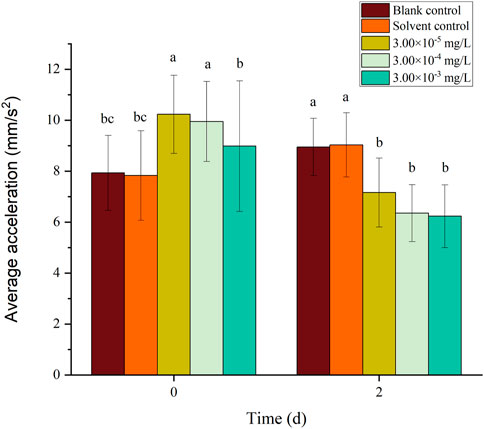
FIGURE 10. Effects of the mixture systems of fenvalerate and tebuconazole on the average acceleration of zebrafish larvae locomotion.
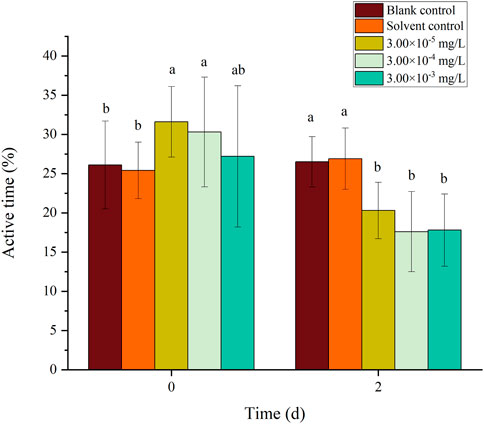
FIGURE 11. Effects of the mixture systems of fenvalerate and tebuconazole on the active time of zebrafish larvae locomotion.
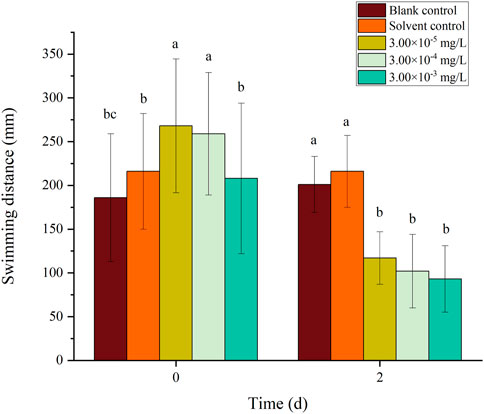
FIGURE 12. Effects of the mixture systems of fenvalerate and tebuconazole on the swimming distance of zebrafish larvae locomotion.
Data are expressed as the mean ± SD and different alphabets denote significant differences between the exposure treatments for each day (p < 0.05, one-way ANOVA). Significant differences were found at 2d (F = 3.62, dfb = 4, dfw = 10).
Data are expressed as the mean ± SD and different alphabets denote significant differences between the exposure treatments for each day (p < 0.05, one-way ANOVA). Significant differences were found at 2d (F = 3.94, dfb = 4, dfw = 10).
Data are expressed as the mean ± SD and different alphabets denote significant differences between the exposure treatments for each day (p < 0.05, one-way ANOVA). Significant differences were found at 2d (F = 4.07, dfb = 4, dfw = 10).
Data are expressed as the mean ± SD and different alphabets denote significant differences between the exposure treatments for each day (p < 0.05, one-way ANOVA). Significant differences were found at 2d (F = 5.42, dfb = 4, dfw = 10).
Data are expressed as the mean ± SD and different alphabets denote significant differences between the exposure treatments for each day (p < 0.05, one-way ANOVA). Significant differences were found at 2d (F = 3.59, dfb = 4, dfw = 10).
Data are expressed as the mean ± SD and different alphabets denote significant differences between the exposure treatments for each day (p < 0.05, one-way ANOVA). Significant differences were found at 2d (F = 3.34, dfb = 4, dfw = 10).
Data are expressed as the mean ± SD and different alphabets denote significant differences between the exposure treatments for each day (p < 0.05, one-way ANOVA). Significant differences were found at 2d (F = 3.87, dfb = 4, dfw = 10).
Data are expressed as the mean ± SD and different alphabets denote significant differences between the exposure treatments for each day (p < 0.05, one-way ANOVA). Significant differences were found at 2d (F = 4.23, dfb = 4, dfw = 10).
Data are expressed as the mean ± SD and different alphabets denote significant differences between the exposure treatments for each day (p < 0.05, one-way ANOVA). Significant differences were found at 0 d (F = 3.55, dfb = 4, dfw = 10) and 2d (F = 4.72, dfb = 4, dfw = 10).
Data are expressed as the mean ± SD and different alphabets denote significant differences between the exposure treatments for each day (p < 0.05, one-way ANOVA). Significant differences were found at 0 d (F = 4.93, dfb = 4, dfw = 10) and 2d (F = 5.12, dfb = 4, dfw = 10).
Data are expressed as the mean ± SD and different alphabets denote significant differences between the exposure treatments for each day (p < 0.05, one-way ANOVA). Significant differences were found at 0 d (F = 2.99, dfb = 4, dfw = 10) and 2d (F = 5.04, dfb = 4, dfw = 10).
Data are expressed as the mean ± SD and different alphabets denote significant differences between the exposure treatments for each day (p < 0.05, one-way ANOVA). Significant differences were found at 0 d (F = 3.53, dfb = 4, dfw = 10) and 2d (F = 4.88, dfb = 4, dfw = 10).
4 Discussion
The acute toxicity test is one of the most basic indicators to evaluate the environmental effects of pesticides and has been widely used. According to Mu et al., the 96 h-LC50 values of triazole fungicide difenoconazole for adult, embryo, and larvae zebrafish were 1.45, 2.34, and 1.17 mg/L, respectively (Mu et al., 2013). However, they did not perform an in-depth statistical analysis based on the confidence interval of the LC50 values to compare the differences in acute toxicity values between every life stage. In the present study, the triazole fungicide tebuconazole exhibits roughly comparable 96 h-LC50 values against adult and larvae zebrafish. But after performing the method by (Sprague and Fogels, 1977), significant differences in the 96 h-LC50 values between two life stages of zebrafish were discovered, with adults being more sensitive. Similarly, fenvalerate and pesticide mixture (fenvalerate:tebuconazole = 8:2) are significantly more poisonous to adults than to larvae zebrafish. This difference may be related to the exposure route, with no food input during the 96 h acute toxicity test. The larvae rely on their yolk for energy during this period, while the adults are unable to maintain normal metabolism without food intake and thus become more sensitive to exogenous hazardous substances.
Some studies have demonstrated that pyrethroids mixed with organophosphorus insecticides can exhibit synergistic toxic effects on zebrafish (Zhang J. et al., 2017; Wang et al., 2017). Wang et al. also reported that the equitoxic mixture of cyprodinil and kresoxim-methyl exhibited synergistic toxic effects to zebrafish embryos (Wang et al., 2018). However, little attention has been paid to the possible differences in the toxic effects of varied ratios of mixtures on organisms. A previous study has found comparable residue concentrations of fenvalerate and tebuconazole (Allinson et al., 2015), but the practical levels may vary. Fenvalerate and tebuconazole have different periods, dosages and frequency of application in pest control. In addition, there are differences in the dissipation patterns of fenvalerate and tebuconazole under environmental conditions. Therefore, we selected three different ratios to evaluate the acute toxicity to zebrafish, aiming at a more comprehensive understanding of their combined toxic effects on fish. Wu et al. reported that fenvalerate and the triazole fungicide triadimefon had a synergistic effect on the toxicity of rare minnow embryos after co-exposure (Wu et al., 2018). The exposure concentrations used in their study were set as a simple summation of the single acute toxicity concentrations of the two pesticides, and exposure of embryos was performed after setting them in a 1:1.4 multiplicative gradient. In contrast to their study, we designed the combined exposure concentration by considering the two pesticides as a whole, and the mixture ratios were based on the environmentally relevant levels of fenvalerate and tebuconazole, which to a certain extent reflects the real situation of the environment. Our study found that mixtures of fenvalerate and tebuconazole in different ratios exhibited different combined toxic effects on zebrafish. Although the mechanisms of toxic effects of fenvalerate and tebuconazole on fish are unknown, fenvalerate is considered to be a potent insect neurotoxicant by affecting interactions with sodium channels (Tripathi and Verma, 2006), and tebuconazole acts by inhibition of fungal lanosterol-14R-demethylase in ergosterol biosynthesis (Maren Kahle et al., 2008). The two pesticides are supposed to have different toxicity mechanisms in fish and interact with different molecular targets. Therefore, different ratios of the mixture may display interactions with multiple targets along an acute toxicity pathway that comprise interaction with molecular targets, modulation of key events associated with the toxic effects (Beyer et al., 2014), and ending with the different LC50 values. The specific mechanism needs to be further explored in toxicogenomics and metabolic activities.
Behavioral indicators are an important bridge between biochemical and ecological effects of environmental pollution. Abnormalities in behavioral endpoints have the capacity to reveal potential effects of drug exposure on organisms. Some studies have reported the effects of pyrethroid insecticides on behavioral changes in zebrafish. It has been documented that the distance and time of movement of zebrafish larvae were significantly reduced after 120 h of 100 μg/L fenvalerate exposure (Han et al., 2017). Han et al. also reported that distance moved of adult zebrafish was shortened and activated time was reduced after fenvalerate exposure, and also the expression of neurogenesis-related genes was significantly downregulated to demonstrate neural damage (Han et al., 2017). Cis-fenvalerate (1,000 μg/L) was also studied by Awoyemi et al. to significantly reduce the distance moved of zebrafish larvae, and they also found that bifenthrin (0.01, 10 μg/L) significantly slowed the average speed of zebrafish larvae (Awoyemi et al., 2019). And Nema et al. showed that cypermethrin significantly reduced the distance moved of zebrafish (Nema and Bhargava, 2018). Studies on the effects of triazole fungicides on the locomotor behavior of zebrafish have been gradually reported. Short-term exposure to tebuconazole was able to cause clinical signs such as loss of motor coordination and sluggishness, and failed to recover after a decontamination period of clear water exposure (Sancho et al., 2010). Tebuconazole also increased the eye distance of juveniles and significantly reduced the distance moved, absolute angle of rotation and average speed of zebrafish (Altenhofen et al., 2017). Cyproconazole significantly reduced the activity of zebrafish larvae (Cao et al., 2019), and Paredes-Zuniga et al. found a concentration-dependent decrease in the locomotor activity of zebrafish larvae after triadimefon exposure (Paredes-Zuniga et al., 2019). Our behavioral assays indicated that after 2 d of exposure to the two pesticides individually and their mixtures, there was basically a significant decrease in the average velocity, average acceleration, activity time and distance moved of larvae. This may be due to the toxic effects on zebrafish after exposure to sublethal doses of the pesticide solution, which leads to a decrease in physical function and consequent decrease in swimming behavior. Generally, pesticide residues in the aquatic environment do not reach doses that cause fish mortality, so it is of greater value to evaluate the sublethal effects of pesticides on fish. Compared with other evaluation methods (growth, development, and reproduction), swimming behavior is a more rapid indicator of the potential effect of pesticides on fish. In the present study, the swimming ability of larvae was reduced under 2d exposure to individual and mixed pesticides, which was consistent with the previous study. But different from previous studies, we also observed the instantaneous effects of individual and mixed pesticides on the swimming behavior of larvae at the beginning of exposure. Significant up-regulation of behavioral indicators in zebrafish larvae was observed in some binary pesticide mixtures groups, while no difference under exposure to two individual pesticides, suggesting that the mixture of pesticides may be more likely to induce instantaneously stress responses. The reason is at present very unclear due to the lack of the molecular mechanism of the relative mixtures. According to Song et al., the responses at low exposure level or short-term exposure may provide valuable information on the stress-induced signal transductions and the defence system for maintaining homeostasis in the organism (Song et al., 2012). In addition, the neuroendocrine system of zebrafish is reported to provide robust physiological responses to stress, and these changes may be associated with the reduced locomotion of zebrafish (Alsop and Vijayan, 2008). Previous studies have revealed induced neural damage and reduced locomotion of zebrafish under fenvalerate and tebuconazole exposure, respectively (Altenhofen et al., 2017; Han et al., 2017). Therefore, it can be hypothesized that the anxious behavior of zebrafish might be stress responses and further study should focus on neuroendocrine system.
5 Conclusion
In conclusion, fenvalerate and tebuconazole had the risk of synergistic toxic effects on zebrafish and induced behavioral abnormalities at environmentally relevant levels in larvae. Based on these findings, it is recommended that the simultaneous use of both pesticides in agricultural activities near water bodies should be scientifically controlled to avoid adverse effects on aquatic organisms.
Data availability statement
The raw data supporting the conclusions of this article will be made available by the authors, without undue reservation.
Author contributions
SP, XL, and DN contributed to conception and design of the study. CY, LH, NC, XG, and CL performed the experiment and statistical analysis. CY, LH, and CL wrote the first draft of the manuscript. YC wrote sections of the manuscript. All authors contribute to manuscript revision, read, and approved the submitted version”.
Acknowledgments
The authors acknowledge technical support from Jia Liu.
Conflict of interest
The authors declare that the research was conducted in the absence of any commercial or financial relationships that could be construed as a potential conflict of interest.
Publisher’s note
All claims expressed in this article are solely those of the authors and do not necessarily represent those of their affiliated organizations, or those of the publisher, the editors and the reviewers. Any product that may be evaluated in this article, or claim that may be made by its manufacturer, is not guaranteed or endorsed by the publisher.
References
Allinson, G., Zhang, P., Bui, A., Allinson, M., Rose, G., Marshall, S., et al. (2015). Pesticide and trace metal occurrence and aquatic benchmark exceedances in surface waters and sediments of urban wetlands and retention ponds in Melbourne, Australia. Environ. Sci. Pollut. Res. 22 (13), 10214–10226. doi:10.1007/s11356-015-4206-3
Alsop, D., and Vijayan, M. M. (2008). Development of the corticosteroid stress axis and receptor expression in zebrafish. Am. J. Physiology-Regulatory Integr. Comp. Physiology 294 (3), R711–R719. doi:10.1152/ajpregu.00671.2007
Altenhofen, S., Nabinger, D. D., Wiprich, M. T., Brandao Pereira, T. C., Bogo, M. R., and Bonan, C. D. (2017). Tebuconazole alters morphological, behavioral and neurochemical parameters in larvae and adult zebrafish (Danio rerio). Chemosphere 180, 483–490. doi:10.1016/j.chemosphere.2017.04.029
Arias-Estevez, M., Lopez-Periago, E., Martinez-Carballo, E., Simal-Gandara, J., Mejuto, J. C., and Garcia-Rio, L. (2008). The mobility and degradation of pesticides in soils and the pollution of groundwater resources. Agric. Ecosyst. Environ. 123 (4), 247–260. doi:10.1016/j.agee.2007.07.011
Awoyemi, O. M., Kumar, N., Schmitt, C., Subbiah, S., and Crago, J. (2019). Behavioral, molecular and physiological responses of embryo-larval zebrafish exposed to types I and II pyrethroids. Chemosphere 219, 526–537. doi:10.1016/j.chemosphere.2018.12.026
Barcellos, L. J. G., Ritter, F., Kreutz, L. C., Quevedo, R. M., da Silva, L. B., Bedin, A. C., et al. (2007). Whole-body cortisol increases after direct and visual contact with a predator in zebrafish, Danio rerio. Aquaculture 272 (1-4), 774–778. doi:10.1016/j.aquaculture.2007.09.002
Barr, D. B., Olsson, A. O., Wong, L.-Y., Udunka, S., Baker, S. E., Whitehead, R. D., et al. (2010). Urinary concentrations of metabolites of pyrethroid insecticides in the general US population: National health and nutrition examination survey 1999-2002. Environ. Health Perspect. 118 (6), 742–748. doi:10.1289/ehp.0901275
Battaglin, W. A., Sandstrom, M. W., Kuivila, K. M., Kolpin, D. W., and Meyer, M. T. (2010). Occurrence of azoxystrobin, propiconazole, and selected other fungicides in US Streams, 2005–2006. Water Air Soil Pollut. 218 (1-4), 307–322. doi:10.1007/s11270-010-0643-2
Beyer, J., Petersen, K., Song, Y., Ruus, A., Grung, M., Bakke, T., et al. (2014). Environmental risk assessment of combined effects in aquatic ecotoxicology: A discussion paper. Mar. Environ. Res. 96, 81–91. doi:10.1016/j.marenvres.2013.10.008
Bossus, M. C., Guler, Y. Z., Short, S. J., Morrison, E. R., and Ford, A. T. (2014). Behavioural and transcriptional changes in the amphipod Echinogammarus marinus exposed to two antidepressants, fluoxetine and sertraline. Aquat. Toxicol. 151, 46–56. doi:10.1016/j.aquatox.2013.11.025
Bradley, P. M., Journey, C. A., Romanok, K. M., Barber, L. B., Buxton, H. T., Foreman, W. T., et al. (2017). Expanded target-chemical analysis reveals extensive mixed-organic-contaminant exposure in U.S. Streams. Environ. Sci. Technol. 51 (9), 4792–4802. doi:10.1021/acs.est.7b00012
Cao, F., Souders, C. L., II, Li, P., Pang, S., Qiu, L., and Martyniuk, C. J. (2019). Developmental toxicity of the triazole fungicide cyproconazole in embryo-larval stages of zebrafish (Danio rerio). Environ. Sci. Pollut. Res. 26 (5), 4913–4923. doi:10.1007/s11356-018-3957-z
Egan, R. J., Bergner, C. L., Hart, P. C., Cachat, J. M., Canavello, P. R., Elegante, M. F., et al. (2009). Understanding behavioral and physiological phenotypes of stress and anxiety in zebrafish. Behav. Brain Res. 205 (1), 38–44. doi:10.1016/j.bbr.2009.06.022
Ge, J., Cong, J., Sun, Y., Li, G., Zhou, Z., Qian, C., et al. (2010). Determination of endocrine disrupting chemicals in surface water and industrial wastewater from Beijing, China. Bull. Environ. Contam. Toxicol. 84 (4), 401–405. doi:10.1007/s00128-010-9958-3
Giari, L., Dezfuli, B. S., Astolfi, L., and Martini, A. (2012). Ultrastructural effects of cisplatin on the inner ear and lateral line system of zebrafish (Danio rerio) larvae. J. Appl. Toxicol. 32 (4), 293–299. doi:10.1002/jat.1691
Gu, A., Shi, X., Yuan, C., Ji, G., Zhou, Y., Long, Y., et al. (2010). Exposure to fenvalerate causes brain impairment during zebrafish development. Toxicol. Lett. 197 (3), 188–192. doi:10.1016/j.toxlet.2010.05.021
Han, J., Ji, C., Guo, Y., Yan, R., Hong, T., Dou, Y., et al. (2017). Mechanisms underlying melatonin-mediated prevention of fenvalerate-induced behavioral and oxidative toxicity in zebrafish. J. Toxicol. Environ. Health A 80 (23-24), 1331–1341. doi:10.1080/15287394.2017.1384167
Hua, N. (2013). Formulation and application of fungicide tebuconazole. Agrochemicals 52 (11), 781–786. doi:10.16820/j.cnki.1006-0413.2013.11.001
Kahle, Maren, Buerge, Ignaz J., Hauser, Andrea, Muller, Markus D., and Poiger, T. (2008). Azole fungicides: Occurrence and fate in wastewater and surface waters. Environ. Sci. Technol. (42), 7193–7200. doi:10.1021/es8009309
Kohler, S. A., Parker, M. O., and Ford, A. T. (2018). Species-specific behaviours in amphipods highlight the need for understanding baseline behaviours in ecotoxicology. Aquat. Toxicol. 202, 173–180. doi:10.1016/j.aquatox.2018.07.013
Konwick, B. J., Garrison, A. W., Avants, J. K., and Fisk, A. T. (2006). Bioaccumulation and biotransformation of chiral triazole fungicides in rainbow trout (Oncorhynchus mykiss). Aquat. Toxicol. 80 (4), 372–381. doi:10.1016/j.aquatox.2006.10.003
Lammer, E., Carr, G. J., Wendler, K., Rawlings, J. M., Belanger, S. E., and Braunbeck, T. (2009). Is the fish embryo toxicity test (FET) with the zebrafish (Danio rerio) a potential alternative for the fish acute toxicity test? Comp. Biochem. Physiology Part C Toxicol. Pharmacol. 149 (2), 196–209. doi:10.1016/j.cbpc.2008.11.006
Lana, R., Vavrova, M., Navratil, S., Brabencova, E., and Vecerek, V. (2010). Organochlorine pollutants in chub, Leuciscus cephalus, from the svratka river, Czech republic. Bull. Environ. Contam. Toxicol. 84 (6), 726–730. doi:10.1007/s00128-010-0005-1
Maloney, E. M., Morrissey, C. A., Headley, J. V., Peru, K. M., and Liber, K. (2018). Can chronic exposure to imidacloprid, clothianidin, and thiamethoxam mixtures exert greater than additive toxicity in Chironomus dilutus? Ecotoxicol. Environ. Saf. 156, 354–365. doi:10.1016/j.ecoenv.2018.03.003
Marking, L. L. (1977). Method for assessing additive toxicity of chemical mixtures. Washington: ASTM special technical publications.
Montagner, C., Sodré, F., Acayaba, R., Vidal, C., Campestrini, I., Locatelli, M., et al. (2018). Ten years-snapshot of the occurrence of emerging contaminants in drinking, surface and ground waters and wastewaters from São Paulo state, Brazil. J. Braz. Chem. Soc. 30 (3), 614–632. doi:10.21577/0103-5053.20180232
Mu, X., Pang, S., Sun, X., Gao, J., Chen, J., Chen, X., et al. (2013). Evaluation of acute and developmental effects of difenoconazole via multiple stage zebrafish assays. Environ. Pollut. 175, 147–157. doi:10.1016/j.envpol.2012.12.029
Nema, S., and Bhargava, Y. (2018). Quantitative assessment of cypermethrin induced behavioural and biochemical anomalies in adult zebrafish. Neurotoxicol. Teratol. 68, 57–65. doi:10.1016/j.ntt.2018.05.003
OECD (2019). Guidelines for the testing of chemicals. Paris, France: Organization for Economic Cooperation and Development. Section 2, Test No. 305: Acute Toxicity for Fish.
Paredes-Zuniga, S., Trost, N., De la Paz, J. F., Alcayaga, J., and Allende, M. L. (2019). Behavioral effects of triadimefon in zebrafish are associated with alterations of the dopaminergic and serotonergic pathways. Prog. Neuropsychopharmacol. Biol. Psychiatry 92, 118–126. doi:10.1016/j.pnpbp.2018.12.012
Pham, B., Miranda, A., Allinson, G., and Nugegoda, D. (2018). Assessing interactive mixture toxicity of carbamate and organophosphorus insecticides in the yabby (Cherax destructor). Ecotoxicology 27 (9), 1217–1224. doi:10.1007/s10646-018-1973-x
Rubinstein, A. L. (2006). Zebrafish assays for drug toxicity screening. Expert Opin. Drug Metab. Toxicol. 2 (2), 231–240. doi:10.1517/17425255.2.2.231
Sancho, E., Villarroel, M. J., Fernandez, C., Andreu, E., and Ferrando, M. D. (2010). Short-term exposure to sublethal tebuconazole induces physiological impairment in male zebrafish (Danio rerio). Ecotoxicol. Environ. Saf. 73 (3), 370–376. doi:10.1016/j.ecoenv.2009.09.020
Seguret, A., Collignon, B., and Halloy, J. (2016). Strain differences in the collective behaviour of zebrafish (Danio rerio) in heterogeneous environment. R. Soc. open Sci. 3 (10), 160451. doi:10.1098/rsos.160451
Shuman-Goodier, M. E., and Propper, C. R. (2016). A meta-analysis synthesizing the effects of pesticides on swim speed and activity of aquatic vertebrates. Sci. Total Environ. 565, 758–766. doi:10.1016/j.scitotenv.2016.04.205
Song, Y., Salbu, B., Heier, L. S., Teien, H. C., Lind, O. C., Oughton, D., et al. (2012). Early stress responses in Atlantic salmon (Salmo salar) exposed to environmentally relevant concentrations of uranium. Aquat. Toxicol. 112-113, 62–71. doi:10.1016/j.aquatox.2012.01.019
Sprague, J., and Fogels, A. (1976). “Watch the Y in bioassay,” in Proceedings of the 3 rd aquatic toxicity workshop, halifax, nova scotia nov. 2-3, 1976 (Halifax, Canada: Environment Canada), 107–118. Tech. Report.(,
Standardization, I. O. f. (1996). Water quality: Determination of the acute lethal toxicity of substances to a fresh water fish [brachydanio rerio Hamilton-buchanan (teleostei, cyprinidae)]. Geneva, Switzerland: ISO.
Standards Press of China (2012). Chemicals—fish acute toxicity test. China, Beijing: Standards Press of China.
Sun, X., Wang, C., Li, X., Zheng, M., and Qiu, L. (2015). Acute toxicity of fenoxycarb to zebrafish(Brachydanio rerio) at different development stages and chronic toxicity to embryo and sac-fry stage. Chin. J. Pesticide Sci. 17 (03), 279–284. doi:10.3969/j.issn.1008-7303.2015.03.05
Tripathi, G., and Verma, P. (2006). Mechanism of fenvalerate-withdrawal-dependent recovery in metabolism of a catfish, Clarias batrachus. Pestic. Biochem. Physiol. 86 (3), 167–171. doi:10.1016/j.pestbp.2006.03.005
van den Bos, R., Mes, W., Galligani, P., Heil, A., Zethof, J., Flik, G., et al. (2017). Further characterisation of differences between TL and AB zebrafish (Danio rerio): Gene expression, physiology and behaviour at day 5 of the larval stage. PLoS One 12 (4), e0175420. doi:10.1371/journal.pone.0175420
Vijverberg, H. P. M., and Vandenbercken, J. (1990). Neurotoxicological effects and the mode of action of pyrethroid insecticides. Crit. Rev. Toxicol. 21(2), 105–126.doi:10.3109/10408449009089875
Wang, Y., Dai, D., Yu, Y., Yang, G., Shen, W., Wang, Q., et al. (2018). Evaluation of joint effects of cyprodinil and kresoxim-methyl on zebrafish, Danio rerio. J. Hazard. Mat. 352, 80–91. doi:10.1016/j.jhazmat.2018.03.023
Wang, Y. H., Lv, L., Yu, Y. J., Yang, G. L., Xu, Z. L., Wang, Q., et al. (2017). Single and joint toxic effects of five selected pesticides on the early life stages of zebrafish (Denio rerio). Chemosphere 170, 61–67. doi:10.1016/j.chemosphere.2016.12.025
Wu, S. G., Hu, G. J., Zhao, X. P., Wang, Q., and Jiang, J. H. (2018). Synergistic potential of fenvalerate and triadimefon on endocrine disruption and oxidative stress during rare minnow embryo development. Environ. Toxicol. 33 (7), 759–769. doi:10.1002/tox.22563
Xiao, Y., Chen, S., Hu, W., and Hu, M. (2012). New progress and prospect for the microbial degradation of pyrethroid pesticides. Chin. Agric. Sci. Bull. 28 (27), 218–224. doi:10.3969/j.issn.1000-6850.2012.27.043
Yang, X. L., Zhang, P. J., Li, X. B., Hu, L., Gao, H. X., Zhang, S. B., et al. (2016). Effervescence-assisted beta-cyclodextrin/attapulgite composite for the in-syringe dispersive solid-phase extraction of pyrethroids in environmental water samples. Talanta 153, 353–359. doi:10.1016/j.talanta.2016.03.007
Yoo, M., Lim, Y.-H., Kim, T., Lee, D., and Hong, Y.-C. (2016). Association between urinary 3-phenoxybenzoic acid and body mass index in Korean adults: 1(st) Korean national environmental health survey. Ann. Occup. Environ. Med. 28, 2. doi:10.1186/s40557-015-0079-7
Yu, L., Chen, M., Liu, Y., Gui, W., and Zhu, G. (2013). Thyroid endocrine disruption in zebrafish larvae following exposure to hexaconazole and tebuconazole. Aquat. Toxicol. 138, 35–42. doi:10.1016/j.aquatox.2013.04.001
Zala, S. M., and Penn, D. J. (2004). Abnormal behaviours induced by chemical pollution: A review of the evidence and new challenges. Anim. Behav. 68, 649–664. doi:10.1016/j.anbehav.2004.01.005
Zhang, J., Liu, L., Ren, L., Feng, W., Lv, P., Wu, W., et al. (2017a). The single and joint toxicity effects of chlorpyrifos and beta-cypermethrin in zebrafish (Danio rerio) early life stages. J. Hazard. Mat. 334, 121–131. doi:10.1016/j.jhazmat.2017.03.055
Keywords: pyrethroid insecticide, triazole fungicide, synergistic effect, fish, acute toxicity, swimming behavior
Citation: Yao C, Huang L, Li C, Nie D, Chen Y, Guo X, Cao N, Li X and Pang S (2022) Exposure to fenvalerate and tebuconazole exhibits combined acute toxicity in zebrafish and behavioral abnormalities in larvae. Front. Environ. Sci. 10:975634. doi: 10.3389/fenvs.2022.975634
Received: 22 June 2022; Accepted: 29 July 2022;
Published: 31 August 2022.
Edited by:
Qiao-Guo Tan, Xiamen University, ChinaReviewed by:
Luís Manuel Félix, University of Trás-os-Montes and Alto Douro, PortugalCarlos Gravato, University of Lisbon, Portugal
Copyright © 2022 Yao, Huang, Li, Nie, Chen, Guo, Cao, Li and Pang. This is an open-access article distributed under the terms of the Creative Commons Attribution License (CC BY). The use, distribution or reproduction in other forums is permitted, provided the original author(s) and the copyright owner(s) are credited and that the original publication in this journal is cited, in accordance with accepted academic practice. No use, distribution or reproduction is permitted which does not comply with these terms.
*Correspondence: Xuefeng Li, OTEwMzBAY2F1LmVkdS5jbg==; Sen Pang, cGFuZ3Nlbjc4MTJAY2F1LmVkdS5jbg==