- Department of Biology, Università degli Studi di Bari Aldo Moro, Bari, Italy
Rare earth elements (REEs) are a group of 15 elements, the lanthanides and Yttrium and Scandium, with similar chemical and physical properties. Their use for many advanced technological applications remarkably increased in the last decades, and it was associated with an intensive extraction of such elements from their ores. Consequently, increasing amounts of either REE-containing by-products, deriving from the extraction process, and REE-containing wastes, deriving from the disposal of REE-containing devices, are reaching the environmental systems both at the local and global levels, as never in the past. Few data in the literature concern the effect (beneficial or toxic) induced by REEs application in terrestrial plant species and at different physiological stages. A set of experiments with exposure to two REEs was performed on some plants to evaluate this. Data of investigations focused on the exposure of lentil (Lens culinaris Medik.) seedlings and onion (Allium cepa L.) bulbs to cerium chloride (CeCl3) and neodymium chloride (NdCl3) at different concentrations for 72 h. Results showed alteration of the growth rate and the levels of some parameters considered as biomarkers of stress (reactive oxygen species and antioxidant systems). An increase in some mitotic aberrations was also observed in the root tissues of both species. The results indicate that the sensitivity of lentil in this bioassay is higher for lower concentrations compared to onion. Also, lower concentrations of these REEs had a positive effect on the growth rate of lentils underlining the complex interaction occurring between REEs and different plant organisms.
Introduction
Rare earth elements (REEs) are metals of the lanthanoid series in the periodic table. They are naturally present at low concentrations in soil, water and atmosphere, where accumulation can occur following anthropogenic inputs from agriculture, animal husbandry and wastes of industrial applications (Tommasi and d’Aquino, 2017). Though they are termed “rare”, REEs are, in fact, commonly found in soils worldwide (Hu et al., 2006); the classification of “rare” solely refers to the lack of large deposits or ores that are characteristic of other elements such as silver and gold (Carpenter et al., 2015). REEs are not individual native metals, but they occur together in numerous ore/accessory minerals as minor or major constituents. Sites in areas impacted by mining activities, not only related to REEs extraction and industry, have been shown to contain REEs with concentrations up to 100 times higher than normal background levels (Gwenzi et al., 2018). In recent decades, the utilization of REEs in a broad array of industrial processes made REEs indispensable ingredients in many technological applications and their utilization in agriculture, animal husbandry and medicine are also well known (Tommasi and d’Aquino, 2017). It was reported that approximately 85 tonnes of neodymium (Nd) were released into the environment from phosphate fertilizer production in the Netherlands in 1994 (Sneller et al., 2000). Currently, REEs do not present any threshold limit values for national and international regulations, but they can be considered as new emerging contaminants with still unknown effects (Galdiero et al., 2019). In a study conducted by Li et al. (2010), it was found that REEs soil pollution due to tailings from a REEs processing plant in China can travel up to approximately 7 km before soil concentrations stabilize to natural levels. Specifically, neodymium (Nd) and praseodymium (Pr) levels concerning the source were found to be, respectively, 5726 and 1614 mg/kg at 0.4 km, 2266 and 650 mg/kg at 0.8 km, 1279 and 373 mg/kg at 1.3 km and 310 and 85 mg/kg at 2.1 km (Li et al., 2010). It was also reported that soils in polluted sites near industrial locations in the Netherlands contain high levels of REEs [800–900 mg/kg cerium (Ce), 500–700 mg/kg lanthanum (La), 400 mg/kg Nd and 100 mg/kg Pr], which are at least 100 times higher than background levels (Sloof et al., 1993).
Although relatively scarce information is available to date on REEs-associated biological effects, including bioassays on model organisms and human health effects (Pagano et al., 2015a; Pagano et al., 2015b; Gravina et al., 2018; Galdiero et al., 2019), an increasing amount of data about the biological effects of REEs including their potential genotoxicity, often controversial, are available from the 1980s about the effects on terrestrial plants (Lopez-Moreno et al., 2010; He and Loh 2000, 2002). Their mechanisms of action and behavior in biological systems are far from being completely understood, but it seems dependent on their concentration and physicochemical conditions of the exposure media. Similarities in their mode of action were evidenced, but not univocally (Siciliano et al., 2021). Previous studies suggested that plants can absorb REEs due to the similar ionic radii these elements share with calcium (Ca) (Pickard, 1970; Hu et al., 2004). Perturbations of the primary metabolisms, including photosynthesis, respiration and photorespiration, occur under environmental stresses, inevitably leading to enhanced production of reactive oxygen species (ROS) (Mittler et al., 2004; Shigeoka and Maruta, 2014). In addition, different authors reported that Ce enhanced photosynthesis (Fashui et al., 2002; Xiaoqing et al., 2009; Yuguan et al., 2009), mitochondrial activity (Dai et al., 2011) and nitrogen metabolism (Weiping et al., 2003). An increase in ROS and inhibition of several antioxidants following Ce treatments was observed in rice (Xu and Chen, 2011) and impairment in macronutrient metabolism has been reported in horseradish (Guo et al., 2007). Therefore, there is strong evidence that REEs can be responsible for oxidative stress in plants and induce a defense response (Zicari et al., 2018). Plants are the most important source of antioxidants that are useful to counteract the ROS produced in plant metabolism and particularly over-generated in response to biotic and abiotic stress. Between them, the components of the ascorbate-glutathione pathway participate in ROS-scavenging (Paradiso et al., 2016; Hasanuzzaman et al., 2019) in which the ascorbate (ASC) is a key component. Few data are available on the potential genotoxic effects of REEs on plants.
The aim of this work was to provide new data on the responses of lentil and onion to Ce and Nd exposure in order to clarify the potential toxicity and genotoxicity of these elements. Rate growth, with particular attention to the root and aerial part, cell division, mitotic aberrations and the role of antioxidant components, were investigated to shed light on the stress response induced by Ce and Nd on these plant species.
Materials and methods
Chemicals
All reagents used in this study were of the highest grade available. They were purchased from Sigma Aldrich Merck Life Science (Milan, Italy) and used without further purification. The chloride hydrate forms of Ce and Nd (Table 1) were selected as the source compound of the REE due to their high solubility in water. Both were dissolved in ultrapure water produced by a Milli-Q system (Millipore, Bedford, MA, United States) to reach the final concentration.
Plant material and experimental setup
Lentil (Lens culinaris Medik. cv. Eston) seeds were sanitized with 0.5% (v/v) sodium hypochlorite solution for 15 min (ISTA, 2012), then thoroughly washed and soaked in distilled water for 20 min (Ghassemi-Golezani et al., 2008). The seeds were allowed to germinate on filter paper (Whatman grade 1) in Petri dishes (12 cm diameter) at 24 ± 1°C and 55–60% relative humidity in the dark. Seedlings were transferred 2 days after germination to a hydroponic set-up in which roots emerged in REEs solution 5, 25, 50 and 100 μM for both Ce and Nd for 72 h before subsequent analyses. Control seedlings were grown in distilled water. Onion (Allium cepa L.) bulbs were grown in distilled water at room temperature according to Ping et al. (2012) and as roots reached 3 cm in length, bulbs were treated with Ce and Nd 0.1 and 1 mM for 48 h. Control bulbs were treated with distilled water.
Mitotic aberrations
Sample preparation was carried out as reported by Sharma and Sharma (1980). Briefly, root tip was washed with distilled water and hydrolyzed with 1 N HCl at 70°C for 5 min, root apex first was excised and treated with aceto orcein 1% for 2 min then squashed with a metal rod and treated again with aceto orcein 1% for 2 min. Photomicrographs were taken and a minimum of 300 cells per slide were analyzed. Three slides were observed for each treatment and for each sample. Mitotic index, micronucleus in interphase and chromosome aberrations in mitotic phases were determined.
The mitotic index was culculated according to Fiskesjö, (1985) as follows:
Mitotic index = number of cells in mitosis/total number of cells.
Anomalies were calculated according to Salazar-Mercado et al. (2019) as follows:
Relative abnormality rate = (Total number of abnormal cells/Total number of cells observed) × 100.
Ascorbate and dehydroascorbate content
Treated and control seedlings (0.5 g) were homogenized with cold 5% metaphosphoric acid in a 1:4 ratio in a porcelain mortar. The homogenate was centrifuged for 15 min at 20,000 × g (4°C) and the supernatant was collected and immediately assayed for ASC and dehydroascorbate according to Loi et al. (2020).
Lipid peroxidation analysis and H2O2 content
For lipid peroxidation, plant material was homogenized with four volumes of 0.1% (w/v) trichloroacetic acid (TCA). The homogenate was centrifuged at 12,000 × g, for 10 min, at 4°C. 1 ml of the supernatant was mixed with 1 ml of 20% TCA containing 0.5% (w/v) thiobarbituric acid (TBA). The level of lipid peroxidation was evaluated in terms of malondialdehyde (MDA) content determined by the TBA reaction. Intracellular H2O2 concentration was evaluated according to Villani et al. (2021).
Statistical analysis
The data presented are the mean of at least three different replicates of three independent experiments. All analyses were run separately for each REE evaluated. One-way ANOVA followed by Dunnett’s multiple comparisons test was performed using GraphPad Prism version 9.0 (GraphPad Software, San Diego, CA, United States). Statistical significance was accepted at p < 0.01 (*), p < 0.001 (**) and p < 0.0001 (***).
Results
Plant growth
Ce and Nd concentrations were chosen for onion according to preliminary results from Romero-Freire et al. (2021) and for lentil after preliminary trials suggesting that concentrations greater than 100 μM were not tolerated by lentil (data not shown). The analyses on onion were performed at higher concentrations. Mobility and bioavailability of lanthanides (Ln) are influenced by factors such as pH, concentration and type of organic and inorganic ligands and temperature. The nitrates, chlorides and sulfates of Ln are soluble, while their carbonates, phosphates and hydroxides are insoluble (Wells and Wells, 2001). Commonly, Ln are present in the trivalent oxidation states (Cotton, 1991), but extreme ecological conditions can change the redox state of elements such as Ce or Eu. In these experiments, the species of REE most bioavailable created after calculating speciation using PHREEQC were Ln+3 (Supplementary Table S1).
Table 2 reports the length of root and aerial part of Ce- and Nd-treated lentil seedlings. Generally, the exposure to both treatments resulted in a significant reduction of root and aerial part lengths, except at 5 µM where the root length did not significantly differ with respect to the control and the aerial part had grown higher (Table 2 and Figure 1). Both root and aerial part showed significant inhibition of elongation at the highest concentration (100 µM) of Ce and Nd. As regards onion, the treatment with REEs at both concentrations, caused a significant reduction of the length of the root compared to the control, with a higher value at 1 mM. Differently by the lentils, in onion, the inhibition of root length at a concentration of 0.1 mM, was found lower in Ce than Nd (Table 2).
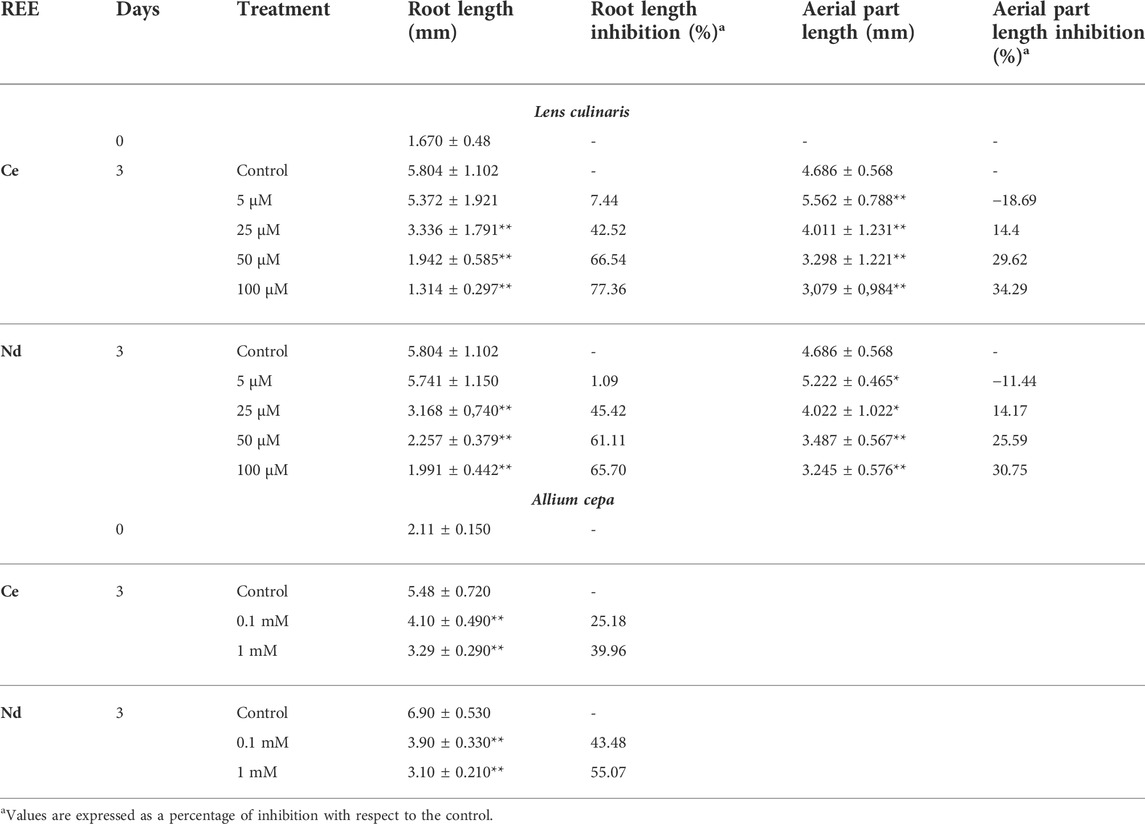
TABLE 2. Effect of different concentrations of Cerium (Ce) and Neodymium (Nd) on root and aerial part length in lentil and on root length in onion. Values are expressed as average ±standard deviation (n = 43).* Statistical significance at p < 0.01 (*), p < 0.001 (**).
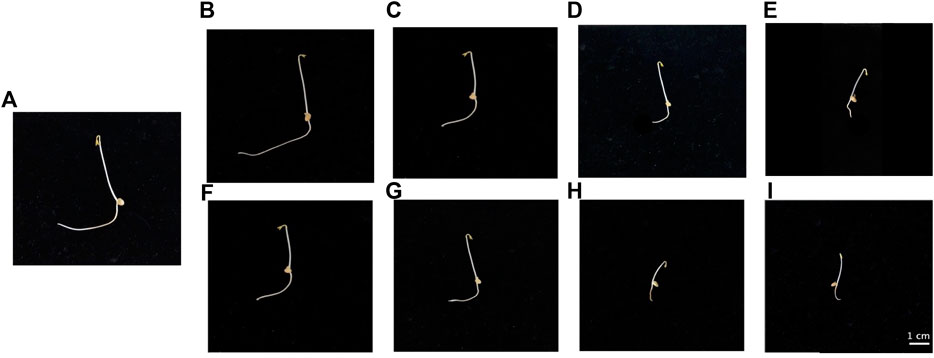
FIGURE 1. Evolution of root length in lentil seedlings following treatment with Ce [(B) 5 μM, (C) 25 μM, (D) 50 μM, (E) 100 µM] and Nd [(F) 5 μM, (G) 25 μM, (H) 50 μM, (I) 100 µM] for 3 days. (A), control.
Genotoxicity and mitotic abnormalities
Four types of mitotic abnormalities were observed (Figure 2) and no stickiness was observed in control and 100 μM Ce. Similarly, vagrant chromosomes in metaphase were the less frequent abnormality, absent with 0, 5 and 25 µM Ce and low value at higher concentrations (50 and 100 µM). In Nd treatment instead, except for 5 μM, with the increment of its concentration, a progressive increase of this abnormality was observed with the maximum value at 100 µM (Table 3). A significant abnormality is the anaphase bridge; generally, both Ce and Nd, caused an increase in their number with the increment of REE concentration utilized, with a minimum value of 1 and a maximum of 24 in presence of Ce respectively at 5 and 100 µM and no bridge was observed at 5 µM Nd. The C-mitosis is the most frequent anomaly in lentil treated with Ce, since all the treatments presented it (with a maximum of 41 at 100 µM) except for the 5 µM.
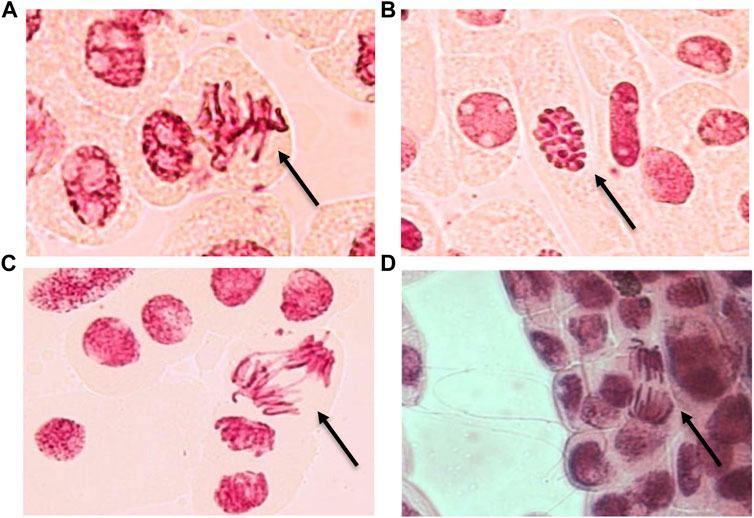
FIGURE 2. Mitotic aberrations observed in onion meristematic cells exposed to Ce (A–C) and Nd (D) at 100 µM. (A) sticky chromosome, (B) C-mitosis, (C) bridge and (D) vagrant chromosome. Magnification ×400.
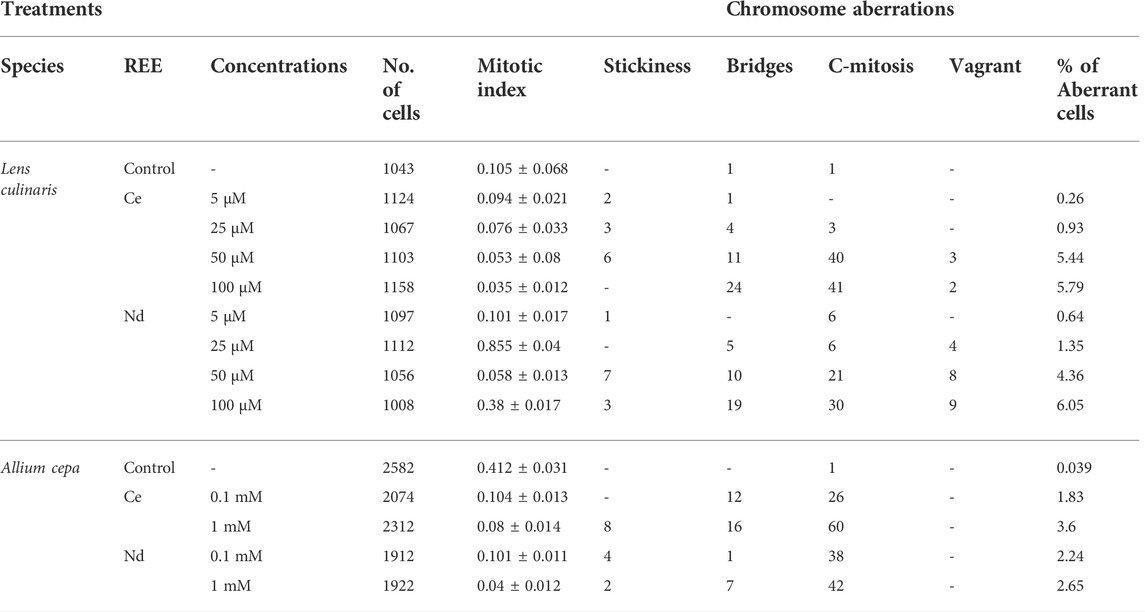
TABLE 3. Cytogenetic analysis of onion and lentil root tips exposed to different concentrations of REE; total cell number, mitotic index and percentage of aberrations after 72 h of treatment with Ce and Nd, and control. The values represent the mean of three experiments ±SD.
Onion seemed to be less sensitive to Ce and Nd than lentil since at lower concentrations (5, 25 and 50 µM) less chromosome aberrations were observed (data not shown). It showed less frequency in the abnormalities and only to higher Ce and Nd concentrations (Table 3). Indeed, onion did not present vagrant chromosomes, although there were observed all other types of chromosome aberrations. Generally, a tendency was observed in which 1 mM treatment of both Ce and Nd caused the highest frequency of abnormalities. 0.1 mM did not cause stickiness when treated with Ce, although 1 mM presented a value of 8 (Table 3). On the other hand, both Nd concentrations produced stickiness, vagrant and bridge chromosomes. Finally, the C-mitosis as observed in lentil root was the most frequent anomaly, with a maximum at 1 mM Ce.
Ascorbate and dehydroascorbate content in lentil seedlings
The ASC content in lentil root significantly increased following exposition to 25 and 100 μM Ce and decreased following exposition to 5 and 50 μM Ce (Figure 3A) whereas in the aerial part a significant increase until 25 µM occurred and a decrease was observed at 50 and 100 µM (Figure 3B). DHA increased significantly at all concentrations in both root and aerial part (Figures 3A,B). Consequently, the ASC/ASC + DHA ratio (Figure 3E) significantly decreased in root at 5, 50 and 100 µM and in aerial part at 25, 50 and 100 µM with respect to the control. A different trend of ASC was observed for Nd treatment (Figures 3C,D). A significant decrease in ASC content was recorded in root and aerial part treated at all concentrations compared to the control. Significant effects on DHA levels on root and aerial part were detected at all concentrations of Nd as compared to the control. The ASC/ASC + DHA ratio (Figure 3F), therefore, significantly decreased in root at all concentrations and in aerial part starting at 25 µM.
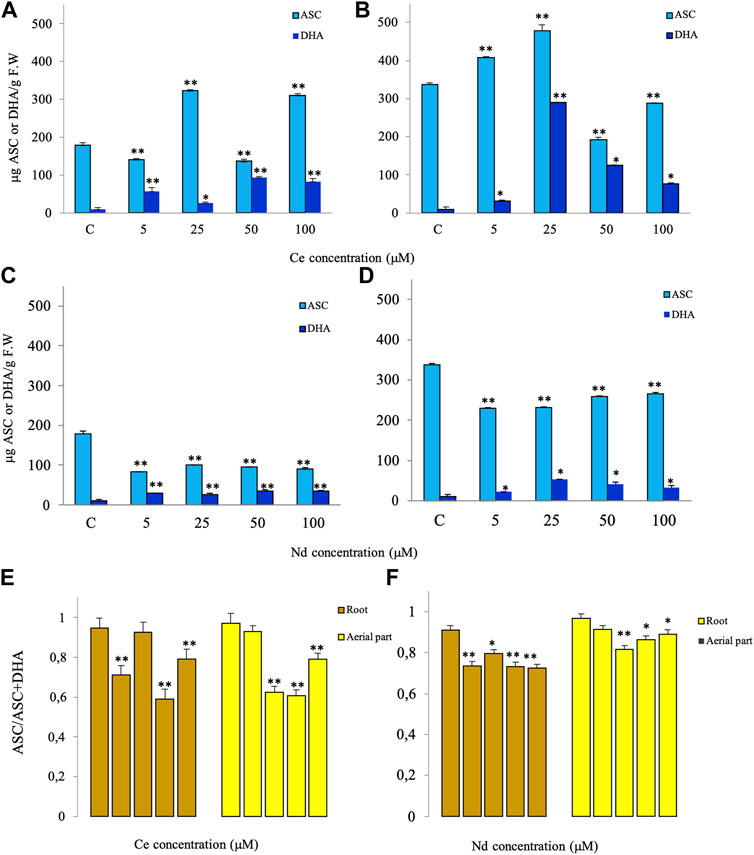
FIGURE 3. Effects of different concentrations of Ce (A,B) and Nd (C,D) on the contents of ascorbate (ASC) and dehydroascorbate (DHA) after 3 days of treatment in roots (A,C) and aerial parts (B,D) and the ratio of ASC/ASC + DHA (E,F) of lentils. Vertical bars indicate the SD of three replicates in each treatment group. * Statistical significance at p < 0.01 (*), p < 0.001 (**), and p < 0.0001 (***). F.W, fresh weight.
Hydrogen peroxide content and lipid peroxidation levels in lentil seedlings
The hydrogen peroxide content increased significantly in root treated with Ce or Nd at concentrations of 25, 50 and 100 µM and in the aerial part when treated with Ce at 5 µM and Nd at 100 µM (Figures 4A,B), as compared to the control. The malondialdehyde content, index of level peroxidation of biological membranes, significantly increased in root at all Ce concentrations being significant at 5, 50 and 100 μM, whereas in the aerial part an increase and decrease were registered to Ce 50 and 100 μM, respectively (Figure 4C), with respect to the control. As regards Nd, it induced a significant increase at 100 µM in root and at 25 and 50 µM in the aerial part (Figure 4D).
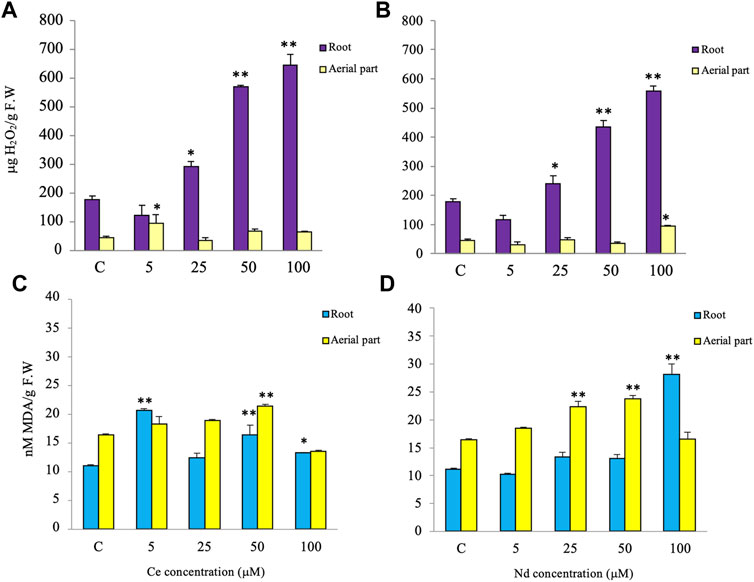
FIGURE 4. Effects of different Ce and Nd concentrations on the hydrogen peroxide (H2O2) (A,B) and malondialdehyde (MDA) (C,D) contents after 3 days of treatment in roots and aerial parts of lentils. Vertical bars indicate the SD of three replicates in each treatment group. * Statistical significance at p < 0.01 (*), p < 0.001 (**), and p < 0.0001 (***). F.W, fresh weight.
Discussion
Over the last decades, particular interest has been focused on the biological effects of the REEs. Although some REEs have been described over the years, many of them lack some knowledge of the cytotoxic effects on plant cells and the molecular mechanisms that are the basis of their toxicity. Some data on sea urchins indicate negative effects of REEs on sperm fertility and embryo development and an increase in toxicity according to the molecular mass of the elements (Oral et al., 2017; Trifuoggi et al., 2017; Gravina et al., 2018). On the other hand, genetic tests with lanthanum (La) nitrate on mice seem to exclude La as a genotoxic hazard (Yang et al., 2016). In plants, physiological effects on the photosynthetic process, growth, enzymes and proteins have been reported (Pickard, 1970; Babula et al., 2008; Xiaoqing et al., 2009). It has been documented the presence of REEs in both the roots and shoots of various plant species growing in soils containing low, natural levels of the REEs (Markert and Li, 1991; Fu et al., 1998; Tyler and Olsson, 2005). This is indicative that REEs can be accumulated in vegetables and horticultural crops and potentially be toxic for humans and animals. To elucidate the mechanism of action of REEs in plants, which is still poorly understood (Yang et al., 2015), concentrations higher than natural levels of REEs were utilized. In this study, therefore, we analyzed the effect of various concentrations of Ce and Nd on plant growth, including mitotic index and mitotic abnormalities and cellular oxidative stress by analyzing the role of ASC, a defense and antioxidant metabolite, in the detoxification process of H2O2 and lipid peroxidation induced by the two REEs.
The two plant species used in this work showed significant inhibition of root elongation. Lentil and onion, however, showed a different tolerance to Ce and Nd, higher in onion (mM in onion vs. µM concentration in lentil). This can be due to different plant tissue utilized (seedlings for lentils and bulbs for onion) but generally presupposes that each plant species can respond differently to REEs (Tommasi and d’Aquino, 2017).
The mitotic index, which reflects the frequency of cell division, is a generally accepted measure of cytotoxicity for all living organisms (d’Aquino et al., 2009; Çavuşoğlu et al., 2012). In our experimental conditions to higher concentrations of Ce and Nd, the mitotic index significantly decreased (Table 3) and dramatically inhibited the root growths of both onion and lentil (Figure 1). Chromosome anomalies are alterations or disorders induced by various agents (Datta et al., 2018). Their frequency is correlated to the concentration of genotoxic agents (Fatma et al., 2018). The observation of chromosome aberrations in root tip cells, such as sticky chromosomes, vagrant chromosomes, bridges, and C-mitosis (Figure 2), with a higher frequency, indicates that higher concentrations of Ce and Nd might cause breakage of the spindle fiber. Therefore, chromosome aberration and the decreases in mitotic index may be major factors in inhibiting root growths of onion exposed to higher concentrations of Ce and Nd. Similarly, cadmium may induce chromosome bridges, stickiness and fragment during mitosis of Allium cepa var. agrogarum L. (Zou et al., 2012; Wang et al., 2014) and treatment with La leads to the appearance of binuclear cells in soybean (de Oliveira et al., 2015). Decreased mitotic activity and increased aberration rates were found also in Paracentrotus lividus embryos reared in Dy, Ho, Er, Yb, Lu (Oral et al., 2017).
The sensitivity to REEs depends from the organism. In sea urchins, negative effects are present at lower concentrations (Oral et al., 2017) respect to those used in this study. Treated at 5 μM, there is no inhibition of the growth in the root, but there is a significant increment in the rate of mitotic aberrations. At 5 μM, the absence of inhibition in lentil root with the higher growth of the aerial part can be explained as hormesis of REEs, as already reported for human effect and sea urchins. Hormetic effects induced by REEs (Park et al., 2008; Calabrese, 2010; Calabrese, 2013; Pagano et al., 2015a) suggest that REEs can exert a protective effect at low concentrations and a toxic effect at higher concentrations. La and Ce exhibit high cytotoxic effects at concentrations of 10–200 and 5–50 mg/L respectively in onion root suggesting that in areas with high soil concentrations of REEs, their effect on the biota must be carefully monitored. In addition, trials on Vicia faba showed that REEs are toxic in water solutions, but the toxicity was mitigated in presence of nutrients (Romero-Freire et al., 2021). Genotoxic effects of Ce oxide micro and nanoparticles in onion roots were reported by (Liman et al. (2019).
The higher H2O2 content and peroxidation level in the roots than in aerial parts of lentils treated with Ce is indicative of a higher oxidative status, probably involved in the higher inhibition of root growth with respect to the aerial part. Generally, the increased ASC observed in both roots and aerial parts highlights that the seedlings attempt to counteract the oxidative stress induced by Ce. Indeed, it is known that ASC, a key molecule in the plant antioxidant system, can directly block the radical species (Paciolla et al., 2019), including the ROS, such as hydrogen peroxide that at high concentrations, are reported to be toxic for the cell (Wojtyla et al., 2016). In addition, due to its redox potential (from +0.40 to +0.50 V) (Matsui et al., 2015; Paciolla et al., 2019), ASC is known as an antioxidant compound involved in the maintenance of cellular redox balance. However, it is observed a concomitant increase in the oxidized form of ASC, DHA, which to a high level is reported to be toxic for the cell and may inhibit the root growth (Paciolla et al., 2001). Additionally, DHA can be alternatively reduced by ferredoxin-, glutathione- and NAD (P) H-dependent pathways underlying a metabolic link between the NAD (P)-dependent redox system and the low molecular weight antioxidant compounds (Sano, 2017). The DHA trend, therefore, brings to shift the ratio ASC/ASC plus DHA towards the oxidized form possibly causing a perturbation of the cell redox state. A higher cell oxidative condition was detected in lentil seedlings treated with Nd compared to Ce. The lower ASC level and the contemporaneous higher DHA content caused a stronger imbalance of the redox ratio of the couple ASC-DHA that together with the higher lipid peroxidation and hydrogen peroxide levels caused a higher cell oxidative stress. Indeed, has been reported that the treatment of REEs could change the activity of the redox system and the permeability of the plasma membrane in the plant cell, leading to the damage of the plasma membrane (Zheng et al., 2002; Peng et al., 2007; Zicari et al., 2018) and a significant increase in ROS and MDA levels were found in embryos of P. lividus treated with Ce (Oral et al., 2017).
All these data indicate that at higher concentrations both plant species suffered despite the antioxidative response being alerted. The increase of REEs in the environment is consistent with a potential accumulation and an increase of toxicity in different organisms. Further biochemical and physiological studies are needed to better understand the specific mechanisms that are the basis of these complex responses. For instance, a deep study of the defense systems such as the ascorbate-glutathione cycle and other antioxidant systems (catalase, peroxidases, phenols, vitamins, pathogenesis-related proteins) could be highly useful. This will allow to understand the action mechanisms of Ce and Nd and to identify potential bioactive compounds able to minimize or neutralize the effects of these REEs and counteract their toxicity.
Data availability statement
The original contributions presented in the study are included in the article/Supplementary Material, further inquiries can be directed to the corresponding author.
Author contributions
IG, CP, and FT contributed to the study’s conception and design. Material preparation, data collection and analysis were performed by IG and SD for lentils and ND for onion The first draft of the manuscript was written by IG; IG, FT, and CP did the writing, review and editing. FT and CP supervisor and funding acquisition. All authors read and approved the final manuscript.
Funding
This project has received funding from European Union’s Horizon 2020 research and innovation program under the Marie Sklodowska-Curie Grant Agreement N°857989.
Conflict of interest
The authors declare that the research was conducted in the absence of any commercial or financial relationships that could be construed as a potential conflict of interest.
Publisher’s note
All claims expressed in this article are solely those of the authors and do not necessarily represent those of their affiliated organizations, or those of the publisher, the editors and the reviewers. Any product that may be evaluated in this article, or claim that may be made by its manufacturer, is not guaranteed or endorsed by the publisher.
Supplementary material
The Supplementary Material for this article can be found online at: https://www.frontiersin.org/articles/10.3389/fenvs.2022.969162/full#supplementary-material
References
Babula, P., Adam, V., Opatrilova, R., Zehnalek, J., Havel, L., Kizek, R., et al. (2008). Uncommon heavy metals, metalloids and their plant toxicity: a review. Environ. Chem. Lett. 6, 189–213. doi:10.1007/s10311-008-0159-9
Calabrese, E. J. (2010). Hormesis is central to toxicology, pharmacology and risk assessment. Hum. Exp. Toxicol. 29, 249–261. doi:10.1177/0960327109363973
Calabrese, E. J. (2013). Hormetic mechanisms. Crit. Rev. Toxicol. 43, 580–606. doi:10.3109/10408444.2013.808172
Carpenter, D., Boutin, C., Allison, J. E., Parsons, J. L., and Ellis, D. M. (2015). Uptake and effects of six rare earth elements (REEs) on selected native and crop species growing in contaminated soils. PLoS ONE 10 (6), e0129936. doi:10.1371/journal.pone.0129936
Çavuşoğlu, K., Kaya, A., Yilmaz, F., and Yalçin, E. (2012). Effects of cypermethrin on Allium cepa. Environ. Toxicol. 27, 583–589. doi:10.1002/tox.20681
Dai, J., Liu, J. J., Zhou, G. Q., Zhang, Y. Z., and Liu, Y. (2011). Effect of Ce(III) on heat production of mitochondria isolated from hybrid rice. Biol. Trace Elem. Res. 143, 1142–1148. doi:10.1007/s12011-010-8902-z
d’Aquino, L., de Pinto, M. C., Nardi, L., Morgana, M., and Tommasi, F. (2009). Effect of some light rare earth elements on seed germination, seeding growth and antioxidant metabolism in Triticum durum. Chemosphere 75, 900–905. doi:10.1016/j.chemosphere.2009.01.026
Datta, R. R., Ling, J., Kurland, J., Ren, X., Xu, Z., Yucel, G., et al. (2018). A feed-forward relay integrates the regulatory activities of bicoid and orthodenticle via sequential binding to suboptimal sites. Genes Dev. 32, 723–736. doi:10.1101/gad.311985.118
de Oliveira, C., Ramos, S. J., Siqueira, J. O., Faquin, V., de Castro, E. M., Amaral, D. C., et al. (2015). Bioaccumulation and effects of lanthanum on growth and mitotic index in soybean plants. Ecotoxicol. Environ. Saf. 122, 136–144. doi:10.1016/j.ecoenv.2015.07.020
Fashui, H., Ling, W., Xiangxuan, M., Zheng, W., and Guiwen, Z. (2002). The effect of cerium (III) on the chlorophyll formation in spinach. Biol. Trace Elem. Res. 89, 263–276. doi:10.1385/bter:89:3:263
Fatma, F., Verma, S., Kamal, A., and Srivastava, A. (2018). Monitoring of morphotoxic, cytotoxic and genotoxic potential of mancozeb using allium assay. Chemosphere 195, 864–870. doi:10.1016/j.chemosphere.2017.12.052
Fiskesjö, G. (1985). The Allium test as a standard in environmental monitoring. Hereditas 102, 99–112. doi:10.1111/j.1601-5223.1985.tb00471.x
Fu, F., Akagi, T., and Shinotsuka, K. (1998). Distribution pattern of rare earth elements in fern: implication for intake of fresh silicate particles by plants. Biol. Trace Elem. Res. 64, 13–26. doi:10.1007/bf02783321
Galdiero, E., Carotenuto, R., Siciliano, A., Libralato, G., Race, M., Lofrano, G., et al. (2019). Cerium and erbium effects on daphnia magna generations: a multiple endpoints approach. Environ. Pollut. 254, 112985. doi:10.1016/j.envpol.2019.112985
Ghassemi-Golezani, K., Aliloo, A. A., Valizadeh, M., and Moghaddam, M. (2008). Effects of hydro and osmo-priming on seed germination and field emergence of lentil (Lens culinaris Medik.). Not. Bot. Horti Agrobot. Cluj-Napoca 36 (1), 29–33. doi:10.15835/nbha36186
Gravina, M., Pagano, G., Oral, R., Guida, M., Toscanesi, M., Siciliano, A., et al. (2018). Heavy rare earth elements affect Sphaerechinus granularis sea urchin early life stages by multiple toxicity endpoints. Bull. Environ. Contam. Toxicol. 100, 641–646. doi:10.1007/s00128-018-2309-5
Guo, X., Zhou, Q., Lu, T., Fang, M., and Huang, X. (2007). Distribution and translocation of 141 Ce(III) in horseradish. Ann. Bot. 100, 1459–1465. doi:10.1093/aob/mcm244
Gwenzi, W., Mangori, L., Danha, C., Chaukura, N., Dunjana, N., Sanganyado, E., et al. (2018). Sources, behaviour, and environmental and human health risks of high-technology rare earth elements as emerging contaminants. Sci. Total Environ. 636, 299–313. doi:10.1016/j.scitotenv.2018.04.235
Hasanuzzaman, M., Bhuyan, M. H. M. B., Anee, T. I., Parvin, K., Nahar, K., Mahmud, J. A., et al. (2019). Regulation of ascorbate-glutathione pathway in mitigating oxidative damage in plants under abiotic stress. Antioxidants 8 (9), 384. doi:10.3390/antiox8090384
He, Y. W., and Loh, C. S. (2000). Cerium and lanthanum promote floral initiation and re- productive growth of Arabidopsis thaliana. Plant Sci. 159, 117–124. doi:10.1016/s0168-9452(00)00338-1
He, Y. W., and Loh, C. S. (2002). Induction of early bolting in Arabidopsis thaliana by triacontanol, cerium and lanthanum is correlated with increased endogenous concentration of isopentenyl adenosine(iPAdos). J. Exp. Bot. 53 (368), 505–512. doi:10.1093/jexbot/53.368.505
Hu, Z., Haneklaus, S., Sparovek, G., and Schnug, E. (2006). Rare earth elements in soils. Commun. Soil Sci. Plant Anal. 37, 1381–1420. doi:10.1080/00103620600628680
Hu, Z., Richter, H., Sparovek, G., and Schnug, E. (2004). Physiological and biochemical effects of rare earth elements on plants and their agricultural significance: a review. J. Plant Nutr. 27 (1), 183–220. doi:10.1081/pln-120027555
ISTA (International Seed Testing Association) (2012). International rules for seed testing, 31. Zürich: Seed Science and Technology.
Li, J., Hong, M., Yin, X., and Liu, J. (2010). Effects of the accumulation of the rare earth elements on soil macrofauna community. J. Rare Earths 28 (6), 957–964. doi:10.1016/s1002-0721(09)60233-7
Liman, R., Acikbas, Y., and Ciğerci, İ. H. (2019). Cytotoxicity and genotoxicity of cerium oxide micro and nanoparticles by allium and comet tests. Ecotoxicol. Environ. Saf. 168, 408–414. doi:10.1016/j.ecoenv.2018.10.088
Loi, M., De Leonardis, S., Mulè, G., Logrieco, A. F., and Paciolla, C. (2020). A novel and potentially multifaceted dehydroascorbate reductase increasing the antioxidant systems is induced by beauvericin in tomato. Antioxidants (Basel, Switz. 9 (5), 435. doi:10.3390/antiox9050435
Lopez-Moreno, M. L., de la Rosa, G., De Hernandez Viezcas, G., Peralta Videa, J., and Guardea Torresdey, J, J. (2010). X-ray Absorption Spectroscopy (XAS) corroboration of the uptake and storage of CeO2 nanoparticles and assessment of their differential toxicity in four edible plant species. J. Agric. Food Chem. 58 (6), 3689–3693. doi:10.1021/jf904472e
Markert, B., and Li, Z. D. (1991). Natural background concentrations of rare-earth elements in a forest ecosystem. Sci. Total Environ. 103, 27–35. doi:10.1016/0048-9697(91)90350-n
Matsui, T., Kitagawa, Y., Okumura, M., and Shigeta, Y. (2015). Accurate standard hydrogen electrode potential and applications to the redox potentials of vitamin C and NAD/NADH. J. Phys. Chem. A 119, 369–376. doi:10.1021/jp508308y
Mittler, R., Vanderauwera, S., Gollery, M., and Van Breusegem, F. (2004). Reactive oxygen gene network of plants. Trends Plant Sci. 9 (10), 490–498. doi:10.1016/j.tplants.2004.08.009
Oral, R., Pagano, G., Siciliano, A., Gravina, M., Palumbo, A., Castellano, I., et al. (2017). Heavy rare earth elements affect early life stages in Paracentrotus lividus and Arbacia lixula sea urchins. Environ. Res. 154, 240–246. doi:10.1016/j.envres.2017.01.011
Paciolla, C., De Tullio, M. C., Chiappetta, A., Innocenti, A. M., Bitonti, M. B., Liso, R., et al. (2001). Short- and long-term effects of dehydroascorbate in Lupinus albus and Allium cepa roots. Plant Cell Physiol. 42, 857–863. doi:10.1093/pcp/pce113
Paciolla, C., Fortunato, S., Dipierro, N., Paradiso, A., De Leonardis, S., Mastropasqua, L., et al. (2019). Vitamin C in plants: from functions to biofortification. Antioxidants 8, 519. doi:10.3390/antiox8110519
Pagano, G., Aliberti, F., Guida, M., Oral, R., Siciliano, A., Trifuoggi, M., et al. (2015a). Rare earth elements in human and animal health: state of art and research priorities. Environ. Res. 142, 215–220. doi:10.1016/j.envres.2015.06.039
Pagano, G., Guida, M., Tommasi, F., and Oral, R. (2015b). Health effects and toxicity mechanisms of rare earth elements- Knowledge gaps and research prospects. Ecotoxicol. Environ. Saf. 115, 40–48. doi:10.1016/j.ecoenv.2015.01.030
Paradiso, A., Caretto, S., Leone, A., Bove, A., Nisi, R., De, Gara L., et al. (2016). ROS production and scavenging under anoxia and Re-oxygenation in arabidopsis cells: a balance between redox signaling and impairment. Front. Plant Sci. 7, 1803. doi:10.3389/fpls.2016.01803
Park, E. J., Choi, J., Park, Y. K., and Park, K. (2008). Oxidative stress induced by cerium oxide nanoparticles in cultured BEAS-2B cells. Toxicology 245, 90–100. doi:10.1016/j.tox.2007.12.022
Peng, L., Zhang, W. Y., Xi, L., and Yi, L. (2007). Structural basis for the biological effects of Pr(III) ions: alteration of cell membrane permeability. Biol. Trace Elem. Res. 120, 141–147. doi:10.1007/s12011-007-8015-5
Pickard, B. G. (1970). Comparison of calcium and lanthanon Ions in the Avena-coleoptile growth test. Planta 91, 314–320. doi:10.1007/BF00387504
Ping, K. Y., Darah, I., Yusuf, U. K., Yeng, C., and Sasidharan, S. (2012). Genotoxicity of Euphorbia hirta and Allium cepa assay. Molecules 17, 7782–7791. doi:10.3390/molecules17077782
Romero-Freire, A., González, V., Groenenberg, J. E., Qiu, H., Auffan, M., Cotelle, S., et al. (2021). Cytotoxicity and genotoxicity of lanthanides for Vicia faba L. are mediated by their chemical speciation in different exposure media. Sci. Total Environ. 790, 148223. doi:10.1016/j.scitotenv.2021.148223
Salazar-Mercado, S. A., Quintero-Caleño, J. D., and Rojas-Suàrez, J. P. (2020). Cytogenotoxic effect of propanil using the Lens culinaris Med and Allium cepa L test. Chemosphere 249, 126193. doi:10.1016/j.chemosphere.2020.126193
Sano, S. (2017). “Molecular and functional characterization of monodehydroascorbate and dehydroascorbate reductases,” in Ascorbic acid in plant growth, development and stress tolerance. Editors M. Hossain, M. Sergi, J. David, D. Pedro, M. Fujita, and A. Lorence (Cham, Switzerland: Springer), 129–156. doi:10.1007/978-3-319-74057-7_5
Sharma, A. K., and Sharma, A. (1980). Chromosome technique theory and practice. 3rd ed. London, UK: Butterworths, 474.
Shigeoka, S., and Maruta, T. (2014). Cellular redox regulation, signaling, and stress response in plants. Biosci. Biotechnol. Biochem. 78, 1457–1470. doi:10.1080/09168451.2014.942254
Siciliano, A., Guida, M., Pagano, G., Trifuoggi, M., Tommasi, F., Lofrano, G., et al. (2021). Cerium, gadolinium, lanthanum, and neodymium effects in simplified acid mine discharges to Raphidocelis subcapitata, Lepidium sativum, and Vicia faba. Sci. Total Environ. 787, 147527. Article 147527. doi:10.1016/j.scitotenv.2021.147527
Slooff, W., Bont, P. F. H., van den Hoop, M. A. G. T., Janus, J. A., and Annema, J. A. (1993). Exploratory report: Rare earth metals and their compounds. Bilthoven, Netherlands: National Institute of Public Health and Environmental Protection. Report No. 710401025.
Sneller, F. E. C., Kalf, D. F., Weltje, L., and Van Wezel, A. P. (2000). Maximum permissible concentrations and negligible concentrations for rare earth elements (REEs). Bilthoven, Netherlands: National Institute of public health and the Environment. RIVM Report 601501 011.
Tommasi, F., and d’Aquino, L. (2017). “Rare earth elements and plants,” in Rare earth elements in human and environmental health: At the crossroad between toxicity and safety. Editors G. Pagano (Singapore: Pan Stanford Publishing Pte. Ltd.), 107.
Trifuoggi, M., Pagano, G., Guida, M., Palumbo, M., Siciliano, A., Gravina, M., et al. (2017). Comparative toxicity of seven rare earth elements in sea urchin early life stages. Environ. Sci. Pollut. Res. 24, 20803–20810. doi:10.1007/s11356-017-9658-1
Tyler, G., and Olsson, T. (2005). Rare earth elements in forest-floor herbs as related to soil conditions and mineral nutrition. Biol. Trace Elem. Res. 106, 177–192. doi:10.1385/bter:106:2:177
Villani, A., Tommasi, F., and Paciolla, C. (2021). The arbuscular mycorrhizal fungus glomus viscosum improves the tolerance to verticillium wilt in artichoke by modulating the antioxidant defense systems. Cells 10 (8), 1944. doi:10.3390/cells10081944
Wang, L., Li, J., Zhou, Q., Yang, G., Ding, X. L., Li, X., et al. (2014). Rare earth elements activate endocytosis in plant cells. Proc. Natl. Acad. Sci. U. S. A. 111, 12936–12941. doi:10.1073/pnas.1413376111
Weiping, S., Fashui, H., Zhigang, W., Yuzhen, Z., Fugen, G., Hongoing, X., et al. (2003). Effects of cerium on nitrogen metabolism of peach plantlet in vitro. Biol. Trace Elem. Res. 95, 259–268. doi:10.1385/bter:95:3:259
Wells, W. H., and Wells, V. (2001). “The lanthanides, rare earth metals,” in Metals and metal compounds, compounds of inorganic nitrogen, carbon, oxygen, and halogens. Editor E. Bingham (New York: Wiley), 423
Wojtyla, Ł., Lechowska, K., Kubala, S., and Garnczarska, M. (2016). Different modes of hydrogen peroxide action during seed germination. Front. Plant Sci. 7, 66. doi:10.3389/fpls.2016.00066
Xiaoqing, L., Hao, H., Chao, L., Min, Z., and Fashui, H. (2009). Physico-chemical property of rare earth—effects on the energy regulation of photosystem II in Arabidopsis thaliana. Biol. Trace Elem. Res. 130, 141–151. doi:10.1007/s12011-009-8321-1
Xu, Q. M., and Chen, H. (2011). Antioxidant responses of rice seedling to Ce4+ under hydroponic cultures. Ecotoxicol. Environ. Saf. 74, 1693–1699. doi:10.1016/j.ecoenv.2011.04.005
Yang, H., Zhang, X., Liu, H., Cui, W., Zhang, Q., Li, Y., et al. (2016). Lanthanum nitrate genotoxicity evaluation: ames test, mouse micronucleus assay, and chromosome aberration test. Mutat. Research/Genetic Toxicol. Environ. Mutagen. 810, 1–5. Epub 2016 Sep 15. doi:10.1016/j.mrgentox.2016.09.008
Yang, Q., Wang, L., Zhou, Q., and Huang, X. (2015). Toxic effects of heavy metal terbium ion on the composition and functions of cell membrane in horseradish roots. Ecotoxicol. Environ. Saf. 111, 48–58. doi:10.1016/j.ecoenv.2014.10.002
Yuguan, Z., Min, Z., Luyang, L., Zhe, J., Chao, L., Sitao, Y., et al. (2009). Effects of cerium on key enzymes of carbon assimilation of spinach under magnesium deficiency. Biol. Trace Elem. Res. 131, 154–164. doi:10.1007/s12011-009-8354-5
Zheng, H. L., Zhang, C. G., Zhao, Z. Q., Ma, J. H., and Huang, X. J. (2002). Effects of lanthanum chloride on activity of redox system in plasma membrane of rice seedling roots. J. Rare Earths 20, 156–157.
Zicari, M. A., d’Aquino, L., Paradiso, A., Mastrolitti, S., and Tommasi, F. (2018). Effect of cerium on growth and antioxidant metabolism of Lemna minor. Ecotoxicol. Environ. Saf. 163, 536–543. doi:10.1016/j.ecoenv.2018.07.113
Keywords: Rare earth elements, toxicology, plant, ascorbate, lipid peroxidation, hydrogen peroxide, aberrations
Citation: Gjata I, Tommasi F, De Leonardis S, Dipierro N and Paciolla C (2022) Cytological alterations and oxidative stress induced by Cerium and Neodymium in lentil seedlings and onion bulbs. Front. Environ. Sci. 10:969162. doi: 10.3389/fenvs.2022.969162
Received: 16 June 2022; Accepted: 14 July 2022;
Published: 19 August 2022.
Edited by:
Giovanni Libralato, University of Naples Federico II, ItalyReviewed by:
Valbona Aliko, University of Tirana, AlbaniaLuigi D'Aquino, Energy and Sustainable Economic Development (ENEA), Italy
Copyright © 2022 Gjata, Tommasi, De Leonardis, Dipierro and Paciolla. This is an open-access article distributed under the terms of the Creative Commons Attribution License (CC BY). The use, distribution or reproduction in other forums is permitted, provided the original author(s) and the copyright owner(s) are credited and that the original publication in this journal is cited, in accordance with accepted academic practice. No use, distribution or reproduction is permitted which does not comply with these terms.
*Correspondence: Franca Tommasi, ZnJhbmNhLnRvbW1hc2lAdW5pYmEuaXQ=