- 1Ecosystems and Environment Research Programme, Faculty of Biological and Environmental Sciences, University of Helsinki, Lahti, Finland
- 2Helsinki Institute of Sustainability Science (HELSUS), Helsinki, Finland
- 3Joint Laboratory of Applied Ecotoxicology, Korea Institute of Science and Technology Europe (KIST EU), Saarbrucken, Germany
- 4Clayton H. Riddell Faculty of Environment, Earth and Resources, University of Manitoba, Winnipeg, MB, Canada
- 5Natural Resources Institute Finland/Luonnonvarakeskus (LUKE), Latokartanonkaari, Helsinki, Finland
With the detection of microplastics in soil, coupled with the various sources continuously contributing to its delivery to and distribution in soils, understanding the effects of microplastics on plants are necessary. Plastics discarded in the environment continually degrade into micro- and nano-sizes, subsequently leaching hazardous chemicals with time. Not only time but also environmental factors related to the climate of the area where the plastic is degrading will contribute to the breakdown process. Thus, this study aimed to understand the phytotoxic effects of microplastic derived from a frequently discarded plastic item, i.e., high-density polyethylene soda bottle caps. The commonly occurring perennial Lolium multiflorum (Italian ryegrass) was exposed to microplastic derived from new and artificially aged bottle caps as well as bottle caps collected from the cities of Lahti, Finland and Gqeberha, South Africa. Additionally, leachates were prepared from these samples and used for exposure. Germination, root and shoot growth, and fresh weight were measured as indicators of adverse effects, and various growth parameters were calculated. Microplastic and leachates from new and Lahti collected bottle caps adversely affected the germination and growth of the plant, indicating that aging and environmental factors affect the phytotoxicity of plastics as environmental pollutants in soil.
1 Introduction
Microplastics (MPs), which are regarded as polymer particles smaller than 5 mm, are abundantly distributed in soils (Scheurer and Bigalke, 2018; Zhang and Lui, 2018; Yang et al., 2021), where they are known to affect physical properties such as aggregation and water holding capacities (de Souza Machado et al., 2019; Lozano et al., 2021a; Lozano et al., 2021b; Bhatt et al., 2021). Several aspects may contribute to the amount of MP deposited into soils, including runoff from roads and urban flooding, plastic mulching, using contaminated compost and fertilizer, fallout from the air, precipitation, and irrigation with contaminated water (Bergmann et al., 2019; Corradini et al., 2019; Kim and An, 2019; Shah and Wu, 2020; Bhatt et al., 2021; Can-Güven, 2021). Furthermore, irrespective of delivery, MP can be distributed within soils through wet-dry cycles, bioturbation, and agricultural activities (O’Connor et al., 2019; Helmberger et al., 2021).
Chemically, intact plastic polymers are considered inert and harmless; however, upon degradation, hazardous additives, often added to the plastics during manufacture to afford desired properties, may be released (Bridson et al., 2021). Except for leaching (Rillig et al., 2021; Zhou et al., 2021), MP can also serve as a vector for concentrating and transporting pollutants adsorbed to the particles’ surfaces (Tourinho et al., 2019). Thus, many publications on the toxic effects of MP exposure highlight the hazardous nature of MP to soil biota (Kim and An, 2019; Kim et al., 2020; Rillig and Lehmann, 2020), threatening biodiversity (He et al., 2018). Organisms have even been shown to actively avoid MP-contaminated soils (Pflugmacher et al., 2020a). However, studies on the direct effects of MPs and their leachates on plants are limited.
Among the plastics, high-density polyethylene (HDPE) is one of the polymers that has the largest annual production (PlasticsEurope, 2019) and is a significant contributor to MP pollution in soil (Kawecki and Nowack, 2019). Globally, approximately 32 million tons of HDPE are produced every year, exceeded only by polypropylene (PP) (45 million tons), low-density (LD) and linear-low-density (LLD) polyethylene (39 million tons), polyvinylchloride (PVC) (37 million tons), and polyethylene terephthalate (PET) (33 million tons). Kawecki and Nowack. (2019) estimated that 98 ± 50 g of HDPE per capita per year is released into the environment (as estimated for Switzerland). Although HDPE is considered one of the least hazardous plastic polymers, plastic additives leaching out of this material have been found to cause acute toxicity in crustaceans (Sridharan et al., 2022). Given the distribution of MPs in soils, the estimated continuous release, as well as the liberal use of HDPE in the agricultural sector (e.g., mulching and irrigation pipes), plants are continuously being exposed and thus, stimulating the need to gather more information on the toxicological implications.
Due to frequent cultivation as a pasture grass and rapid, competitive growth, Lolium multiflorum (Italian ryegrass) can be found in temperate regions globally. Previously, Boots et al. (2019) exposed Lolium perenne (perennial ryegrass) to HDPE, causing negative growth and development effects. The studies by Pflugmacher et al. (2020b) and Pflugmacher et al. (2021a) highlighted that the effects of aging should also not be neglected when assessing the adverse toxicological effects of MPs. Furthermore, the need to consider more relevant exposure materials and not just virgin primary MPs to assess risks was also noted. The preceding research prompted the present study to delve deeper into the effect of HDPE, its leachates, and the effects of aging. L. multiflorum reproduces only by seed, producing large quantities with little dormancy and high germination rates (Beddows, 1973), and the seedlings typically thrive. Due to its high degree of phenotypic plasticity and, thus, high adaptability, L. multiflorum, a model organism in phytotoxicity studies, was chosen to investigate the effects of MP on germination and seedling growth. Typically, in plants, germination and growth are recorded as suitable indicators of changes in environmental conditions and stress, including nutrient and water depletion and toxicants (Gvozdenac et al., 2011).
HDPE used in this study was derived from new as well as naturally and artificially aged soda bottle caps, i.e., to use MP that represents “real-life” samples. The naturally aged caps were collected from two cities with very distinct climates, i.e., Lahti, Finland (continental climate) and Gqeberha (previously Port Elizabeth, PE), South Africa (subtropical oceanic). Therefore, the study aimed to evaluate the effects of new and artificially aged HDPE MP as well as naturally aged HDPE MP and leachates administered to soil on the germination and growth of L. multiflorum. The study contributes to understanding the phytotoxic effects of MP by considering real-life plastic materials collected from the environment.
2 Materials and methods
2.1 Virgin and aged HDPE and leachates
Commercial soda bottle caps marked as high-density polyethylene (HDPE) (i.e., stamped with a number two inside the three-arrow recycling symbol) were used as the plastic material for the experiments. The four HDPE treatments consisted of four types of caps, i.e., new, artificially aged, as well as naturally aged bottle caps collected from urban areas in Lahti, Finland and on a beach in Gqeberha (Port Elizabeth, PE), South Africa. All plastic materials were first washed with ISO-reconstituted water (pH 7.2) (International Organization for Standardization, 1996) and dried at 25°C to remove any adhering particles. To obtain test material, the bottle caps were ground to an average size of 4 mm ± 1 mm with an SHR3D IT compact plastic shredder (3devo B.V. Utrecht, Netherlands). The larger size was chosen as this size was shown to induce the highest response in a study by Bosker et al. (2019) investigating the adverse effects of MP on Lepidium sativum.
Artificial aging of the new HDPE MP material was accomplished according to Fejdyś et al. (2011) using (PN-EN 12280-1:2002, 1997). In short, the accelerated aging was carried out in a dynamic climate chamber (TK 720 Binder GmbH, Tuttlingen, Germany) heated at 70°C ± 0.5°C and a humidity of 50 ± 1.5% for 160 days.
To induce leaching, the four HDPE MP samples (new, aged, Lahti, and PE) were treated according to the Swedish standard 12,457: 2003 (Swedish Standards Institute, 2003), with minor modifications. Each of the HDPE MP samples (200 g) was mixed with ISO-reconstituted water (pH 7.2) (International Organization for Standardization, 1996) in a liquid-to-solid ratio (L/S) of 10 and leached for 72 h at 50°C; the temperature based on the surface temperature of plastic material reaching beyond 50°C outdoors (Wypych, 1999). The whole process was conducted in round bottom flasks on a rotary mixer (Hei-VAP Valve, Heidolph-Instruments, Schwabach, Germany) at 21 rpm in the absence of light. After leaching, the liquid was separated from the solids by vacuum filtration using Whatman borosilicate glass microfiber filters (grade GF/F; particle retention 0.7 µm), according to the EPA method TCLP 1311 (United States Environmental Protection Agency, 1992).
2.2 Lolium multiflorum seeds
Commercially available L. multiflorum var. westerwoldicum seeds were used for the experiments. The seeds were allowed to imbibe in tap water for 24 h before sowing to induce germination. After soaking, whole and undamaged seeds were selected and used for the exposure experiments.
2.3 Exposure treatments and experimental setup
Individual imbibed seeds were planted in 16 mm × 100 mm soda glass test tubes containing soil and the different exposure treatments. Eight treatments (four MP treatments: new HDPE MP, artificially aged MP, Lahti MP, PE MP; four leachate treatments: leachate from new HDPE MP, leachate from aged HDPE MP, leachate from Lahti collected HDPE MP, and leachate from PE collected HDPE MP) were conducted in parallel to untreated controls (n = 24). For the four HDPE MP treatments, 9 g of soil was used per test tube containing 3% w/w of each of the HDPE MP treatments, respectively, and watered with 4 ml of tap water. The MP concentration applied to the soil in the treatments was based on MP concentrations (ranging from 0.03% to 6.7% (w/w)) reported for highly contaminated sites (Fuller and Gautam, 2016). For the leachate treatments, 9 g of soil (without MP) was watered with 4 ml of leachate from each of the treatments (n = 24). The control consisted of pure garden soil (9 g) irrigated with 4 ml of tap water. For each exposure treatment, a total of 24 replicates were used. The seeds were allowed to germinate for 7 days at 23°C ± 1°C, a light intensity of 1,500 lux, and a light-dark cycle of 14:10 h.
2.4 Measured parameters
The germinated seeds per exposure treatment were counted every day for 7 days since planting. Each seedling was carefully removed from the test tube on day seven and gently washed. The root and shoot lengths were manually measured with a digital caliper, and the fresh weight of each seedling was recorded.
Based on the data recorded, germination parameters were calculated, including total Germination Percentage (G%), Mean Germination Rate (MGR), Synchronization Index (Z), Germination Index (GI), and Time to 50% germination (T50).
2.4.1 Total germination percentage (G%)
The total germination percentage (G%) measures the proportion of seeds germinating within a given time, in this case, 7 days. The total G% was calculated according to the following formula by Dastanpoor et al. (2013):
where n is the number of germinated seeds in 7 days and N is the total number of seeds in the population (n = 24).
2.4.2 Mean germination rate
The mean germination rate is the inverse of the mean germination time (MGT), defined as when 50% of all germinating seeds have completed germination (Bewley et al., 2013; Soltani et al., 2015). MGR was calculated as described by Bewley et al. (2013):
where t is the time in days, and n is the number of seeds germinating on day t.
2.4.3 Synchronization index (Z)
The synchronization index (Z) measures the degree of overlap in the germination of two seeds within the same replicated treatment (Ranal and De Santana, 2006). The synchronization index (Z) was calculated according to Ranal and De Santana. (2006):
where Cni,2 = ni, (ni − 1)⁄2 and N = ∑ ni (∑ ni − 1⁄2). Cni, 2 is a combination of the seeds germinated in the time i, two together, and ni is the number of seeds germinated in the time i.
2.4.4 Germination index
The germination index (GI) is a measure of both quantity and rate of germination. The GI of L. multiflorum was determined according to the calculation proposed by the Association of Official Seed Analysts. (1983):
2.4.5 Half germination time (T50)
The T50 describes either time to 50% germination of the final germination percentage within a given period or time until 50% germination of the total seed population (Bewley et al., 2013). The formula used to calculate T50 (Farooq et al., 2005) in the present study utilizes the time to 50% germination of the total germination percentage as described below:
where N is the final number of germination and ni, nj cumulative numbers of seeds germinated by adjacent counts at times ti and tj, respectively, when ni < N/2 < nj.
2.4.6 Root to shoot ratio (R/S ratio)
The R/S ratio was calculated for every seedling by dividing the root length by the shoot length. Non-germinated seeds were excluded from this calculation.
2.5 Statistical analysis
The means and standard deviations were calculated for all recorded empirical data. The ungerminated seeds were considered in the calculations as having root and shoot lengths of 0 mm and fresh weight of 0 g.
Statistical analyses were conducted using IBM® SPSS Statistics version 28.0.0.0 (190) (SPSS Inc. IBM). The data pertaining to the percentage of germinated seed with time were analyzed by Repeated measures analysis of variance (ANOVA) with Bonferroni correction, followed by Tukey posthoc analysis. The remaining data comparing the germination and growth on the seventh day were analyzed with non-parametric Kruskal–Wallis and pairwise comparison using the Bonferroni correction. The alpha value used for designating significance was 0.05 (Kruskal and Wallis, 1952).
3 Results and discussion
3.1 Germination
3.1.1 Growth percentage
The percentage of L. multiflorum seeds germinating with exposure to the eight HDPE MP and leachate treatments over time is shown in Figure 1. After 3 days, the first seeds started germinating in the control and treatments with aged MP, aged leachate, PE MP, and PE leachate (Figure 1). On the fourth day, seedlings exposed to new MP, Lahti MP, and Lahti leachate started germinating. In contrast, the first seeds from the group treated with new leachate did not start germinating until day five. The results indicated that exposure to leachate from new HDPE significantly retarded germination.
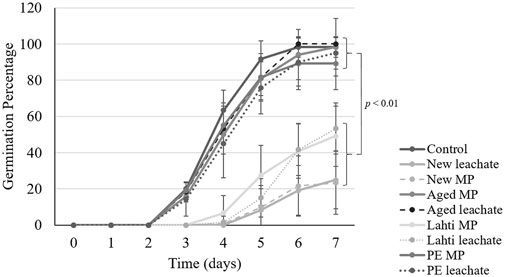
FIGURE 1. Percentage of germinated seeds with time exposed to eight treatments against an untreated control. Data represent mean germination percentages ± standard deviation (n = 24).
On the seventh day, there was a clear division among the treatments where exposures to new MP and new leachate, as well as Lahti MP and leachate, resulted in significantly reduced germination compared to untreated seeds in the control and seeds exposed to aged and PE MP and leachates (p < 0.05). The lowest germination percentage was observed in seeds exposed to new MP, which was 75% lower than the control (p < 0.001) (Figure 1; Table 1). However, exposure to aged MP (p = 0.264) and aged leachate (p = 1), as well as exposure to PE MP (p = 0.073), did not significantly lower the germination percentage compared to the control over time (Figure 1).
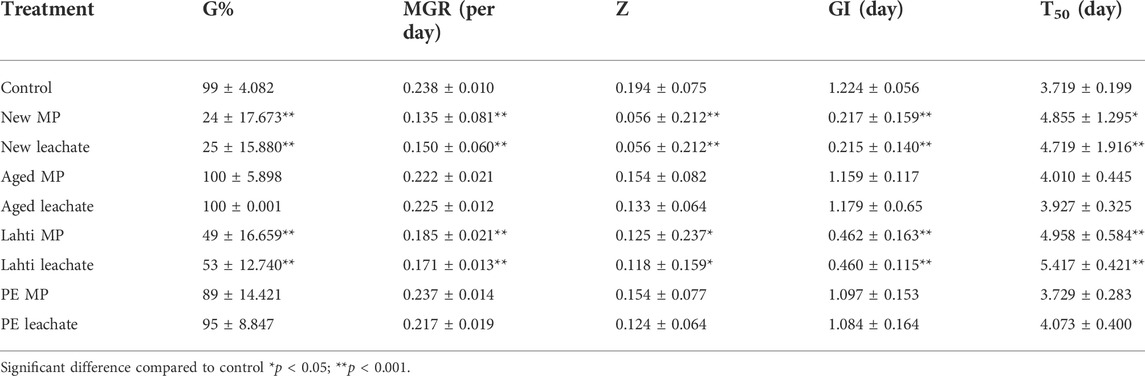
TABLE 1. Calculation of parameters related to germination; germination percentage (G%), mean germination rate (MGR), synchronization index (Z), germination index (GI), and 50% germination time (T50), expressed as mean ± standard deviation (n = 24).
In accordance with Figure 1, the G% and MGR values calculated on the seventh day (Table 1) were significantly reduced when L. multiflorum seeds were exposed to new MP, new leachate, Lahti MP, and Lahti leachate (p < 0.05). Similarly, Boots et al. (2019) reported that exposure to virgin HDPE (0.1% w/w) reduced the number of germinating L. perenne seeds compared to the control.
There were no significant differences in the G% between seeds exposed to MP particles or MP leachates of the same source (new, aged, Lahti, or PE, respectively) (p > 0.05).
The MGRs of the L. multiflorum seeds (Table 1) were 29% lower with exposure to new leachate, 28% lower with Lahti leachate, 25% lower with new MP, 23% lower with Lahti MP, and 9% lower with PE leachate (p < 0.05), indicating more offset and spread germination compared to the control with these exposures. Again, there was no statistical effect on the MGR between MP and leachate samples from the same origin (p > 0.05).
Z values closer to one indicate that seeds exposed to a given treatment germinated simultaneously, whereas a Z value closer to zero represents at least two seeds germinating at different times (Ranal and De Santana, 2006). In general, the Z value was closer to zero for all treatments, including the control (Table 1). The new and Lahti MPs and leachates treated samples had statistically lower Z values than the control (p < 0.05). Nevertheless, based on these data, considerably more seeds germinated simultaneously in the control group than with new and Lahti MPs and leachates.
A higher GI value indicates a large number of seeds germinating at a faster rate. Thus compared to the control, exposure to new and Lahti MPs and leachates caused reduced and slower germination as the GI values were significantly lower (p < 0.05). GI values for seeds exposed to MP particles or the corresponding leachate for the same respective bottle caps were the same (p > 0.05). The GI values obtained for the seeds treated with the aged and PE samples (both MP and leachate) did not significantly differ (p > 0.05).
The T50 values, or time to germination of 50% of the seeds, were 25.1% ± 4.4% higher for seeds treated with both new and Lahti MP and leachate compared to the untreated controls (p < 0.05). As with the other parameters, treatment with either particles or leachate originating from the same source produced indistinguishable T50 values (p > 0.05).
In this experiment, higher values for total G%, MGR, Z, and GI correlate with lower T50 values and vice versa. Exposure to new and Lahti MPs and leachates results in lower and slower germination indicated across the calculated germination parameters for these exposures. Exposure to aged MP, aged leachate, and PE MP and leachate did not significantly affect any germination parameters compared to the controls. These results indicate significant inhibition in the germination of L. multiflorum when exposed to MP particles and leachates of new bottle caps and bottle caps collected in Lahti. Studies by Pignattelli et al. (2020) and Bosker et al. (2019) reported reduced inhibition in seedlings with exposure to MP. Furthermore, Bosker et al. (2019) found that in L. sativum, adverse effects were directly related to increasing plastic sizes; however, in their study, adverse effects were evident after 8 hours but no longer detectable after 24 h. Even though studies on plastic leachates are scarce, Schiavo et al. (2020) demonstrated that the leachates of oxo-degradable PP negatively affect Sorghum saccharatum germination, and Pflugmacher et al. (2020b) demonstrated that the leachates of PC affected L. sativum germination.
Because treatment with MP particles resulted in a similar total G% as treatment with MP leachates, the particles themselves likely have little to no effect on the extent of germination. Instead, findings imply that the substances leaching out of the MPs are responsible for their ecotoxicity. Pflugmacher et al. (2021b) showed that L. sativum exposed to new and short-term aged polycarbonate (PC) suffered more severe germination reductions than with exposure to long-term aged PC, and the adverse effects associated with PC exposure were reduced as a function of aging of the exposure material. The authors similarly hypothesized that the toxicological effects may be attributed to leached chemicals. Pflugmacher et al. (2021a) showed that climate affected the phytotoxicity of MP, where MP from HDPE bottle caps collected from a cooler climate caused a higher percentage of inhibition on Triticum aestivum (wheat) growth compared to MP from caps collected from a warmer climate. The authors postulated that inherently toxic chemicals had already been prompted to leach out of the MP, accelerated by the ambient conditions, into the environment from where it was collected. Their findings agree with the data presented here, showing that the PE MP and leachate were less toxic than the Lahti MP and leachate in terms of germination. The reduced effect of PE MPs on germination compared to Lahti MPs could be explained by the warmer climate in South Africa, which contributed to quicker degradation of the toxic components than in the colder environment of Lahti. Another reason could be that the bottle caps collected in Gqeberha (PE) were older than those collected from Lahti.
3.2 Growth
3.2.1 Root and shoot length
The root and shoot lengths for the different exposure treatments are presented in Figure 2. The root lengths of L. multiflorum seedlings were significantly reduced after 7 days of exposure to new MP and leachate, aged leachate, as well as Lahti collected MP and leachate compared to the untreated controls (p < 0.05). The seedlings most severely affected were exposed to new MP and new leachate, and their root lengths were 84.3% (p < 0.001, new MP) and 91.4% (p < 0.001, leachate from new MP) shorter than the controls. The reductions in root length were 73.2% for Lahti MP (p < 0.001) and 66.8% for Lahti leachate (p < 0.001), and 23.9% for aged leachate (p = 0.023). Soil containing 3% w/w new MP particles and 4 ml leachate from bottle caps collected in PE, as well as aged MP were thus the only treatments that did not significantly reduce the root lengths of L. multiflorum. As observed for germination, there was no statistically significant difference in root growth whether the seedlings had been exposed to MP particles or MP leachate of the same origin for any group (p = 1). Reduced root lengths were also demonstrated for the plant Vicia faba when exposed to 5 µm polystyrene MPs at concentrations of 50 and 100 mg/L (Jiang et al., 2019). In another study by Bosker et al. (2019), significant differences in the root growth of L. sativum were observed after 24 h but not after 48 or 72 h of exposure to MP particles. The effects on the root lengths were both positive and negative depending on the particle size, and there was little effect on the shoot growth.
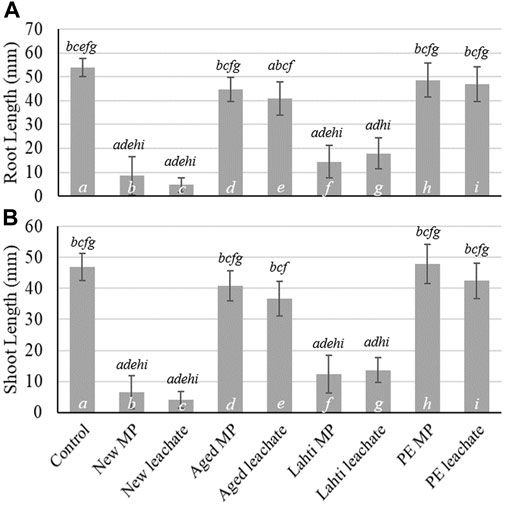
FIGURE 2. (A) Root and (B) shoot length of L. multiflorum seeds exposed to the eight treatments versus an unexposed control. Bar graphs represent mean length (in mm) ± standard deviation (n = 24). Significant differences are indicated by the letter above the bars (p < 0.05).
In the present study, the shoot growth was significantly inhibited on day seven for seedlings treated with all exposure treatments (p < 0.05), except aged and PE MP and leachate (p > 0.05), compared to the controls. The shoot lengths were most severely reduced when exposed to MP and leachate from new bottle caps, followed by MP and leachate from Lahti collected bottle caps. Seedlings exposed to new MP and new leachate had an average reduction in shoot length of 86.1% (p < 0.001) and 91.2% (p < 0.001). For the Lahti MP and leachate, the shoot length was reduced by 73.6% (p < 0.001) and 70.9% (p < 0.001). No statistically significant differences were observed in shoot length between seedlings treated with MP particles and leachate of the same origin (p = 1). Similarly, Boots et al. (2019) and Pignattelli et al. (2020) reported reduced shoot length of L. perenne exposed to polylactic acid (PLA) MPs and L. sativum exposure to various MPs polymer types, respectively.
According to these findings, MP particles and leachate could adversely affect the root and shoot growth of plants. The difference between new and aged MP and leachates indicates that aging drastically decreases the toxicological effect on root and shoot growth. Again, the findings agree with studies by Pflugmacher et al. (2020b); Pflugmacher et al. (2021a); Pflugmacher et al. (2021b), showing that the reduction in phytotoxicity was directly related to aging.
In this experiment, the root and shoot growth inhibition was similar regardless of exposure to particles or leachates from the same MPs. Interestingly, even though the study by Pflugmacher et al. (2020b) did not directly compare the root and shoot lengths of L. sativum with exposure to PC MP and leachate, there is a visible difference in the length distinguishable between the two exposure types. It may indicate that this observation is species-specific and related to the resilience and adaptability of the plant species and probably also the variant in question.
The mechanisms through which MP particles affect plant growth are largely unknown; however, Rillig et al. (2019) postulated, among others, that this could be related to changed soil properties affecting water availability and microbes, MP acting as vectors for other pollutants or uptake by the roots leading to physical damage. Due to the MP particle size used in the present study (4 mm) and the fact that particles and leachate from the same MP caused the same magnitude of effects, uptake and altered soil properties seem unlikely.
3.2.2 Root to shoot ratio
The ratios of the roots to the shoots per treatment are presented in Figure 3. This ratio represents the relationship between the amounts of tissue with supportive functions versus those with growth functions. A more significant root proportion indicates a more effective uptake of nutrients, whereas higher fractions of shoots are associated with more light energy uptake (Allaby, 2019).
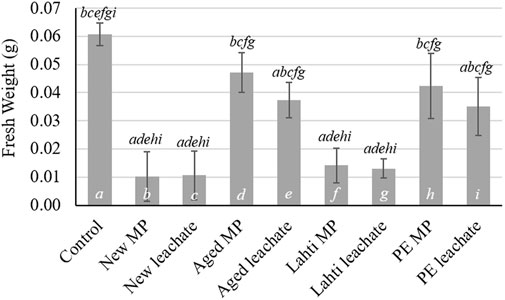
FIGURE 3. Ratio of the root and shoot lengths. Data represent mean ± standard deviation (n = 24). Significant differences are indicated by the letter above the bars (p < 0.05).
Stress is known to induce changes to the R/S ratio in plants; however, no consistent evidence exists on whether the ratio increases, decreases, or remains unaffected under certain stress conditions (Agathokleous et al., 2019). In the presented study, none of the treatments differed from the ratio of the control (p > 0.05). Nevertheless, the exposure to PE MP resulted in a significantly lower R/S ratio than new MP (23.4%; p = 0.002) as well as Lahti MP (16.3%; p = 0.006) and leachate (22.9%; p < 0.001).
In a study by Boots et al. (2019), L. perenne planted in soil containing 0.1% w/w HDPE MP (size range 0.48–316 μm) resulted in seedlings with a 35% higher R/S ratio than the controls. Treatment with synthetic fibers (acrylic and nylon mixture) and biodegradable polylactic acid (PLA) did not significantly affect the R/S ratio (Boots et al., 2019), indicating that the ecotoxicity of MPs varies depending on the polymer type. In the present study, however, a higher concentration of 3% (w/w) HDPE with larger particles (4 mm) did not elicit a response in terms of the R/S ratio.
3.2.3 Fresh weight
In Figure 4, the fresh weights of the seedlings are presented. Seedlings exposed to all MP particles and leachates had reduced weights (p < 0.05) compared to the control, except for seedlings exposed to aged MP and Lahti MP. Exposure to new MP and leachate caused L. multiflorum seedlings’ fresh weights to decrease by 83.4% (p < 0.001) and 82.6% (p < 0.001), respectively. Treatment with Lahti MP and Lahti leachate also resulted in severe reductions in seedlings’ fresh weight, amounting to 76.8% (p < 0.001) and 78.5% (p < 0.001). The fresh weight of seedlings exposed to aged MP remained unaffected (p = 1); however, exposure to aged leachate caused a 38.5% reduction (p = 0.004). For PE leachate, the fresh weight was 42.3% lower than the controls (p = 0.001), and the seedling exposed to PE MP remained unaffected (p = 0.126).
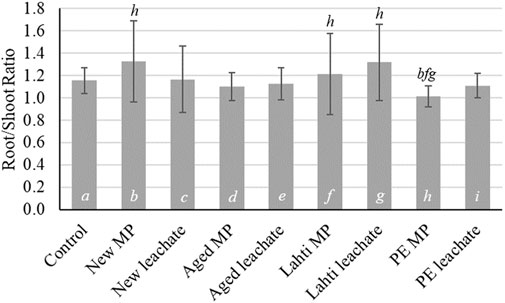
FIGURE 4. Fresh weight of the seedlings exposed to the eight different treatments compared to an unexposed control. Bars represent mean fresh weight (in grams) ± standard deviation (n = 24). Significant differences are indicated by the letter above the bars (p < 0.05).
Previous experiments measuring plant weights after exposure to MPs have demonstrated varying effects. In a study on T. aestivum (wheat) by Qi et al. (2018), the shoot biomass was lower than the controls when exposed to starch-based biodegradable MPs but not when exposed to LDPE MPs after a period of 14–40 days. The biodegradable MPs negatively affected the shoot biomass also after 2 months. Both plastic materials resulted in lower root biomass after 2 months, but neither exhibited lower root or shoot biomass after 4 months, indicating that the adverse effects in terms of weight may only be observable after a longer exposure time than was used in the present study. In support of this, Pignattelli et al. (2020) reported that the shoot biomass of L. sativum was negatively affected when exposed to polyethylene and PP MPs (0.02% w/w) smaller than 0.125 mm during chronic exposure (21 days). However, Jiang et al. (2019) found that exposure to 5 µm polystyrene MP in concentrations of 10, 50, and 100 mg/L resulted in reduced fresh weight of V. faba roots after 48 h. On the contrary, in a study by Boots et al. (2019), exposure to HDPE MPs (0.1 w/w; size 0.6–363 μm) resulted in higher root biomass of L. perenne after an exposure period of 30 days. Therefore, the effect and onset time of the effect may be species-specific; therefore, more data is needed to understand how plants are affected by MP exposure.
The results generally indicated that plastics are the most phytotoxic in their virgin state due to little exposure to the elements to facilitate breakdown and leaching. Because exposure to the particles and leachate from the same origin caused the same adverse effects, chemicals released from the polymer are likely responsible for the inhibited germination and growth observed.
Life completely devoid of plastics is currently inconceivable due to the ubiquitous use of plastics in quotidian products and activities. However, harmless alternatives must be sought to curb the adverse phytotoxic effects. Additionally, development in the approaches to removing plastic pollution from terrestrial and aquatic environments, such as bioremediation, seems promising (Bhatt et al., 2021). Using natural packaging materials may also alleviate the burden of plastic pollution on the various ecosystems.
4 Conclusion
This study showed that new HDPE MP particles and leachates severely inhibit the germination and growth of L. multiflorum. Similar adverse effects were recorded on germination and growth with exposure to MP and subsequent leachate from HDPE bottle caps collected from a location with a cooler climate. Adverse effects were reduced with artificial aging. When exposed to bottle caps collected from a warmer climate, toxic chemicals had already leached from the material prior to exposure. Compared to the existing literature, various responses were observed among the species exposed, indicating that the phytotoxicity of MP may be species-specific, and additionally depend on the polymer type and size. Thus, more data need to be collected to assess the risk posed to plants with exposure to MPs in soil.
Data availability statement
The raw data supporting the conclusion of this article will be made available by the authors, without undue reservation.
Author contributions
Conceptualization: SP, ME; Data curation: ME, SP, SV; Investigation, Methodology and Formal analysis: SV, SP, ME; Funding acquisition: SP, ME, YK; Project administration SP, ME; Supervision: SP, O-PP, ME; Resources: SP, ME; Writing—original draft: ME; Writing—review and editing: ME, SV, O-PP, YK, SP. All authors contributed to the article and approved the submitted version.
Funding
This work was supported by the Korean Institute of Science and Technology (KIST) Europe’s Nanomaterial Technology Development Program (NRF-2017M3A7B6052455), funded by the South Korean Ministry of Science and ICT. The University of Helsinki provided open access funding.
Conflict of interest
The authors declare that the research was conducted in the absence of any commercial or financial relationships that could be construed as a potential conflict of interest.
Publisher’s note
All claims expressed in this article are solely those of the authors and do not necessarily represent those of their affiliated organizations, or those of the publisher, the editors and the reviewers. Any product that may be evaluated in this article, or claim that may be made by its manufacturer, is not guaranteed or endorsed by the publisher.
References
Agathokleous, E., Belz, R., Kitao, M., Koike, T., and Calabrese, E. (2019). Does the root to shoot ratio show a hormetic response to stress? An ecological and environmental perspective. J. For. Res. 30 (5), 1569–1580. doi:10.1007/s11676-018-0863-7
Association of Official Seed Analysts (AOSA) (1983). “Seed vigor testing handbook,” in Contribution No 32 to the handbook on seed testing (Ithaca, NY: AOSA Seed Vigor Test Committee).
Bergmann, M., Mützel, S., Primpke, S., Tekman, M. B., Trachsel, J., and Gerdts, G. (2019). White and wonderful? Microplastics prevail in snow from the alps to the arctic. Sci. Adv. 5, eaax1157. doi:10.1126/sciadv.aax1157
Bewley, J., Bradford, K., Hilhorst, H., and Nonogaki, H. (2013). Seeds physiology of development, germination and dormancy. Berlin, Germany: Springer.
Bhatt, P., Pathak, V. M., Bagheri, A. R., and Bilal, M. (2021). Microplastic contaminants in the aqueous environment, fate, toxicity consequences, and remediation strategies. Environ. Res. 200, 111762. doi:10.1016/j.envres.2021.111762
Boots, B., Russell, C., and Green, D. (2019). Effects of microplastics in soil ecosystems: Above and below ground. Environ. Sci. Technol. 53 (19), 11496–11506. doi:10.1021/acs.est.9b03304
Bosker, T., Bouwman, L., Brun, N., Behrens, P., and Vijver, M. (2019). Microplastics accumulate on pores in seed capsule and delay germination and root growth of the terrestrial vascular plant Lepidium sativum. Chemosphere 226 (1), 774–781. doi:10.1016/j.chemosphere.2019.03.163
Bridson, J. H., Gaugler, E. C., Smith, D. A., Northcott, G. L., and Gaw, S. (2021). Leaching and extraction of additives from plastic pollution to inform environmental risk: A multidisciplinary review of analytical approaches. J. Hazard. Mat. 414, 125571. doi:10.1016/j.jhazmat.2021.125571
Can-Güven, E. (2021). Microplastics as emerging atmospheric pollutants: A review and bibliometric analysis. Air Qual. Atmos. Health 14 (2), 203–215. doi:10.1007/s11869-020-00926-3
Corradini, F., Meza, P., Eguiluz, R., Casado, F., Huerta-Lwanga, E., and Geissen, V. (2019). Evidence of microplastic accumulation in agricultural soils from sewage sludge disposal. Sci. Total Environ. 671, 411–420. doi:10.1016/j.scitotenv.2019.03.368
Dastanpoor, N., Fahimi, H., Shariati, M., Davazdahemami, S., and Hashemi, S. (2013). Effects of hydropriming on seed germination and seedling growth in sage (Salvia officinalis L.). Afr. J. Biotechnol. 12 (11), 1223–1228. doi:10.5897/AJB12.1941
De Souza Machado, A. A., Lau, C. W., Kloas, W., Bergmann, J., Bachelier, J. B., Faltin, E., et al. (2019). Microplastics can change soil properties and affect plant performance. Environ. Sci. Technol. 53, 6044–6052. doi:10.1021/acs.est.9b01339
Farooq, M., Basra, S., Ahmad, N., and Hafeez, K. (2005). Thermal hardening: A new seed vigor enhancement tool in rice. J. Integr. Plant Biol. 47 (1), 187–193. doi:10.1111/j.1744-7909.2005.00031.x
Fejdyś, M., Landwijt, M., and Strusczyk, M. (2011). Effects of accelerated ageing conditions on the degradation process of Dyneema® polyethylene composites. Fibres Text. East. Eur. 19 (1), 60–65.
Fuller, S., and Gautam, A. A. (2016). A procedure for measuring microplastics using pressurized fluid extraction. Environ. Sci. Technol. 50 (11), 5774–5780. doi:10.1021/acs.est.6b00816
Gvozdenac, S., Inđić, D., Slavica, V., Grahovac, M., Vrhovac, M., Bošković, Ž., et al. (2011). Germination root and shoot length as indicators of water quality. Acta Agric. serb. 16 (31), 33–41.
He, D., Luo, Y., Lu, S., Liu, M., Song, Y., and Lei, L. (2018). Microplastics in soils: Analytical methods, pollution characteristics and ecological risks. TrAC Trends Anal. Chem. 109, 163–172. doi:10.1016/j.trac.2018.10.006
Helmberger, M. S., Tiemann, L. K., and Grieshop, M. J. (2021). Towards an ecology of soil microplastics. Funct. Ecol. 34, 550–560. doi:10.1111/1365-2435.13495
International Organization for Standardization (ISO) (1996). Water quality - determination of the acute lethal toxicity of substances to a freshwater fish [brachydanio rerio Hamilton-buchanan (teleostei, cyprinidae)]. Available At: http://www.iso.org (accessed on April 2, 2022).
Jiang, X., Chen, H., Liao, Y., Ye, Z., Li, M., and Klobučar, G. (2019). Ecotoxicity and genotoxicity of polystyrene microplastics on higher plant Vicia faba. Environ. Pollut. 250 (1), 831–838. doi:10.1016/j.envpol.2019.04.055
Kawecki, D., and Nowack, B. (2019). Polymer-specific modeling of the environmental emissions of seven commodity plastics as macro- and microplastics. Environ. Sci. Technol. 53 (16), 9664–9676. doi:10.1021/acs.est.9b02900
Kim, S. W., and An, Y.-J. (2019). Soil microplastics inhibit the movement of springtail species. Environ. Int. 126, 699–706. doi:10.1016/j.envint.2019.02.067
Kim, S. W., Waldman, W. R., Kim, T. Y., and Rillig, M. C. (2020). Effects of different microplastics on nematodes in the soil environment: Tracking the extractable additives using an ecotoxicological approach. Environ. Sci. Technol. 54, 13868–13878. doi:10.1021/acs.est.0c04641
Kruskal, W. H., and Wallis, W. A. (1952). Use of ranks in one-criterion variance analysis. J. Am. Stat. Assoc. 47 (260), 583–621. doi:10.1080/01621459.1952.10483441
Lozano, Y. M., Aguilar‐Trigueros, C. A., Onandia, G., Maaß, S., Zhao, T., and Rillig, M. C. (2021b). Effects of microplastics and drought on soil ecosystem functions and multifunctionality. J. Appl. Ecol. 58, 988–996. doi:10.1111/1365-2664.13839
Lozano, Y. M., Lehnert, T., Linck, L. T., Lehmann, A., and Rillig, M. C. (2021a). Microplastic shape, polymer type, and concentration affect soil properties and plant biomass. Front. Plant Sci. 12, 616645. doi:10.3389/fpls.2021.616645
O’Connor, D., Pan, S., Shen, Z., Song, Y., Jin, Y., Wu, W. M., et al. (2019). Microplastics undergo accelerated vertical migration in sand soil due to small size and wet-dry cycles. Environ. Pollut. 249, 527–534. doi:10.1016/j.envpol.2019.03.092
Pflugmacher, S., Huttunen, J. H., von Wolff, M. A., Penttinen, O. P., Kim, Y. J., Kim, S., et al. (2020a). Enchytraeus crypticus avoid soil spiked with microplastic. Toxics 8 (1), 10. doi:10.3390/toxics8010010
Pflugmacher, S., Sulek, A., Mader, H., Heo, J., Noh, J. H., Penttinen, O. P., et al. (2020b). The influence of new and artificial aged microplastic and leachates on the germination of Lepidium sativum. L. Plants 9 (3), 339. doi:10.3390/plants9030339
Pflugmacher, S., Tallinen, S., Kim, Y. J., Kim, S., and Esterhuizen, M. (2021b). Ageing affects microplastic toxicity over time: Effects of aged polycarbonate on germination, growth, and oxidative stress of Lepidium sativum. Sci. Total Environ. 790, 148166. doi:10.1016/j.scitotenv.2021.148166
Pflugmacher, S., Tallinen, S., Mitrovic, S. M., Penttinen, O. P., Kim, Y. J., Kim, S., et al. (2021a). Case study comparing effects of microplastic derived from bottle caps collected in two cities on Triticum aestivum (Wheat). Environments 8 (7), 64. doi:10.3390/environments8070064
Pignattelli, S., Broccoli, A., and Renzi, M. (2020). Physiological responses of garden cress (L. sativum) to different types of microplastics. Sci. Total Environ. 727 (1), 138609. doi:10.1016/j.scitotenv.2020.138609
PlasticsEurope (2019). Plastics – The facts 2019. An analysis of European plastics production demand and waste data. Available At: https://www.plasticseurope.org/en/resources/publications/1804-plastics-facts-2019 (accessed on January 22, 2022).
PN-EN 12280-1:2002 (1997). Rubber- or plastics-coated fabrics—accelerated ageing tests—Part 1: Heat ageing; EN 12280-1:1997. Geneva, Switzerland: International Organization for Standards.
Qi, Y., Yang, X., Pelaez, A., Huerta Lwanga, E., Beriot, N., Gertsen, H., et al. (2018). Macro- and micro- plastics in soil-plant system: Effects of plastic mulch film residues on wheat (Triticum aestivum) growth. Sci. Total Environ. 645 (1), 1048–1056. doi:10.1016/j.scitotenv.2018.07.229
Ranal, M. A., and De Santana, D. G. (2006). How and why to measure the germination process. Rev. Bras. Bot. 29 (1), 1–11. doi:10.1590/S0100-84042006000100002
Rillig, M. C., Kim, S. W., Kim, T. -Y., and Waldman, W. R. (2021). The global plastic toxicity Debt. Environ. Sci. Technol. 55, 2717–2719. doi:10.1021/acs.est.0c07781
Rillig, M. C., and Lehmann, A. (2020). Microplastic in terrestrial ecosystems. Science 368, 1430–1431. doi:10.1126/science.abb5979
Rillig, M., Lehmann, A., Souza Machado, A., and Yang, G. (2019). Microplastic effects on plants. New Phytol. 223 (3), 1066–1070. doi:10.1111/nph.15794
Scheurer, M., and Bigalke, M. (2018). Microplastics in Swiss floodplain soils. Environ. Sci. Technol. 52, 3591–3598. doi:10.1021/acs.est.7b06003
Schiavo, S., Oliviero, M., Chiavarini, S., and Manzo, S. (2020). Adverse effects of oxo-degradable plastic leachates in freshwater environment. Environ. Sci. Pollut. Res. 27 (8), 8586–8595. doi:10.1007/s11356-019-07466-z
Shah, F., and Wu, W. (2020). Response to: Grant re: “Precipitation and climate zone explains the geographical disparity in the invasive cancer incidence rates in the United States”. Environ. Eng. Sci. 164, 231–287. doi:10.1089/ees.2020.0012
Soltani, E., Ghaderi-Far, F., Baskin, C., and Baskin, J. (2015). Problems with using mean germination time to calculate rate of seed germination. Aust. J. Bot. 63 (8), 631–635. doi:10.1071/BT15133
Sridharan, S., Kumar, M., Saha, M., Kirkham, M. B., Singh, L., and Bolan, N. S. (2022). The polymers and their additives in particulate plastics: What makes them hazardous to the fauna? Sci. Total Environ. 824, 153828. doi:10.1016/j.scitotenv.2022.153828
Swedish Standards Institute (SIS) (2003). Characterization of waste - leaching - compliance test for leaching of granular waste materials and sludges svensk standard SS-en 12457-3. Available Ate: https://www.sis.se (accessed on April 12, 2022).
Tourinho, P. S., Kočí, V., Loureiro, S., and van Gestel, C. A. M. (2019). Partitioning of chemical contaminants to microplastics: Sorption mechanisms, environmental distribution and effects on toxicity and bioaccumulation. Environ. Pollut. 252, 1246–1256. doi:10.1016/j.envpol.2019.06.030
United States Environmental Protection Agency (USEPA). (1992). Method 1311: Toxicity characteristic leaching procedure, part of test methods for evaluating solid waste, physical/chemical methods USEPA. Available At: https://www.epa.gov/sites/production/files/2015-12/documents/1311.pdf (accessed on April 12, 2022).
Wypych, G. (1999). Weathering of plastics: Testing to mirror life performance. Scarborough, Canada: ChemTec Publishing.
Yang, L., Zhang, Y., Kang, S., Wang, Z., and Wu, C. (2021). Microplastics in soil: A review on methods, occurrence, sources, and potential risk. Sci. Total Environ. 780, 146546. doi:10.1016/j.scitotenv.2021.146546
Zhang, G., and Liu, Y. (2018). The distribution of microplastics in soil aggregate fractions in southwestern China. Sci. Total Environ. 642 (1), 12–20. doi:10.1016/j.scitotenv.2018.06.004
Keywords: Italian ryegrass, microplastic, leachate, ecotoxicology, sprouting, growth, phytotoxicity of microplastic and leachate
Citation: Esterhuizen M, Vikfors S, Penttinen O-P, Kim YJ and Pflugmacher S (2022) Lolium multiflorum germination and growth affected by virgin, naturally, and artificially aged high-density polyethylene microplastic and leachates. Front. Environ. Sci. 10:964230. doi: 10.3389/fenvs.2022.964230
Received: 08 June 2022; Accepted: 18 October 2022;
Published: 28 October 2022.
Edited by:
Andrew Hursthouse, University of the West of Scotland, United KingdomReviewed by:
Pankaj Bhatt, Purdue University, United StatesGrzegorz Żurek, Plant Breeding and Acclimatization Institute, Poland
Copyright © 2022 Esterhuizen, Vikfors, Penttinen, Kim and Pflugmacher. This is an open-access article distributed under the terms of the Creative Commons Attribution License (CC BY). The use, distribution or reproduction in other forums is permitted, provided the original author(s) and the copyright owner(s) are credited and that the original publication in this journal is cited, in accordance with accepted academic practice. No use, distribution or reproduction is permitted which does not comply with these terms.
*Correspondence: Maranda Esterhuizen, Maranda.esterhuizen@helsinki.fi