- 1Gulf Fisheries Centre, Fisheries and Oceans Canada, Moncton, NB, Canada
- 2Great Lakes Laboratory for Fisheries and Aquatic Sciences, Fisheries and Oceans Canada, Burlington, ON, Canada
- 3Department of Biology, University of Waterloo, Waterloo, ON, Canada
Continued urban development is inevitable with ongoing human population growth. Urbanization has profound negative impacts on aquatic environments and the organisms that occupy those environments. Urbanization results in habitat stressors including sedimentation, alterations to flow, increases in water temperature, changes in riparian habitat, and changes in the food supply of freshwater systems. In the face of continuing urbanization there is an urgent need to employ effective and practical tools to evaluate the impacts on freshwater fish populations and to better understand the implications of urban development for lotic aquatic ecosystems. Stable isotope analyses (δ13C, δ15N, δ18O δ34S and δ2H) are useful and cost-effective tools that can help highlight and evaluate urbanization impacts on fish populations. Here we review recent developments in stable isotope-based methods for studying urbanization impacts on freshwater fish populations. We identified and review published studies that have implemented stable isotopes to evaluate urban impacts, discuss promising stable isotope-based approaches that have not been commonly applied to the assessment of urban impacts, and highlight the advantages and limitations of the various approaches. In particular, we show how stable isotope analyses can help address both the ecological and physiological impacts of urban development on freshwater fishes in hopes of inspiring more frequent use of the approaches in urbanization studies. We elucidate how stable isotopes can be used to understand the impacts of urbanization on the feeding ecology, habitat and thermal occupancy, and field metabolic rates of freshwater fishes. It was found that stable isotope-based approaches are underutilized to examine the impacts of urbanization on freshwater fishes. We suggest such approaches can be applied more frequently in freshwater environments globally to help assess and actively mitigate the negative impacts of urbanization on freshwater fishes.
Introduction
The rapid growth of human populations and the increasing concentration of people in urban centres has had significant consequences for the environmental integrity of surrounding ecosystems (Cohen, 2003). Often occurring near water for practical (i.e., food, travel and power) and leisure (i.e., recreational fishing, aquatic sports, beauty) reasons, urbanization has had notable impacts on aquatic environments, in particular for freshwaters, and has been implicated as one of the major causes of biodiversity loss worldwide (Tickner et al., 2020). Rivers are especially threatened given projections that up to 60% of the world’s population will eventually reside in cities located on rivers (Young et al., 1994), which is expected to cause changes in river ecosystems through habitat destruction, changes in water chemistry, industrialization, land-use change and river channel alterations (Malmqvist and Rundle, 2002). Many of the impacts will act synergistically to alter both physical habitats and the biological processes occurring within affected rivers and streams, thereby confounding the detection and determination of cause and effect (Baer and Pringle, 2000). In the face of such changes, it is critically important that additional analytical tools are developed and used for characterizing and describing the mechanisms and effects of urbanization impacts on freshwater biota. Here we review recent developments in stable isotope methods for studying urbanization impacts on freshwater fish populations, which often integrate the direct and indirect stressors acting on the ecosystems in which they are found (e.g., Adams, 2002; Power, 2007).
Urban development is known to negatively impact freshwater fishes through a wide variety of mechanisms, including the release of contaminants (Collier et al., 1998), the introduction of non-native invasive species (Santana Marques et al., 2020), channelization (Brookes and Gregory, 1983) and hydrological disruptions (Booth and Jackson, 1997), increased impervious surfaces (Wang et al., 2000), increased sedimentation (Poff et al., 2006), changes in thermal regimes (Nelson and Palmer, 2007), modifications to nutrient and energy flows (Carpenter et al., 2011) and the degradation of riparian habitat (Booth et al., 2016). Most, if not all, of the effects will have direct and indirect implications for the feeding ecology, habitat occupancy, growth, survival, reproduction, and migration patterns of affected fishes (Figure 1), either as individuals, populations, or communities. Adjustments to feeding and habitat occupancy can happen quickly as realized niches are constrained (Woodland et al., 2022), either by the physical impacts of urbanization (i.e., imposed thermal constraints) or changes in the competitive dynamics within or between species.
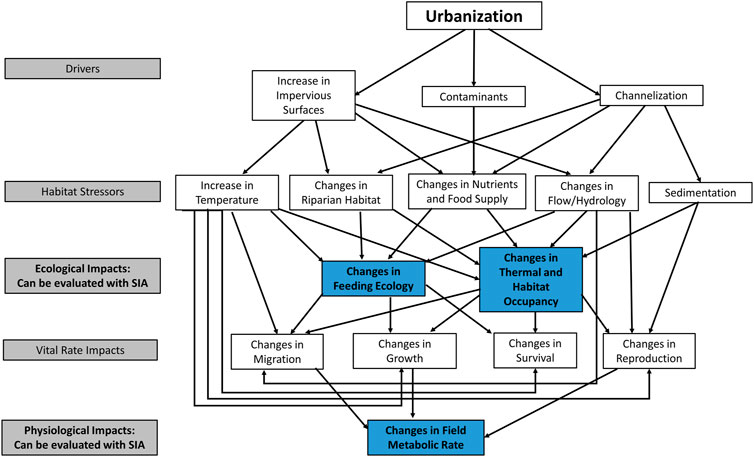
FIGURE 1. Multifactorial conceptual pathways for urbanization effects on freshwater fish. Aspects that can be directly evaluated using stable isotope analysis are shown in blue. Aspects more generally assessed using methods aimed at quantification of vital rates are shown in white. Links and feedbacks between stressors were not represented to enhance readability.
Understanding the impacts of urbanization on freshwater fishes can help determine the overall influence of urbanization on freshwater ecosystems given the strong top-down and bottom-up effects of fishes on freshwater ecosystem processes (Gido et al., 2010). Given the continued and growing presence of urbanization threats to freshwater ecosystems (Carpenter et al., 2011) there is an urgent need to develop and apply effective and practical tools to understand the various impacts of urban development on freshwater fishes. Many tools including the quantification of life history traits (Winemiller, 2005) and stable isotope analyses (Post, 2002; Boecklen et al., 2011) have been widely used for characterizing the response of fish species to other environmental stressors, and are likely to be equally valuable for evaluating the negative impacts of urban development on fish populations.
Changes in life history traits related to survival (e.g., age-specific survival rates, year class strength, age structure), growth (e.g., mean weight-at-age, growth curves, specific growth rates, condition factor) and reproduction (e.g., age-at-maturity, fecundity, egg size) have historically been used to examine responses to stressor exposure (e.g., Adams, 2002) on individual fish and fish populations. These life history based assessments are often the default method for determining stressor impacts on fish populations. Power (2002) provides an in-depth review of assessment frameworks based on the use of life history traits and population biological characteristics that can be broadly grouped into those affecting the vitals rates of growth, survival or reproduction known to influence fish populations (Table 1). Based on the theory and techniques used for assessing harvesting impacts, such frameworks can be valuable when applied to the impacts of urbanization-induced stressors on fish vital rates given that changes in vital rates will eventually impact fish population status (Shelton and Mangel, 2011). A comprehensive review of the methods listed in Table 1 can be found in Guy and Brown (2007).
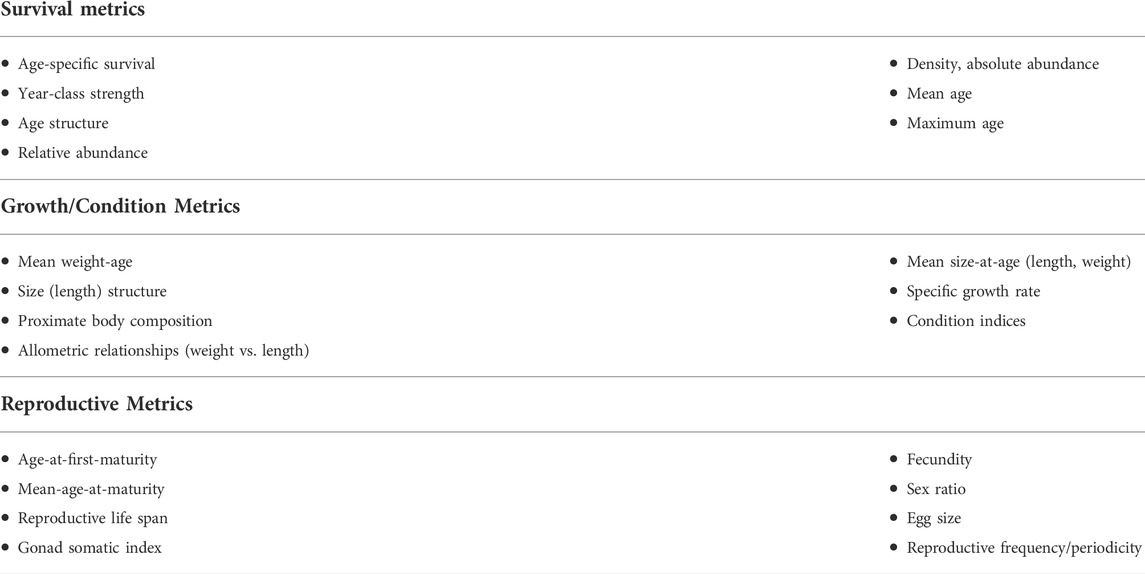
TABLE 1. Traditional metrics used to assess fish population status based on Power (2002). Metrics can be derived from survey measurements of the population from either point-in-time cross-sectional surveys or from repeated surveys through time. Metrics are grouped by the key vital rates (survival, growth or reproduction) responsible for determining the intrinsic rate of population increase. The measures within each group are not necessarily independent of each other either within or between categories. As an example, changes in maximum age (survival) will affect age-structure similarly used to assess survival. This can hold direct implications for life-time fecundity (reproduction) and may affect age-specific survival rates (survival) in subsequent generations. Stable isotope-based approaches can be used to understand changes in feeding ecology, habitat use, and temperature use that might lead to changes in vital rates.
The quantification of life history traits focuses on assessing the individual or cumulative effects of stressors after the stressors have begun to elicit population-level responses. Focusing on changes in life history traits may fail to address the more immediate ecological impacts of urban development on freshwater fishes such as changes in feeding ecology or variation in habitat occupancy and activity levels that may eventually lead to shifts in vital rates. Here we examine how the methods and techniques associated with stable isotope analyses can be adapted to address important aspects of the pathways of effects of urbanization on freshwater fish populations and communities (see Figure 1).
Stable isotope analysis to address the effects of urbanization
Stable isotope analyses have become a well-established, efficient, and cost-effective tool in ecological studies for estimating dietary composition (Boecklen et al., 2011), trophic niche attributes (Newsome et al., 2007), thermal habitat occupancy (Høie et al., 2004) and the field metabolic rate (Chung M.-T. et al., 2019b) of fishes. While the use of stable isotopes to describe wastewater impacts on the chemistry of flowing waters (e.g., Kaushal et al., 2011) and macroinvertebrate assemblages (e.g., Singer and Battin, 2007; Calizza et al., 2012; Smucker et al., 2018) is common, there have been relatively few applications of stable isotope analyses to evaluate the impacts of urbanization on freshwater fishes (but see Mackintosh et al., 2017; Kupilas et al., 2021; Burbank et al., 2022). Urban-driven alterations to the thermal regime, riparian habitat, nutrients, hydrology and channel structure will all have implications for the availability and consumption of dietary items by freshwater fishes as well as for the habitat occupancy and the stoichiometry (Montaña and Schalk, 2018) of the affected ecosystems (Figure 1). Such stressors will alter feeding ecology and habitat occupancy of individual species and can result in changes in fish populations and their vital rates, community interactions, and physiology (e.g., O’Gorman et al., 2016; Korman et al., 2021). In this review paper we evaluate studies that have implemented stable isotope approaches to examine urban impacts, discuss promising stable isotope-based approaches that have not been commonly applied to the assessment of urban impacts, and highlight the advantages and limitations of the various approaches. In particular we discuss how stable isotope analyses can be used to understand and assess changes in the feeding ecology, habitat and thermal occupancy, and field metabolic rates of fishes as they respond to urban development impacts on lotic freshwater systems.
Trophic level and diet reconstructions
Urbanization can impact the availability of prey and result in diet shifts of freshwater fishes (Hall-Scharf et al., 2016; Kupilas et al., 2021). For instance, changes in riparian habitat associated with urbanization can reduce the proportion of high quality terrestrial prey consumed by freshwater fishes and impact prey production sources, with potential implications for growth, survival, and overall ecosystem function (Francis and Schindler, 2009; Burbank et al., 2022). Additionally, channelization and addition of contaminants can impact the prey species available to fishes resulting in altered diets (Northington and Hershey, 2006; de Carvalho et al., 2019). Stable isotope analyses provide insights into the trophic level, food chain length, the type of habitat prey are sourced from, the relative contribution of prey to the diet of fishes (i.e., diet reconstruction), and the resulting dietary niche breadth of freshwater fishes, all of which help understand shifts in the feeding ecology associated with urbanization. In general, nitrogen stable isotopes (δ15N) is indicative of trophic level and approaches have been developed to quantify trophic position based on the δ15N of fish muscle tissue (e.g., see Post (2002)). Shifts in the trophic position of urban-affected freshwater fishes have been estimated and quantified in several freshwater ecosystems such as the urbanized streams of Piedmont, United States (Helms et al., 2018), the Hawaiian islands (Lisi et al., 2018), the Kansas River (Eitzmann and Paukert, 2010) and constructed wetlands in Melbourne, Australia (Mackintosh et al., 2017). Additionally, there have been several studies that have examined trophic shifts experienced by freshwater fishes and invertebrates in response to wastewater impacts (Di Lascio et al., 2013; Loomer et al., 2014; de Carvalho et al., 2019). Such studies typically quantify the trophic shifts associated with the stressor. Estimates of mean trophic position and trophic shifts can be extended and applied to evaluate important ecological impacts such as to infer and examine changes in food chain length in response to urban activity (Singer and Battin, 2007; El-Sabaawi, 2018). Reported estimates of trophic position in urbanization impact studies have often failed to account for uncertainty arising from assumptions concerning trophic discrimination factors (e.g., McCutchan et al., 2003; Hussey et al., 2014) and isotopic baselines (Post, 2002). Instead of simply using point estimates, considerations of parameter variation (i.e., variance about the mean parameter estimate) for trophic discrimination factors and isotopic baselines can now be included in the estimation of trophic position using an R package called tROPHICPOSITION (Quezada-Romegialli et al., 2018). The package allows researchers to estimate trophic position from stable isotope data within a Bayesian framework using Markov Chain Monte Carlo (MCMC) simulations (Quezada-Romegialli et al., 2018). As an example, the package was used to estimate and evaluate trophic position of urban affected freshwater fish species occupying streams in South Africa to show decreases in food chain length with increasing temperature (Jackson et al., 2020).
Carbon stable isotopes (δ13C) of muscle tissue can also be used for understanding the habitat a fish is feeding in or sourcing its dietary items from (Phillips and Gregg, 2001). This is because δ13C values at the base of different food webs such as pelagic versus littoral (France, 1995) and terrestrial versus aquatic (France, 1997; Burbank et al., 2022) can be sufficiently distinct to allow for differentiation of the source of individuals diets (Figure 2). Depending on the type of food or environment food is sourced from, other stable isotopes such as hydrogen (δ2H) and sulfur (δ34S) can be valuable. δ2H can be particularly helpful when attempting to distinguish between autochthonous and allochthonous sources (Figure 2) because they have distinct δ2H values (e.g., allochthonous δ2H = ∼ −100 to −75 and autochthonous δ2H = ∼ −225 to −150; Burress et al., 2016; Hayden et al., 2021). In contrast δ34S can help identify estuarine or marine contributions to diet as δ34S values are typically elevated in marine food sources, thus δ34S can be valuable for examining anadromy (Figure 2). Biogeochemical differences in sediments (i.e., benthic habitats) versus plankton found in the water column can further facilitate use of δ34S for broadscale differentiation within and among complex freshwater habitats (e.g., Carr et al., 2017). For example, δ34S have been successfully used to help identify the contribution of sewage particles to the diet of mussels (Tucker et al., 1999) but not the diet of invertebrates in urban rivers (Morrissey et al., 2013). Nevertheless, one-isotope, two-source linear mixing models (Phillips and Gregg, 2001) can be used with any of the aforementioned isotopes to estimate the relative proportion of diet sourced from two different habitats using the equations (Phillips and Gregg, 2001):
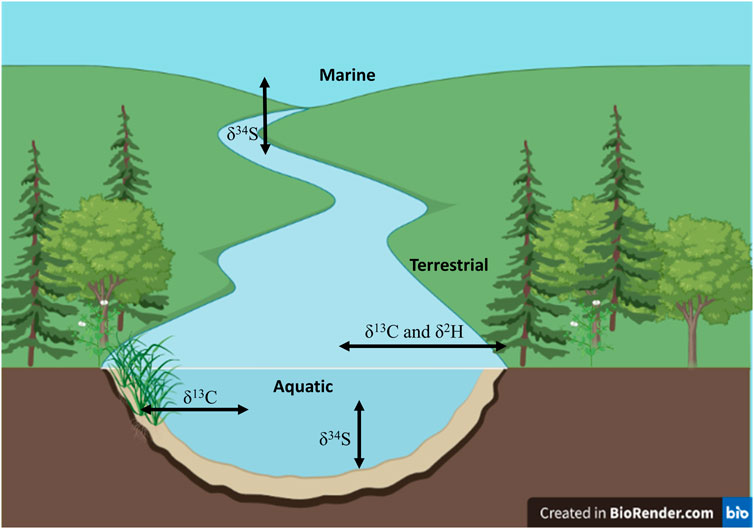
FIGURE 2. Schematic drawing illustrating the various stable isotopes that can be used to differentiate between use of different coupled environments (i.e. benthic-pelagic, littoral-pelagic, aquatic-terrestrial and freshwater-marine). The figure was created with BioRender.com.
Where αA and αB are the proportion of the diet sourced from habitat A and B respectively, δM is the isotopic value of the consumer (i.e., fish), δA is the mean end-member isotope value for habitat A and δB is the mean end-member isotope value for habitat B.
Generally, mixing model frameworks using stable isotopes have been developed to better understand and reconstruct the diet of fishes. Some of the most basic mixing model approaches are linear mixing models that incorporate one stable isotope and two sources or two isotopes and three sources (Phillips and Gregg, 2001). Linear mixing models have been expanded to include multiple isotopes with the ability to determine source contributions from a range of dietary sources using tools such as IsoSource (Phillips and Gregg, 2003). The isotopes commonly included in multi-isotope mixing models can include combinations of δ15N, δ13C, δ2H and δ34S depending on the objective of the study, the environment fish occupy and where the food resources are sourced from (e.g., aquatic vs. terrestrial). To further expand the capacity of multi-isotope mixing models, and better incorporate source uncertainty, researchers have formulated approaches to develop the mixing models in a Bayesian framework using MCMC simulations (Moore and Semmens, 2008). The expanded modelling approach, referred to as Bayesian stable isotope mixing models, is a powerful tool that allows for the reconstruction of fish diets as long as isotope values are available for both the fish (consumers) and their potential food resources (sources) (Parnell et al., 2013). Having suitable values available for the latter is important insofar as mixing modelling frameworks are constrained by their input data (Robinson et al., 2018). Bayesian stable isotope mixing models can be run using packages developed in R (Table 2) such as MixSIR (Moore and Semmens, 2008), IsotopeR (Hopkins and Zimmerman, 2014), SIAR (Parnell et al., 2010), simmr, and MixSIAR (Stock et al., 2018). The package creators have typically developed relatively straightforward and in-depth vignettes or manuals (e.g., Stock and Semmens, 2016) that help potential users learn to implement the package with their own data. To date the most flexible and advanced package for developing and implementing stable isotope mixing models is the MixSIAR package, which allows for the incorporation of prior information on diet with the use of informative prior distributions in a Bayesian modelling framework. The package further allows for consideration of both fixed and random effects and has the ability to use information criterion to estimate the relative support for different models developed with input data (Stock and Semmens, 2016; Stock et al., 2018). Bayesian stable isotope mixing model packages have been employed extensively by freshwater fish ecologists to reconstruct the diet of numerous fishes including Lake Trout (Salvelinus namaycush) in Lake Ontario (Colborne et al., 2016), Lake Sturgeon (Acipenser fulvescens) in Rainy River, Ontario Canada (Smith et al., 2016), and Eastern Sand Darter (Ammocrypta pellucida) in the Thames River, Ontario, Canada (Burbank et al., 2019), and would be equally useful to reconstruct diet of fishes occupying urbanized environments. In this respect, there have been few applications MixSIAR models to reconstruct the diet of urban affected freshwater fishes (but see Alonso et al., 2019; de Carvalho et al., 2019; Woodland et al., 2022). de Carvalho et al. (2019) found exotic species of guppies were consuming and assimilating notable amounts of sewage in wastewater impacted systems. Alonso et al. (2019) determined algae and periphyton were the most assimilated resource in polluted sites of the Rio de Velhas basin, Brazil. Furthermore, Woodland et al. (2022) identified that White Perch (Morone americana) decoupled from the benthic trophic pathway in the urban impacted creeks, indicating a shift in prey resource use in response to urbanization. Table 3 and Figure 3 illustrate tabular and graphical examples of the output provided by the MixSIAR package that consists of the posterior estimates of the relative contribution of each diet item to the diet of the consumer, in this case Eastern Sand Darter (Burbank et al., 2019). Given that the results are derived from a Bayesian model, users can obtain the median (50%) and 95% credible interval (lower:2.5% and upper:97.5%) for reporting results.
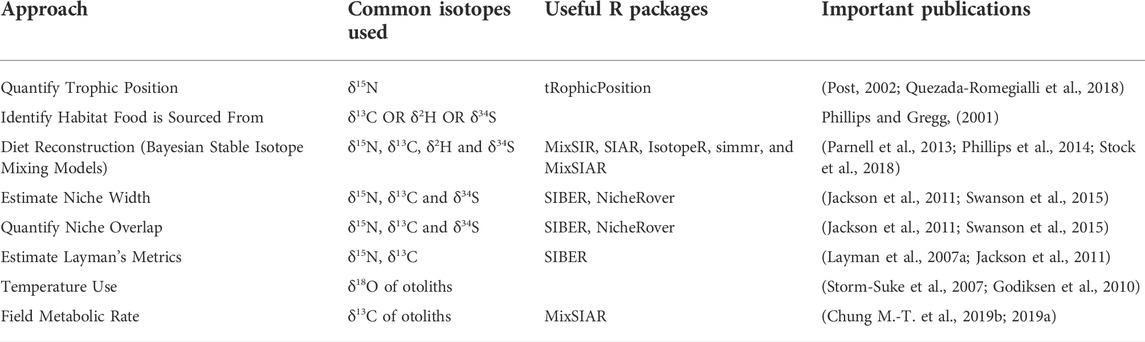
TABLE 2. Stable isotope-based approaches proposed for evaluating urbanization impacts on freshwater fishes, the isotopes commonly used for each approach, and useful R packages that can be used to implement each approach.
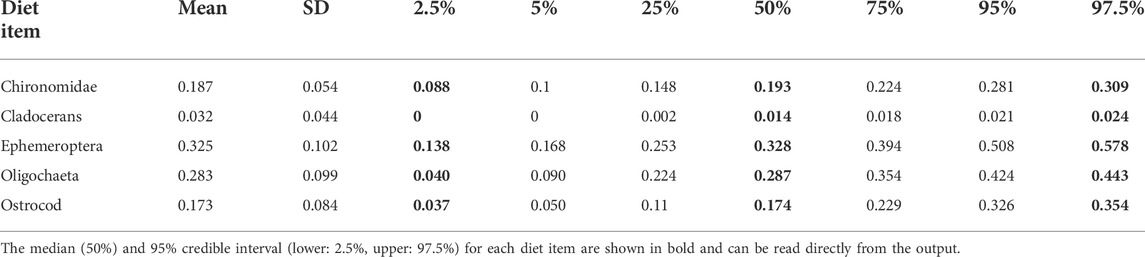
TABLE 3. An example of the output provided by the MixSIAR package, which shows the posterior estimates of the percent of each prey item consumed by Eastern Sand Darter in the Thames River, Ontario, Canada estimated in Burbank et al. (2019). The median (50%) and 95% credible interval (lower:2.5%, upper:97.5%) for each diet item are bolded and can be read directly from the output.
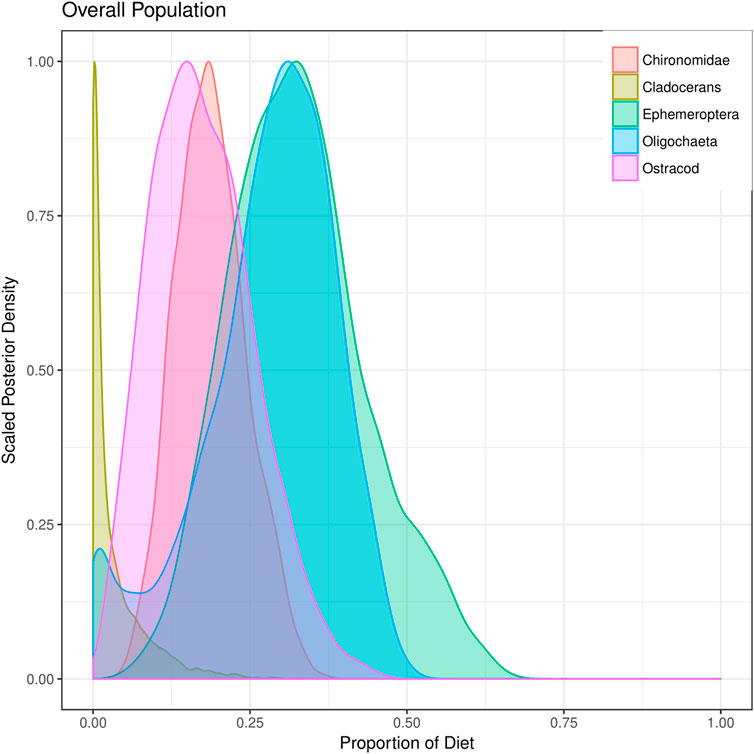
FIGURE 3. An example of the graphical output provided by the MixSIAR package, which shows the posterior density distributions of each prey item consumed by Eastern Sand Darter in the Thames River, Ontario, Canada estimated in Burbank et al. (2019).
It is important to note that stable isotope approaches for quantifying trophic position and diet reconstructions require caution with regard to the data to be collected. Appropriate baseline samples should be collected from sample sites and relevant trophic discrimination factors must be used (Post, 2002; Kristensen et al., 2016). When comparing among locations baseline isotope values must be accounted for and, when appropriate, baseline corrections should be implemented to ensure comparisons are valid (Cabana and Rasmussen, 1996; Kristensen et al., 2016). Understanding baselines in urbanized streams and rivers is particularly important given possible point source inputs (i.e., sewage plants) that can significantly alter downstream isotope values (e.g., Ulseth and Hershey, 2005). Lipid corrections should also be considered and employed when the C:N ratio is > 3.5 (Skinner et al., 2016). Substantial literature exists to guide researchers on the need and use of lipid corrections (Post et al., 2007; De Lecea and De Charmoy, 2015). Additionally, when employing Bayesian stable isotope mixing models to reconstruct diets, all relevant potential diet sources should be included to ensure models converge and appropriately represent a species diet (Stock et al., 2018). It is essential that the assumptions and data requirements of each R package are thoroughly followed to ensure the proper use of each tool.
Despite several important technical considerations, the quantification of trophic position, identification of the habitat where dietary items are sourced from, and diet reconstruction can be valuable for understanding shifts in the feeding ecology of freshwater fishes in response to urban development (Steffy and Kilham, 2004; Eitzmann and Paukert, 2010). For example, Helms et al. (2018) used δ15N to determine that redbreast sunfish (Lepomis auritus) occupied a lower trophic position in urban streams compared to non-urban streams, highlighting altered functional roles in response to urbanization. Lisi et al. (2018) used δ15N to demonstrate that an omnivorous goby species (Awaous stamineus) occupied a higher trophic position in urban catchments compared to forested catchments, highlighting that the species exhibits a variable diet that can help buffer it against the environmental degradation associated with urbanization. In contrast, Kupilas et al. (2021) evaluated δ13C in Brown Trout (Salmo trutta) occupying urban sites with and without riparian buffers in the Oslo Fjord Basin, Norway, to show that reaches with a riparian buffer facilitated a greater reliance on terrestrially-sourced carbon and suggested that riparian buffers may be important for the persistence of Brown Trout in urban streams. These examples show that stable isotopes are useful for understanding the impacts of urbanization on the feeding ecology of affected fishes and demonstrating how freshwater fishes respond to urban development (Table 4). Our literature review suggests that urbanization typically impacts the feeding ecology of freshwater fishes, but the impacts are regionally specific and are not consistent across fishes. It is also evident from reviewing the literature that stable isotope-based approaches are underutilized for understanding urban impacts. For example, searches on the Web of Science using urbanization and fishes and stable isotopes yield only 3.3% of the results compared to searches using urbanization and fishes. After an extensive search of the literature, we identified only 68 papers that examined stable isotope values of freshwater fish in urban impacted systems. Stable isotope-based diet reconstructions in urban streams are particularly lacking. However, the implementation of this tool can help to better understand how urbanization impacts the feeding ecology of a range of freshwater fishes given that changes in feeding are often precursors to changes in vital rates such as growth. More recently researchers have used stable isotopes from specimens at museum collections to evaluate alterations in basal resource use (e.g., Turner et al., 2015) and feeding ecology of freshwater fish populations and communities (e.g., Kishe-Machumu et al., 2017) in response to colonization and historical urban development over longer temporal scales. To date, studies that exist have largely focused on single species assessments and most often highlight general shifts in food sources (i.e., allochthonous versus autochthonous) and trophic position (e.g., Helms et al., 2018; Lisi et al., 2018; Kupilas et al., 2021). While useful, stable isotope analyses can be used to assess more subtle shifts, such as changes in prey consumption, and can be applied to understand the implications of the simplification of invertebrate communities for freshwater fishes in response to urbanization. For instance, the availability and consumption of high quality benthic and terrestrially derived prey that would be expected to be negatively affected by habitat loss and degradation associated with urban development can be evaluated with the methods described above. Future research in urban environments should employ the stable isotope-based approaches described in this section to highlight current, future or past changes in feeding ecology that can arise in response to urban development.
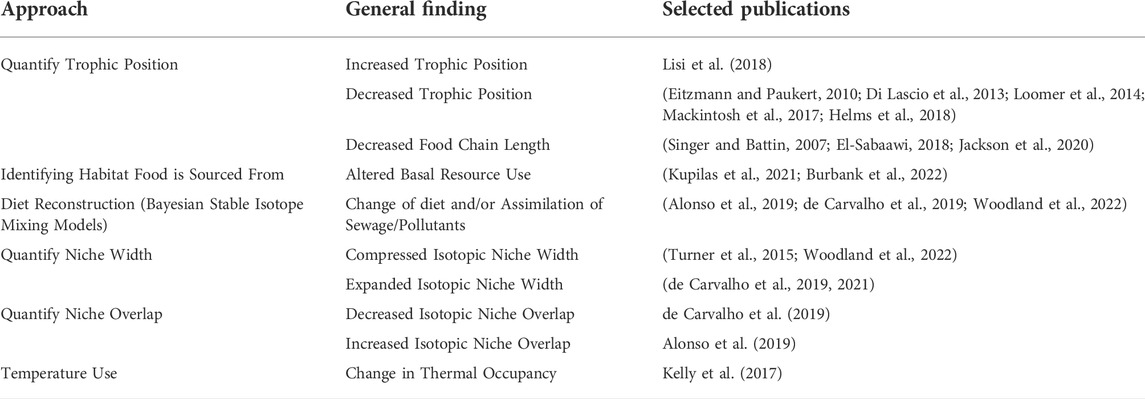
TABLE 4. Selected publications that use various stable isotope-based approaches to evaluate the impacts of urbanization on freshwater fishes. The general findings were organized based on the approaches outlined in Table 2.
Isotopic niche width and overlap
Urban development can have substantial impacts on the realized niche of fishes (Woodland et al., 2022) via alterations to the competitive interactions among species. Understanding shifts in realized niche and competitive interactions is foundational for evaluating the impacts of urbanization. The isotopic niche concept, first formalized in 2007 (Newsome et al., 2007), is representative of the Hutchinson’s niche concept (Hutchinson, 1957) within isotopic (typically δ13C and δ15N) space as it encompasses both bionomic (i.e., diet) and scenopoetic (i.e., habitat) axes. Thus, the isotopic niche width of a species is a useful metric for understanding the breadth of dietary resources used and/or the habitat exploited. As niche constriction is often associated with increased risks of local extirpation (e.g., Scheele et al., 2017; Le Feuvre et al., 2021), changes in the isotopic niche breadth can be used as a measure of the potential resilience of species to changes in resource and habitat availability (Layman et al., 2007b) arising from urban development (Figure 1). A narrow niche implies that a species is a specialist (Vander Zanden et al., 2010) and may indicate increased vulnerability to changes in resource availability due to urbanization and other threats (e.g., invasive species) compared to generalist species with wider niches (McKinney, 1997; Burbank et al., 2019).
Isotopic niche overlap is another valuable tool for assessing the extent to which species within a community, or individuals within populations, exploit similar space and dietary resources (Layman et al., 2007a; Newsome et al., 2007). Understanding isotopic niche overlap may help inform the potential for competitive interactions and resource partitioning that might occur following urban development. For instance, if species exhibit high overlap within isotopic space, it indicates they exploit similar resources, which is a precursor to competition. Alternatively, low isotopic niche overlap among species is indicative of differential resource use and niche segregation, which could help buffer against the negative impacts of urban development (Lisi et al., 2018). In response to urbanization, a changing prey base may influence the dietary niche of fishes (whether overlapping or segregated), so the examination of isotopic niche overlap can be a valuable tool for understanding shifts in species trophic interactions. Layman et al. (2007a) proposed a set of metrics associated with isotopic niche among community members deemed “Layman’s metrics” that include δ15N range, δ13C range, total area of the convex hull, mean distance to centroid, mean nearest neighbour distance and standard deviation of the nearest neighbor distance. δ15N range helps provide information on the trophic length of a community and δ13C range can provide insight into the diversity of basal resources (see Trophic Level and Diet Reconstruction section). Total area of the convex hull represents the total amount of isotopic niche space occupied by a fish community. Mean distance to centroid quantifies the average extent of trophic diversity in a community, mean nearest neighbor distance represents how densely species cluster in isotopic space, and standard deviation of nearest neighbor distance represents the evenness of species clustering. Together these metrics can be helpful for understanding how community members interact in isotopic space but also for understanding changes in community structure associated with urbanization (Layman et al., 2007a; Layman, 2007; Jackson et al., 2011).
Several useful methods and R packages have been developed to estimate isotopic niche width, evaluate isotopic niche overlap, and compute Layman metrics (Table 2). Initially, isotopic niche was estimated as the convex hull area occupied by a species in bi-variate isotope space (Newsome et al., 2007). Jackson et al. (2011) then developed the Stable Isotope Bayesian Ellipses in R (SIBER) package, which placed isotopic niche width estimates and Layman’s metrics into a Bayesian framework. The Bayesian approach reduced result sensitivity to sample size and allowed uncertainty to be incorporated into the estimates of niche width. The SIBER package estimates niche width as a standard ellipse area corrected for small sample sizes (SEAC), with the standard ellipse in bivariate space being conceptually equivalent to the standard deviation in univariate space. SEAC is the area of an ellipse that contains 40% of the data in bivariate space with the incorporation of a correction for small sample sizes and is representative of the niche width of a species in isotopic space (Jackson et al., 2011; Mumby et al., 2018), but may be scaled to include more or less of the data as required. The SIBER package also facilitates estimates of SEAC in a Bayesian framework (SEAB) with a user specified number of iterations. Additionally, the package allows for the geometric estimation of the area of overlap among two standard ellipse areas that is representative of niche overlap (Jackson et al., 2012; Guzzo et al., 2013). More recently, Swanson et al. (2015) developed NicheRover to incorporate n ≥ 2 information vectors allowing for the use of more than two isotopes or the combination of isotopes with other information (e.g., temperature) to estimate niches more compatible with Hutchinson’s original n-dimensional hypervolume definition (Hutchinson, 1957). NicheRover also computes bi-directional overlap by estimating the extent of niche overlap from the perspective of either species (i.e., species A overlap on species B niche and vice versa). With this approach, the asymmetry of niche overlap can be assessed and interpreted (see NicheRover vignette). For instance, if species A has a large niche and species B has a small niche, with the niche of B almost entirely contained within the niche of A, the probability of B overlapping with A would be very high, but the probability of A overlapping with B would be low (e.g., Swanson et al., 2015; Burbank et al., 2019). One interpretation of such asymmetries is that one species (B) is more exposed to resource competition with the other species (A). Such advancements significantly improve the capacity to define niche space and overlap in isotopic space and can help elucidate both potential competitive interactions and resource portioning among freshwater fishes. Thus, we suggest using the NicheRover package when estimating niche width and overlap in urbanized ecosystems. As with the isotope mixing models, authors of the SIBER and NicheRover packages provide in-depth and easy to follow vignettes to teach practitioners how to use the packages with their data. It is essential that these vignettes are examined thoroughly and that all relevant assumptions and cautions are taken when using the above packages for estimating isotopic niche width and overlap. For instance, the SIBER package is relatively insensitive to sample size but recommends that a minimum of n = 10 samples are used per group (Jackson et al., 2011).
Stable isotope techniques can be applied to understand how fish populations and communities are impacted by the range of stressors associated with urbanization that affects both feeding ecology and habitat occupancy of freshwater fishes (Figure 1; Table 4). For example, de Carvalho et al. (2019) quantified niche width and overlap of native (Phalloceros uai) and exotic (Poecilia reticulata) guppies in polluted Brazilian waters and found the species exhibited wider isotopic niches but less isotopic niche overlap in polluted compared to unpolluted sites, indicating increased niche segregation in polluted waters. de Carvalho et al. (2021) found increases in the isotopic niche width of fish communities in polluted compared to non-polluted sites of the Rio das Velhas basin, Brazil. Whereas, Alonso et al. (2019) observed increased overlap of the isotopic niche of Astyanax taeniatus and Astyanax lacustris in polluted waters in Brazil, suggesting niche compression in response to reduced prey diversity. Woodland et al. (2022) found the isotopic niche width of white perch (Morone americana) contracted in response to increases in impervious surfaces, likely as a result of homogenization of diet and a less diverse forage base, in urbanized sub-estuaries of the Patapsco River, Maryland. Furthermore, Turner et al. (2015) used museum collections gathered for over 70 years to identify reductions in isotopic niche width of freshwater fishes and increased δ15N of the entire fish community inhabiting urban affected reaches in response to sedimentation and channelization in the highly urbanized and regulated Rio Grande system, United States.
It is evident that measures of isotopic niche width and overlap provide valuable insight into how different species respond to increased urban pressure and how interspecific interactions may be impacted by continued urban development. Quantification of isotopic niche width and overlap can be particularly valuable for predicting the sensitivity of species to urban impacts and quantifying changes in diet, habitat use, competitive interactions and niche segregation in response to urbanization.
Temperature use
Urban-driven changes in stream and river temperatures (e.g., Kinouchi et al., 2007; Nelson and Palmer, 2007; Kaushal et al., 2010) will have consequences for resident fish species, which may include acute and chronic thermal stress for fish unable to disperse to suitable thermal refugia (Timm and Ouellet, 2021) and changes in seasonal phenology such as emergence date and growth-related effects (Spanjer et al., 2018). Given the importance of temperature for fish (e.g., Magnuson et al., 1979) it is important to understand whether individual fish can exploit habitat heterogeneity (i.e., find thermal refugia) to offset the effects of warming waters. One method of doing so is through the use of otolith thermometry which allows the oxygen isotope measures (δ18O) of otolith calcium carbonate to be translated to temperature (°C) via experimentally validated empirical relationships (e.g., Godiksen et al., 2010—Arctic Charr (Salvelinus alpinus); Willmes et al., 2019—Delta Smelt (Hypomesus transpacificus); Burbank et al., 2020—Silver Shiner). The approach has proven useful for separating fish species by thermal habitat use (Power et al., 2012; Kelly et al., 2015) and could be effectively applied to understand the thermal habitats occupied by freshwater fishes in anthropogenic affected environments. For instance, otolith δ18O has been used to evaluate the temperature use of Brook Charr (Salvelinus fontinalis) and Slimy Sculpin (Cottus cognatus) in response to dam operation by comparing temperature use in both regulated and unregulated rivers in Ontario, Canada (Kelly et al., 2017). The study found Slimy Sculpin, but not Brook Charr, used significantly cooler water temperatures below dams. Additionally, otolith δ18O can be useful for understanding thermal niche segregation that may help facilitate coexistence (Power et al., 2012) under changing environmental conditions, or in response to new biotic interactions that can arise from species invasion (Kelly et al., 2015), or changes in fish community interactions. Changes in riparian habitat and channelization associated with urban development typically lead to changes in the thermal regime (Nelson and Palmer, 2007) and otolith thermometry can be applied to quantify how relative temperature use changes through time or across migration routes. The exploitation of thermal refugia within urban streams such as localized regions of groundwater upwelling (Power et al., 1999) can be examined and identified by comparing occupied temperature to the dominant thermal regime. Otolith thermometry is a relatively cost-effective approach that can provide insight into how species cope with increasing temperatures in the face of urbanization and may help refine estimates of habitat use.
There are several important considerations when applying δ18O in otoliths to understand temperature use. For example, when attempting to reconstruct realized temperature use with previously developed otolith thermometry equations, quantification of δ18O in the water where fish were captured is required to calculate otolith isotope fractionation (Godiksen et al., 2010). Additionally, the method is confined to situations where archived otoliths are available or lethal sampling is permitted (Burbank et al., 2020). Nevertheless, otolith thermometry can be applied to a wide range of fishes to understand realized or relative temperature use within and among species in the face of continuing urbanization. The approach is well suited to small bodied fishes when alternative technologies such as telemetry are not practical because of tag size. Additionally, otoliths of specimens archived in museum collections can be examined to retrospectively quantify historical impacts of urbanization on thermal occupancy of fishes that experienced shifts in the thermal regime long before the widespread availability of thermal tagging technology (Surge and Walker, 2005). Otolith thermometry may be particularly useful in understanding shifts in temperature use in response to urban development because micro-milling or secondary ion mass spectrometry (SIMS) techniques allow for thermal occupancy to be estimated over the course of an individual’s life (Helser et al., 2018).
Field metabolic rates
Field metabolic rate is a critical measure for evaluating how species are impacted by environmental stressors (Chung M.-T. et al., 2019a). Urban development is expected to have significant impacts on fish metabolic rates through a range of pathways (Figure 1) i.e., via diet quality (Francis and Schindler, 2009) or changes to the thermal envelop in which fish reside (Nelson and Palmer, 2007). Quantifying the impacts of urbanization on the field metabolic rate of freshwater fishes is difficult as a result of the significant lack of metabolic information for fish derived under field conditions (Treberg et al., 2016). However, the analysis of δ13C in fish otoliths has been shown to be representative of fish field metabolic rates (Chung M.-T. et al., 2019a; Martino et al., 2020) and is a promising tool for quantifying changes in metabolic rate in response to urbanization and environmental stressors. For example, Sinnatamby et al. (2015) observed increases in otolith δ13C estimated field metabolic rates in young-of-year Arctic Charr as a function of increasing latitude, suggesting increased food consumption in high-latitude populations during the summer feeding period. Wurster et al. (2005) used otolith δ13C values of Chinook Salmon (Oncorhynchus tshawytscha) in Lake Ontario to track shifts in field metabolic rate that were associated with alterations in habitat use. It should be noted, however, that otolith δ13C based estimates of field metabolic rate are influenced by many processes such as feeding, movement, thermoregulation, reproduction, growth and aggression, and as a consequence may be difficult to interpret (Sinnatamby et al., 2015; Chung M.-T. et al., 2019b). Nevertheless, metabolic rates inferred from field-based otolith δ13C are advantageous when compared to laboratory-based measures because they relate directly to cumulative fish behaviours in the wild (Chung M.-T. et al., 2019b).
Otolith δ13C field metabolic rate can be estimated using a two component mixing model that includes consideration of dietary δ13C (i.e., muscle tissue δ13C), otolith δ13C and the δ13C of dissolved organic carbon (DIC) in occupied waters (Schwarcz et al., 1998; Solomon et al., 2006; Chung M.-T. et al., 2019a, Chung M.-T. et al., 2019 M. T.b). The MixSIAR package in R (Stock and Semmens, 2016; Stock et al., 2018) can be used to perform the two component mixing model within a Bayesian framework (Chung M.-T. et al., 2019b). While there are several measurements required to estimate otolith δ13C metabolic rate (diet δ13C, otolith δ13C and δ13C of DIC in water), if researchers are conducting stable isotope-based feeding ecology investigations and/or otolith δ18O thermal reconstructions, otolith-based field metabolic rate estimates are a relatively straightforward add-on to such studies and may be a valuable tool for tracking the influence of urbanization stresses on fish physiology.
Synthesis and recommendations
The most drastic human interventions on aquatic ecosystems tends to be realized within urban environments, thus freshwater fish populations and communities are subjected to a wide variety of stressors in the face of continued urbanization (Cohen, 2003; Tickner et al., 2020). Therefore, ecologists must take an active approach when evaluating and quantifying the impacts of urbanization on freshwater ecosystems by using the wide variety of tools available. Doing so can help understand and mitigate the effects of urbanization on freshwater fishes and ecosystems. We have highlighted stable isotope analyses as useful tools for improving our understanding of the impacts of urbanization on freshwater fishes (Table 4). Stable isotope analyses can be used in conjunction with other approaches such as life-history based, contaminant-based and habitat-based approaches to gain a broader and more comprehensive understanding of how fish populations and communities are responding to the myriad of habitat stressors associated with urbanization. Stable isotope-based approaches are particularly useful for understanding urban impacts as they can equally point to urban-driven habitat degradation and the response of populations and communities to habitat change. Based on surveys of the literature to date, it is evident that stable isotope approaches are underutilized by researchers in assessing urbanization impacts on freshwater fish populations and communities. To further their use in that regard, we have provided an overview of how stable isotope analyses can be effectively and easily applied to investigate, quantify, and understand the impacts of urban development on freshwater fish populations and communities. In particular, we show how stable isotope analyses can help address both the ecological and physiological impacts of urban development on freshwater fishes in hopes of inspiring more frequent use of the approaches in urbanization studies. In addition to assessing impacts of urbanization, stable isotope analyses can be useful for assessing the ecological response of fishes in urban systems that have undergone habitat restoration.
Stable isotope-based examination of fish feeding ecology in response to urban development is a promising approach for quantifying how changes in riparian habitat, instream temperature, nutrients, contaminants, food supply, hydrology, and sedimentation can impact prey consumption by freshwater fishes. Stable isotope-based methods can be easily implemented to investigate potential shifts in the trophic level, which habitats food is sourced from, and the overall diet of freshwater fishes to better understand impacts on the feeding ecology of fish populations and communities. As such shifts are typically important predecessors of wider population effects, stable isotopes have the potential to be used as important indicator metrics. Additionally, isotopic niche width and overlap measures provide valuable tools for understanding how fish cope with urban stressors, for evaluating potential competitive interactions and niche segregation among species, and for assessing changes in habitat use in the face of urbanization. Otolith-based work such a δ18O analysis can help researchers uncover and track changes in temperature and habitat use in response to shifts in thermal regimes associated with urbanization and should be considered for use with archived otolith collections or future sampling programs. Otolith δ13C data also has promise for understanding changes in field metabolic rate in response to the wide range of urban related stressors and providing insights into urban impacts on freshwater fish physiology. Critically, advancements in mass spectrometry have made stable isotope analyses both a widely available and cost-effective tool for use in urban impact studies. As we continue to face increasing urban development globally, researchers should make more frequent use of stable isotope analyses to gain a more comprehensive understanding of the impacts of urbanization on freshwater fish populations and communities and to advance our knowledge of urban impacts on freshwater ecosystems as a whole.
Author contributions
JB helped conceptualize the idea and wrote the manuscript, DD helped conceptualize the idea, reviewed the manuscript and provided funding, MP helped conceptualize the idea, helped with writing, reviewed the manuscript and provided funding.
Funding
For this study was provided by a NSERC discovery grant to MP, and NSERC PGS-D scholarship to JB, and Fisheries and Oceans Canada.
Acknowledgments
We thank two reviewers for comments that improved the manuscript.
Conflict of interest
The authors declare that the research was conducted in the absence of any commercial or financial relationships that could be construed as a potential conflict of interest.
Publisher’s note
All claims expressed in this article are solely those of the authors and do not necessarily represent those of their affiliated organizations, or those of the publisher, the editors and the reviewers. Any product that may be evaluated in this article, or claim that may be made by its manufacturer, is not guaranteed or endorsed by the publisher.
References
Adams, S. M. (2002). Biological indicators of aquatic ecosystem stress. Bethesda, Maryland: American Fisheries Society.
Alonso, M. B., de Carvalho, D. R., Alves, C. B. M., Moreira, M. Z., and Pompeu, P. S. (2019). Changes in trophic characteristics of two fish species of astyanax (teleostei: Characidae) in response to aquatic pollution. Zoologia 36, 1–12. doi:10.3897/zoologia.36.e30445
Baer, K. E., and Pringle, C. M. (2000). “Special problems of urban river conservation: The encroaching megapolis,” in Global perspectives in river conservation. Editors P. J. Boon, B. Davies, and G. E. Petts (Chichester, UK: John Wiley & Sons), 385–402.
Boecklen, W. J., Yarnes, C. T., Cook, B. A., and James, A. C. (2011). On the use of stable isotopes in trophic ecology. Annu. Rev. Ecol. Evol. Syst. 42, 411–440. doi:10.1146/annurev-ecolsys-102209-144726
Booth, D. B., and Jackson, C. R. (1997). Urbanization of aquatic systems: Degradation thresholds, stormwater detection and the limits of mitigation. J. Am. Water Resour. Assoc. 33, 1077–1090. doi:10.1111/j.1752-1688.1997.tb04126.x
Booth, D. B., Roy, A. H., Smith, B., and Capps, K. A. (2016). Global perspectives on the urban stream syndrome. Freshw. Sci. 35, 412–420. doi:10.1086/684940
Brookes, A., Gregory, K. J., and Dawson, F. (1983). An assessment of river channelization in England and Wales. Sci. Total Environ. 27, 97–111. doi:10.1016/0048-9697(83)90149-3
Burbank, J., Drake, D. A. R., and Power, M. (2020). Field-based oxygen isotope fractionation for the conservation of imperilled fishes: An application with the threatened silver shiner Notropis photogenis. Endanger. Species Res. 42, 83–93. doi:10.3354/ESR01040
Burbank, J., Drake, D. A. R., and Power, M. (2022). Seasonal consumption of terrestrial prey by a threatened stream fish is influenced by riparian vegetation. Endanger. Species Res. 47, 15–27. doi:10.3354/esr01161
Burbank, J., Finch, M., Drake, D. A. R., and Power, M. (2019). Diet and isotopic niche of eastern sand darter (Ammocrypta pellucida) near the northern edge of its range: A test of niche specificity. Can. J. Zool. 97, 763–772. doi:10.1139/cjz-2018-0291
Burress, E. D., Holcomb, J. M., and Armbruster, J. W. (2016). Ecological clustering within a diverse minnow assemblage according to morphological, dietary and isotopic data. Freshw. Biol. 61, 328–339. doi:10.1111/fwb.12710
Cabana, G., and Rasmussen, J. B. (1996). Comparison of aquatic food chains using nitrogen isotopes. Proc. Natl. Acad. Sci. U. S. A. 93, 10844–10847. doi:10.1073/pnas.93.20.10844
Calizza, E., Costantini, M. L., Rossi, D., Carlino, P., and Rossi, L. (2012). Effects of disturbance on an urban river food web. Freshw. Biol. 57, 2613–2628. doi:10.1111/fwb.12033
Carpenter, S. R., Stanley, E. H., and Vander Zanden, M. J. (2011). State of the world’s freshwater ecosystems: Physical, chemical, and biological changes. Annu. Rev. Environ. Resour. 36, 75–99. doi:10.1146/annurev-environ-021810-094524
Carr, M. K., Jardine, T. D., Doig, L. E., Jones, P. D., Bharadwaj, L., Tendler, B., et al. (2017). Stable sulfur isotopes identify habitat-specific foraging and mercury exposure in a highly mobile fish community. Sci. Total Environ. 586, 338–346. doi:10.1016/j.scitotenv.2017.02.013
Chung, M.-T., Trueman, C. N., Godiksen, J. A., Holmstrup, M. E., and Grønkjær, P. (2019a). Field metabolic rates of teleost fishes are recorded in otolith carbonate. Commun. Biol. 2, 24. doi:10.1038/s42003-018-0266-5
Chung, M.-T., Trueman, C. N., Godiksen, J. A., and Grønkjær, P. (2019b). Otolith δ 13 C values as a metabolic proxy: Approaches and mechanical underpinnings. Mar. Freshw. Res. 70, 1747. doi:10.1071/MF18317
Cohen, J. E. (2003). Human population: The next half century. Sci. (80-. ) 302, 1172–1175. doi:10.1126/science.1088665
Colborne, S. F., Rush, S. A., Paterson, G., Johnson, T. B., Lantry, B. F., and Fisk, A. T. (2016). Estimates of lake trout (Salvelinus namaycush) diet in Lake Ontario using two and three isotope mixing models. J. Gt. Lakes. Res. 42, 695–702. doi:10.1016/j.jglr.2016.03.010
Collier, T. K., Johnson, L. L., Stehr, C. M., Myers, M. S., and Stein, J. E. (1998). A comprehensive assessment of the impacts of contaminants on fish from an urban waterway. Mar. Environ. Res. 46, 243–247. doi:10.1016/S0141-1136(98)00005-1
de Carvalho, D. R., Flecker, A. S., Alves, C. B. M., Sparks, J. P., and Pompeu, P. S. (2019). Trophic responses to aquatic pollution of native and exotic livebearer fishes. Sci. Total Environ. 681, 503–515. doi:10.1016/j.scitotenv.2019.05.092
de Carvalho, D. R., Sparks, J. P., Flecker, A. S., Alves, C. B. M., Moreira, M. Z., and Pompeu, P. S. (2021). Nitrogen pollution promotes changes in the niche space of fish communities. Oecologia 197, 485–500. doi:10.1007/s00442-021-05029-z
De Lecea, A. M., and De Charmoy, L. (2015). Chemical lipid extraction or mathematical isotope correction models: Should mathematical models be widely applied to marine species? Rapid Commun. Mass Spectrom. 29, 2013–2025. doi:10.1002/rcm.7310
Di Lascio, A., Rossi, L., Carlino, P., Calizza, E., Rossi, D., and Costantini, M. L. (2013). Stable isotope variation in macroinvertebrates indicates anthropogenic disturbance along an urban stretch of the river Tiber (Rome, Italy). Ecol. Indic. 28, 107–114. doi:10.1016/j.ecolind.2012.04.006
Eitzmann, J. L., and Paukert, C. P. (2010). Urbanization in a great plains river: Effects on fishes and food webs. River Res. Appl. 26, 948–959. doi:10.1002/rra.1326
El-Sabaawi, R. (2018). Trophic structure in a rapidly urbanizing planet. Funct. Ecol. 32, 1718–1728. doi:10.1111/1365-2435.13114
France, R. L. (1995). Differentiation between littoral and pelagic food webs in lakes using stable carbon isotopes. Limnol. Oceanogr. 40, 1310–1313. doi:10.4319/lo.1995.40.7.1310
France, R. L. (1997). Stable carbon and nitrogen isotopic evidence for ecotonal coupling between boreal forests and fishes. Ecol. Freshw. Fish. 6, 78–83. doi:10.1111/j.1600-0633.1997.tb00147.x
Francis, T. B., and Schindler, D. E. (2009). Shoreline urbanization reduces terrestrial insect subsidies to fishes in North American lakes. Oikos 118, 1872–1882. doi:10.1111/j.1600-0706.2009.17723.x
Gido, K. B., Bertrand, K. N., Murdock, J. N., Dodds, W. K., and Whiles, M. R. (2010). Community Ecol. stream fishes concepts, approaches Tech, 593–617.Disturbance-mediated effects of fishes on stream ecosystem processes: Concepts and results from highly variable prairie streamsAm. Fish. Soc. Bethesda, Maryl.
Godiksen, J. A., Svenning, M. A., Dempson, J. B., Marttila, M., Storm-Suke, A., and Power, M. (2010). Development of a species-specific fractionation equation for arctic charr (Salvelinus alpinus (L.)): An experimental approach. Hydrobiologia 650, 67–77. doi:10.1007/s10750-009-0056-7
Guy, C., and Brown, M. (2007). Analysis and interpertation of freshwater Fisheries data. Bethesda, Maryland: American Fisheries Society.
Guzzo, M. M., Haffner, G. D., Legler, N. D., Rush, S. A., and Fisk, A. T. (2013). Fifty years later: Trophic ecology and niche overlap of a native and non-indigenous fish species in the Western basin of lake erie. Biol. Invasions 15, 1695–1711. doi:10.1007/s10530-012-0401-z
Hall-Scharf, B. J., Switzer, T. S., and Stallings, C. D. (2016). Ontogenetic and long-term diet shifts of a generalist juvenile predatory fish in an urban estuary undergoing dramatic changes in habitat availability. Trans. Am. Fish. Soc. 145, 502–520. doi:10.1080/00028487.2016.1143396
Hayden, B., Tongnunui, S., Beamish, F. W. H., Nithirojpakdee, P., Soto, D. X., and Cunjak, R. A. (2021). Functional and trophic diversity of tropical headwater stream communities inferred from carbon, nitrogen and hydrogen stable isotope ratios. Food Webs 26, e00181. doi:10.1016/j.fooweb.2020.e00181
Helms, B. S., Bickford, N. A., Tubbs, N. W., and Feminella, J. W. (2018). Feeding, growth, and trophic position of redbreast sunfish (Lepomis auritus) in watersheds of differing land cover in the lower Piedmont, USA. Urban Ecosyst. 21, 107–117. doi:10.1007/s11252-017-0696-8
Helser, T. E., Kastelle, C. R., McKay, J. L., Orland, I. J., Kozdon, R., and Valley, J. W. (2018). Evaluation of micromilling/conventional isotope ratio mass spectrometry and secondary ion mass spectrometry of δ 18 O values in fish otoliths for sclerochronology. Rapid Commun. Mass Spectrom. 32, 1781–1790. doi:10.1002/rcm.8231
Høie, H., Otterlei, E., and Folkvord, A. (2004). Temperature-dependent fractionation of stable oxygen isotopes in otoliths of juvenile cod (Gadus morhua L.). ICES J. Mar. Sci. 61, 243–251. doi:10.1016/j.icesjms.2003.11.006
Hopkins, R. L., and Zimmerman, B. (2014). First observation of the eastern Sand darter (Ammocrypta pellucida) in raccoon creek (Ohio river basin) in southeastern Ohio in 57 years. Northeast. Nat. (Steuben). 21, N13–N17. doi:10.1656/045.021.0116
Hussey, N. E., Macneil, M. A., Mcmeans, B. C., Olin, J. A., Dudley, S. F. J., Cliff, G., et al. (2014). Rescaling the trophic structure of marine food webs. Ecol. Lett. 17, 239–250. doi:10.1111/ele.12226
Hutchinson, G. E. (1957). Concluding remarks. Cold Spring Harb. Symp. Quant. Biol. 22, 415–427. doi:10.1101/sqb.1957.022.01.039
Jackson, A. L., Inger, R., Parnell, A. C., and Bearhop, S. (2011). Comparing isotopic niche widths among and within communities: SIBER - stable isotope bayesian ellipses in R. J. Anim. Ecol. 80, 595–602. doi:10.1111/j.1365-2656.2011.01806.x
Jackson, M. C., Donohue, I., Jackson, A. L., Britton, J. R., Harper, D. M., and Grey, J. (2012). Population-level metrics of trophic structure based on stable isotopes and their application to invasion ecology. PLoS One 7, e31757. doi:10.1371/journal.pone.0031757
Jackson, M. C., Fourie, H. E., Dalu, T., Woodford, D. J., Wasserman, R. J., Zengeya, T. A., et al. (2020). Food web properties vary with climate and land use in South African streams. Funct. Ecol. 34, 1653–1665. doi:10.1111/1365-2435.13601
Kaushal, S. S., Groffman, P. M., Band, L. E., Elliott, E. M., Shields, C. A., and Kendall, C. (2011). Tracking nonpoint source nitrogen pollution in human-impacted watersheds. Environ. Sci. Technol. 45, 8225–8232. doi:10.1021/es200779e
Kaushal, S. S., Likens, G. E., Jaworski, N. A., Pace, M. L., Sides, A. M., Seekell, D., et al. (2010). Rising stream and river temperatures in the United States. Front. Ecol. Environ. 8, 461–466. doi:10.1890/090037
Kelly, B., Amundsen, P. A., and Power, M. (2015). Thermal habitat segregation among morphotypes of whitefish (Coregonus lavaretus: Salmonidae) and invasive vendace (C. albula): A mechanism for co-existence? Freshw. Biol. 60, 2337–2348. doi:10.1111/fwb.12660
Kelly, B., Smokorowski, K. E., and Power, M. (2017). Downstream effects of hydroelectric dam operation on thermal habitat use by Brook Trout (Salvelinus fontinalis) and Slimy Sculpin (Cottus cognatus). Ecol. Freshw. Fish. 26, 552–562. doi:10.1111/eff.12299
Kinouchi, T., Yagi, H., and Miyamoto, M. (2007). Increase in stream temperature related to anthropogenic heat input from urban wastewater. J. Hydrol. X. 335, 78–88. doi:10.1016/j.jhydrol.2006.11.002
Kishe-Machumu, M. A., van Rijssel, J. C., Poste, A., Hecky, R. E., and Witte, F. (2017). Stable isotope evidence from formalin–ethanol-preserved specimens indicates dietary shifts and increasing diet overlap in Lake Victoria cichlids. Hydrobiologia 791, 155–173. doi:10.1007/s10750-016-2925-1
Korman, J., Yard, M. D., Dzul, M. C., Yackulic, C. B., Dodrill, M. J., Deemer, B. R., et al. (2021). Changes in prey, turbidity, and competition reduce somatic growth and cause the collapse of a fish population. Ecol. Monogr. 91, 1–20. doi:10.1002/ecm.1427
Kristensen, P. B., Riis, T., Dylmer, H. E., Kristensen, E. A., Meerhoff, M., Olesen, B., et al. (2016). Baseline identification in stable-isotope studies of temperate lotic systems and implications for calculated trophic positions. Freshw. Sci. 35, 909–921. doi:10.1086/687284
Kupilas, B., Burdon, F. J., Thaulow, J., Håll, J., Mutinova, P. T., Forio, M. A. E., et al. (2021). Forested riparian zones provide important habitat for fish in urban streams. Water 13, 877. doi:10.3390/w13060877
Layman, C. A., Arrington, D. A., Montaña, C. G., Post, D. M., Ecology, S., Jan, N., et al. (2007a). Can stable isotope ratios provide for community-wide measures of trophic structure. Ecology 88, 42–48. doi:10.1890/0012-9658(2007)88[42:csirpf]2.0.co;2
Layman, C. A., Quattrochi, J. P., Peyer, C. M., and Allgeier, J. E. (2007b). Niche width collapse in a resilient top predator following ecosystem fragmentation. Ecol. Lett. 10, 937–944. doi:10.1111/j.1461-0248.2007.01087.x
Layman, C. A. (2007). What can stable isotope ratios reveal about mangroves as fish habitat? Bull. Mar. Sci. 80, 513–527.
Le Feuvre, M. C., Dempster, T., Shelley, J. J., Davis, A. M., and Swearer, S. E. (2021). Range restriction leads to narrower ecological niches and greater extinction risk in Australian freshwater fish. Biodivers. Conserv. 30, 2955–2976. doi:10.1007/s10531-021-02229-0
Lisi, P. J., Childress, E. S., Gagne, R. B., Hain, E. F., Lamphere, B. A., Walter, R. P., et al. (2018). Overcoming urban stream syndrome: Trophic flexibility confers resilience in a Hawaiian stream fish. Freshw. Biol. 63, 492–502. doi:10.1111/fwb.13091
Loomer, H. A., Oakes, K. D., Schiff, S. L., Taylor, W. D., and Servos, M. R. (2014). Use of stable isotopes to trace municipal wastewater effluents into food webs within a highly developed river system. River Res. Appl. 31, 1093–1100. doi:10.1002/rra.2826
Mackintosh, T. J., Davis, J. A., and Thompson, R. M. (2017). The effects of urbanization on trophic relationships in constructed wetlands. Freshw. Sci. 36, 138–150. doi:10.1086/690674
Magnuson, J. J., Crowder, L. B., and Medvick, P. A. (1979). Temperature as an ecological resource. Am. Zool. 19, 331–343. doi:10.1093/icb/19.1.331
Malmqvist, B., and Rundle, S. (2002). Threats to the running water ecosystems of the world. Environ. Conserv. 29, 134–153. doi:10.1017/S0376892902000097
Martino, J. C., Doubleday, Z. A., Chung, M. T., and Gillanders, B. M. (2020). Experimental support towards a metabolic proxy in fish using otolith carbon isotopes. J. Exp. Biol. 223, jeb217091. doi:10.1242/jeb.217091
McCutchan, J. H., Lewis, W. M., Kendall, C., and Mcgrath, C. C. (2003). Variation in trophic shift for stable isotope ratios of carbon, nitrogen, and sulfur. Oikos 102, 378–390. doi:10.1034/j.1600-0706.2003.12098.x
McKinney, M. L. (1997). Extinction vulnerability and selectivity:combining ecological and paleontological views. Annu. Rev. Ecol. Syst. 28, 495–516. doi:10.1146/annurev.ecolsys.28.1.495
Montaña, C. G., and Schalk, C. M. (2018). Correlates of elemental-isotopic composition of stream fishes: The importance of land-use, species identity and body size. J. Fish. Biol. 92, 944–960. doi:10.1111/jfb.13554
Moore, J. W., and Semmens, B. X. (2008). Incorporating uncertainty and prior information into stable isotope mixing models. Ecol. Lett. 11, 470–480. doi:10.1111/j.1461-0248.2008.01163.x
Morrissey, C. A., Boldt, A., Mapstone, A., Newton, J., and Ormerod, S. J. (2013). Stable isotopes as indicators of wastewater effects on the macroinvertebrates of urban rivers. Hydrobiologia 700, 231–244. doi:10.1007/s10750-012-1233-7
Mumby, J. A., Larocque, S. M., Johnson, T. B., Stewart, T. J., Fitzsimons, J. D., Weidel, B. C., et al. (2018). Diet and trophic niche space and overlap of Lake Ontario salmonid species using stable isotopes and stomach contents. J. Gt. Lakes. Res. 44, 1383–1392. doi:10.1016/j.jglr.2018.08.009
Nelson, K. C., and Palmer, M. A. (2007). Stream temperature surges under urbanization and climate change: Data, models, and responses. J. Am. Water Resour. Assoc. 43, 440–452. doi:10.1111/j.1752-1688.2007.00034.x
Newsome, S. D., Martinez del Rio, C., Bearhop, S., and Phillips, D. L. (2007). A niche for isotopic ecology. Front. Ecol. Environ. 5, 429–436. doi:10.1890/1540-9295(2007)5[429:anfie]2.0.co;2
Northington, R. M., and Hershey, A. E. (2006). Effects of stream restoration and wastewater treatment plant effluent on fish communities in urban streams. Freshw. Biol. 51, 1959–1973. doi:10.1111/j.1365-2427.2006.01626.x
O’Gorman, E. J., Ólafsson, Ó. P., Demars, B. O. L., Friberg, N., Guðbergsson, G., Hannesdóttir, E. R., et al. (2016). Temperature effects on fish production across a natural thermal gradient. Glob. Chang. Biol. 22, 3206–3220. doi:10.1111/gcb.13233
Parnell, A. C., Inger, R., Bearhop, S., and Jackson, A. L. (2010). Source partitioning using stable isotopes: Coping with too much variation. PLoS One 5, e9672–5. doi:10.1371/journal.pone.0009672
Parnell, A. C., Phillips, D. L., Bearhop, S., Semmens, B. X., Ward, E. J., Moore, J. W., et al. (2013). Bayesian stable isotope mixing models. Environmetrics 24, 387–399. doi:10.1002/env.2221
Phillips, D. L., and Gregg, J. W. (2003). Source partitioning using stable isotopes: Coping with too many sources. Oecologia 136, 261–269. doi:10.1007/s00442-003-1218-3
Phillips, D. L., and Gregg, J. W. (2001). Uncertainty in source partitioning using stable isotopes. Oecologia 127, 171–179. doi:10.1007/s004420000578
Phillips, D. L., Inger, R., Bearhop, S., Jackson, A. L., Moore, J. W., Parnell, A. C., et al. (2014). Best practices for use of stable isotope mixing models in food-web studies. Can. J. Zool. 835, 823–835. doi:10.1139/cjz-2014-0127
Poff, N. L. R., Bledsoe, B. P., and Cuhaciyan, C. O. (2006). Hydrologic variation with land use across the contiguous United States: Geomorphic and ecological consequences for stream ecosystems. Geomorphology 79, 264–285. doi:10.1016/j.geomorph.2006.06.032
Post, D. M., Layman, C. A., Arrington, D. A., Takimoto, G., Quattrochi, J., and Montaña, C. G. (2007). Getting to the fat of the matter: Models, methods and assumptions for dealing with lipids in stable isotope analyses. Oecologia 152, 179–189. doi:10.1007/s00442-006-0630-x
Post, D. M. (2002). Using stable isotopes to estimate trophic position: Models, methods, and assumptions. Ecology 83, 703–718. doi:10.1890/0012-9658(2002)083[0703:usitet]2.0.co;2
Power, G., Brown, R. S., and Imhof, J. G. (1999). Groundwater and fish - insights from northern north America. Hydrol. Process. 13, 401–422. doi:10.1002/(sici)1099-1085(19990228)13:3<401::aid-hyp746>3.0.co;2-a
Power, M. (2002). “Assessing fish population responses to stress,” in Biological indicators of aquatic ecosystem stress. Editor S. M. Adams (Bethesda, Maryland: American Fisheries Society), 379–429.
Power, M., O’Connell, M. F., and Dempson, B. (2012). Determining the consistency of thermal habitat segregation within and among Arctic charr morphotypes in Gander Lake, Newfoundland. Ecol. Freshw. Fish. 21, 245–254. doi:10.1111/j.1600-0633.2011.00543.x
Quezada-Romegialli, C., Jackson, A. L., Hayden, B., Kahilainen, K. K., Lopes, C., and Harrod, C. (2018). tRophicPosition, an R package for the Bayesian estimation of trophic position from consumer stable isotope ratios. Methods Ecol. Evol. 9, 1592–1599. doi:10.1111/2041-210X.13009
Robinson, B. G., Franke, A., and Derocher, A. E. (2018). Stable isotope mixing models fail to estimate the diet of an avian predator. Auk 135, 60–70. doi:10.1642/AUK-17-143.1
Santana Marques, P., Resende Manna, L., Clara Frauendorf, T., Zandonà, E., Mazzoni, R., and El-Sabaawi, R. (2020). Urbanization can increase the invasive potential of alien species. J. Anim. Ecol. 89, 2345–2355. doi:10.1111/1365-2656.13293
Scheele, B. C., Foster, C. N., Banks, S. C., and Lindenmayer, D. B. (2017). Niche contractions in declining species: Mechanisms and consequences. Trends Ecol. Evol. 32, 346–355. doi:10.1016/j.tree.2017.02.013
Schwarcz, H. P., Gao, Y., Campana, S. E., Browne, D., Knyf, M., and Brand, U. (1998). Stable carbon isotope variations in otoliths of Atlantic cod (Gadus morhua). Can. J. Fish. Aquat. Sci. 55, 1798–1806. doi:10.1139/f98-053
Shelton, A. O., and Mangel, M. (2011). Fluctuations of fish populations and the magnifying effects of fishing. Proc. Natl. Acad. Sci. U. S. A. 108, 7075–7080. doi:10.1073/pnas.1100334108
Singer, G. A., and Battin, T. J. (2007). Anthropogenic subsidies alter stream consumer-resource stoichiometry, biodiversity, and food chains. Ecol. Appl. 17, 376–389. doi:10.1890/06-0229
Sinnatamby, R. N., Brian Dempson, J., Reist, J. D., and Power, M. (2015). Latitudinal variation in growth and otolith-inferred field metabolic rates of Canadian young-of-the-year Arctic charr. Ecol. Freshw. Fish. 24, 478–488. doi:10.1111/eff.12166
Skinner, M. M., Martin, A. A., and Moore, B. C. (2016). Is lipid correction necessary in the stable isotope analysis of fish tissues? Rapid Commun. Mass Spectrom. 30, 881–889. doi:10.1002/rcm.7480
Smith, A., Smokorowski, K., Marty, J., and Power, M. (2016). Stable isotope characterization of Rainy River, Ontario, lake sturgeon diet and trophic position. J. Gt. Lakes. Res. 42, 440–447. doi:10.1016/j.jglr.2015.12.016
Smucker, N. J., Kuhn, A., Cruz-Quinones, C. J., Serbst, J. R., and Lake, J. L. (2018). Stable isotopes of algae and macroinvertebrates in streams respond to watershed urbanization, inform management goals, and indicate food web relationships. Ecol. Indic. 90, 295–304. doi:10.1016/j.ecolind.2018.03.024
Solomon, C. T., Weber, P. K., Cech, , Ingram, B. L., Conrad, M. E., Machavaram, M. V., et al. (2006). Experimental determination of the sources of otolith carbon and associated isotopic fractionation. Can. J. Fish. Aquat. Sci. 63, 79–89. doi:10.1139/f05-200
Spanjer, A. R., Moran, P. W., Larsen, K. A., Wetzel, L. A., Hansen, A. G., and Beauchamp, D. A. (2018). Juvenile coho salmon growth and health in streams across an urbanization gradient. Sci. Total Environ. 625, 1003–1012. doi:10.1016/j.scitotenv.2017.12.327
Steffy, L. Y., and Kilham, S. S. (2004). Elevated δ15N in stream biota in areas with septic tank systems in an urban watershed. Ecol. Appl. 14, 637–641. doi:10.1890/03-5148
Stock, B. C., Jackson, A. L., Ward, E. J., Parnell, A. C., Phillips, D. L., and Semmens, B. X. (2018). Analyzing mixing systems using a new generation of Bayesian tracer mixing models. PeerJ 6, e5096. doi:10.7717/peerj.5096
Storm-Suke, A., Dempson, J. B., Reist, J. D., and Power, M. (2007). A field-derived oxygen isotope fractionation equation for Salvelinus species. Rapid Commun. Mass Spectrom. 21, 4109–4116. doi:10.1002/rcm.3320
Surge, D., and Walker, K. J. (2005). Oxygen isotope composition of modern and archaeological otoliths from the estuarine hardhead catfish (Ariopsis felis) and their potential to record low-latitude climate change. Palaeogeogr. Palaeoclimatol. Palaeoecol. 228, 179–191. doi:10.1016/j.palaeo.2005.03.051
Swanson, H. K., Lysy, M., Power, M., Stasko, A. D., Johnson, J. D., and Reist, J. D. (2015). A new probabilistic method for quantifying n -dimensional ecological niches and niche overlap. Ecology 96, 318–324. doi:10.1890/14-0235.1
Tickner, D., Opperman, J. J., Abell, R., Acreman, M., Arthington, A. H., Bunn, S. E., et al. (2020). Bending the curve of global freshwater biodiversity loss: An emergency recovery plan. Bioscience 70, 330–342. doi:10.1093/biosci/biaa002
Timm, A., Ouellet, V., and Daniels, M. (2021). Riparian land cover, water temperature variability, and thermal stress for aquatic species in urban streams. Water 13, 2732. doi:10.3390/w13192732
Treberg, J. R., Killen, S. S., MacCormack, T. J., Lamarre, S. G., and Enders, E. C. (2016). Estimates of metabolic rate and major constituents of metabolic demand in fishes under field conditions: Methods, proxies, and new perspectives. Comp. Biochem. Physiol. Part A Mol. Integr. Physiol. 202, 10–22. doi:10.1016/j.cbpa.2016.04.022
Tucker, J., Sheats, N., Giblin, A. E., Hopkinson, C. S., and Montoya, J. P. (1999). Using stable isotopes to trace sewage-derived material through Boston Harbor and Massachusetts Bay. Mar. Environ. Res. 48, 353–375. doi:10.1016/S0141-1136(99)00069-0
Turner, T. F., Krabbenhoft, T. J., Collyer, M. L., Krabbenhoft, C. A., Edwards, M. S., and Sharp, Z. D. (2015). Retrospective stable isotope analysis reveals ecosystem responses to river regulation over the last century. Ecology 96, 3213–3226. doi:10.1890/14-1666.1
Ulseth, A. J., and Hershey, A. E. (2005). Natural abundances of stable isotopes trace anthropogenic N and C in an urban stream. J. North Am. Benthol. Soc. 24, 270–289. doi:10.1899/03-080.1
Vander Zanden, H. B., Bjorndal, K. A., Reich, K. J., and Bolten, A. B. (2010). Individual specialists in a generalist population: Results from a long-term stable isotope series. Biol. Lett. 6, 711–714. doi:10.1098/rsbl.2010.0124
Wang, L., Lyons, J., Kanehi, P., Bannerman, R., and Emmons, E. (2000). Watershed urbanization and changes in fish communities in southeastern Wisconsin streams. J. Am. Water Resour. Assoc. 36, 1173–1189. doi:10.1111/j.1752-1688.2000.tb05719.x
Willmes, M., Lewis, L. S., Davis, B. E., Loiselle, L., James, H. F., Denny, C., et al. (2019). Calibrating temperature reconstructions from fish otolith oxygen isotope analysis for California’s critically‐endangered Delta Smelt. Rapid Commun. Mass Spectrom. 33, 1207–1220. doi:10.1002/rcm.8464
Winemiller, K. O. (2005). Life history strategies, population regulation, and implications for fisheries management. Can. J. Fish. Aquat. Sci. 62, 872–885. doi:10.1139/f05-040
Woodland, R. J., Harris, L., Reilly, E., Fireman, A., Schott, E., and Heyes, A. (2022). Food web restructuring across an urban estuarine gradient. Ambio 51, 888–900. doi:10.1007/s13280-021-01610-1
Wurster, C. M., Patterson, W. P., Stewart, D. J., Bowlby, J. N., and Stewart, T. J. (2005). Thermal histories, stress, and metabolic rates of chinook salmon (Oncorhynchus tshawytscha) in Lake Ontario: Evidence from intra-otolith stable isotope analyses. Can. J. Fish. Aquat. Sci. 62, 700–713. doi:10.1139/f04-241
Keywords: anthropogenic, diet, ecology, freshwater fishes, habitat use, SIA, temperature use, urban impacts
Citation: Burbank J, Drake DAR and Power M (2022) Use of stable isotopes for assessing urbanization impacts on freshwater fishes. Front. Environ. Sci. 10:963693. doi: 10.3389/fenvs.2022.963693
Received: 07 June 2022; Accepted: 02 September 2022;
Published: 26 September 2022.
Edited by:
Juan Jose Rosso, Consejo Nacional de Investigaciones Científicas y Técnicas (CONICET), ArgentinaReviewed by:
Maria De Los Angeles González Sagrario, CONICET Mar del Plata, ArgentinaCarmen Montana, Stephen F. Austin State University, United States
Copyright © 2022 Michael Power and His Majesty the King in Right of Canada, as represented by the Ministry of Fisheries and Oceans Canada for the contribution of Jacob Burbank and D. Andrew R. Drake. This is an open-access article distributed under the terms of the Creative Commons Attribution License (CC BY). The use, distribution or reproduction in other forums is permitted, provided the original author(s) and the copyright owner(s) are credited and that the original publication in this journal is cited, in accordance with accepted academic practice. No use, distribution or reproduction is permitted which does not comply with these terms.
*Correspondence: Jacob Burbank, jdburban@uwaterloo.ca