- 1Key Laboratory of Vegetation Ecology, Ministry of Education, Jilin Songnen Grassland Ecosystem National Observation and Research Station, Northeast Normal University, Changchun, China
- 2Agriculture College of Yanbian University, Yanji, China
- 3State Environmental Protection Key Laboratory of Wetland Ecology and Vegetation Restoration, Northeast Normal University, Changchun, China
- 4Heilongjiang Provincial Key Laboratory of Ecological Restoration and Resource Utilization for Cold Region, Heilongjiang University, Harbin, China
- 5School of Ecology and Environmental Science, Yunnan University, Kunming, China
Mid-latitude areas such as Northeast China are predicted to have warmer temperature according to IPCC sixth which may influence the duration of the snowmelt season. What remains unknown, however, is how annual snowmelt can influence the biodiversity of macroinvertebrate communities, especially in headwater streams in Northeast China. Here, we investigated the temporal changes (before, during, and after the snowmelt period) of macroinvertebrate communities and stream physicochemical characteristics in five headwater streams in Northeast China. We found that: (1) Macroinvertebrate taxa richness, density, Shannon diversity index, and functional diversity were lower in the melting period than in the other two periods, while biomass and percentage of dominant species were significantly higher in this period; (2) As to functional traits, “erosional”, “strong swimming ability”, “some attachment”, “streamlined shape” and “large size at maturity” traits of the macroinvertebrates taxa were significantly higher in the melting period than in the other two periods; and (3) Redundancy analysis (RDA) and multiple linear regression model found that temperature, turbidity, and velocity were key environmental factors affecting the functional traits of macroinvertebrates during the whole snowmelt process. This study could provide crucial data to support biodiversity conservation and ecosystem restoration of freshwater ecosystems in mid-to high-latitude and alpine areas.
Introduction
The Intergovernmental Panel on Climate Change sixth report states that global warming caused by carbon emissions from human activities is irreversible, with the survival of life on earth potentially facing severe challenges (Zhou and Zhai, 2021). It also predicted that mid-latitude areas such as Northeast China, will experience warmer temperatures, which may influence the duration of the snowmelt season, and thereby further affect river ecosystems. The annual melt of snow and ice, contributing to water supply and nutrient replenishment is of great significance for maintaining the balance of macroinvertebrate biodiversity in mid-to high-latitudes and high-altitude freshwater ecosystems (Palmer et al., 2010; Fodelianakis et al., 2021; Vione et al., 2021). During the snowmelt season, stream habitats are changed in various ways such as faster stream velocity, lower sediment stability, and higher organic matter content (enriching the food supply for aquatic organisms) (Boyero et al., 2011; Oliveira et al., 2020), The melted water gathering over a short period of time rapidly increases the water flow speed, which reduces the stability of the original stream substrates (Scotti et al., 2019). In addition, large amounts of litter and sand/silt are mixed in the melted water on both sides of the river bank, which increases the concentration of organic matter in the stream and changes the composition of the streambed substrate accordingly (Fu et al., 2016), all of which have potential impacts on aquatic organism communities.
However, most of the previous studies on this topic have focused on glacier-fed streams (Fell et al., 2017; Brown et al., 2018; Tolotti et al., 2020; Lencioni et al., 2021) while snowmelt streams (non-glacier-fed) have been relatively overlooked. Glacier-fed and snowmelt streams differ in the three following ways: (1) Differences in snowmelt duration. Glacier melting is a relatively continuous process (Brighenti et al., 2021). Giant glaciers continue to melt throughout non-freezing periods to replenish streams. Furthermore, as the atmospheric temperature continues to increase, glacier melting has a more significant impact on the water temperature, flow rate, substrate stability, and turbidity of headwater streams (Fureder and Niedrist, 2020). In contrast, the duration of non-glacial snowmelt is relatively short. After the ice and snow of the streambed and both sides of the stream are fully melted and enter into the stream, the process ends. Although the ice and snow melting duration in non-glacier-fed streams is relatively shorter than that in glacier-fed streams, it also has a significant impact on macroinvertebrates (Herbst and Cooper, 2010). (2) Differences in the ecological environments of headwater streams (Alemneh et al., 2017). The upstream surrounding environment of glacier-fed streams is covered with ice and snow all year round, and there is very little vegetation on both sides (Martyniuk et al., 2019), while non-glacier-fed streams are mostly located in mid-to high-latitude and alpine areas with riparian forest (Jacobsen et al., 2020). The low plants (mainly herbs) on the slopes during the melting period of ice and snow have not yet turned green, and they have less resistance to the water flow formed by the ice and snow melt (Giulivo et al., 2019). Water flow mixed with a large amount of litter quickly enters the stream, providing a large amount of organic matter and nutrients to headwater streams (Wang et al., 2019). (3) Differences in stream reaches. Research into the macroinvertebrates of glacier-fed streams, has mainly focused on headwater streams. Chironomids can grow faster and have higher individual biomass in glacier-fed headwater streams (Lods-Crozet et al., 2001; Niedrist et al., 2018; Schutz and Fureder, 2018). This may be due to fewer macroinvertebrates, low competition levels, and abundant food resources in glacial streams (Milner et al., 2001; Niedrist and Fureder, 2018). Sueyoshi et al. (2014) found that the abundance and diversity of macroinvertebrates in riffles decreased significantly during ice and snow melt (non-glacier-fed), but were hardly affected in the ‘backwater’, ‘slow’, and ‘side channels’ of floodplains (Sueyoshi et al., 2014). To our best knowledge, research to date has only focused on the middle and lower reaches of streams, with little known regarding non-glacier-fed headwater streams. It is unclear how snow and ice melt in headwater streams (non-glacier-fed) affects macroinvertebrate community structure.
Headwater streams are mainly distributed in nature reserves and experience little interference from human activities. There have healthy vegetation and natural conditions on both sides of the stream bank, which can objectively reflect the impact of melting ice and snow on the stream environment and ecosystem. We intended to reveal the exact biodiversity maintaining mechanisms of macroinvertebrate communities and how they are influenced by the major environmental factors (temperatures, flow discharge, etc.) in non-glacier-fed headwater streams of mid-to high-latitude and alpine areas and to provide new field data. Therefore, in this study, we measured the water environment of headwater streams and assessed the impact of the snow and ice melting process on macroinvertebrate colonization (specifically community structure and functional characteristics). By analyzing the relationship between headwater streams, and the structure and functional characteristics of the macroinvertebrate communities in the freezing period (FP), melting period (MP), and after the melting period (AM), we revealed how the ice and snow melting process affects the macroinvertebrate community. We hypothesized that: (1) During the ice and snow melting in the headwater streams, the macroinvertebrate taxa richness and density showed a trend of first decreasing and then increasing due to changes in water environmental factors, and was lowest in the MP; (2) The functional traits of the macroinvertebrate communities are closely related to the changes in environmental factors. Among them, macroinvertebrate taxa with “Strong swimming ability (Swim 3)", “Some attachment (Atch 2)" and “Large size at maturity (Size 3)" traits are significantly more abundant during the MP; and (3) During the ice and snow melting, temperature and velocity of the stream are key environmental factors affecting the community structure of macroinvertebrates.
In order to test these hypotheses, the water environment variables of a headwater stream in different periods of ice and snow melt were investigated, and the ecological effects of this process were evaluated in terms of the species richness, community structure, and functional diversity of macroinvertebrates. The findings can improve our understanding of how ice and snow melt impacts the community structure and functional diversity of macroinvertebrates in headwater streams and provide crucial data to support biodiversity conservation and ecosystem restoration of freshwater ecosystems in mid-to high-latitude and alpine areas.
Materials and methods
Study area
The experimental streams (42°19′41″-42°20′13″N, 126°28′41″-126°30′22″E, 760–776 m a.s.l.) were located in Longwan National Nature Reserve, Huinan County, Jilin Province, China. The area has a northern temperate continental monsoon climate with a mean annual precipitation of 704.2 mm, mean annual water temperature of 7°C, and a monthly water temperature range of 0.5°C in January to 15°C in July (Wang et al., 2019). The freezing and snowmelt periods in this area occur from late November to late March of the following year, and from early to late April, respectively.
In order to reduce the effects of differences in microhabitats caused by stream pooling and changes in the external environment, in this experiment, a total of five headwater streams were selected as the research sites and five test sections were set up for each stream (Figure 1). All sections were located in first-order tributaries, and the distance between them was less than 100 m. The streams were crystal clear, and the substrate comprised mainly pebbles. The average flow of the streams was 0.04 m3˙s−1, and the average velocity was 0.16 m˙s−1. The vegetation on both sides of the stream bank of each section was similar, and mainly including Quercus mongolica, Pinus densiflora, and Syringa oblata.
Field experiment
Macroinvertebrates were sampled by using Surber-net (mesh size: 50 μm; sampling area: 0.09 m2) on 25 January 2019 (FP, the stream was completely frozen and covered with snow), 26 March 2019 (MP, the snow and ice on the stream and the slopes on both sides began to melt, but some ice and snow had not yet completely melted), and 25 April 2019 (AM, the snow and ice on the stream and the hillsides on both sides were completely melted, and the spring flood season ended). Five sites (50–100 m apart from each other) that had similar microhabitats were selected at each stream, and three replicates of macroinvertebrates were sampled at each site. In the FP, the ice surface (the mean thickness was 24.6 ± 3.7 cm) was carefully cut with a chainsaw (to avoid excessive movement and disturbance of the substrate), and then samples were taken. The collected samples were placed in plastic zipper bags, fixed with 75% ethanol, and brought back to the laboratory for further processing. In the laboratory, the macroinvertebrates in the plastic zipper bags were picked out and transported into a 50 ml specimen bottle with 75% ethanol for further identification. The macroinvertebrates were identified to the lowest possible taxonomic level (usually genus) under a stereoscopic microscope (Olympus SZX7), counted and weighed with an electronic balance (accuracy 0.1 mg).
Stream physiochemical characteristics were measured before collecting macroinvertebrates to avoid any potential disturbances. Three cross-sections were selected at each sampling point, and firstly the stream wet width was measured at each cross-section. Then the stream wet width was divided into quarters, and water depth, velocity, temperature, pH, dissolved oxygen (DO), electrical conductivity (EC), and turbidity were measured in three quartering points (Wang et al., 2019). The mean value of all the data was taken as the corresponding value of each environmental variables for this cross-section. The stream wet width was measured with an electronic rangefinder (FLUKE-406E), the water depth was measured by a self-made sounding rod, and the flow velocity was measured by a portable velocity analyzer (Globalwater-FP211). Stream water temperature, pH, DO, EC, and turbidity were measured in-situ by using a multiparameter water quality meter (Hanna HI9829). In addition, three water samples were taken at each site to analyze ammonia nitrogen (NH3-N), total phosphorus (TP), and total nitrogen (TN), by using a UV spectrophotometer in the laboratory.
Data analysis
The average substrate score (MSUBSTD) was calculated based on the composition of the substrate obtained during sampling. It was divided into five types of substrates: boulder, cobble, pebble, gravel, and sand/silt according to the particle size of the substrate (Fu et al., 2016), then the percentage of each type of substrate was estimated and multiplied by the corresponding coefficient to obtain the MSUBSTD value (Fu et al., 2016; Wang et al., 2019). The specific calculation formula is as follows:
where TOTSUB indicates the sum of different substrate coverage percentages. BOLDCOBB, PEBBGRAV, SAND, and SILTCLAY indicate the percentage cover of boulder/cobble, pebble/gravel, sand, and silt/clay, respectively. A higher score indicates higher proportions of sand and silt, whereas a lower score indicates a higher proportion of large rocks and cobble.
Species richness, density, biomass, and percentage of dominant species were used to evaluate the community composition of macroinvertebrate communities during different periods of snowmelt. Among them, the dominant species were determined by the Berger-Parker dominance index (Y) (Zhang et al., 2019). The specific calculation method is as follows:
where N is the habitat density of all macroinvertebrates in each station; ni is the habitat density of species i; fi is the frequency of occurrence of species i at each station. When Y ≥ 0.02, it was identified as the dominant species.
Shannon diversity index (H′), Margalef diversity index (dM), Pielou evenness index (J), and Simpson diversity index (D) were used to evaluate the diversity of macroinvertebrates in each period of ice and snow melt. All the macroinvertebrates were classified into four groups of functional traits: life history (voltinism), ecology (rheophily, thermal preference, habits, and trophic habits), mobility (occurrence in drift, swimming ability), and morphology (attachment, shape, size at maturity) (Poff et al., 2006; Wang et al., 2019). The classification standards of ten functional trait indicators in four functional traits were listed in Supplementary Table S1. The abundance and proportion of macroinvertebrates of each functional index were calculated. Regarding the functional diversity of macroinvertebrates, we calculated functional richness (FRic), functional evenness (FEve), functional divergence (FDiv), functional dispersion (FDis), and Rao’s quadratic entropy (RQE) using the FD package of the R version 4.0.5 software. Additionally, we calculated functional redundancy (FR) (Wang et al., 2019), the specific calculation method is as follows:
where FR is functional redundancy; FRic is functional richness; H′ is the Shannon diversity index.
One-way ANOVA was used to analyze the differences in stream environmental factors and macroinvertebrate communities with sampling date as a fixed factor. Tukey’s post hoc test was performed after one-way ANOVA was performed on datasets with normal distribution and homogeneity of variance. When the data set did not have normality (Shapiro-Wilk test) and homogeneity of variance (Levene’s test), the data were log10 (x+1) transformed, and the nonparametric Kruskal–Wallis test was used to evaluate the difference between means. The one-way ANOVA was used to compare stream conditions during freezing, melting, and after the melting periods. To test the relationship between functional trait structures and environmental factors, a constrained ordination method was employed. Detrended Correspondence Analysis (DCA) of the dataset using CANOCO for windows version 5.0 software was used to determine the appropriate model type for direct gradient analysis (Braak and Verdonschot, 1995). When the gradient lengths of DCA were less than 3, linear model RDA was used to further analyze the data. The 999 permutations of the Monte Carlo randomization test in RDA were used to screen the key environmental factors for the three periods of ice and snow melting (p < 0.05). DCA and RDA of the analyses were conducted with log10 (x+1) transformed abundance data (Fu et al., 2016; Wang et al., 2019).
A multiple linear regression model was used for the analysis of the correlation between environmental factors and community structure characteristics of macroinvertebrates, including species richness, density, biomass, percentage of dominant species, H′, D, dM, J, FRic, FEve, FDiv, FDis, RQE, and FR. Stepwise regression with forwarding selection was used to determine key factors driving the structural characteristics of macroinvertebrate communities during the melting of ice and snow. All analyses of variance and multiple linear regression models were performed with SPSS version 22.0 software (SPSS Inc., Chicago, IL, United States). Drawing graphics with Origin version 2019b software (OriginLab Corporation, Northampton, MA, United States).
Results
Stream physiochemical characteristics
All measured stream physiochemical characteristics showed temporal changes except for TN and NH3-N (Figure 2). Stream wet width, water depth, flow velocity, DO, MSUBSTD, turbidity, and TP were higher during MP than in the other two periods. Water temperature and pH shared the same trend: the two indices were the lowest in the FP and highest in the AM. In contrast, EC was higher in the FP than in the other two periods.
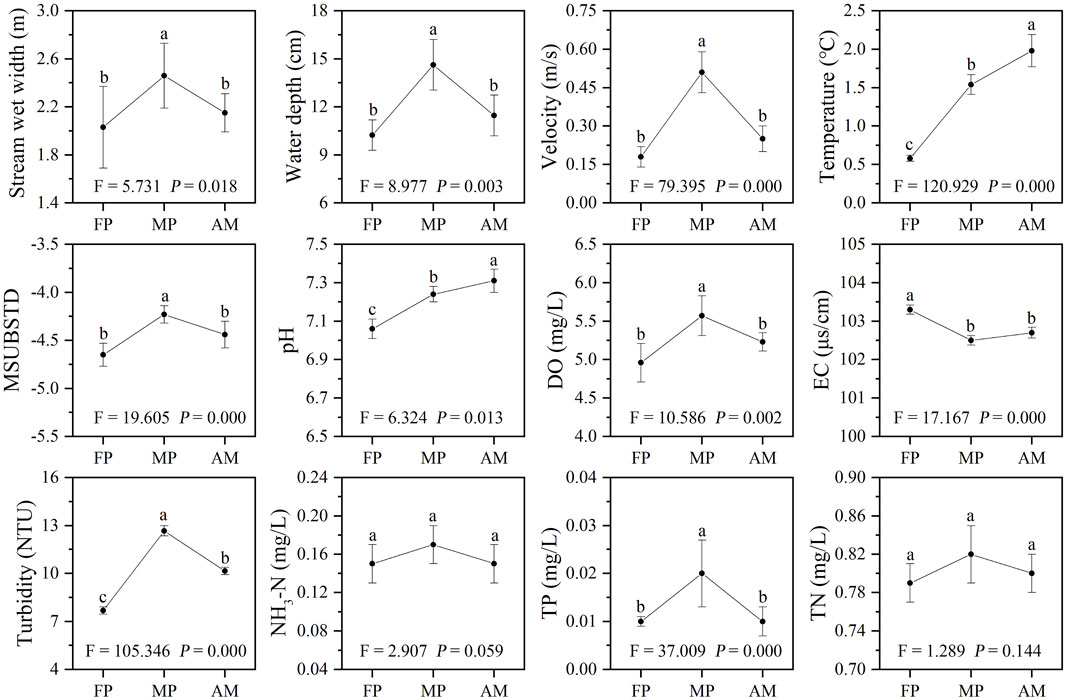
FIGURE 2. Temporal changes (FP, freezing period; MP, melting period; AM, after the melting period) of stream physicochemical characteristics. Data were shown as mean ± SE. Lowercase letters above each data point denote significant differences after one-way ANOVA followed by post hoc Tukey (parameters with the same letter are not significantly different between studies). Different lowercase letters indicate significant differences in invertebrate community structure caused by different periods.
Macroinvertebrates
We found a total of 9,655 macroinvertebrates, including 41 taxa and 13 families. 32 taxa in the FP, 25 taxa in the MP, and 41 taxa in the AM (Supplementary Table S2). The main taxonomic groups were Choroperlidae, Perlodidae, Ephemerellidae, Heptageniidae, Chironomidae, and Nemouridae. All measured macroinvertebrate indices differed among the three periods (Figure 3). Species richness, density, H′, and dM were the highest in the AM, medium in the FP, and lowest in the MP (p < 0.05, Figures 3A,B,E,F). The biomass and percentage of dominant species were higher in the MP than in the other two periods (p < 0.05, Figures 3C,D). J was highest in the AM (0.81 ± 0.04); it was 14.77 and 13.06% higher than that in the FP and MP, respectively (p < 0.05, Figure 3G). D was higher in the AM and FP than in the MP (p < 0.05, Figure 3H).
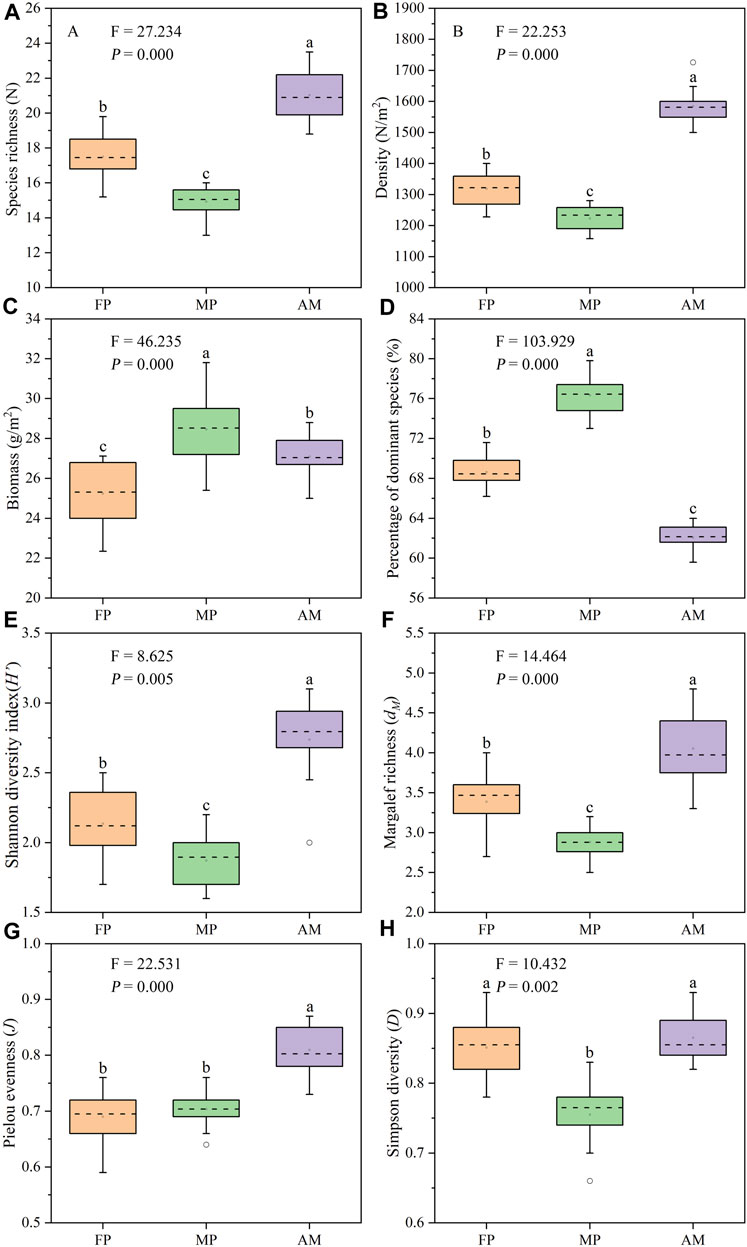
FIGURE 3. Temporal (FP, freezing period; MP, melting period; AM, after the melting period) changes of macroinvertebrate species richness (A), density (B), biomass (C), and proportion of dominant species (D) of stream macroinvertebrate species in three periods of snow melting, Shannon diversity index (E), Margalef diversity (F), Pielou evenness (G), and Simpson diversity (H). Box plots indicated interquartile ranges (box part), 25th and 75th percentiles (lower and upper error bars), and mean values (dash lines in the box). Different lowercase letters indicate significant differences in invertebrate community structure caused by different periods.
Functional traits and functional diversity indices
Regarding life history traits, the probability of " Bi-or multivoltine (Volt 3)" was higher in the AM than in the other two periods (p < 0.05, Figure 4A). From the perspective of ecological traits, the “erosional (Rheo 3)" macroinvertebrate characteristics were higher in the MP than in the other two periods. The proportion of taxa with the characteristics of “cool/warm eurythermal (Ther 2)” and “sprawl (Habi 3)” were higher in the AM than in the other two periods, while the proportion of “predators (Trop 4)” was significantly lower in the AM than in the other two periods (p < 0.05, Figures 4B–E). From the perspective of mobility traits, the proportion of macroinvertebrate taxa of “Rare occurrence in drift (Drft 1)" was higher in the AM than in the other two periods (p < 0.05, Figure 4F). The proportion of macroinvertebrate taxa with a “Strong swimming ability (swim 3)" trait was higher in the MP than in the other two periods (p < 0.05, Figure 4G). Regarding morphology traits, the proportion of macroinvertebrate taxa with “Some attachment (Atch 2)", “Streamlined shape (Shpe 1)", and “Large size at maturity (Size 3)" traits in the MP were significantly higher than in the AM and FP (p < 0.05, Figures 4H–J). The proportion of taxa of “Small size at maturity (Size 1)" traits were higher in the AM than in the other two periods (p < 0.05, Figure 4J).
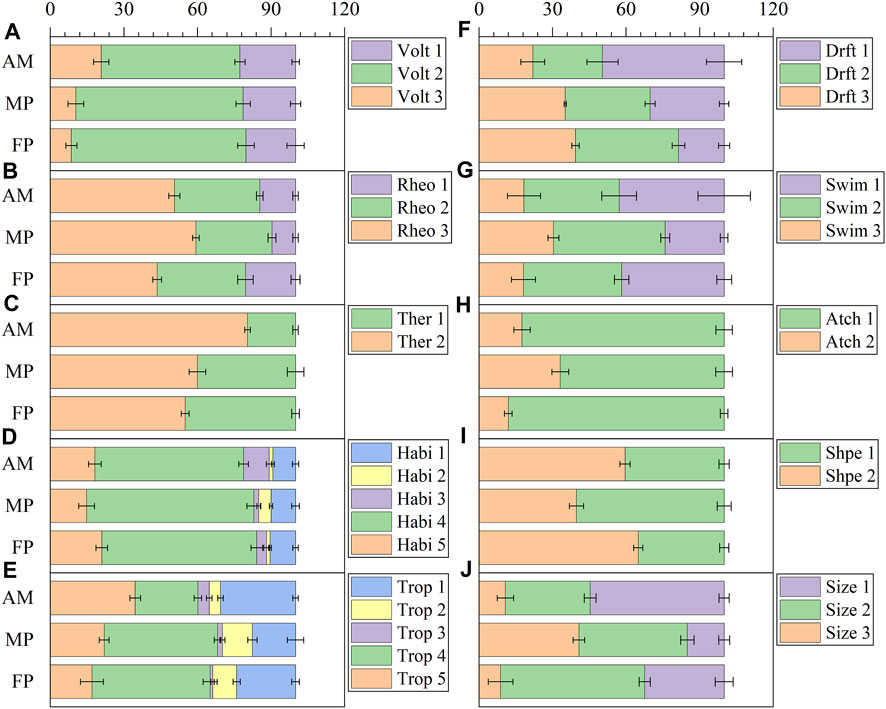
FIGURE 4. Average percentage of functional traits of macroinvertebrates during three periods of snow melt (FP, freezing period; MP, melting period; AM, after the melting period): voltinism (A), rheophily (B), thermal preference (C), habit (D), trophic habit (E), occurrence in drift (F), swimming ability (G), attachment (H), shape (I) and size at maturity (J). Different lowercase letters indicate significant differences in invertebrate community structure caused by different periods. Data are shown as mean ± SE.
The structural and functional diversity of macroinvertebrates declined and increased significantly in the MP and in the AM, respectively (Figure 5). Among them, Fric, Fdis, and RQE were the highest in the AM, second highest in the FP, and lowest in the MP (FRic, 0.11; FDis, 1.10; RQE, 4.30; all p < 0.05, Figures 5A,D,E). The FEve and FDiv were the highest during the FP (FEve, 0.63; FDiv, 0.59), and the lowest in the MP (FEve, 0.44; FDiv, 0.39; all p < 0.05, Figures 5B,C). Although FR varied among the three periods, the difference was not significant (Figure 5F).
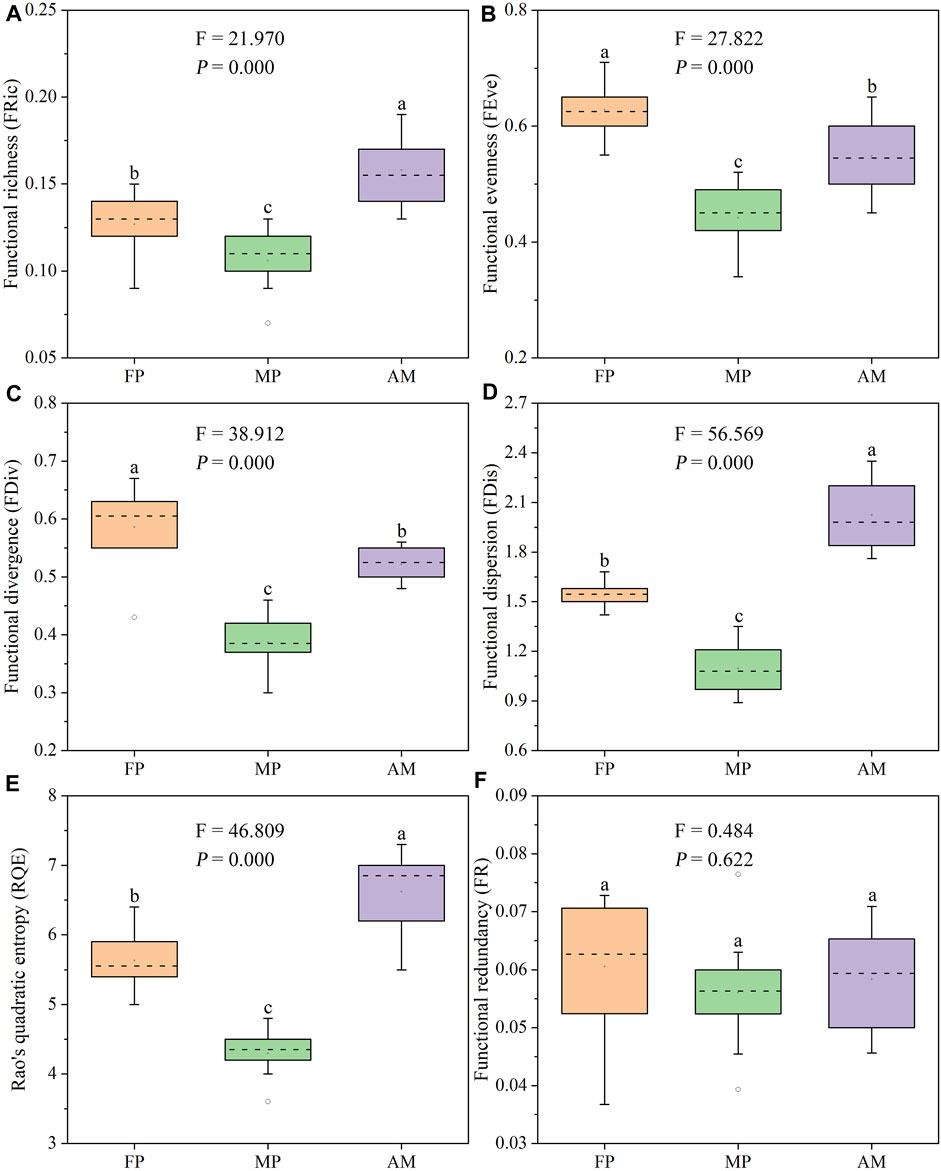
FIGURE 5. Differences in functional richness (A), functional evenness (B), functional divergence (C), functional dispersion (D), Rao’s quadratic entropy (E), and functional redundancy (F) of stream macroinvertebrates during three periods of snow melting. FP, freezing period; MP, melting period; AM, after the melting period. Different lowercase letters indicate significant differences in invertebrate community structure caused by different periods.
Relationship between environmental factors and macroinvertebrate communities
RDA ordering explained 84.0% of the variation in the functional shape composition of macroinvertebrates (Table 1). The relationship between the functional composition of macroinvertebrates and environmental factors during the freezing and melting of ice and snow in headwater streams is shown in Figure 6. The MP and AM sites were distributed along with the positive and negative directions of the first axis, respectively, which showed distinct habitat characteristics between the two periods. FP sites were mainly distributed on both sides of the positive direction of the second axis, reflecting the characteristics of low water temperature, low flow velocity and clean water of the headwater stream during the FP. According to the Monte Carlo permutation test results, three environmental factors (temperature, F = 30, p = 0.002; flow velocity, F = 18, p = 0.009; turbidity, F = 5.8, p = 0.014) were identified as the main factors explaining the variation in macroinvertebrate functional composition in the three periods of freezing and melting ice and snow.
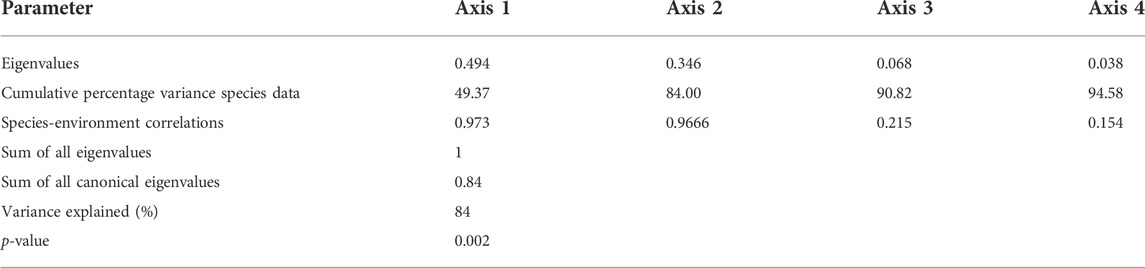
TABLE 1. Summary of the statistical characteristics of the four axes of the RDA analysis for the total data, FP data, MP data, and AM data.
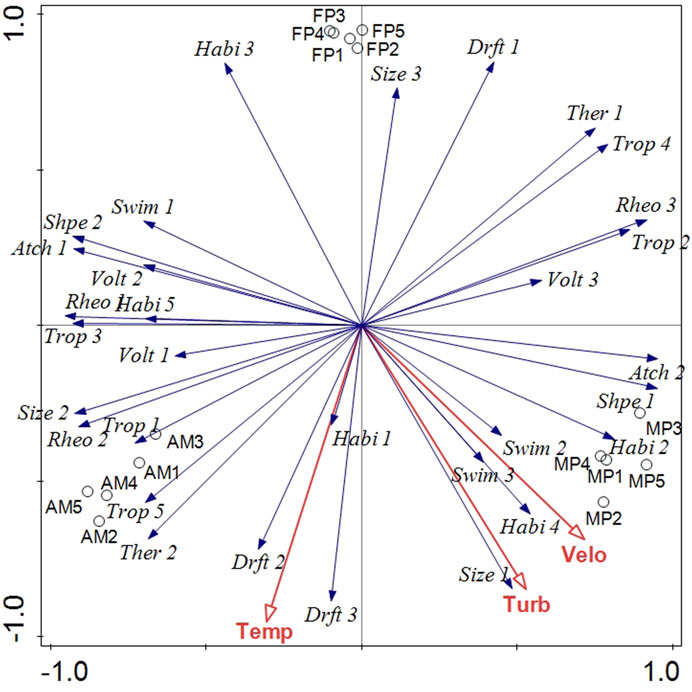
FIGURE 6. Redundancy analysis (RDA) ordination biplots of functional traits (blue arrows), sampling sites (black circles), and forward-selected environmental factors (red arrows) for three periods of melting ice and snow. The summary of ordination results is presented in Table 1 and the codes for each functional trait category are shown in Supplementary Table S1. Temp, Temperature; Turb, Turbidity; Velo, Velocity. FP, freezing period; MP, melting period; AM, after the melting period.
A multiple linear regression analysis identified the key environmental factors affecting stream macroinvertebrate community characteristics during the melting of ice and snow (Table 2). Except for biomass, D, and FDiv, there was a significant positive between temperature and other macroinvertebrate community characteristics. Turbidity had a significant positive predictive effect with biology and percentage of dominant species, but a significant negative predictive effect with other macroinvertebrate community characteristics in the model. Biomass, percentage of dominant species, D, FRic, FDiv, and RQE were positively affected by velocity. Biomass and H′ were positively affected by NH3-N, H′, J, and FDiv were negatively affected by TP. Thus, temperature, turbidity, velocity, NH3-N, and TP were the main environmental factors affecting the macroinvertebrate community during the melting of ice and snow.
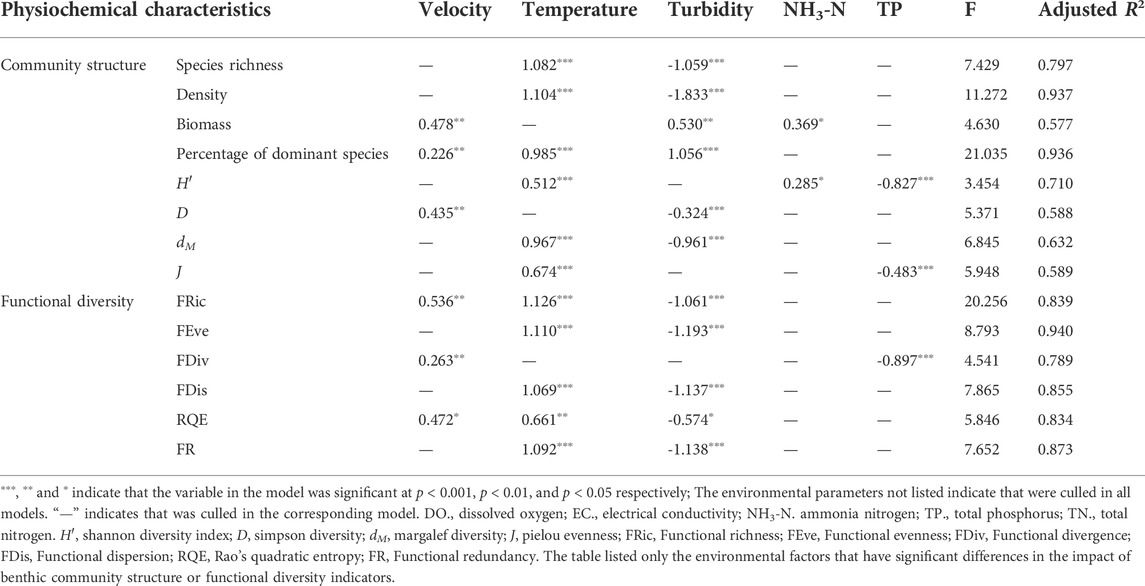
TABLE 2. Multiple linear regression analysis of macroinvertebrate community parameters and environmental factors (n = 75).
Discussion
Changing environmental factors in headwater streams during ice and snow melt
During the ice and snow melting period, the flow velocity of the headwater stream increased, consistent with the findings of the previous study (Khamis et al., 2015). This can be attributed to the rapid increase in the amount of water in the stream channel due to the melting of ice and snow on both sides of the stream channel and bank as the temperature increased (Kuhn et al., 2011; Khamis et al., 2015). In addition, the stream water turbidity also showed an increasing trend in the MP. Previous studies have shown that the status of food webs in headwater streams is closely related to the quality and quantity of organic matter transported into streams from surrounding terrestrial ecosystems (Hagen et al., 2010; Ledesma et al., 2018). This is because a large amount of litter residue and sediment on both sides quickly enters the stream channel during ice and snow melt (Brighenti et al., 2019). Although this process increases the turbidity of stream water, it also simultaneously transports a large amount of organic matter into the stream (Brighenti et al., 2019; Sargac et al., 2021). Climate change in the surrounding environment can affect the environmental characteristics of stream ecosystems (Brown et al., 2018; Brighenti et al., 2019), which has a significant impact on stream hydrology and water quality in particular (Lencioni et al., 2021).
Effects of snow and ice melt on macroinvertebrate community structure
Compared to the FP, the species richness, density, Hʹ, and dM were lower in the MP, but higher in the AM. This supports our first hypothesis. Previous studies on the effects of ice and snow melt on macroinvertebrate community structure in headwater streams have mainly focused on glacier melting (Brown et al., 2018; Tolotti et al., 2020; Brighenti et al., 2021; Fodelianakis et al., 2021). Melting glaciers can affect the stability of stream substrates, reducing the density and abundance of macroinvertebrates (Milner et al., 2017). However, when a headwater stream is rich in substrate structure, the probability of organic debris as a result of the ice and snow melt on both sides of the stream will be greatly enhanced in the stream channel, which is conducive to the colonization and increased abundance of macroinvertebrates (Brighenti et al., 2021). Our study also derived a similar conclusion. The melting of ice and snow in the headwater stream transports a large amount of organic matter such as litter into the stream (Rosi-Marshall et al., 2016). However, due to the low temperature in winter, the survival of macroinvertebrates is under pressure, with no positive organism responses (Herbst and Cooper, 2010). During ice and snow melt, the water flow is faster (Cauvy-Fraunie et al., 2016), at this time, the ability of smaller macroinvertebrates or those with weak attachment ability to resist drastic changes in the external environment is generally reduced, resulting in a lower abundance and diversity of macroinvertebrates in the MP compared with the FP (Milner et al., 2017). However, with the gradual increase in water temperature in the AM, the eggs of some macroinvertebrates turn into larvae (Füreder et al., 2005), and the organic matter entering the stream channel provides them with the necessary food (Rosi-Marshall et al., 2016). Thus, the species richness, density, and community diversity index of macroinvertebrates all increase significantly within a few weeks in the AM (Suren and Jowett, 2006). Macroinvertebrate diversity (H′, dM, and D) also showed a similar trend in the present study. However, although the species and density of macroinvertebrates decreased during the MP, the biomass and abundance of dominant species increased significantly, as hypothesized. Smaller or less resistant macroinvertebrates may not have been able to survive in the original substrate when the stream flow suddenly increases, while larger or swimmer taxa increased significantly. Since the key factor determining community biomass is body size rather than density (Brown et al., 2004), the biomass of macroinvertebrates and the number of dominant species in the MP were significantly higher than those in the other two periods. This also indicates that when a headwater stream is affected by the melting of ice and snow, the diversity of macroinvertebrate species decreases and a homogenization trend emerges (Piano et al., 2020; Prokin and Seleznev, 2021).
Effects of snow and ice melt on functional characteristics
The species composition of macroinvertebrate communities is closely related to individual species’ physiological, behavioral, and biological characteristics of predation strategies (Maclean and Beissinger, 2017). In our study, macroinvertebrate taxa with “Univoltine (Vlot 2)”, “Cool/warm eurythermal (Ther 2)”, “Cling (Habi 4)” and “Some attachment (Atch 2)” traits during the MP were dominant, reflecting the main functional traits of macroinvertebrates pre and post ice and snow melt in the headwater streams of Changbai Mountain (Wang et al., 2019). During the MP, although the density of macroinvertebrates decreased, the proportion of macroinvertebrate taxa with “Strong swimming ability (Swim 3)”, “Some attachment (Atch 2)”, “Streamlined shape (Shpe 1)”, and “Large size at maturity (Size 3)” traits increased. This supports our second hypothesis and indicates the survival strategies adopted by macroinvertebrates to adapt to environmental changes. During this period, the water flow was faster and more suitable for the survival of macroinvertebrates with strong swimming ability. At the same time, the macroinvertebrates with good attachment ability, streamlined shape, and large body length also showed good adaptability to changes in water flow velocity (Poff et al., 2006). However, the FRic, FEve, FDiv, Fdis, and RQE in this period were significantly reduced, which indicated that the functional traits of macroinvertebrates in headwater streams during the MP were greatly affected by environmental changes. The main reason for this was that the macroinvertebrates had just experienced low temperature stress during the FP, and were also facing the pressure of spring floods. The groups and individuals with weak resistance could not continue to survive in the original substrate. The increased proportion of macroinvertebrates with “Bi-or multivoltine (Volt 3)”, “Cool/warm eurythermal (Ther 2)” and “Small size at maturity (Size 1)” traits in the AM indicated that, the gradual increase in water temperature, the thermophilic macroinvertebrate eggs began to hatch (Füreder et al., 2005). In addition, macroinvertebrate taxa with the “Shredders (Trop 5)” traits also increased significantly due to the input of organic matters into streams (Wang et al., 2018). The rapid rebound in FRic, FDis, and RQE during this period reflected the quick response of macroinvertebrates to environmental changes and the gradual improvement in functional diversity under increasing temperature and food resources.
Environmental drivers of macroinvertebrate community characteristics
Our findings reveal that the key environmental drivers of changes in macroinvertebrate communities during the melting of ice and snow in headwater streams were water temperature, turbidity, and flow velocity. This supports our third hypothesis. Numerous studies have shown that macroinvertebrates adopt different survival strategies in order to adapt to environmental changes (Brown et al., 2018; Di Sabatino et al., 2018; Brighenti et al., 2021). For example, during the FP, due to the decrease in water temperature and scarcity of food (the stream surface is covered with ice and snow, and it is difficult for organic matter on both sides of the stream bank to enter the stream), macroinvertebrates will reduce the number of generations per year, overwinter in the form of eggs, or find suitable substrates to withstand severe cold. Therefore, the number of macroinvertebrates decreased significantly during the FP (Brown et al., 2018; Wang et al., 2019). Excessive flow velocity also poses a certain threat to the survival of macroinvertebrates. In particularly, when spring floods arrive, the lower water temperature and the drastic change in current speed make it difficult for most macroinvertebrates to continue to survive in the original substrate (Scotti et al., 2019). In addition, during the MP, the vegetation on both sides of the stream bank has not yet turned green, and the soil-fixing ability of the plants is relatively poor. The silt/sand enters the stream channel with the melting water of the ice and snow, which increases the turbidity and MSUBSTD of the water significantly, resulting in the destruction of the original habitat and food source of macroinvertebrates (Milner et al., 2017; Zou et al., 2019). The temperature, flow velocity, and turbidity of the stream water environment in the AM became more suitable for the survival of macroinvertebrates. Therefore, the species richness and functional diversity of macroinvertebrates were also significantly improved (Brighenti et al., 2021).
Conclusion
Based on traditional biodiversity research, and incorporating functional traits and functional diversity indicators, we analyzed the structure and functional characteristics of macroinvertebrate communities and their relationship with environmental factors during the melting of ice and snow in headwater streams. This is valuable for determining the potential ecological value of the ice and snow melt process, and can provide a scientific basis for further understanding the impact of ice and snow melt in headwater streams on stream biodiversity. The results showed that the melting of ice and snow in headwater streams had a significant impact on hydrology and water quality. Among them, water temperature, turbidity, and flow velocity were the key drivers affecting the structural and functional characteristics of macroinvertebrate communities. Although the species richness and functional diversity of macroinvertebrates decreased significantly during the MP, this period brought in additional organic substances to the headwater stream. These organic substances were a necessary food source for the survival and reproduction of macroinvertebrates, which facilitated an increase in macroinvertebrate diversity after the ice and snow melt. Therefore, from a long-term perspective, melting ice and snow is beneficial to macroinvertebrate communities in headwater streams. The experimental sites in this study were located in headwater streams in a high-latitude cold region, and these experimental results can be utilized as reference values for studying the impact of the headwater streams on macroinvertebrates in this region or in similar conditions during the melting of ice and snow (non-glacial melting).
Data availability statement
The original contributions presented in the study are included in the article/Supplementary Material further inquiries can be directed to the corresponding authors.
Author contributions
Conceptualization, ZZ, YG, and HY; methodology, data collection: YG, LR, LC, KL, and CL; writing—original draft preparation, YG and LR; writing—review and editing, ZZ, HX, and HY; funding acquisition, LR, ZZ, HX, and HY. All authors have read and agreed to the published version of the manuscript.
Funding
This study was supported by Science Foundation of Science and Technology of Education Department of Jilin Province (grant no. JJKH20200517KJ, JJKH20211293KJ); National Natural Science Foundation of China (grant no. 32071587, 32101310); The fellowship of China Postdoctoral Science Foundation (grant no. 2021M692728); China Scholarship Council (grant no. 202006625001); and Program of Introducing Talents of Discipline to Universities (grant no. B16011).
Acknowledgments
We thank International Science Editing (http://www.internationalscienceediting.com) for editing this manuscript.
Conflict of interest
The authors declare that the research was conducted in the absence of any commercial or financial relationships that could be construed as a potential conflict of interest.
Publisher’s note
All claims expressed in this article are solely those of the authors and do not necessarily represent those of their affiliated organizations, or those of the publisher, the editors and the reviewers. Any product that may be evaluated in this article, or claim that may be made by its manufacturer, is not guaranteed or endorsed by the publisher.
Supplementary Material
The Supplementary Material for this article can be found online at: https://www.frontiersin.org/articles/10.3389/fenvs.2022.960254/full#supplementary-material
References
Alemneh, T., Ambelu, A., Bahrndorff, S., Mereta, S. T., Pertoldi, C., and Zaitchik, B. F. (2017). Modeling the impact of highland settlements on ecological disturbance of streams in Choke Mountain Catchment: Macroinvertebrate assemblages and water quality. Ecol. Indic. 73, 452–459. doi:10.1016/j.ecolind.2016.10.019
Boyero, L., Pearson, R. G., Dudgeon, D., Graca, M. a. S., Gessner, M. O., Albarino, R. J., et al. (2011). Global distribution of a key trophic guild contrasts with common latitudinal diversity patterns. Ecology 92, 1839–1848. doi:10.1890/10-2244.1
Braak, T., and Verdonschot, P. (1995). Canonical correspondence analysis and related multivariate methods in aquatic ecology. Aquat. Sci. 57, 255–289. doi:10.1007/bf00877430
Brighenti, S., Tolotti, M., Bertoldi, W., Wharton, G., and Bruno, M. C. (2021). Rock glaciers and paraglacial features influence stream invertebrates in a deglaciating Alpine area. Freshw. Biol. 66, 535–548. doi:10.1111/fwb.13658
Brighenti, S., Tolotti, M., Bruno, M. C., Wharton, G., Pusch, M. T., and Bertoldi, W. (2019). Ecosystem shifts in alpine streams under glacier retreat and rock glacier thaw: A review. Sci. Total Environ. 675, 542–559. doi:10.1016/j.scitotenv.2019.04.221
Brown, J. H., Gillooly, J. F., Allen, A. P., Savage, V. M., and West, G. B. (2004). Toward a metabolic theory of ecology. Ecology 85, 1771–1789. doi:10.1890/03-9000
Brown, L. E., Khamis, K., Wilkes, M., Blaen, P., Brittain, J. E., Carrivick, J. L., et al. (2018). Functional diversity and community assembly of river invertebrates show globally consistent responses to decreasing glacier cover. Nat. Ecol. Evol. 2, 325–333. doi:10.1038/s41559-017-0426-x
Cauvy-Fraunie, S., Andino, P., Espinosa, R., Calvez, R., Jacobsen, D., and Dangles, O. (2016). Ecological responses to experimental glacier-runoff reduction in alpine rivers. Nat. Commun. 7, 12025. doi:10.1038/ncomms12025
Di Sabatino, A., Cristiano, G., Vignini, P., Miccoli, F. P., and Cicolani, B. (2018). A modification of the leaf-nets method for sampling benthic invertebrates in spring habitats. J. Limnol. 77, 82–87. doi:10.4081/jlimnol.2017.1675
Fell, S. C., Carrivick, J. L., and Brown, L. E. (2017). The multitrophic effects of climate change and glacier retreat in mountain rivers. Bioscience 67, 897–911. doi:10.1093/biosci/bix107
Fodelianakis, S., Washburne, A. D., Bourquin, M., Pramateftaki, P., Kohler, T. J., Styllas, M., et al. (2021). Microdiversity characterizes prevalent phylogenetic clades in the glacier-fed stream microbiome. ISME J. 16, 666–675. doi:10.1038/s41396-021-01106-6
Fu, L., Jiang, Y., Ding, J., Liu, Q., Peng, Q.-Z., and Kang, M.-Y. (2016). Impacts of land use and environmental factors on macroinvertebrate functional feeding groups in the Dongjiang River basin, southeast China. J. Freshw. Ecol. 31, 21–35. doi:10.1080/02705060.2015.1017847
Fureder, L., and Niedrist, G. H. (2020). Glacial stream ecology: Structural and functional assets. Water, 12, 376. doi:10.3390/w12020376
Füreder, L., Wallinger, M., and Burger, R. (2005). Longitudinal and seasonal pattern of insect emergence in alpine streams. Aquat. Ecol. 39, 67–78. doi:10.1007/s10452-004-2969-6
Giulivo, M., Stella, E., Capri, E., Esnaola, A., De Alda, M. L., Diaz-Cruz, S., et al. (2019). Assessing the effects of hydrological and chemical stressors on macroinvertebrate community in an Alpine river: The Adige River as a case study. River Res. Appl. 35, 78–87. doi:10.1002/rra.3367
Hagen, E. M., Mctammany, M. E., Webster, J. R., and Benfield, E. F. (2010). Shifts in allochthonous input and autochthonous production in streams along an agricultural land-use gradient. Hydrobiologia 655, 61–77. doi:10.1007/s10750-010-0404-7
Herbst, D. B., and Cooper, S. D. (2010). Before and after the deluge: Rain-on-snow flooding effects on aquatic invertebrate communities of small streams in the sierra Nevada, California. J. North Am. Benthol. Soc. 29, 1354–1366. doi:10.1899/09-185.1
Jacobsen, D., Wiberg-Larsen, P., Brodersen, K. P., Hansen, S. B., Lindegaard, C., Friberg, N., et al. (2020). Macroinvertebrate communities along the main stem and tributaries of a pre-alpine river: Composition responds to altitude, richness does not. Limnologica 84, 125816. doi:10.1016/j.limno.2020.125816
Khamis, K., Brown, L. E., Milner, A. M., and Hannah, D. M. (2015). Heat exchange processes and thermal dynamics of a glacier-fed alpine stream. Hydrol. Process. 29, 3306–3317. doi:10.1002/hyp.10433
Kuhn, J., Andino, P., Calvez, R., Espinosa, R., Hamerlik, L., Vie, S., et al. (2011). Spatial variability in macroinvertebrate assemblages along and among neighbouring equatorial glacier-fed streams. Freshw. Biol. 56, 2226–2244. doi:10.1111/j.1365-2427.2011.02648.x
Ledesma, J. L. J., Futter, M. N., Blackburn, M., Lidman, F., Grabs, T., Sponseller, R. A., et al. (2018). Towards an improved conceptualization of riparian zones in boreal forest headwaters. Ecosystems 21, 297–315. doi:10.1007/s10021-017-0149-5
Lencioni, V., Franceschini, A., Paoli, F., and Debiasi, D. (2021). Structural and functional changes in the macroinvertebrate community in Alpine stream networks fed by shrinking glaciers. Fundam. Appl. Limnol. 194, 237–258. doi:10.1127/fal/2020/1315
Lods-Crozet, B., Lencioni, V., Ólafsson, J. S., Snook, D. L., Rossaro, B., Brittain, J. E., et al. (2001). Chironomid (Diptera: Chironomidae) communities in six European glacier‐fed streams. Freshw. Biol. 46, 1791–1809. doi:10.1046/j.1365-2427.2001.00859.x
Maclean, S. A., and Beissinger, S. R. (2017). Species' traits as predictors of range shifts under contemporary climate change: A review and meta-analysis. Glob. Chang. Biol. 23, 4094–4105. doi:10.1111/gcb.13736
Martyniuk, N., Modenutti, B., and Balseiro, E. G. (2019). Seasonal variability in glacial influence affects macroinvertebrate assemblages in North-Andean Patagonian glacier-fed streams. Inland Waters 9, 522–533. doi:10.1080/20442041.2019.1624115
Milner, A. M., Brittain, J. E., Castella, E., and Petts, G. E. (2001). Trends of macroinvertebrate community structure in glacier‐fed rivers in relation to environmental conditions: A synthesis. Freshw. Biol. 46, 1833–1847. doi:10.1046/j.1365-2427.2001.00861.x
Milner, A. M., Khamis, K., Battin, T. J., Brittain, J. E., Barrand, N. E., Fuereder, L., et al. (2017). Glacier shrinkage driving global changes in downstream systems. Proc. Natl. Acad. Sci. U. S. A. 114, 9770–9778. doi:10.1073/pnas.1619807114
Niedrist, G. H., Cantonati, M., and Fureder, L. (2018). Environmental harshness mediates the quality of periphyton and chironomid body mass in alpine streams. Freshw. Sci. 37, 519–533. doi:10.1086/699480
Niedrist, G. H., and Fureder, L. (2018). When the going gets tough, the tough get going: The enigma of survival strategies in harsh glacial stream environments. Freshw. Biol. 63, 1260–1272. doi:10.1111/fwb.13131
Oliveira, P. C. D. R., Kraak, M. H. S., Pena-Ortiz, M., Van Der Geest, H. G., and Verdonschot, P. F. M. (2020). Responses of macroinvertebrate communities to land use specific sediment food and habitat characteristics in lowland streams. Sci. Total Environ. 703, 135060. doi:10.1016/j.scitotenv.2019.135060
Palmer, M. A., Menninger, H. L., and Bernhardt, E. (2010). River restoration, habitat heterogeneity and biodiversity: A failure of theory or practice? Freshw. Biol. 55, 205–222. doi:10.1111/j.1365-2427.2009.02372.x
Piano, E., Doretto, A., Mammola, S., Falasco, E., Fenoglio, S., and Bona, F. (2020). Taxonomic and functional homogenisation of macroinvertebrate communities in recently intermittent Alpine watercourses. Freshw. Biol. 65, 2096–2107. doi:10.1111/fwb.13605
Poff, N. L., Olden, J. D., Vieira, N. K. M., Finn, D. S., Simmons, M. P., and Kondratieff, B. C. (2006). Functional trait niches of North American lotic insects: Traits-based ecological applications in light of phylogenetic relationships. J. North. Am. Benthol. Soc. 25, 730–755. doi:10.1899/0887-3593(2006)025[0730:FTNONA]2.0.CO;2
Prokin, A. A., and Seleznev, D. G. (2021). Structure of macrozoobenthos in floodplain lakes under conditions of different durations of spring flooding. Inland Water Biol. 14, 573–580. doi:10.1134/s1995082921050126
Rosi-Marshall, E. J., Vallis, K. L., Baxter, C. V., and Davis, J. M. (2016). Retesting a prediction of the river continuum concept: Autochthonous versus allochthonous resources in the diets of invertebrates. Freshw. Sci. 35, 534–543. doi:10.1086/686302
Sargac, J., Johnson, R. K., Burdon, F. J., Truchy, A., Risnoveanu, G., Goethals, P., et al. (2021). Forested riparian buffers change the taxonomic and functional composition of stream invertebrate communities in agricultural catchments. Water 13, 1028. doi:10.3390/w13081028
Schutz, S. A., and Fureder, L. (2018). Unexpected patterns of chironomid larval size in an extreme environment: A highly glaciated, alpine stream. Hydrobiologia 820, 49–63. doi:10.1007/s10750-018-3579-y
Scotti, A., Tappeiner, U., and Bottarin, R. (2019). Stream benthic macroinvertebrates abundances over a 6-year monitoring period of an Italian glacier-fed stream. Biodivers. Data J. 7, e33576. doi:10.3897/BDJ.7.e33576
Sueyoshi, M., Nakano, D., and Nakamura, F. (2014). The relative contributions of refugium types to the persistence of benthic invertebrates in a seasonal snowmelt flood. Freshw. Biol. 59, 257–271. doi:10.1111/fwb.12262
Suren, A. M., and Jowett, I. G. (2006). Effects of floods versus low flows on invertebrates in a New Zealand gravel‐bed river. Freshw. Biol. 51, 2207–2227. doi:10.1111/j.1365-2427.2006.01646.x
Tolotti, M., Cerasino, L., Donati, C., Pindo, M., Rogora, M., Seppi, R., et al. (2020). Alpine headwaters emerging from glaciers and rock glaciers host different bacterial communities: Ecological implications for the future. Sci. Total Environ. 717, 137101. doi:10.1016/j.scitotenv.2020.137101
Vione, D., Colombo, N., Said-Pullicino, D., Bocchiola, D., Confortola, G., Salerno, F., et al. (2021). Seasonal variations in the optical characteristics of dissolved organic matter in glacial pond water. Sci. Total Environ. 759, 143464. doi:10.1016/j.scitotenv.2020.143464
Wang, L., Gao, Y., Han, B.-P., Fan, H., and Yang, H. (2019). The impacts of agriculture on macroinvertebrate communities: From structural changes to functional changes in Asia's cold region streams. Sci. Total Environ. 676, 155–164. doi:10.1016/j.scitotenv.2019.04.272
Wang, L., Yang, H. J., Li, K., Li, L., Nan, X. F., and Zhang, Z. X. (2018). Seasonal dynamics of macroinvertebrate community structure in a headwater stream in the Changbai Mountains. Acta eco. Sin. 38, 4834–4842. doi:10.5846/stxb201707121259
Zhang, H., Zhang, Y., Diao, S., Ye, J., Xu, B., Feng, C., et al. (2019). Difference of macrobenthos community composition and diversity in different sub- habitats in salt marsh wetland of the Yangtze River Estuary. Chin. J. Ecol. 38, 3102–3109. doi:10.13292/j.1000-4890.201910.037
Zhou, B. Q., and Zhai, P. M. (2021). The constraint methods for projection in the IPCC Sixth Assessment Report on climate change. Acta Meteorol. Sin. 79, 1063–1070. doi:10.11676/qxxb2021.069
Keywords: macroinvertebrate biodiversity, functional traits, climate change, freshwater, functional diversity, mid-latitude alpine streams
Citation: Gao Y, Rong L, Cao L, Li K, Lin C, Zhang Z, Xiang H and Yang H (2022) Temporal changes in headwater streams macroinvertebrate assemblages during the snowmelt season in northeast china. Front. Environ. Sci. 10:960254. doi: 10.3389/fenvs.2022.960254
Received: 06 June 2022; Accepted: 25 July 2022;
Published: 22 August 2022.
Edited by:
Hamid Gholami, University of Hormozgan, IranReviewed by:
Binbin Ren, Beijing Academy of Forestry and Landscape Architecture, ChinaChunyang Zhu, Huazhong Agricultural University, China
Martin Pfeiffer, University of Bayreuth, Germany
Copyright © 2022 Gao, Rong, Cao, Li, Lin, Zhang, Xiang and Yang. This is an open-access article distributed under the terms of the Creative Commons Attribution License (CC BY). The use, distribution or reproduction in other forums is permitted, provided the original author(s) and the copyright owner(s) are credited and that the original publication in this journal is cited, in accordance with accepted academic practice. No use, distribution or reproduction is permitted which does not comply with these terms.
*Correspondence: Zhenxing Zhang, zhangzx725@nenu.edu.cn; Hongyong Xiang, Xianghy480@nenu.edu.cn; Haijun Yang, yang@nenu.edu.cn
†These authors have contributed equally to this work