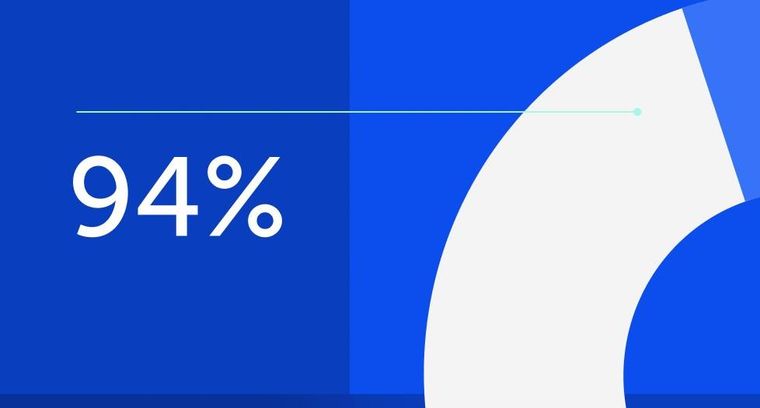
94% of researchers rate our articles as excellent or good
Learn more about the work of our research integrity team to safeguard the quality of each article we publish.
Find out more
ORIGINAL RESEARCH article
Front. Environ. Sci., 19 August 2022
Sec. Toxicology, Pollution and the Environment
Volume 10 - 2022 | https://doi.org/10.3389/fenvs.2022.954158
This article is part of the Research TopicPollutants and Heat Treatment of Livestock Manure: Emission, Control, and Environmental Risk AssessmentView all 5 articles
Composting can convert organic materials into stable humus (HS), resulting in high-fertility organic fertilizers that were widely used in the treatment of agricultural wastes. Therefore, it would be beneficial to strengthen the degree of composting humification by exploring the formation law and the main influencing factors of composting humification for different agricultural wastes. In this study, straw, cabbage, chicken manure, and pig manure were used as research objects, and the industrial mixed-material strip-stack composting and the single-material laboratory reactor composting were carried out, respectively. The results showed that the industrial mixed-material composting mode was beneficial to the continuation of the high-temperature period for composting (the heat lasts for 20 days), and the high lignin treatment T2 (straw + chicken manure composting) helped to promote the degradation of lignin and formation of humic acid (HA). At the end of composting, the absolute degradation of lignin in T2 treatment increased by 13.18% and 120.02% (p < 0.01) compared with T1 (straw + cabbage + chicken manure) and T3 (straw + pig manure) treatments, respectively (p < 0.01), and the humic acid (HA) content increased by 11.78% and 26.59% (p < 0.05). In the single-material laboratory reactor composting system, the continuous high-temperature period (31 days) contributed to the degradation of the composting materials and the formation of HA in the straw (TS) composting treatment; at the end of composting, the absolute degradation amount of TS lignin increased by 137.98%, 170.99%, and 196.82% compared with TV (cabbage), TC (chicken manure), and TP (pig manure) treatments (p < 0.01), and the HA content increased by 281.76%, 377.57%, and 460.71% (p < 0.01), respectively. Redundancy analysis showed that temperature was the main environmental factor affecting the decomposition and humification process of composting organic materials; the increase of lignin absolute degradation was helpful to promote the formation of HA. This study found that temperature was the main environmental factor that drove lignin degradation and promoted the humification process of agricultural waste composting; the lignin content in the composting material was the basis for the formation of HA, and the absolute degradation amount of lignin was the main material factor that promoted the humification process.
With the rapid development of the social economy and population, the output of agricultural waste in China is growing increasingly, which is harmful to human life, production, and the environment (Gao et al., 2021). So far, the annual output of straw wastes is as high as 900 million tons, of which about 30% are unused (Chang et al., 2021); the annual output of vegetable waste reached 500 million tons, and the unused proportion exceeded 90% (Chang et al., 2019); the annual output of livestock and poultry manure is as high as 3.8 billion tons, and the comprehensive utilization rate is less than 60% (Baldi et al., 2020). As a process of degradation of organic waste in an aerobic environment, composting has become an eco-friendly and economically feasible way to treat organic waste (Cheng et al., 2013; Awasthi et al., 2016). The main goal of composting is to convert efficiently organic matter into more stable humus (HS) (Bhatia et al., 2013; Jurado et al., 2015). HS is not only an important product of aerobic composting but also one of the most important criteria for evaluating the aerobic composting process (Wu et al., 2017b). As a part of HS, humic acid (HA) mainly contains abundant functional groups such as carboxyl, quinone, and hydroxyl (Gao et al., 2019; Wu et al., 2018; Wu et al., 2017a), which can complex with various pollutants, thereby reducing the migration and transformation of toxic and harmful substances in the environment (Wong et al., 2009; Guo et al., 2016; Jurado et al., 2015; Canellas et al., 2015; Guo et al., 2019). Therefore, it is very essential for environmental management to deal effectively with agricultural wastes from different sources and explore the formation law of HA in composting.
Agricultural waste composting treatment usually uses straw with high carbon content and livestock manure with high nitrogen content to adjust the appropriate C/N ratio and moisture co-composting (Guerra-Rodríguez et al., 2006; Wu et al., 2019; Bai et al., 2020). Straw was generally considered to be the main factor limiting the rapid and efficient composting of agricultural waste due to a large amount of aromatic compound lignin (McCann and Carpita, 2015; Yang et al., 2016; Kurata et al., 2018). However, when composting with materials that are rich in lignocellulose, lignin is not only the source but also the skeleton of HA (Wang et al., 2021). The addition of higher lignin materials will enhance the temperature and duration of the high-temperature phase of composting to a certain extent (Hemati et al., 2021) and sustain high temperature, which is contributed to the degradation of lignocellulose and the formation of HA (Chen et al., 2019; Ning et al., 2020). The formation of HA in composting takes place in two parts: the degradation of lignin and the condensation of precursor substances (Guo et al., 2019). On the one hand, lignin can be partially oxidized into polyphenols and quinones under the action of microorganisms (Paradelo et al., 2013; Kulikowska, 2016); on the other hand, lignin and its oxidation products can participate in the formation of HS through condensation or polymerization together with microbial degradation products such as amino acids and reducing sugars (Zhang et al., 2019). However, this process is affected by various physicochemical parameters, such as feedstock type, temperature, etc. (Petric et al., 2012; Silva et al., 2014; Guo et al., 2019; Ge et al., 2022).
Different sources and types of organic materials used for composting can lead to different lignocellulose contents and the sources of HA precursors, and thus has different effects on the microbial community and structure. Ultimately, this results in the synthesis of different HA contents that produced were by composting (Gao et al., 2019); the composting temperature was the main environmental factor that dominated this process (Rich et al., 2018).
Considering the abovementioned reasons, it is clear that the lignin content played a major role in composting humification and increasing the amount of lignin degradation in composting materials was expected to improve the degree of composting humification. At present, many studies have explored the enhancement of the composting humification process by regulating environmental factors and exogenous inoculation of microorganisms (Chen et al., 2019; Xu et al., 2022b; He et al., 2022), but there are few studies on the formation law of HA and the main impact factors during the composting process of different raw materials. Therefore, it is speculated that increasing the lignocellulose content and degradation amount of composting organic materials may strengthen the composting humification process and increase the HA content of the compost. On this basis, straw, cabbage, chicken manure, and pig manure were used as the main raw materials in this study, and the HA formation rules and influencing factors were studied by the industrial mixed-material composting and the single-material laboratory reactor composting mode, respectively. The research objectives are (1) to explore the relationship between lignocellulose degradation and HA formation during aerobic composting and (2) to analyze the main material factors that drive the formation of HA.
In this study, straw, cabbage, chicken manure, and pig manure were used as the main raw materials. The basic physical and chemical properties are shown in Table 1.
In this study, two composting methods were set up: one was a factory-based mixed-material composting mode and the other was a single-material laboratory reactor composting mode.
In the industrial mixed-material composting system, straw was used as the main carbon source for composting, and cabbage, chicken-manure, and pig-manure were the nitrogen sources. The method of stacking composting was adopted (the length, width, and height of the pile body are 50, 2, and 1.5 m, respectively.), and three groups of treatments, T1 (straw + cabbage + chicken manure), T2 (straw + chicken manure), and T3 (straw + pig manure) were set up, and each group was repeated three times. For every 100 kg of the composting material, the additional amounts of straw added were 31.13, 47.28, and 15.43 kg, respectively. The initial C/N of the composting material was adjusted to 25 kg and the moisture content was adjusted to about 60% for compost fermentation. The mixed material was loaded into a mesh bag, weighed, and then buried in the upper, middle, and lower positions of each composting system to calculate the degradation rate of the composting material. The ingredients were turned once a day during the composting process until fermentation was complete. In the single-material laboratory reactor composting system, the straw and cabbage were crushed to 2–3 cm, and the pig and chicken manures were sun-dried. The four single-material composting treatments, TS (straw), TV (cabbage), TC (chicken manure), and TP (pig manure) were designed, respectively, and each group was repeated three times; the moisture content was adjusted to 55% for composting and fermentation. At different positions of the upper, middle, and lower parts of each composting reactor, a mesh bag containing 100.00 g of the composting material was buried, and sampling was executed to measure the degradation rate of the organic material. The composting reactor was mainly made of 304 stainless, steel with a diameter of 0.42 m and a height of 0.72 m. Each fermenter was equipped with an aerobic sensor, a temperature probe, a display, and a metal grid of 1 × 1 mm two holes was fixed at 7 cm from the bottom to support the composting material and allow composting and aeration from the bottom (Figure 1). During the fermentation process, the aeration rate was set to 5 L min−1 and the ventilation frequency was 6 min h−1; forced aeration was conducted and the heap was turned every 3 days until the fermentation was completed.
FIGURE 1. Schematic diagram of the lab-scale forced ventilation circular cylinder reactor (100 L capacity).
Compost sampling was carried out using the five-point sampling method after each pile turnover was completed. Also, the five samples were mixed absolutely and divided into two parts, one of which was stored at 4°C for the humification progress indicator determination, and the other part was air-dried and passed through a 0.1 mm sieve for other chemical analyses. In the industrial mixed-material composting system, samples were collected on days 0, 3, 6, 15, 20, 25, 30, 35, and 40 of composting, respectively, and samples were taken on days 0, 3, 6, 12, 24, and 40of composting in a single-material laboratory reactor composting system.
The composting temperature was measured and recorded with a thermometer (JRS-LCD 105). The seed germination index (GI) of the composting samples was determined according to the method of Yu et al. (2011). The lignin content was determined according to the analytical method reported by Xu Y. et al. (2022). After the air-dried composting samples were made into powder, it was treated with acidic detergents, neutral detergents, and sulfuric acid to extract the lignin and determine its content. HS, FA, and HA were extracted and determined according to the method described by Zhang and Sun (2015).
Physicochemical properties were determined on three parallel samples. Differences in the physicochemical properties of samples were expressed as means and compared statistically using ANOVA testing at the 5% level of significance, and by the Least Significant Difference (LSD) test in SPSS 19 for Windows® software (IBM Corp., Armonk, NY, United States) to determine changes in the parameters with time. Excel® software (Microsoft, Inc., Redmond, WA, United States) was used to plot the analytical results. Differences between values at p > 0.05 were considered not significantly different.
The change in the pile temperature was the main indicator to characterize the decomposing process and the microbial activity of organic materials, and it is also a key factor in the whole composting process (Rich et al., 2018). As shown in Figure 2A, in the industrial mixed-material composting system, the T1, T2, and T3 treatments entered the high-temperature phase (≥50°C) on days 5, 5, and 7, respectively, and the high-temperature stage lasted for 20 days, which met the standard requirements of the composting harmless treatment (Joseph et al., 2018). Compared with the composting systems of straw + cabbage + chicken manure (T1) and straw + chicken manure (T2), the time of straw + pig manure (T3) composting entering the high-temperature stage (≥50°C) was delayed by 2 days (Figure 2A). Due to the different compositions of composting raw materials, different heterogeneous environments were formed to mediate the metabolic activities of organisms, which was the main reason that affected the slow warming of the T3 treatment (Gabhane et al., 2012). Therefore, further understanding of the degradation of organic materials in different material composting systems due to temperature changes may be helpful to evaluate the process and law of composting humification.
The seed germination index (the GI value) has always been considered to be the most reliable, sensitive, and effective indicator to reflect the maturity and harmlessness of the compost (Banegas et al., 2007; Meng et al., 2018). When GI ≥50%, the toxicity of the composting materials was low; when GI ≥80%, the complete decomposing of the composting materials will not affect the growth and development of plants (Saidi et al., 2009; Wong et al., 2017). As shown in Figure 2B, at the end of composting, the T1, T2, and T3 treatments could all meet the fermentation standard (GI≥80%), and the GI values were 92.3%, 97.5%, and 88.3%, respectively, among which the T1 and T2 treatments were significantly higher than that of T3 treatment (p <0.05); the results showed that there were differences in the composting maturity of different material compositions. Among them, the decomposing effect of the T2 treatment was the best, which may be related to the relatively high content and the lower toxicity of lignin in this system (Figure 4B).
In this study, the variation trend of the HS content in the industrial mixed-material composting system followed the typical evolution of aerobic composting. During the composting process, the HS content of all treatments increased rapidly (Figure 3A), and at the end of composting, the HS contents of T1, T2, and T3 treatments were 104.2, 110.03, and 100.97 g kg−1, respectively, in which the HS content in the T2 treatment was significantly higher than those of T1 and T3 treatments (p < 0.05),. The reason may be directly related to the relatively high lignin content in the T2 treatment (Figure 4B), which indicated the importance of lignin content in the composting material to HS formation.
FIGURE 3. Changes in (A) humus, (B) fulvic acid, and (C) humic acid of composting in mixed material.
Fulvic acid (FA) is a component of HS with a relatively small molecular weight and simple structure, which was decomposed easily by microorganisms (Li et al., 2015; Wong et al., 2017; Zhang et al., 2018). In the industrial mixed-material composting system, the FA content of each treatment increased first and then decreased slowly (Figure 3B), and the rapid decomposition of lignin by microorganisms at high temperature was the main reason for the rapid increase of FA. Subsequently, one part was decomposed by microorganisms, and the other part was used by microorganisms as an energy material to form more stable HA, which was the main reason for the slow decline of FA in the cooling and decomposing period (Wu et al., 2018; Xie et al., 2019); these results were consistent with the research of Li et al. (2015).
As shown in Figure 3C, the changing trend of the HA content was consistent with that of conventional composting (Zhang et al., 2018). In an industrial mixed-material composting system, at the end of composting, the HA content of the T2 treatment was significantly higher than those of T1 and T3 treatments (p < 0.05), reaching 67.37 g kg−1, an increase of 11.78% and 26.59% compared with T1 and T3, respectively. Since lignin as the source and skeleton of HA (Stevenson, 1982; Wang et al., 2021) played an important role in the condensation or polymerization of composting HA, the significant increase of lignin absolute degradation (Figure 4D) can well explain the increase of HA in the T2 treatment (Lu et al., 2018).
The degradation rate of raw materials during the composting process can reflect the decomposing progress of composting (Xu et al., 2016; Zhao et al., 2016). As shown in Figure 4A, the cumulative degradation of organic materials showed an upward trend in the whole process of mixed-materials composting; the long duration of the high-temperature period affected the respiration and the release of the heat of composting microorganisms, promoting the biodegradation of composting organic materials, which was the main reason for the rapid degradation of organic materials in each treatment of composting (Qiao et al., 2019). At the end of composting, the degradation rates of organic materials in T1, T2, and T3 treatments were 21.2%, 18.5%, and 21.5%, respectively, and the cumulative degradation of organic materials in T2 treatment was 14.59% and 16.22% lower than that of T1 and T3 treatments, respectively (p < 0.05). Due to the abundant presence of the aromatic compound lignin (Figure 4B), the strong resistance to biodegradation during the composting process was the main reason that the cumulative degradation of organic materials in straw + chicken manure (T2) treatments was relatively slow (Kurata et al., 2018; McCann and Carpita et al., 2015; Yang et al., 2016). The decomposition of lignin played an important role in the condensation of HA in composting (Stevenson, 1982), and the decomposition of macromolecular organic carbon such as lignocellulose was a key factor determining the composting maturity process (Zhu et al., 2021). As shown in Figure 4B, the lignin content of T1, T2, and T3 treatments decreased from 10.837 kg/100 kg, 12.728 kg/100 kg, and 8.105 kg/100 kg at the beginning of secondary fermentation to 5.23 kg/100 kg, 6.24 kg/100 kg and 5.9 kg/100 kg at the end of composting, respectively. In terms of relative degradation rates, they were significantly higher in T1 and T2 treatments than that in the T3 treatment (p < 0.01), which were 51.74%, 50.97%, and 27.21% at the end of composting, respectively (Figure 4C). The content and degradation rate of lignin significantly correlated with the formation of HA (p < 0.05), which was consistent with the research by Kulikowska and Sindrewicz (2018). Lignin biodegradation products (phenols and quinones) are important precursors for HA formation (Guo et al., 2019), and at the end of composting, the absolute degradation amounts (referred to as the quality of lignin degraded during the composting) of lignin were 6.72 kg/100 kg, 7.64 kg/100 and 3.47 kg/100 kg, respectively, in the T2 treatment, which were 13.70% and 120.17% higher than those in T1 and T3 treatments (Figure 3D), which were positively correlated with HA (Figure 3A); this result further validated the importance of lignin in HA formation.
In the single-material laboratory reactor composting system, the change curve of the composting temperature also followed the evolution law of the aerobic composting process (Figure 5A). The temperature of all treatments increased sharply during the composting process. TS, TV, TC, and TP treatments reached the high-temperature phase (≥50°C) on days 4, 2, 2, and 1, respectively, and the duration of the high-temperature period was 31, 8, 7, and 9 days, respectively. Among them, the highest temperature of single-straw (TS) composting reached 73°C, and the highest temperature of the other treatments did not exceed 57°C (Figure 5A), which may be directly related to the abundant existence of lignocellulose in straw to provide a sufficient carbon source for microbial respiration and metabolism. The results showed that aerobic composting of lignin-rich organic materials can enhance the temperature and prolong the duration of the high-temperature period of composting, thereby accelerating the decomposition of lignocellulose.
FIGURE 5. Changes in (A) the temperature and (B) germination index of composting in a single material.
The seed germination index can reflect the maturity degree of the compost to a certain extent, as shown in Figure 5B; the GI value of the single-material laboratory reactor composting decreased first and then increased, and the reason for the decrease was related to the secretion of organic acids and the production of ammonia (Awasthi et al., 2014; Wang et al., 2016); at the end of composting, only TC composting failed to meet the maturity standard (GI<80%), which may be related to the release of a large amount of inorganic salt in the chicken-manure fermentation process on the one hand (Chen et al., 2020), and the relatively low-fermentation intensity on the other hand (Figure 5A). The longer duration of the high-temperature period (31 days) was the main reason that the GI value of the straw compost (TS) was higher than those of other treatments (p < 0.05).
In the single-material laboratory reactor composting system, the HS content showed an overall upward trend in the straw (TS) and cabbage (TV) composting treatment. In terms of the increasing rate, TS was 286.67% of the TV treatment at the end of composting; the HS content in TC (chicken-manure) and TP (pig-manure) treatments showed a decreasing trend in the whole fermentation process (Figure 6A). The prolonged high-temperature period (31 days) (Figure 5A) accelerated the continuous decomposition of lignin (Figure 7D), which was the main reason for the significant increase of FA in the TS treatment (Figure 6B). At the end of composting, the HS contents of TS, TV, TC, and TP treatments were 179.8, 46.5, 27.6, and 26.5 g kg−1, respectively; the continuous high temperature promotes the rapid degradation of lignin to form a large number of HA precursors (phenols and quinones) to strengthen the polymerization of HA, which was the main reason for the significant increase in the HS TS treatment (Wu et al., 2017b). At the end of composting, the HA content of the TS treatment reached 125.6 g kg−1, an increase of 281.76%, 377.57%, and 460.71% compared with each treatment, respectively (Figure 6C), which was positively correlated with the absolute degradation of lignin (p < 0.05). Lignin is the source and skeleton of HA formation (Stevenson, 1982; Wang et al., 2021), and the excessive lignin content in straw (Figure 7B) provided a possibility for the formation of HA during the straw composting process. In addition, the longer duration of the high-temperature period and the higher temperature (Figure 5A) enhanced the massive degradation of lignin (Figure 7D), which was the main reason why the HA content in the TS treatment was significantly higher than those of TV, TC, and TP treatments. The abovementioned results further verified the importance of the lignin content in the composting material to the humification process.
FIGURE 6. Changes in (A) humus, (B) fulvic acid, and (C) humic acid of composting in a single material.
FIGURE 7. The degradation rate changes in the raw material and lignin in single material composting.
Exploring the degradation of organic materials was helpful for further analysis of the composting fermentation process. As shown in Figure 7A, the cumulative degradation rate of organic materials in the single-material laboratory reactor composting system was consistent with the changing trend of the industrial mixed-material composting mode (Figure 4A). However, the degradation of organic materials in each treatment of single-material composting mainly was concentrated in the early stage of composting (0–12 days), which was basically consistent with the duration of the high-temperature period of composting (Figure 5A), further indicating the importance of temperature on the decomposition of organic materials, consistent with the research results of Zhao X. et al. (2022) and He et al. (2022). In terms of the change in the lignin content, the lignin content of the straw (TS) composting treatment was most rapidly decomposed (Figure 7B) in the whole single-material composting system, which may be related to the long duration of the high-temperature period (31 days) during the composting process to promote the continuous decomposition of lignin (Figure 5A). As shown in Figure 7C, the relative degradation rate of lignin in the TV treatment was significantly higher than those of TS, TP, and TC treatments (p < 0.05), which may be directly related to the relatively low lignin content in the cabbage composting system (Figure 7B) and the relatively suitable initial C/N of cabbage composting (initial C/N was 29.1). In terms of the absolute degradation amount of lignin, it was significantly higher in the TS treatment than those in other treatments (p < 0.01); at the end of composting, the absolute degradation amounts of the TS, TV, TC, and TP lignin per 100 kg material were 11.98, 4.91, 4.31, and 3.95 kg, respectively (Figure 7D), which positively correlated with the formation of HA (p < 0.05). In addition, it was found that the decomposition of lignin played an important role in the formation of HA in composting, and the significant increase in the absolute degradation amount of lignin in the straw composting system was the main reason for the significant increase in the HA content in the TS treatment (Figure 6C).
In general, composting humification processes were affected by changes in environmental parameters (Bhatia et al., 2013). Furthermore, the breakdown of lignocellulose was very important for the HA synthesis because their degradation products served as the basic skeleton for HA formation (Stevenson, 1982). In order to study the key factors that affected the humification process, redundancy analysis (RDA) was used to evaluate the industrial mixed-materials composting of organic materials from different sources and the correlation between the degradation of organic materials and HA formation in the single-material laboratory reactor composting mode (Figure 8). The results showed that the relative degradation rate, material degradation amount, and absolute degradation amount of lignin were positively correlated with the HA content (p < 0.01), while the content of lignin was negatively correlated with the HA content (p < 0.01) under the conditions of the industrial mixed-materials composting; in addition, temperature also had a significant effect on the humification process (p < 0.01) (Figure 8A), which was consistent with the result found in the reports of Zhao Y. et al. (2022) and Xu et al. (2022c), indicating that the lignin content during the composting process was the basis for the formation of HA. The temperature was the main environmental factor affecting the humification process, and the degradation amount of lignin was another major factor for HA formation. The redundancy analysis (RDA) of the single-material laboratory reactor composting system showed that the absolute degradation amount and the relative degradation rate of lignin had the most significant effects on the formation of HA (p < 0.01), followed by the degradation of organic materials (Figure 8B), which further proved the important role of the degradation amount of lignin in the composting humification process.
FIGURE 8. Redundancy analysis (RDA) of two composting methods: mixed material (A) and single material (B). Red and black arrows represent environmental and humic acid parameters, respectively. Note: T: temperature; LC: lignin content; DR: the cumulative degradation rate of organic materials; A-d: absolute degradation of lignin; R-d: the relative amount of lignin degradation; HS, humus; FA, fulvic acid; HA, humic acid.
Temperature is the main environmental factor driving the lignin degradation and promoting the humification process for agricultural waste composting. Also, the lignin degradation can promote the increase in the composting temperature. The lignin content was the basis for the formation of composting HA, and the absolute degradation amount of lignin was an important factor driving the formation of HA. Therefore, increasing the lignin content and absolute degradation amount in composting materials is one of the main means to strengthen the composting humification process.
The original contributions presented in the study are included in the article/Supplementary Material; further inquiries can be directed to the corresponding author.
BZ: data curation, methodology, writing the original draft. YW: data curation, methodology, writing original draft, and funding acquisition. HS: methodology, software, formal analysis, writing review, and editing. ZX: supervision and funding acquisition.
This work was supported by the Major Science and Technology Special Project of Yunnan Province (202202AE090025), the National Natural Science Foundation of China (32160744) and Top Young Talents of Yunnan High-level Talent Training and Support Program (A3012020057).
The authors declare that the research was conducted in the absence of any commercial or financial relationship that could be construed as a potential conflict of interest.
All claims expressed in this article are solely those of the authors and do not necessarily represent those of their affiliated organizations, or the publisher, the editors, and the reviewers. Any product that may be evaluated in this article, or claim that may be made by its manufacturer, is not guaranteed or endorsed by the publisher.
Awasthi, M. K., Pandey, A. K., Bundela, P. S., Wong, J. W. C., Li, R., and Zhang, Z. (2016). Co-composting of gelatin industry sludge combined with organic fraction of municipal solid waste and poultry waste employing zeolite mixed with enriched nitrifying bacterial consortium. Bioresour. Technol. 213, 181–189. doi:10.1016/j.biortech.2016.02.026
Awasthi, M. K., Pandey, A. K., Khan, J., Bundela, P. S., Wong, J. W. C., and Selvam, A. (2014). Evaluation of thermophilic fungal consortium for organic municipal solid waste composting. Bioresour. Technol. 168, 214–221. doi:10.1016/j.biortech.2014.01.048
Bai, L., Deng, Y., Li, J., Ji, M., and Ruan, W. (2020). Role of the proportion of cattle manure and biogas residue on the degradation of lignocellulose and humification during composting. Bioresour. Technol. 307, 122941. doi:10.1016/j.biortech.2020.122941
Baldi, E., Luciano, C., Martina, M., Claudio, M., and Moreno, T. (2020). Fourteen years of compost application in a commercial nectarine orchard: Effect on microelements and potential harmful elements in soil and plants. Sci. Total Environ. 752, 141894. doi:10.1016/j.scitotenv.2020.141894
Banegas, V., Moreno, J. L., Moreno, J. I., García, C., León, G., and Hernández, T. (2007). Composting anaerobic and aerobic sewage sludges using two proportions of sawdust. Waste Manage 27, 1317–1327. doi:10.1016/j.wasman.2006.09.008
Bhatia, A., Madan, S., Sahoo, J., Ali, M., Pathania, R., and Kazmi, A. A. (2013). Diversity of bacterial isolates during full scale rotary drum composting. Waste Manage 33, 1595–1601. doi:10.1016/j.wasman.2013.03.019
Canellas, L. P., Olivares, F. L., Aguiar, N. O., Jones, D. L., Nebbioso, A., Mazzei, P., et al. (2015). Humic and fulvic acids as biostimulants in horticulture. Sci. Hortic-Amsterdam 2015, 15–27. doi:10.1016/j.scienta.2015.09.013
Chang, H., Zhu, X., Wu, J., Guo, D., Zhang, L., and Feng, Y. (2021). Dynamics of microbial diversity during the composting of agricultural straw. J. Integr. Agric. 20, 1121–1136. doi:10.1016/S2095-3119(20)63341-X
Chang, R., Li, Y., Chen, Q., Guo, Q., and Jia, J. (2019). Comparing the effects of three in situ methods on nitrogen loss control, temperature dynamics and maturity during composting of agricultural wastes with a stage of temperatures over 70 °C. J. Environ. Manage. 230, 119–127. doi:10.1016/j.jenvman.2018.09.076
Chen, H., Awasthi, S. K., Liu, T., Duan, Y., Ren, X., Zhang, Z., et al. (2020). Effects of microbial culture and chicken manure biochar on compost maturity and greenhouse gas emissions during chicken manure composting. J. Hazard. Mat. 389, 121908. doi:10.1016/j.jhazmat.2019.121908
Chen, Y., Wang, Y., Xu, Z., Liu, Y., and Duan, H. (2019). Enhanced humification of maize straw and canola residue during composting by inoculating Phanerochaete chrysosporium in the cooling period. Bioresour. Technol. 293, 122075. doi:10.1016/j.biortech.2019.122075
Cheng, W., Lu, H., Dong, D., Deng, H., Strong, P. J., Wang, H., et al. (2013). Insight into the effects of biochar on manure composting: Evidence supporting the relationship between N2O emission and denitrifying community. Environ. Sci. Technol. 47, 7341–7349. doi:10.1021/es305293h
Gabhane, J., William, S. P. M. P., Bidyadhar, R., Bhilawe, P., Anand, D., Vaidya, A. N., et al. (2012). Additives aided composting of green waste: Effects on organic matter degradation, compost maturity, and quality of the finished compost. Bioresour. Technol. 114, 382–388. doi:10.1016/j.biortech.2012.02.040
Gao, X., Tan, W., Zhao, Y., Wu, J., Sun, Q., Qi, H., et al. (2019). Diversity in the mechanisms of humin formation during composting with different materials. Environ. Sci. Technol. 53, 3653–3662. doi:10.1021/acs.est.8b06401
Gao, X., Xu, Z., Li, Y., Zhang, L., and Luo, W. (2021). Bacterial dynamics for gaseous emission and humification in bio-augmented composting of kitchen waste. Sci. Total Environ. 801, 149640. doi:10.1016/j.scitotenv.2021.149640
Ge, M., Shen, Y., Ding, J., Meng, H., Zhou, H., Zhou, J., et al. (2022). New insight into the impact of moisture content and pH on dissolved organic matter and microbial dynamics during cattle manure composting. Bioresour. Technol. 344, 126236. doi:10.1016/j.biortech.2021.126236
Guerra-Rodríguez, E., Alonso, J., Melgar, M. J., and Vázquez, M. (2006). Evaluation of heavy metal contents in co-composts of poultry manure with barley wastes or chestnut burr/leaf litter. Chemosphere 65, 1801–1805. doi:10.1016/j.chemosphere.2006.04.023
Guo, X., Huang, J., Lu, Y., Shan, G., and Li, Q. (2016). The influence of flue gas desulphurization gypsum additive on characteristics and evolution of humic substance during co-composting of dairy manure and sugarcane pressmud. Bioresour. Technol. 219, 169–174. doi:10.1016/j.biortech.2016.07.125
Guo, X., Liu, H., and Wu, S. (2019). Humic substances developed during organic waste composting: Formation mechanisms, structural properties, and agronomic functions. Sci. Total. Environ. 662, 501–510. doi:10.1016/j.scitotenv.2019.01.137
He, J., Zhu, N., Xu, Y., Wang, L., Zheng, J., and Li, X. (2022). The microbial mechanisms of enhanced humification by inoculation with Phanerochaete chrysosporium and Trichoderma longibrachiatum during biogas residues composting. Bioresour. Technol. 351, 126973. doi:10.1016/j.biortech.2022.126973
Hemati, A., Aliasgharzad, N., Khakvar, R., Khoshmanzar, E., Asgari Lajayer, B., and van Hullebusch, E. D. (2021). Role of lignin and thermophilic lignocellulolytic bacteria in the evolution of humification indices and enzymatic activities during compost production. Waste Manag. 119, 122–134. doi:10.1016/j.wasman.2020.09.042
Joseph, S., Kammann, C. I., Shepherd, J. G., Conte, P., Schmidt, H.-P., Hagemann, N., et al. (2018). Microstructural and associated chemical changes during the composting of a high temperature biochar: Mechanisms for nitrate, phosphate and other nutrient retention and release. Sci. Total Environ. 618, 1210–1223. doi:10.1016/j.scitotenv.2017.09.200
Jurado, M. M., Suárez-Estrella, F., López, M. J., Vargas-García, M. C., López-González, J. A., and Moreno, J. (2015). Enhanced turnover of organic matter fractions by microbial stimulation during lignocellulosic waste composting. Bioresour. Technol. 186, 15–24. doi:10.1016/j.biortech.2015.03.059
Kulikowska, D. (2016). Kinetics of organic matter removal and humification progress during sewage sludge composting. Waste Manage 49, 196–203. doi:10.1016/j.wasman.2016.01.005
Kulikowska, D., and Sindrewicz, S. (2018). Effect of barley straw and coniferous bark on humification process during sewage sludge composting. Waste Manage 79, 207–213. doi:10.1016/j.wasman.2018.07.042
Kurata, Y., Mori, Y., Ishida, A., Nakajima, M., Ito, N., Hamada, M., et al. (2018). Variation in hemicellulose structure and assembly in the cell wall associated with the transition from earlywood to latewood in cryptomeria japonica. J. Wood Chem. Technol. 2018, 1–10. doi:10.1080/02773813.2018.1434206
Li, R., Wang, Q., Zhang, Z., Zhang, G., Li, Z., Wang, L., et al. (2015). Nutrient transformation during aerobic composting of pig manure with biochar prepared at different temperatures. Environ. Technol. 36, 815–826. doi:10.1080/09593330.2014.963692
Lu, Q., Zhao, Y., Gao, X., Wu, J., Zhou, H., Tang, P., et al. (2018). Effect of tricarboxylic acid cycle regulator on carbon retention and organic component transformation during food waste composting. Bioresour. Technol. 2018, 128–136. doi:10.1016/j.biortech.2018.01.142
McCann, M. C., and Carpita, N. C. (2015). Biomass recalcitrance: A multi-scale, multi-factor, and conversion-specific property. J. Exp. Bot. 66, 4109–4118. doi:10.1093/jxb/erv267
Meng, X., Liu, B., Xi, C., Luo, X., Yuan, X., Wang, X., et al. (2018). Effect of pig manure on the chemical composition and microbial diversity during co-composting with spent mushroom substrate and rice husks. Bioresour. Technol. 251, 22–30. doi:10.1016/j.biortech.2017.09.077
Ning, Z., Jun, G., Dong, L., Yanyun, Z., Bingqing, L., and Hongmei, J. (2020). Thermal pretreatment enhances the degradation and humification of lignocellulose by stimulating thermophilic bacteria during dairy manure composting. Bioresour. Technol. 319, 124149. doi:10.1016/j.biortech.2020.124149
Paradelo, R., Moldes, A. B., and Barral, M. T. (2013). Evolution of organic matter during the mesophilic composting of lignocellulosic winery wastes. J. Environ. Manage 116, 18–26. doi:10.1016/j.jenvman.2012.12.001
Petric, I., Helić, A., and Avdić, E. A. (2012). Evolution of process parameters and determination of kinetics for co-composting of organic fraction of municipal solid waste with poultry manure. Bioresour. Technol. 117, 107–116. doi:10.1016/j.biortech.2012.04.046
Qiao, C., Penton, C. R., Liu, C., Shen, Z., and Shen, Q. (2019). Key extracellular enzymes triggered high-efficiency composting associated with bacterial community succession. Bioresour. Technol. 288, 121576. doi:10.1016/j.biortech.2019.121576
Rich, N., Bharti, A., and Kumar, S. (2018). Effect of bulking agents and cow dung as inoculant on vegetable waste compost quality. Bioresour. Technol. 252, 83–90. doi:10.1016/j.biortech.2017.12.080
Saidi, N., Kouki, S., M’Hiri, F., Jedidi, N., Mahrouk, M., Hassen, A., et al. (2009). Microbiological parameters and maturity degree during composting of Posidonia oceanica residues mixed with vegetable wastes in semi-arid pedo-climatic condition. J. Environ. Sci. 21, 1452–1458. doi:10.1016/S1001-0742(08)62439-0
Silva, M. E. F., de Lemos, L. T., Nunes, O. C., and Cunha-Queda, A. C. (2014). Influence of the composition of the initial mixtures on the chemical composition, physicochemical properties and humic-like substances content of composts. Waste Manage 34, 21–27. doi:10.1016/j.wasman.2013.09.011
Stevenson, F. J. (1982). Humus chemistry; Genesis, composition, reactions. Soil Sci. 135, 129–130. doi:10.1097/00010694-198302000-00014
Wang, W., Hou, Y., Huang, W., Liu, X., Wen, P., Wang, Y., et al. (2021). Alkali lignin and sodium lignosulfonate additives promote the formation of humic substances during paper mill sludge composting. Bioresour. Technol. 320, 124361. doi:10.1016/j.biortech.2020.124361
Wang, X., Selvam, A., and Wong, J. W. C. (2016). Influence of lime on struvite formation and nitrogen conservation during food waste composting. Bioresour. Technol. 217, 227–232. doi:10.1016/j.biortech.2016.02.117
Wong, J. W. C., Karthikeyan, O. P., and Selvam, A. (2017). Biological nutrient transformation during composting of pig manure and paper waste. Environ. Technol. 38, 754–761. doi:10.1080/09593330.2016.1211747
Wong, W. C., Fung, S. O., and Selvam, A. (2009). Coal fly ash and lime addition enhances the rate and efficiency of decomposition of food waste during composting. Bioresour. Technol. 100, 3324–3331. doi:10.1016/j.biortech.2009.01.063
Wu, J., Qi, H., Huang, X., Dan, W., Yue, Z., Wei, Z., et al. (2018). How does manganese dioxide affect humus formation during bio-composting of chicken manure and corn straw? Bioresour. Technol. 269, 169–178. doi:10.1016/j.biortech.2018.08.079
Wu, J., Zhao, Y., Qi, H., Zhao, X., Yang, T., Du, Y., et al. (2017a). Identifying the key factors that affect the formation of humic substance during different materials composting. Bioresour. Techno. 244, 1193–1196. doi:10.1016/j.biortech.2017.08.100
Wu, J., Zhao, Y., Zhao, W., Yang, T., Zhang, X., Xie, X., et al. (2017b). Effect of precursors combined with bacteria communities on the formation of humic substances during different materials composting. Bioresour. Technol. 226, 191–199. doi:10.1016/j.biortech.2016.12.031
Wu, Y., Chen, Y., Shaaban, M., Zhu, D., Hu, C., Chen, Z., et al. (2019). Evaluation of microbial inoculants pretreatment in straw and manure co-composting process enhancement. J. Clean. Prod. 239, 118078. doi:10.1016/j.jclepro.2019.118078
Xie, X., Gao, X., Chaonan, P., Zimin, W., Yue, Z., Xu, Z., et al. (2019). Assessment of multiorigin humin components evolution and influencing factors during composting. J. Agric. food Chem. 67, 4184. doi:10.1021/acs.jafc.8b07007
Xu, J., Xu, X., Liu, Y., Li, H., and Liu, H. (2016). Effect of microbiological inoculants DN-1 on lignocellulose degradation during co-composting of cattle manure with rice straw monitored by FTIR and SEM. Environ. Prog. Sustain. Energy. 35, 345–351. doi:10.1002/ep.12222
Xu, Y., Bi, Z., Zhang, Y., Wu, H., Zhou, L., and Zhang, H. (2022a). Impact of wine grape pomace on humification performance and microbial dynamics during pig manure composting. Bioresour. Technol. 358, 127380. doi:10.1016/j.biortech.2022.127380
Xu, Z., Li, R., Wu, S., He, Q., Ling, Z., Liu, T., et al. (2022b). Cattle manure compost humification process by inoculation ammonia-oxidizing bacteria. Bioresour. Technol. 344, 126314. doi:10.1016/j.biortech.2021.126314
Xu, Z., Ma, Y., Li, Y., Li, G., Nghiem, L. D., and Luo, W. (2022c). Comparison between cold plasma, ultrasonication, and alkaline hydrogen peroxide pretreatments of garden waste to enhance humification in subsequent composting with kitchen waste: Performance and mechanisms. Bioresour. Technol. 2022, 127228. doi:10.1016/j.biortech.2022.127228
Yang, W. T., Gu, J. F., Zou, J. L., Zhou, H., Zeng, Q. R., and Liao, B. H. (2016). Impacts of rapeseed dregs on Cd availability in contaminated acid soil and Cd translocation and accumulation in rice plants. Environ. Sci. Pollut. R. 23 (20), 20853–20861. doi:10.1007/s11356-016-7326-5
Yu, Z., Zeng, G. M., Chen, Y. N., Zhang, J. C., Yu, Y., Li, H., et al. (2011). Effects of inoculation with Phanerochaete chrysosporium on remediation of pentachlorophenol-contaminated soil waste by composting. Process Biochem. 46, 1285–1291. doi:10.1016/j.procbio.2011.02.018
Zhang, L., and Sun, X. (2015). Effects of earthworm casts and zeolite on the two-stage composting of green waste. Waste Manage 39, 119–129. doi:10.1016/j.wasman.2015.02.037
Zhang, Z., Zhao, Y., Wang, R., Lu, Q., Wu, J., Zhang, D. Y., et al. (2018). Effect of the addition of exogenous precursors on humic substance formation during composting. Waste Manage 79, 462–471. doi:10.1016/j.wasman.2018.08.025
Zhang, Z., Zhao, Y., Yang, T., Wei, Z., Li, Y., Wei, Y., et al. (2019). Effects of exogenous protein-like precursors on humification process during lignocellulose-like biomass composting: Amino acids as the key linker to promote humification process. Bioresour. Technol. 291, 121882. doi:10.1016/j.biortech.2019.121882
Zhao, X., Li, B., Ni, J., and Xie, D. (2016). Effect of four crop straws on transformation of organic matter during sewage sludge composting. Integr. Agric. 15, 232–240. doi:10.1016/S2095-3119(14)60954-0
Zhao, X., Xu, K., Wang, J., Wang, Z., Pan, R., Wang, Q., et al. (2022a). Potential of biochar integrated manganese sulfate for promoting pig manure compost humification and its biological mechanism. Bioresour. Technol. 357, 127350. doi:10.1016/j.biortech.2022.127350
Zhao, Y., Weng, Q., and Hu, B. (2022b). Microbial interaction promote the degradation rate of organic matter in thermophilic period. Waste Manage 144, 11–18. doi:10.1016/j.wasman.2022.03.006
Keywords: different agricultural wastes, lignin degradation, humification process, humic acid, composting
Citation: Zhao B, Wang Y, Sun H and Xu Z (2022) Analysis of humus formation and factors for driving the humification process during composting of different agricultural wastes. Front. Environ. Sci. 10:954158. doi: 10.3389/fenvs.2022.954158
Received: 27 May 2022; Accepted: 22 July 2022;
Published: 19 August 2022.
Edited by:
Hua-jun Huang, Jiangxi Agricultural University, ChinaReviewed by:
Suyun Xu, University of Shanghai for Science and Technology, ChinaCopyright © 2022 Zhao, Wang, Sun and Xu. This is an open-access article distributed under the terms of the Creative Commons Attribution License (CC BY). The use, distribution or reproduction in other forums is permitted, provided the original author(s) and the copyright owner(s) are credited and that the original publication in this journal is cited, in accordance with accepted academic practice. No use, distribution or reproduction is permitted which does not comply with these terms.
*Correspondence: Zhi Xu, eHV6aGk5OTEwQDEyNi5jb20=
†These authors have contributed equally to this work and share the first authorship.
Disclaimer: All claims expressed in this article are solely those of the authors and do not necessarily represent those of their affiliated organizations, or those of the publisher, the editors and the reviewers. Any product that may be evaluated in this article or claim that may be made by its manufacturer is not guaranteed or endorsed by the publisher.
Research integrity at Frontiers
Learn more about the work of our research integrity team to safeguard the quality of each article we publish.