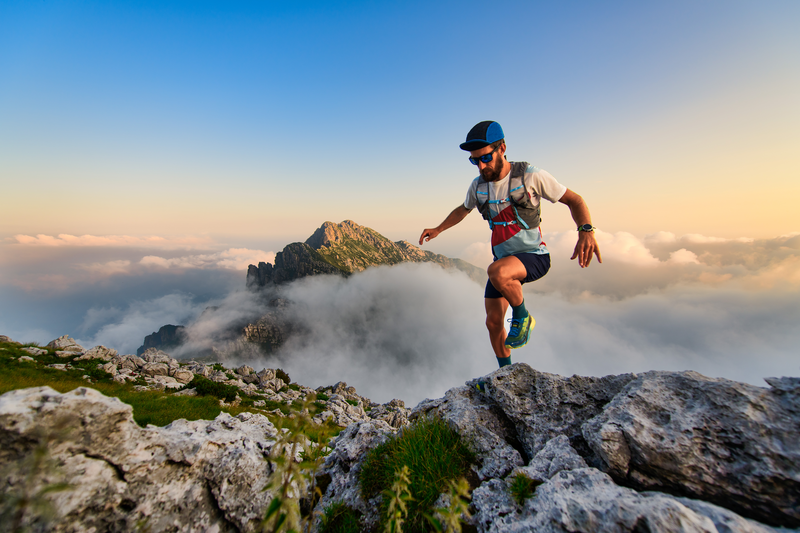
94% of researchers rate our articles as excellent or good
Learn more about the work of our research integrity team to safeguard the quality of each article we publish.
Find out more
ORIGINAL RESEARCH article
Front. Environ. Sci. , 02 September 2022
Sec. Drylands
Volume 10 - 2022 | https://doi.org/10.3389/fenvs.2022.954004
This article is part of the Research Topic Understanding Soil Wind Erosion and Control Practices in Arid and Semiarid Environments View all 10 articles
Wind erosion is a serious problem in agricultural regions and threatens the regional food production in Northeast China. However, limited information is available on the characteristics of wind erosion in arable soil in Northeast China. As a result, field-based research during periods of vulnerability to wind erosion is essential. This study quantified the characteristics of soil wind erosion under no-tillage (NT) and conventional tillage (CT) treatments in China’s northern “corn-belt.” The results determined the wind erosion transport mode of Mollisols to be generally characterized by creep and supplemented by saltation and suspension in Northeast China. The erodible particles of the creep accounted for 80.37% and 85.42% of the total wind erosion under the NT and CT treatments, respectively. During experiments with erodible particles in the saltation mode from the soil surface to 2 m, the majority of the particles were collected by the sampler at 0.5 m height, with the NT and CT treatments collecting 5.82 kg·m−2 and 6.93 kg·m−2 of erodible particles per unit area, respectively. Wind erosion on agricultural land was observed to be influenced by tillage practices, rainfall, wind speed, and soil moisture content. Average and maximum wind speeds exhibited significant positive correlations with wind erosion during April and May. Moreover, the erodible particles of each wind erosion transport mode (creep, saltation, and suspension) under CT were higher (1.73, 1.41, and 1.35 times) than those under the NT treatment. With less damage and greater protection of the surface soil, the NT treatment was able to decrease the occurrence of wind erosion and influence its outcome on farmland. Therefore, NT treatment should be encouraged as a key initiative for the reduction of wind erosion of arable soil in the Chinese Mollisol region.
Wind erosion is the movement of coarse and fine particles by wind across a landscape (Jarrah et al., 2020) and involves three transport processes: creep, saltation, and suspension (Cornelis, 2006). It is a global environmental problem faced by many countries (Katra et al., 2016; Yildiz et al., 2017) and not only causes serious on-site problems, such as a reduction in soil and crop productivity, but also induces off-site problems (e.g., dust storms) (Blanco and Lal, 2008). Furthermore, wind erosion brings great harm to industrial and agricultural production. Therefore, the measurement and prevention of wind erosion are crucial for sustainable land use and environmental protection (Uzun et al., 2020).
The Mollisol region in Northeast China possesses fertile and productive soil and is the country’s ecological security base (Liu et al., 2019). Due to highly intensive cultivation, irrational farming, and improper land use practices in the short term, the Mollisol region is easily eroded by rain, wind, freeze–thaw, snowmelt runoff, and other external agents occurring in this special geographical environment in Northeast China (Xie et al., 2019). The arable land fertility of this region is currently decreasing, which consequently threatens the grain production and food security in Northeast China (Shen et al., 2020). Numerous studies have focused on the underlying formation mechanism, influential factors, and hazards of water erosion and freeze-thaw erosion in this region (Jia et al., 2015; Yao et al., 2017; Xu et al., 2018; Shen et al., 2020). However, the wind eroded area in the Mollisol zone makes up 11.1% of the total area in Northeast China (Chi et al., 2019). Large amounts of fine-grained soil and its adsorbed organic matter are transported by wind erosion. This severely damages the productivity of the land, resulting in bare crop seeds following spring sowing and ultimately affects the seeding emergence rate (Sharratt et al., 2018). Thus, the agricultural production and ecological environment in the arable Mollisol region are seriously threatened by wind erosion, and consequently, exploring the characteristics of wind erosion on arable land is crucial for the Mollisol region in Northeast China.
As a widely promoted tillage treatment in Northeast China, no-tillage (NT) has been the focus of much research. In particular, NT is considered to have an important role in retaining soil water (Sekaran et al., 2021), controlling soil and water losses (Jia et al., 2019), improving soil structure, providing nutrients (Wang et al., 2019b), reducing straw burning, and protecting the ecological environment (Wang and Wang, 2022). Nevertheless, due to the constraints in the observation methods and research techniques, studies on the wind erosion in the arable Mollisol region in Northeast China are limited. Wind erosion is typically measured via wind tunnel tests and field measurements. More specifically, wind tunnel tests are employed to explore the quantitative relationships of the wind erosion influencing factors (Feizi et al., 2019). For example, Lyu et al. (2021) determined wind speed as the initiating force of wind erosion, with a positive correlation between wind speed and wind erosion intensity. Soil moisture content in arable land is reported to visually reflect the climatic state of the field and is negatively correlated with the wind erosion rate (Shahabinejad et al., 2019). Wassif et al. (2020) demonstrated the ability of straw mulching in NT to effectively reduce wind erosion efficiency, with a wind-induced soil erosion rate under NT observed to be 62.9% lower than that with conventional tillage (CT). However, compared to wind tunnel tests, field observations have the advantage of fully revealing the entire wind erosion process on arable land. The key influencing factors of wind erosion of arable land are a function of climatic conditions and tillage practices (Hong et al., 2020). These factors vary greatly across regions, and hence research on the primary controlling factors of wind erosion and the correlation between them is essential for the arable land in the Mollisol region.
The research in the current study was performed in the Mollisol region of Northeast China, a common experimental site for water erosion (Chen et al., 2019a) and freeze-thaw erosion (Chen et al., 2019b) under different tillage treatments. A revised wind erosion sampler was created by our research group to collect and measure soil particles by wind erosion, with specific applicability in Northeast China (Yang, 2018; Chen et al., 2019c). The principal aims of this study are to: 1) identify the wind erosion characteristics under NT and CT in the arable Mollisol region in Northeast China; and 2) analyze the factors influencing wind erosion under different tillage treatments and the correlation between them.
The study site is located at the Hailun Monitoring and Research Station of Soil and Water Conservation, Northeast Institute of Geography and Agroecology, Chinese Academy of Sciences, Heilongjiang Province (Figure 1). It is situated in the center of the typical Mollisol zone within Northeast China (47º23′ N, 126º51′ E; 240 m altitude) (Chen et al., 2019a). The mean annual temperature is 1.5°C (34.7℉), and the mean annual precipitation is approximately 530 mm, with 65% occurring from June to August. Total annual solar radiation is 4600 MJ m−2, and annual available accumulated temperature (≥10°C [50℉]) is 2,450°C (4,442℉). The soil is a loess-derived fine, mixed, superactive Oxyaquic Haplocryoll with a light silty clay texture, slightly acidic reaction, and 4.4% SOM (Table 1). The soil classification information was obtained from Liu et al. (2015) and the guidelines from the Key to Soil Taxonomy (Soil Survey Staff, 2014).
Wind erosion occurs during high wind speed events. The maximum wind speeds were measured in order to clarify the occurrence of high wind events from 2009 to 2018 occurring at the study site (Figure 2). Approximately 7% of wind speed observations exceed 14 m·s−1 from April to June. This period corresponds to less rainfall and field coverage in the black soil region of Northeast China compared to the rest of the year. Therefore, the subsequent field observations were performed from April to June.
FIGURE 2. Wind rose diagrams for January–March, April–June, July–September, and October–December during 2007–2018 in the study area.
Experiments were performed under no-tillage (NT) and conventional tillage (CT) treatments in the field during 2016–2018. The size of each experiment plot was 3.2 h m2. For the NT treatment, all biomass (with the exception of the harvested seeds) were cut and uniformly distributed across the plot in autumn. No other soil tillage practices were implemented. For the CT treatment, all aboveground biomass was removed manually and ridged via rotary tillage in the autumn. The field was ridged twice every 15 days after sowing (Chen et al., 2011).
Different field measurements were adopted to collect the erodible particles based on the three wind erosion transport modes, namely, creep, saltation, and suspension. In particular, the large-sized particles (0.5–1 mm diameter) generally creep with the rolling movement along the surface. Small-sized particles (<0.1 mm diameter) are suspended in the air for a long time, moving with the wind and subsequently falling slowly. New small particles are constantly added, and these particles move forward in a suspended state. Medium-sized particles (0.1–0.5 mm diameter) break away from the surface and make continuous leaping movements, denoted as saltation (Cornelis, 2006; Blanco and Lal, 2008; Avecilla et al., 2018). Based on the existing research and current field situation in the study area (Fryrear, 1986; Shao, 2001; Bagnold, 2012), we selected a set of devices to collect and measure the soil particles under the three transport forms (creep, saltation, and suspension) of the actual wind erosion in the field (Figure 3).
FIGURE 3. Collection system for soil erosion used in this study. (A-1, A-2, and A-3 are the collection subsystems of soil particle by suspension, creep, and saltation, respectively; (B) is the rBSNE sampler in the field).
The collection system for the wind erosion particles consists of three subsystems (Yang, 2018). The suspension subsystem includes one bucket (0.2 m height, 0.16 m diameter) and one bracing piece (2 m height), and collects the deposition of suspended materials (Figure 3-A-1). The creep subsystem contains one bucket (0.2 m height, 0.16 m diameter, Figure 3-A-2), with the barrel mouth level to the ground in order to increase accuracy (Bagnold, 1941; Liu et al., 2014; Kang et al., 2017). The last collecting subsystem is the revised Big Spring Number Eight (rBSNE) sampler for collecting materials by saltation at five heights (0.2, 0.5, 1.0, 1.5, 2.0 m). This system is based on the Big Spring Number Eight (BSNE) sampler (Figure 3A-3). However, we altered the original internal baffle from a large pore screen structure to a reverse louver baffle structure. Once air enters the sampler, the air speed is reduced and the suspended soil particles settle into the collection pan. A 60-mesh screen is used as the air discharge channel. The louver baffle reduces the movement of deposited soil particles, possibly increasing the loss of fine soil particles from the top of the 60-mesh screen (Chen et al., 2019c). Wind tunnel pretests reveal the retention efficiency of the soil particles by the rBSNE sampler to be significantly higher than that of the BSNE sampler under different wind speeds, particularly for particle sizes less than 0.25 mm at wind speeds above 10 m·s−1 (Table 2). In addition, the rBSNE sampler has a tail and can be freely rotated (Figure 3A-3). Thus, it can always be freely rotated with the main wind direction and the entrance of the sampler always directly facing the erosional wind direction.
TABLE 2. Retention rate of soil particles (%) by the BSNE sampler and rBSNE samplers for different material sizes under different wind speeds.
The wind erosion measurements were repeated three times for each tillage management. In order to avoid the influence of long distance transport of wind erosion on the results, each collection device was separated by a distance of 50 m. To further analyze the relationship between soil, wind erosion, and environmental factors, we measured soil water content, precipitation, and wind speed in the field during the observation period.
All statistical analyses were performed using the SPSS 16.0 (SPSS Inc., USA). Independent sample t-tests were performed to compare the retention rate of the material by the BSNE and rBSNE samplers. The correlation between the total erodible particles collected by the sampler, rainfall, average wind speed, maximum wind speed, and soil water content was also evaluated based on the linear correlation analysis. Differences were considered significant based on Tukey’s multiple comparison using a 0.05 level of probability. All data were expressed as means.
Figure 4 presents the total amount of erodible particles (creep, saltation, and suspension) for the NT and CT treatments in Northeast China from April to June during 2016–2018. Wind erosion was mainly caused by creep (81.15% and 84.07%, respectively), followed by saltation (18.30% and 15.51%, respectively), and suspension (0.55% and 0.42%, respectively).
FIGURE 4. Total erodible particles (creep, saltation, and suspension) during 1st April to 1st June under different tillage treatments in Northeast China by the rBSNE sampler (2016–2018).
Erodible particles in saltation mode were collected at five heights (0.2, 0.5, 1.0, 1.5, and 2.0 m) in the NT and CT treatments (Figure 5). The most erodible particles were collected by the sampler at 0.5 m height, with the NT and CT treatments collecting 5.82 kg·m−2 and 6.93 kg·m−2 of erodible particles per unit area, respectively. Above 0.5 m, the amount of erodible particles collected by the sampler was observed to decrease with increasing height.
FIGURE 5. Total erodible particles (saltation) by the rBSNE sampler at different height (0.2, 0.5, 1.0, 1.5, and 2.0 m) from the ground under different tillage during 1st April to 1st June in 2017.
NT and CT are currently the dominant tillage practices for the Mollisol region in Northeast China. Our results reveal the erodible particles of creep, saltation, and suspension under the CT treatment to exceed those of the NT treatment by 1.73, 1.41, and 1.35 times, respectively (Figure 4). Moreover, the amount of erodible particles of saltation under the NT treatment was significantly lower than that of the CT treatment within the height range from the surface to 0.5 m (Figure 5).
Figure 6 depicts the wind erosion and environmental factors observations in farmland from 2016 to 2018. The total daily precipitation from April to June was determined as 34.4, 40.6, and 51.6 mm, for 2016–2018, respectively. Due to the uncertainty of natural conditions, the distribution of rainfall and wind varied greatly from 2016 to 2018. The maximum wind speed from 2016 to 2018 ranged between 6.0-24.5 m·s−1, 6.4–23.1 m·s−1, and 4.0–12.3 m·s−1, respectively. The soil moisture content increased with the increase in precipitation. Furthermore, more erodible particles (creep, saltation, and suspension) were observed during periods of higher wind speeds and lower soil moisture content (e.g., 1st to 13th April in 2016; third to fifth May in 2017; and 16th to 20th May in 2018), while the reverse was observed for periods of lower wind speeds and higher soil moisture content (e.g., second to sixth May in 2016; fifth to sixth May in 2017; and 15th to 21st April in 2018).
FIGURE 6. Rainfall, average wind speed, maximum wind speed, soil water content, and erodible particles (creep, saltation, and suspension) under different tillage treatment over the period from 1st April to 1st June (2016–2018).
Table 3 reports the correlation between meteorological factors, soil water content, and sand transport under different tillage treatments. A significant positive correlation was observed between the total erodible particles collected by the sampler and both mean and maximum wind speeds under both the NT and CT treatments. In addition, wind erosion under the CT treatment exhibited a stronger relationship with rainfall and soil moisture than under the NT treatment.
TABLE 3. Correlation between the total erodible particles collected by the sampler (TMC, kg·m−2), rainfall (RF, mm), average wind speed (AWS, m·s−1), maximum wind speed (MWS, m·s−1), and soil water content (SWC, %) in NT and CT.
Affected by wind erosion and tillage, large quantities of soil particles are transported and lost from the surface of arable land each year during spring cultivation. Our results indicate surface creep as the major erosion type during the total transport of the Mollisol region in Northeast China. This is in agreement with Zobeck et al. (2003), who determined that creep transport accounted for 40% of total wind erosion. However, other studies have found saltation to be the dominant transport form of wind erosion, with the potential to provide power for creep and suspension (Martin and Kok, 2017; Liu and Bo, 2019). Under natural environments, a gradual transition is observed between the three erodible particles’ movement patterns, and each transition mode is related to the surface shear stress and size of the erodible particles (Zhang et al., 2021). In general, the particle size cut-off points for suspension, saltation, and creep wind erosion are approximately 100 and 500 μm (Fattahi et al., 2020). Previous research has suggested that when erodible particles gain momentum and change their resting state, the majority experience creep motion prior to saltation (Jarrah et al., 2020; Swann et al., 2020). As the creeping motion of the particles continues, more particles collide with each other, leading to a gradual increase in the energy of some erodible particles. The particles move faster than their wind-up velocity, inducing the saltation motion of some of the small particles (Andreotti et al., 2002; Pähtz et al., 2020). In addition, erodible particles moving under saltation can continuously strike up fine particles during wind erosion, which are blown away in the form of suspension under high winds, eventually falling back to the land surface (Kheirabadi et al., 2018). Small diameter (<100 μm), long transport distances, and prolonged suspension of erodible particles under suspension can complicate the monitoring and collection of suspension, resulting in sand transport rates from suspension often being neglected in wind erosion field studies (Jarrah et al., 2020). Similar to many studies, only a small number of erodible particles under suspension was observed at the height of 2 m in our results. In addition, clear differences were observed in the number of erodible particles under saltation between heights (Figure 5). The vertical distribution of erodible particles under saltation is influenced by two major factors, namely, the wind speed and the number of erodible particles (Yang et al., 2019). A large number of erodible particles were observed in the soil surface layer. The high roughness value of the surface layer slowed down the wind speed at this layer, and thus there was not enough power to carry the erodible particles out of the farmland surface. This agrees with Yang et al. (2019), who suggested that the high roughness value of the surface layer relative to other heights slowed down the wind speed and makes it less likely to erode particles, resulting in fewer erodible particles in the near-surface airflow relative to other heights in the vertical profile. The complex and microscopic exchanges of erodible particles and airflow increase with height (Tan et al., 2014). The current literature has not reached a consensus on the erodible particle distribution in the vertical profile of the saltation layers. Li et al. (2008) determined a relationship between the transport flux of erodible particles and the sand grain diameter from the soil surface to 20 cm height. The authors demonstrated that for erodible particles with diameters greater than 355 μm, the particle mass decreases with height according to a power law; otherwise, the mass decreases exponentially with height. Thus, the erodible particles must overcome the effects of gravity in order to maintain the mass in the saltation layer vertical profiles. Yang et al. (2019) identified a turning point for the vertical profile distribution of erodible particles. However, the number of erodible particles was observed to decrease, reducing the materials collected by the sampler. The launch velocities of erodible particles vary with their size (Li and Zhou, 2007), and thus the aeolian sand movement of the saltation layer fluctuates. This phenomenon is complex during the process of wind erosion. Our results are consistent with the work of Jia et al. (2015), who determined the wind erosion height of the wind-sand flow during wind erosion in black soil to be within 60 cm. This may be attributed to the similar grain-size fraction observed in both studies.
Different tillage treatments can produce highly variable results in terms of the wind erosion degree of agricultural soils. Our results agree with Xiao et al. (2021), who demonstrated that the sand transport of farmland under NT was significantly smaller than that under CT. Mozaffari et al. (2021) also reported wind erosion of tilled farmland to be more severe than untilled farmland, with the wind erosion rate of farmland soils exhibiting a decreasing trend as the no-tillage treatment duration increased. Agricultural soils affected by wind erosion can form a rough surface layer. When this occurs, the tillage treatment of the farmland can be a key factor influencing the further development of wind erosion. The NT treatment was observed to exert less damage to the surface soil. The surface stubble and crop straw mulch of NT may play a protective role on the surface, improving the surface aerodynamic roughness and weakening surface wind erosion (Asensio et al., 2015; Wang et al., 2019a). In addition, crop straw can effectively slow down soil evaporation, maintain soil moisture and increase the soil compactness, which is conducive to increasing the initial wind speed of eroded particles on the surface and strengthening the resistance of the soil surface to wind erosion (Shojaei et al., 2019; Xiao et al., 2021). However, the CT treatment requires frequent tilling of the surface layer of the farmland, which can create a ridge-like undulating terrain in the farmland and cause greater damage to the surface layer of the farmland soil. Thus, the CT treatment may increase the roughness of the soil surface, changing the airflow field structure and significantly affecting the magnitude and distribution of airflow-soil surface interaction forces (Yang et al., 2020; Carretta et al., 2021). Furthermore, the soil surface layer induced damage as the tillage process may have provided more erodible soil particles for wind erosion, promoting the occurrence of wind erosion on farmland and ultimately influencing the outcome of wind erosion on farmland (Labiadh, 2017; Pi et al., 2021). Thus, tillage treatment is a key anthropogenic influencing factor on wind erosion. The black soil region of Northeast China is an important commodity grain production base and its output is fundamental in ensuring national food security (Shen et al., 2020). As the tillage treatment is one of the most important factors affecting crop yield (Wang et al., 2019a), our research group also measured the annual output of the NT and CT treatments investigated in the current study. In particular, the yield from corn planted in 2018 under NT and CT was determined as 9,000 kg·hm−2 and 10,000 kg·hm−2, respectively. Although the crop yield of NT was lower than that of CT, which started in the year of 2007, the prevention effect of NT on wind erosion must be considered. Moreover, our research group also observed higher yields under NT compared to CT in the arable Mollisol region of Northeast China following 13 years of NT treatment (Guo et al., 2021). Therefore, NT can be potentially applied to enhance crop yield and effectively prevent wind erosion in the Mollisol region of Northeast China.
Zhang et al. (2019) determined wind erosion to be a result of a combination of factors. Our results suggest natural (wind speed and precipitation) and human (tillage management) factors to be the main influences behind wind erosion in arable land (Figure 6). We observed that the number of erodible particles on farmland increases with wind speed (e.g., 16th to 20th May in 2017 and 20th to 25th April in 2018) and decreases with increasing rainfall (e.g., 4th to 7th May in 2017 and 4th to 16th April in 2018). This has been confirmed in previous literature, whereby the amount of material accumulated each year by wind erosion was closely related to the wind speed and the rainfall amount in a given year (Pierre et al., 2018; de Oro et al., 2019; Pi et al., 2021). The soil particles begin to move when the wind speed is greater than the maximum threshold of frictional velocity between the soil particles and the surface of the farmland (Bergametti et al., 2016).
In addition to wind speed, differences in soil water content are also a major factor influencing wind erosion on agricultural soils. Our results reveal rainfall and soil moisture content to be maximized from 7 to 9 May in 2017 and from 19th to 21st April in 2018, corresponding to relatively lower levels of wind erosion (Figures 6b, Figures 6c). This agrees with the work of Uzun et al. (2017), who reported the force required to separate soil particles to be greater at higher soil moisture content under the action of water in the film between the soil particles. Under wet conditions, the tension between water molecules and soil particles in the soil increases the cohesion between the particles, and thus an increase in soil moisture content enhances the cohesion and agglomeration of the soil, which ultimately increases the starting wind speed of the soil (Yuge and Anan, 2019; Wang et al., 2021). Therefore, when soil moisture content is high, it is difficult for soil particles to move even if the wind reaches the starting wind speed of the soil particles.
In the current study, we measured the three transport modes of wind erosion (i.e., creep, saltation, and suspension) under NT and CT on the arable Mollisol region in Northeast China during the years 2016–2018. The results identify creep as the dominant transport mode of wind erosion in the study region, causing 81.15% and 84.07% of the entire wind erosion under the NT and CT treatments. The most erodible particles by saltation were collected at 0.5 m above the ground (when comparing heights from 0.2 to 2 m). These observations can help our team to implement future prevention programs for the wind erosion of the arable Mollisol region in Northeast China.
Our results also reveal wind erosion on agricultural land to be influenced by tillage practices, rainfall, wind speed, and soil moisture content. We found a significant (p ≤ 0.05) positive correlation between the total erodible particles collected by the sampler and both maximum and average wind speeds in the study area. Compared to the CT treatment, the NT treatment was more effective in reducing the erodible particles of creep and saltation in the surface soil of the cropland. This indicates the ability of mulching straw to effectively reduce wind erosion near arable soil surfaces. As the CT treatment lacked soil surface cover, wind erosion under the CT treatment was more susceptible to rainfall and soil moisture than that under the NT treatment. This may increase the occurrence of soil erosion on farmland.
This work concludes that the use of NT treatment can reduce soil loss by wind erosion on the farmland of Mollisols in Northeast China.
The original contributions presented in the study are included in the article/Supplementary Material; further inquiries can be directed to the corresponding author.
SC designed the field observation and wrote the manuscript. XZ and WH conducted statistical analysis. JL and MG performed the observation and collected data. All authors contributed to the writing—reviewing and editing.
This research was funded by the projects of the National Key Research and Development Program of China (Grant No. 2021YFD1500700), the National Science Foundation of China (Grant No. 42177312), the project of Scientific and Technological Re-search Program of Chongqing Municipal Education Commission (Grant No. KJQN20200837), the project of Scientific Research Foundation of Chongqing Technology and Business University (Grant No. 1956027), and Major Theoretical Research Projects in Philosophy and Social Sciences of Chongqing Education Commission (Grant No. 19SKZDZX10) and Major Projects of Key Research Base of Humanities and Social Sciences of the Ministry of Education (Grant No. 16JJD790064).
The authors would like to thank the National Key Research and Development Program of China, Scientific and Technological Research Program of the Chongqing Municipal Education Commission, and Chongqing Technology and Business University for funding this research. They also wish to thank the Scientific Data Center of Northeast Black Soil, National Earth System Science Data Sharing Infrastructure, National Science and Technology Infrastructure of China (http://northeast.geodata.cn), for supporting the meteorological data.
The authors declare that the research was conducted in the absence of any commercial or financial relationships that could be construed as a potential conflict of interest.
All claims expressed in this article are solely those of the authors and do not necessarily represent those of their affiliated organizations, or those of the publisher, the editors and the reviewers. Any product that may be evaluated in this article, or claim that may be made by its manufacturer, is not guaranteed or endorsed by the publisher.
Andreotti, B., Claudin, P., and Douady, S. (2002). Selection of dune shapes and velocities Part 1: Dynamics of sand, wind and barchans. Eur. Phys. J. B - Condens. Matter 28 (3), 321–339. doi:10.1140/epjb/e2002-00236-4
Asensio, C., Lozano, F. J., Ortega, E., and Kikvidze, Z. (2015). Study on the effectiveness of an agricultural technique based on aeolian deposition, in a semiarid environment. Environ. Eng. Manag. J. 14 (5), 1143–1150. doi:10.30638/eemj.2015.125
Avecilla, F., Panebianco, J. E., Buschiazzo, D. E., and de, O. (2018). A study on the fragmentation of saltating particles along the fetch distance during wind erosion. Aeolian Res. 35, 85–93. doi:10.1016/j.aeolia.2018.10.003
Bergametti, G., Rajot, J.-L., Pierre, C., Bouet, C., and Marticorena, B. (2016). How long does precipitation inhibit wind erosion in the Sahel? Geophys. Res. Lett. 43 (12), 6643–6649. doi:10.1002/2016gl069324
Carretta, L., Tarolli, P., Cardinali, A., Nasta, P., Romano, N., and Masin, R. (2021). Evaluation of runoff and soil erosion under conventional tillage and no-till management: A case study in Northeast Italy. Catena 197, 104972. doi:10.1016/j.catena.2020.104972
Chen, S., Burras, C. L., Lili, E., and Zhang, X. (2019a). Interrelationship among slope steepness, tillage practice and rainfall properties with surface runoff and soil loss on Mollisols in Northeast China. Arch. Agron. Soil Sci. 65 (13), 1860–1872. doi:10.1080/03650340.2019.1579310
Chen, S., Burras, C. L., and Zhang, X. (2019b). Soil aggregate response to three freeze-thaw methods in a northeastern China Mollisol. Pol. J. Environ. Stud. 28 (5), 3635–3645. doi:10.15244/pjoes/99205
Chen, S., Zhang, X., Hu, W., Yang, R., Li, H., and Zhai, X. (2019c). Measurement device of soil wind erosion on arable Mollisols regionChnia. Patent No 2018219787883. Beijing: China: China National Intellectual Property Administration.
Chen, Y., Liu, S., Li, H., Li, X., Song, C., Cruse, R., et al. (2011). Effects of conservation tillage on corn and soybean yield in the humid continental climate region of Northeast China. Soil Tillage Res. 115, 56–61. doi:10.1016/j.still.2011.06.007
Chi, W., Zhao, Y., Kuang, W., and He, H. (2019). Impacts of anthropogenic land use/cover changes on soil wind erosion in China. Sci. Total Environ. 668, 204–215. doi:10.1016/j.scitotenv.2019.03.015
Cornelis, W. M. (2006). Hydroclimatology of wind erosion in arid and semiarid envirmonments, Dryland ecohydrology. New York: Springer, 141–159.
de Oro, L. A., Colazo, J. C., Avecilla, F., Buschiazzo, D. E., and Asensio, C. (2019). Relative soil water content as a factor for wind erodibility in soils with different texture and aggregation. Aeolian Res. 37, 25–31. doi:10.1016/j.aeolia.2019.02.001
Fattahi, S. M., Soroush, A., and Huang, N. (2020). Biocementation control of sand against wind erosion. J. Geotech. Geoenviron. Eng. 146 (6), 04020045. doi:10.1061/(ASCE)GT.1943-5606.0002268
Feizi, Z., Ayoubi, S., Mosaddeghi, M. R., Besalatpour, A. A., Zeraatpisheh, M., and Rodrigo-Comino, J. (2019). A wind tunnel experiment to investigate the effect of polyvinyl acetate, biochar, and bentonite on wind erosion control. Arch. Agron. Soil Sci. 65 (8), 1049–1062. doi:10.1080/03650340.2018.1548765
Guo, M., Li, J., Li, J., and Zhang, X. (2021). Changes of soil structure and function after 16-year conservation tillage in black soil. Trans. Chin. Soc. Agric. Eng. 37 (22), 108–118. (In Chinese). doi:10.11975/j.issn.1002-6819.2021.22.012
Hong, C., Chenchen, L., Xueyong, Z., Huiru, L., Liqiang, K., Bo, L., et al. (2020). Wind erosion rate for vegetated soil cover: A prediction model based on surface shear strength. Catena 187, 104398. doi:10.1016/j.catena.2019.104398
Jarrah, M., Mayel, S., Tatarko, J., Funk, R., and Kuka, K. (2020). A review of wind erosion models: Data requirements, processes, and validity. Catena 187, 104388. doi:10.1016/j.catena.2019.104388
Jia, H., Wang, G., Guo, L., Zhuang, J., and Tang, L. (2015). Wind erosion control utilizing standing corn residue in Northeast China. Soil Tillage Res. 153, 112–119. doi:10.1016/j.still.2015.05.009
Jia, L., Zhao, W., Zhai, R., Liu, Y., Kang, M., and Zhang, X. (2019). Regional differences in the soil and water conservation efficiency of conservation tillage in China. Catena 175, 18–26. doi:10.1016/j.catena.2018.12.012
Kang, Y., He, Q., Yang, X., Yang, F., Ali, M., and Huo, W. (2017). Research on saltation and creeping laws of wind - blown currents based on field observations. J. Arid. Land Resour. Environ. 31 (5), 119–125. (In Chinese). doi:10.13448/j.cnki.Jalre.2017.154
Katra, I., Gross, A., Swet, N., Tanner, S., Krasnov, H., and Angert, A. (2016). Substantial dust loss of bioavailable phosphorus from agricultural soils. Sci. Rep. 6 (1), 24736–24737. doi:10.1038/srep24736
Kheirabadi, H., Mahmoodabadi, M., Jalali, V., and Naghavi, H. (2018). Sediment flux, wind erosion and net erosion influenced by soil bed length, wind velocity and aggregate size distribution. Geoderma 323, 22–30. doi:10.1016/j.geoderma.2018.02.042
Labiadh, M. (2017). Impact of ploughing techniques on wind erosion: Field experiments assessment, euro-mediterranean conference for environmental integration. New York: Springer, 1269–1270.
Li, W., and Zhou, Y. (2007). Statistical behaviors of different–sized grains lifting off in stochastic collisions between mixed sand grains and the bed in aeolian saltation. J. Geophys. Res. 112 (D22), D22106–D22456. doi:10.1029/2006JD007888
Li, Z., Feng, D., Wu, S., Borthwick, A. G. L., and Ni, J. R. (2008). Grain size and transport characteristics of non-uniform sand in aeolian saltation. Geomorphology 100 (3-4), 484–493. doi:10.1016/j.geomorph.2008.01.016
Liu, F., Hao, Y., Xin, Z., Chen, H., Xu, J., and Zhao, Y. (2014). The surface aeolian-sand flow structure in the noetheastern margin of the Ulanbuh Desert. J. Desert Res. 34 (5), 1200–1207. (In Chinese). doi:10.7522/j.issn.1000-694X.2014.00096
Liu, L., and Bo, T.-L. (2019). A study on the initiation of saltation in the model of wind-blown sand transport considering the effect of turbulence. Granul. Matter 21 (3), 78–15. doi:10.1007/s10035-019-0934-2
Liu, S., Zhang, X.-Y., Kravchenko, Y., and Iqbal, M. A. (2015). Maize (Zea mays L.) yield and soil properties as affected by no tillage in the black soils of China. Acta Agric. Scand. Sect. B —. Soil & Plant Sci. 65 (6), 554–565. doi:10.1080/09064710.2015.1036304
Liu, X., Li, H., Zhang, S., Cruse, R. M., and Zhang, X. (2019). Gully erosion control practices in Northeast China: A review. Sustainability 11 (18), 5065. doi:10.3390/su11185065
Lyu, X., Li, X., Wang, H., Gong, J., Li, S., Dou, H., et al. (2021). Soil wind erosion evaluation and sustainable management of typical steppe in Inner Mongolia, China. J. Environ. Manage. 277, 111488. doi:10.1016/j.jenvman.2020.111488
Martin, R. L., and Kok, J. F. (2017). Wind-invariant saltation heights imply linear scaling of aeolian saltation flux with shear stress. Sci. Adv. 3 (6), e1602569–10. doi:10.1126/sciadv.1602569
Mozaffari, H., Rezaei, M., and Ostovari, Y. (2021). Soil sensitivity to wind and water erosion as affected by land use in southern Iran. Earth 2 (2), 287–302. doi:10.3390/earth2020017
Pähtz, T., Clark, A. H., Valyrakis, M., and Durán, O. (2020). The physics of sediment transport initiation, cessation, and entrainment across aeolian and fluvial environments. Rev. Geophys. 58 (1), e2019RG000679. doi:10.1029/2019RG000679
Pi, H., Webb, N. P., Huggins, D. R., and Sharratt, B. (2021). Influence of physical crust cover on the wind erodibility of soils in the inland Pacific Northwest, USA. Earth Surf. Process. Landf. 46 (8), 1445–1457. doi:10.1002/esp.5113
Pierre, C., Kergoat, L., Hiernaux, P., Baron, C., Bergametti, G., Rajot, J. L., et al. (2018). Impact of agropastoral management on wind erosion in Sahelian croplands. Land Degrad. Dev. 29 (3), 800–811. doi:10.1002/ldr.2783
Sekaran, U., Sagar, K. L., and Kumar, S. (2021). Soil aggregates, aggregate-associated carbon and nitrogen, and water retention as influenced by short and long-term no-till systems. Soil Tillage Res. 104885, 104885. doi:10.1016/j.still.2020.104885
Shahabinejad, N., Mahmoodabadi, M., Jalalian, A., and Chavoshi, E. (2019). In situ field measurement of wind erosion and threshold velocity in relation to soil properties in arid and semiarid environments. Environ. Earth Sci. 78 (16), 501–522. doi:10.1007/s12665-019-8508-5
Shao, Y. (2001). A model for mineral dust emission. J. Geophys. Res. 106 (D17), 20239–20254. doi:10.1029/2001JD900171
Sharratt, B. S., Kennedy, A. C., Hansen, J. C., and Schillinger, W. F. (2018). Soil carbon loss by wind erosion of summer fallow fields in Washington's dryland wheat region. Soil Sci. Soc. Am. J. 82 (6), 1551–1558. doi:10.2136/sssaj2018.06.0214
Shen, H., Wang, D., Wen, L., Zhao, W., and Zhang, Y. (2020). Soil erosion and typical soil and water conservation measures on hillslopes in the Chinese Mollisol region. Eurasian Soil Sc. 53 (10), 1509–1519. doi:10.1134/S1064229320100178
Shojaei, S., Ardakani, M. A. H., Sodaiezadeh, H., jafari, M., and afzali, S. f. (2019). Optimization of parameters affecting organic mulch test to control erosion. J. Environ. Manage. 249, 109414. doi:10.1016/j.jenvman.2019.109414
Soil Survey Staff (2014). Keys to soil Taxonomy. 12th ed. Washington, DC: USDA-Natural Resources Conservation Service.
Swann, C., Sherman, D., and Ewing, R. (2020). Experimentally derived thresholds for windblown sand on Mars. Geophys. Res. Lett. 47 (3), e2019GL084484. doi:10.1029/2019GL084484
Tan, L., Zhang, W., Qu, J., Du, J., Yin, D., and An, Z. (2014). Variation with height of aeolian mass flux density and grain size distribution over natural surface covered with coarse grains: A mobile wind tunnel study. Aeolian Res. 15, 345–352. doi:10.1016/j.aeolia.2014.06.008
Uzun, O., Kaplan, S., Basaran, M., Deviren Saygın, S., Youssef, F., Nouri, A., et al. (2017). Spatial distribution of wind-driven sediment transport rate in a fallow plot in Central Anatolia, Turkey. Arid Land Res. Manag. 31 (2), 125–139. doi:10.1080/15324982.2016.1276487
Uzun, O., Kaplan, S., Ince, K., Basaran, M., and Erpul, G. (2020). Spatially and temporally assessing event-based wind erosion in adjacent plots of fallow and wheat cultivation in the Central Anatolia, Turkey. Arch. Agron. Soil Sci. 1-15, 661–675. doi:10.1080/03650340.2020.1849624
Wang, K., and Wang, H. (2022). Integrated cost dataset under the whole life cycle of biogas, straw and coal power generation. J. Glob. Change Data Discov. 1, 53–57. doi:10.3974/geodp.2022.01.07
Wang, X., Fan, J., Xing, Y., Xu, G., Wang, H., Deng, J., et al. (2019a). The effects of mulch and nitrogen fertilizer on the soil environment of crop plants. Adv. Agron. 153, 121–173. doi:10.1016/bs.agron.2018.08.003
Wang, X., Li, S., Sun, Y., Zhang, C., and Liu, G. (2021). Influence of freeze–thaw cycling on the soil mechanical properties of open-pit mine dump under different moisture contents. Environ. Earth Sci. 80 (7), 1–10. doi:10.1007/s12665-021-09592-9
Wang, X., Qi, J.-Y., Zhang, X.-Z., Li, S.-S., Virk, A. L., Zhao, X., et al. (2019b). Effects of tillage and residue management on soil aggregates and associated carbon storage in a double paddy cropping system. Soil Tillage Res. 194, 104339. doi:10.1016/j.still.2019.104339
Wassif, O. M., Meselhy, A. A., Sharkawy, S. F., and Ali, A. A. (2020). Quantify impact of wind erosion on organic matter content under management practices, Wadi El Raml, NWCZ, Egypt. Egypt. Egypt. J. Desert Res. 70 (1), 83–102. doi:10.21608/EJDR.2020.33688.1073
Xiao, L., Li, G., Zhao, R., and Zhang, L. (2021). Effects of soil conservation measures on wind erosion control in China: A synthesis. Sci. Total Environ. 778, 146308. doi:10.1016/j.scitotenv.2021.146308
Xie, Y., Lin, H., Ye, Y., and Ren, X. (2019). Changes in soil erosion in cropland in northeastern China over the past 300 years. Catena 176, 410–418. doi:10.1016/j.catena.2019.01.026
Xu, X., Zheng, F., Wilson, G., He, C., Lu, J., and Bian, F. (2018). Comparison of runoff and soil loss in different tillage systems in the Mollisol region of Northeast China. Soil Tillage Res. 177, 1–11. doi:10.1016/j.still.2017.10.005
Yang, C., Geng, Y., Fu, X. Z., Coulter, J. A., and Chai, Q. (2020). The effects of wind erosion depending on cropping system and tillage method in a semi-arid region. Agron. (Basel). 10 (5), 732. doi:10.3390/agronomy10050732
Yang, R. (2018). Monitoring study on wind erosion in hailun of the typical Mollisols farmland in Northeast China. China: Northeast Agricultural University. [master’s thesis]. [Harbin (Hei Longjiang)](In Chinese).
Yang, Y., Liu, L., Li, X., Shi, P., Zhang, G., Xiong, Y., et al. (2019). Aerodynamic grain‐size distribution of blown sand. Sedimentology 66 (2), 590–603. doi:10.1111/sed.12497
Yao, Q., Liu, J., Yu, Z., Li, Y., Jin, J., Liu, X., et al. (2017). Three years of biochar amendment alters soil physiochemical properties and fungal community composition in a black soil of northeast China. Soil Biol. Biochem. 110, 56–67. doi:10.1016/j.soilbio.2017.03.005
Yildiz, Ş., Enc, V., Kara, M., Tabak, Y., and Acet, E. (2017). Assessment of the potential risks of airbone microbial contamination in solid recovered fuel plants: A case study in istanbul. Environ. Eng. Manag. J. 16 (7), 1415–1421. doi:10.30638/eemj.2017.154
Yuge, K., and Anan, M. (2019). Evaluation of the effect of wind velocity and soil moisture condition on soil erosion in andosol agricultural fields (Model experiment). Water 11 (1), 98. doi:10.3390/w11010098
Zhang, H., Gao, Y., Sun, D., Liu, L., Cui, Y., and Zhu, W. (2019). Wind erosion changes in a semi-arid sandy area, Inner Mongolia, China. Sustainability 11 (1), 188. doi:10.3390/su11010188
Zhang, P., Sherman, D. J., and Li, B. (2021). Aeolian creep transport: A review. Aeolian Res. 51, 100711. doi:10.1016/j.aeolia.2021.100711
Keywords: tillage treatment, wind erosion, interrelationship, arable, Mollisols
Citation: Chen S, Zhang X, Li J, Guo M and Hu W (2022) Effect of tillage management on the wind erosion of arable soil in the Chinese Mollisol region. Front. Environ. Sci. 10:954004. doi: 10.3389/fenvs.2022.954004
Received: 26 May 2022; Accepted: 08 August 2022;
Published: 02 September 2022.
Edited by:
Huawei Pi, Henan University, ChinaReviewed by:
Worradorn Phairuang, Kanazawa University, JapanCopyright © 2022 Chen, Zhang, Li, Guo and Hu. This is an open-access article distributed under the terms of the Creative Commons Attribution License (CC BY). The use, distribution or reproduction in other forums is permitted, provided the original author(s) and the copyright owner(s) are credited and that the original publication in this journal is cited, in accordance with accepted academic practice. No use, distribution or reproduction is permitted which does not comply with these terms.
*Correspondence: Wei Hu, d2VpaHU5ODdAMTYzLmNvbQ==
Disclaimer: All claims expressed in this article are solely those of the authors and do not necessarily represent those of their affiliated organizations, or those of the publisher, the editors and the reviewers. Any product that may be evaluated in this article or claim that may be made by its manufacturer is not guaranteed or endorsed by the publisher.
Research integrity at Frontiers
Learn more about the work of our research integrity team to safeguard the quality of each article we publish.