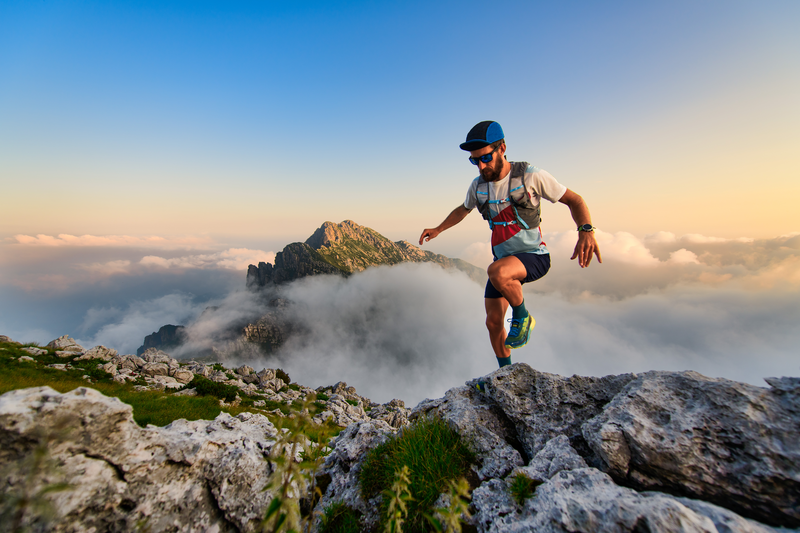
95% of researchers rate our articles as excellent or good
Learn more about the work of our research integrity team to safeguard the quality of each article we publish.
Find out more
ORIGINAL RESEARCH article
Front. Environ. Sci. , 08 August 2022
Sec. Soil Processes
Volume 10 - 2022 | https://doi.org/10.3389/fenvs.2022.953579
This article is part of the Research Topic Better Management of Phosphorus Fertilizer in Intensive Cropping Systems: An Approach Basing on Integrated Agronomic, Ecological and Environmental Compromises View all 11 articles
It is possible to simultaneously reduce both food security and environmental impact by understanding the relation between rice (Oryza sativa L.) grain yield and phosphorus (P) uptake requirements. The goal of this study was to determine P uptake requirements and relationship of P accumulation with yield formation at different rice grain yield levels under saline-sodic stress. A database comprising measurements in 28 plots in four on-farm research station located in saline-sodic soil area during the period 2018–2019 in Jilin province of Northeast China was used for the analyses. The grain yields of rice averaged 9.0 Mg ha−1 and varied from 5.11 to 13.41 Mg ha−1. The P uptake at late growth stages (heading and maturity) of rice gradually increased with increasing grain yield levels. The P requirement for producing 1 Mg grain (Preq.) were 4.61, 4.60, and 4.21 kg Mg−1 for grain yields ranging from <7.0, 8.0–9.5, and >10.0 Mg ha−1, respectively. The decrease in Preq. values with increasing grain yield was mainly attributable to the increase in the harvest index from 0.25 to 0.33. A larger proportion of the P was accumulated from heading to maturity stage when grain yields were higher than 8.0 Mg ha−1. The P uptake in leaves, stems and panicles at the maturity stage gradually increased with increasing grain yield levels. The results give a contribution to rice production in saline-sodic soils, and greatly optimize P management especially in high-yielding rice systems, furtherly improving food security in the Jilin province of China.
Food production needs to increase 100% by 2050 to meet projected food demands (Pastor et al., 2019). Marginal lands have received an increased attention for world food crisis, especially in China, which has a large population and relatively limited cultivated land. Saline-sodic soils are the typical marginal land, which have a serious adverse impacts on agricultural productivity and sustainability in arid and semi-arid climates (Qadir et al., 2007; Chi et al., 2012; Huang et al., 2017). Rice-planting could potentially reduce the adverse effects of these soil degradation, and has been recommended as the preferred method of biological improvement for saline-sodic soils (Chi et al., 2012; Huang et al., 2016; Huang et al., 2017). So far, the area of saline-sodic paddy fields in the Western Songnen Plain of China had reached 1.1 million ha, accounting for 3.67% of China’s total rice area (Huang et al., 2016). However, the yield of rice under saline-sodic stress responses to nutrient supply are not well understood. Especially about phosphorus (P), which as an essential macronutrient plays an important role for plant growth (Wu et al., 2015; Dai et al., 2022; Javed et al., 2022). Under saline-sodic conditions, the soil organic matter is low (Huang et al., 2016), and soils contain extremely high ratios of Na+/Ca2+, Na+/K+, Ca2+/Mg2+, and Cl−/NO3− (Huang et al., 2017), the Olsen-P is easily immobilized by Ca2+, and competes with Na+, Cl−, CO32-, and other salt ions (Hu and Schmidhalter, 2010; Tian et al., 2016). The high pH conditions of saline-sodic soils generally result in the reduction of phosphorus absorption (Tian et al., 2016). The availability of P to the plant is limited by insoluble state of tricalcium phosphate in saline-sodic soils (Saito et al., 2019; Guo et al., 2020), so the P use efficiency (PUE) in saline-sodic soils are very low. P has become the main limiting factor affecting the growth and yield of rice under saline-sodic stress (Guo et al., 2020).
To improve the crop yields and maintain soil fertility, phosphate fertilizers are regularly recommended based on target yields and soil test values (Zhang et al., 2008; Zhang et al., 2015). The typical P fertilization rate applied for rice paddy in Northeastern China is about 40–70 kg P2O5 ha−1 under a agronomist’s recommendation (Zhang et al., 2010). But, most farmers and even agricultural extension staff still blindly apply P fertilizer. Applying excess P fertilizer beyond crop needs increases P accumulation in soil and the risk of P loss, which leads to serious environmental problems, like eutrophication of water bodies (Conley et al., 2009; Bai et al., 2013). The consequences of these soil and water problems are meaningful on a global scale. Therefore, understanding the yield formation of rice under saline-sodic stress from the aspect of P uptake requirements, and improving PUE while increasing crop productivity and reducing environmental risk are very important for efficient use of marginal land and food security (Li et al., 2011; Zhang et al., 2012; Tian et al., 2017).
Grain yield of rice are associated with P concentrations in grains. Previous studies have reported differences in the rice grain yield -P uptake relationships over regions (environments), genotypes, irrigation, and nutrient (nitrogen and phosphorus) management strategies (Dobermann et al., 1996; Vandamme et al., 2016; Wang et al., 2017; Wei et al., 2017; Song et al., 2019; Guo et al., 2020). For example, Dobermann et al. (1996) reported that uptake of 1.8–4.2 kg P was required to produce one ton of grain yield by using the data from long-term experiments at 11 sites in five countries of Asia. Wei et al. (2017) found that the higher yield produced by japonica/indica hybrid of “super rice” was accompanied by a higher total P accumulation, and the grain yield was positively correlated with P accumulation from stem elongation to heading stage of rice. Wang et al. (2017) reported low straw P concentrations improved grain yield by enhancing P translocation into grain. In addition, the yield of rice in saline-alkaline soils was positively correlated with P accumulation and rates of nutrient mobilization to the leaves, stem-sheaths, and panicles at maturity (Guo et al., 2020).
Many studies have provided information about P requirements and the relationships with grain yield of rice, but quantitative physiological P requirements in terms of yield relationships across a wide range of farming environments under saline-sodic stress are very few. In this study, we collected data in 28 plots of saline-sodic paddy field from 2018 to 2019 in Jilin province of Northeastern China. The objectives of this study were to investigate P uptake during main growth periods and relationship of P accumulation with grain yield, quantify the P requirement per ton grain produced for different yield levels, explore the key factors affecting the formation of rice yield under saline-sodic stress.
The data were collected from on-farm research station experiments conducted in four locations in the saline-sodic soil area during 2018–2019 in Jilin province (41°–46°N, 121°–130°E), Northeastern China. Measurements were conducted in 28 plots of saline-sodic paddy field in total. Specific detailed information of these on-farm studies are shown in Table 1. Rice was transplanted in early May and harvested in early October. Annual precipitation in this area is 500–800 mm, with 60–70% of the rainfall occurring during the rice growth periods. Fertilizer were recommended by agronomists and were based on targeted yield and soil fertility. All experimental fields received phosphorus applications that ranged from 50 to 130 kg P2O5 ha−1, nitrogen application rates range from 97 to 278 kg N ha−1 and potassium application rates range from 52 to 129 kg K2O ha−1. Plant densities ranged from 200,000 to 300,000 plants ha−1. Commercial rice varieties with high yield potential that were considered suitable at each site were used. All experiments were managed according to the individual farmers’ practices, and no manure was applied. Insects were intensively controlled using chemicals to avoid biomass and yield losses.
At the rice tillering, stem elongation, heading and maturity stages, ten consecutive plants of each plot were sampled to determine the aboveground total dry matter (DM) and phosphorus (P) content. These plants were separated into leaves, stems (culm and sheath) at four stages, and panicles at the heading and maturity stages. All plant samples were oven-dried at 105°C for 0.5 h and then dried at 80°C until a constant weight was reached for biomass measurements. After weighing, the samples were ground to fine powder (0.5 mm sieve), and the P concentrations (mg g−1) of each sample were determined by the molybdate-blue colorimetric method (Murphy and Riley, 1962). The P accumulation (kg ha−1) were calculated by multiplying the P concentration by the aboveground total dry weight.
At the maturity stage, plants covering an area of 1 m2 (excluding the border rows) in each plot were collected to determine the grain yield components, includes number of panicles per hectare, the number of spikelets per panicle, filled-grain percentage (%) and 103-grain weight (g). The percentage of filled grains was calculated in the ratio of filled grains (specific gravity ≥1.06 g cm−3) to the total number of spikelets (Wei et al., 2017). All plants in each plot were harvested and grain yield was reported with 14% moisture content.
The Preq. (kg Mg−1 grain) was defined as the amount of aboveground P needed to produce 1 Mg grain. The harvest index (HI) was determined by dividing the grain yield by the total aboveground plant dry matter. The partial factor productivity (PFPp, kg kg−1) was calculated as the grain yield divided by the amount of P fertilizer applied. The P harvest index (PHI) was calculated as the ratio of the grain P accumulation to the total P accumulation of the aboveground parts at the maturity stage (Tang et al., 2011; Wu et al., 2015; Wang et al., 2017).
According to the rice yield of Jilin province of China in recent 5 years, the data of grain yields were split into three groups: <7.0, 8.0–9.5, and >10.0 Mg ha−1. Experiments with P accumulation data collected at four stages, and grain yield components at the maturity stage were also grouped on the basis of grain yield (<7.0, 8.0–9.5, and >10.0 Mg ha−1). A one-way ANOVA was used to compare the means of P accumulation, grain yield components and yield levels based on the least significant difference at a 0.05 level of probability with SPSS 18.0. And the relationship between yields and P accumulation were calculated using Pearson correlation coefficient.
Considering all 28 observations, the average rice grain yields of <7.0, 8.0–9.5, and >10.0 Mg ha−1 were 6.3 ± 0.5, 8.7 ± 0.4 and 11.4 ± 1.4 (Table 2). The number of panicles per hectare and the number of spikelets per panicle gradually increased with increasing grain yield levels, and the yields of 8.0–9.5, and >10.0 Mg ha−1 were significantly higher than the yield of <7.0 Mg ha−1. The filled-grain percentages remained stable at ∼96.0% among the three yield levels. However, the 103-grain weight decreased with increasing grain yield levels. Overall, there was a significant positive correlation between rice yield and the number of spikelets per panicle (Table 2).
For total DM accumulation, the greatest difference among the three yield ranges was observed at maturity stage (Table 3). The total DM gradually increased with increasing grain yield levels at the heading and maturity stages, which caused P uptake to increase with an increase in grain yield (Figure 1). The yield level of >10.0 Mg ha−1 showed higher P uptake at heading stage (39.5 kg ha−1) and maturity stage (64.5 kg ha−1) compared with the yields of <7.0, and 8.0–9.5 Mg ha−1. The P uptake at late growth stages of rice gradually increased with increasing grain yield levels (Figure 1).
FIGURE 1. Aboveground P uptake at main growth stages of rice with different yield levels. Different letters above the bars are significantly different for different yield levels (p < 0.05). Yield level: <7.0, 8.0–9.5, and >10.0 Mg ha−1.
The Preq. were decreased with increase in grain yield, average Preq. values were 4.61, 4.60, and 4.21 kg Mg−1 for grain yields ranging from <7.0, 8.0–9.5, and >10 Mg ha−1, respectively (Table 4). The increase in HI also contributed to the decrease in Preq. with increasing yield levels. Average PHI around 0.75 for all of the yield levels. The PFPp were 125.4 kg kg−1, 109.5 kg kg−1 and 126.5 kg kg−1 as yield increased from <7.0 Mg ha−1 to 8.0–9.5 Mg ha−1 to >10.0 Mg ha−1, respectively (Table 4).
TABLE 4. The P requirement (Preq.), harvest index (HI), P harvest index (PHI), and partial factor productivity (PFPp) of rice with different yield levels.
To better understand the P accumulation and partitioning pattern of rice under saline-sodic stress, P accumulation during main growth periods (Table 5) and P uptake in each organ at the maturity stage (Figure 2) were calculated. From tillering to stem elongation stage, the P accumulation rate in the yield of <7.0 Mg ha−1was highest (58.1%), followed by the yield of >10.0, and 8.0–9.5 Mg ha−1. From stem elongation to heading stage, the P accumulation rate increased from 9.2% to 19.7% to 21.3% as yield increased from <7.0 Mg ha−1 to 8.0–9.5 Mg ha−1 to >10.0 Mg ha−1, respectively, while the same trend was observed from heading stage to maturity stage. The yield level of >10.0, and 8.0–9.5 Mg ha−1 had the highest P accumulation rate from heading stage to maturity stage, which were 40.3 and 54.5%, respectively (Table 5).
FIGURE 2. P accumulation in each organ at the maturity stages of rice with different yield levels. Different letters above the bars are significantly different for different yield levels (p < 0.05). Yield level: <7.0, 8.0–9.5, and >10.0 Mg ha−1.
At the maturity stage, leaves dry matter in the yield of <7.0 Mg ha−1was highest, followed by the yield of 8.0–9.5, and >10.0 Mg ha−1 (Table 6). Dry matter of stems and panicles gradually increased with increasing grain yield levels. For the P concentration, grain yield levels did not show the difference on leaf, stem, and panicle P concentration at the heading stage. At the maturity stage, the P concentration in leaves, stems, and panicles also increased with increasing grain yield levels, but there was no significantly different among the three yield levels (Table 6). A considerable amount of stem and leaf P was translocated to the grain in the yield of >10.0 Mg ha−1 (Table 6). For the P uptake in each organ at the maturity stage, the P uptake in leaves, stems and panicles gradually increased with increasing grain yield levels. There was no significant difference among the three yield levels in leaves, while was significantly different between the yield of >10.0 Mg ha−1 and <7.0 Mg ha−1 in stems and panicles (Figure 2).
TABLE 6. The total dry matter (DM) and P concentration in each organ at the maturity stages of rice with different yield levels.
Overall, the relationship between aboveground P accumulation and grain yield of rice under saline-sodic stress were shown in Figure 3. The grain yield was significantly and positively affected by the P accumulation from stem elongation to heading stage, and P uptake in panicle at the maturity stage (P < 0.01).
FIGURE 3. Pearson correlation between aboveground P accumulation and grain yield of rice (n = 28). * and ** indicate significance at P < 0.05 and P < 0.01, respectively. TE-P, P accumulation from tillering to stem elongation stage; EH-P, P accumulation from stem elongation to heading stage; HM-P, P accumulation from heading to maturity stage; ML-P, P uptake in leaf at maturity stage; MS-P, P uptake in stem at maturity stage; MP-P, P uptake in panicle at maturity stage.
Among yield components, panicle and spikelet number was the basis of stable high yield of rice (Sheehy et al., 2001; Yan et al., 2010; Guo et al., 2020; Li et al., 2021). Our results showed that the number of panicles per hectare and spikelets per panicle were significantly increased with increasing grain yield levels (Table 2). Generally, the number of spikelets per panicle was negatively correlated with the filled-grain percentage generally (Mohapatra and Sahu, 1991). But in the present study, there was no significant difference in filled-grain percentage among the three yield levels (Table 2), which probably means that the high-yielding rice in our study can achieve the synchronous improvement of spikelet number and filled-grain percentage.
Soil salinity and sodicity can affect the absorption of essential nutrients, and cause nutritional disorders and reduction of grain yield. Especially about P, which is easily immobilized by Ca2+, and competes with salt ions (Huang et al., 2017). In addition, the organic matter (OM) in soils are able to rapidly sorb applied P fertilizer and improved P availability of plants (Guppy et al., 2005). However, OM in saline-sodic soils (6–8 g kg−1) were lower than that of conventional paddy field (>20 g kg−1) (Huang et al., 2016; Wei et al., 2017; Song et al., 2019). In practice, to maximize grain yield of rice in saline-sodic soils, the application of P fertilizer was higher than that of conventional paddy field, so the PUE in saline-sodic soils are very low. In the present study, the yield level of >10.0 Mg ha−1 showed higher total DM and P uptake at late growth stages of rice compared with other two yield levels (Table 3 and Figure 1). A similar observation of “super rice” was also reported by Wei et al. (2016). Our results showed that Preq. of rice decreased as grain yield improved (Table 4), and the Preq. value in this study (4.2–4.6 kg Mg−1) was higher than the 1.8–4.2 kg Mg−1 reported by Dobermann et al. (1996), based on the data from long-term experiments of rice in Asia. Moreover, some similar trend about Preq. of maize (Zea mays L.) was reported by Wu et al. (2015), and decreased Preq. as grain yield improved means that improvements in grain yield do not require proportional increases in the use of chemical P fertilizers. Therefore, the Preq. of rice as affected by grain yield level should be of consideration in appropriate P fertilizer recommendation for simultaneously reducing cost and P losses of rice in saline-sodic soils.
Rice HI have been documented in several previous studies, which range of 0.17–0.56 (Bueno and Lafarge, 2009; Ju et al., 2009; Yang and Zhang, 2010). In the present study, HI (0.25–0.33) increased when the yield range increased (Table 4). An improvement of HI means an increase in the economic portion of the plant, and rice HI is the result of various integrated processes, including the number of panicles per unit area, the number of spikelets per panicle, filled-grain percentage, and 103-grain weight (Li et al., 2012). The PHI (0.72–0.78) in this study was consistent with the results of 0.73–0.83 (indica rice) reported by Song et al. (2019), and higher than the 0.50–0.68 of two indica rice reported by Wang et al. (2017). Our results showed that the HI increased as yield increased, but PHI has no significant difference (Table 4), which probably related with the greater increasing in the allocation of carbohydrates (Wu et al., 2015).
P accumulation during main growth periods and the relationship of P accumulation with grain yield have been documented in several previous studies (Rose et al., 2010; Bi et al., 2013; Wang et al., 2017; Wei et al., 2017; Guo et al., 2020). 60–90% of P taken up by the rice is typically accumulated in the grains at maturity (Rose et al., 2010; Bi et al., 2013). Wei et al. (2017) found that the grain yield of “super rice” was positively correlated with P accumulation from stem elongation to heading stage of rice. Wang et al. (2017) reported that low straw P concentrations improved grain yield by enhancing P translocation into grain. In saline-alkaline soils, there was a positively correlation between grain yield with P accumulation and rates of nutrient mobilization to the leaves, stem-sheaths, and panicles at maturity were reported by Guo et al. (2020). In this study, a larger proportion of the P (40.3%, 54.5%) was accumulated from heading to maturity stage when grain yields were higher than 8.0 Mg ha−1 (Table 5). The P uptake in leaves, stems and panicles at the maturity stage gradually increased with increasing grain yield levels (Figure 2). A considerable amount of stem and leaf P was translocated to the grain in the yield of >10.0 Mg ha−1 (Table 6). Moreover, We found that the grain yield was positively affected by the P accumulation from stem elongation to heading stage, and P uptake in panicle at the maturity stage (Figure 3), which was consistent with previous studies (Wang et al., 2017; Wei et al., 2017; Guo et al., 2020). This results highlights the importance of maintaining P accumulation at late growth stages in high-yielding rice system under saline-sodic stress.
In summary, from the data analysis, the P uptake at late growth stages of rice were gradually increased with increasing grain yield levels. The Preq. were decreased with increase in grain yield. The grain yield was significantly and positively affected by the P accumulation from stem elongation to heading stage, and P uptake in panicle at the maturity stage. The knowledge with relationship between P requirement and grain yield can play a vital role in increasing rice yield in saline-sodic soils and in optimizing P management especially in high-yielding rice system.
The original contributions presented in the study are included in the article/supplementary material, further inquiries can be directed to the corresponding author.
NC and ZW designed the research and supervised the project. YZ, ZL, TW, and MP were key players for the field trials and collected data. ZL and TW analyzed the data and verified the analytical methods. YZ, ZW, and NC wrote the manuscript.
This study was funded by the Science and Technology Planning Project of Qinghai Province (Grant No. 2019-ZJ-7063), National Key Research and Development Program of China (2017YFD0300608-2).
The authors declare that the research was conducted in the absence of any commercial or financial relationships that could be construed as a potential conflict of interest.
All claims expressed in this article are solely those of the authors and do not necessarily represent those of their affiliated organizations, or those of the publisher, the editors and the reviewers. Any product that may be evaluated in this article, or claim that may be made by its manufacturer, is not guaranteed or endorsed by the publisher.
Bai, Z., Li, H., Yang, X., Zhou, B., Shi, X., Wang, B., et al. (2013). The critical soil P levels for crop yield, soil fertility and environmental safety in different soil types. Plant Soil 372, 27–37. doi:10.1007/s11104-013-1696-y
Bi, J., Liu, Z., Lin, Z., Alim, M. A., Ding, Y., Li, G., et al. (2013). Phosphorus accumulation in grains of japonica rice as affected by nitrogen fertilizer. Plant Soil 369 (1-2), 231–240. doi:10.1007/s11104-012-1561-4
Bueno, C. S., and Lafarge, T. (2009). Higher crop performance of rice hybrids than of elite inbreds in the tropics: 1. Hybrids accumulate more biomass during each phenological phase. Field Crops Res. 112 (2-3), 229–237. doi:10.1016/j.fcr.2009.03.006
Chi, C., Zhao, C., Sun, X., and Wang, Z. (2012). Reclamation of saline-sodic soil properties and improvement of rice (Oriza sativa L.) growth and yield using desulfurized gypsum in the west of Songnen Plain, northeast China. Geoderma 187-188, 24–30. doi:10.1016/j.geoderma.2012.04.005
Conley, D. J., Paerl, H. W., Howarth, R. W., Boesch, D. F., Seitzinger, S. P., Havens, K. E., et al. (2009). Controlling eutrophication: Nitrogen and phosphorus. Science 323 (5917), 1014–1015. doi:10.1126/science.1167755
Dai, C., Dai, X., Qu, H., Men, Q., Liu, J., Yu, L., et al. (2022). The rice phosphate transporter OsPHT1;7 plays a dual role in phosphorus redistribution and anther development. Plant Physiol. 188 (4), 2272–2288. doi:10.1093/plphys/kiac030
Dobermann, A., Cassman, K. G., Cruz, P., Adviento, M., and Pampolino, M. F. (1996). Fertilizer inputs, nutrient balance and soil nutrient supplying power in intensive, irrigated rice system. III. Phosphorus. Nutr. Cycl. Agroecosyst. 46 (2), 111–125. doi:10.1007/BF00704311
Guo, X., Jiang, H., Lan, Y., Wang, H., Lv, Y., Yin, D., et al. (2020). Optimization of nitrogen fertilizer management for improving rice grain yield and nutrient accumulation and mobilization in saline-alkaline soils. Crop Sci. 60, 2621–2632. doi:10.1002/csc2.20169
Guppy, C. N., Menzies, N. W., Moody, P. W., and Blamey, F. (2005). Competitive sorption reactions between phosphorus and organic matter in soil: A review. Soil Res. 43 (2), 189. doi:10.1071/SR04049
Hu, Y., and Schmidhalter, U. (2010). Drought and salinity: A comparison of their effects on mineral nutrition of plants. J. Plant Nutr. Soil Sci. 168 (4), 541–549. doi:10.1002/jpln.200420516
Huang, L. H., Liang, Z. W., Suarez, D. L., Wang, Z. C., Wang, M. M., Yang, H. Y., et al. (2016). Impact of cultivation year, nitrogen fertilization rate and irrigation water quality on soil salinity and soil nitrogen in saline-sodic paddy fields in Northeast China. J. Agric. Sci. 154 (04), 632–646. doi:10.1017/S002185961500057X
Huang, L., Liu, X., Wang, Z., Liang, Z., Wang, M., Liu, M., et al. (2017). Interactive effects of pH, EC and nitrogen on yields and nutrient absorption of rice (Oryza sativa L.). Agric. Water Manag. 194, 48–57. doi:10.1016/j.agwat.2017.08.012
Javed, T., I, I., Singhal, R. K., Shabbir, R., Shah, A. N., Kumar, P., et al. (2022). Recent advances in agronomic and physio-molecular approaches for improving nitrogen use efficiency in crop plants. Front. Plant Sci. 13. doi:10.3389/fpls.2022.877544
Ju, J., Yamamoto, Y., Wang, Y., Shan, Y., Dong, G., Miyazaki, A., et al. (2009). Genotypic differences in dry matter accumulation, nitrogen use efficiency and harvest index in recombinant inbred lines of rice under hydroponic culture. Plant Prod. Sci. 12 (2), 208–216. doi:10.1626/pps.12.208
Li, H., Huang, G., Meng, Q., Ma, L., Yuan, L., Wang, F., et al. (2011). Integrated soil and plant phosphorus management for crop and environment in China. A review. Plant Soil 349 (1-2), 157–167. doi:10.1007/s11104-011-0909-5
Li, X., Cao, J., Huang, J., Xing, D., and Peng, S. (2021). Effects of topsoil removal on nitrogen uptake, biomass accumulation, and yield formation in puddled-transplanted rice. Field Crops Res. 265, 108130. doi:10.1016/j.fcr.2021.108130
Li, X., Yan, W., Agrama, H., Jia, L., Jackson, A., Moldenhauer, K., et al. (2012). Unraveling the complex trait of harvest index with association mapping in rice (oryza sativa L.). Plos One 7 (1), e29350. doi:10.1371/journal.pone.0029350
Mohapatra, P. K., and Sahu, S. K. (1991). Heterogeneity of primary branch development and spikelet survival in rice panicle in relation to assimilates of primary branches. J. Exp. Bot. 42 (7), 871–879. doi:10.1093/jxb/42.7.871
Murphy, J., and Riley, J. P. (1962). A modified single solution method for the determination of phosphate in natural waters. Anal. Chim. Acta X. 27 (C), 31–36. doi:10.1016/S0003-2670(00)88444-5
Pastor, A. V., Palazzo, A., Havlik, P., Biemans, H., Wada, Y., Obersteiner, M., et al. (2019). The global nexus of food–trade–water sustaining environmental flows by 2050. Nat. Sustain. 2 (6), 499–507. doi:10.1038/s41893-019-0287-1
Qadir, M., Oster, J. D., Schubert, S., Noble, A. D., and Sahrawat, K. L. (2007). Phytoremediation of sodic and saline-sodic soils. Adv. Agron. 96 (07), 197–247. doi:10.1016/S0065-2113(07)96006-X
Rose, T. J., Pariasca-Tanaka, J., Rose, M. T., Fukuta, Y., and Wissuwa, M. (2010). Genotypic variation in grain phosphorus concentration, and opportunities to improve P-use efficiency in rice. Field Crops Res. 119 (1), 154–160. doi:10.1016/j.fcr.2010.07.004
Saito, k., Vandamme, E., Johnson, J., Tanaka, A., Senthilkumar, K., Dieng, I., et al. (2019). Yield-limiting macronutrients for rice in sub-Saharan Africa. Geoderma 338, 546–554. doi:10.1016/j.geoderma.2018.11.036
Sheehy, J. E., Dionora, M., and Mitchell, P. L. (2001). Spikelet numbers, sink size and potential yield in rice. Field Crops Res. 71 (2), 77–85. doi:10.1016/S0378-4290(01)00145-9
Song, T., Xu, F., Yuan, W., Chen, M., Xu, W., Tian, Y., et al. (2019). Combining alternate wetting and drying irrigation with reduced phosphorus fertilizer application reduces water use and promotes phosphorus use efficiency without yield loss in rice plants. Agric. Water Manag. 223, 105686. doi:10.1016/j.agwat.2019.105686
Tang, X., Shi, X., Ma, Y., and Hao, X. (2011). Phosphorus efficiency in a long-term wheat–rice cropping system in China. J. Agric. Sci. 149 (03), 297–304. doi:10.1017/S002185961000081X
Tian, Z., Li, J., He, X., Jia, X., Yang, F., Wang, Z., et al. (2017). Grain yield, dry weight and phosphorus accumulation and translocation in two rice (oryza sativa L.) varieties as affected by salt-alkali and phosphorus. Sustainability 9, 1461. doi:10.3390/su9081461
Tian, Z., Li, J., Jia, X., Yang, F., and Wang, Z. (2016). Assimilation and translocation of dry matter and phosphorus in rice genotypes affected by salt-alkaline stress. Sustainability 8 (6), 568. doi:10.3390/su8060568
Vandamme, E., Wissuwa, M., Rose, T., Dieng, I., Drame, K. N., Fofana, M., et al. (2016). Genotypic variation in grain P loading across diverse rice growing environments and implications for field P balances. Front. Plant Sci. 7, 1435. doi:10.3389/fpls.2016.01435
Wang, K., Cui, K., Liu, G., Luo, X., Huang, J., Nie, L., et al. (2017). Low straw phosphorus concentration is beneficial for high phosphorus use efficiency for grain production in rice recombinant inbred lines. Field Crops Res. 203, 65–73. doi:10.1016/j.fcr.2016.12.017
Wei, H., Meng, T., Li, C., Xu, K., Huo, Z., Wei, H., et al. (2017). Comparisons of grain yield and nutrient accumulation and translocation in high-yielding japonica/indica hybrids, indica hybrids, and japonica conventional varieties. Field Crops Res. 204, 101–109. doi:10.1016/j.fcr.2017.01.001
Wei, H., Meng, T., Li, C., Zhang, H., Dai, Q., Ma, R., et al. (2016). Accumulation, distribution, and utilization characteristics of phosphorus in yongyou 12 yielding over 13.5 t ha-1. Acta Agron. Sin. 42 (6), 886. (in Chinese with English abstract). doi:10.3724/sp.J.1006.2016.00886
Wu, L., Cui, Z., Chen, X., Yue, S., Sun, Y., and Zhao, R. (2015). Change in phosphorus requirement with increasing grain yield for Chinese maize production. Field Crops Res. 180, 216–220. doi:10.1016/j.fcr.2015.06.001
Yang, J., and Zhang, J. (2010). Crop management techniques to enhance harvest index in rice. J. Exp. Bot. 61 (12), 3177–3189. doi:10.1093/jxb/erq112
Yan, J., Yu, J., Tao, G., Vos, J., Bouman, B., Xie, G., et al. (2010). Yield formation and tillering dynamics of direct-seeded rice in flooded and nonflooded soils in the Huai River Basin of China. Field Crops Res. 116 (3), 252–259. doi:10.1016/j.fcr.2010.01.002
Zhang, F., Chen, X., and Chen, Q. (2010). Fertilization guide of major crop species in China. Beijing: China Agric. Univ. Press. (In Chinese).
Zhang, F., Cui, Z., Chen, X., Ju, X., Jiang, R., Chen, Q., et al. (2012). Integrated nutrient management for food security and environmental quality in China. Adv. Agron. 116, 1–40. doi:10.1016/B978-0-12-394277-7.00001-4
Zhang, W., Ma, W., Ji, Y., Fan, M., Oenema, O., Zhang, F., et al. (2008). Efficiency, economics, and environmental implications of phosphorus resource use and the fertilizer industry in China. Nutr. Cycl. Agroecosyst. 80, 131–144. doi:10.1007/s10705-007-9126-2
Keywords: phosphorus uptake requirements, yield level, rice, saline-sodic stress, yield formation
Citation: Wei Z, Zhang Y, Liu Z, Peng M, Wang T and Cao N (2022) Change in phosphorus requirement with increasing grain yield for rice under saline-sodic stress in Northeast China. Front. Environ. Sci. 10:953579. doi: 10.3389/fenvs.2022.953579
Received: 26 May 2022; Accepted: 06 July 2022;
Published: 08 August 2022.
Edited by:
Haigang Li, Inner Mongolia Agricultural University, ChinaReviewed by:
Shanchao Yue, Northwest A&F University, ChinaCopyright © 2022 Wei, Zhang, Liu, Peng, Wang and Cao. This is an open-access article distributed under the terms of the Creative Commons Attribution License (CC BY). The use, distribution or reproduction in other forums is permitted, provided the original author(s) and the copyright owner(s) are credited and that the original publication in this journal is cited, in accordance with accepted academic practice. No use, distribution or reproduction is permitted which does not comply with these terms.
*Correspondence: Ning Cao, Y2FvbmluZ2puQDE2My5jb20=
†These authors have contributed equally to this work
Disclaimer: All claims expressed in this article are solely those of the authors and do not necessarily represent those of their affiliated organizations, or those of the publisher, the editors and the reviewers. Any product that may be evaluated in this article or claim that may be made by its manufacturer is not guaranteed or endorsed by the publisher.
Research integrity at Frontiers
Learn more about the work of our research integrity team to safeguard the quality of each article we publish.