- 1Integrated Regional Office, Ministry of Environment, Forest and Climate Change (MoEFCC), Hyderabad, India
- 2Department of Environmental Science, Parul Institute of Applied Sciences, Parul University, Vadodara, Gujarat, India
- 3Department of Physics, Faculty of Sciences, University 20 Août 1955, Skikda, Algeria
- 4College of Medicine, King Khalid University, Abha, Saudi Arabia
- 5Department of Biosciences, School of Liberal Arts and Sciences, Mody University of Science and Technology, Sikar, India
- 6School of Environment and Sustainable Development, Central University of Gujarat, Gandhinagar, India
- 7Faculty of Science and Technology, Madhyanchal Professional University, Bhopal, India
- 8Department of Electrical and Computer Engineering, Faculty of Engineering, King Abdulaziz University, Jeddah, Saudi Arabia
- 9Department of Microbiology and Biotechnology, Sardar Vallabh Bhai Patel College, Mandleshwar, Madhya Pradesh, India
- 10Department of Chemistry, SBS Government PG College, Hoshangabad, India
- 11Department of Earth Resources and Environmental Engineering, Hanyang University, Seoul, South Korea
- 12Geobiotec Research Centre, Department of Geoscience, University of Aveiro, Aveiro, Portugal
Environmental pollution is becoming more prevalent in both human life and the ecosystem. The increased use of fossil fuels, mining, and the burning of wastes, as well as industrial wastewater discharge, are polluting natural resources such as water, soil, and air. Metals (loid)s (Cu, Cr, Cd, Zn, Ni, Pb, Hg, Sb, Sn, and As) contribute to several ecological problems when exposed to humans and the environment resulting in serious health and environmental risks. The pollution of aquatic and terrestrial sites with these elements is an issue of environmental as well as public health significance. The present review highlights environmental problems instigated by the toxic metal (loid)s, their source, and respective health/environmental concern along with the importance of creating low-cost, environmentally acceptable clean-up technologies for treating household and industrial wastewater. Various physical, chemical, biological, and/or biochemical as well as their various combinations have been described from the sustainable technological point of view. Techniques such as ion exchange, membrane filtration, photocatalysis, bioremediation, phytoremediation, economical biosorbents, and nanomaterials have been discussed in detail along with respective recent case studies to gain a significant inside towards the solution of the environmental problems focused and action-oriented sustainable technologies development. Thus, this article significantly provides a deep insight into metal (loid)s toxicity, source identification, and their influences on the ecosystem and human health along with conventional and sustainable clean-up technologies.
1 Introduction
The basic necessity across the planet is represented by the environmental significance of water. Water is the most fragile component of our environment, and it is essential for both human and industrial progress. As the population rises, so does the demand for clean water for drinking purposes. This precious resource must be handled in a viable mode to safeguard the expansion of the human population in an environment with limited resources (Boretti and Rosa, 2019). Water resource management is a challenge in many developing nations owing to a lack of integration and a comprehensive strategy, generally with minimal engagement from the general public and other stakeholders other than the government (Loucks and van Beek, 2017).
The severe environmental problem is growing as a result of water pollution and insufficiency, and its restricted availability is growing as a result of the devastation of natural water supplies. During the last 50 years, industrialization has expanded at a rapid pace, increasing the need for indiscriminate exploitation of the Earth’s natural resources, and exacerbating the worldwide problem of environmental pollution (Ahmed et al., 2022). Toxins such as organic and inorganic contaminants, organometallic mixtures, radioactive isotopes, and gaseous pollutants have severely polluted the aquatic environment (Briffa et al., 2020). Industrial activities have increased in developing countries in modern times, thus contributing to more metal (loid)s pollution. The metal (loid)s contamination is mostly instigated by the discharge of wastewater from different industries such as power plants, mining, pharmaceutical, metal finishing as well as waste disposal activities (Srivastava et al., 2017; Kinuthia et al., 2020).
Several water quality issues are waiting to be solved including metal(loid)s, pesticides, fluoride, and many more (Islam et al., 2018a; Wołowiec et al., 2019). Due to the unrestricted release of industrial wastes, raw sewage, and other waste contaminants, the majority of the environment disrupts organism survival and biological activity (Akhtar et al., 2021).
The assurance of clean water is a big challenge. Sustainable technologies are having potential and these technologies can create huge changes. Sustainable technologies are a powerful tool for managing environmental problems and offering remarkable solutions. These technologies are surely a great promise and a hope for advancements of existing technologies to gain maximum outputs (Rene et al., 2021).
Soil pollution by metal (loid)s is becoming a critical environmental concern due to industrial revolution and urbanization (Alengebawy et al., 2021). The persistent and non-biodegradable nature of metal (loid)s facilitates their accumulation in the soil and can poses harmful effects on the human health (Sarwar et al., 2017). The distribution of metal (loid)s in soils is associated with both natural and anthropogenic inputs. Natural sources include volcanic eruptions, geological weathering of parent rock materials, etc. Whereas, anthropogenic sources of metal (loid)s are extensive use of fertilizers and pesticides in agriculture farms, mining and smelting of metals, industrial emissions, aerosol deposition, spillage of petrochemicals, leaded gasoline and paints, and atmospheric deposition (Chibuike and Obiora, 2014). Soils are the primary sink for metal (loid)s released into the environment, where metal (loid)s go through several complex physicals and chemical processes such as co-precipitation, adsorption, desorption, metal oxide or hydroxide formation, hydrolysis, and biological uptake (Delshab et al., 2016). Accumulation of metal (loid)s in soil is boosted by the different components such as organic matter, phyllosilicate, carbonate, and charged minerals. Moreover, they show ion exchange with clay particles, where clay minerals bearing several oxides and humic matter form organo-metal compounds, which escalates metal (loid)s accumulation in soil (Violante et al., 2010). All over the world, there are over 5 million areas where metal (loid)s concentrations are now over the allowable levels (Li et al., 2019). Metal (loid)s pollution has induced several threats to the ecosystem and people, and it impacts food chain security (soil-plant-human or soil-plant-animal-human), reduction in food quality via phytotoxicity, and diminishing the ability to use the land for agricultural production, all of which have an impact on food security and exacerbate issues of land tenure crises (Gonzalez Henao and Ghneim-Herrera, 2021). Therefore, characterization and clean-up of metal (loid)s contaminated soil are necessary for their effective conservation and restoration. Risk assessment is a potent scientific technique that helps decision-makers to manage highly contaminated areas efficiently and affordably while conserving the ecosystem and human health.
This article scientifically reflects the properties of potentially toxic elements (metalloids) as harmful components in water and soil particularly highlighting their ecological persistence and their toxicity on human health. Further, the article describes several available clean-up technologies such as ion exchange, membrane filtration, photocatalysis, economical biosorbents, nanomaterials, bioremediation, and phytoremediation for potentially toxic elements (Cu, Cr, Cd, Zn, Ni, Pb, Hg, Sb, Sn, and As).
2 Metal(loid)s
Metal (loid)s is a term used to describe a group of elements having a high molecular weight and density (Punia et al., 2022). These elements are considered major environmental contaminants even in little quantities. Metal (loid)s such as Cd, Ni, Hg, As, Cr, Th, Zn, Sb, Cu, and Pb have a comparatively great density extending from 3.5 to 7 g cm3 (Briffa et al., 2020). Metal (loid)s contamination poses a severe hazard and concern to the bio-network (Hashem et al., 2017). As per USEPA, the eight most prevalent metal (loid)s are lead, chromium, arsenic, zinc, copper, cadmium, nickel, and mercury (Tchounwou et al., 2012). A broad categorization of metal (loid)s based on their classes is demonstrated in Figure 1.
Metal(loid)s tend to accumulate in the environment which results in their higher concentrations than the allowed limits (Rzymski et al., 2014). The maximum permissible limit of different metals such as Cr, Cd, Hg, Pb, and Ag in an aqueous medium is 0.01, 0.05, 0.002, 0.015, and 0.05 mg/L respectively, as per the Comprehensive Environmental Response Compensation and Liability Act of the United States (Jaishankar et al., 2014). If the concentrations exceed acceptable levels, they can cause various problems such as Cancer, Alzheimer, and Parkinson disease (Muszyńska and Hanus-Fajerska, 2015). The problem of metal (loid)s contamination has prompted several researchers to create numerous remediation techniques to keep pollution levels below the acceptable limit (Table 1).
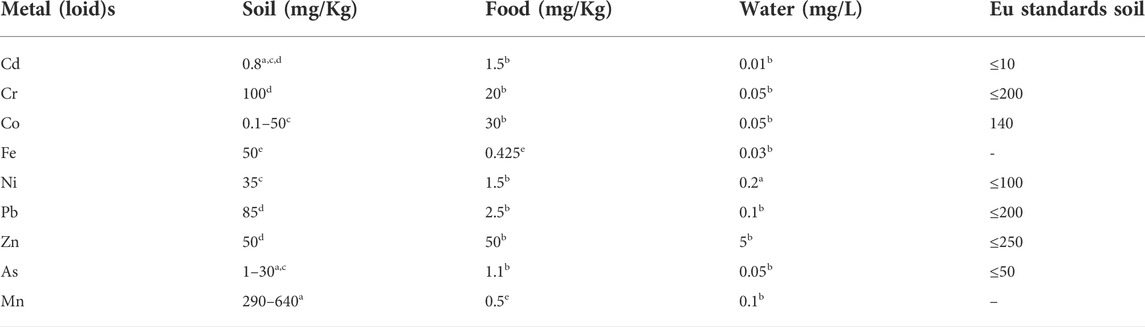
TABLE 1. Standards criteria for Maximum permissible limits for Metal(loid)s [Source: Kabata-Pendias and Pendias, (2011)a; Liu et al. (2018)b; WHO (1996)c; Denneman and Robberse (1990)d; Chiroma et al., (2014)e].
These metal(loid)s are abundant in the earth’s shell and are not decomposable. These metals come into the human body via air, water, and food. Some of the metal(loid)s have an important role in human metabolism at trace amounts but in higher concentrations, they can cause toxicity (Palansooriya et al., 2020; Ahmed et al., 2022). They can be emitted into the atmosphere via mining and industrial activities and end up adjacent to water bodies and soils (Alengebawy et al., 2021; Elbasiouny et al., 2021).
2.1 Metal(loid)s sources and contamination of environment
Metal(loid)s may originate from both natural/geogenic/lithogenic and man-made sources. Natural causes comprise weathering of metal-containing rocks and volcanic explosions, whereas major man-made sources consist of industrial emissions, excavating, smelting, and agronomic operations such as pesticide and phosphate fertilizer application (Gupta et al., 2019). The burning of fossil oils also leads to metal (loid)s emissions into the environment (Balali-Mood et al., 2021; Chheang et al., 2021). Metal (loid)s remain in the atmosphere, contaminate food, and create various health concerns (Ali et al., 2019). Paper companies, insecticides, tanneries, metal plating industries, mining activities, and other sectors release metal (loid)s into the environment, which are non-degradable and toxic to biological structures. However, metal (loid)s can be converted into less toxic elements and can persist in either inorganic or diverse forms (Han et al., 2016; Kumar et al., 2017). Landfill leachates, liquid disposal, industrial waste, etc., contaminate the groundwater. Temperature, pH, live organisms, cation exchange, evaporation, absorption, and other factors will all affect the metal (loid)s content of the water (Selvi et al., 2019). Different sources of metal (loid)s in the environment are presented in Figure 2.
2.2 Importance of Metal(loid)s in life
Metal(loid)s are categorized as essential or non-essential based on their roles in various biochemical and physiological functions. Essential metal(loid)s are required for living beings in very little quantities and metal(loid)s with no recognized biotic purpose are referred to as non-essential elements (Yan et al., 2020). The essential elements for living beings include Cu, Mn, Zn, and Fe, whereas Cd, Pb, Hg, As, and Cr are toxic and considered non-essential (Crichton, 2017). The chemical elements such as Cu, Fe, Ni, Mn, and Zn are vital for plants for their growth, stress tolerance, production, and functioning of diverse biomolecules like carbohydrates, chlorophyll, nucleic acids, and secondary metabolites (Rai et al., 2021a). Diseases or abnormal states result from either a shortage or an excess of an important heavy metal. Though, the necessary elements for various kinds of plant species, and microbes may differ. It indicates that an element may be required for one organism but not for another (Yan et al., 2020).
2.3 Environmental and human health risks of Metal(loid)s
Metal(loid)s are the utmost studied pollutants in the environment. Depending on the quantity and period of exposure, almost every metal(loid)s can be harmful to the biotic system. Cr, Ni, Cu, Zn, Pb, Cd, As, and Hg are the utmost harmful metal(loid)s in the environment (Egorova and Ananikov, 2017). China proposed Cr, Cd, Pb, As, and Hg as the major contaminants for management in 2009 in their 5-years plan for comprehensive prevention and control of metal(loid)s pollution because these metal(loid)s can be harmful to humans depending on the amount and exposure time (Fu et al., 2017; Kumar and Fulekar, 2017).
Metal(loid)s kills the aquatic organism, and cause oxygen deficiency, and algal blooms (Bashir et al., 2020). Once metal(loid)s are dumped into rivers, they become hydrated ions, which are significantly more dangerous than metal ions. Those ions interrupt the enzymatic activities of the aquatic microorganism and make absorption faster. Therefore, metal(loid)s removal is required to reduce public hazards (Yan et al., 2020).
Metal(loid)s ions such as Cd2+, Pb2+, Hg2+, Ag+, and As3+ react with bioparticles in the human body to create hazardous chemicals (Shimizu et al., 2019). The toxicity of metal(loid)s reduces cerebral and neurological function and harms lungs, and kidneys, loss of memory, and cause allergies in the human body. Cell death is also caused by the production of free radicals, which are responsible for oxidative stress (Wang and Du, 2013; Rai et al., 2019). Table 2 describes potential human health concerns of metal (loid)s.
The toxicity of metal(loid)s in the human body, food chains and ecosystems has been considered a serious threat to their safety and integrity. The high-level toxicities of various metal(loid)s and their multi-trophic transfer into the ecosystems (agriculture, soil, plants, etc.) are serious issues that need to be addressed (Alengebawy et al., 2021; Sarker et al., 2022). Various global policies are also important to address these issues with the respect to developing and developed countries and the presence of various technologies (Yap and Al-Mutairi, 2022). Various recent reports have been explaining the ecotoxicology of metal(loid)s, their source, transport, and health-environmental risks (Parker et al., 2022). The human health impact and eco-toxicity of various metal (loid)s are given below.
2.3.1 Cadmium
Cadmium (Cd) can be found in the earth’s crust together with other elements. Cd is not required for biotic processes. In addition, it is the most hazardous metal found in industrial discharge. It is used extensively in sectors such as electroplating, Cd-Ni batteries, phosphate fertilizers, stabilizers, and composites (Haider et al., 2021). Even at little concentrations, Cd compounds are very toxic and accumulate in the environment. The “Itai-Itai” illness is caused by Cd build-up in the river and causes bone weakening and fractures in humans. It can potentially cause lung cancer as well as damage to the respiratory system, liver, and kidney (Rafati Rahimzadeh et al., 2017; Genchi et al., 2020b).
2.3.2 Chromium
Chromium (Cr) exists as ore, which is made up of ferric chromite (FeCr2O4), crocoite (PbCrO4), and chromeochre (Cr2O3). It is widely utilized in the leather industries, as well as in paper, pulp, and rubber manufacture. The high Cr concentrations can affect the liver and kidneys, create skin ulcers and affect the nervous system of humans. Cr (VI) is more hazardous than Cr (III) for human health (Demim et al., 2013; Carolin et al., 2017). Cr (VI) is generally found in the chromate salt manufacturing sector. Cr (III) is beneficial in fat breakdown and shows a vital role in the digestion of sugar (Król et al., 2020; Li et al., 2021).
2.3.3 Lead
Lead (Pb) poisoning is extremely harmful to humans. The higher Pb concentrations have the potential to harm the foetus and be toxic to the central nervous system (Kumar et al., 2020). Pb may be found in the forms of sulphide, cerussite (PbCl2), and galena. The primary reason for Pb contamination in industrial waste is lead-acid battery discharge. It causes illnesses such as renal and neurological system damage, intellectual disability, and cancer (Qu et al., 2013; Cechinel et al., 2014; Briffa et al., 2020). Airborne Pb can pollute food by deposition on soils and water (Kumar et al., 2020).
2.3.4 Copper
At high concentrations, Copper (Cu) is often regarded as an extremely toxic metal. It is an essential element for people which has a significant role in enzyme activities, bone formation, and tissue development (Balali-Mood et al., 2021). It is utilized in the fabrication of kitchenware, electric cables, tubes, brass, and bronze. Mining, element manufacture, steel trades, circuits, coating trades, dyes, fertilizers, and other sectors are important contributors to Cu (Gao et al., 2013; Mehta et al., 2015). Cu causes the loss of hair, anemia, renal injury, and headaches and also causes mortality when accumulated in the liver, brain, and pancreas, resulting in mortality (Grzeszczak et al., 2020).
2.3.5 Mercury
Mercury (Hg) is generally found in industrial discharge in several forms, such as elemental mercury (Hg0), mercurous ion (Hg22+), and mercuric ion (Hg2+). In general, a considerable quantity of Hg is supplemented in sectors such as paper and pulp, plastics, chloro-alkali, pharmaceuticals, oil refineries, etc. In its organic form, Hg is a very poisonous metal that has been linked to Minamata illness in Japan (Parham et al., 2012). Hg can harm the kidneys, the brain, the reproductive system, and the respiratory system.
2.3.6 Zinc
Zinc (Zn) contributes to the regulation of biological processes and the functioning of tissues in humans. It protects other metals by acting as a protective coating (Hernández-Camacho et al., 2020). Corrosion can be avoided by adding Zn to the steel. It is used in several manufacturing procedures, including steel production, mining, and coal burning. Although Zn is necessary for humans in trace amounts but it can cause vomiting, skin irritation, fever, and anemia (Cristian et al., 2015).
2.3.7 Nickel
Nickel (Ni) is released from several industrial sources such as printing, electroplating, silver refineries, battery manufacture, and alloy sectors (Demim et al., 2013; Malamis and Katsou, 2013). It is required for human blood cell production; nevertheless, the excessive quantity of Ni is hazardous. Trace quantities of Ni may not harm biological cells, but prolonged exposure to a high quantity may harm cells, reduce weight, and harm the heart and liver. Ni toxicity could result in decreased cell development and cancer (Genchi et al., 2020a).
2.3.8 Arsenic
Arsenic (As) occurs naturally in the earth’s crust in the form of arsenite and arsenopyrite across the planet. As is mostly found in groundwater as arsenate and arsenite (Shankar et al., 2014; Awasthi et al., 2017). The movement of As in water is generally controlled by pH and adsorption. As adsorption in aquatic bodies is aided by metal oxides of Fe, Al, and Mn. As has been discovered at significant concentrations naturally in groundwater in India, Bangladesh, Taiwan, Brazil, and Chile. Because of its higher quantity in drinking water, it is hazardous to both people and animals. The groundwater pollution from As initiated by weathering of rocks and sediments, as well as consuming arsenic-contaminated water, causes blood poisoning, lung cancer, and breathing problems (Nicomel et al., 2015; Yeo et al., 2021).
2.3.9 Cobalt
Volcanic outbreaks, ocean spray, and forest fires are all-natural causes of Cobalt (Co) in the environment. Coal-fired power stations as well as car exhaust, are man-made causes of Co in the atmosphere. Co processing operations, the manufacture of cobalt-containing composites, sewages, domestic and agronomic overflow are all important sources of Co to the river ecosystem (Farjana et al., 2019; Dehaine et al., 2021; Horn et al., 2021). The human health consequences of Co are defined by a complicated clinical syndrome that includes neurological, cardiovascular, and endocrine abnormalities (Leyssens et al., 2017).
2.3.10 Iron
Iron (Fe) is a substantial component of the lithosphere, accounting for roughly 5% of the total. It is often discovered in municipal discharge, mainly in locations where Fe and steel are produced. Even at little quantities of around 1.8 mg/L, the taste of Fe in water may be perceived. Iron enters the environment mostly through industrial effluents such as pharmaceutical goods, mining trades, mineral treating, and plating industries (Briffa et al., 2020). The occurrence of Fe(II) in water promotes the proliferation of iron microorganisms, which results in unpleasant water color, taste, and odour. Fe is important for humans for the normal working of the biological system (Gülay et al., 2018). Anemia is caused by Fe deficiency. Excess iron, on the other hand, is harmful to humans. Excessive Fe consumption causes haemochromatosis, which causes tissue damage owing to Fe build-up. The first signs of Fe poisoning in humans include vomiting, diarrhea, and intestinal damage (Smith et al., 2017).
3 Clean-up technologies for metal(loid)s
The metal(loid)s are openly discharged into the environment and affect humans, animals, and plants. The most common routes of contact are breathing and skin interaction in humans. The increasing danger of human exposure to these metal(loid)s has significant health concerns as well as ecological degradation (Rzymski et al., 2014; Kumar, 2018). Therefore, their detection and clean-up from the environment is a very important aspect (Figure 3). Several clean-up technologies for metal(loid)s removal have been developed during the last few decades (Figure 4).
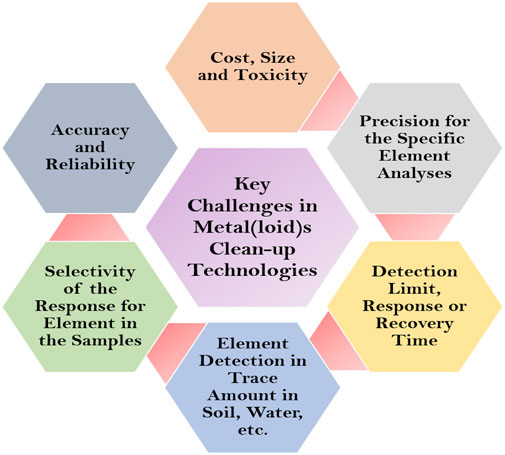
FIGURE 3. Key challenges to improve sustainable technologies for metal(loid)s detection and clean-up from the environment.
Since metal(loid)s ion concentrations in the environment repeatedly exceed acceptable limits, therefore many treatment approaches are being applied to protect the environment. Ion exchange, adsorption, filtration, electrodialysis, precipitation, microbiological, electrochemical method, and membrane bioreactors are more interesting and dependable clean-up treatment technologies for these potentially toxic elements (Rajasulochana and Preethy, 2016; Qasem et al., 2021). These techniques are classified as physical technologies, chemical technologies, and biological technologies. Different treatment techniques may vary depending on the types of elements, however, a combination of different techniques may be used for the successful removal of metal(loid)s. Table 3 summarises the merits and drawbacks of these technologies.
3.1 Chemical precipitation
It is the process of adding compounds to convert the physical state of dissolved particles to enable their removal via sedimentation (Pohl, 2020). These precipitates are separated during the sedimentation process, and the residual solution is utilized for various uses. It is a proficient way of removing Cu, Cd, Mn, and Zn. Tanong et al. (2017) examined the removal of Ni and Mn by the addition of Na2Co3, which was shown to be fully precipitated at pH 9. To eliminate precipitates from water, further techniques such as filtering and sedimentation are necessary. The treated water may then be used again or released into the environment. Though, this procedure needs a huge number of compounds to precipitate the metals. They are commonly applied in industries due to their simplicity and cost-effectiveness. Hydroxide and sulphide precipitation are the modern chemical precipitation processes (Estay et al., 2021). Several researchers have used different techniques involved in chemical precipitation for removing metal(loid)s from wastewater with the application of different materials (Table 4).
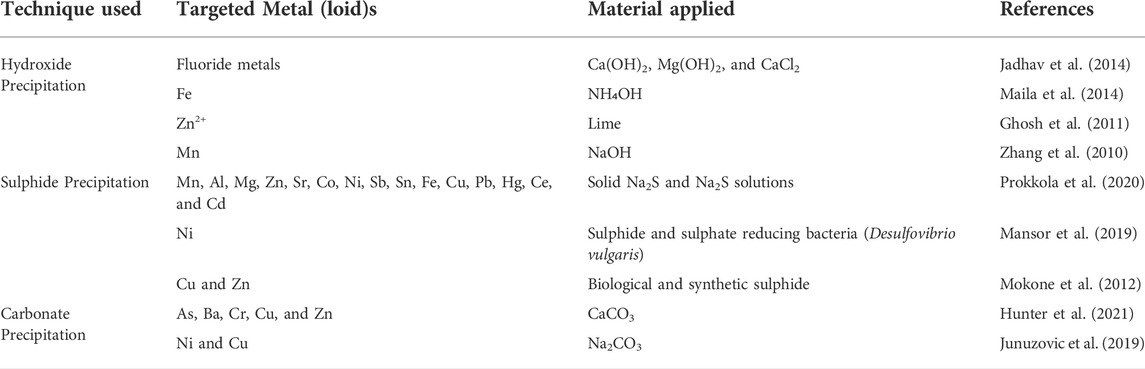
TABLE 4. Application of different materials used in the chemical precipitation technique for metal(loid)s removal.
3.1.1 Hydroxide precipitation
Chemical precipitation of metal(loid)s as hydroxides using lime or sodium hydroxide is extensively applied due to its ease of handling and low cost. The metal hydroxides that are inexplicable in the alkaline medium are increased when the pH is changed. For metal(loid)s removal, layered double hydroxides are produced in the case of trivalent ions (Thomas et al., 2021). To create a hydroxide precipitate, several precipitants such as lime, calcium hydroxide, ammonium hydroxide, and sodium hydroxide are presented. Due to their ease of accessibility, lime and limestone are often employed (Kumar and Jana, 2021). The drawbacks of this approach include differences in the optimal pH for hydroxide production across metals, which may cause issues in the treatment of effluents comprising mixed metal ions. Other disadvantages include variable metal hydroxide solubility at a given pH and the production of a considerable sludge volume (Vidu et al., 2020).
3.1.2 Sulphide precipitation
Sulphide precipitation can provide better metal (loid)s removal because most metal(loid)s create stable sulphides. The benefit of employing sulphide precipitation is that sulphide precipitate is less soluble in alkaline conditions than hydroxide precipitate. Solid (ferrous and calcium sulphide), aqueous (sodium sulphide, sodium hydrosulphide, ammonium hydrosulphide), or gaseous sulphide sources (hydrogen sulphide) are the most commonly utilized sulphide precipitants (Günes et al., 2021).
3.1.3 Carbonate precipitation
Metal carbonate precipitation using Ca or Na carbonates is relatively limited. Several scientists described superior results for Cd (II) and Pb (II) from electroplating wastes using carbonate precipitate. Once the pH was raised to 7.5, the remaining Pb (II) and Cd (II) concentrations were 0.60 and 0.25 mg/L, correspondingly (Egbosiuba et al., 2021).
3.2 Membrane filtration
It is a pressure-driven technology that is now applied in the treatment of wastewater. This process includes disinfection in addition to metal(loid)s removal (Lyu et al., 2016). The purification process may be accelerated by treating the membrane with chemical substances. The membrane is constructed of a permeable substance that shows a vital part in metal(loid)s removal from wastewater (Patil et al., 2016). Membrane materials are divided into ceramic and polymer. Ceramics, due to their chemical resilience are mostly employed for industrial wastewater treatment. It is a favourable technology for metal(loid)s removal due to its effectiveness and easy process as compared to other methods. It is also effective in removing suspended particles and chemical substances. Despite its benefits, membrane filtration is restricted to metal(loid)s elimination due to membrane fouling, frequent membrane replacement, and high cost (Kotobuki et al., 2021). Membranes are complicated structures that incorporate nanometer-scale active components. The partitioning of water and dissolved salts across the membrane are impacted by the chemical characteristics and the physical structures of the membrane on nano to microscales. This transitional range comprises colloidal solids, big organic molecules, and polymers from the standpoint of filtration. Ultrafiltration, nanofiltration, electrodialysis, and reverse osmosis are the membrane techniques applied to clean up the metal (loid)s (Khulbe and Matsuura, 2018; Algieri et al., 2021). Different types of membranes applied by the researchers for metal(loid)s clean-up have been illustrated in Table 5.
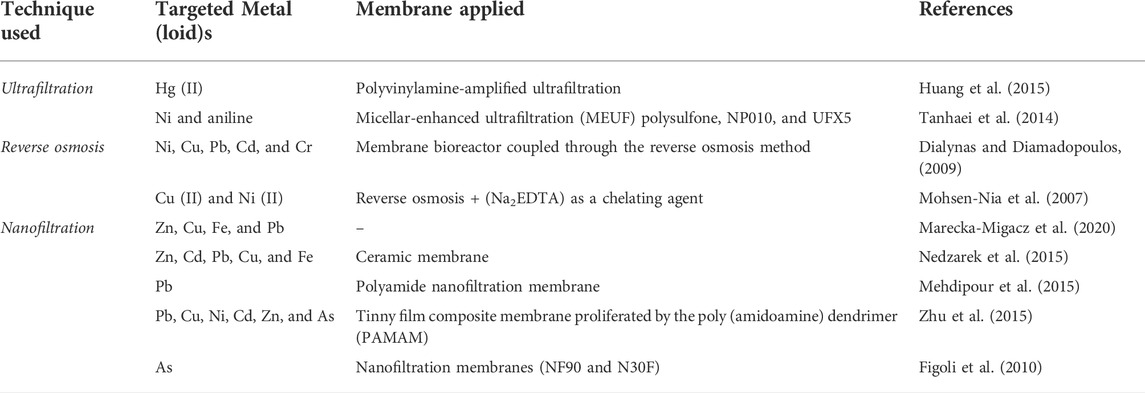
TABLE 5. Different membranes applied in the membrane filtration technique for the removal of metal (loid)s.
3.2.1 Ultrafiltration
Ultrafiltration is a separation technology that uses less energy to clean wastewater. This technique removes particles in the 10–100 nm size range. The particles quickly separate from the aqueous solutions due to hydrophobic and electrostatic interfaces. Since it has a bigger hole size membrane that is larger than metal(loid)s ions size, this technology is usually utilized in the form of a combination technique. Thus, the metal(loid)s ions can readily flow through the UF membrane. Chemical and polymeric agents were employed to increase the ultrafiltration process, which is known as micellar-enhanced ultrafiltration (MEUF) and polymer enhanced ultrafiltration (PEUF) (Warsinger et al., 2016; Naseem and Durrani, 2021). MEUF was developed in the 1980s and used to remove the metal(loid)s and organic pollutants from the wastewater. It is a good separation method for extracting metal(loid)s. Surfactants adding is the simple principle of this method (El Batouti et al., 2021). Samper et al. (2009) utilized miceller-enhanced ultrafiltration to eradicate Cd2+, Pb2+, Ni2+, Zn2+, and Cu2+ from synthetic water in a lab-scale system utilizing SDS and linear alkylbenzene sulfonate (LAS). PEUF is presented as a sustainable technique for extracting metal(loid)s ions from an aqueous medium. It employs a water-soluble polymer with a greater molecular weight than complex metallic ions and produces macromolecular (Meng et al., 2021; Oyarce et al., 2022). Lin et al. (2020) applied micellar-enhanced ultrafiltration (MEUF) to extract Ni ions from aqueous solutions, utilizing the surfactant sodium dodecyl sulphate (SDS) as a chelating agent. Using the Box-Behnken design, a response surface method was applied to model and optimize process variables and indicators. The response surface method might sufficiently represent the performance pointers within the evaluated ranges of the procedure variables, according to experimental verification. To estimate MEUF performance and confirm the RSM results, an artificial neural network (ANN) model was used. The generated ANN models suited the experimental data well.
3.2.2 Reverse osmosis
This method employs a semi-permeable film which permits the cleansed liquid to flow while rejecting impurities. It helps in removing the different microorganisms from water and is also responsible for about 20% of the world’s salt removal capability. The pore size of a RO membrane ranges from 0.1 to 1.0 nm. It's commonly utilized in the desalination process (Feria-Díaz et al., 2021). The capacity of a RO membrane is determined by the membrane’s composition, temperature, pressure, pH, and clogging properties. To prevent membrane entangling, the wastewater is pre-treated, which eliminates shallow and colloidal particles. This technology has been exclusively applied in water treatment for the past 10 years (Obotey Ezugbe and Rathilal, 2020). Petrinic et al. (2015) reported that the wastewater treatment from the metal finishing sector utilizes integrated membrane techniques like ultrafiltration and reverse osmosis to eliminate suspended particles and metal(loid)s. This dual membrane technique eliminates 91.3 percent to 99.8% of pollutants from effluent, including metals, organic and inorganic composites, and the ultrafiltration reduces fouling of the RO membrane.
3.2.3 Nanofiltration
Nanofiltration is a pressure-driven technique and is widely utilized in the chemical and biotech sectors. It is a technology that falls in between reverse osmosis and ultrafiltration. The benefits of this technique include low energy consumption, efficiency, and easiness of operation. It also requires less pressure than reverse osmosis (Tao et al., 2016; Abdel-Fatah, 2018). Its effectiveness is determined by pH, temperature, membrane propensity, and design (Hosseini et al., 2016). The membranes are typically prepared by synthetic polymers and externally charged, which aids in the dissociation of metal (loid)s. Size exclusion and charge exclusion are the separation mechanisms used in nanofiltration (Siddique et al., 2020; Suhalim et al., 2022). This technology is favourable for eliminating metal (loid)s from wastewater, including Ni (Murthy and Chaudhari, 2008), Cr (Muthukrishnan and Guha, 2008), Cu (Ahmad and Ooi, 2010); and As (Nguyen et al., 2009; Figoli et al., 2010).
3.3 Chemical coagulation/flocculation
Coagulation/flocculation might be used to clean up the metal(loid)s from wastewater, (Tang et al., 2016). The coagulation treatment disrupts colloidal elements by applying a coagulant, resulting in sedimentation. In general, coagulation is trailed by flocculation of the unsteady elements into large floccules to enlarge particle size (Teh et al., 2016). The enormous floccules will finally settle in the sedimentation tank. Alum, ferric chloride and ferrous sulfate are widely utilized in conventional wastewater treatment systems (Shewa and Dagnew, 2020). This technique has a few drawbacks, such as high functioning expenses owing to the broad utilization of chemicals. Macromolecule flocculants are being applied as efficient flocculants for metal(loid)s remediation from wastewater (Maćczak et al., 2020; Jiang et al., 2021). Shen et al. (2015) investigated the removal of Cr (III), Zn (II), and Cd (II) metal ions using flocculation. Cationic polymers and anionic surfactants were applied in the investigation. The resulting structure uses cationic polymers and anionic surfactants known as polymer-surfactant aggregates (PSAs). These PSAs exhibited the capability to eliminate metal(loid)s from the system. Diallyldimethylammonium chloride (PolyDADMAC) or poly ethylenimine (PEI) and sodium dodecyl sulphate (SDS) were used as cationic polymers and anionic surfactants respectively. All three elements were easily removed using this system and 99% of efficiency was achieved in 20 min under optimum conditions. Pang et al. (2011) used aluminium sulfate, polyaluminium chloride, and magnesium chloride coagulants for the removal of Pb, Zn, and Fe. Koaret PA 3230 was also used as polyelectrolyte in the experiment. The individual metal and the mixture of selected metals along with pH effect, the dosage of coagulant, and flocs settling time were examined. The outcomes concluded that PACI was found to be the most effective coagulant among all three coagulants.
3.4 Ion-exchange
It is a separation technique that replaces one ion with another for treating wastewater with higher metal(loid)s ion elimination efficiency with less sludge production as compared to the coagulation process (Luhar et al., 2021). Ion exchange resin is a metal(loid)s recovery or removal substance divided into two groups: synthetic resins and natural resins. Both resins are used to swap metal ions with cations. Synthetic resins are far more preferred for infinite metal separation. The main usage of synthetic resins is to remove As from wastewater. Fouling of the matrix happens in the presence of an extremely rigorous metal solution, which is a drawback of these resins (Dixit et al., 2021). Zeolites and silicate minerals are extensively utilized to eradicate metal(loid)s from wastewater in addition to manufactured resins because of little price and availability. Several studies have shown that zeolites have high cation-exchange abilities for metal(loid)s ions in various conditions (Jiang et al., 2018; Morante-Carballo et al., 2021). The foremost constraints of ion exchange are the higher cost and the need for adequate pre-treatment schemes. It can deliver metal(loid)s ion concentrations down to parts per million (Crini and Lichtfouse, 2019). Thakare and Jana (2015) applied high-density ion exchange resin (INDION225H) in aqueous media to remove Cu (II). The physical properties of adsorbent, equilibrium, kinetic and thermodynamic investigations have been carried out. The surface of INDION225H resin is made up of 49.9% carbon, 36.1% oxygen, 13.8% sulphur, and 0.18% copper. The equilibrium results indicated 79.49 kJ/mol activation energy for Cu (II) ion adsorption and Freundlich as well as Redlich–Peterson Isotherm followed by the system. Pseudo first and second-order kinetic was responsible for adsorption phenomena. The rate of reaction rises with the primary concentration of metal. The adsorption of metal also depended on the temperature of the system. Zewail and Yousef (2015) eliminated Ni (II) and Pb (II) metal ions using cation exchange resins (AMBERJET 1200 Na). The batch conical air spouted bed was used for the investigation purpose. The findings indicated that 98 and 99% of Ni (II) and Pb (II) metal ions were easily removed under optimum conditions. The air velocity, time, and primary concentration of metals were also examined during the experiment. The findings recommended that the pseudo-second-order kinetic model easily explains the adsorption phenomena under investigation.
3.5 Electrodialysis
Electrodialysis (ED) is a membrane technology that uses an electric field as a dynamic force to discrete ions through charged films (Gurreri et al., 2020; Shen and Badireddy, 2021). Ion-exchange membranes are engaged in the majority of ED procedures. Cation and anion-exchange membranes are two categories of membranes. This method is being extensively utilized to produce drinking water from seawater, as well as to treat industrial effluents, recover valuable minerals, and produce salt. This process has similarly demonstrated a potential technique in the treatment of metal (loid)s (Hassanvand et al., 2017). Sivakumar et al. (2014) remediated Cd and Sn using electrodialysis. The trial was performed at a different temperature and agitation speed and this method easily removed the metal(loid)s ions present in the electroplating wastewater. Both the metal(loid)s ions easily disappeared within 8 h of reaction at 50°C and 100 rpm. This study established that the electro-dialysis technique magnificently reduced Cd and Sn from wastewater collected from the electroplating industry. Moura et al. (2012) removed Cr using the electrodialysis method. The effluent samples were collected from the metal finishing and tannery industry. The two different prepared membranes (MCS and MTS) and one commercial membrane (Nafion 450) were used for comparative analysis. The findings concluded that all three membranes were having almost similar efficiency for tannery effluents whereas Nafion 450 showed superior efficiency than MCS and MTS in the case of metal finishing samples.
3.6 Electrochemical treatment
Electrochemical techniques can recuperate metal(loid)s in their elemental state by plating out ions on a cathode surface. Because electrochemical wastewater systems need a relatively substantial financial investment as well as a costly energy source, they have not been extensively used. However, because of severe environmental laws governing wastewater disposal, electrochemical methods have regained prominence globally (Rai et al., 2021b; Zavahir et al., 2021). Zuo et al. (2020) used MOF@rGO nanocomposite electrode material to remove Pb (II). In a typical electrochemical treatment method, rGO and Co-MOF endow the conductivity and selectivity for the process. MOF@rGO functioned as the cathode in the cell. The results revealed the reduction of Pb (II) concentration from 50 to 0.21 ppm. Song et al. (2019) applied the chronoamperometry method for the removal of As (III). The double potential step chronoamperometry approach was used for adsorption and oxidation of As (III). Initially As (III) has been easily converted into H2AsO3 (deprotonated from). At basic pH (pH = 10), this deprotonated easily adsorbed and oxidized into As (V). In a very simple electrochemical removal of As, 91% efficiency was achieved under experimental conditions.
3.7 Adsorption
Adsorption is currently regarded as a viable and low-cost approach for treating wastewater. This technique is flexible in terms of functioning, and it produces high-grade treated waste in many situations. Furthermore, since adsorption is occasionally rescindable, adsorbents could be renewed using an appropriate desorption method (Younas et al., 2021). Several researchers have conducted different metal (loid)s removal studies and they have used various types of adsorbents such as activated carbon, carbon nanotubes, and biosorbents like algal biomass, fungal biomass, bacterial adsorbent, and agricultural residues (Table 6).
3.7.1 Activated carbon adsorbents
Adsorbents based on activated carbon are commonly utilized to remove metal(loid)s. Its utility stems mostly from its enormous micropore and mesopore contents, as well as the consequent high surface area. Many researchers are investigating the application of alternate current for the removal of metal(loid)s (De Gisi et al., 2016; Lucaci et al., 2019; Petrovic et al., 2021).
3.7.2 Carbon nanotubes adsorbents
CNTs (carbon nanotubes) were discovered by Iijima in 1991 and received great attention due to their exceptional characteristics and uses. Because of their excellent characteristics and applications, CNTs adsorbents are extensively employed in the removal of metal(loid)s (Carolin et al., 2017). CNTs have shown a higher ability for eradicating Pb Cd, Cr, Cu, and Ni (Badawi et al., 2021). There are two kinds of carbon nanotubes (CNTs): 1) single-walled CNTs (SWCNTs) and 2) multi-walled CNTs (MWCNTs) (Odom et al., 1998). These methods are complex due to electrostatic force, precipitation, and chemical interface amid metal(loid)s ions and CNT external functional sets. The presence of carbon nanotubes might influence the metabolic action of microorganisms, posing a health hazard to humans (Tran and Mulchandani, 2016; Arora and Attri, 2020; Rahamathulla et al., 2021). With regards to sensitive detection a novel technology, the Nanobiosensor, and associated advancements are very effective and in trend to detect trace amounts of pollutants (Figure 5). The combination of biology with nanotechnology has allowed the sensing technology to enable faster, cheaper as well as more reliable evaluations and applications in various scientific domains specifically metal (loid)s detection.
3.7.3 Biosorbents
Biosorption is a novel technology for eliminating metal(loid)s from wastewater. This technique is thought to be an operational and detoxifying procedure even at low concentrations of metal(loid)s. Biosorption is a type of adsorption procedure that involves both solid and liquid states. To remove metal(loid)s, both viable and nonviable biological organisms are required (Saravanan et al., 2022). The main benefit of employing dead material over viable material is that it does not need any growing medium. Because of their high effectiveness and cheap cost, possible sorbents such as bacteria, yeast, fungus, algae, sawdust, seed shells, sugar beet pectin gels, and potato peels are utilized in this process (Bădescu et al., 2018; Okoro et al., 2022; Savastru et al., 2022).
3.7.3.1 Algal biomass
The investigators have focused on both living and non-living algal biomasses for metal(loid)s remediation (Diep et al., 2018). The adsorption capability of living biomass is restricted in metal(loid)s elimination due to the adsorption procedure occurs during the growth phase and metal(loid)s absorption occurs during this phase alone, which is regarded as an intracellular procedure with more sophisticated adsorption mechanisms (Gupta and Diwan, 2017; Igiri et al., 2018; Medfu Tarekegn et al., 2020). However, for non-living algal biomass, the extracellular procedure occurs because the metal(loid)s become adsorbent on the cell wall. The adsorption ability of non-living algae could be influenced by pH, temperature, and interaction duration, among others (Mohammed et al., 2019).
3.7.3.2 Fungal biomass
Due to the higher fraction of cell wall components in fungal biomass, it has a high sorbing ability. It can grow in its native habitat (Garcia-Rubio et al., 2020). The cell wall is composed of chitin, glucan, mannan, proteins, and other polymers, which increase the fungi’s adsorption prospective (Blaga et al., 2021). Metal (loid)s can be absorbed by fungi via intracellular precipitation, valence conversion, ion exchange, and complexation (Ayangbenro and Babalola, 2017).
3.7.3.3 Bacterial adsorbent
The wastewater treatment via bacterial adsorbent is an excellent biological method. Bacteria have features such as lower size, accessibility, and tractability, which prompts investigators to emphasize microbial adsorbents for metal (loid)s removal (Younas et al., 2021). Bacterial cell walls include functional groups such as ketones, aldehydes, and carboxyl groups and its biomass is typically utilized as a binding or supportive material to the adsorbent for metal(loid)s removal (El-Naggar et al., 2018).
3.7.3.4 Agricultural residues or plant material
Although the adsorbents mentioned above are cheap and renewable, they may have a disposal difficulty and the least commercial benefit. As a result, the researchers focused primarily on the usage of cost-effective adsorbents such as agricultural residues, industrial by-products, and natural compounds for metal(loid)s remediation. It develops an alternative to activated carbon by employing these low-cost adsorbents. The application of low-cost adsorbents is being inspected as a low neutralization method for treating wastewater (Saleem et al., 2019; Sabzehmeidani et al., 2021). The use of agricultural residues in the biosorption technique is an eco-friendly procedure. These agricultural residues or plant materials act as a replacement for traditional adsorbents (Torres, 2020). It is a suitable choice for metal(loid)s removal because the waste becomes more efficient after using these agricultural residues. Agricultural waste such as cashew nut shells, palm oil fruit shells, orange peel, kenaf fiber, and barley straw may be applied for the recovery of metal(loid)s from wastewater (Kwikima et al., 2021).
3.8 Sustainable green technologies for Metal(loid)s clean-up
The sustainable biotic approach to remove metal(loid)s includes: 1) using microbes to detoxify metal(loid)s through valence conversion, extracellular chemical precipitation, or volatilization, and 2) using specific plants to purify the water or soil by deactivating metal (loid)s in the rhizospheric zone or transfer them to the above-ground parts of plants. Phytoremediation is an attractive choice with the respect to the cost as many times the use of selective absorbents or tailoring agents or modification agents etc. made other techniques comparatively cost-enhancing (Sharma et al., 2016). It may remove metal(loid)s, radionuclides, and organic contaminants. Phytoremediation is an inexpensive, proficient, environmentally friendly, and in-situ technology (Nedjimi, 2021). The capability to accumulate metal(loid)s varies substantially between plant species because each plant species has a specific mechanism to remove metal(loid)s ions based on genetic, morphological, physiological, and anatomical features (Kumar Yadav et al., 2018; Suman et al., 2018). Depending on the remediation technique, several phytoremediation approaches such as phytoextraction, rhizofiltration, phytostabilization, and phytovolatilization are illustrated in Figure 6 and their strategy is presented in Figure 7. Some specific plants species used in the phytoremediation technology for clean-up of specific metal(loid)s are demonstrated in Table 7.
3.8.1 Phytoextraction
It is a method in which plants absorb, translocate, and store metal(loid)s in their below-ground and above-ground parts. The plants are then either burned or composted to recover the metal(loid)s. If plants are burned, the ash essentially is discarded in a hazardous waste landfill (Sarwar et al., 2017; Lee et al., 2021). Benavides et al. (2021) evaluated Cd accumulating species: Helianthus annuus, Brassica napus, and Chyrsopogon zizanioides. The plants were cultivated in two pot trials, each with a distinct Cd-amended growth medium: (sand + perlite) and natural soil. The total Cd and Cd absorption in shoot biomass augmented linearly with increasing levels of additional Cd in both studies. Plant total Cd was highest in Brassica napus, whereas Cd absorption in shoot biomass was highest in Helianthus annuus. Jacobs et al. (2018) used Noccaea caerulescensis to remediate Zn in the field and demonstrated that the concentration of Zn in the plant’s leaves exceeded 300 g Cd ha−1 in 2 months. Fourati et al. (2016) removed Ni using Sesuvium portulacastrum by phytoextraction and showed that Ni was accumulated up to 1,050 μg g−1 dry weight in the shoots of the selected plant. Herzig et al. (2014) conducted a Zn phytoextraction study using tobacco (Nicotiana tabacum) and sunflower (Helianthus annuus). The experiment was carried out for 5 years. The findings demonstrated that both plants exhibited good phytoextraction efficiency for Zn. The concentration of Zn was easily reduced by 45–70% in soil. Macci et al. (2013) evaluated the clean-up of a contaminated industrial region using a combination of metal(loid)s, hydrocarbons, and polychlorinated biphenyls. Populus sp., Paulownia tomentosa, and Cytisus scoparius were planted among naturally occurring plants with the addition of horse manure. The vegetation of Populus sp., Paulownia tomentosa, and Cytisus scoparius reduced pollution by up to 35, 40, and 70% for metal (loid)s, hydrocarbons, and polychlorinated biphenyls (PCBs), respectively in 2 years. Tlustoš et al. (2006) conducted a phytoextraction experiment for As, Cd, Pb, and Zn using Melilotus alba, Trifolium Pratense, Malva verticillata, Carthamus tinctorius, and Cannabis sativa. The findings showed that the selected plants efficiently removed As, Cd, Pb, and Zn, and the highest efficiency was demonstrated by Carthamus tinctorius.
3.8.2 Rhizofiltration/Rhizodegradation
Rhizofiltration is the adsorption or precipitation of metal (loid)s onto plant roots. This technique is employed to clean the contaminated water rather than soil (Subba Rao et al., 2022). The plants are grown with roots immersed in water. The contaminated water is delivered to the plants, or the plants are put in the contaminated region, when the roots absorb the water and pollutants dissolved in it, plants are collected (Tiwari et al., 2018). Kumar and Fulekar (2022) demonstrated the rhizoremediation capability of Pennisetum pedicellatum for Cd removal in a pot culture experiment and the findings indicated that the selected plant species was effectively removed 83% of Cd within 2 months. Han et al. (2021) used uranium-polluted water and Lactuca sativa, Brassica campestris L., Raphanus sativus L., and Oenanthe javanica in a rhizofiltration study. The findings showed that as the U concentration in the groundwater increases, so does in the plant roots. Kodituwakku and Yatawara (2020) used Eichhornia crassipes, Salvinia molesta, and Pistia stratiotes to eliminate Cu, Cr, Cd, Ni, and Zn from industrial sludge. The findings indicated that S. molesta exhibited the highest reductions of Zn (36.0%), Fe (26.6%), Cu (32.6%), Cr (58.6%), and Ni, (26.9%) whereas P. startiotes and E. crassipes, respectively, displayed the highest Cd (27.1%) and Pb (42.4%) reductions. Yang et al. (2015) demonstrated that metals like U and Cs were efficiently removed from the groundwater using Phaseolus vulgaris. Oustriere et al. (2017) specified that the Arundo donax is a proficient candidate to remove Cu from the constructed wetlands using the rhizofiltration technique. Vera Tomé et al. (2008) removed U and Ra (226) using sunflower (Helianthus annuus) and they reported that the rhizofiltration process was responsible for the elimination of metals. They reported 50 % and 70% of U and Ra (226) removal after 2 days, respectively.
3.8.3 Phytostabilization
In this technique, plants immobilize contaminants in soil and water by root absorption, accumulation, adsorption, or precipitation inside the root zone of the plants. This technique decreases pollutant mobility and inhibits relocation to water or air, as well as bioavailability for entrance into the food chain (Radziemska et al., 2017). This method may be applied to restore a vegetative cover in areas where natural vegetation has been lost owing to high metal(loid)s concentrations. Metal(loid)s-tolerant plants are utilized to decrease the possibility of pollutants movement by wind erosion and transfer of surface soils, as well as soil contamination seeping into groundwater (Shackira and Puthur, 2019). Sarath et al. (2022) attempted a phytostabilization experiment to remove As by Acanthus ilicifolius L. and described that this plant species to variable concentrations of As is the result of morpho-physiological and anatomical characteristics, which make the plant favourable for As removal, particularly in wetlands. Mataruga et al. (2020) assessed Salix alba, Juglans regia, and Populus nigra for the biomonitoring of trace elements in the Sava River. The concentrations of As, Cd, Cr, Cu, Ni, Pb, and Zn were analyzed in the soils, roots, and leaves of plants. The findings demonstrated that Salix alba is a beneficial plant species for the phytostabilization of Cd and Cu. Zgorelec et al. (2020) determined the removal potential of Miscanthus × giganteus (MxG) for Cd and Hg. The concentrations of Cd (0, 10, and 100 mg kg−1 soil) and Hg (0, 2, and 20 mg kg−1 soil) were supplemented to the soil. MxG was able to accumulate (293.8 µg Cd and 4.7 µg Hg) in shoots. The findings suggested that Miscanthus × giganteus (MxG) is a potential species for phytostabilization on soils polluted with Cd and Hg. Bacchetta et al. (2018) specified that the uptake of Zn, Cd, and Pb was limited mainly in the roots of Helichrysum microphyllum which is appropriate for the phytostabilization process. Al Chami et al. (2015) evaluated the removal efficiency of Sorghum bicolor and Carthamus tinctorius for Ni, Pb, and Zn. The findings specified that both the species were capable to accumulate these elements in their roots.
3.8.4 Phytovolatilization
In this technique, plants absorb contaminants and transform them into less toxic volatile forms. The contaminants are absorbed by the roots, transferred to the shoot, then volatized in the atmosphere via the stomata of leaves (Nedjimi, 2021). Phytovolatilization has been applied to remove volatile elements such as Hg and Se (Cristaldi et al., 2017). Mahar et al. (2016) stated that this technology may be implemented to remove different organic contaminants and metal(loid)s such as Se, As, and Hg from the environment. Guarino et al. (2020) described that Arundo donax has the potential to volatilize more than 50% of As aided by Stenotrophomonas maltophilia and Rhyzobacterium sp. Moreno et al. (2008) described Hg removal using Brassica juncea (L.) grown in hydroponic conditions with Hg concentrations of 0–10 mg/L. The findings concluded that Brassica juncea has the potential to remove Hg up to 95% by phytovolatilization. The volatilization of Hg mainly happened from the roots in the Hg (0) vapour form.
3.9 Advantages and limitations of phytoremediation technology
3.9.1 Advantages
The phytoremediation technology is efficient with lots of benefits (Figure 8).
1. Phytoremediation is less expensive since it does not need the purchase of large equipment.
2. Planting trees on contaminated sites make these areas more attractive.
3. Plants can be grown quickly and simply with little effort, and they can also be checked effortlessly.
4. The precious metal(loid)s can be recovered and reused.
5. The phytoremediation technology uses natural organisms to protect the environment, thus it is potentially the least destructive technology.
6. It protects the topsoil and thus maintains soil fertility.
7. It increases the plant phytochemicals and improves the health of soil and yield.
3.9.2 Limitations of phytoremediation
Phytoremediation is simple to use and cost-efficient, however, it does have significant technical limitations (Figure 9).
1. Phytoremediation just relocates hazardous metal (loid)s rather than complete removal.
2. The zone and depth engaged by the roots are the only areas where phytoremediation may take place.
3. A long-term commitment is required due to the slow growth of the plants.
4. It’s impossible to prevent metal(loid)s from seeping into groundwater using this technique.
5. To be accessible to the roots, the contaminant must be present inside the root zone.
6. The pollutants may transfer to the food chain during phytoremediation.
7. The phytoremediation technique is less efficient for the higher concentration of contaminants.
3.10 Future prospects of phytoremediation technology
Phytoremediation is the most favourable technology for the eco-restoration of contaminated environments, however, more studies and analyses are necessary to develop our understanding of effective phytoremediation of metal(loid)s. More study into metal(loid)s remediation would contribute insights into phytotoxicity limits, and genetic engineering may enable plants to withstand larger metal(loid)s concentrations. In field trials, it is essential to look for new approaches to understand the processes, metabolites, and genes employing cutting-edge omics techniques. Further study in the following areas looks to be worthwhile in the future to secure further advantages.
i. Identification of potential plants containing compounds that may prevent herbivores, followed by transformation of such plants with altered or increased metal tolerance capacities; such a system will aid in avoiding metal transmission to the food chain.
ii. Transgenic phytoremediation experiments must be applied in the contaminated areas to deal with the metal (loid)s as well as other types of pollutants.
iii. A multigene technique that involves the parallel delivery of numerous genes into eligible candidate plants could aid in the removal of pollutants.
iv. Cropping system identification with targeting elements and site-based phytoremediation.
v. Farmers, local populations, academics, industrial sectors, and environmental specialists may contribute to the success and dependability of phytoremediation through teaching initiatives, ensuring the long-term sustainability of this environmentally beneficial green technology.
4 Conclusion
The presence of metal(loid)s in the environment is a challenging issue associated with ecological and human health and their clean-up is a global concern that needs to be addressed. The understanding of metal(loid)s contamination concerning their recent global scenario, sources, the necessities for removal, and impacts on human health and the ecosystem are very important to predict the future strategies to deal with this problem. According to the findings, anthropogenic activities have considerably contributed to excessive quantities of metal(loid)s discharge into the environment. Various technologies to eliminate these harmful metal(loid)s from the environment are significant and functional according to the need, types, and conditions of remediation sites. However, the search for better clean-up technologies either in the combination or modification of existing ones or new technologies with cutting-edge scientific advancements is the need of the hour. Selective technologies like phytoremediation, ion exchange, membrane filtration, and adsorption, all have benefits and drawbacks when it comes to metal(loid)s removal from the environment. A systematic understanding of metal (loid)s sources, their chemistry, and possible hazard to the ecosystem and humans are required to choose a proficient clean-up technology and its proper management to make better strategies and sustainable policies for the future.
Author contributions
PK: Methodology, Writing- Original Draft. AG: Supervision. MA: Conceptualization, Methodology, Writing- Original Draft. VY: Writing- Original Draft, Formal analysis. SS: Formal analysis, Resources, Writing- Review, and Editing. VD: Writing- Original Draft, Formal analysis. MA: Writing- Original Draft, Formal analysis. KY: Writing- Original Draft, Formal analysis. SP: Data curation, Formal analysis. PM: Formal analysis, Writing- Review, and Editing. MC-P: Formal analysis, Writing- Review, and Editing. YA: Formal analysis, Writing- Review, and Editing. B-HJ: Supervision, Formal analysis.
Funding
The authors extend their appreciation to the Deanship of Scientific Research at King Khalid University, Saudi Arabia for funding this work through Small Groups Project under grant number RGP.1/62/43. This work was supported by the National Research Foundation of Korea (NRF) grant funded by the Korea government (MSIT) (2022R1C1C2003376). Marina Cabral Pinto thanks FCT (UIDB/04035/2020).
Conflict of interest
The authors declare that the research was conducted in the absence of any commercial or financial relationships that could be construed as a potential conflict of interest.
Publisher’s note
All claims expressed in this article are solely those of the authors and do not necessarily represent those of their affiliated organizations, or those of the publisher, the editors and the reviewers. Any product that may be evaluated in this article, or claim that may be made by its manufacturer, is not guaranteed or endorsed by the publisher.
References
Abdel -Aty, A. M., Ammar, N. S., Abdel Ghafar, H. H., and Ali, R. K. (2013). Biosorption of cadmium and lead from aqueous solution by fresh water alga Anabaena sphaerica biomass. J. Adv. Res. 4, 367–374. doi:10.1016/j.jare.2012.07.004
Abdel-Fatah, M. A. (2018). Nanofiltration systems and applications in wastewater treatment: Review article. Ain Shams Eng. J. 9, 3077–3092. doi:10.1016/j.asej.2018.08.001
Ahmad, A. L., and Ooi, B. S. (2010). A study on acid reclamation and copper recovery using low pressure nanofiltration membrane. Chem. Eng. J. 156, 257–263. doi:10.1016/j.cej.2009.10.014
Ahmaruzzaman, M. (2009). Role of fly ash in the removal of organic pollutants from wastewater. Energy fuels. 23, 1494–1511. doi:10.1021/ef8002697
Ahmed, M. J. K., and Ahmaruzzaman, M. (2016). A review on potential usage of industrial waste materials for binding heavy metal ions from aqueous solutions. J. Water Process Eng. 10, 39–47. doi:10.1016/j.jwpe.2016.01.014
Ahmed, S. F., Kumar, P. S., Rozbu, M. R., Chowdhury, A. T., Nuzhat, S., and Rafa, N. (2022). Heavy metal toxicity, sources, and remediation techniques for contaminated water and soil. Environ. Technol. Innov. 25, 102114. doi:10.1016/j.eti.2021.102114
Akhtar, N., Syakir Ishak, M. I., Bhawani, S. A., and Umar, K. (2021). Various natural and anthropogenic factors responsible for water quality degradation: A review. Water 13, 660. doi:10.3390/w13192660
Al Chami, Z., Amer, N., Al Bitar, L., and Cavoski, I. (2015). Potential use of Sorghum bicolor and Carthamus tinctorius in phytoremediation of nickel, lead and zinc. Int. J. Environ. Sci. Technol. 12, 3957–3970. doi:10.1007/s13762-015-0823-0
Alengebawy, A., Abdelkhalek, S. T., Qureshi, S. R., and Wang, M.-Q. (2021). Heavy metals and pesticides toxicity in agricultural soil and plants: Ecological risks and human health implications. Toxics 9, 42. doi:10.3390/toxics9030042
Algieri, C., Chakraborty, S., and Candamano, S. (2021). A way to membrane-based environmental remediation for heavy metal removal. Environ 8, 52. doi:10.3390/environments8060052
Ali, H., Khan, E., and Ilahi, I. (2019). Environmental chemistry and ecotoxicology of hazardous heavy metals: Environmental persistence, toxicity, and bioaccumulation. J. Chem. 2019, 6730305. doi:10.1155/2019/6730305
Arora, B., and Attri, P. (2020). Carbon nanotubes (CNTs): A potential nanomaterial for water purification. J. Compos. Sci. 4, 135. doi:10.3390/jcs4030135
Ashraf, M. A., Wajid, A., Mahmood, K., Maah, M. J., and Yusoff, I. (2011). Low cost biosorbent banana peel (Musa sapientum) for the removal of heavy metals. Sci. Res. Essays 6, 4055–4064. doi:10.5897/sre11.303
Awasthi, S., Chauhan, R., Srivastava, S., and Tripathi, R. D. (2017). The journey of arsenic from soil to Grain in Rice. Front. Plant Sci. 8, 1007. doi:10.3389/fpls.2017.01007
Ayangbenro, A. S., and Babalola, O. O. (2017). A new strategy for heavy metal polluted environments: A review of microbial biosorbents. Int. J. Environ. Res. Public Health 14, 94. doi:10.3390/ijerph14010094
Bacchetta, G., Boi, M. E., Cappai, G., De Giudici, G., Piredda, M., and Porceddu, M. (2018). Metal tolerance capability of Helichrysum microphyllum cambess. subsp. tyrrhenicum bacch., brullo & giusso: A candidate for phytostabilization in abandoned mine sites. Bull. Environ. Contam. Toxicol. 101, 758–765. doi:10.1007/s00128-018-2463-9
Badawi, A. K., Abd Elkodous, M., and Ali, G. A. M. (2021). Recent advances in dye and metal ion removal using efficient adsorbents and novel nano-based materials: An overview. RSC Adv. 11, 36528–36553. doi:10.1039/D1RA06892J
Bădescu, I. S., Bulgariu, D., Ahmad, I., and Bulgariu, L. (2018). Valorisation possibilities of exhausted biosorbents loaded with metal ions – a review. J. Environ. Manage. 224, 288–297. doi:10.1016/j.jenvman.2018.07.066
Balali-Mood, M., Naseri, K., Tahergorabi, Z., Khazdair, M. R., and Sadeghi, M. (2021). Toxic mechanisms of five heavy metals: Mercury, lead, chromium, cadmium, and arsenic. Front. Pharmacol. 12. doi:10.3389/fphar.2021.643972
Bashir, I., Lone, F. A., Bhat, R. A., Mir, S. A., Dar, Z. A., and Dar, S. A. (2020). “Concerns and threats of contamination on aquatic ecosystems,” in Bioremediation biotechnol. Sustain. Approaches to pollut. Degrad., 1–26. doi:10.1007/978-3-030-35691-0_1
Benavides, B. J., Drohan, P. J., Spargo, J. T., Maximova, S. N., Guiltinan, M. J., and Miller, D. A. (2021). Cadmium phytoextraction by Helianthus annuus (sunflower), Brassica napus cv Wichita (rapeseed), and Chyrsopogon zizanioides (vetiver). Chemosphere 265, 129086. doi:10.1016/j.chemosphere.2020.129086
Blaga, A. C., Zaharia, C., and Suteu, D. (2021). Polysaccharides as support for microbial biomass-based adsorbents with applications in removal of heavy metals and dyes. Polym. (Basel) 13, 893. doi:10.3390/polym13172893
Boretti, A., and Rosa, L. (2019). Reassessing the projections of the world water development report. npj Clean. Water 2, 15. doi:10.1038/s41545-019-0039-9
Briffa, J., Sinagra, E., and Blundell, R. (2020). Heavy metal pollution in the environment and their toxicological effects on humans. Heliyon 6, e04691. doi:10.1016/j.heliyon.2020.e04691
Carolin, C. F., Kumar, P. S., Saravanan, A., Joshiba, G. J., and Naushad, M. (2017). Efficient techniques for the removal of toxic heavy metals from aquatic environment: A review. J. Environ. Chem. Eng. 5, 2782–2799. doi:10.1016/j.jece.2017.05.029
Cechinel, M. A. P., Ulson de Souza, S. M. A. G., and Ulson de Souza, A. A. (2014). Study of lead (II) adsorption onto activated carbon originating from cow bone. J. Clean. Prod. 65, 342–349. doi:10.1016/j.jclepro.2013.08.020
Chheang, L., Thongkon, N., Sriwiriyarat, T., and Thanasupsin, S. P. (2021). Heavy metal contamination and human health implications in the chan thnal reservoir, Cambodia. Sustain. 13, 13538. doi:10.3390/su132413538
Chibuike, G. U., and Obiora, S. C. (2014). Heavy metal polluted soils: Effect on plants and bioremediation methods. Appl. Environ. soil Sci. 2014, 752708. doi:10.1155/2014/752708
Chiroma, T. M., Ebewele, R. O., and Hymore, F. K. (2014). Comparative assessment of heavy metal levels in soil, vegetables and urban grey waste water used for irrigation in Yola and Kano. Int. refereed J. Eng. Sci. 3 (2), 01–09.
Concas, S., Lattanzi, P., Bacchetta, G., Barbafieri, M., and Vacca, A. (2015). Zn, Pb and Hg contents of Pistacia lentiscus L. grown on heavy metal-rich soils: Implications for phytostabilization. Water. Air. Soil Pollut. 226, 340. doi:10.1007/s11270-015-2609-x
Crichton, R. R. (2017). “Metal toxicity - an introduction,” in RSC metallobiology (The Royal Society of Chemistry), 1–23. doi:10.1039/9781782623892-00001
Crini, G., and Lichtfouse, E. (2019). Advantages and disadvantages of techniques used for wastewater treatment. Environ. Chem. Lett. 17, 145–155. doi:10.1007/s10311-018-0785-9
Cristaldi, A., Conti, G. O., Jho, E. H., Zuccarello, P., Grasso, A., Copat, C., et al. (2017). Phytoremediation of contaminated soils by heavy metals and PAHs. A brief review. Environ. Technol. Innov. 8, 309–326. doi:10.1016/j.eti.2017.08.002
Cristian, P., Violeta, P., Anita-Laura, R., Raluca, I., Alexandrescu, E., Andrei, S., et al. (2015). Removal of zinc ions from model wastewater system using bicopolymer membranes with fumed silica. J. Water Process Eng. 8, 1–10. doi:10.1016/j.jwpe.2015.08.001
da Silva, A. A., de Oliveira, J. A., de Campos, F. V., Ribeiro, C., Farnese, F., dos, S., et al. (2018). Phytoremediation potential of Salvinia molesta for arsenite contaminated water: Role of antioxidant enzymes. Theor. Exp. Plant Physiol. 30, 275–286. doi:10.1007/s40626-018-0121-6
De Gisi, S., Lofrano, G., Grassi, M., and Notarnicola, M. (2016). Characteristics and adsorption capacities of low-cost sorbents for wastewater treatment: A review. Sustain. Mat. Technol. 9, 10–40. doi:10.1016/j.susmat.2016.06.002
Dehaine, Q., Tijsseling, L. T., Glass, H. J., Törmänen, T., and Butcher, A. R. (2021). Geometallurgy of cobalt ores: A review. Min. Eng. 160, 106656. doi:10.1016/j.mineng.2020.106656
Delshab, H., Farshchi, P., and Keshavarzi, B. (2016). Geochemical distribution, fractionation and contamination assessment of heavy metals in marine sediments of the Asaluyeh port, Persian Gulf. Mar. Pollut. Bull. 115 (1–2), 401–411. doi:10.1016/j.marpolbul.2016.11.033
Demim, S., Drouiche, N., Aouabed, A., Benayad, T., Dendene-Badache, O., and Semsari, S. (2013). Cadmium and nickel: Assessment of the physiological effects and heavy metal removal using a response surface approach by L. gibba. Ecol. Eng. 61, 426–435. doi:10.1016/j.ecoleng.2013.10.016
Denneman, C. A., and Robberse, J. G. (1990). “Ecotoxicological risk assessment as a base for development of soil quality criteria,” in Contaminated Soil’90 (Dordrecht: Springer), 157–164. doi:10.1007/978-94-011-3270-1_28
Dialynas, E., and Diamadopoulos, E. (2009). Integration of a membrane bioreactor coupled with reverse osmosis for advanced treatment of municipal wastewater. Desalination 238, 302–311. doi:10.1016/j.desal.2008.01.046
Diep, P., Mahadevan, R., and Yakunin, A. F. (2018). Heavy metal removal by bioaccumulation using genetically engineered microorganisms. Front. Bioeng. Biotechnol. 6, 157. doi:10.3389/fbioe.2018.00157
Dixit, F., Dutta, R., Barbeau, B., Berube, P., and Mohseni, M. (2021). PFAS removal by ion exchange resins: A review. Chemosphere 272, 129777. doi:10.1016/j.chemosphere.2021.129777
Egbosiuba, T. C., Abdulkareem, A. S., Kovo, A. S., Afolabi, E. A., Tijani, J. O., Bankole, M. T., et al. (2021). Adsorption of Cr(VI), Ni(II), Fe(II) and Cd(II) ions by KIAgNPs decorated MWCNTs in a batch and fixed bed process. Sci. Rep. 11, 75. doi:10.1038/s41598-020-79857-z
Egorova, K. S., and Ananikov, V. P. (2017). Toxicity of metal compounds: Knowledge and myths. Organometallics 36, 4071–4090. doi:10.1021/acs.organomet.7b00605
Eissa, M. A. (2017). Phytoextraction mechanism of Cd by Atriplex lentiformis using some mobilizing agents. Ecol. Eng. 108, 220–226. doi:10.1016/j.ecoleng.2017.08.025
El Batouti, M., Al-Harby, N. F., and Elewa, M. M. (2021). A review on promising membrane technology approaches for heavy metal removal from water and wastewater to solve water crisis. Water 13, 241. doi:10.3390/w13223241
El-Naggar, N. E. A., Hamouda, R. A., Mousa, I. E., Abdel-Hamid, M. S., and Rabei, N. H. (2018). Biosorption optimization, characterization, immobilization and application of Gelidium amansii biomass for complete Pb2+ removal from aqueous solutions. Sci. Rep. 8, 13456. doi:10.1038/s41598-018-31660-7
Elbasiouny, H., Darwesh, M., Elbeltagy, H., Abo-alhamd, F. G., Amer, A. A., Elsegaiy, M. A., et al. (2021). Ecofriendly remediation technologies for wastewater contaminated with heavy metals with special focus on using water hyacinth and black tea wastes: A review. Environ. Monit. Assess. 193, 449. doi:10.1007/s10661-021-09236-2
Estay, H., Barros, L., and Troncoso, E. (2021). Metal sulfide precipitation: Recent breakthroughs and future outlooks. Minerals 11. doi:10.3390/min11121385
Farjana, S. H., Huda, N., and Mahmud, M. A. P. (2019). Life cycle assessment of cobalt extraction process. J. Sustain. Min. 18, 150–161. doi:10.1016/j.jsm.2019.03.002
Farooq, U., Kozinski, J. A., Khan, M. A., and Athar, M. (2010). Biosorption of heavy metal ions using wheat based biosorbents – a review of the recent literature. Bioresour. Technol. 101, 5043–5053. doi:10.1016/j.biortech.2010.02.030
Fathollahi, A., Khasteganan, N., Coupe, S. J., and Newman, A. P. (2021). A meta-analysis of metal biosorption by suspended bacteria from three phyla. Chemosphere 268, 129290. doi:10.1016/j.chemosphere.2020.129290
Feria-Díaz, J. J., Correa-Mahecha, F., López-Méndez, M. C., Rodríguez-Miranda, J. P., and Barrera-Rojas, J. (2021). Recent desalination technologies by hybridization and integration with reverse osmosis: A review. Water 13, 1369. doi:10.3390/w13101369
Figoli, A., Cassano, A., Criscuoli, A., Mozumder, M. S. I., Uddin, M. T., Islam, M. A., et al. (2010). Influence of operating parameters on the arsenic removal by nanofiltration. Water Res. 44, 97–104. doi:10.1016/j.watres.2009.09.007
Fourati, E., Wali, M., Vogel-Mikuš, K., Abdelly, C., and Ghnaya, T. (2016). Nickel tolerance, accumulation and subcellular distribution in the halophytes Sesuvium portulacastrum and Cakile maritima. Plant Physiol. biochem. 108, 295–303. doi:10.1016/j.plaphy.2016.07.024
Frérot, H., Lefèbvre, C., Gruber, W., Collin, C., Santos, A. D., and Escarré, J. (2006). Specific interactions between local metallicolous plants improve the phytostabilization of mine soils. Plant Soil 282, 53–65. doi:10.1007/s11104-005-5315-4
Fu, Z., Guo, W., Dang, Z., Hu, Q., Wu, F., Feng, C., et al. (2017). Refocusing on nonpriority toxic metals in the aquatic environment in China. Environ. Sci. Technol. 51, 3117–3118. doi:10.1021/acs.est.7b00223
Gao, J., Liu, F., Ling, P., Lei, J., Li, L., Li, C., et al. (2013). High efficient removal of Cu(II) by a chelating resin from strong acidic solutions: Complex formation and DFT certification. Chem. Eng. J. 222, 240–247. doi:10.1016/j.cej.2013.02.055
Garcia-Rubio, R., de Oliveira, H. C., Rivera, J., and Trevijano-Contador, N. (2020). The fungal cell wall: Candida, cryptococcus, and Aspergillus species. Front. Microbiol. 10, 02993. doi:10.3389/fmicb.2019.02993
Genchi, G., Carocci, A., Lauria, G., Sinicropi, M. S., and Catalano, A. (2020a). Nickel: Human health and environmental toxicology. Int. J. Environ. Res. Public Health 17, 679. doi:10.3390/ijerph17030679
Genchi, G., Sinicropi, M. S., Lauria, G., Carocci, A., and Catalano, A. (2020b). The effects of cadmium toxicity. Int. J. Environ. Res. Public Health 17, 3782. doi:10.3390/ijerph17113782
Ghosh, P., Samanta, A. N., and Ray, S. (2011). Reduction of COD and removal of Zn2+ from rayon industry wastewater by combined electro-Fenton treatment and chemical precipitation. Desalination 266, 213–217. doi:10.1016/j.desal.2010.08.029
Gonzalez Henao, S., and Ghneim-Herrera, T. (2021). Heavy metals in soils and the remediation potential of bacteria associated with the plant microbiome. Front. Environ. Sci. 15, 4216. doi:10.3389/fenvs.2021.604216
Grzeszczak, K., Kwiatkowski, S., and Kosik-Bogacka, D. (2020). The role of Fe, Zn, and Cu in pregnancy. Biomolecules 10, 1–33. doi:10.3390/biom10081176
Guarino, F., Miranda, A., Castiglione, S., and Cicatelli, A. (2020). Arsenic phytovolatilization and epigenetic modifications in Arundo donax L. assisted by a PGPR consortium. Chemosphere 251, 126310. doi:10.1016/j.chemosphere.2020.126310
Gülay, A., Çekiç, Y., Musovic, S., Albrechtsen, H. J., and Smets, B. F. (2018). Diversity of iron oxidizers in groundwater-fed rapid sand filters: Evidence of Fe(II)-Dependent growth by curvibacter and undibacterium spp. Front. Microbiol. 9, 2808. doi:10.3389/fmicb.2018.02808
Günes, E., Çifçi, D. I., Dinçer, A. R., and Günes, Y. (2021). Removal of cod, aromaticity and color of a pretreated chemical producing industrial wastewater: A comparison between adsorption, ozonation, and advanced oxidation processes. Turk. J. Chem. 45, 551–565. doi:10.3906/kim-2010-48
Gupta, A., and Balomajumder, C. (2015). Biosorptive performance of Escherichia coli supported on waste tea biomass (wtb) for removal of Cr(VI) to avoid the contamination of ground water: A comparative study between biosorption and SBB system. Groundw. Sustain. Dev. 1, 12–22. doi:10.1016/j.gsd.2016.01.001
Gupta, N., Yadav, K. K., Kumar, V., Kumar, S., Chadd, R. P., and Kumar, A. (2019). Trace elements in soil-vegetables interface: Translocation, bioaccumulation, toxicity and amelioration - a review. Sci. Total Environ. 651, 2927–2942. doi:10.1016/j.scitotenv.2018.10.047
Gupta, P., and Diwan, B. (2017). Bacterial exopolysaccharide mediated heavy metal removal: A review on biosynthesis, mechanism and remediation strategies. Biotechnol. Rep. 13, 58–71. doi:10.1016/j.btre.2016.12.006
Gurreri, L., Tamburini, A., Cipollina, A., and Micale, G. (2020). Electrodialysis applications in wastewater treatment for environmental protection and resources recovery: A systematic review on progress and perspectives. Membr. (Basel) 10, 1–93. doi:10.3390/membranes10070146
Haider, F. U., Liqun, C., Coulter, J. A., Cheema, S. A., Wu, J., Zhang, R., et al. (2021). Cadmium toxicity in plants: Impacts and remediation strategies. Ecotoxicol. Environ. Saf. 211, 111887. doi:10.1016/j.ecoenv.2020.111887
Hajahmadi, Z., Younesi, H., Bahramifar, N., Khakpour, H., and Pirzadeh, K. (2015). Multicomponent isotherm for biosorption of Zn(II), CO(II) and Cd(II) from ternary mixture onto pretreated dried Aspergillus niger biomass. Water Resour. Ind. 11, 71–80. doi:10.1016/j.wri.2015.07.003
Han, W., Fu, F., Cheng, Z., Tang, B., and Wu, S. (2016). Studies on the optimum conditions using acid-washed zero-valent iron/aluminum mixtures in permeable reactive barriers for the removal of different heavy metal ions from wastewater. J. Hazard. Mat. 302, 437–446. doi:10.1016/j.jhazmat.2015.09.041
Han, Y., Lee, J., Kim, C., Park, J., Lee, M., and Yang, M. (2021). Uranium rhizofiltration by Lactuca sativa, Brassica campestris L., Raphanus sativus L., Oenanthe javanica under different hydroponic conditions. Minerals 11, 1–14. doi:10.3390/min11010041
Hashem, M. A., Nur-A-Tomal, M. S., and Mondal, N. R. (2017). Hair burning and liming in tanneries is a source of pollution by arsenic, lead, zinc, manganese and iron. Environ. Chem. Lett. 15, 501–506. doi:10.1007/s10311-017-0634-2
Hassanvand, A., Wei, K., Talebi, S., Chen, G. Q., and Kentish, S. E. (2017). The role of ion exchange membranes in membrane capacitive deionisation. Membr. (Basel). 7, 54. doi:10.3390/membranes7030054
Hernández-Camacho, J. D., Vicente-García, C., Parsons, D. S., and Navas-Enamorado, I. (2020). Zinc at the crossroads of exercise and proteostasis. Redox Biol. 35, 101529. doi:10.1016/j.redox.2020.101529
Herzig, R., Nehnevajova, E., Pfistner, C., Schwitzguebel, J. P., Ricci, A., and Keller, C. (2014). Feasibility of labile Zn phytoextraction using enhanced tobacco and sunflower: Results of five- and one-year field-scale experiments in Switzerland. Int. J. Phytoremediation 16, 735–754. doi:10.1080/15226514.2013.856846
Horn, S., Gunn, A. G., Petavratzi, E., Shaw, R. A., Eilu, P., Törmänen, T., et al. (2021). Cobalt resources in Europe and the potential for new discoveries. Ore Geol. Rev. 130, 103915. doi:10.1016/j.oregeorev.2020.103915
Hosseini, S. S., Bringas, E., Tan, N. R., Ortiz, I., Ghahramani, M., and Alaei Shahmirzadi, M. A. (2016). Recent progress in development of high performance polymeric membranes and materials for metal plating wastewater treatment: A review. J. Water Process Eng. 9, 78–110. doi:10.1016/j.jwpe.2015.11.005
Huang, Y., Du, J. R., Zhang, Y., Lawless, D., and Feng, X. (2015). Removal of mercury (II) from wastewater by polyvinylamine-enhanced ultrafiltration. Sep. Purif. Technol. 154, 1–10. doi:10.1016/j.seppur.2015.09.003
Hunter, H. A., Ling, F. T., and Peters, C. A. (2021). Coprecipitation of heavy metals in calcium carbonate from coal fly ash leachate. ACS ES&T Water 1, 339–345. doi:10.1021/acsestwater.0c00109
Ibrahim, W. M., Hassan, A. F., and Azab, Y. A. (2016). Biosorption of toxic heavy metals from aqueous solution by Ulva lactuca activated carbon. Egypt. J. Basic Appl. Sci. 3, 241–249. doi:10.1016/j.ejbas.2016.07.005
Igiri, B. E., Okoduwa, S. I. R., Idoko, G. O., Akabuogu, E. P., Adeyi, A. O., and Ejiogu, I. K. (2018). Toxicity and bioremediation of heavy metals contaminated ecosystem from tannery wastewater: A review. J. Toxicol. 2018, 2568038. doi:10.1155/2018/2568038
Ihsanullah, I., Abbas, A., Al-Amer, A. M., Laoui, T., Al-Marri, M. J., Nasser, M. S., et al. (2016). Heavy metal removal from aqueous solution by advanced carbon nanotubes: Critical review of adsorption applications. Sep. Purif. Technol. 157, 141–161. doi:10.1016/j.seppur.2015.11.039
Islam, M. M., Karim, M. R., Zheng, X., and Li, X. (2018a). Heavy metal and metalloid pollution of soil, water and foods in Bangladesh: A critical review. Int. J. Environ. Res. Public Health 15, 825. doi:10.3390/ijerph15122825
Jacobs, A., De Brabandere, L., Drouet, T., Sterckeman, T., and Noret, N. (2018). Phytoextraction of Cd and Zn with Noccaea caerulescens for urban soil remediation: Influence of nitrogen fertilization and planting density. Ecol. Eng. 116, 178–187. doi:10.1016/j.ecoleng.2018.03.007
Jadhav, S. V., Gadipelly, C. R., Marathe, K. V., and Rathod, V. K. (2014). Treatment of fluoride concentrates from membrane unit using salt solutions. J. Water Process Eng. 2, 31–36. doi:10.1016/j.jwpe.2014.04.004
Jaishankar, M., Tseten, T., Anbalagan, N., Mathew, B. B., and Beeregowda, K. N. (2014). Toxicity, mechanism and health effects of some heavy metals. Interdiscip. Toxicol. 7, 60–72. doi:10.2478/intox-2014-0009
Jiang, N., Shang, R., Heijman, S. G. J., and Rietveld, L. C. (2018). High-silica zeolites for adsorption of organic micro-pollutants in water treatment: A review. Water Res. 144, 145–161. doi:10.1016/j.watres.2018.07.017
Jiang, X., Li, Y., Tang, X., Jiang, J., He, Q., Xiong, Z., et al. (2021). Biopolymer-based flocculants: A review of recent technologies. Environ. Sci. Pollut. Res. 28, 46934–46963. doi:10.1007/s11356-021-15299-y
Jones, B. O., John, O. O., Luke, C., Ochieng, A., and Bassey, B. J. (2016). Application of mucilage from Dicerocaryum eriocarpum plant as biosorption medium in the removal of selected heavy metal ions. J. Environ. Manage. 177, 365–372. doi:10.1016/j.jenvman.2016.04.011
Junuzovic, H., Begic, S., Selimovic, A., Dozic, A., Cvrk, R., and Ahmetovi, M. (2019). Efficiency of carbonate precipitation and removal of copper and nickel ions from their monocomponent and two-component aqueous solutions. Int. J. Res. Appl. Sci. Biotechnol. 06, 11–15. doi:10.31033/ijrasb.6.6.3
Kabata-Pendias, A., and Pendias, H. (2011). Trace elements in soils and plants. 4th Edition. Boca Raton: CRC Press.
Khulbe, K. C., and Matsuura, T. (2018). Removal of heavy metals and pollutants by membrane adsorption techniques. Appl. Water Sci. 8, 19. doi:10.1007/s13201-018-0661-6
Kinuthia, G. K., Ngure, V., Beti, D., Lugalia, R., Wangila, A., and Kamau, L. (2020). Levels of heavy metals in wastewater and soil samples from open drainage channels in nairobi, Kenya: Community health implication. Sci. Rep. 10, 8434. doi:10.1038/s41598-020-65359-5
Kodituwakku, K. A. R. K., and Yatawara, M. (2020). Phytoremediation of industrial sewage sludge with Eichhornia crassipes, Salvinia molesta and Pistia stratiotes in batch fed free water flow constructed wetlands. Bull. Environ. Contam. Toxicol. 104, 627–633. doi:10.1007/s00128-020-02805-0
Kongsuwan, A., Patnukao, P., and Pavasant, P. (2009). Binary component sorption of Cu(II) and Pb(II) with activated carbon from Eucalyptus camaldulensis Dehn bark. J. Ind. Eng. Chem. 15, 465–470. doi:10.1016/j.jiec.2009.02.002
Kosa, S. A., Al-Zhrani, G., and Abdel Salam, M. (2012). Removal of heavy metals from aqueous solutions by multi-walled carbon nanotubes modified with 8-hydroxyquinoline. Chem. Eng. J. 181–182, 159–168. doi:10.1016/j.cej.2011.11.044
Kotobuki, M., Gu, Q., Zhang, L., and Wang, J. (2021). Ceramic-polymer composite membranes for water and wastewater treatment: Bridging the big gap between ceramics and polymers. Molecules 26, 331. doi:10.3390/molecules26113331
Król, E., Krejpcio, Z., Okulicz, M., and Śmigielska, H. (2020). Chromium(III) glycinate complex supplementation improves the blood glucose level and attenuates the tissular copper to zinc ratio in rats with mild hyperglycaemia. Biol. Trace Elem. Res. 193, 185–194. doi:10.1007/s12011-019-01686-7
Kumar, L., and Jana, S. K. (2021). Advances in absorbents and techniques used in wet and dry fgd: A critical review. Rev. Chem. Eng. doi:10.1515/revce-2020-0029
Kumar, P., and Fulekar, M. (2017). Assessment of physico chemical, microbial characteristics and heavy metals contamination at E waste dumping sites at ahmedabad, Gujarat. Int. J. Adv. Res. 5, 1601–1609. doi:10.21474/ijar01/4577
Kumar, P., and Fulekar, M. H. (2022). Cadmium phytoremediation potential of Deenanath grass (Pennisetum pedicellatum) and the assessment of bacterial communities in the rhizospheric soil. Environ. Sci. Pollut. Res. 29, 2936–2953. doi:10.1007/s11356-021-15667-8
Kumar, P., Kumar, R., and Reddy, M. V. (2017). Assessment of sewage treatment plant effluent and its impact on the surface water and sediment quality of river Ganga at Kanpur. Int. J. Sci. Eng. Res. 8, 1315–1324. doi:10.14299/ijser.2018.01.003
Kumar, P. (2018). Electronic waste—hazards, management and available green technologies for remediation-a review. Int. Res. J. Environ. Sci. 7 (5), 57–68.
Kumar, A., Kumar, A., Cabral-Pinto, M., Chaturvedi, A. K., Shabnam, A. A., Subrahmanyam, G., et al. (2020). Lead toxicity: Health hazards, influence on food Chain, and sustainable remediation approaches. Int. J. Environ. Res. Public Health 17, 2179. doi:10.3390/ijerph17072179
Kumar Yadav, K., Gupta, N., Kumar, A., Reece, L. M., Singh, N., Rezania, S., et al. (2018). Mechanistic understanding and holistic approach of phytoremediation: A review on application and future prospects. Ecol. Eng. 120, 274–298. doi:10.1016/j.ecoleng.2018.05.039
Kwikima, M. M., Mateso, S., and Chebude, Y. (2021). Potentials of agricultural wastes as the ultimate alternative adsorbent for cadmium removal from wastewater. A review. Sci. Afr. 13, e00934. doi:10.1016/j.sciaf.2021.e00934
Lee, J., Kaunda, R. B., Sinkala, T., Workman, C. F., Bazilian, M. D., and Clough, G. (2021). Phytoremediation and phytoextraction in Sub-Saharan Africa: Addressing economic and social challenges. Ecotoxicol. Environ. Saf. 226, 112864. doi:10.1016/j.ecoenv.2021.112864
Leyssens, L., Vinck, B., Van Der Straeten, C., Wuyts, F., and Maes, L. (2017). Cobalt toxicity in humans—a review of the potential sources and systemic health effects. Toxicology 387, 43–56. doi:10.1016/j.tox.2017.05.015
Li, C., Zhou, K., Qin, W., Tian, C., Qi, M., Yan, X., et al. (2019). A review on heavy metals contamination in soil: Effects, sources, and remediation techniques. Soil Sediment Contam. Int. J. 28 (4), 380–394. doi:10.1080/15320383.2019.1592108
Li, X., He, S., Zhou, J., Yu, X., Li, L., Liu, Y., et al. (2021). Cr (VI) induces abnormalities in glucose and lipid metabolism through ROS/Nrf2 signaling. Ecotoxicol. Environ. Saf. 219, 112320. doi:10.1016/j.ecoenv.2021.112320
Lin, W., Jing, L., and Zhang, B. (2020). Micellar-enhanced ultrafiltration to remove nickel ions: A response surface method and artificial neural network optimization. Water 12, 1269. doi:10.3390/w12051269
Liu, L., Li, W., Song, W., and Guo, M. (2018). Remediation techniques for heavy metal-contaminated soils: Principles and applicability. Sci. Total Environ. 633, 206–219. doi:10.1016/j.scitotenv.2018.03.161
Liu, L., Liu, J., Liu, X., Dai, C., Zhang, Z., Song, W., et al. (2019). Kinetic and equilibrium of U(VI) biosorption onto the resistant bacterium Bacillus amyloliquefaciens. J. Environ. Radioact. 203, 117–124. doi:10.1016/j.jenvrad.2019.03.008
Lo, S.-F., Wang, S.-Y., Tsai, M.-J., and Lin, L.-D. (2012). Adsorption capacity and removal efficiency of heavy metal ions by Moso and Ma bamboo activated carbons. Chem. Eng. Res. Des. 90, 1397–1406. doi:10.1016/j.cherd.2011.11.020
Loucks, D. P., and van Beek, E. (2017). “Water resources planning and management: An overview,” in Water resource systems planning and management. Editors D. P. Loucks,, and E. van Beek (Cham: Springer International Publishing), 1–49. doi:10.1007/978-3-319-44234-1_1
Lucaci, A. R., Bulgariu, D., Ahmad, I., Lisă, G., Mocanu, A. M., and Bulgariu, L. (2019). Potential use of biochar from various waste biomass as biosorbent in Co(II) removal processes. Water 11, 1565. doi:10.3390/w11081565
Luhar, I., Luhar, S., Abdullah, M. M., Razak, R. A., Vizureanu, P., Sandu, A. V., et al. (2021). A state-of-the-art review on innovative geopolymer composites designed for water and wastewater treatment. Mater 14, 456. doi:10.3390/ma14237456
Lyu, S., Chen, W., Zhang, W., Fan, Y., and Jiao, W. (2016). Wastewater reclamation and reuse in China: Opportunities and challenges. J. Environ. Sci. 39, 86–96. doi:10.1016/j.jes.2015.11.012
Macci, C., Doni, S., Peruzzi, E., Bardella, S., Filippis, G., Ceccanti, B., et al. (2013). A real-scale soil phytoremediation. Biodegradation 24, 521–538. doi:10.1007/s10532-012-9608-z
Maćczak, P., Kaczmarek, H., and Ziegler-Borowska, M. (2020). Recent achievements in polymer bio-based flocculants for water treatment. Mater 13, 951. doi:10.3390/ma13183951
Mahar, A., Wang, P., Ali, A., Awasthi, M. K., Lahori, A. H., Wang, Q., et al. (2016). Challenges and opportunities in the phytoremediation of heavy metals contaminated soils: A review. Ecotoxicol. Environ. Saf. 126, 111–121. doi:10.1016/j.ecoenv.2015.12.023
Maila, M. D., Maree, J. P., and Cele, L. M. (2014). Acid mine water neutralisation with ammonium hydroxide and desalination with barium hydroxide. Water sa. 40, 521–528. doi:10.4314/wsa.v40i3.16
Malamis, S., and Katsou, E. (2013). A review on zinc and nickel adsorption on natural and modified zeolite, bentonite and vermiculite: Examination of process parameters, kinetics and isotherms. J. Hazard. Mat. 252–253, 428–461. doi:10.1016/j.jhazmat.2013.03.024
Mansor, M., Winkler, C., Hochella, M. F., and Xu, J. (2019). Nanoparticulate nickel-hosting phases in sulfidic environments: Effects of ferrous iron and bacterial presence on mineral formation mechanism and solid-phase nickel distribution. Front. Earth Sci. 7, 151. doi:10.3389/feart.2019.00151
Marecka-Migacz, A., Mitkowski, P. T., Nędzarek, A., Różański, J., and Szaferski, W. (2020). Effect of pH on total volume membrane charge density in the nanofiltration of aqueous solutions of nitrate salts of heavy metals. Membr. (Basel) 10, 235. doi:10.3390/membranes10090235
Mataruga, Z., Jarić, S., Marković, M., Pavlović, M., Pavlović, D., Jakovljević, K., et al. (2020). Evaluation of Salix alba, Juglans regia and Populus nigra as biomonitors of PTEs in the riparian soils of the Sava River. Environ. Monit. Assess. 192, 131. doi:10.1007/s10661-020-8085-9
Matouq, M., Jildeh, N., Qtaishat, M., Hindiyeh, M., and Al Syouf, M. Q. (2015). The adsorption kinetics and modeling for heavy metals removal from wastewater by Moringa pods. J. Environ. Chem. Eng. 3, 775–784. doi:10.1016/j.jece.2015.03.027
Medfu Tarekegn, M., Zewdu Salilih, F., and Ishetu, A. I. (2020). Microbes used as a tool for bioremediation of heavy metal from the environment. Cogent Food Agric. 6, 1783174. doi:10.1080/23311932.2020.1783174
Mehdipour, S., Vatanpour, V., and Kariminia, H.-R. (2015). Influence of ion interaction on lead removal by a polyamide nanofiltration membrane. Desalination 362, 30. doi:10.1016/j.desal.2015.01.030
Mehta, D., Mazumdar, S., and Singh, S. K. (2015). Magnetic adsorbents for the treatment of water/wastewater—a review. J. Water Process Eng. 7, 244–265. doi:10.1016/j.jwpe.2015.07.001
Mena Aguilar, K. M., Amano, Y., and Machida, M. (2016). Ammonium persulfate oxidized activated carbon fiber as a high capacity adsorbent for aqueous Pb(II). J. Environ. Chem. Eng. 4, 4644–4652. doi:10.1016/j.jece.2016.10.028
Meng, Q., Nan, J., Mu, Y., Zu, X., and Guo, M. (2021). Study on the treatment of sudden cadmium pollution in surface water by a polymer enhanced ultrafiltration process. RSC Adv. 11, 7405–7415. doi:10.1039/D0RA10818A
Meyers, D. E. R., Auchterlonie, G. J., Webb, R. I., and Wood, B. (2008). Uptake and localisation of lead in the root system of Brassica juncea. Environ. Pollut. 153, 323–332. doi:10.1016/j.envpol.2007.08.029
Milojković, J., Pezo, L., Stojanović, M., Mihajlović, M., Lopičić, Z., Petrović, J., et al. (2016). Selected heavy metal biosorption by compost of Myriophyllum spicatum-A chemometric approach. Ecol. Eng. 93, 112–119. doi:10.1016/j.ecoleng.2016.05.012
Moghaddam, M. R., Fatemi, S., and Keshtkar, A. (2013). Adsorption of lead (Pb2+) and uranium (UO22+) cations by Brown algae; experimental and thermodynamic modeling. Chem. Eng. J. 231, 294–303. doi:10.1016/j.cej.2013.07.037
Mohammed, A. A., Najim, A. A., Al-Musawi, T. J., and Alwared, A. I. (2019). Adsorptive performance of a mixture of three nonliving algae classes for nickel remediation in synthesized wastewater. J. Environ. Heal. Sci. Eng. 17, 529–538. doi:10.1007/s40201-019-00367-w
Mohsen-Nia, M., Montazeri, P., and Modarress, H. (2007). Removal of Cu2+ and Ni2+ from wastewater with a chelating agent and reverse osmosis processes. Desalination 217, 276–281. doi:10.1016/j.desal.2006.01.043
Mokone, T. P., van Hille, R. P., and Lewis, A. E. (2012). Metal sulphides from wastewater: Assessing the impact of supersaturation control strategies. Water Res. 46, 2088–2100. doi:10.1016/j.watres.2012.01.027
Morante-Carballo, F., Montalván-Burbano, N., Carrión-Mero, P., and Espinoza-Santos, N. (2021). Cation exchange of natural zeolites: Worldwide research. Sustain 13, 751. doi:10.3390/su13147751
Moreno, F. N., Anderson, C. W. N., Stewart, R. B., and Robinson, B. H. (2005). Mercury volatilisation and phytoextraction from base-metal mine tailings. Environ. Pollut. 136, 341–352. doi:10.1016/j.envpol.2004.11.020
Moreno, F. N., Anderson, C. W. N., Stewart, R. B., and Robinson, B. H. (2008). Phytofiltration of mercury-contaminated water: Volatilisation and plant-accumulation aspects. Environ. Exp. Bot. 62, 78–85. doi:10.1016/j.envexpbot.2007.07.007
Moura, R. C. A., Bertuol, D. A., Ferreira, C. A., and Amado, F. D. R. (2012). Study of chromium removal by the electrodialysis of tannery and metal-finishing effluents. Int. J. Chem. Eng. 2012, 179312. doi:10.1155/2012/179312
Murthy, Z. V. P., and Chaudhari, L. B. (2008). Application of nanofiltration for the rejection of nickel ions from aqueous solutions and estimation of membrane transport parameters. J. Hazard. Mat. 160, 70–77. doi:10.1016/j.jhazmat.2008.02.085
Muszyńska, E., and Hanus-Fajerska, E. (2015). Why are heavy metal hyperaccumulating plants so amazing? BioTechnologia 96, 265–271. doi:10.5114/bta.2015.57730
Muthukrishnan, M., and Guha, B. K. (2008). Effect of pH on rejection of hexavalent chromium by nanofiltration. Desalination 219, 171–178. doi:10.1016/j.desal.2007.04.054
Naseem, T., and Durrani, T. (2021). The role of some important metal oxide nanoparticles for wastewater and antibacterial applications: A review. Environ. Chem. Ecotoxicol. 3, 59–75. doi:10.1016/j.enceco.2020.12.001
Nedjimi, B. (2018). “Heavy metal tolerance in two Algerian saltbushes: A review on plant responses to cadmium and role of calcium in its mitigation,” in Plant nutrients and abiotic stress tolerance. Editors M. Hasanuzzaman, M. Fujita, H. Oku, K. Nahar, and B. Hawrylak-Nowak (Singapore: Springer Singapore), 205–220. doi:10.1007/978-981-10-9044-8_9
Nedjimi, B. (2021). Phytoremediation: A sustainable environmental technology for heavy metals decontamination. SN Appl. Sci. 3, 286. doi:10.1007/s42452-021-04301-4
Nedzarek, A., Drost, A., Harasimiuk, F. B., and Tórz, A. (2015). The influence of pH and BSA on the retention of selected heavy metals in the nanofiltration process using ceramic membrane. Desalination 369, 62. doi:10.1016/j.desal.2015.04.019
Nguyen, C. M., Bang, S., Cho, J., and Kim, K.-W. (2009). Performance and mechanism of arsenic removal from water by a nanofiltration membrane. Desalination 245, 82–94. doi:10.1016/j.desal.2008.04.047
Nguyen, T. A. H., Ngo, H. H., Guo, W. S., Zhang, J., Liang, S., Yue, Q. Y., et al. (2013). Applicability of agricultural waste and by-products for adsorptive removal of heavy metals from wastewater. Bioresour. Technol. 148, 574–585. doi:10.1016/j.biortech.2013.08.124
Nicomel, N. R., Leus, K., Folens, K., Van Der Voort, P., and Du Laing, G. (2015). Technologies for arsenic removal from water: Current status and future perspectives. Int. J. Environ. Res. Public Health 13, ijerph13010062. doi:10.3390/ijerph13010062
Obotey Ezugbe, E., and Rathilal, S. (2020). Membrane technologies in wastewater treatment: A review. Membr. (Basel) 10, 89. doi:10.3390/membranes10050089
Odom, T., Huang, J. L., and Kim, P. (2020). Atomic structure and electronic properties of single-walled carbon nanotubes. Nature 391, 62–64. doi:10.1038/34145
Okoro, H. K., Pandey, S., Ogunkunle, C. O., Ngila, C. J., Zvinowanda, C., Jimoh, I., et al. (2022). Nanomaterial-based biosorbents: Adsorbent for efficient removal of selected organic pollutants from industrial wastewater. Emerg. Contam. 8, 46–58. doi:10.1016/j.emcon.2021.12.005
Oustriere, N., Marchand, L., Roulet, E., and Mench, M. (2017). Rhizofiltration of a Bordeaux mixture euent in pilot-scale constructed wetland using Arundo donax L. coupled with potential Cu-ecocatalyst production. Ecol. Eng. 105, 296–305.
Oyarce, E., Roa, K., Boulett, A., Salazar-Marconi, P., and Sánchez, J. (2022). Removal of lithium ions from aqueous solutions by an ultrafiltration membrane coupled to soluble functional polymer. Sep. Purif. Technol. 288, 120715. doi:10.1016/j.seppur.2022.120715
Palansooriya, K. N., Shaheen, S. M., Chen, S. S., Tsang, D. C. W., Hashimoto, Y., Hou, D., et al. (2020). Soil amendments for immobilization of potentially toxic elements in contaminated soils: A critical review. Environ. Int. 134, 105046. doi:10.1016/j.envint.2019.105046
Pang, F., Kumar, P., Teng, T. T., Kadir, M., and Wasewar, K. (2011). Removal of lead, zinc and iron by coagulation–flocculation. J. Taiwan Inst. Chem. Eng. - J. TAIWAN Inst. Chem. Eng. 42, 809–815. doi:10.1016/j.jtice.2011.01.009
Parham, H., Zargar, B., and Shiralipour, R. (2012). Fast and efficient removal of mercury from water samples using magnetic iron oxide nanoparticles modified with 2-mercaptobenzothiazole. J. Hazard. Mat. 205–206, 94–100. doi:10.1016/j.jhazmat.2011.12.026
Parker, D. R., Feist, L. J., Varvel, T. W., Thomason, D. N., and Zhang, Y. (2003). Selenium phytoremediation potential of Stanleya pinnata. Plant Soil 249, 157–165. doi:10.1023/A:1022545629940
Parker, G. H., Gillie, C. E., Miller, J. V., Badger, D. E., and Kreider, M. L. (2022). Human health risk assessment of arsenic, cadmium, lead, and mercury ingestion from baby foods. Toxicol. Rep. 9, 238–249. doi:10.1016/j.toxrep.2022.02.001
Patil, D. S., Chavan, S. M., and Oubagaranadin, J. U. K. (2016). A review of technologies for manganese removal from wastewaters. J. Environ. Chem. Eng. 4, 468–487. doi:10.1016/j.jece.2015.11.028
Petrinic, I., Korenak, J., Povodnik, D., and Hélix-Nielsen, C. (2015). A feasibility study of ultrafiltration/reverse osmosis (UF/RO)-based wastewater treatment and reuse in the metal finishing industry. J. Clean. Prod. 101, 292–300. doi:10.1016/j.jclepro.2015.04.022
Petrovic, B., Gorbounov, M., and Masoudi Soltani, S. (2021). Influence of surface modification on selective CO2 adsorption: A technical review on mechanisms and methods. Microporous Mesoporous Mater 312, 110751. doi:10.1016/j.micromeso.2020.110751
Pohl, A. (2020). Removal of heavy metal ions from water and wastewaters by sulfur-containing precipitation agents. Water. Air. Soil Pollut. 231, 503. doi:10.1007/s11270-020-04863-w
Prokkola, H., Nurmesniemi, E. T., and Lassi, U. (2020). Removal of metals by sulphide precipitation using na2s and hs−-solution. ChemEngineering 4, 1–10. doi:10.3390/chemengineering4030051
Punia, P., Bharti, M. K., Dhar, R., Thakur, P., and Thakur, A. (2022). Recent advances in detection and removal of heavy metals from contaminated water. ChemBioEng Rev. doi:10.1002/cben.202100053
Qasem, N. A. A., Mohammed, R. H., and Lawal, D. U. (2021). Removal of heavy metal ions from wastewater: A comprehensive and critical review. npj Clean. Water 4, 36. doi:10.1038/s41545-021-00127-0
Qu, X., Alvarez, P. J. J., and Li, Q. (2013). Applications of nanotechnology in water and wastewater treatment. Water Res. 47, 3931–3946. doi:10.1016/j.watres.2012.09.058
Radziemska, M., Vaverková, M. D., and Baryła, A. (2017). Phytostabilization-management strategy for stabilizing trace elements in contaminated soils. Int. J. Environ. Res. Public Health 14, 958. doi:10.3390/ijerph14090958
Rafati Rahimzadeh, M., Rafati Rahimzadeh, M., Kazemi, S., and Moghadamnia, A.-A. (2017). Cadmium toxicity and treatment: An update. Casp. J. Intern. Med. 8, 135–145. doi:10.22088/cjim.8.3.135
Rahamathulla, M., Bhosale, R. R., Osmani, R. A. M., Mahima, K. C., Johnson, A. P., Hani, U., et al. (2021). Carbon nanotubes: Current perspectives on diverse applications in targeted drug delivery and therapies. Mat. (Basel, Switz. 14, 6707. doi:10.3390/ma14216707
Rai, P. K., Lee, S. S., Zhang, M., Tsang, Y. F., and Kim, K. H. (2019). Heavy metals in food crops: Health risks, fate, mechanisms, and management. Environ. Int. 125, 365–385. doi:10.1016/j.envint.2019.01.067
Rai, S., Singh, P. K., Mankotia, S., Swain, J., and Satbhai, S. B. (2021a). Iron homeostasis in plants and its crosstalk with copper, zinc, and manganese. Plant Stress 1, 100008. doi:10.1016/j.stress.2021.100008
Rai, V., Liu, D., Xia, D., Jayaraman, Y., and Gabriel, J. C. P. (2021b). Electrochemical approaches for the recovery of metals from electronic waste: A critical review. Recycling 6, 30053. doi:10.3390/recycling6030053
Rajasulochana, P., and Preethy, V. (2016). Comparison on efficiency of various techniques in treatment of waste and sewage water – a comprehensive review. Resour. Technol. 2, 175–184. doi:10.1016/j.reffit.2016.09.004
Rehman, M., Maqbool, Z., Peng, D., and Liu, L. (2019). Morpho-physiological traits, antioxidant capacity and phytoextraction of copper by ramie (Boehmeria nivea L.) grown as fodder in copper-contaminated soil. Environ. Sci. Pollut. Res. 26, 5851–5861. doi:10.1007/s11356-018-4015-6
Rene, E. R., Bui, X. T., Ngo, H. H., Nghiem, L. D., and Guo, W. (2021). Green technologies for sustainable environment: An introduction. Environ. Sci. Pollut. Res. 28, 63437–63439. doi:10.1007/s11356-021-16870-3
Ruihua, L., Lin, Z., Tao, T., and Bo, L. (2011). Phosphorus removal performance of acid mine drainage from wastewater. J. Hazard. Mat. 190, 669–676. doi:10.1016/j.jhazmat.2011.03.097
Rzymski, P., Niedzielski, P., Klimaszyk, P., and Poniedziałek, B. (2014). Bioaccumulation of selected metals in bivalves (Unionidae) and Phragmites australis inhabiting a municipal water reservoir. Environ. Monit. Assess. 186, 3199–3212. doi:10.1007/s10661-013-3610-8
Sabzehmeidani, M. M., Mahnaee, S., Ghaedi, M., Heidari, H., and Roy, V. A. L. (2021). Carbon based materials: A review of adsorbents for inorganic and organic compounds. Mat. Adv. 2, 598–627. doi:10.1039/d0ma00087f
Saleem, J., Shahid, U. B., Hijab, M., Mackey, H., and McKay, G. (2019). Production and applications of activated carbons as adsorbents from olive stones. Biomass Convers. Biorefinery 9, 775–802. doi:10.1007/s13399-019-00473-7
Sampaio, C. J. S., de Souza, J. R. B., Damião, A. O., Bahiense, T. C., and Roque, M. R. A. (2019). Biodegradation of polycyclic aromatic hydrocarbons (PAHs) in a diesel oil-contaminated mangrove by plant growth-promoting rhizobacteria. 3 Biotech. 9, 155. doi:10.1007/s13205-019-1686-8
Samper, E., Rodríguez, M., De la Rubia, M. A., and Prats, D. (2009). Removal of metal ions at low concentration by micellar-enhanced ultrafiltration (MEUF) using sodium dodecyl sulfate (SDS) and linear alkylbenzene sulfonate (LAS). Sep. Purif. Technol. 65, 337–342. doi:10.1016/j.seppur.2008.11.013
Sankararamakrishnan, N., Jaiswal, M., and Verma, N. (2014). Composite nanofloral clusters of carbon nanotubes and activated alumina: An efficient sorbent for heavy metal removal. Chem. Eng. J. 235, 1–9. doi:10.1016/j.cej.2013.08.070
Sarath, N. G., Shackira, A. M., El-Serehy, H. A., Hefft, D. I., and Puthur, J. T. (2022). Phytostabilization of arsenic and associated physio-anatomical changes in Acanthus ilicifolius L. Environ. Pollut. 298, 118828. doi:10.1016/j.envpol.2022.118828
Saravanan, A., Kumar, P. S., Hemavathy, R. V., Jeevanantham, S., Harikumar, P., Priyanka, G., et al. (2022). A comprehensive review on sources, analysis and toxicity of environmental pollutants and its removal methods from water environment. Sci. Total Environ. 812, 152456. doi:10.1016/j.scitotenv.2021.152456
Sardella, F., Gimenez, M., Navas, C., Morandi, C., Deiana, C., and Sapag, K. (2015). Conversion of viticultural industry wastes into activated carbons for removal of lead and cadmium. J. Environ. Chem. Eng. 3, 253–260. doi:10.1016/j.jece.2014.06.026
Sarker, A., Kim, J.-E., Islam, A. R. M. T., Bilal, M., Rakib, M. R. J., Nandi, R., et al. (2022). Heavy metals contamination and associated health risks in food webs—A review focuses on food safety and environmental sustainability in Bangladesh. Environ. Sci. Pollut. Res. 29, 3230–3245. doi:10.1007/s11356-021-17153-7
Sarwar, N., Imran, M., Shaheen, M. R., Ishaque, W., Kamran, M. A., Matloob, A., et al. (2017). Phytoremediation strategies for soils contaminated with heavy metals: Modifications and future perspectives. Chemosphere 171, 710–721. doi:10.1016/j.chemosphere.2016.12.116
Savastru, E., Bulgariu, D., Zamfir, C.-I., and Bulgariu, L. (2022). Application of Saccharomyces cerevisiae in the biosorption of Co(II), Zn(II) and Cu(II) ions from aqueous media. Water 14, 976. doi:10.3390/w14060976
Selvi, A., Rajasekar, A., Theerthagiri, J., Ananthaselvam, A., Sathishkumar, K., Madhavan, J., et al. (2019). Integrated remediation processes toward heavy metal removal/recovery from various environments-A review. Front. Environ. Sci. 7, 66. doi:10.3389/fenvs.2019.00066
Shackira, A. M., and Puthur, J. T. (2019). “Phytostabilization of heavy metals: Understanding of principles and practices,” in Plant-metal interactions. Editors S. Srivastava, A. K. Srivastava, and P. Suprasanna (Cham: Springer International Publishing), 263–282. doi:10.1007/978-3-030-20732-8_13
Shankar, S., Shanker, U., and Shikha, (2014). Arsenic contamination of groundwater: A review of sources, prevalence, health risks, and strategies for mitigation. Sci. World J. 2014, 304524. doi:10.1155/2014/304524
Sharma, S., Rana, S., Thakkar, A., Baldi, A., Rsr, M., and Rk, S. (2016). iMedPub journals physical , chemical and phytoremediation technique for removal of heavy metals keywords : Removal of heavy metals : Strategies and mechanisms. J. Heavy Mater. Toxic. Dis. 1, 1–15. doi:10.21767/2473-6457.100010
Shen, L.-C., Nguyen, X.-T., and Hankins, N. P. (2015). Removal of heavy metal ions from dilute aqueous solutions by polymer–surfactant aggregates: A novel effluent treatment process. Sep. Purif. Technol. 152, 101–107. doi:10.1016/j.seppur.2015.07.065
Shen, Y., and Badireddy, A. R. (2021). A critical review on electric field-assisted membrane processes: Implications for fouling control, water recovery, and future prospects. Membr. (Basel) 11, 820. doi:10.3390/membranes11110820
Shewa, W. A., and Dagnew, M. (2020). Revisiting chemically enhanced primary treatment of wastewater: A review. Sustain 12, 928. doi:10.3390/su12155928
Shimizu, F. M., Braunger, M. L., and Riul, A. (2019). Heavy metal/toxins detection using electronic tongues. Chemosensors 7, 1–19. doi:10.3390/CHEMOSENSORS7030036
Siddique, T. A., Dutta, N. K., and Roy Choudhury, N. (2020). Nanofiltration for arsenic removal: Challenges, recent developments, and perspectives. Nanomater 10, 71323. doi:10.3390/nano10071323
Sivakumar, D., Shankar, D., Gomathi, V., and Nandakumaar, A. (2014). Application of electro-dialysis on removal of heavy metals. Pollut. Res. 33, 627–631.
Smith, M. R., Golden, C. D., and Myers, S. S. (2017). Potential rise in iron deficiency due to future anthropogenic carbon dioxide emissions. GeoHealth 1, 248–257. doi:10.1002/2016GH000018
Song, Z., Garg, S., Ma, J., and Waite, T. D. (2019). Modified double potential step chronoamperometry (DPSC) method for as(III) electro-oxidation and concomitant as(V) adsorption from groundwaters. Environ. Sci. Technol. 53, 9715–9724. doi:10.1021/acs.est.9b01762
Srivastava, V., Sarkar, A., Singh, S., Singh, P., de Araujo, A. S. F., and Singh, R. P. (2017). Agroecological responses of heavy metal pollution with special emphasis on soil health and plant performances. Front. Environ. Sci. 5, 64. doi:10.3389/fenvs.2017.00064
Subba Rao, T., Panigrahi, S., and Velraj, P. (2022). “Transport and disposal of radioactive wastes in nuclear industry,” in Microbial biodegradation and bioremediation. Editor S. Das. Second Edision (Dash (Elsevier), 419–440. doi:10.1016/b978-0-323-85455-9.00027-8
Suhalim, N. S., Kasim, N., Mahmoudi, E., Shamsudin, I. J., Mohammad, A. W., Mohamed Zuki, F., et al. (2022). Rejection mechanism of ionic solute removal by nanofiltration membranes: An overview. Nanomater 12, 437. doi:10.3390/nano12030437
Suman, J., Uhlik, O., Viktorova, J., and Macek, T. (2018). Phytoextraction of heavy metals: A promising tool for clean-up of polluted environment? Front. Plant Sci. 871, 1476. doi:10.3389/fpls.2018.01476
Tang, X., Zheng, H., Teng, H., Sun, Y., Guo, J., Xie, W., et al. (2016). Chemical coagulation process for the removal of heavy metals from water: A review. Desalin. Water Treat. 57, 1733–1748. doi:10.1080/19443994.2014.977959
Tanhaei, B., Pourafshari Chenar, M., Saghatoleslami, N., Hesampour, M., Laakso, T., Kallioinen, M., et al. (2014). Simultaneous removal of aniline and nickel from water by micellar-enhanced ultrafiltration with different molecular weight cut-off membranes. Sep. Purif. Technol. 124, 26–35. doi:10.1016/j.seppur.2014.01.009
Tanong, K., Tran, L.-H., Mercier, G., and Blais, J.-F. (2017). Recovery of Zn (II), Mn (II), Cd (II) and Ni (II) from the unsorted spent batteries using solvent extraction, electrodeposition and precipitation methods. J. Clean. Prod. 148, 233–244. doi:10.1016/j.jclepro.2017.01.158
Tao, W., Chen, G., Zeng, G., Yan, M., Chen, A., Guo, Z., et al. (2016). Influence of silver nanoparticles on heavy metals of pore water in contaminated river sediments. Chemosphere 162, 117–124. doi:10.1016/j.chemosphere.2016.07.043
Tchounwou, P. B., Yedjou, C. G., Patlolla, A. K., and Sutton, D. J. (2012). Heavy metal toxicity and the environment. Exp. Suppl. 101, 133–164. doi:10.1007/978-3-7643-8340-4_6
Teh, C. Y., Budiman, P. M., Shak, K. P. Y., and Wu, T. Y. (2016). Recent advancement of coagulation–flocculation and its application in wastewater treatment. Ind. Eng. Chem. Res. 55, 4363–4389. doi:10.1021/acs.iecr.5b04703
Thakare, Y., and Jana, A. (2015). Performance of high density ion exchange resin (INDION225H) for removal of Cu(II) from waste water. J. Environ. Chem. Eng. 3, 1393–1398. doi:10.1016/j.jece.2015.01.002
Thomas, M., Kozik, V., Bąk, A., Barbusiński, K., Jazowiecka‐rakus, J., and Jampilek, J. (2021). Removal of heavy metal ions from wastewaters: An application of sodium trithiocarbonate and wastewater toxicity assessment. Mater. (Basel) 14, 1–18. doi:10.3390/ma14030655
Tiwari, J., Ankit, S., Kumar, S., Korstad, J., and Bauddh, K. (2018). “Ecorestoration of polluted aquatic ecosystems through rhizofiltration,” in Phytomanagement of polluted sites: Market opportunities in sustainable phytoremediation. Editors V. C. Pandey, and S. Bauddh (Elsevier), 179–201. doi:10.1016/B978-0-12-813912-7.00005-3
Tlustoš, P., Száková, J., Hrubý, J., Hartman, I., Najmanová, J., Nedělník, J., et al. (2006). Removal of As, Cd, Pb, and Zn from contaminated soil by high biomass producing plants. Plant, Soil Environ. 52, 413–423. doi:10.17221/3460-pse
Torres, E. (2020). Biosorption: A review of the latest advances. Processes 8, 1–23. doi:10.3390/pr8121584
Tran, T.-T., and Mulchandani, A. (2016). Carbon nanotubes and graphene nano field-effect transistor-based biosensors. Trac. Trends Anal. Chem. 79, 222–232. doi:10.1016/j.trac.2015.12.002
Vera Tomé, F., Blanco Rodríguez, P., and Lozano, J. C. (2008). Elimination of natural uranium and 226Ra from contaminated waters by rhizofiltration using Helianthus annuus L. Sci. Total Environ. 393, 351–357. doi:10.1016/j.scitotenv.2008.01.013
Vidu, R., Matei, E., Predescu, A. M., Alhalaili, B., Pantilimon, C., Tarcea, C., et al. (2020). Removal of heavy metals from wastewaters: A challenge from current treatment methods to nanotechnology applications. Toxics 8, 1–37. doi:10.3390/toxics8040101
Violante, A., Cozzolino, V., Perelomov, L., Caporale, A. G., and Pigna, M. (2010). Mobility and bioavailability of heavy metals and metalloids in soil environments. J. Soil Sci. Plant Nutr. 10 (3), 268–292. doi:10.4067/S0718-95162010000100005
Wang, B., and Du, Y. (2013). Cadmium and its neurotoxic effects. Oxid. Med. Cell. Longev. 2013, 898034. doi:10.1155/2013/898034
Wang, G., Zhang, S., Yao, P., Chen, Y., Xu, X., Li, T., et al. (2018). Removal of Pb(II) from aqueous solutions by Phytolacca americana L. biomass as a low cost biosorbent. Arab. J. Chem. 11, 99–110. doi:10.1016/j.arabjc.2015.06.011
Warsinger, D. M., Chakraborty, S., Tow, E. W., Plumlee, M. H., Bellona, C., Loutatidou, S., et al. (2016). A review of polymeric membranes and processes for potable water reuse. Prog. Polym. Sci. 81, 209–237. doi:10.1016/j.progpolymsci.2018.01.004
Who, (1996). Permissible limits of heavy metals in soil and plants. Switzerland: Geneva: World Health Organization.
Wołowiec, M., Komorowska-Kaufman, M., Pruss, A., Rzepa, G., and Bajda, T. (2019). Removal of heavy metals and metalloids from water using drinking water treatment residuals as adsorbents: A review. Minerals 9, 487. doi:10.3390/min9080487
Yan, A., Wang, Y., Tan, S. N., Mohd Yusof, M. L., Ghosh, S., and Chen, Z. (2020). Phytoremediation: A promising approach for revegetation of heavy metal-polluted land. Front. Plant Sci. 11, 359. doi:10.3389/fpls.2020.00359
Yan, G., and Viraraghavan, T. (2008). Mechanism of biosorption of heavy metals by Mucor rouxii. Eng. Life Sci. 8, 363–371. doi:10.1002/elsc.200820237
Yang, M., Jawitz, J. W., and Lee, M. (2015). Uranium and cesium accumulation in bean (Phaseolus vulgaris L. var. vulgaris) and its potential for uranium rhizofiltration. J. Environ. Radioact. 140, 42–49. doi:10.1016/j.jenvrad.2014.10.015
Yang, W. J., Gu, J. F., Zhou, H., Huang, F., Yuan, T. Y., Zhang, J. Y., et al. (2020). Effect of three Napier grass varieties on phytoextraction of Cd- and Zn-contaminated cultivated soil under mowing and their safe utilization. Environ. Sci. Pollut. Res. 27, 16134–16144. doi:10.1007/s11356-020-07887-1
Yap, C. K., and Al-Mutairi, K. A. (2022). Ecological-health risk assessments of heavy metals (Cu, pb, and zn) in aquatic sediments from the asean-5 emerging developing countries: A review and synthesis. Biol. (Basel) 11, 10007. doi:10.3390/biology11010007
Yeo, K. F. H., Li, C., Zhang, H., Chen, J., Wang, W., and Dong, Y. (2021). Arsenic removal from contaminated water using natural adsorbents: A review. Coatings 11, 1407. doi:10.3390/COATINGS11111407
Younas, F., Mustafa, A., Farooqi, Z. U. R., Wang, X., Younas, S., Mohy-Ud-din, W., et al. (2021). Current and emerging adsorbent technologies for wastewater treatment: Trends, limitations, and environmental implications. WaterSwitzerl. 13, 215. doi:10.3390/w13020215
Zavahir, S., Elmakki, T., Gulied, M., Ahmad, Z., Al-Sulaiti, L., Shon, H. K., et al. (2021). A review on lithium recovery using electrochemical capturing systems. Desalination 500, 114883. doi:10.1016/j.desal.2020.114883
Zewail, T. M., and Yousef, N. S. (2015). Kinetic study of heavy metal ions removal by ion exchange in batch conical air spouted bed. Alex. Eng. J. 54, 83–90. doi:10.1016/j.aej.2014.11.008
Zgorelec, Z., Bilandzija, N., Knez, K., Galic, M., and Zuzul, S. (2020). Cadmium and Mercury phytostabilization from soil using Miscanthus × giganteus. Sci. Rep. 10, 6685. doi:10.1038/s41598-020-63488-5
Zhang, W., Cheng, C. Y., and Pranolo, Y. (2010). Investigation of methods for removal and recovery of manganese in hydrometallurgical processes. Hydrometallurgy 101, 58–63. doi:10.1016/j.hydromet.2009.11.018
Zhang, D., Yin, C., Abbas, N., Mao, Z., and Zhang, Y. (2020). Multiple heavy metal tolerance and removal by an earthworm gut fungus Trichoderma brevicompactum QYCD-6. Sci. Rep. 10, 6940. doi:10.1038/s41598-020-63813-y
Zhu, W.-P., Gao, J., Sun, S.-P., Zhang, S., and Chung, T.-S. (2015). Poly(amidoamine) dendrimer (PAMAM) grafted on thin film composite (TFC) nanofiltration (NF) hollow fiber membranes for heavy metal removal. J. Memb. Sci. 487, 117–126. doi:10.1016/j.memsci.2015.03.033
Keywords: toxicity, aquatic environment, sustainability, metal(loid)s, clean-up technologies
Citation: Kumar P, Gacem A, Ahmad MT, Yadav VK, Singh S, Yadav KK, Alam MM, Dawane V, Piplode S, Maurya P, Ahn Y, Jeon B-H and Cabral-Pinto MMS (2022) Environmental and human health implications of metal(loid)s: Source identification, contamination, toxicity, and sustainable clean-up technologies. Front. Environ. Sci. 10:949581. doi: 10.3389/fenvs.2022.949581
Received: 21 May 2022; Accepted: 19 July 2022;
Published: 26 August 2022.
Edited by:
Mubarak Mujawar, University of Technology Brunei, BruneiReviewed by:
Lakhan Kumar, Delhi Technological University, IndiaBulgariu Laura, Gheorghe Asachi Technical University of Iași, Romania
Gordana Gajic, University of Belgrade, Serbia
Sandeep K. Malyan, National Institute of Hydrology (Roorkee), India
Ram Naresh Bharagava, Babasaheb Bhimrao Ambedkar University, India
Copyright © 2022 Kumar, Gacem, Ahmad, Yadav, Singh, Yadav, Alam, Dawane, Piplode, Maurya, Ahn, Jeon and Cabral-Pinto. This is an open-access article distributed under the terms of the Creative Commons Attribution License (CC BY). The use, distribution or reproduction in other forums is permitted, provided the original author(s) and the copyright owner(s) are credited and that the original publication in this journal is cited, in accordance with accepted academic practice. No use, distribution or reproduction is permitted which does not comply with these terms.
*Correspondence: Pankaj Kumar, cGFua2FqYjQzNEB5YWhvby5jb20=; Virendra Kumar Yadav, eWFkYXZhOTRAZ21haWwuY29tJiN4MDIwMGE7; Byong-Hun Jeon, YmhqZW9uQGhhbnlhbmcuYWMua3I=