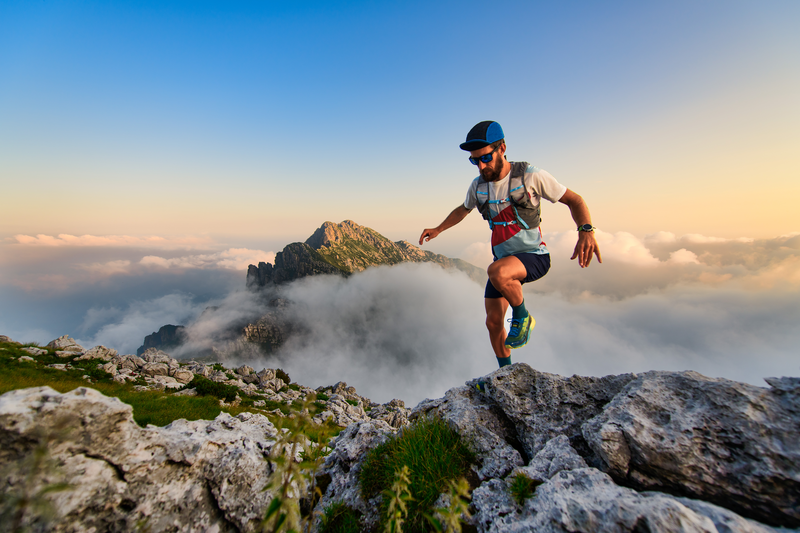
95% of researchers rate our articles as excellent or good
Learn more about the work of our research integrity team to safeguard the quality of each article we publish.
Find out more
ORIGINAL RESEARCH article
Front. Environ. Sci. , 27 September 2022
Sec. Freshwater Science
Volume 10 - 2022 | https://doi.org/10.3389/fenvs.2022.949353
This article is part of the Research Topic Freshwater Science in Africa View all 10 articles
The introduction of alien predatory fish such as rainbow trout (Oncorhynchus mykiss) can have a significant effect on indigenous fish communities such as altering the structure and dynamics of food webs. Quantifying the trophic niche utilised by the alien fish species is therefore important to aid our understanding of how their feeding strategies might aid establishment, define their functional role and inform on potential impacts. This study assessed food web interactions between fish communities in river reaches that are invaded by O. mykiss and sections that are free of invasions in the upper Blyde River catchment, South Africa. It specifically evaluated the hypothesis that O. mykiss invasion is likely to lead to a decrease in the trophic functional diversity through predation and that indigenous fish will shift their trophic niche to either minimise competition with, or avoid predation, by O. mykiss. Gut content and stable isotope analyses were used to determine trophic interactions. Fish communities in uninvaded areas utilised fewer and similar food sources and occupied lower trophic levels, than fish communities in invaded areas. Oncorhynchus mykiss fed mainly on invertebrates and at trophic levels similar to and or lower than indigenous fishes. This suggests that their current impact on indigenous fish communities is mainly through competition for resources. We posit that O. mykiss invasions reduced the abundance of indigenous fishes and thereby reduced predation pressure on aquatic invertebrates, with a knock-on effect on the trophic interrelationships among fish assemblages. Our findings are consistent with other studies in South Africa that have shown that the impacts of O. mykiss invasion can lead to a decline and fragmentation of indigenous species populations and niche shifts.
Predatory freshwater fish species have been introduced worldwide to enhance angling and inland fisheries (Cambray, 2003; Arlinghaus and Cooke, 2009). Rainbow trout (Oncorhynchus mykiss) is one of the most widely distributed fish species for angling (Crawford and Muir, 2008). It is native to the western seaboard of the United States, Canada, and northern Mexico (Page and Burr, 2011). In its introduced range, O. mykiss has been implicated in causing major impacts on indigenous fauna and ecosystems mainly through competition and predation (Drenner and Hambright, 2002; Simon and Townsend, 2003; McDowall, 2006; Fausch, 2007), modifying aquatic-terrestrial linkages (e.g., Epanchin et al., 2010; Jackson et al., 2016), altering food-web structure (Flecker and Townsend, 1994), and disrupting nutrient cycles (Schindler et al., 2001; Eby et al., 2006).
Oncorhynchus mykiss was introduced into South Africa in 1897, mainly for angling and aquaculture (Cambray, 2003; Ellender et al., 2014). Currently, O. mykiss has established populations in the mountain streams and upland reservoirs in over 80% of the major drainage basins in the country (Weyl et al., 2020). There is, however, limited information on the impacts of O. mykiss invasions in South Africa (Zengeya et al., 2020; Van Wilgen et al., 2022). The few available studies have, however, shown that O. mykiss can cause a decline, and in some cases local extirpation of indigenous invertebrates, frogs, and fishes (Karssing et al., 2012; Rivers-Moore et al., 2013; Shelton et al., 2015a; Jackson et al., 2016; Weyl et al., 2020). For example, O. mykiss invasions in the uKhahlamba Drakensberg Park in Kwa-Zulu Natal have been implicated in the reduction of macroinvertebrate communities and the abundance of amphibians probably likely through predation (Karssing et al., 2012; Rivers-Moore et al., 2013). Similarly, O. mykiss invasions have also been implicated in the decline in the abundance of populations of amphibians in the upper Krom River in the Western Cape (Avidon et al., 2018). Oncorhynchus mykiss invasions have also led to a decline in the abundance of river minnows such as the Breede River redfin (Pseudobarbus burchelli), the Cape kurper (Sandelia capensis), and the Cape galaxies (Galaxias zebratus) in the Breede River system, Western Cape (Shelton et al., 2015a). Also, in the Breede River, O. mykiss invasions were shown to induce a trophic cascade by releasing some herbivorous invertebrates from predation, leading to an increase in grazing pressure and lower algal biomass at invaded sites (Shelton et al., 2015b). Oncorhynchus mykiss invasions in several river systems such as the Keiskamma River (Eastern Cape), the Thukela and the Umgeni River systems (KwaZulu Natal) have been shown to modify aquatic-terrestrial food-web linkages by competing for and reducing the trophic subsidies available for riparian consumers such as spiders (Jackson et al., 2016).
In this study, we examined the trophic structure of fish communities in the upper Blyde River catchment in Mpumalanga Province where O. mykiss was introduced into several headwater streams of the catchment in the early 1900s for sport fishing (Engelbrecht and Roux, 1998). The subsequent establishment of naturalised populations of O. mykiss poses a risk to endangered indigenous fish species through predation and competition for habitat and food resources as a result of resource overlaps with O. mykiss. For example, the Treur River barb (Enteromius treurensis) is endemic to the upper reaches of the Blyde River (Kleynhans, 1987). It was extirpated from several sections of the Blyde River system invaded by O. mykiss, brown trout (Salmo trutta), and smallmouth bass (Micropterus dolomieu) (Gaigher, 1969; Kleynhans, 1987; Engelbrecht and Roux, 1998). The remnant populations of the Treur River barb are fragmented but largely intact, restricted to the upper river sections of the catchment because a series of waterfalls have prevented upstream migrations of invasive predatory fishes from lower river sections where introductions occurred. A recent study in the upper Blyde River catchment demonstrated that O. mykiss invasions have caused a decline in the abundance of populations of indigenous fish species such as E. treurensis (Maimela et al., 2021). However, the mechanism(s) through which these impacts have manifested remains unknown. Trophic niche analysis has been used to assess the ecological opportunities available to alien species in terms of food resources and how factors that affect access to, and utilisation of, food resources facilitate the successful establishment and adverse impacts in a recipient ecosystem (e.g., Tonella et al., 2018). This study, therefore, investigated the trophic interrelationship between O. mykiss and the indigenous fish species in the upper Blyde River catchment, by assessing whether O. mykiss invasion altered community structure and function, such as trophic functional diversity and trophic niche size of indigenous fish species. It is likely, as demonstrated elsewhere, that O. mykiss invasions could lead to niche shifts and a decline in indigenous fish populations through either predation or competitive exclusion from food resources (e.g., McDowall, 2006; Kadye et al., 2013; Shelton et al., 2015a; Weyl et al., 2020). This study, therefore, tested the hypotheses that the O. mykiss invasion in the upper Blyde River catchment has decreased the trophic functional diversity of indigenous fish species through predation and that indigenous species have shifted their niche width (i.e., the variety of food items utilised by a species) and position (i.e., type of food resources utilised) to minimise competition with, or avoid predation, by O. mykiss.
The study area included the headwaters of the Blyde and Treur rivers in the northern part of the Great Drakensburg escarpment, Mpumalanga Province, South Africa (Figure 1). The study sites were located on the upper slopes of the escarpment (1,200–1,500 m above sea level (a.s.l.)) that are characterised by grassland vegetation that is interspaced by Afromontane forests. The climate is cool to moderate (10–18°C), moderate to high annual rainfall (800–1,200 mm), and mean annual precipitation of more than 1,000 mm (WRC, 2001).
FIGURE 1. A map showing the location of sites sampled. Sites invaded by rainbow trout (Oncorhynchus mykiss) (•) and sites free of invasion (o) in the Blyde and Treur rivers in Mpumalanga Province, South Africa.
Fish were sampled between September 2017 and October 2018 from 7 sampling sites (Figure 1; Table 1) and each site was sampled once every three months on five different occasions. To assess the impact of O. mykiss invasion on trophic niche utilised by indigenous fishes, sites were grouped based on their invasion status. A site was categorised as invaded if O. mykiss was present at the site or at upstream sites where downstream dispersal is possible. Four sampling sites were selected from the upper Blyde River, two in uninvaded sites (sites 1 and 2) and two in invaded river sections (sites 2–4) (Table 1). Three additional sites (sites 5–7) were located in the upper sections of the Treur River that are free of alien fish. All the sites were sampled using a SAMUS725MP electrofisher (Samus Special Electronics, Warsaw, Poland), while fyke nets were used at all sites on the Blyde River but not in the Treur River because of low water levels. A river stretch of ∼50 m was blocked off to prevent fish escapes, and electrofishing was conducted for ∼30 min, with the electrofisher being operated in an upstream direction with a 1 m deep x 2 m wide, 5 mm mesh mobile block net behind to catch stunned fish missed by the operator (Kimberg et al., 2014). Fyke nets were set in the evening and retrieved the following morning. Fish were sorted according to species, weighed (g), and total length (TL, mm) recorded, and then preserved in ethanol for further analysis.
TABLE 1. Sampling sites on the Blyde and Treur rivers in Mpumalanga Province, South Africa and their associated rainbow trout (Oncorhynchus mykiss) invasion status, geographic coordinates, and elevation.
Gut content and stable isotope analyses were used to determine diet and trophic interactions between O. mykiss and indigenous fish species. The simultaneous use of gut content analysis and stable isotope analysis (SIA) allows for a better estimate of the overall fish diet and better insights into the trophic levels of fish communities that reflect different spatial and temporal scales (see Hyslop, 1980; Fry, 2006; Finlay and Kendall, 2007; Marshall et al., 2007; Zengeya et al., 2011; Kadye and Booth, 2012). Gut content analysis was used to identify possible food sources, and this was achieved by sampling fish from both invaded and uninvaded sites to obtain an overall diet spectrum for each sampled species, SIA was then used to differentiate food niches among fish species concerning trophic space, width, and the degree of overlap.
Fish gut contents were examined using a microscope and identified to the lowest possible taxonomic level. The diet of each fish species was determined using the frequency of occurrence (% F) (Hyslop, 1980). The approach provides estimates of the number of stomachs containing a specific prey item as a percentage of all analysed stomachs. It has been shown to provide a more robust and interpretable measure of diet composition than other quantitative approaches (Baker et al., 2014; Buckland et al., 2017; Amundsen and Sánchez-Hernández, 2019). The observed food items included algae, plant material and insects (Supplementary Table S1). The food items identified in the gut content analysis were then collected from the environment to delineate the food web structure in the river system. Algae and plant material were collected by hand, while aquatic invertebrates were collected using kick-net sampling (mesh size = 1 mm) on all available substrates at each site following the SASS protocol (Dickens and Graham, 2002). The aquatic invertebrates were identified up to family level using a photographic identification manual developed for South African river systems (Gerber and Gabriel, 2002). The differences in community structure of fish and invertebrates among the sites were tested using multivariate PERMANOVA based on a Bray–Curtis similarity matrix of fourth root transformed abundance data with 9,999 permutations. The PERMANOVA was based on algorithms in PAST v3 (Hammer et al., 2001).
Muscle tissue was excised from all fish samples while insects were analysed whole following similar studies done in the region (e.g., Lübcker et al., 2016; Taylor et al., 2017; Lombard et al., 2018; Bokhutlo et al., 2021). Insects were combined so that they included representatives from the following seven orders: Coleoptera, Diptera, Ephemeroptera, Hemiptera, Lepidoptera, Odonata and Trichoptera. Algae, plant material, insect samples and muscle tissue were oven-dried at 70°C for 24 h. SIA was undertaken on a Flash EA 1112 Series coupled to a Delta V Plus stable light isotope ratio mass spectrometer via a ConFlo IV system (all equipment supplied by Thermo Fischer, Bremen, Germany), housed at the Stable Isotope Laboratory, Mammal Research Institute (MRI), University of Pretoria, Pretoria, South Africa. A laboratory running standard (Merck Gel: δ13C = - 20.57%; δ15N = 6.8%; C% = 43.83; N% = 14.64) and blank sample were run after every 12 samples. The standards were Vienna Pee Dee Belemnite limestone for δ13C (Craig, 1957) and atmospheric nitrogen for δ15N (Ehleringer and Rundell, 1989). Results were expressed in delta notation using a parts per thousand scale following the standard equation by Craig (1961) (Eq. 1):
Where X = 15N or 13C and R = 15N/14N or 13C/12C, respectively. Analytical precision was <0.05‰ for δ13C and <0.09‰ for δ15N.
Stable isotope values for basal resources can vary considerably along spatial and temporal gradients, and to correct for these differences, nitrogen values (δ15N) were converted to the trophic position of each consumer (TP), and carbon values (δ13C) were corrected to the carbon of each consumer (Ccorr) following Olsson et al. (2009); (Eqs 2, 3)
where δ15Nconsumer = the isotopic ratio of each consumer, and δ15Nbaseline = the isotopic ratio and trophic position of a primary consumer. The trophic enrichment factor was set 3.4 following the meta-analysis of Post (2002).
where δ13Cconsumer = the isotopic ratio of each consumer, δ13Cmeaninv = the mean invertebrate isotope ratio, and CRinv = the invertebrate carbon range (δ13Cmax - δ13Cmin).
Ideally, the δ15Nbaseline is estimated using the isotopic signature of a long-lived filter-feeding primary consumer (e.g., bivalves; Vander Zanden and Rasmussen, 1999; Post, 2002), but the absence of long-lived filter-feeding primary consumers at the study sites necessitated the use of mean nitrogen values of aquatic insects as recommended by Jackson and Britton (2014). These include several families of dragonflies (Odonata), damselflies (Odonata), mayflies (Ephemeroptera), crane flies (Diptera), giant water bug (Hemiptera), and whirligig beetles (Coleoptera). The insect families that occurred at all sampling sites were dragonflies, mayflies, crane flies, and whirligig beetles. The isotopic signatures of mayfly insects showed no significant differences for δ15N (Welch: F = 1.12, df = 12.92, p > 0.05) and δ13C values (Welch: F = 0.02, df = 13.62, p > 0.05) between sampling sites, and were used to determine nitrogen baseline (δ15Nbaseline), mean carbon invertebrate ratio (δ13Cmeaninv), and the invertebrate carbon range (CRinv). The isotopic baseline correction for nitrogen (δ15N) and carbon values (δ13C) to trophic position (TP) and carbon corrected values (Ccorr) allowed for comparisons of trophic interactions among fish communities across a stream where sampling sites were grouped into qualitative categories based on their invasion status (i.e., free of, or invaded by O. mykiss) and location (Figure 1).
A Bayesian mixing model (simmr, Parnell et al., 2010; R Core Team 2021) was used to estimate the relative proportion of basal food resources that were assimilated by O. mykiss and indigenous fish species. The mixing model was calibrated using food sources that were identified from the gut content analysis. Food items were grouped into broad taxonomic categories: algae, plant material, and insects were grouped into orders (Coleoptera, Diptera, Ephemeroptera, Hemiptera, and Odonata). Models were constructed using uncorrected stable isotope data. The trophic enrichment factors were set at 3.37 ± 1.30% for nitrogen and 0.54 ± 0.53% for carbon following Taylor et al. (2017) and Bokhutlo et al. (2021). Model performance and fit were checked using the convergence diagnostic tool and by visually checking the posterior probability distributions.
Shifts in trophic niche position for each fish species were determined using measures of central tendency (i.e., the difference in centroid position between species) following the approach by Turner et al. (2010). Several metrics, trophic position range (TP range), corrected carbon (Ccorr range), mean distance to the centroid (CD) and mean nearest neighbour distance (NND) were used to calculate niche dispersion (see Layman et al., 2007). TP range provides an estimate of the trophic length of the community, the Ccorr range provides an estimate of the diversity of food resources, the CD represents the mean of Euclidean distance of each species to the sample bi-plot centroid, where the mean of TP values and Ccorr of all species in the food web represent the sample centroid. NND represents the mean of Euclidean distances to each species’ nearest neighbour in a TP and Ccorr - bi-plot. A lower dispersion in the two dispersion metrics of trophic diversity of a system indicates trophic redundancy. The significance of the test statistics for the niche position and dispersion metrics were then evaluated using null distributions generated from nested linear models and a residual permutation procedure (Turner et al., 2010). Post-hoc analysis of significant differences was done using one-way analysis of variance (ANOVA) posthoc test.
A residual permutation procedure was used to assess if changes in the trophic niche position of a species were due to the invasion status of the sampling site (see Turner et al., 2010). This was done by comparing the magnitude (path length) and direction of change (path direction) in centroid positions for each species sampled in the invaded and uninvaded sites. Path length was taken as the Euclidean distance between a pair of centroid positions sampled from different sites, and path direction was defined as the angle (θ) between the first principal component (PC1) vectors (see Collyer and Adams, 2007; Adams and Collyer, 2009).
The niche space utilised by the fish species was delineated using sample size-corrected standard ellipse area (SEAc) that were derived using the R package Stable Isotope Ellipses (SIBER) (Jackson et al., 2011). The SEAc were constructed using variance and covariance of the isotope biplot that contained only 40% of the data, which represents the core isotopic and typical resources utilised by a species. A Markov Chain Monte Carlo (MCMC) simulation with 104 iterations that provides 95% confidence limits of the isotopic niche size for each species sampled was used for a Bayesian estimate of SEAc and its area (Jackson et al., 2011). A maximum likelihood-fitted SEAc was used to quantify the magnitude of niche size overlap between species and was expressed as the proportional area that overlapped between a pair of SEAc divided by the total area of both ellipses (Jackson et al., 2011). Extent of overlap was classified as low (<40%), intermediate (40–60%) and high (>60%) (Langton, 1982).
There were no significant differences in composition (PERMANOVA: p > 0.05) and abundance (PERMANOVA: p > 0.05) among the invertebrate assemblages based on the invasion status of the site (Table 2). The most abundant taxa across all sampling sites were Odonata (64% of the total) and Diptera (11%) which collectively made up 76% of the total number of invertebrates collected (Table 2). There were however, significant differences in species composition, (PERMANOVA: p < 0.05) and abundance (PERMANOVA: p < 0.05) among fish assemblages based on invasion status and site location (Blyde or Treur River) (Table 2). In the Blyde River, sites (1 and 2) were uninvaded by O. mykiss and only two species were collected from these sites, E. treurensis and the stargazer mountain catfish (Amphilius uranoscopus), of which E. treurensis was highly abundant. In contrast, O. mykiss was abundant at sites 3 and 4 but A. uranoscopus and E. treurensis were sparse. Sites 5-7 were located on the Treur River and were uninvaded by O. mykiss. The sites had a higher species diversity because of three additional species chubbyhead barb (E. anoplus), E. lineomaculatus (line spotted barb) and banded tilapia (Tilapia sparrmanii) that were caught from these sites but not on the Blyde River (Table 2).
TABLE 2. The composition and abundance (by numbers) of invertebrate and fish taxa sampled from sites invaded by rainbow trout (Oncorhynchus mykiss) on the Blyde River and sites free of invasion both the Blyde River and Treur rivers. The numbers of fish outside parentheses indicate the total number of individuals collected using electrofishing and those in parentheses using fyke nets.
Fish communities in the uninvaded river sections of the Blyde River comprised only two species, E. treurensis and A. uranoscopus, that both primarily fed on Diptera and Ephemeroptera (Table 3). The two species, however occupied significantly different centroid positions (p < 0.05), because of differences in Ccorr values (ANOVA: F2, 56 = 29.8; p < 0.05) but the TP values were similar (ANOVA: F 2, 56 = 3.3; p > 0.05) (Figure 2A).
TABLE 3. Proportion (% of isotopic value) of food sources utilised by rainbow trout (Oncorhynchus mykiss) and indigenous fish species in the catchments of the Blyde and Treur rivers, Mpumalanga Province, South Africa. ± denotes standard deviation.
FIGURE 2. Centroid positions, and estimates of niche size and overlap using sample size-corrected standard ellipse areas (SEAc) of fish species sampled from sites invaded by rainbow trout (Oncorhynchus mykiss) on the Blyde River (B,E) and sites free of invasion both the Blyde (A,D) and Treur rivers (C,F).
Fish communities in the invaded river reach of the Blyde River comprised three species that fed mainly on insects but also consumed algae, and macrophytes (Supplementary Table S4). The three species occupied significantly different centroid positions (p < 0.05) because of differences in TP values (ANOVA: F2, 37 = 9.29; p < 0.05) but Ccorr values were similar (ANOVA: F5, 120 = 3.9; p > 0.05) (Figure 2B). Enteromius treurensis (SEAc = 0.05) and O. mykiss (SEAc = 0.03) utilised similar-sized isotopic niche spaces (p > 0.05) that were significantly larger than the isotopic niche space utilised by A. uranoscopus (SEAc = 0.01) (Figure 2E). The extent of overlap between A. uranoscopus - E. treurensis (0.11), and A. uranoscopus - O. mykiss (0.11) was low but the was an intermediate overlap between E. treurensis and O. mykiss (0.55) (Figure 2E).
The fish species from the uninvaded Treur River could be separated into two groups. Four insectivores, A. uranoscopus, E. anoplus, E. treurensis, and E. lineomaculatus, fed mainly on insects while T. sparrmanii was an omnivore and fed on algae and insects (Table 3). Amphilius uranoscopus had the highest TP values (TP = 2.72 ± 0.14, Ccorr = 0.53 ± 0.09) that was significantly different from E. lineomaculatus (TP = 2.35 ± 0.12, Ccorr = 0.57 ± 0.11) and E. treurensis (TP = 2.51 ± 0.11, Ccorr = 0.63 ± 0.10), while T. sparrmanii (TP = 2.15 ± 0.27, Ccorr = 0.58 ± 0.12) had the lowest TP values that were significantly different from all the other species (ANOVA: F5,120 = 18.24; p < 0.05) (Figure 2C, Supplementary Table S2). There were no significant differences in Ccorr values (range = 0.41–0.63) among the five species (ANOVA: F5, 120 = 3.9; p > 0.05). Tilapia sparrmanii utilised the largest isotopic niche (SEAc = 0.11 ± 0.1) while the rest of the species utilised smaller but similar-sized isotopic niches (A. uranoscopus = 0.03 ± 0.03, E. anoplus = 0.03 ± 0.02, E. lineomaculatus = 0.04 ± 0.04, and E. treurensis = 0.04 ± 0.03) (SIBER p < 0.05) (Figure 2F, Supplementary Table S2). The extent of overlap was low for most species except for intermediate overlap between E. lineomaculatus and E. treurensis (45%) and high overlap between E. anoplus and E. treurensis (62%) (Supplementary Table S4).
The isotopic food web varied in structure across the invaded and uninvaded sites from the Blyde and Treur rivers. The fish communities from the invaded section of the Blyde River had smaller niche metrics [total area of the convex hull (TA), trophic range (TP range), and Ccorr range] than fish communities in the uninvaded sections in the Blyde and Treur rivers (Supplementary Table S5). In addition, there were significant differences (p < 0.05) in the mean community centroid positions across sites in the uninvaded (Ccorr = 0.19, TP = 2.55) and invaded (Ccorr = 0.38, TP = 2.88) sections of the Blyde River and invasion free Treur River (Ccorr = 0.58, TP = 2.37). The mean distance to the centroid of fish communities in uninvaded sections of the Blyde (CD = 0.22) and Treur River (CD = 0.24) was higher and significantly different (p < 0.05) from fish communities in the invaded section of the Blyde River (0.15). In contrast, the nearest-neighbour distance (NND) was not significantly different (p > 0.05) (Supplementary Table S5).
Only two species (A. uranoscopus and E. treurensis) were sampled across invaded and uninvaded sites in the Blyde and Treur rivers. The two species occupied significantly different centroid positions from each other across the uninvaded and invaded sites (Figure 3). The centroid position of A. uranoscopus from uninvaded sections of the Blyde River was characterised by depleted TP (2.37 ± 0.06) and Ccorr values (0.16 ± 0.01) relative to invaded sections (TP = 3.15 ± 0.09 and Ccorr values = 0.33 ± 0.03) and invasion free Treur River (TP = 2.72 ± 0.14 and Ccorr = 0.53 ± 0.09). A similar trend was also observed for E. treurensis were the centroid position in invaded section of the Blyde river had enriched TP and Ccorr values (TP = 2.91 ± 0.11 and Ccorr = 0.39 ± 0.15) relative to invasion free sections of both the Blyde (TP = 2.56 ± 0.17, Ccorr = 0.22 ± 0.11) and Treur rivers (TP = 2.51 ± 0.11 and Ccorr values = 0.63 ± 0.1). There were no significant differences (p > 0.05) in path length, direction and shape between the centroid positions of the two species among invaded and uninvaded sites across the two rivers (Figure 3).
FIGURE 3. The magnitude and direction of change in centroid positions of Enteromius treurensis and Amphilius uranoscopus across invaded and uninvaded sites on the Blyde and Treur rivers in Mpumalanga Province, South Africa.
This study assessed food web interactions between fish communities in river sections invaded by O. mykiss and sections that are still free of invasion in the upper reaches of the Blyde River catchment. Oncorhynchus mykiss was an insectivore and fed at a similar trophic level to that of indigenous fish species. Similar results have been reported elsewhere in the uKhahlamba Drakensberg Park and Cape Floristic Region of South Africa where introduced trout (S. trutta and O. mykiss) have been observed to feed at similar and or lower trophic levels to indigenous fishes (e.g., Rivers-Moore, et al., 2013; Jackson et al., 2016; Weyl et al., 2020). Oncorhynchus mykiss is known to impact indigenous fish fauna through various mechanisms such as competition for resources (food and space), predation, alteration of food web structures and changes in habitat coupling, as well as overall ecosystem-level effects (e.g., Eby et al., 2006; McDowall, 2006; Karssing et al., 2012; Ellender, 2013; Shelton et al., 2015a). Isolating the relative individual contribution of each of these mechanisms to the overall impact on the food web is difficult (e.g., see Crowl et al., 1992; Townsend, 2003). The fact that O. mykiss and indigenous fish communities were feeding at the same trophic level suggests that their present impact on indigenous fish communities in the upper Blyde River Catchment is more likely through competition for resources.
Competition for food and space between Oncorhynchus mykiss and indigenous species can lead to adverse impacts on community structure and function (e.g. Eby et al., 2006). Such impacts include a decline and fragmentation of indigenous species populations and niche shifts (e.g., Kadye et al., 2013; Shelton et al., 2015a). Oncorhynchus mykiss and E. treurensis utilised a similar-sized isotopic niche that had intermediate overlap (55%). Oncorhynchus mykiss is a general predator but it has been shown to feed primarily on terrestrial invertebrates, while E. treurensis utilises a wider variety of invertebrate prey (Kleynhans, 1987). This is consistent with observations from gut contents in this study where O. mykiss fed mainly on aquatic invertebrates but the E. treurensis had a broader diet spectrum that included algae and aquatic invertebrates. The high proportion of aquatic invertebrates in the diets of O. mykiss and E. treurensis likely lead to the observed high niche overlap between the two species. This indicates that O. mykiss is not using a vacant trophic niche in this system, but is instead using similar food resources to E. treurensis, potentially competitively displacing it. This notion is supported by the fact that river sections with high O. mykiss densities were characterised by a low abundance of E. treurensis (96% lower) relative to uninvaded areas.
In addition, competition often leads to niche shifts by the weaker competitor to minimise competition (Kramer and Drake, 2014). However, the effects of interspecific competition on the niche widths of consumers are often context-specific (Araújo et al., 2011). For example, competing species can utilise smaller niche spaces to reduce competition by minimising overlap in resource use (e.g., Bolnick et al., 2010; Jackson et al., 2016) or increase their niche width in order to maintain access to limited resources (e.g., Svanbäck and Bolnick, 2007). In this study, A. uranoscopus and E. treurensis shifted their niche and fed at a higher trophic level in invaded areas than in uninvaded areas. The increase in trophic level was likely associated with an increase in the variety of food items utilised by a species in invaded sections relative to uninvaded areas. Both species fed predominately on Ephemeroptera larvae in uninvaded sections of the Blyde River and on Hemiptera and Odonata in the uninvaded Treur River but increased their niche width in invaded sections of the Blyde River to include greater proportions of algae and other aquatic invertebrates such as Diptera, Hemiptera and Odonata. The niche shift by A. uranoscopus and E. treurensis between invaded and uninvaded river sections may be linked to differences in access to food resources rather than food availability as there were no clear differences in food resources (aquatic invertebrates) available to fishes based on the invasion status. There were however significant differences in fish composition and abundance among sites. Indigenous fish species such as E. treurensis occurred at high densities at uninvaded sites relative to invaded sites. It is therefore likely that intraspecific competition for food resources might have constricted the niche width of indigenous fishes at uninvaded sites and conversely, niche expansion occurred at invaded sites because of increased access to food resources that may have been otherwise depleted or monopolised by competitors (Bolnick et al., 2010). This notion is supported by other comparative studies that have observed increases in invertebrate diversity in areas invaded by O. mykiss that might be due to O. mykiss invasions reducing the abundance of indigenous fish and thereby reducing predation pressure on aquatic invertebrates, with knock-on effect on the structure of these assemblages. For example, in the upper Breede catchment, O. mykiss were characterised as weaker regulators of invertebrate abundance than the indigenous river minnows which they replace (Shelton et al., 2015b, 2017). Similar observations were found in mountain headwater streams in eastern Zimbabwe where there was a higher abundance of some invertebrate taxa in areas invaded by trout which could be linked to decreased predation pressure from indigenous invertebrates and fish that occurred in lower abundance in invaded areas (Kadye et al., 2013).
Although not observed in this study, O. mykiss has also been observed to selectively consume small river minnows (McIntosh et al., 2010; Shelton et al., 2015a). The processes of competition and predation by alien fish are often linked and interact to determine net effects in invaded systems (e.g., Eby et al., 2006; David et al., 2017). It is, therefore, possible that the decline in populations of indigenous fishes in the upper Blyde River catchment might have been through a combination of predation and competition from O. mykiss invasions. The trophic niche concept has been used to assess the impacts of alien species on community structure and function but its use can be hindered by several limitations some of which were evident in this study. These include a lack of long-term monitoring studies to track trends. The sites sampled in this study have been invaded for over a century and there are no monitoring studies that have been done to measure long-term trends in community absolute abundance, predator demands and community compensation mechanisms. Therefore the observed trophic interrelationships may represent a point-in-time snapshot of foodweb dynamics that may shift over time. Second, there are difficulties in determining the food utilised by fish species from sites that are in a continuum along a river profile as upstream river segments often affect nutrient dynamics in downstream segments. In this study, to minimise spatial dependencies of food webs along a river continuum, an isotopic baseline correction was done to allow for comparisons of trophic interactions among fish communities along spatial gradients (see Olsson et al., 2009; Jackson and Britton, 2014). Third, the number of individuals varied significantly among sites, where a species could be abundant at one site but rare at other sites. To avoid data dependency problems among sites, sampling effort was standardised and sites were pooled together based on the invasion status (presence or absence of O. mykiss) and location. However, some sites had low sample sizes and the confidence level in some of the estimates of food web matrices could be improved by increasing the sample sizes. Despite these caveats, this study demonstrated that there are spatial differences in trophic functional diversity among the fish communities in the upper Blyde River catchment that are correlated to O. mykiss invasions. Fish communities in uninvaded areas had greater trophic redundancy than fish communities in invaded areas. These spatial differences in trophic functional diversity are likely a result of O. mykiss invasion through competition for resources with indigenous fishes. We posit O. mykiss invasions have reduced the abundance of indigenous fishes and thereby reducing predation pressure on aquatic invertebrates, with a knock-on effect on the trophic interrelationships among fish assemblages. This is consistent with observations elsewhere that have noted that O. mykiss invasions can influence the diet and trophic niches of indigenous fish by altering the structure and dynamics of food webs through various mechanisms such as predation and competition.
The original contributions presented in the study are included in the article/Supplementary Material, further inquiries can be directed to the corresponding author.
The animal study was reviewed and approved by the University of Pretoria Animal Ethics Committee.
All authors listed have made a substantial, direct, and intellectual contribution to the work and approved it for publication.
This study was funded by the DSI-NRF Centre of Excellence for Invasion Biology and the South African Department of Forestry, Fisheries, and the Environment (DFFE) noting that this publication does not necessarily represent the views or opinions of DFFE or its employees. TZ acknowledges support from the National Research Foundation (Grant 103602).
The authors declare that the research was conducted in the absence of any commercial or financial relationships that could be construed as a potential conflict of interest.
The handling editor TD declared a past co-authorship with the author TZ.
All claims expressed in this article are solely those of the authors and do not necessarily represent those of their affiliated organizations, or those of the publisher, the editors and the reviewers. Any product that may be evaluated in this article, or claim that may be made by its manufacturer, is not guaranteed or endorsed by the publisher.
The Supplementary Material for this article can be found online at: https://www.frontiersin.org/articles/10.3389/fenvs.2022.949353/full#supplementary-material
Supplementary Table S1 | Frequency of occurrence (%) of prey items in the diets of indigenous and alien invasive fish species in the catchments of the Blyde and Treur rivers, Mpumalanga Province, South Africa.
Supplementary Table S2 | Trophic position (TP), corrected Carbon (Ccorr) and values of isotopic niche (SEAc) for indigenous and alien invasive (bold) fish species sampled in uninvaded and invaded catchments of the Blyde and Treur rivers, Mpumalanga Province, South Africa.
Supplementary Table S3 | Isotopic niche overlap among fish species in river sections invaded by rainbow trout (Oncorhynchus mykiss) on the Blyde River, South Africa. Extent of overlap was classified as low (< 40%), intermediate (40 to 60%) and high (> 60%) (Langton, 1982).
Supplementary Table S4 | Isotopic niche overlap among fish species in the Treur River, South Africa that is free of rainbow trout (Oncorhynchus mykiss) invasion. Extent of overlap was classified as low (< 40%), intermediate (40 to 60%) and high (> 60%) (Langton, 1982).
Supplementary Table S5 | Isotopic matrices of food webs of fish communities sampled in sites invaded by rainbow trout and sites free of invasion on the Blyde and Treur rivers, Mpumalanga Province, South Africa.
Adams, D., and Collyer, M. (2009). A general framework for the analysis of phenotypic trajectories in evolutionary studies. Evol 63 (5), 1143–1154. doi:10.1111/j.1558-5646.2009.00649.x
Amundsen, P., and Sánchez-Hernández, J. (2019). Feeding studies take guts – critical review and recommendations of methods for stomach contents analysis in fish. J. Fish. Biol. 95 (6), 1364–1373. doi:10.1111/jfb.14151
Araújo, M. S., Bolnick, D. I., and Layman, C. A. (2011). The ecological causes of individual specialisation. Ecol. Lett. 14, 948–958. doi:10.1111/j.1461-0248.2011.01662.x
Arlinghaus, R., and Cooke, S. J. (2009). “Recreational fisheries: Socioeconomic importance, conservation issues and management challenges,” in Recreational hunting, conservation and rural livelihoods: Science and practice. Editors B. Dickson, J. Hutton, and W. A. Adams (Oxford: Blackwell Publishing), 39–58.
Avidon, S., Shelton, J. M., Sean, M. M., Bellingan, T. A., Karen, E., and Weyl, O. L. F. (2018). Preliminary evaluation of non-native rainbow trout (Oncorhynchus mykiss) impact on the Cederberg ghost frog (Heleophryne depressa) in South Africa’s Cape Fold Ecoregion. Afr. J. Aquat. Sci. 43 (3), 313–318. doi:10.2989/16085914.2018.1507898
Baker, R., Buckland, A., and Sheaves, M. (2014). Fish gut content analysis: Robust measures of diet composition. Fish. Fish. 15 (1), 170–177. doi:10.1111/faf.12026
Bokhutlo, T., Keppeler, F. W., and Winemiller, K. O. (2021). Seasonal hydrology influences energy channels in food webs of rivers in the lower Okavango Delta. Environ. Biol. Fishes 104, 1303–1319. doi:10.1007/s10641-021-01156-x
Bolnick, D. I., Ingram, T., Stutz, W. E., Snowberg, L. K., Lau, O. L., and Paull, J. S. (2010). Ecological release from interspecific competition leads to decoupled changes in population and individual niche width. Proc. R. Soc. B 277, 1789–1797. doi:10.1098/rspb.2010.0018
Buckland, A., Baker, R., Loneragan, N., and Sheaves, M. (2017). Standardising fish stomach content analysis: The importance of prey condition. Fish. Res. 196, 126–140. doi:10.1016/j.fishres.2017.08.003
Cambray, J. A. (2003). The global impact of alien trout species-a review; with reference to their impact in South Africa. Afr. J. Aquat. Sci. 28 (1), 61–67. doi:10.2989/16085914.2003.9626601
Collyer, M. L., and Adams, D. C. (2007). Analysis of two-state multivariate phenotypic change in ecological studies. Ecology 88 (3), 683–692. doi:10.1890/06-0727
Craig, H. (1957). Isotopic standards for carbon and oxygen and correction factors for mass spectrometric analysis of carbon dioxide. Geochim. Cosmochim. Acta 12 (1-2), 133–149. doi:10.1016/0016-7037(57)90024-8
Craig, H. (1961). Standard for reporting concentrations of deuterium and oxygen-18 in natural waters. Science 133, 1833–1834. doi:10.1126/science.133.3467.1833
Crawford, S. S., and Muir, A. M. (2008). Global introductions of salmon and trout in the genus Oncorhynchus: 1870-2007. Rev. Fish. Biol. Fish. 18 (3), 313–344. doi:10.1007/s11160-007-9079-1
Crowl, T. A., Townsend, C. R., and McIntosh, A. R. (1992). The impact of introduced Brown and rainbow trout on native fish: The case of australasia. Rev. Fish. Biol. Fish. 2 (3), 217–241. doi:10.1007/BF00045038
David, P., Thébault, E., Anneville, O., Duyck, P. F., Chapuis, E., and Loeuille, N. (2017). Impacts of invasive species on food webs: A review of empirical data. Adv. Ecol. Res. 56, 1–60. doi:10.1016/bs.aecr.2016.10.001
Dickens, C., and Graham, P. M. (2002). The South African scoring system (SASS) version 5 rapid bioassessment method for rivers. Afr. J. Aquat. Sci. 27 (1), 1–10. doi:10.2989/16085914.2002.9626569
Drenner, R. W., and Hambright, K. D. (2002). Piscivores, trophic cascades, and lake management. Sci. World J. 2, 284–307. doi:10.1100/tsw.2002.138
Eby, L. A., Roach, W. J., Crowder, L. B., and Stanford, J. A. (2006). Effects of stocking-up freshwater food webs. Trends Ecol. Evol. 21 (10), 576–584. doi:10.1016/j.tree.2006.06.016
Ehleringer, J. E., and Rundell, P. W. (1989). “Stable isotopes: History and instrumentation,” in Stable isotopes in ecological research. Ecological studies. Editors P. W. Rundel, J. R. Ehleringer, and K. A. Nagy (New York, NY: Springer), 68. doi:10.1007/978-1-4612-3498-2_1
Ellender, B. R. (2013). Ecological consequences of non‐native fish invasion in Eastern Cape headwater streams. PhD thesis. Grahamstown: Rhodes University.
Ellender, B. R., Woodford, D. J., Weyl, O. L. F., and Cowx, I. G. (2014). Managing conflicts arising from fisheries enhancements based on non-native fishes in southern Africa. J. Fish. Biol. 85 (6), 1890–1906. doi:10.1111/jfb.12512
Engelbrecht, J. S., and Roux, F. (1998). A note on the reintroduction of Enteromius treurensis groenewald 1958 (pisces: Cyprinidae) into tiie Treur river, Mpumalanga, South Africa. South. Afr. J. Aquatic Sci. 24 (1-2), 153–156. doi:10.1080/10183469.1998.9631421
Epanchin, P. N., Knapp, R. A., and Lawler, S. P. (2010). Non-native trout impact an alpine-nesting bird by altering aquatic-insect subsidies. Ecology 91 (8), 2406–2415. doi:10.1890/09-1974.1
Fausch, K. D. (2007). Introduction, establishment and effects of non-native salmonids: Considering the risk of rainbow trout invasion in the United Kingdom. J. Fish. Biol. 71, 1–32. doi:10.1111/j.1095-8649.2007.01682.x
Finlay, J. C., and Kendall, C. (2007). “Stable isotope tracing of temporal and spatial variability in organic matter sources to freshwater ecosystems,” in Stable isotopes in ecology and environmental science. Editors R. Michener, and K. Lajtha. 2 ed. (Oxford: Blackwell Publishing). doi:10.1002/9780470691854.ch10
Flecker, A. S., and Townsend, C. R. (1994). Community-wide consequences of trout introduction in New Zealand streams. Ecol. Appl. 4 (4), 798–807. doi:10.2307/1942009
Gaigher, I. G. (1969). Aspekte met betrekking tot die ekologie, geografie en taksonomie van varswatervissoorte in die Limpopo- en Incomatiriviersisteem. Ph.D. thesis. Johannesburg: Rand Afrikaans University.
Gerber, A., and Gabriel, M. J. M. (2002). Aquatic invertebrates of South African rivers: A field guide. Pretoria, South Africa: Department of Water Affairs and Forestry, Resource Quality Services, pp150.
Hammer, Ø., Harper, A. T., and Ryan, P. D. (2001). Past: Paleontological statistical software package for education and data analysis. Paleontol. Electron. 4, 1–9. Available at: http://palaeo-electronica.org/2001_1/past/issue1_01.htm.
Hyslop, E. J. (1980). Stomach contents analysis-a review of methods and their application. J. Fish. Biol. 17 (4), 411–429. doi:10.1111/j.1095-8649.1980.tb02775.x
Jackson, M. C., and Britton, J. R. (2014). Divergence in the trophic niche of sympatric freshwater invaders. Biol. Invasions 16 (5), 1095–1103. doi:10.1007/s10530-013-0563-3
Jackson, A. L., Inger, R., Parnell, A. C., and Bearhop, S. (2011). Comparing isotopic niche widths among and within communities: SIBER - stable isotope bayesian ellipses in R. J. Anim. Ecol. 80 (3), 595–602. doi:10.1111/j.1365-2656.2011.01806.x
Jackson, M. C., Woodford, D. J., Bellingan, T. A., Weyl, O. L. F., Potgieter, M. J., Rivers‐Moore, N. A., et al. (2016). Trophic overlap between fish and riparian spiders: Potential impacts of an invasive fish on terrestrial consumers. Ecol. Evol. 6 (6), 1745–1752. doi:10.1002/ece3.1893
Kadye, W. T., and Booth, A. J. (2012). Integrating stomach content and stable isotope analyses to elucidate the feeding habits of non-native sharp tooth catfish Clarias gariepinus. Biol. Invasions 14 (4), 779–795. doi:10.1007/s10530-011-0116-6
Kadye, W. T., Chakona, A., Marufu, L. T., and Samukange, T. (2013). The impact of non-native rainbow trout within Afro-montane streams in eastern Zimbabwe. Hydrobiologia 720 (1), 75–88. doi:10.1007/s10750-013-1624-4
Karssing, R. J., Rivers‐Moore, N. A., and Slater, K. (2012). Influence of waterfalls on patterns of association between trout and Natal cascade frog Hadromophryne natalensis tadpoles in two headwater streams in the Ukhahlamba Drakensberg Park World Heritage Site, South Africa. Afr. J. Aquat. Sci. 37 (1), 107–112. doi:10.2989/16085914.2012.666381
Kimberg, P. K., Woodford, D. J., Roux, H., and Weyl, O. L. F. (2014). Species-specific impact of introduced largemouth bass Micropterus salmoides in the groot marico freshwater ecosystem priority area, South Africa. Afr. J. Aquat. Sci. 39 (4), 451–458. doi:10.2989/16085914.2014.976169
Kleynhans, C. (1987). A preliminary study of aspects of the ecology of a rare minnow Barbus treurensis Groenewald 1958 (Pisces: Cyprinidae) from the eastern Transvaal, South Africa. J. Limnological Soc. South. Afr. 13 (1), 7–13. doi:10.1080/03779688.1987.9634537
Kramer, A. M., and Drake, J. M. (2014). Time to competitive exclusion. Ecosphere 5, art52–16. doi:10.1890/es14-00054.1
Langton, R. (1982). Diet overlap between Atlantic cod, Gadus morhua, silver hake, Merluccius bilinearis, and fifteen other northwest Atlantic finfish. Fish. Bull. 80 (4), 745–759.
Layman, C. A., Arrington, D. A., Montaña, C. G., and Post, D. M. (2007). Can stable isotope ratios provide for community-wide measures of trophic structure? Ecol 88 (1), 42–48. doi:10.1890/0012-9658(2007)88[42:CSIRPF]2.0.CO;2
Lombard, R. J., Chimimba, C. T., and Zengeya, T. A. (2018). Niche complementarity between an alien predator and native omnivorous fish in the Wilge River, South Africa. Hydrobiologia 817 (6), 329–340. doi:10.1007/s10750-017-3352-7
Lübcker, N., Dabrowski, J., Zengeya, T. A., Oberholster, P. J., Woodborne, S., Hall, G., et al. (2016). Trophic ecology and persistence of invasive silver carp Hypophthalmichthys molitrix in an oligotrophic impoundment, South Africa. Afr. J. Aquat. Sci. 41, 399–411. doi:10.2989/16085914.2016.1246356
Maimela, L. T., Chimimba, C. T., and Zengeya, T. A. (2021). The effect of rainbow trout (Oncorhynchus mykiss) invasions on native fish communities in the subtropical Blyde River, Mpumalanga province, South Africa. Afr. J. Aquat. Sci. 47, 78–87. doi:10.2989/16085914.2021.1949260
Marshall, J. D., Brooks, J. R., and Lajtha, K. (2007). “Sources of variation in the stable isotope composition of plants,” in Stable isotopes in ecology and environmental science. Editors R. Michener, and K. Lajtha. 2nd ed. (Carlton: Blackwell Publishing). doi:10.1002/9780470691854.ch2
McDowall, R. M. (2006). Crying wolf, crying foul, or crying shame: Alien salmonids and a biodiversity crisis in the southern cool-temperate galaxioid fishes? Rev. Fish. Biol. Fish. 16 (3), 233–422. doi:10.1007/s11160-006-9017-7
McIntosh, A. R., Mchugh, P., Dunn, N. R., Goodman, J. M., Howard, S., Jellyman, P. G., et al. (2010). The impact of trout on galaxiid fishes in New Zealand. N. Z. J. Ecol. 34 (1), 195–206. Available at: https://newzealandecology.org/nzje/2909.pdf.
Olsson, K., Stenroth, P., Nyström, P., and Granéli, W. (2009). Invasions and niche width: Does niche width of an introduced crayfish differ from a native crayfish? Freshw. Biol. 54 (8), 1731–1740. doi:10.1111/j.1365-2427.2009.02221.x
Page, L. M., and Burr, B. M. (2011). A field guide to freshwater fishes of North America north of Mexico. Boston: Houghton Mifflin Harcourt, 663p.
Parnell, A. C., Inger, R., Bearhop, S., and Jackson, A. L. (2010). Source partitioning using stable isotopes: Coping with too much variation. PloS ONE 5 (3), e9672. doi:10.1371/journal.pone.0009672
Post, D. M. (2002). Using stable isotopes to estimate trophic position: Models, methods, and assumptions. Ecol 83 (3), 703–718. doi:10.1890/0012-9658(2002)083[0703:USITET]2.0.CO
R Core Team (2021). R: A language and environment for statistical computing. Vienna, Austria: R Foundation for Statistical Computing. Available at: https://www.R-project.org/.
Rivers‐Moore, N. A., Fowles, B., and Karssing, R. J. (2013). Impacts of trout on aquatic macroinvertebrates in three Drakensberg rivers in KwaZulu–Natal, South Africa. Afr. J. Aquat. Sci. 38 (1), 93–99. doi:10.2989/16085914.2012.750592
Schindler, D. E., Knapp, R. A., and Leavitt, P. R. (2001). Alteration of nutrient cycles and algal production resulting from fish introductions into mountain lakes. Ecosystems 4 (4), 308–321. doi:10.1007/s10021-001-0013-4
Shelton, J. M., Samways, M. J., and Day, J. A. (2015a). Predatory impact of non-native rainbow trout on endemic fish populations in headwater streams in the Cape Floristic Region of South Africa. Biol. Invasions 17 (1), 365–379. doi:10.1007/s10530-014-0735-9
Shelton, J. M., Samways, M. J., and Day, J. A. (2015b). Non-native rainbow trout change the structure of benthic communities in headwater streams of the Cape Floristic Region, South Africa. Hydrobiologia 745 (1), 1–15. doi:10.1007/s10750-014-2067-2
Shelton, J. M., Bird, M. S., Samways, M. J., and Day, J. (2017). Non-native rainbow trout (Oncorhynchus mykiss) occupy a different trophic niche to native Breede River redfin (Pseudobarbus burchelli) which they replaced in South African headwater streams. Ecol. Freshw. Fish. 26 (3), 484–496. doi:10.1111/eff.12293
Simon, K. S., and Townsend, C. R. (2003). Impacts of freshwater invaders at different levels of ecological organisation, with emphasis on salmonids and ecosystem consequences. Freshw. Biol. 48 (6), 982–994. doi:10.1046/j.1365-2427.2003.01069.x
Svanbäck, R., and Bolnick, D. I. (2007). Intraspecific competition drives increased resource use diversity within a natural population. Proc. R. Soc. B 274, 839–844. doi:10.1098/rspb.2006.0198
Taylor, G. C., Hill, J. M., Jackson, M. C., Peel, R. A., and Weyl, O. L. F. (2017). Estimating δ15N fractionation and adjusting the lipid correction equation using Southern African freshwater fishes. PLoS ONE 12 (5), e0178047. doi:10.1371/journal.pone.0178047
Tonella, L. H., Fugi, R., Vitorino, O. B., Suzuki, H. I., Gomes, L. C., and Agostinho, A. (2018). Importance of feeding strategies on the long-term success of fish invasions. Hydrobiologia 817, 239–252. doi:10.1007/s10750-017-3404-z
Townsend, C. R. (2003). Individual, population, community, and ecosystem consequences of a fish invader in New Zealand streams. Conserv. Biol. 17 (1), 38–47. doi:10.1046/j.1523-1739.2003.02017.x
Turner, T. F., Collyer, M. L., and Krabbenhoft, T. J. (2010). A general hypothesis-testing framework for stable isotope ratios in ecological studies. Ecology 91 (8), 2227–2233. doi:10.1890/09-1454.1
Van Wilgen, B. W., Zengeya, T. A., and Richardson, D. (2022). A review of the impacts of biological invasions in South Africa. Biol. Invasions 24 (2), 27–50. doi:10.1007/s10530-021-02623-3
Vander Zanden, M. J., and Rasmussen, J. B. (1999). Primary consumer d13C and d15N and the trophic position of aquatic consumers. Ecol 80 (4), 1395–1404. doi:10.1890/0012-9658(1999)080[1395:PCCANA]2.0.CO;2
Water Research Commission (2001). State of the rivers report: Crocodile, sabie-sand and olifants river systems. WRC report No. TT 147/01. Pretoria, South Africa: Water Research Commission.
Weyl, O. L. F., Ellender, B., Wassermann, R. J., Truter, M., Dalu, T., Zengeya, T. A., et al. (2020). “Alien freshwater fauna in South Africa,” in Biological invasions in South Africa. Editors B. W. van Wilgen, J. Measey, D. M. Richardson, J. R. Wilson, and T. A. Zengeya (Berlin: Springer), 153–183. doi:10.1007/978-3-030-32394-3_6
Zengeya, T. A., Booth, A. J., Bastos, A. D. S., and Chimimba, C. T. (2011). Trophic interrelationships between the exotic Nile tilapia, Oreochromis niloticus and indigenous tilapiine cichlids in a subtropical African river system (Limpopo River, South Africa). Environ. Biol. Fishes 92 (4), 479–489. doi:10.1007/s10641-011-9865-4
Zengeya, T. A., Kumschick, S., Weyl, O. L. F., and van Wilgen, B. W. (2020). “The impact of alien species on biodiversity in South Africa,” in Biological invasions in South Africa. Editors B. W. van Wilgen, J. Measey, D. M. Richardson, J. R. Wilson, and T. A. Zengeya (Berlin: Springer), 489–512. doi:10.1007/978-3-030-32394-3_17
Keywords: competition, predation, trophic dispersion, niche position, Enteromius treurensis, Treur River
Citation: Maimela LT, Chimimba CT and Zengeya TA (2022) Niche expansion by indigenous fish species following the introduction of rainbow trout (Oncorhynchus mykiss) in a subtropical river system, upper Blyde River, South Africa. Front. Environ. Sci. 10:949353. doi: 10.3389/fenvs.2022.949353
Received: 20 May 2022; Accepted: 23 August 2022;
Published: 27 September 2022.
Edited by:
Tatenda Dalu, University of Mpumalanga, South AfricaReviewed by:
Sergio Makrakis, Universidade Estadual do Oeste do Paraná, BrazilCopyright © 2022 Maimela, Chimimba and Zengeya. This is an open-access article distributed under the terms of the Creative Commons Attribution License (CC BY). The use, distribution or reproduction in other forums is permitted, provided the original author(s) and the copyright owner(s) are credited and that the original publication in this journal is cited, in accordance with accepted academic practice. No use, distribution or reproduction is permitted which does not comply with these terms.
*Correspondence: Lerato T. Maimela, bGVyYXRvLm1haW1lbGFAem9vbG9neS51cC5hYy56YQ==
†ORCID: Lerato T. Maimela, orcid.org/0000-0002-3691-9399; Christian T. Chimimba, orchid.org/0000-0002-8366-9994; Tsungai A. Zengeya, orcid.org/0000-0003-0946-0452
Disclaimer: All claims expressed in this article are solely those of the authors and do not necessarily represent those of their affiliated organizations, or those of the publisher, the editors and the reviewers. Any product that may be evaluated in this article or claim that may be made by its manufacturer is not guaranteed or endorsed by the publisher.
Research integrity at Frontiers
Learn more about the work of our research integrity team to safeguard the quality of each article we publish.