- 1College of Geography and Environmental Science, Henan University, Kaifeng, China
- 2Miami College, Henan University, Kaifeng, China
- 3Henan Key Laboratory of Earth System Observation and Modeling, Henan University, Kaifeng, China
- 4BNU-HKUST Laboratory for Green Innovation, Beijing Normal University, Zhuhai, China
In order to understand the heavy metal pollution and potential ecological risk of farmland soil around the waste dump in the eastern suburb of Kaifeng clearly and provide a scientific basis for the remediation of heavy metals in farmland soil, the single factor pollution index method, Nemero comprehensive pollution index method and Hakanson potential ecological risk method were used to evaluate the heavy metal pollution status and potential ecological risk degree of farmland in this area at the same time. The ArcGIS software was employed to map the spatial distribution of heavy metal pollution and potential ecological risks. The results show that the average values of heavy metals in this area are higher than the soil Background Value of Kaifeng City, and the problem of excessive heavy metals in the soil is significant. Of the 41 surface soil samples, Cd’s heavy and moderate pollution rates were up to 15% and 38%, respectively, and the potential ecological risk caused by Cd element was quite serious, and more than 35% of the soil samples were at higher potential ecological risk. The comprehensive review results showed that 38% of the samples were heavy pollution, and 23% of the sample soils were at higher potential ecological risk. The spatial distribution characterized by the levels of most heavy metals and the extent of potential ecological risk showed high levels in the center of the dump, with a gradual decrease towards the outward radiation zone. This means dust and leachate from waste dump are major causes of heavy metal contamination and potential ecological risks. In addition, the retrieval of the waste mound soil from landfills by villagers as soil fertilizer for rapeseed also caused, to some extent, the transfer of the waste mound contaminants.
Introduction
As China’s reform and opening-up progress continue to accelerate, the economy is rapidly developing, and its increasingly large city size, urban population, and area are increasing year by year. The consequent urban domestic waste is increasing, which has become a serious problem in urban development in China (Hogland et al., 2002; Huang et al., 2019). Kaifeng City is an important tourism city in Central China, where population growth and urbanization are also very rapid. Since 1990, the annual rise in waste production has been fast, among which municipal solid waste (MSW) is increasing rapidly. Meanwhile, limited by the technical conditions for domestic waste disposal in Kaifeng City, a large volume of domestic waste is mainly disposed of in simple landfills or indirect stacks. Therefore, a large number of hazardous materials, such as heavy metals (HMs), organic pollutants, microorganisms, etc., will be generated during the decomposition of domestic, municipal waste (Yahya et al., 2019), and the hazardous materials can seriously contaminate the local soil and the surrounding environment through fluctuations and downwelling fluids (Alam et al., 2020). Heavy metals in soils are toxic to plants, animals, and humans, and longer exposure leads to bioconcentration (Gao et al., 2019). MSW is usually composed of different forms of toxic and carcinogenic heavy metal elements that may penetrate soil and water bodies. Arsenic (As), cadmium (Cd), chromium (Cr), and nickel (Ni) are classified as class 1 carcinogens by the IARC and are also toxic in nature (IARC, 2018).
Moreover, heavy metal enrichment would be absorbed by different crops and thus enter into a complex food chain, thus causing serious ecological risk (Wakeel et al., 2020). In humans, long-term exposure to contaminated soil tends to negatively affect the central nervous, gastric, and respiratory systems (Khanam R. et al., 2019; Tseng et al., 2019). In addition, previous studies have reported that long-term exposure to heavy metals such as chromium, cadmium, and nickel can cause allergies, asthma, dermatitis, diarrhea, and even lung cancer (Bhattacharya et al., 2015; Moreira et al., 2018).
Current studies on MSW are mainly focused on the physicochemical properties of soil and soil heavy metal contamination after waste stacks (Wan et al., 2012; Li et al., 2014). Zhang et al. (2020) Studies have found that long-term dumps of waste cause a decrease in soil pH, while soil organic matter, total N, available P, and available K increase. Evaluation of the environmental quality of soils from landfill sites in Bangou County, Tibet, revealed that the levels of heavy metals were significantly elevated in the soil surrounding the landfill (Zeng et al. 2021). Li et al. (2015) measured Cu, Zn, Pb, and Cd levels in soil and vegetables from Kaifeng Eastern suburban agricultural soils and showed that Cd contamination was very severe and Zn and Pb genera were slightly contaminated, which was confirmed by Jiang Y. L. et al. (2020). Chen et al. (2020) determined that sewage irrigation and industrial and mining pollution of farmland soil in the eastern suburb of Kaifeng are the actual sources of soil heavy metal pollution in Kaifeng and proposed a multiple linear regression model with an attenuation function to quantify the accumulation of heavy metals in soil by previously determined pollution sources.
This research was focused on the open-air waste dump in the eastern suburb of Kaifeng. High-density samples were carried out on the surrounding farmland by concentric circle sampling, and the content characteristics and spatial distribution of heavy metals in soil were investigated and analyzed, the degree of soil heavy metal pollution and potential ecological risk were evaluated so as to provide a theoretical basis for a reasonable treatment of domestic waste and soil remediation. The study aims to provide data support and model determination for heavy metal pollution assessment of farmland around open-air waste dump.
Materials and methods
Study area
Kaifeng is located in the Middle East of Henan Province. The overall terrain of the whole city is relatively flat. The altitude range is 69–78m. The average annual precipitation is about 630mm, and the average annual temperature is 14°C. It is dominated by the southerly wind in summer and northerly wind in winter, belonging to the continental monsoon climate of the warm temperate zone. The soil quality of the Kaifeng area is formed by the alluvial of the Yellow River. The basic type belongs to yellow tide soil, with a deep soil layer and sandy texture. This study takes the waste dump (34°45′47.46″ N, 114°24′14.93″ E, 72m) as the center, and there are many villages nearby. Almost every village has several farmlands and grows a variety of agricultural products. The waste dump (This place is called Yangzhengmen Waste Mountain) covers an area of 0.066 km2. Due to the limited capacity of urban garbage treatment, it has been storing urban domestic garbage since 1960, forming a high garbage mountain. A total of 300000 m3 of garbage are accumulated in the waste dump. The Songlou, Hengchuanwan, Yangzhengmen villages, and other villages are located within 1 km of radiation. Within one kilometer around the waste dump, the color steel insulation board processing plant is located in the East, the vegetable planting area and atmospheric particulate matter settlement monitoring point are built in the southwest. Within 1–2 km around the waste dump, liulizhuang village is in the west, Baita village is in the northwest, and yuxiuqi ecological wetland park is also built in the southeast. Within 2–3 km around the waste dump, chenyanli village, Xiazhang village, Taohua village and other villages are located within the radiation range, and the East is the junction of urban and rural areas, with commercial, residential communities, primary and secondary schools and cash crop planting areas. The open stacking of waste dumps in the eastern suburb of Kaifeng has already affected the region’s ecological environment. The content of heavy metals in the surrounding farmland soil may reach the pollution level. Due to the enrichment of heavy metals in crops it has a potential threat to the health of local residents.
Sample collection
This study has taken the waste dump in the eastern suburb of Kaifeng as the center and investigates the pollution of farmland soil within 2 km around in a concentric circle (the sampling time was November 2020). The land is subordinate to Nanjiao Township, with a population of 23120. As shown in Figure 1, taking the waste dump as the center of the study area, the study is divided into an inner circle (500 m range, No. 1–17 samples), a middle circle (0.5–1 km range, No. 18–30 samples) and an outer circle (1–2.5 km, No. 31–41 samples). Soil samples are taken from waste dump and farms in the study area and shown in Figure 1 and Table 1. Soil samples were randomly collected by the plum blossom distribution method from 0 to 20 cm in the surface layer and 20–40 cm in the middle layer of croplands, and as shown in Figure 1, a total of 80 point soil samples were taken. Sample 5 kg soil at each point by quartering method, record the longitude, latitude, altitude, and other information of the sampling point, and mark it. The soil samples were air-dried (at room temperature 25°C), crushed, and stones were removed, and then screened with 20 mesh.
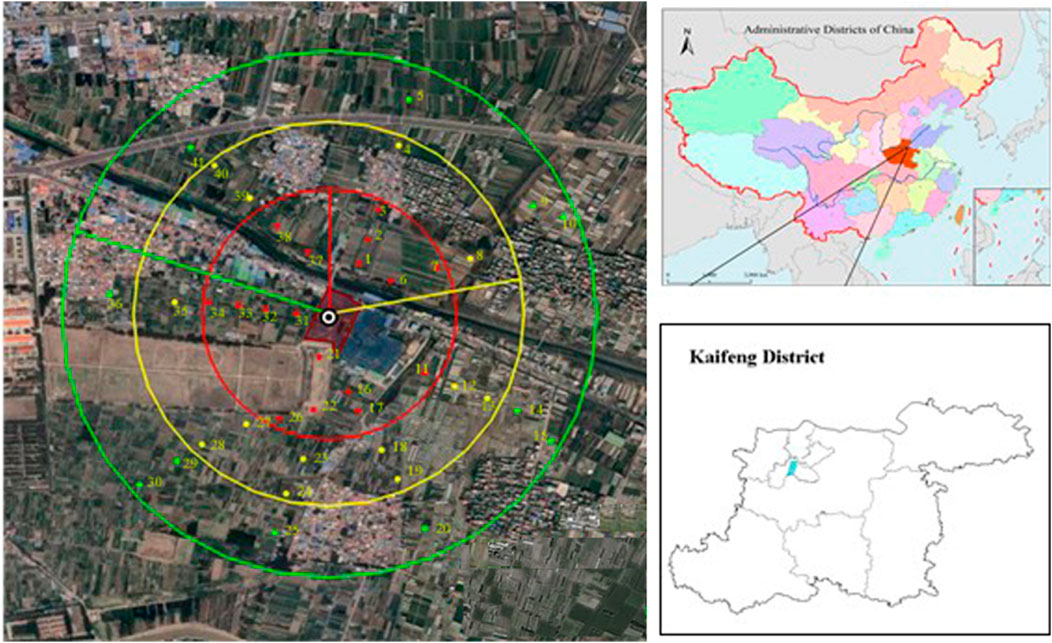
FIGURE 1. Map of the study area and distribution of sampling points. *The center of the concentric circle is the waste dump.
Methods of sample analysis
The pH was determined in deionized water with a ratio of 1:2.5 (w/v), using a pH meter (pHS-3C, Leici, China) (Biswas and Mukherjee, 2008). Available phosphorous (AP) and total nitrogen (TN) were extracted with sodium bicarbonate and determined by molybdenum antimony anti Colorimetry. Organic matter (OM) was titrated with potassium dichromate according to the trial implementation of standards for pollution risk control of agricultural land for soil environmental quality (Biswas and Mukherjee, 2008). The farmland pollution risk screening value items were mandatory items, including Cd, As, Pb, Cr, Cu, Ni, and Zn. The total amount of Cd, As, Pb, Cr, Cu, Ni, and Zn were determined by digestion of HNO3, HClO4 and HF (volume ratio 8:5:2). All samples were replicated 3 times, and the average value was taken.
Evaluation method of soil heavy metal pollution
In this study, the single factor pollution index method and Nemero comprehensive pollution index method were used to jointly evaluate the heavy metal pollution of farmland in this area (Khademi et al., 2019). The screening value of farmland pollution risk is shown in Table 2 (Egbueri et al., 2020; Jiang H. et al., 2020; Monged et al., 2020).
Single-factor pollution load index (Pi) and integrated pollution load index (IPi) were employed to assess the overall level of HMs pollution in the soil samples of the studied area. The pollution load index was determined using the Eq. 1:
where Pi is the pollution load index for the examined HMs, Ci is the concentration of HMs in a soil sample (mg kg−1), and Si is the permitted standard of the same metal (mg kg−1) (Men et al., 2018). For calculation of integrated pollution load index (PN), Eq. 2 was used as follows:
Pimax is the maximum value of Pi, and Piave is the average value of the sum of all Pi, i.e., the three HMs from the 20 sampling sites. If the Pi value is greater than unity, it suggests the existence of pollution or the presence of pollutants, while no pollution loads are inferred. Suppose the value is lower than or equal to unity. Among them, the grading criteria of PN are shown in Table 3 (Yari et al., 2021).
Combined with the principle of the single factor pollution index method, it is considered that as long as one pollutant exceeds the standard, the soil sample exceeds the standard, so the main heavy metals and their harm degree can be determined. The Nemero-comprehensive pollution index method considers a single factor with the most serious pollution and avoids the influence of subjective factors in the weight coefficient in the weighting process. It can more comprehensively reflect the soil environmental quality of the farmland (Yari et al., 2021). The classification and evaluation criteria of the heavy metal pollution index are shown in Table 3.
Potential ecological risk assessment method of soil heavy metals
The potential ecological risk index (PERI) is based on the necessary test item - farmland pollution risk screening value in the trial implementation of standards for pollution risk control of agricultural land for soil environmental quality. Hakanson’s potential ecological risk index method evaluated the ecological risk of heavy metal pollution in farmland soil in this area. The classification evaluation standard of the potential risk of heavy metals was established (Hakanson, 1980), as shown in Table 4.
In Table 4, the calculation formula of single factor potential ecological risk index
where is the corresponding Toxicity Coefficient of heavy metal element i (Cd is 30, As is 10, Pb is 5, Cr is 2, Cu is 5, Ni is 5, and Zn is 1). The calculation formula of comprehensive potential ecological risk index RI is
The single factor potential ecological risk index can reflect the risk degree of each heavy metal, respectively, and the comprehensive potential ecological risk index can reflect the comprehensive risk of a variety of heavy metals.
Data analysis
Through Excel 2019 software, the contents of 7 kinds of necessary heavy metal elements, soil pollution index, and potential ecological risk index of farmland soil around 40 sampling points of an open-air waste dump in the eastern suburb of Kaifeng are statistically analyzed and calculated, and the sampling points are located and mapped by GISMAP software. The classification and classification standard of colors are divided by different degrees corresponding to the single factor pollution index method, Nemero comprehensive pollution index method, and Hakanson potential ecological risk index method (explained by figure legend). According to the observed values of the known sample points in the area, the inverse distance weight method is used to predict the values other than the sample points in the area (Tong et al., 2012). Due to the low complexity of the data, in order to quickly interpolate from the sparse data on irregularly spaced samples, this study selects the inverse distance weight method (IDW) for spatial interpolation analysis and mapping through Arc Map software (Xiao et al., 2019).
Results and discussion
General characteristics of soils contaminated with municipal solid wastes
Through the potentiometric method for determining soil pH, the pH values of 40 farmland soil in the study area were measured. The results showed that the soil pH values of 80 samples (topsoil and middle soil) ranged from 7.21 to 8.52, the median was 7.73, and the standard deviation was 0.36, indicating that the soil in this area was slightly alkaline or alkaline. The average organic matter content of 80 soil samples in the study area was 2.03%, and the coefficient of variation was 46.96%; the average value of total nitrogen content was 16.00%, and the coefficient of variation was 23.10%; The average value of available phosphorus content was 0.24 g kg−1 and the coefficient of variation was 21.23%. Table 5 shows the specific physical and chemical properties and contents of the soil.
Spatial distribution of heavy metals
As shown in the data results in Table 6, the samples in the waste dump are seriously polluted, among which Cd, Pb, As and Zn exceed the standard seriously. The contents of 7 necessary heavy metals of farmland soil in the study area are shown in Table 6, in which the Cd concentration in surface soil ranges from 0.37 mg kg−1–3.00 mg kg−1. The range of Cd of 20–40 cm soil is 0.37–3.00 mg kg−1 and the median is 1.27 mg kg−1, which is similar to the median value of soil Cd (0.83 mg kg−1) of soil around Kaifeng reported by Li et al. (2015). The range of Ni in topsoil is 148.63–254.28 mg kg−1, with a median of 198.75 mg kg−1. The range of Ni in the middle soil is 168.33–245.37 mg kg−1, with a median of 1.27 mg kg−1, which is similar to the median value of soil Ni (0.83 mg kg−1) reported by Li et al. (2015). Based on the variation coefficients of these seven different heavy metals, it is found that the variation coefficient pattern is Cu (55.77%) > Cd (42.36%) > As (41.07%) > Zn (38.70%) > Cr (28.39%) > Pb (27.43%) > Ni (11.40%). Cu and Cd have strong variation coefficients, indicating that Cu and Cd are most affected by external pollution factors and human activities in this area. The variation coefficient of Ni is the smallest, indicating that it is less affected by human activities and the content changes little in space. In addition, the range of soil heavy metal content at each sampling point varies greatly. The average values of heavy metals measured are higher than the soil background value of Kaifeng City except for Cr. Among them, the more serious Cd, Cu, Pb, Ni, and Zn are 17.16, 2.65, 1.72, 6.67, and 4.29 times the background value, respectively, indicating that the long-term open-air waste stacking and atmospheric deposition in this area have caused heavy metal pollution in farmland soil, which may increase the potential ecological risk of farmland. The experimental results show that the average values of Cd and Ni in the measured heavy metals are higher than the risk screening values of farmland soil pollution, indicating that Cd and Ni elements may be risky to the quality and safety of agricultural products, crop growth or soil ecology, and soil environmental testing and agricultural products should be strengthened in collaboration Monitoring (Egbueri et al., 2020; Monged et al., 2020).
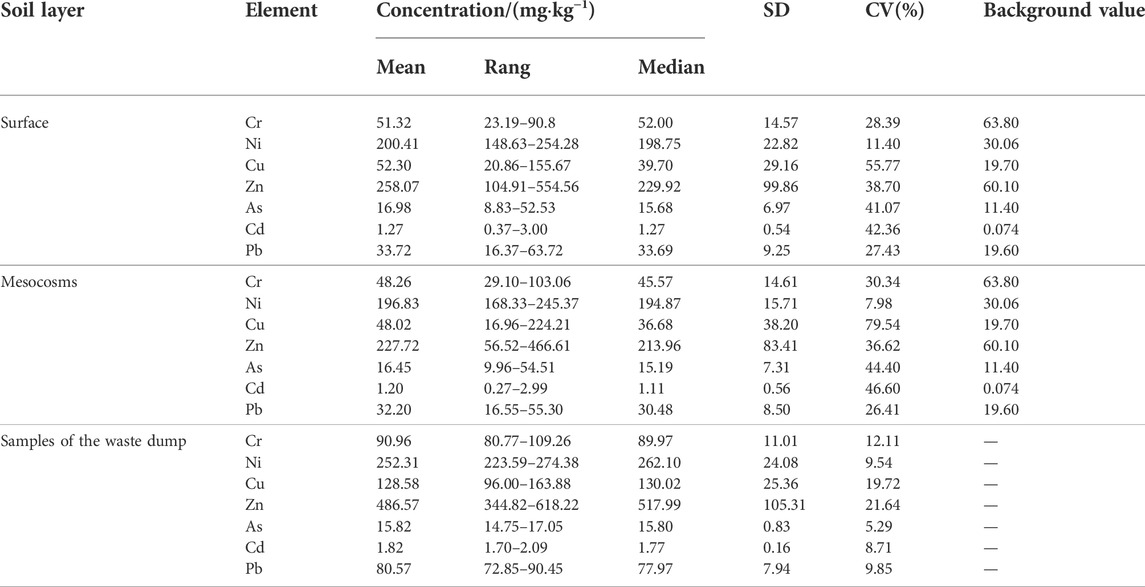
TABLE 6. Statistical results of heavy metals content in farmland soil and the waste dump samples in the study area.
Soil heavy metal pollution evaluation
In this study, the single factor pollution index method and the Nemero comprehensive pollution index method are used to evaluate the heavy metal pollution of farmland in this area. It can be seen from Table 6 that the average values of the single factor pollution index of the measured heavy metal elements are 0.21, 1.05, 0.52, 0.86, 0.68, 2.12, and 0.20, respectively. The value of Ni is greater than 1, indicating that there is slight pollution of Ni in the area, and the value of Cd is greater than 2, indicating that there is moderate pollution of Cd in the area. The floating dust formed by waste transportation and open stacking may be the main cause of soil heavy metal pollution. According to the Nemero comprehensive pollution index method principle, 1.0 < PN≤2.0 can be judged as mild pollution, while the survey results show that the average value is 1.61 and the maximum value is as high as 3.66. In terms of the total amount of heavy metal elements, the heavy metal pollution of farmland in this area is serious, and the main contributors to exceeding heavy metals are Cd and Cu. Li et al. (2015) conducted crop and soil investigation on the farmland in the eastern suburb of Kaifeng and found that the Cd content in the local farmland soil was very high, which was basically consistent with the conclusion of this paper. Heavy metals are potential long-term pollutants in soil, which are not easy to leach with water and cannot be decomposed by soil microorganisms but can be enriched by organisms, which often makes heavy metals accumulate gradually in the soil environment and difficult to remove transfer. Therefore, soil heavy metal pollution is more difficult and harmful than water environment heavy metal pollution. This study analyzes the content of heavy metals and Nemero comprehensive pollution index at 40 sampling points by spatial interpolation through the inverse distance weight method in ArcGIS. The results are shown in Figure 2. It can be seen that the content of Cd and Ni in farmland soil in this area is the highest, the pollution degree is the most serious, and the pollution distribution is the most extensive. This is due to the non-standard management of open-air waste dump for many years and the leakage of dust and leachate from the dump (Khademi et al., 2019). It can be seen that in addition to Cu and As, the spatial distribution characteristics of the content of most other heavy metals are high in the central area of the waste dump, and the content in the outward radiation area gradually decreases, indicating that the content distribution is indeed affected by the dust and leachate of the waste dump in the open air (Mutafela et al., 2020). The leachate from the waste dump will also cause heavy metal pollution to the surrounding farmland through surface runoff and underground runoff (Cossu et al., 2018). By comparison, it is found that the content, pollution degree, and pollution distribution of heavy metals in middle soil and surface soil are similar. Therefore, it shows light pollution above the middle soil under the dual action of continuous farmland tillage and open-air discharge of waste dump in this area. This phenomenon also explains the fact that there is long-term pollution in the waste dump. In addition, during the sampling survey, this study found that part of the farmland soil in the village came from the crushed waste residue retrieved by the villagers from the waste dump for crop fertilizer, which also caused the transfer of farmland soil near the center of the waste dump to a certain extent.
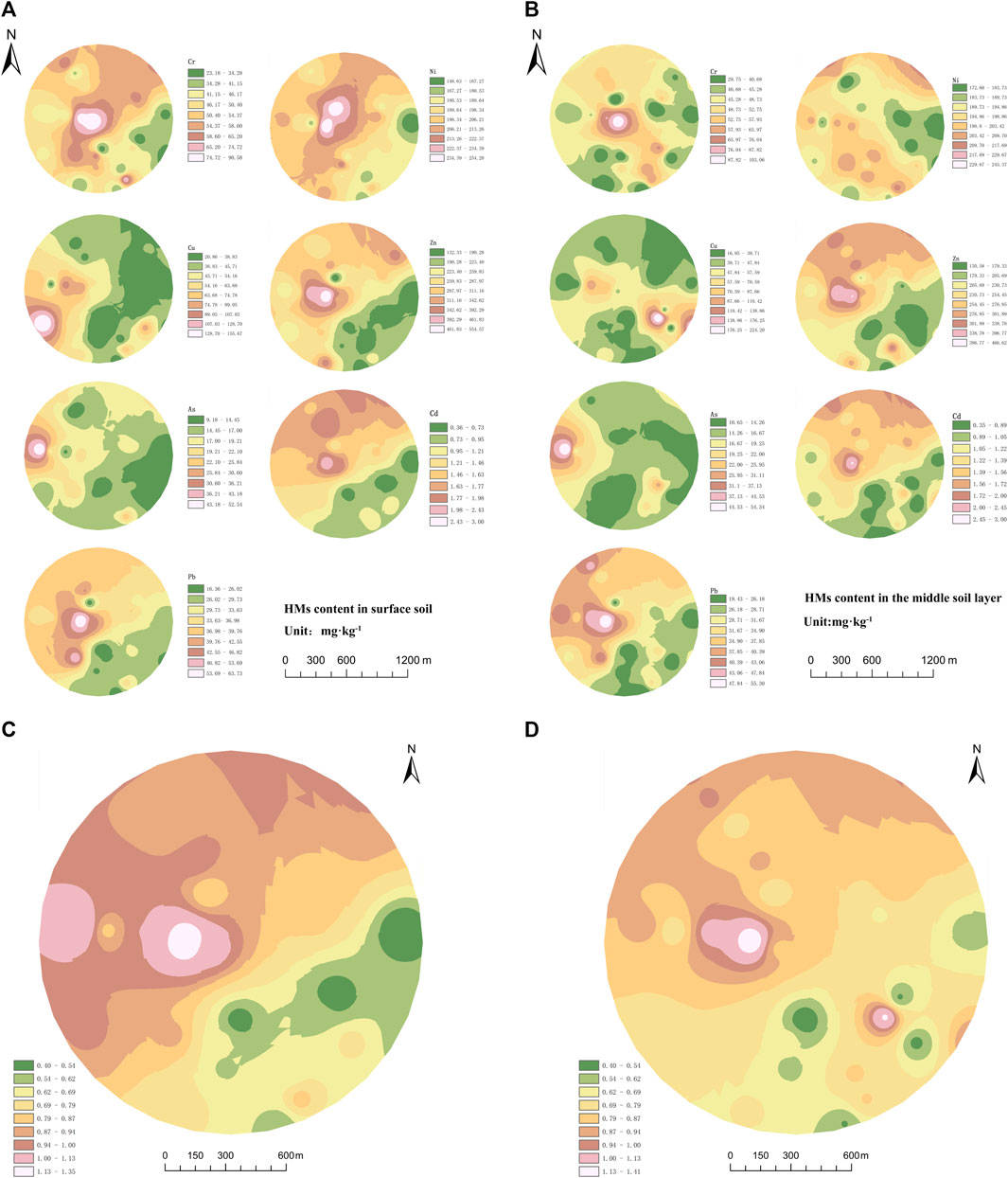
FIGURE 2. Spatial distribution of Single-factor pollution index and Nemero comprehensive pollution index. (A) Single-factor pollution index in surface soil; (B) Single-factor pollution index in the middle soil layer; (C) Nemero comprehensive pollution index in surface soil; (D) Nemero comprehensive pollution index in the middle soil layer. *Stars represent the waste dumps.
The pollution degree of the single factor pollution index and Nemero comprehensive pollution index of different elements in the total sample is shown in Figure 3. It can be seen that according to the evaluation of the single factor pollution index method, there are serious pollution phenomena in the total amount of Cd and Cu, including 62% mild pollution of Ni and 15% severe pollution of Cd. However, there is no pollution of Cr and Pb in the sampled farmland soil. As and Cu in farmland in most areas are pollution-free, and only 5% of farmland is slightly polluted. The distribution of Cd pollution is relatively complex. 8% of farmland is free of pollution, 38% of farmland is slightly polluted, 38% of farmland is moderately polluted, and 15% of farmland is heavily polluted. In addition, according to the Nemero comprehensive pollution index evaluation, 3% of the farmland soils sampled in this area are in a safe state, 8% are in a warning state, 56% are slightly polluted, and 21% are moderately polluted, and 3% are heavily polluted. Chen et al. (2020) analyzed the distribution characteristics of heavy metals in the soil around the fertilizer plant in the eastern suburb of Kaifeng and found that the contents of Cu, Pb, Zn, Cd, and other heavy metals in the soil around the fertilizer plant are significantly high, which has been seriously polluted by heavy metals. Ma et al. (2014) have concluded that there is no pollution of Ni, Zn, Cu, and Pb in the farmland soil far away from the industrial park in different functional areas of Kaifeng County. The overall environmental quality of the core area is excellent, and only Cd is good and average in some areas. Most areas in the core area of the comprehensive evaluation are pollution-free, and the soil’s environmental quality is excellent. This is because the farmland in the western suburb is far away from the industrial park and open-air landfill, which reduces the heavy metal pollution and potential ecological risk, which is consistent with the conclusion of this paper.
Potential ecological risk assessment of soil heavy metals
Considering the toxicity differences of different heavy metals, this study adopts Hakanson’s potential ecological risk index method to evaluate the ecological risk of heavy metal pollution in farmland soil in this area. The potential ecological risk index of different heavy metals is shown in Table 7. The average values of the single factor potential ecological risk indexes of the measured heavy metal elements are 0.42, 5.27, 2.61, 0.86, 6.79, 63.49, and 0.99, respectively. Among them, the potential ecological risk indexes of as, Pb, Cr, Cu, Ni, and Zn are all less than 40, and the values of Pb, Cr, Ni, and Zn are far less than 40, so the ecological risk is very low. However, the average value of Cd is greater than 80 but less than 160, and the maximum value is as high as 155, indicating that there is a high potential ecological risk of Cd in this area. In addition, the average value of the comprehensive potential ecological risk index is 80.43, less than 150. However, there are more than 150 sample points in the central area of the landfill, indicating that there is a medium potential ecological risk under the comprehensive consideration of the pollution of these seven heavy metals in this area.
The potential ecological risk index is analyzed by spatial interpolation through the inverse distance weight method in ArcGIS. The results are shown in Figures 4A,B. It can be clearly shown the potential ecological risk degree of different areas in the study area. The content of Cd in farmland soil in this area is high, and the Toxicity Coefficient of Cd is as high as 30. Therefore, the potential ecological risk caused by Cd is very serious, and other elements have a lower toxicity coefficient than Cd. Therefore, although heavy metal pollution exceeds the standard to varying degrees, the potential ecological risk is relatively low. Generally speaking, the relatively high potential ecological risks caused by different elements are mainly concentrated around the Tonglushan site, and the farther the distance is, the lower the potential ecological risk is. This also proves that the transportation of dust, leachate, and residue produced by open-air stacking waste is the main reason for the potential ecological risk to the surrounding soil. It can be seen from the comparison of Figures 4C,D that the potential health risk index of Mesocosms soil is lower than that of surface soil.
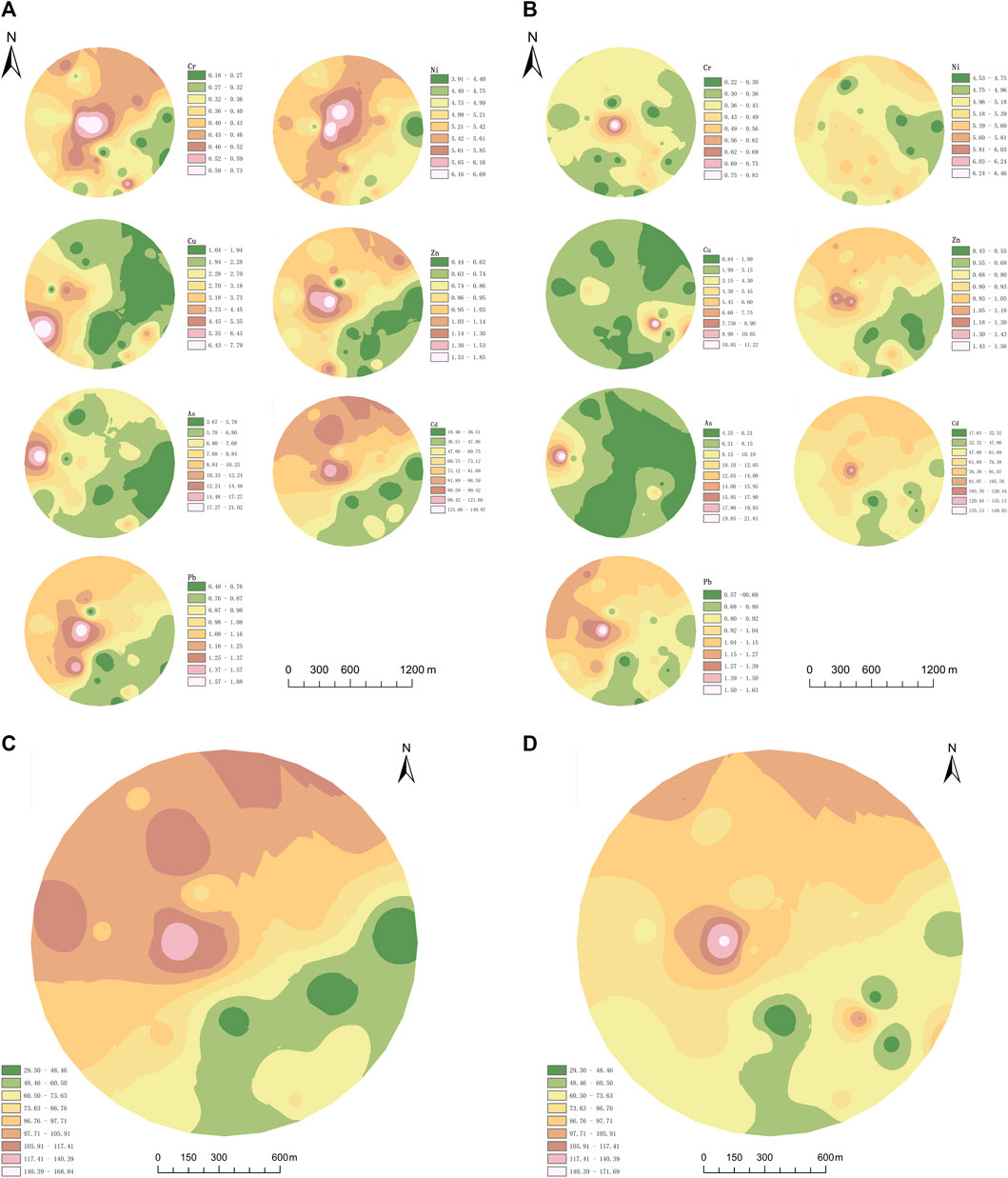
FIGURE 4. Spatial distribution of potential ecological risks of soil heavy metals. *Stars represent the waste dumps.
For the potential ecological risk caused by Cd, 30% of the sample soil is at low potential ecological risk, 35% of the sample soil is at medium potential ecological risk, 23% of the sample soil is at high potential ecological risk, 5% of the sample soil is at high potential ecological risk, and 7% of the sample soil is at ultra-high potential ecological risk, which is similar to the conclusion of “potential ecological risk of Cd in farmland soil around Daye Lake” studied by (Jiang et al., 2020). Cadmium is a non-essential element of the human body. It can be enriched in the body to form cadmium, this protein, which accumulates in the liver and kidney, resulting in cadmium poisoning. Cadmium is widely used in industrial production, which is easier to be absorbed by crops than other heavy metals and is easy to be discharged into the environment through waste gas, wastewater, and waste residue, resulting in pollution (Essien et al., 2019). Pollution sources are mainly mining and transportation of mineral resources, smelting and electroplating non-ferrous metals, and factories using cadmium compounds as raw materials or catalysts (Shao and Yang, 2020). Many industrial products in municipal solid waste need cadmium-containing raw materials or catalysts. Therefore, the dust and leachate of these wastes cause cadmium pollution in local soil in different ways. Another main reason may be closely related to the application of phosphorus fertilizer and pesticide, which have a long history, wide range, and large amount. Therefore, cadmium from phosphorus fertilizer and some pesticides in crops may exceed cadmium from other pollution sources (Shi et al., 2019). The potential ecological risk of other elements is low, and only Ni has a low proportion of high-risk sample soil. Considering the potential ecological risks, among the 80 sample varieties, 92% of the sample soil is at low risk, 8% of the sample soil is at medium risk, and there is no high-risk sample soil. Soledad et al. analyzed the content and distribution of Hg, Pb, Cd, Cr, as, Cu, and Zn in the surface sediment of Texcoco saline lake and found that the comprehensive potential ecological risk index in this area is 65.70–128.72, with an average value of 227.63, most of which belong to low risk, which is basically consistent with the conclusion of this study (Soledad et al., 2020).
Analysis on correlation and source of heavy metals in soil
Because the source, migration, and transformation of heavy metal elements in soil usually have a certain relationship, the correlation analysis can intuitively reflect the degree of correlation between various elements. It can be seen from Figure 5A that the total amount of heavy metals in the topsoil of Cr, Ni, Zn, Cd, and Pb in the study area has a significant positive correlation with each other at the level of 0.05, and the correlation coefficient is greater than 0.5; The total amount of heavy metals in Cd has a significant positive correlation with Zn at the level of 0.05. The total amount of heavy metals in Ni has a significant positive correlation with Cr at the level of 0.05. The total amount of heavy metals in Cu has a weak or no correlation with other elements. It can be seen from Figure 5B that the total amount of heavy metals in the middle soil of Cr, Ni, Zn, Cd and Pb in the study area has a significant positive correlation with each other at the level of 0.05, and the correlation coefficient is greater than 0.5; The total amount of heavy metals in Cd has a significant positive correlation with Zn at the level of 0.05, and the correlation coefficients are greater than 0.8; The total amount of heavy metals in Cu has weak or no correlation with other elements. According to the correlation analysis of the total amount of heavy metals in surface and middle soil, the correlation between Ni, Zn, and Cd is significant. There is a significant positive correlation between Zn and Cd elements, and their spatial distribution characteristics of potential ecological risk index are basically the same, but their correlation with other elements is weak or no correlation. Combined with the characteristics of high Cd and Pb elements in individual points in the central and western regions, the two sources are more in line with the law of local leakage of dust activities in the open-air stacking of waste.
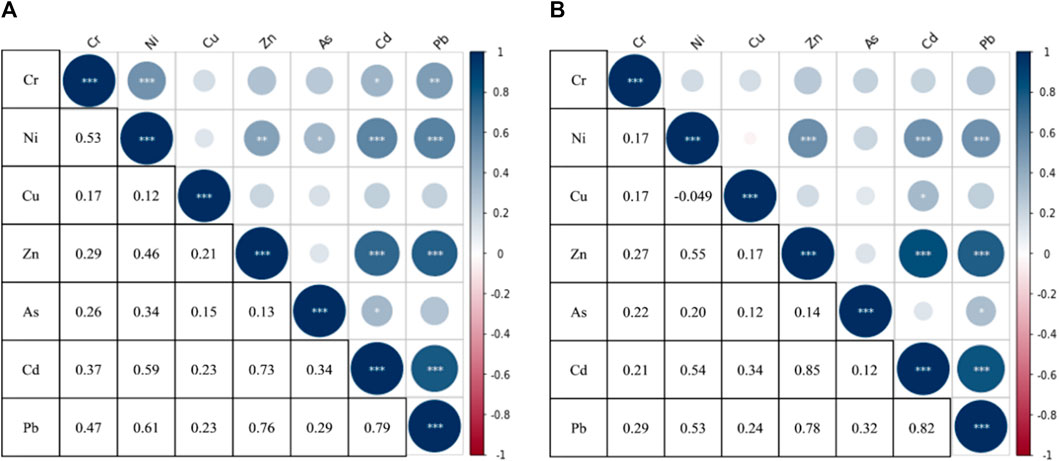
FIGURE 5. Pearson correlation matrix between total heavy metals. (A) Suface; (B) Mesocosms; *p ≤ 0.05, **p ≤ 0.01, and the size of the circle represents the absolute value of the correlation coefficient.
According to the above analysis, the correlation between Cr, Ni, Cu, Zn, As, Cd, and Pb elements is good, which is suitable for principal component analysis. KMO and Bartlett methods were used to test the total data of heavy metals Cr, Ni, Cu, Zn, As, CD, and Pb in soil. The results showed that KMO was 0.762 and 0.747, respectively, and the companion probability of the Bartlett sphericity test was 0.000, which met the data requirements of principal component analysis. The results of principal component analysis show that: Two factors with large eigenvalues are extracted from the upper soil, accounting for 66.67% of the total variance of the explanatory variable. Three factors with large eigenvalues are extracted from the middle soil, accounting for 77.68% of the total variance of the explanatory variable. Factor 1 and factor 2 can reflect most of the information from the original data. The elements with a high first-factor load of total heavy metals are Cr, Cu, Zn, as, Cd, and Pb, and the variance contribution rate can reach 81.8%, indicating that Cr, Cu, Zn, as, Cd, and Pb have homology (Ma et al., 2018). The pollution of heavy metals in the soil near a municipal waste dump in northern China was studied. The results showed that the high contents of heavy metals were mainly as, Cd, Pb, Zn and Cu, and their sources were mainly floating dust and leachate caused by the open stacking of municipal waste in the region. Combined with the statistical analysis and spatial distribution law of heavy metals, it can be seen that the main sources of Cr, Cu, Zn, as, Cd and Pb are waste dust and leachate input caused by the non-standard management of waste dumps in the eastern suburbs. The elements with the higher load of the second factor are Ni and Cr, and the contribution rate of variance is 14.8%. Combined with the previous research on ecological risk and spatial distribution, the ecological risk of Ni and Cr is low, the coefficient of variation is small, and Cr is usually in the residual form (Wang et al., 2019; Jiang T. et al., 2020). Therefore, the main source of the second factor is more in line with the law of natural input. This result is consistent with the research conclusion of Sun et al. (2018) and María et al. (2011). that is, Ni and Cr are more derived from the parent material and background of soil formation and belong to natural source metals.
Conclusions
1) The single factor pollution index of each element is between 0.01 and 5.58, the single factor indexes of Cd and as are 1.50 and 2.13, respectively, and the evaluation results are light pollution and light pollution, respectively. The single factor index of Pb exceeds 5, which belongs to heavy pollution. The enrichment factors of As, Hg, and Zn are 3.73, 10.4, and 22.4, which belong to moderate pollution, heavy pollution, and serious pollution, respectively. The enrichment factor of Cd and Pb exceeds 40, which belongs to extremely heavy pollution and is obviously polluted by artificial pollution.
2) The content of heavy metals in the soil measured in the study area is higher than the background value of the soil in Kaifeng City, and the variation range is large. The heavy metal pollution problem is significant. Cd has the strongest coefficient of variation, which is strongly affected by human activities. Among the detected heavy metal elements, Cd and Ni have serious pollution phenomena, but due to the high toxicity of Cd, the heavy metal pollution and potential ecological risks caused by Cd are the most serious. The spatial distribution characteristics of the content of most heavy metals and the degree of potential ecological risk in the area are as follows: the central area of the mining area is high, and the outward radiation area gradually decreases, indicating that the floating dust and leachate caused by the open-air stacking of urban waste in the area are the main causes of heavy metal pollution and potential ecological risk. In addition, the villagers took the soil from the waste dump as farmland soil, which also caused the transfer of heavy metal pollution to a certain extent.
3) The results of correlation and principal component analysis of total and available heavy metals in soil showed that the correlation between Cu, Zn, as, Cd, and Pb was significant, and the main source was the input of industrial production activities; There is a significant positive correlation between Cd and Zn, but there is no correlation with other elements; The main source of Ni is more in line with the law of natural input.
4) In the next study, different spatial interpolation methods of soil heavy metals [such as ordinary Kriging (OK), inverse distance weight method (IDW), and radial basis function method (RBF)] can be used to improve the reliability of the analysis results. In addition, increasing the scope of the study area and sampling density will also help to improve the analysis accuracy further.
Data availability statement
The original contributions presented in the study are included in the article/Supplementary Material, further inquiries can be directed to the corresponding authors.
Author contributions
Conceptualization, ZW and MX. Investigation and methodology, ZW. Formal analysis, ZW. Resources, MX and WL. Supervision, MX and WL. Writing—original draft, LW. Writing—review and editing, MZ. All authors have read and agreed to the published version of the manuscript.
Conflict of interest
The authors declare that the research was conducted in the absence of any commercial or financial relationships that could be construed as a potential conflict of interest.
Publisher’s note
All claims expressed in this article are solely those of the authors and do not necessarily represent those of their affiliated organizations, or those of the publisher, the editors and the reviewers. Any product that may be evaluated in this article, or claim that may be made by its manufacturer, is not guaranteed or endorsed by the publisher.
Supplementary material
The Supplementary Material for this article can be found online at: https://www.frontiersin.org/articles/10.3389/fenvs.2022.946298/full#supplementary-material
References
Alam, P., Sharholy, M., and Ahmad, K. (2020). “A study on the landfill leachate and its impact on groundwater quality of Ghazipur area, New Delhi, India,” in Recent developments in waste management. Editor A. Kalamdhad (Singapore: Springer), 345–358. doi:10.1007/978-981-15-0990-2_27
Bhattacharya, B. D., Nayak, D. C., Sarkar, S. K., Biswas, S. N., Rakshit, D., and Ahmed, M. K. (2015). Distribution of dissolved trace metals in coastal regions of Indian sundarban mangrove wetland: a multivariate approach. J. Clean. Prod. 96, 233–243. doi:10.1016/j.jclepro.2014.04.030
Biswas, T. D., and Mukherjee, S. K. (2008). Textbook of soil science. Second ed. New Delhi, India: Tata McGraw-Hill publishing company.
Chen, Z. F., Hua, Y. X., Wei, Z. D., and Pei, J. C. (2020). Analysis of heavy metal pollution sources in peri-urban farmland based on positive definite matrix factor Analysis model. Acta Sci. Circumstantiae) 40 (01), 276–283. doi:10.13671/j.hjkxxb.2019.0380
Cossu, R., Zuffianò, L. E., Limoni, P. P., Giorgio, G. D., Pizzardini, P., Miano, T., et al. (2018). How can the role of leachate on nitrate concentration and groundwater quality be clarified? An approach for landfills in operation (Southern Italy). Waste Manag. 77, 156–165. doi:10.1016/j.wasman.2018.05.014
Egbueri, J. C., Ukah, B. U., Ubido, O. E., and Unigwe, C. O. (2020). A chemometric approach to source apportionment, ecological and health risk assessment of heavy metals in industrial soils from southwestern Nigeria. Int. J. Environ. Anal. Chem. 98, 1–19. doi:10.1080/03067319.2020.1769615
Essien, J. P., Inam, E. D., Ikpe, D. I., Udofia, G. E., and Benson, N. U. (2019). Ecotoxicological status and risk assessment of heavy metals in municipal solid wastes dumpsite impacted soil in Nigeria. Environ. Nanotechnol. Monit. Manag. 11 (100), 100215. doi:10.1016/j.enmm.2019.100215
Gao, M., Lin, Y., Shi, G. Z., Li, H. H., Yang, Z. B., Xu, X. X., et al. (2019). Bioaccumulation and health risk assessments of trace elements inhousefly (Musca domestica L.) larvae fed with food wastes. Sci. Total Environ. 682, 485–493. doi:10.1016/j.scitotenv.2019.05.182
Hakanson, L. (1980). An ecological risk index for aquatic pollution control.a sedimentological approach. Water Res. 14, 975–1001. doi:10.1016/00431354(80)90143-8
Hogland, W. (2002). Remediation of an old landsfill site: soil analysis, leachate quality and gas production. Environ. Sci. Pollut. Res. Int. 1, 49–54. doi:10.1007/bf02987426
Huang, Y., Wang, Y., Sun, Y., Kai, Y., and Chen, L. (2019). Microbial community structure and population distribution characteristics in short-term landfill-age garbage dumps. J. Environ. Sci. (Beijing, China) 39 (12), 4122–4131. doi:10.13671/j.hjkxxb.2019.0318
IARC (2018). Chromium (IV) compounds. IARC Monograph 100C. Available at: https://monographs.iarc.fr/wp-content/uploads/2018/06/mono100C-9.pdf (Accessed January 24, 2022).
Jiang, H., Cai, L., Wen, H., Hu, G., Chen, L., and Luo, J. (2020). An integrated approach to quantifying ecological and human health risks from different sources of soil heavy metals. Sci. Total Environ. 701, 134466. doi:10.1016/j.scitotenv.2019.134466
Jiang, T., Lin, W. W., Cao, Y. J., Li, K., Xuan, Y. X., Li, R., et al. (2020). Pollution and ecological risk assessment and source apportionment of heavy metals in sediments of Qingliangshan Reservoir in the Meijiang Basin. Chin. J. Environ. Sci. 41 (12), 5410–5418. doi:10.13227/j.hjkx.202003018
Jiang, Y. L., Ruan, X. L., and Ma, J. H. (2020). Characteristics and classification management of heavy metal pollution in polluted farmland near a battery factory in Xinxiang City. Acta Sci. Circumstantiae. 40 (02), 645–654. doi:10.13671/j.hjkxxb.2019.0343
Khademi, H., Gabarrón, M., Abbaspour, A., Martínez-Martínez, S., Faz, A., and Acosta, J. A. (2019). Environmental impact assessment of industrial activities on heavy metals distribution in street dust and soil. Chemosphere 217, 695–705. doi:10.1016/j.chemosphere.2018.11.045
Khanam, R., Kumar, A., Nayak, A., Shahid, M., Tripathi, R., Vijayakumar, S., et al. (2019). Metal(loid)s (As, Hg, Se, Pb and Cd) in paddy soil: bioavailability and potential risk to human health. Sci. Total Environ. 699, 134330. doi:10.1016/j.scitotenv.2019.134330
Li, Y. M., Ma, J. H., Liu, D. X., Sun, Y. L., and Chen, Y. F. (2015). Assessment of heavy metal pollution and potential ecological risks in soil in Kaifeng City. Chin. J. Environ. Sci. 36 (03), 1037–1044. doi:10.13227/j.hjkx.2015.03.037
Li, Y. X., Huang, Y., Wang, N., Yang, J., Xu, M. M., Sun, B., et al. (2014). Characteristics of heavy metal pollution and health risk assessment of domestic waste dump near the outskirts of a city in Shandong Province. Environ. Chem. (Beijing, China) 33 (09), 1476–1483. doi:10.7524/j.issn.0254-6108.014.09.009
Ma, J. H., Ma, S. Y., and Chen, Y. Z. (2014). Migration and accumulation of heavy metals in soil-crop-human hair system in a polluted irrigation area of Henan Province. Acta Sci. Circumstantiae. 34 (06), 1517–1526. doi:10.13671/j.hjkxxb.2014.0216
Ma, W., Tai, L., Qiao, Z., Zhong, L., Wang, Z., Fu, K., et al. (2018). Contamination source apportionment and health risk assessment of heavy metals in soil around municipal solid waste incinerator: a case study in North China. Sci. Total Environ. 631, 348–357. doi:10.1016/j.scitotenv.2018.03.011
María, T. R., Ofelia, M., Elizabeth, H., Lozano, R., and Tapia-Cruz, V. (2011). The study of metal contamination in urban topsoils of Mexico City using GIS. Environ. Earth Sci. 62 (5), 899–905. doi:10.1007/s12665-010-0584-5
Men, C., Liu, R., Xu, F., Wang, Q., Guo, L., and Shen, Z. (2018). Pollution characteristics, risk assessment, and source apportionment of heavy metals in road dust in Beijing, China. Sci. Total Environ. 612, 138–147. doi:10.1016/j.scitotenv.2017.08.123
Monged, M. H. E., Hassan, H. B., and El-Sayed, S. A. (2020). Spatial distribution and ecological risk assessment of natural radionuclides and trace elements in agricultural soil of northeastern nile valley, Egypt. Water Air Soil Pollut. 231, 338. doi:10.1007/s11270-020-04678-9
Moreira, L. J., da Silva, E. B., Fontes, M. P., Liu, X., and Ma, L. (2018). Speciation, bio-accessibility and potential risk of chromium in Amazon forest soils. Environ. Pollut. 239, 384–391. doi:10.1016/j.envpol.2018.04.025
Mutafela, R. N., Mantero, J., Jani, Y., Thomas, R., Holm, E., and Hogland, W. (2020). Radiometrical and physico-chemical characterisation of contaminated glass waste from a glass dump in Sweden. Chemosphere 241, 124964. doi:10.1016/j.chemosphere.2019.124964
Shao, L., and Yang, X. (2020). Analysis of water pollution sources in copper smelting industry. Chin. J. Min. Mag. 29 (S1), 551–553. doi:10.12075/j.issn.1004-4051.2020.S1.04
Shi, T., Zhang, Y., Gong, Y., Ma, J., Wei, H., Wu, X., et al. (2019). Status of cadmium accumulation in agricultural soils across China (1975–2016): from temporal and spatial variations to risk assessment. Chemosphere 230, 136–143. doi:10.1016/j.chemosphere.2019.04.208
Soledad, M. S., Meza-Olvera, E., Shruti, V. C., and Sedeno-Diaz, J. E. (2020). Assessment of metal contamination and their ecological risks in wetland sediments of the former Texcoco saline lake, Mexico. J. Soils Sediments 20, 2912–2930. doi:10.1007/s11368-020-02613-3
Sun, H., Bi, R. T., Guo, Y., Cai, M., and Guo, Z. X. (2018). Source apportionment analysis of trace metal contamination in soils of Guangdong province, China. Acta Sci. Circumstantiae. 38 (2), 704–714. doi:10.13671/j.hjkxxb.2017.0351
Tong, Z. Q., Gu, L., Duan, H. J., and Ma, J. H. (2012). Spatial distribution of heavy metals in roadside soils based on kriging interpolation: a case study of zhengzhou-kaifeng section along the 310th national highway. Acta Sci. Circumstantiae. 32 (12), 3030–3038. doi:10.13671/j.hjkxxb.2012.12.020
Tseng, C. H., Lee, I. H., and Chen, Y. (2019). Evaluation of hexavalent chromium concentration in water and its health risk with a system dynamics model. Sci. Total Environ. 669, 103–111. doi:10.1016/j.scitotenv.2019.03.103
Wakeel, A., Xu, M., and Gan, Y. (2020). Chromium-induced reactive oxygen species accumulation by altering the enzymatic antioxidant system and associated cytotoxic, genotoxic, ultrastructural, and photosynthetic changes in plants. Int. J. Mol. Sci. 21, 728. doi:10.3390/ijms21030728
Wan, S. M., Xi, B. D., Li, M. X., and Xia, X. F. (2012). Effects of long-term accumulation of rural domestic waste on soil nitrification rate and respiration rate. J. Northeast Agric. Univ. Chin. Ed. . 43 (11), 67–71. doi:10.19720/j.cnki.issn.1005-9369.2012.11.014
Wang, Y. Y., Li, F. F., Wang, X. Y., Yang, Z. H., Han, K., and Ruan, X. L. (2019). Spatial distribution and risk assessment of heavy metal contamination in surface farmland soil around a lead and zinc smelter. J. Environ. Sci. (Beijing, China) 40 (1), 437–444. doi:10.13227/j.hjkx.201803031
Xiao, Y. T., Zhang, R. X., and Wu, P. (2019). Review on common spatial interpolation methods for soil heavy metal analysis. Environ. Sci. Technol. (Wuhan, China) 42 (3), 198–205. doi:10.19672/j.cnki.1003-6504.2019.03.028
Yahya, J., Burlakovs, J., Augustsson, A., Marcia, M., and Hogland, W. (2019). Physicochemical and toxicological characterization of hazardous wastes from an old glasswork dump at southeastern part of Sweden. Chemosphere 237, 124568. doi:10.1016/j.chemosphere.2019.124568
Yari, A. A., Varvani, J., and Zare, R. (2021). Assessment and zoning of environmental hazard of heavy metals using the Nemerow integrated pollution index in the vineyards of Malayer city. Acta Geophys. 69 (1), 149–159. doi:10.1007/s11600-020-00514-0
Zeng, D., Meng, D., and Zhou, W. (2021). Comprehensive analysis and evaluation of environmental impact of landfill in Bangor County, Tibet. J. Environ. Eng. Technol. (Beijing, China) 11 (1), 202–208. doi:10.12153/j.issn.1674-991X.20200062
Keywords: waste dump, agricultural soils, heavy metals, pollution investigation, ecological risk
Citation: Wang L, Zeraatpisheh M, Wei Z and Xu M (2022) Heavy metal pollution and risk assessment of farmland soil around abandoned domestic waste dump in Kaifeng City. Front. Environ. Sci. 10:946298. doi: 10.3389/fenvs.2022.946298
Received: 17 May 2022; Accepted: 29 July 2022;
Published: 19 August 2022.
Edited by:
Chunhao Gu, University of Delaware, United StatesReviewed by:
Juris Burlakovs, University of Latvia, LatviaŁukasz Jurczyk, University of Rzeszow, Poland
Copyright © 2022 Wang, Zeraatpisheh, Wei and Xu. This is an open-access article distributed under the terms of the Creative Commons Attribution License (CC BY). The use, distribution or reproduction in other forums is permitted, provided the original author(s) and the copyright owner(s) are credited and that the original publication in this journal is cited, in accordance with accepted academic practice. No use, distribution or reproduction is permitted which does not comply with these terms.
*Correspondence: Zhangdong Wei, 10380012@vip.henu.edu.cn; Ming Xu, mingxu@henu.edu.cn