- 1Plateau Atmosphere and Environment Key Laboratory of Sichuan Province, College of Atmospheric Science, Chengdu University of Information Technology, Chengdu, China
- 2Institute of Plateau Meteorology, China Meteorological Administration, Chengdu, China
- 3Sichuan Lightning Protection Center, Chengdu, China
- 4Guangzhou Institute of Tropical and Marine Meteorology, China Meteorological Administration, Guangzhou, China
This study discussed the influence of aerosols on the relative frequency of positive cloud-to-ground (CG) lightning and its dependence on thermodynamic and cloud-related factors in Sichuan during the warm season from 2005 to 2017. The relative frequency of positive CG lightning is defined as the proportion of positive CG lightning flashes to total CG lightning flashes. Although the total CG lightning density in the Western Sichuan Plateau is significantly lower than that in the Sichuan Basin, the relative frequency of positive CG lightning is higher than that in the basin. Convective available potential energy (CAPE) and vertical wind shear in the low-to-mid level of the troposphere (SHEAR-5 km) are the controlling factors of positive CG lightning frequency. A small CAPE and a large SHEAR-5 km represent weak convection, which is more conducive to the generation of positive CG lightning. The upper main positive charge region in a thriving thunderstorm is higher from the ground, which is not conducive to the transport of positive charge to the ground, so it is not conducive to the generation of positive CG lightning. In the basin, the relationship between aerosols and positive lightning is not significant, which may be due to the strong total CG lightning and the low proportion of positive CG lightning. In the plateau, both sulfate aerosol and black carbon (BC) aerosol have a significant inhibition effect on the positive CG lightning relative frequency. Sulfate aerosol stimulates the ice-phase process through a microphysical effect and promotes the development of convection. The distribution of the main positive charge center is higher, which is not conducive to the transport of positive charge to the ground and the generation of positive CG lightning. The significant heating effect of BC aerosol on the lower troposphere makes the convective development more vigorous and is not conducive to the occurrence of positive CG lightning.
Introduction
Observational evidence and numerical simulation studies indicated that aerosol has an outstanding effect on lightning activity. One view holds that aerosols could stimulate lightning activity through a microphysical effect (Wang et al., 2011; Mansell and Ziegler, 2013; Yang and Li, 2014; Liu et al., 2020), more aerosols serve as cloud condensation nuclei to reduce the radius of cloud droplets and then reduce the collision–coalescence efficiency, thus inhibiting the warm rain process. The small cloud droplets are transported above the freezing level to participate in the ice-phase microphysical processes, forming more ice particles and releasing a large amount of latent heat to further stimulate convection. Stronger convection and more ice particles are involved in the non-inductive electrification process, making lightning more vigorous. Another view suggests that aerosols inhibit lightning activity through a radiative effect (Yang et al., 2013; Tan et al., 2016; Altaratz et al., 2017), the scattered aerosols reduce the solar radiation reaching the ground, which weakens the thermal conditions in the lower troposphere, and the relatively stable atmospheric conditions are not conducive to the development of convection, thus inhibiting lightning activity. The radiative forcing effect of BC aerosol affects the structure of atmospheric thermal stability and convective development, which is closely related to its vertical distribution and heating rate (Lu et al., 2020). Sulfate aerosols have different effects on lightning activity through the microphysical effect and radiation effect. Sulfate aerosols could stimulate lightning activity through microphysical effect, and could inhibit lightning activity through radiation effect (Yang et al., 2013; Yang and Li, 2014; Zhao et al., 2020).
There were also two different views on the effect of aerosols on positive CG lightning. On the one hand, previous studies suggested that anthropogenic aerosols in the urban increase the percentage of positive CG lightning (Steiger et al., 2002; Naccarato et al., 2003). According to their urban aerosol hypothesis, urban aerosols influence the polarity of lightning by influencing the charge separation process in thunderstorms. Atmospheric pollutants in super cooled cloud droplets would cause graupel particles to be charged negatively in a warmer temperature region, and a wider negative charge region is conducive to the generation of negative CG lightning, thus reducing the percentage of positive CG lightning. On the other hand, the effect of smoke aerosol from fires on the percentage of positive CG lightning is opposite to that of urban aerosols (Lyons, 1998; Murray et al., 2000; Lang and Rutledge, 2006). The smoke aerosol hypothesis considers that smoke aerosol would narrow the droplet spectrum, and the narrow droplet spectrum leads to the graupel particles charging positively between −10°C and −20°C in the thunderstorm, which would lead to a wider distribution of lower positive charge region, which is conducive to the generation of positive CG lightning.
Previous studies (Eddy, 2018; MacGorman et al., 2018) have suggested that thermodynamic and moisture factors, such as thermal stability, vertical wind shear, and moisture in the low-to the mid-level troposphere, are crucial to the generation of positive CG lightning. Weaker thermal instability conditions mean weaker thunderclouds, while the distribution of the main positive charge region is relatively low, which is conducive to the triggering of positive CG lightning from there (Orville and Huffines, 1999; Rakov, 2003; Wang et al., 2018). Stronger vertical wind shear causes the cloud body to tilt, so that positively charged ice particles in the upper part of the cloud are repositioned and are no longer blocked by the negative charged region below, making it easier to transport positive charge to the ground and generate positive CG lightning (Brook et al., 1982; Zhao et al., 2021). Dry low-to mid-level troposphere also has an excitation effect on positive CG lightning (Carey and Buffalo, 2007).
The west of Sichuan is the West Sichuan Plateau, and the east is the Sichuan Basin. On the one hand, the special terrain and thermal dynamic conditions lead to the obvious spatial difference in lightning activity in this region (Xia et al., 2015; Zhao et al., 2022b). On the other hand, the topographic conditions unfavorable to the diffusion of atmospheric pollutants and the prominent anthropogenic emissions result in the aerosol concentration in the basin area being significantly higher than that in the plateau area (Ning et al., 2018). The influence of aerosols on CG lightning density in Sichuan has obvious spatial differences (Zhao et al., 2020), so, what is the relationship between aerosols and positive CG lightning activity? In this study, we focus on this interesting issue, analyzing the possible influence of black carbon aerosols and sulfate aerosols on the relative frequency of positive CG lightning and the role of environmental conditions in this effect.
Data and Methodology
CG Lightning
The CG lightning data were obtained from the Sichuan Lightning Detection Network (SCLDN) of the Sichuan Province Lightning protection center, including the flash number of CG lightning, CG lightning polarity, and peak current. SCLDN consists of 25 Advanced Time of Arrival and Direction (ADTD) ground-based sensors with an average detection efficiency of more than 90% [CMA (China Meteorological Administration), 2009; Yang et al., 2015]. The CG lightning data employed in this study was processed with quality control, which was based on previous studies (Cummins et al., 1998; Yang et al., 2015; Zhao et al., 2020; Zhao et al., 2021). Lightning data during the warm season (May to October) from 2005 to 2017 were applied. To match environmental factors data, lightning data were processed to a temporal of 1 month and spatial resolution of 0.25 × 0.25. The positive CG lightning relative frequency is defined as the percentage of positive CG lightning flashes in total CG lightning flashes.
where PRF is the relative frequency of positive CG lightning, Npositive is the positive CG lightning flashes, Ntotal is the total CG lightning flashes.
Aerosol Optical Depth
AODs data sets were derived from MERRA-2 (Modern-Era Retrospective analysis for Research and Applications, version 2) and include the optical thickness of various aerosols, such as total aerosol, sulfate, black carbon (BC), organic carbon, sea salt, and dust (Buchard et al., 2017; Randles et al., 2017). Our previous study (Zhao et al., 2020) compared AOD products of MISR (Multi-angle Imaging SpectroRadiometer) with AOD products of MERRA-2 over Sichuan, showing good consistency. Sulfate AOD and BC AOD with a spatial resolution of 0.25 × 0.25 interpolated from the original spatial resolution of 0.5 × 0.625 were selected in this study to discuss the relationship between aerosol and positive CG lightning.
Thermodynamic and Cloud-Related Factors
Convective available potential energy (CAPE), vertical wind shear in the low-to middle troposphere (SHEAR-5 km), liquid water path (LWP), and ice water path (IWP) with a spatial resolution of 0.25 × 0.25 from ERA5 were selected as thermodynamic and cloud-related factors. These environmental factors are more representative to control positive CG lightning (Zhao et al., 2021; Zhao et al., 2022a). SHEAR-5 km is defined as vertical wind shear from the ground to 5 km level above the ground, the specific calculation method referred to in our previous studies (Zhao et al., 2020; Zhao et al., 2021).
where u5km, u5km, v0km, and v0km are zonal and meridional wind speeds interpolated to 5 km level above ground and the ground level from isobaric layers, respectively.
Results and Discussions
Positive CG Lightning and Aerosols in Sichuan
Due to the special terrain difference, the thermal dynamic conditions of the western Plateau and the eastern basin of Sichuan are different, which leads to the obvious difference in lightning activity characteristics in the two regions. Figure 1 illustrates the spatial distribution characteristics of total CG lightning density, positive CG lightning density, the peak current of positive CG lightning, and the relative frequency of positive CG lightning in Sichuan during the warm season. Influenced by the high topography of the Qinghai-Tibet Plateau, the Sichuan Basin has become a region with a high concurrence of cyclonic circulation (such as the southwest vortex and plateau vortex), the abundant thermal and water vapor conditions make the convective systems and heavy precipitation in this region very vigorous (Yu et al., 2007; Zhang et al., 2014). As can be seen in Figure 1A, the Sichuan Basin is an area with exuberant lightning activity, and the total CG lightning density is significantly higher than that of the Western Sichuan Plateau, the maximum lightning density is more than 5 flashes km−2 year−1 in the southeastern part of the Basin, the southern part of Sichuan is an area with secondary lightning activity, and the maximum lightning density in the Western Sichuan Plateau is about 1 flash km−2 year−1. As a whole, in the Sichuan region, the positive CG lightning density is about one-tenth of the total CG lightning density. The difference in positive CG lightning density between the basin region and plateau region is not as obvious as the difference in total CG lightning density (Figure 1B). The maximum positive CG lightning density is approximately 0.4 flashes km−2year−1 in the central part of the basin, and the maximum positive CG lightning density is approximately 0.1–0.2 flashes km−2 year−1 in the plateau.
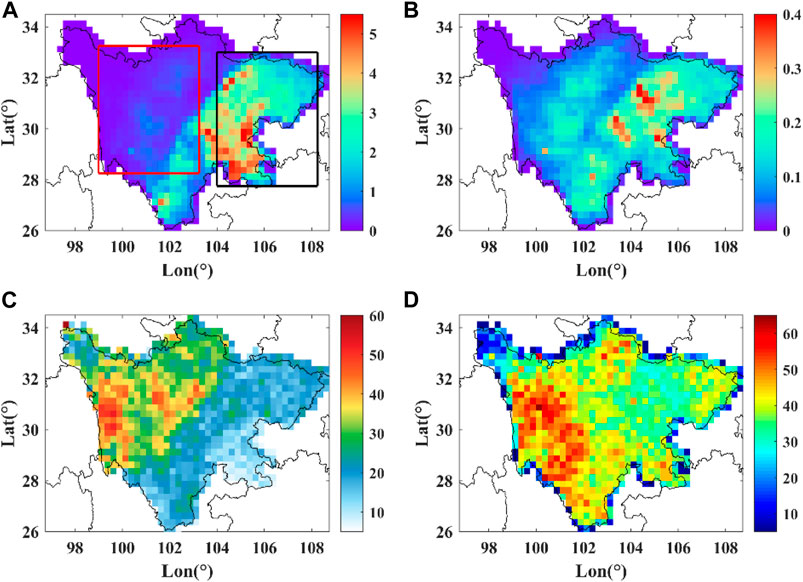
FIGURE 1. Spatial distribution of (A) total CG lightning density (in flashes km−2 year−1), (B) positive CG lightning density (in flashes km−2 year−1), (C) positive CG lightning peak current (in kA), and (D) positive CG lightning relative frequency (in %) in Sichuan during the warm season. The red rectangle represents the plateau region, and the black rectangle represents the basin region.
Although the occurrence probability of positive CG lightning is much lower than that of negative CG lightning, it has become the focus of many studies because of the large current generated by it (Nag and Rakov, 2012). Although the positive CG lightning activity in the plateau region is not as strong as that in the basin region, the positive CG lightning producing a large peak current is concentrated in the plateau region (Figure 1C). In the main plateau region, the peak current of positive CG lightning is over 30 kA, and the maximum is about 60 kA, while in the basin region, the peak current of positive lightning is below 25 kA. The relative frequency of positive CG lightning in the plateau region is also significantly higher than that in the basin region (Figure 1D). The relative frequency of positive CG lightning in the main plateau region is more than 40%, and the maximum value is approximately 65%, while the relative frequency of positive CG lightning in the main basin region is approximately 30%.
Although the positive CG lightning density in the plateau is lower than that in the basin region, the peak current and relative frequency are higher than that in the basin region. Aerosols have an outstanding influence on the total CG lightning density in Sichuan (Zhao et al., 2020). Is there a potential connection between aerosols and positive lightning activities? To probe this interesting issue, we discuss the relationship between the relative frequency of positive CG lightning and aerosols in the following sections.
Sichuan Basin is one of the most densely populated areas in Southwest China, which is one of the most prominent air pollution areas in China due to many anthropogenic emissions and special terrain that is not conducive to the diffusion of air pollutants (Zhang et al., 2012; Ning et al., 2018). Figure 2 shows the sulfate AOD, BC AOD, sulfate AOD ratio in total AOD, and BC AOD ratio in total AOD in Sichuan during the warm season. The aerosol loading in the basin region is significantly higher than that in the Plateau region (Figures 2A,B), and the sulfate AOD and BC AOD in the basin region are over 0.4 and 0.04, respectively, while the sulfate AOD and BC AOD in the Plateau area are approximately 0.1 and 0.01, respectively. The sulfate ratio in the basin is larger than 70%, while in the main part of the plateau is approximately 40%–65%. The difference in BC AOD between basin and plateau is not obvious, and the ratio is approximately 8%. Although the ratio of BC aerosols in total aerosols is not prominent, the influence of BC aerosols on atmospheric thermal structure through heating cannot be ignored (Jacobson, 2001; Nenes et al., 2002), so the influence of sulfate aerosols and BC aerosols on positive lightning is considered simultaneously in our study.
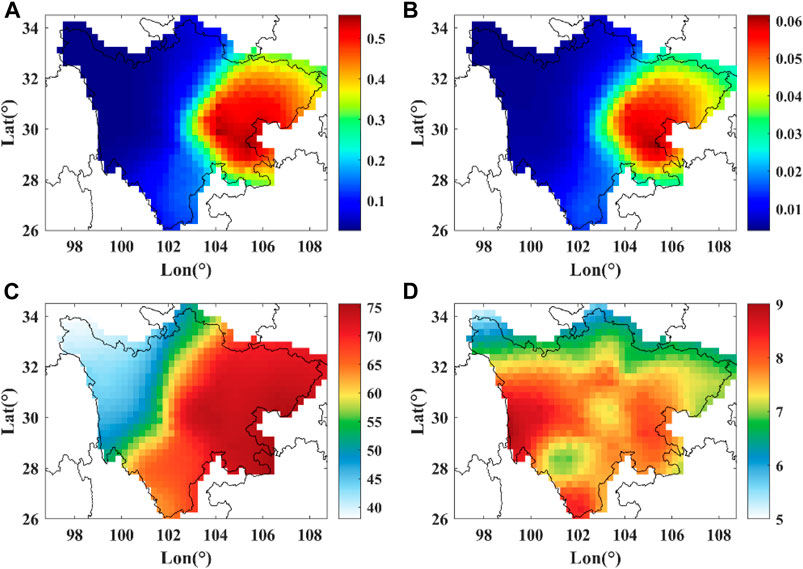
FIGURE 2. Spatial distribution of (A) sulfate AOD, (B) BC AOD, (C) sulfate AOD ratio (in %), and (D) BC AOD ratio (in %) in Sichuan during warm season.
Figure 3 shows the annual variation of total CG lightning density, the relative frequency of CG positive lightning (PRF), sulfate AOD and BC in the basin and plateau during the warm season from 2005 to 2017. From 2005 to 2010, the total CG lightning density in the basin and plateau showed an obvious upward trend and fluctuated after that. The relative frequency of CG positive lightning showed a significant upward trend from 2006 to 2010 and remained at a high value after that. The annual change trend of sulfate AOD increased firstly and then decreased, it increased significantly from 2005 to 2010, and then decreased significantly. In both plateau and basin, BC AOD showed a monotonically decreasing annual trend, and the decreasing range was relatively prominent.
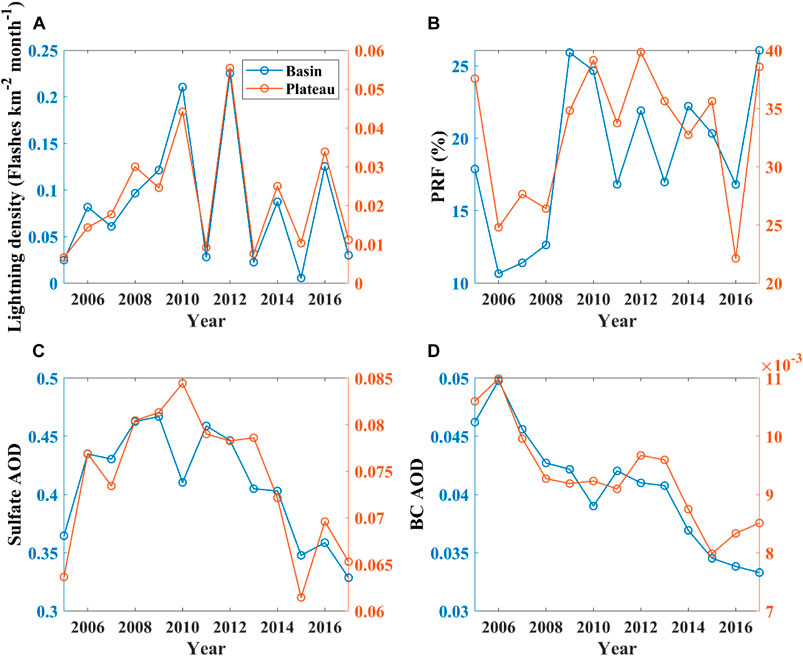
FIGURE 3. Annual variations of (A) total CG lightning density, (B) relative frequency of positive CG lightning (PRF), (C) sulfate AOD, and (D) BC AOD in the basin and plateau regions during the warm season.
Relationship Between Aerosols and Positive CG Lightning
Figure 4 shows the Pearson correlation coefficients between sulfate aerosol and positive CG lightning relative frequency and between BC aerosol and positive CG lightning relative frequency in Sichuan. As shown in Figure 4A, the correlation between sulfate aerosol and positive CG lightning is spatially different in Sichuan, in the Western Sichuan Plateau region, sulfate aerosol shows a significant negative correlation with positive CG lightning relative frequency suggesting that increases in sulfate aerosol loading would reduce the proportion of positive CG lightning in total CG lightning, and in the basin region of eastern Sichuan, the correlation between sulfate aerosol and positive CG lightning is not significant, but a few areas show a positive correlation. In Figure 4B, there is a significant negative correlation between BC aerosol and positive CG lightning in the Western Sichuan Plateau, suggesting that the increase in BC aerosol would lead to a decrease in the relative frequency of positive CG lightning. In the basin, there is a negative correlation between BC aerosol and positive CG lightning relative frequency, but the correlation is weak.
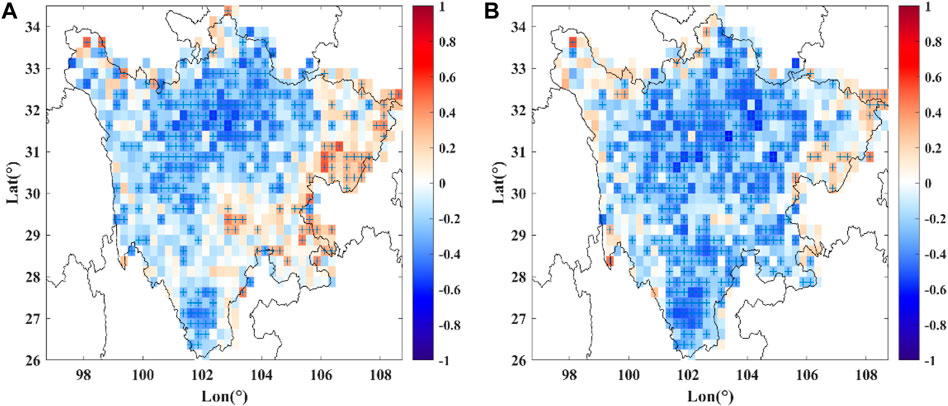
FIGURE 4. Pearson correlation coefficients between (A) the sulfate aerosol and positive CG lightning relative frequency, and correlation coefficients between (B) the BC aerosol and positive CG lightning relative frequency in Sichuan during the warm season. The cross symbol indicates that the grid has passed the 95% significance test.
Previous studies have indicated that less developed thunderstorms are more likely to produce positive CG lightning because the upper main positive charge region of the cloud is closer to the ground, which is easier for transporting positive charge to the ground (Rakov, 2003; Wang et al., 2018). Zhao et al. (2020) suggested that in the Western Sichuan Plateau with low aerosol loading, sulfate aerosol stimulated the ice-phase microphysical processes through microphysical effect, making the lightning activity more vigorous; while in the Sichuan Basin, with high aerosol loading, sulfate aerosol reduced the solar radiation reaching the ground through radiation effect, inhibiting lightning activity. In the Western Sichuan Plateau, the negative correlation between sulfate aerosol and positive CG lightning relative frequency is mainly since sulfate aerosols stimulate thunderstorms, and strong thunderclouds are not conducive to positive lightning. In the Sichuan Basin, the correlation between sulfate aerosol and positive CG lightning is not significant, which may be related to the low relative frequency of positive CG lightning in this area, the relationship between sulfate aerosol and positive CG lightning relative frequency is covered by other potentially influencing factors. Black carbon aerosol and positive CG lightning ratio showed a consistent negative correlation over the Sichuan region, suggesting that excitation effects of black carbon aerosol for lightning activities, thereby inhibiting the positive CG lightning, this may be due to the heating effects of black carbon aerosol for the lower atmosphere, the heating effect is beneficial to convection development. Liang et al. (2021) also indicated that the heating effect of black carbon aerosol on the lower atmosphere stimulated the convective intensity.
Previous observational evidence suggested that urban atmospheric particulate pollutants lead to a decline in the proportion of positive lightning (Steiger et al., 2022; Naccarato et al., 2003). They speculated that many anthropogenic atmospheric pollutants in super cooled water make graupel particles charging negatively have a wider area, and the main negative charge region extends to the ground, so more negative lightning is formed, and positive lightning activities are suppressed. These results explained the decrease in the proportion of positive lightning caused by aerosols from the perspective of the electrification process of thunderstorms, and further illustrate the important contribution of aerosols to the polarity of lightning. However, in our study, we tend to consider that aerosols change the development intensity of thunderstorms by influencing microphysical and radiation processes, thus affecting CG lightning polarity.
Dependence of the Relation Between Aerosol and Positive CG Lightning on Environmental Factors
To further analyze the dependence of aerosol influence on the relative frequency of positive CG lightning relative frequency on environmental factors, thermodynamic factors, and cloud factors were selected. Figure 5 shows the effects of CAPE, SHEAR-5km, LPW, and IWP on positive CG lightning relative frequency in the basin and plateau regions (Figure 1A). CAPE in the plateau region is smaller than that in the basin region, and CAPE has a significant inhibition effect on positive CG lightning in both regions. CAPE explains 40% and 44% of positive CG lightning relative frequency variances in the basin and plateau regions, respectively. SHEAR-5 km in the plateau region is higher than that in the basin region, and the larger vertical wind shear is favorable to the occurrence of positive CG lightning. SHEAR-5 km explains 51% of positive lightning relative frequency variance in the basin region and 60% of positive CG lightning relative frequency variance in the plateau region. In the basin, higher LWP is favorable to positive lightning, LWP explains 31% of positive CG lightning relative frequency variance, while in the plateau, the relationship between LWP and positive CG lightning is not significant. This may be since the topography of the plateau compresses the clouds, making the warm clouds thinner. In the basin, the higher LWP means the liquid-phase process is more vigorous than the ice-phase process, and the convective activity is weaker. The correlation between LWP and positive CG lightning is obviously different between the plateau and basin, which may be related to two aspects. On the one hand, due to the compression effect of the plateau terrain on a cloud, the warm cloud in the plateau is relatively thin, and LWP is relatively low. On the other hand, a thermal condition in the plateau area is weaker than that in the basin, resulting in relatively weak lightning activity. As a result, the correlation between LWP and positive CG lightning is weak over the plateau. There is a negative correlation between IWP and positive CG lightning relative frequency. IWP explains 19% and 15% of positive CG lightning relative frequency variances in basin and plateau regions, respectively. Higher ice water content indicates stronger convection, while strong convection is not conducive to positive CG lightning because a higher upper main positive charge region is not conducive to the transfer of positive charge to the ground.
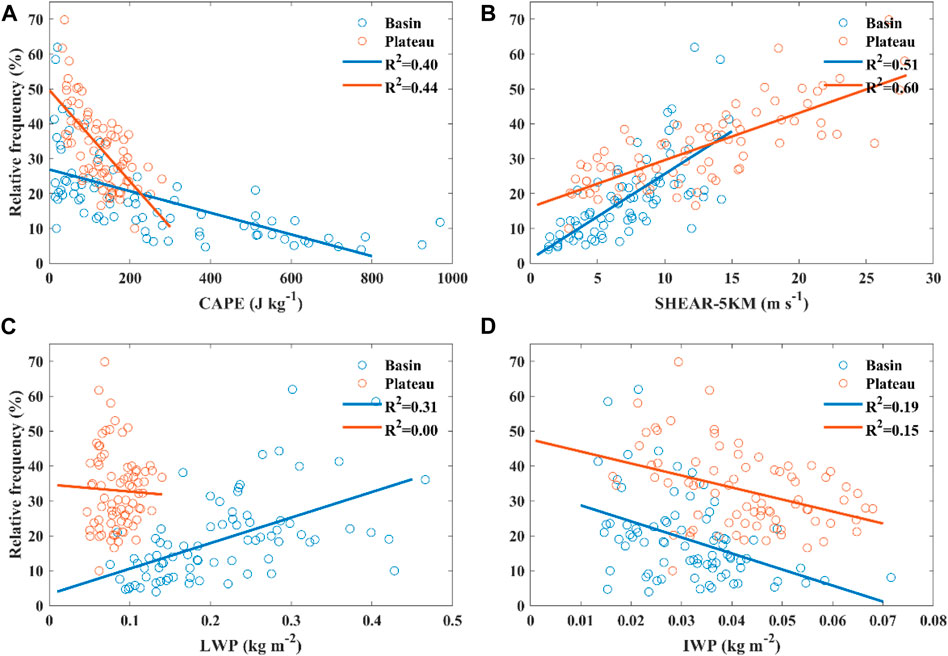
FIGURE 5. The function of CAPE (A), SHEAR-5 km (B), LWP (C), and IWP (D) with the relative frequency of positive CG lightning in the basin and plateau regions during the warm season.
In general, CAPE and SHEAR-5 km are the most prominent factors affecting positive CG lightning, weak convection is more likely to form positive lightning, and the environmental factors conducive to the development of convection have a certain inhibitory effect on positive CG lightning. Previous studies have (Wang et al., 2018; Zhao et al., 2021) also indicated that the main positive charge region at the upper part of the thunderstorm with weak development is closer to the ground, which is conducive to the transport of positive charge to the ground, meanwhile, the positive charge area of the thunderstorm which is affected by large vertical wind shear is no longer blocked by the main negative charge area at the lower part, which is more conducive to the occurrence of positive CG lightning (Brook et al., 1982; Zhao et al., 2021).
Although aerosols may have a potential relationship with positive lightning, it is still the atmospheric thermodynamic conditions that play a major role in controlling positive CG lightning. In plateau and basin areas, the relative frequency of positive CG lightning is strongly dependent on CAPE, SHEAR-5km, and IWP. Figure 6 shows the dependence of the correlation between positive CG lightning relative frequency and aerosols on CAPE, SHEAR-5 km, and IWP in the basin region. Overall, the proportion of positive CG lightning in the basin region is more dependent on environmental factors than on aerosols. When CAPE is greater than 200 J kg−1, the relative frequency of positive CG lightning is about 5%–20%, while when CAPE is less than 200 J kg−1, the relative frequency of positive CG lightning increases significantly, about 25%–65%. When SHEAR-5 km is between 5 and 15 m s−1, the relative frequency of positive CG lightning is significantly higher, about 20%, while when SHEAR-5 km is less than 5 m s−1, the relative frequency of positive CG lightning is about 10%. Compared with the thermodynamic factors, the positive lightning is less dependent on ice water content, the average relative frequency of positive CG lightning is about 20% when IPW is greater than 0.03 kg m−2, and about 30% when IWP is less than 0.03 kg m−2. For sulfate AOD, the probability of forming more positive lightning increases when AOD is greater than 0.5. For BC aerosol, the proportion of positive lightning is not significantly dependent on it. In the basin area, the lightning activity is more vigorous than that in the plateau area, but the relative frequency of positive lightning is lower than that in the plateau area. Although Zhao et al. (2020) indicated that sulfate aerosol could inhibit the lightning activity in the Sichuan Basin area through aerosol radiation interaction, for the region with a low frequency of positive lightning. The effect of aerosols on positive lightning relative frequency may be overridden by the effect of thermodynamic factors controlling lightning activities.
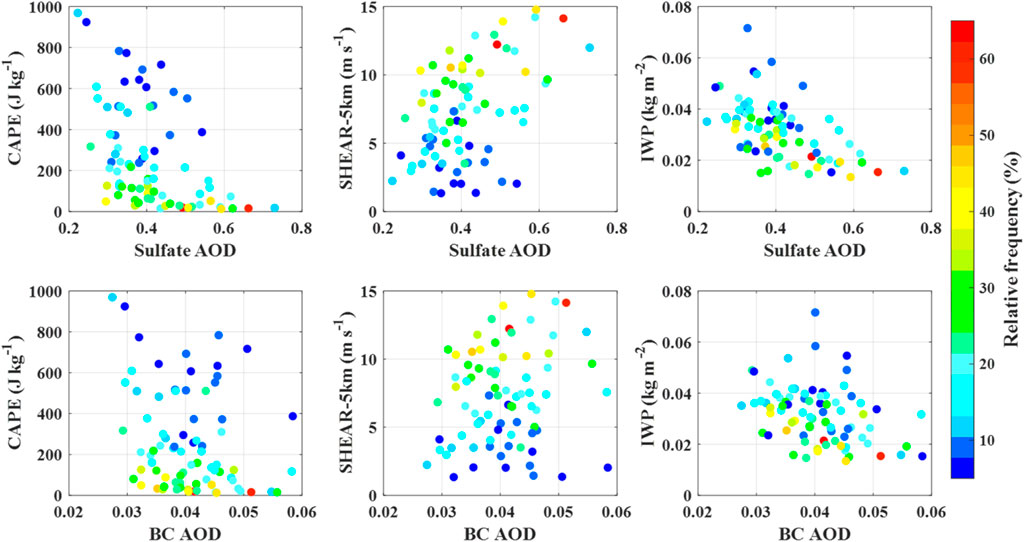
FIGURE 6. Function of positive CG lightning relative frequency with sulfate AOD, BC AOD, CAPE, SHEAR-5 km, and IWP in the basin region.
Figure 7 displays the dependence of aerosols effects on the relative frequency of positive CG lightning on CAPE, SHEAR-5 km, and IWP over the plateau. Low sulfate aerosol loading is favorable for positive lightning in the plateau region. When the sulfate AOD is less than 0.08, the average relative frequency of positive CG lightning is approximately 40%. When the sulfate AOD is less than 0.08 and CAPE is less than 100 J kg−1, the relative frequency of positive CG lightning is approximately 40%–70%. When SHEAR-5 km exceeds 10 m s−1, IWP is less than 0.04 kg m−2, and sulfate AOD is less than 0.08, the relative frequency of positive CG lightning is higher, with an average of more than 50%. A similar phenomenon exists for BC aerosols. Under small CAPE and IWP and large SHEAR-5 km conditions, low BC aerosol loading is more conducive to the occurrence of positive lightning.
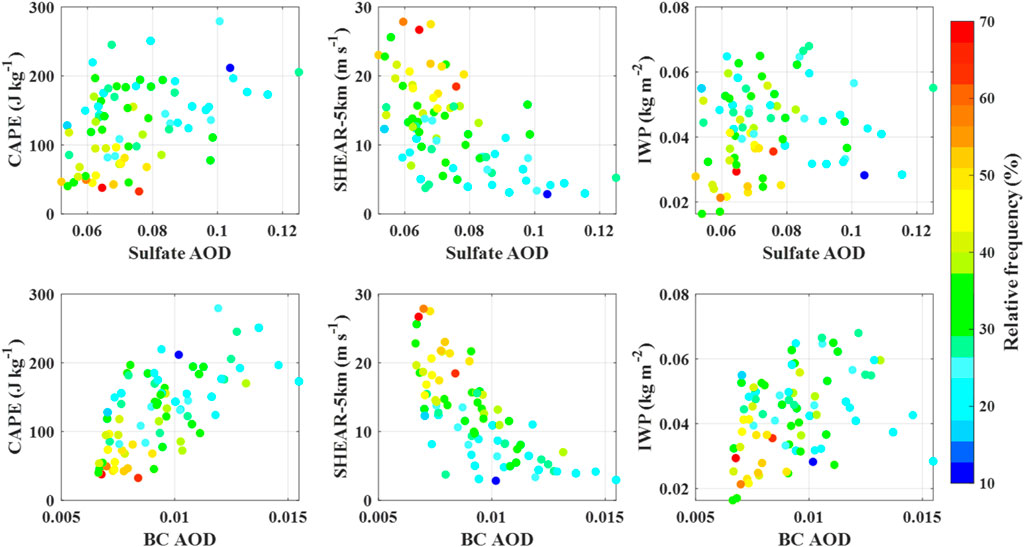
FIGURE 7. Function of positive CG lightning relative frequency with sulfate AOD, BC AOD, CAPE, SHEAR-5km, and IWP in the plateau region.
From another perspective, aerosols may have a potential influence on thermodynamic and cloud-related factors, and thus on lightning polarity. Over the plateau, sulfate aerosols may act as cloud condensation nuclei, regulating cloud thermodynamic structures by influencing microphysical processes. More sulfate aerosols make the cloud droplet radius reduced, and the number concentration increases, the cloud droplet’s collision-coalescence efficiency decreases, thus inhibiting the warm rain process. Smaller cloud droplets to be delivered to the ice-phase region form more ice particles, The release of a large latent heat of freezing process further stimulates convection development. Strong convective motion means higher positive charge distribution is not conducive for positive lightning. BC aerosols may influence the thermodynamic and microphysical processes of a thunderstorm by heating the lower atmosphere and then influencing the lightning activity. By heating the lower atmosphere, BC aerosol increases the convective energy of the local environment and then reduces the vertical wind shear, making the convective development more vigorous, promoting the formation of ice particles, and stimulating the lightning activity, while the proportion of positive lightning decreases.
Conclusion
Data on CG lightning, AOD, and environmental factors during the warm season from 2005 to 2017 in Sichuan, Southwest China, were employed to investigate the possible influence of aerosols on the relative frequency of positive CG lightning, and the dependence of this effect on thermodynamic and cloud-related factors.
In the Sichuan region, the spatial difference in thermal and water vapor conditions caused by the special terrain leads to the obvious spatial difference in lightning activity characteristics (Xia et al., 2015; Yang et al., 2015). The total CG lightning and positive CG lightning density in Sichuan Basin are significantly higher than that in the Western Sichuan Plateau, while the relative frequency and peak current of positive CG lightning in the plateau are significantly higher than that in the basin, that is, although the lightning activity is relatively weak in higher area, there are more severe positive CG lightning. The relatively high positive CG lightning relative frequency in the plateau region is dominated by multiple thermal and cloud-related factors, and relatively frequent weak convection produces more positive CG lightning (Qie et al., 2003; Qie et al., 2005; Qie et al., 2014; Zhao et al., 2021). The Sichuan Basin is one of the areas with serious air pollution in China because of the prominent anthropogenic emissions and the special terrain that is not conducive to the diffusion of atmospheric pollutants (Zhang et al., 2012; Ning et al., 2018). The sulfate aerosol and BC aerosol loads in the basin are significantly higher than those in the plateau. The ratio of sulfate AOD in total AOD is higher in the basin, while the spatial difference of BC AOD ratio in Sichuan is not significant. Observational evidence suggested that aerosols are associated with lightning activity in Sichuan, and aerosols affect the intensity of lightning activity through microphysical and radiation effects (Zhao et al., 2020). In this study, we focus on the potential impact of sulfate aerosols and BC aerosols on the relative frequency of positive CG lightning.
In the plateau region, both sulfate aerosols and BC aerosols have a significant inhibition effect on the relative frequency of CG positive lightning, while in the basin region, the correlation between aerosols and relative frequency of positive CG lightning is not significant. Due to abundant thermodynamic and water vapor conditions, convective cloud and lightning activities are vigorous in the basin, while the relative frequency of positive CG lightning is low. The influence of aerosol on positive lightning may be covered by the strong total CG lightning and the complex relationship between aerosol and convection systems. Previous studies (Lyons, 1998; Murray et al., 2000; Steiger et al., 2002; Naccarato et al., 2003; Lang and Rutledge, 2006) have found that urban anthropogenic aerosols and smoke from forest fires have significant effects on positive lightning, and it is believed that aerosols affect positive lightning by affecting the electrification process of cloud particles. In our study, we tend to consider that aerosols affect charge structure and thus positive lightning by influencing convective intensity. In the plateau region, sulfate aerosols could act as cloud condensation nuclei and stimulate thunderclouds through a microphysical effect (Zhao et al., 2020). In the highly developed thunderclouds, the distribution of the main positive charge is higher, and the positive charge is more difficult to transport to the ground, which is not conducive to the generation of positive CG lightning. BC aerosols have a prominent heating effect on the lower atmosphere, which would promote the further development of convection (Liang et al., 2021), which is not conducive to positive CG lightning. Therefore, BC aerosols are negatively correlated with the relative frequency of positive CG lightning over the Plateau. Thermodynamic and cloud-related factors play an important role in the influence of aerosols on positive lightning, and smaller CAPE and larger vertical wind shear are more conducive to the generation of positive lightning. In other words, aerosols may influence the generation of positive lightning by influencing thermodynamics and cloud structure.
Data Availability Statement
The MERRA-2 AODs data can be found in online repositories. The names of the repository/repositories and accession number(s) can be found at: https://disc.gsfc.nasa.gov/datasets/M2TMNXAER_5.12.4/summary, and the ERA5 dataset can be found at https://cds.climate.copernicus.eu/cdsapp#!/dataset/10.24381/cds.f17050d7?tab=overview; the CG lightning dataset can be obtained by contacting the first author (zpg@cuit.edu.cn).
Author Contributions
PZ and YZ contributed to the conception and design of this study. CL and YJZ contributed to data analysis and visualization. PZ, PWZ, and HX wrote the manuscript.
Funding
This research was jointly supported by the Second Tibetan Plateau Scientific Expedition and Research (STEP) program (2019QZKK0104), the Sichuan Science and Technology Program (2021YJ0393), and the National Natural Science Foundation of China (41905126 and 42075086).
Conflict of Interest
The authors declare that the research was conducted in the absence of any commercial or financial relationships that could be construed as a potential conflict of interest.
Publisher’s Note
All claims expressed in this article are solely those of the authors and do not necessarily represent those of their affiliated organizations, or those of the publisher, the editors, and the reviewers. Any product that may be evaluated in this article, or claim that may be made by its manufacturer, is not guaranteed or endorsed by the publisher.
References
Altaratz, O., Kucienska, B., Kostinski, A., Raga, G. B., and Koren, I. (2017). Global Association of Aerosol with Flash Density of Intense Lightning. Environ. Res. Lett. 12, 114037. doi:10.1088/1748-9326/aa922b
Brook, M., Nakano, M., Krehbiel, P., and Takeuti, T. (1982). The Electrical Structure of the Hokuriku Winter Thunderstorms. J. Geophys. Res. 87 (C2), 1207–1215. doi:10.1029/jc087ic02p01207
Buchard, V., Randles, C. A., Da Silva, A. M., Darmenov, A., Colarco, P. R., Govindaraju, R., et al. (2017). The MERRA-2 Aerosol Reanalysis, 1980 Onward. Part II: Evaluation and Case Studies. J. Clim. 30, 6851–6872. doi:10.1175/jcli-d-16-0613.1
Carey, L. D., and Buffalo, K. M. (2007). Environmental Control of Cloud-To-Ground Lightning Polarity in Severe Storms. Mon. Wea. Rev. 135, 1327–1353. doi:10.1175/mwr3361.1
CMA (China Meteorological Administration) (2009). China Lightning Monitoring Reports. Beijing, China: China Meteorological Press, 142.
Cummins, K. L., Murphy, M. J., Bardo, E. A., Hiscox, W. L., Pyle, R. B., and Pifer, A. E. (1998). A Combined TOA/MDF Technology Upgrade of the U.S. National Lightning Detection Network. J. Geophys. Res. 103, 9035–9044. doi:10.1029/98jd00153
Eddy, A. (2018). Environmental Conditions Producing Thunderstorms with Anomalous Vertical Polarity of Charge Structure. Master dissertation. Norman, OK: University of Oklahoma.
Jacobson, M. Z. (2001). Strong Radiative Heating Due to the Mixing State of Black Carbon in Atmospheric Aerosols. Nature 409, 695–697. doi:10.1038/35055518
Lang, T. J., and Rutledge, S. A. (2006). Cloud-to-ground Lightning Downwind of the 2002 Hayman Forest Fire in Colorado. Geophys. Res. Lett. 33 (3), L03804. doi:10.1029/2005gl024608
Liang, Z., Ding, J., Fei, J., Cheng, X., and Huang, X. (2021). Direct/indirect Effects of Aerosols and Their Separate Contributions to Typhoon Lupit (2009): Eyewall versus Peripheral Rainbands. Sci. China Earth Sci. 64 (12), 2113–2128. doi:10.1007/s11430-020-9816-7
Liu, Y., Guha, A., Said, R., Williams, E., Lapierre, J., Stock, M., et al. (2020). Aerosol Effects on Lightning Characteristics: A Comparison of Polluted and Clean Regimes. Geophys. Res. Lett. 47, e2019GL086825. doi:10.1029/2019gl086825
Lu, Q., Liu, C., Zhao, D., Zeng, C., Li, J., Lu, C., et al. (2020). Atmospheric Heating Rate Due to Black Carbon Aerosols: Uncertainties and Impact Factors. Atmos. Res. 240, 104891. doi:10.1016/j.atmosres.2020.104891
Lyons, W. A., Nelson, T. E., Williams, E. R., Cramer, J. A., and Turner, T. R. (1998). Enhanced Positive Cloud-To-Ground Lightning in Thunderstorms Ingesting Smoke from Fires. Science 282 (5386), 77–80. doi:10.1126/science.282.5386.77
MacGorman, D. R., Eddy, A. J., Homeyer, C. R., and Williams, E. (2018). “Environmental Conditions Producing Thunderstorms with Anomalous Vertical Polarity of Charge Structure,” in 25th International Lightning Detection Conference & 7th International Lightning Meteorology Conference, Florida, USA, March 12–15.
Mansell, E. R., and Ziegler, C. L. (2013). Aerosol Effects on Simulated Storm Electrification and Precipitation in a Two-Moment Bulk Microphysics Model. J. Atmos. Sci. 70 (7), 2032–2050. doi:10.1175/jas-d-12-0264.1
Murray, N. D., Orville, R. E., and Huffines, G. R. (2000). Effect of Pollution from Central American Fires on Cloud-To-Ground Lightning in May 1998. Geophys. Res. Lett. 27 (15), 2249–2252. doi:10.1029/2000gl011656
Naccarato, K. P., Pinto, O., and Pinto, I. R. C. A. (2003). Evidence of Thermal and Aerosol Effects on the Cloud-to-Ground Lightning Density and Polarity Over Large Urban Areas of Southeastern Brazil. Geophys. Res. Lett. 30, 13–1674. doi:10.1029/2003GL017496
Nag, A., and Rakov, V. A. (2012). Positive Lightning: An Overview, New Observations, and Inferences. J. Geophys. Res. 117, D08109. doi:10.1029/2012jd017545
Nenes, A., Conant, W. C., and Seinfeld, J. H. (2002). Black Carbon Radiative Heating Effects on Cloud Microphysics and Implications for the Aerosol Indirect Effect, 2, Cloud Microphysics. J. Geophys. Res. 107 (D21), 4605. doi:10.1029/2002jd002101
Ning, G., Wang, S., Ma, M., Ni, C., Shang, Z., Wang, J., et al. (2018). Characteristics of Air Pollution in Different Zones of Sichuan Basin, China. Sci. Total Environ. 612, 975–984. doi:10.1016/j.scitotenv.2017.08.205
Orville, R. E., and Huffines, G. R. (1999). Lightning Ground Flash Measurements over the Contiguous United States: 1995-97. Mon. Wea. Rev. 127, 2693–2703.
Qie, X., Kong, X., Zhang, G., Zhang, T., Yuan, T., Zhou, Y., et al. (2005). The Possible Charge Structure of Thunderstorm and Lightning Discharges in Northeastern Verge of Qinghai-Tibetan Plateau. Atmos. Res. 76 (1–4), 231–246. doi:10.1016/j.atmosres.2004.11.034
Qie, X., Liu, D., and Sun, Z. (2014). Recent Advances in Research of Lightning Meteorology. J. Meteorol. Res. 28 (5), 983–1002. doi:10.1007/s13351-014-3295-0
Qie, X., Toumi, R., and Zhou, Y. (2003). Lightning Activity on the Central Tibetan Plateau and its Response to Convective Available Potential Energy. Chin. Sci. Bull. 48 (3), 296–299. doi:10.1007/bf03183302
Rakov, V. A. (2003). A Review of Positive and Bipolar Lightning Discharges. Bull. Amer. Meteor. Soc. 84 (6), 767–776. doi:10.1175/BAMS-84-6-767
Randles, C. A., Da Silva, A. M., Buchard, V., Colarco, P. R., Darmenov, A., Govindaraju, R., et al. (2017). The MERRA-2 Aerosol Reanalysis, 1980 Onward. Part I: System Description and Data Assimilation Evaluation. J. Clim. 30, 6823–6850. doi:10.1175/jcli-d-16-0609.1
Steiger, S. M., Orville, R. E., and Huffines, G. R. (2002). Cloud-to-ground Lightning Characteristics over Houston, Texas: 1989–2000. J. Geophys. Res. 107 (D11), 4117. doi:10.1029/2001jd001142
Tan, Y., Peng, L., Shi, Z., and Chen, H. (2016). Lightning Flash Density in Relation to Aerosol over Nanjing (China). Atmos. Res. 174, 1–8.
Wang, D., Wu, T., and Takagi, N. (2018). Charge Structure of Winter Thunderstorm in Japan: A Review and an Update. IEEJ Trans. PE 138 (5), 310–314. doi:10.1541/ieejpes.138.310
Wang, Y., Wan, Q., Meng, W., Liao, F., Tan, H., and Zhang, R. (2011). Long-term Impacts of Aerosols on Precipitation and Lightning over the Pearl River Delta Megacity Area in China. Atmos. Chem. Phys. 11, 12421–12436. doi:10.5194/acp-11-12421-2011
Xia, R., Zhang, D.-L., and Wang, B. (2015). A 6-yr Cloud-To-Ground Lightning Climatology and its Relationship to Rainfall over Central and Eastern China. J. Appl. Meteorol. Clim. 54, 2443–2460. doi:10.1175/jamc-d-15-0029.1
Yang, X., and Li, Z. (2014). Increases in Thunderstorm Activity and Relationships with Air Pollution in Southeast China. J. Geophys. Res. 119, 1835–1844. doi:10.1002/2013jd021224
Yang, X., Sun, J., and Li, W. (2015). An Analysis of Cloud-To-Ground Lightning in China during 2010–13. Weather Forecast 30 (6), 1537–1550. doi:10.1175/WAF-D-14-00132.1
Yang, X., Yao, Z., Li, Z., and Fan, T. (2013). Heavy Air Pollution Suppresses Summer Thunderstorms in Central China. J. Atmos. Solar-Terrestrial Phys. 95-96, 28–40. doi:10.1016/j.jastp.2012.12.023
Yu, R., Xu, Y., Zhou, T., and Li, J. (2007). Relation between Rainfall Duration and Diurnal Variation in the Warm Season Precipitation over Central Eastern China. Geophys. Res. Lett. 34, L13703. doi:10.1029/2007gl030315
Zhang, X. Y., Wang, Y. Q., Niu, T., Zhang, X. C., Gong, S. L., Zhang, Y. M., et al. (2012). Atmospheric Aerosol Compositions in China: Spatial/temporal Variability, Chemical Signature, Regional Haze Distribution and Comparisons with Global Aerosols. Atmos. Chem. Phys. 12, 779–799. doi:10.5194/acp-12-779-2012
Zhang, Y., Sun, J., and Fu, S. (2014). Impacts of Diurnal Variation of Mountain-Plain Solenoid Circulations on Precipitation and Vortices East of the Tibetan Plateau during the Mei-Yu Season. Adv. Atmos. Sci. 31, 139–153. doi:10.1007/s00376-013-2052-0
Zhao, P., Li, Z., Xiao, H., Wu, F., Zheng, Y., Cribb, M. C., et al. (2020). Distinct Aerosol Effects on Cloud-To-Ground Lightning in the Plateau and Basin Regions of Sichuan, Southwest China. Atmos. Chem. Phys. 20, 13379–13397. doi:10.5194/acp-20-13379-2020
Zhao, P., Xiao, H., Liu, C., and Zhou, Y. (2021). Dependence of Warm Season Cloud-To-Ground Lightning Polarity on Environmental Conditions over Sichuan, Southwest China. Adv. Meteorology 2021, 1–12. doi:10.1155/2021/1500470
Zhao, P., Xiao, H., Liu, C., Zhou, Y., Xu, X., and Hao, K. (2022a). Evaluating a Simple Proxy for Climatic Cloud‐to‐ground Lightning in Sichuan Province with Complex Terrain, Southwest China. Intl J. Climatol. 42 (7), 3909–3927. doi:10.1002/joc.7451
Keywords: sulfate aerosol, black carbon aerosol, positive lightning, thermodynamic factors, Sichuan
Citation: Zhao P, Zhang Y, Liu C, Zhang P, Xiao H and Zhou Y (2022) Potential Relationship Between Aerosols and Positive Cloud-to-Ground Lightning During the Warm Season in Sichuan, Southwest China. Front. Environ. Sci. 10:945100. doi: 10.3389/fenvs.2022.945100
Received: 16 May 2022; Accepted: 23 June 2022;
Published: 01 August 2022.
Edited by:
Honglei Wang, Nanjing University of Information Science and Technology, ChinaReviewed by:
Zheng Shi, Nanjing University of Information Science and Technology, ChinaYue Zhou, China Meteorological Administration, China
Copyright © 2022 Zhao, Zhang, Liu, Zhang, Xiao and Zhou. This is an open-access article distributed under the terms of the Creative Commons Attribution License (CC BY). The use, distribution or reproduction in other forums is permitted, provided the original author(s) and the copyright owner(s) are credited and that the original publication in this journal is cited, in accordance with accepted academic practice. No use, distribution or reproduction is permitted which does not comply with these terms.
*Correspondence: Yuanyang Zhang, enl5c2d0Y0B5ZWFoLm5ldA==