- 1School of Food Science Environmental Health, Technological University Dublin, Dublin, Ireland
- 2Environmental Sustainability and Health Institute, Technological University Dublin, Dublin, Ireland
- 3Green Restoration Ireland Cooperative Society Ltd., Carlow, Ireland
- 4FOCAS Research Institute, Technological University Dublin, Dublin, Ireland
Degraded peatland has reduced many ecosystem services, such as water quality and quantity, biodiversity, carbon storage, climate regulations, and other cultural benefits. Therefore, several initiatives for the restoration of degraded peatland (RDP) have attempted to restore the ecosystem processes, productivity, and services of the degraded peatland to its original natural condition. Notwithstanding the popularity of RDP research among researchers and industry practitioners, a quantitative technique to map a comprehensive survey of the intellectual core and the general body landscape of knowledge on RDP research does not exist. In this study, a scientometric analysis was employed to analyze 522 documents using VOSviewer and CiteSpace. The Web of Science database was used to retrieve bibliographic records using the advanced search “TS (topic) = (‘drained peatland restoration’ OR ‘drained bog restoration’ OR ‘drained mire restoration’ OR degraded peatland restoration’ OR ‘degraded bog restoration’ OR ‘drained peatland reclamation’ OR ‘drained bog restoration’ OR‘ degraded peatland reclamation’ OR ‘degraded bog reclamation’ OR‘ drained mire restoration’ OR ‘degraded mire reclamation’ OR ‘degraded fen restoration’ OR ‘drained fen reclamation’). “The outcome sought to provide relevant information in RDP research, such as (i) publication trends, (ii) research outlets, (iii) most influential keywords, (iv) most influential institutions and authors, and (v) top influential countries active in RDP research. In addition, four clusters were identified to ascertain the central theme of RDP research, in which cluster one is linked to the central research theme-“ impact of drainage on peatland ecosystem services; cluster two focused on the impact of peatland restoration on greenhouse gas emissions; cluster three is associated with peatland restoration and biogeochemical properties; and cluster four is related to peatland restoration and species richness. A new research hotspot, such as soil respiration, was identified via the keywords with the strongest citation bursts. This study will provide various stakeholders (e.g., industry, journal editors, policymakers, and researchers) with an instinctive understanding of the research status and the development frontier of RDP research.
1 Introduction
Peatlands are found in an estimated 180 countries (Parish et al., 2008), covering 4.23 million km2, or 2.84% of the global land area. They play a significant role in mediating the ecosystem functions, such as carbon storage, rich biodiversity, water retention and water quality, and livelihood (Joosten, 2009; Xu et al., 2018).
Nevertheless, most countries have degraded more than half of their original peatland coverage for agriculture and energy. One of the major causes of degradation of peatlands is the drainage for timber production, affecting approximately 15 million hectares in the northern boreal subarctic regions (Strack et al., 2008). It has been estimated that 50 million ha (13%) of peatlands have been directly altered by human land use in most European countries (Lappalainen 1996; Strack et al., 2008; Tanneberger and Wichtmann 2011). For example, Germany has degraded more than 85% of its original peatlands, an estimated 930,000 ha of drained peatlands (Joosten et al., 2017). In Ireland, peat soils cover 21% of the total land area, with a total peatland area of 1,564,650 hectares, but it is estimated that only 10% of raised bogs and 28% of blanket bogs are in a natural state (Pike, 2021). Peatlands make up 12% of the Southeast Asia, estimated to be around 27 million peatlands (Joosten et al., 2017). However, out of 27 million hectares of peatland, 12 million hectares (45%) are currently degraded. For example, in the past 15 years, it has been estimated that 3 million hectares of Indonesia’s peatlands have been burned to make way for farming and logging. In Scotland, 70% of blanket bogs and 90% of raised bogs have been damaged.
Degraded peatland has reduced many ecosystem functions, such as water quality and quantity, biodiversity, and climate regulations (Bonn et al., 2016). Many studies have indicated that huge amounts of carbon have been emitted into the atmosphere due to peatland deforestation and degradation, draining, and repeated fires (Ballhorn et al., 2009; Jaenicke et al., 2010; Hooijer et al., 2014). The removal of above- and below-ground biomass, peat decomposition and oxidation caused by drainage, and peat combustion are all major sources of carbon loss and CO2 emissions into the atmosphere. Meanwhile, the application of N fertilizers on degraded peatland acts as a source of N2O emission, especially when nitrogen fertilizers have been added to promote agricultural productivity (Dohong et al., 2017; Mishra et al., 2021). Tree harvesting by clear-cutting on drained peatland has also been reported to enhance the leaching of dissolved organic C (DOC), dissolved organic N (DON), and mineral N to surface waters and the nearest catchment in a boreal peatland (Könönen et al., 2018). The clear-cutting of trees exposes the peat surfaces to direct sunlight, which and stimulates the decomposition of the drier peat through increased aerobic mineralization, causing high concentrations of DOC and associated watercolor to upland and seminatural catchments (Hooijer et al., 2014). Therefore, several restorations of degraded peatland (RDP) initiative has been attempted to restore the ecosystem processes, productivity, and services of the degraded peatland to its original natural condition (Haapalehto et al., 2011; Bonn et al., 2014).
RDP research has received considerable attention from researchers but is not limited to industry practitioners, with several researchers publishing traditional review papers from different aspects to summarize its development and effectiveness. Several review studies have made significant contributions to the RDP research discourse (Prince et al., 2003; Andersen et al., 2017; Chimner et al., 2017; Dohong et al., 2018; Leifeld and Menichetti, 2018; Harrison et al., 2020). Regardless, using the traditional reviews has some limitations because it has been qualitative and is subjected to manual evaluation according to the researcher’s experience, which has been disparaged for irreproducibility and predilection to subjective biases (Hammersley, 2001; Yu and Liao, 2016).
According to Markoulli et al. (2017), traditional reviews seek to investigate the “trees,” but do not provide a wide overview of the “forest.” In addition, most existing review work has narrowed perspectives focusing on specificity, limiting aspects of RDP research. For example, Yuwati et al. (2021) review work focused on the restoration of degraded tropical peatland in Indonesia, while Dohong et al. (2017) study focused on a review of the drivers of tropical peatland degradation in South-East Asia. However, to the best of our knowledge, the existing literature on RDP is restricted to specific locations and review studies that provide a complete insight into RDP using the quantitative technique are inadequately examined. To fill this gap, the present study employs analytical algorithms to map a comprehensive survey of the intellectual core and the landscape of the general body of knowledge on RDP research. Specifically, a scientometric analysis was examined to evaluate the intellectual discourse and landscape of the general body of knowledge of (i) publication trend of RDP; (ii) country’s co-authorship analysis, keywords co-occurrence analysis, research outlet analysis and document citation analysis, and (iii) a comprehensive discussion on key themes using the keywords co-occurrence analysis.
1.1 World peatland coverage
Peatlands are a distinctive wetland type that are characterized by the accumulation of partially decayed organic matter, resulting in the formation of peat layers (Likens, 2009). To date, there is no accepted standard definition of “peat” and “peatland,” with different interest groups often using their definitions for peatland. For example, Burton & Hodgson (1987) define peat or peatland as > 50% OM, measured as a loss on ignition, while Joosten and Clarke (2002) determined peatland or peat as sedentarily accumulated material which consists of about 30% (dry mass) of dead organic material. In Ireland, 45-cm thick peat moss is considered a peatland, while Germany’s minimum thickness is approximately 30 cm (Steffens, 1996).
Peatlands cover 2.84% of the global land area, mainly in the mid-high latitudes of the Northern Hemisphere, with Canada containing 27% of the world’s peatlands and is the second country with the most peatlands after Russia (Xu et al., 2018). Bogs and fens are the dominant peatland types in Canada. Bogs cover 67% (762 × 103 km2) of the total peatland area and fens cover 32% (367 × 103 km2), while marshes and swamps cover the remaining 1% (Tarnocai, 2006). Boreal (and polar), temperate, and tropical zone contribute about 83.3%, 4.0, and 12.7%, respectively, to the total peatland coverage (Leifeld and Menichetti, 2018). Active peat formation is found in the moist temperate climates predominate in the northern areas of the circumboreal taiga zone of coniferous forests, Finland, Alaska, and Canada (Tarnocai and Stolbovoy, 2006). The percentages of the global breakdown of peatland area coverage (4.23 million km2) are shown in Figure 1, with the highest and the lowest percentage of peatland coverage from Asia and the Ocean, respectively.
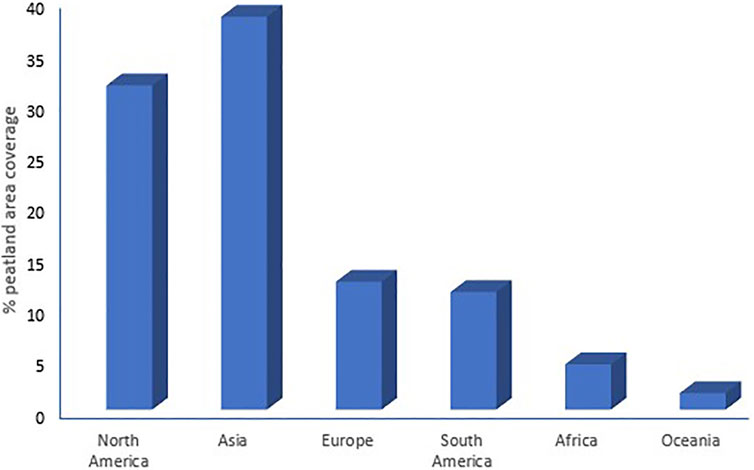
FIGURE 1. Percentage of the world’s peatland coverage. Data used was retrieved from PEATMAP (Xu et al., 2018). As a result, 4.23 million km2 was used as the total global peatland area coverage.
In addition, peatlands are predominant outside the boreal zone proper in Ireland, Scotland, Germany, southwest Sweden, and northern Poland, northern Minnesota, and the Everglades of Florida (Sjörs, 1980). Canada contains an estimated of 27% of the world’s peatlands and is the country with the most peatlands after Russia. Generally, peatlands are widespread in Asia, North America, Europe, and South America (FAO, 2012).
1.2 Types of peatland
Peatland formation results from flooding or waterlogged conditions, which results in the inhibition of organic materials decompositions, specifically plant material, due to the oxygen diffusion being impeded by the flooding conditions (Dise, 2009). There are many different ways of classifying peatland based on the purposes of the classifications. Recently, peat-forming systems have been classified into two broad peat-forming types based on the hydrogenetic type: the ombrogenous (nutrient-poor bogs) and geogenous bogs (fen), which differ in vegetation cover, water availability, and nutrient supply, geography and climate, and many more (Flores-Moreno et al., 2016). Water availability on peatland surfaces is significant for the distribution of vegetation, biomass productivity, nutrient transfer, decomposition, and preservation of organic matter in the peatland types. Nutrients (chemical elements, compounds, and metals) supplied to the peatland stimulate the growth metabolism of plants, either positively or negatively affecting the biodiversity, thereby influencing the type of peatland form (Lamers et al., 2012).
Topography and climate conditions are factors that cannot be ignored during peatland formation because they affect the productivity of the ecosystem by influencing the phytosociology, biomass production, energy flux, plant dynamics, and soil and nutrient cycling (Graniero and Price, 1999). For example, herbaceous plant remains in the temperate climatic region produce fibric to hemic peats during decomposition; while in the humid climatic region highly decayed woody plant remains produce hemic to sapric peats, influencing the nutrient flux and types of peat form (Flores, 2014).
Ombrogenous peatlands (bog) receive water and nutrients from the atmosphere because they are isolated from the groundwater. They are found mainly in the temperate and the tropics region, with differing peatland types, vegetation cover, peat depth, and topography (Joosten & Clarke, 2002; Lavoie et al., 2005a). Unlike fen, ombrogenous bog depends only on rainfall for water and nutrient supplies, which influence the vegetation and the plant biomass cover. This results in the formation of an above-ground biomass, which is known as raised or blanket bogs (Nykänen et al., 1998: Flores, 2014).
A raised bog is characterized by complex structures of organic debris reaching an estimated thickness of 12 m, comprising three sediments stratigraphy, namely a basal tier of the bog formed under minerotrophic groundwater, sub-surface, and upper tiers (Hammond, 1981). In addition, the layers formed in the raised bog consist of a loose living moss at the surface, dead moss, and a partially decomposed peat that retards evapotranspiration during the intense temperature rise, hence protecting the bog from water loss (Minayeva and Sirin, 2011). Raised bogs consist of low concentrations of dissolved nutrients and low pH (<5.0) with sphagnum mosses, but can also contain patches of sedge (Carex sp.), small shrubs, and trees (Bubier et al., 1995).
A blanket bog consists of peat in a moderately deep accumulation of 2.6 m thick that is deposited on gentle to steep slopes (Hammond, 1981), having a low pH (<5.0), and a predominate vegetation cover of Vaccinium myrtillus, t’mpetrum nigrum, and Diplophyllum_albican. The onset of the blanket bog formation is closely correlated with climatic deterioration within the post-glacial period. Its formation is controlled by climatic factors such as rainfall (>1,250 mm), high atmospheric humidity, and topography (Hammond, 1981). The topography influences the flow of nutrients leached by rain and the vegetation distribution, as reported by Hammond (1981). The high acidity of ombrotrophic bogs originates from their organic acids, predominately fulvic and humic acid, and the sequestration of cations in peat. Generally, the pH of the blanket and the raised bogs is dependent on the carboxylic acid (R−COOH) content of dissolved organic matter (DOM) originating in the peat, ion exchange with living plants (e.g., bryophytes), and the nutrient content received from their atmosphere (Sjörs and Gunnarsson, 2002).
Fens are wetlands with accumulated peat of 40 cm thick, receiving water and nutrients from rainfall, inflowing streams, and groundwater, making them fertile for plant growth compared to the ombrogenous peatlands. The enriched water from the inflowing streams and the surrounding watershed provides an array of minerals for a diverse plant community and stimulates the degree of oxygenation to the organic substrate, thereby accelerating peat decomposition (Miettinen & Liew, 2010). The formation of geogenous bogs occurs at raised bogs bases (ombrogenous bogs) and the central plain, in river valleys, and poorly drained hollows adjacent to raised bogs. The fens are characterized by acidic to alkaline water ranging from 4.5 to 7.5, with brown mosses and herbaceous plants as the common vegetation (Crum, & Planisek, 1992). The high pH of the fen is due to the input of water and minerals from the ground, overland runoff, and the nearby watershed serving as a source of more mineral-derived alkalinity and higher pH (Bridgham et al., 1999). With increasing pH, Sjörs (1950) subdivided fen into acidic fens, intermediate fens, and rich fens. Sjörs and Gunnarsson (2002) conclude that the high pH of an intact or undisturbed fen peatland is due to the release of bicarbonate and carbonate from the groundwater to buffer the pH of the fen.
1.3 Effect of degraded peatland on ecological functions
The impact of peatlands that have been degraded or disturbed has changed in physical characteristics, biology, and chemistry, resulting in a loss of the ecological function of the peat, putting environmental and social-economic development at risk (Maftu’ah et al., 2019). Some of the repercussions of disturbing peatland include hydrophobicity, increased soil acidity, and decreased total organic carbon (TOC) and total organic nitrogen (TON) (Anshari 2010). Irreversible drying conditions indicate degraded peat features (Salmah et al., 1991). According to Valat et al. (1991), the hydrophobic character of peat soil is caused by: the presence of humic acid, which is naturally hydrophobic because its particles are covered by wax; the presence of non-polar groups, such as ethyl, methyl, and temporary aromatic compounds, which cause the hydrophilic group to decrease; and the absorption of hydrophobic substances, such as oil, fat, and N-organic fractions on the surface of the humic fraction. Soil hydrophobicity can aid in evaluating soil quality and fertility factors (Matejková and Simon, 2012). According to Maftu’ah et al. (2019), soil water content at the study site showed a substantial variation. Compared to natural peatlands and agricultural land, there was a considerable decrease in water content in degraded peatlands, reaching more than 3 times lower.
Soil pH in degraded peatlands is 3.62, whereas in agricultural soils it is 4.70 (Maftu’ah et al., 2019). According to Maftu’ah et al. (2019), the overall pH of soil differed significantly. Ion H+ and Al3+ were the main sources of the low pH in the peat in the examined area (Maftu’ah et al., 2019). The dissociation of organic acids, specifically plant-derived acids, which are usually dominated by fulvic and humic acid in peat was the source of the H+ ion. Organic acids play a significant role in peat soil’s low pH. Carboxylates (R-COOH) and phenols (C6H4OH) are the most abundant reactive groups in decomposed organic matter, and they dominate the exchange complex. Strong organic acids can dissociate and produce enormous amounts of ions. The presence of Al ions is also a source of higher acidity (Maftu’ah et al., 2019).
In an experiment, disturbed or degraded peatlands had the highest total N values, while natural peatlands had the lowest (Maftu’ah et al., 2019). This happens because plant materials contribute to organic matter in the soil, and natural peatlands have low decomposition rates, which may help maintain high organic matter (Maftu’ah et al., 2019). Because bog drainage has been demonstrated to boost production rates, dissolved organic carbon (DOC) concentrations are a particular concern in degraded peatlands (Bussell et al., 2010). This could be due to increased microbial activity and phenolic component breakdown caused by oxygenation (Fenner and Freeman, 2011) and changes in peat structure (Minkkinen and Laine, 1998; Holden, 2005). Other nutrients, such as nitrogen (N) and phosphorus (P), which are held in substantial amounts in the upper layers of peat soils are also prone to losses as a result of oxygenation and subsequent mineralization (Miller et al., 1996; Tiemeyer et al., 2007).
Peatland is one of the World Wildlife Fund’s internationally important ecological regions (Brooks et al., 2006). The majority of wild animals and flora have been known to thrive in this location due to ideal habitats (e.g., fertile soil, rainforest, and a favorable environment) (Adesiji, et al., 2015). According to Parish and Looi (1999), agricultural development causes peatland removal, which results in a loss of biodiversity and habitat for some indigenous flora and wildlife. Prior to the invasion of peatlands for logging and expansion of oil palm plantations, there was a high level of vegetation and fauna (Adesiji, et al., 2015).
Emissions from degraded peatlands are estimated at 2 gigatonnes of CO 2e annually, due to peatland deforestation and degradation, draining, and repeated fires (Zheng et al., 2021). The removal of above- and below-ground biomass, peat decomposition and oxidation caused by drainage, and peat combustion are all major sources of carbon loss and CO2 emissions; Hooijer et al., 2012; Hooijer et al., 2006). Draining peatland for large-scale agriculture and industrial plantations has been a global concern in recent decades due to significant CO2 emissions from peat oxidation and decomposition, which contribute to global climate change (Hooijer et al., 2012; Biancalani and Avagyan, 2014).
2 Methodology
This current study employs analytical algorithms that are known as scientometrics to detect the intellectual structure of RDP by analyzing the major topic studied, and the connections among researchers and major studies (Nikolenko et al., 2017). Scientometric is a subset of informetric analysis process and visualization of bibliometric data of a particular research theme. This method is useful for visualizing significant patterns and trends in RDP research discourse. Scientometric studies produce an authentic and less skewed result that is not influenced by any individual’s or author’s perspective (Martinez et al., 2019; Şenel, 2019; Baker et al., 2021). Furthermore, it provides solutions to the difficulties experienced by scholars doing traditional reviews and connects journals, keywords, writers, publications, and nations within a specific study topic (Darko et al., 2020; Zhang et al., 2021). Therefore, this study uses maps and bibliometric data to quantify research trends and highlight research hotspots in the RDP research discourse.
2.1 Data collection and search strategy
The Web of Science (WOS) database was selected to retrieve bibliography information of documents in RDP research. The WOS was chosen for the data collection because its research entails over 3,300 carefully selected publishers and over 12,000 high-impact journals, indexed in the database since 1900 (Li et al., 2021). The data used for this study was extracted on 15 January 2022, from the online library of the Technological University Dublin using the advanced search “TS (topic) = (‘drained peatland restoration’ OR ‘drained bog restoration’ OR ‘drained mire restoration’ OR degraded peatland restoration’ OR ‘degraded bog restoration’ OR ‘drained peatland reclamation’ OR ‘drained bog restoration’ OR ‘degraded peatland reclamation’ OR ‘degraded bog reclamation’ OR ‘drained mire restoration’ OR ‘degraded mire reclamation’ OR ‘degraded fen restoration’ OR ‘drained fen reclamation’). If the defined terms appeared in the title, keywords, or abstracts, then the documents would be identified. In addition, data refinement techniques were employed such that the “document type” was limited to “review” and “article” only, while the language type was limited to English only and the “timespan” was set to “1945 to 2021.” As a result, 522 documents were retrieved by keyword based-bibliometric for the scientometric analysis. Retrieved documents from the WOS database were then saved in “plain text” with “full record and cited references”.
2.2 Science mapping
Scientometric techniques such as network construction through keywords co-occurrence analysis, document co-citation analysis, citation burst analysis, outlets direct citation analysis, and co-authorship analysis were carried out via VOSviewer and CiteSpace. Vosviewer and the CiteSpace were selected as science mapping tools to analyze the retrieved bibliographic data in the RDP research discourse. Vosviewer is a software tool that offers basic functionality for producing, visualizing, and exploring bibliometric networks (Van Eck & Waltman, 2020).
The output of the VOSviewer is a distance-based map that consists of nodes and edges, whereas the node size reveals the frequency of occurrence of a topic in the abstract and titles of published documents in relation to specific research topics and the edges indicate the relations among the nodes, strength, and weight of the relations (Perianes-Rodriguez et al., 2016; Oraee et al., 2017). In addition, the total length strength is attributed to the strength of the relationship between nodes, as reported in Elisha and Viljoen (2021), while a link referred to a connection or a relationship between two items.
Fractional counting was selected to create the distance-based map. The idea of selecting fractional counting is to decrease the influence of documents having many authors such that the co-authorship link strength between two authors is evaluated not only by the number of documents co-authored by the authors but also by the total number of authors of each of the co-authored documents (Van Eck & Waltman, 2013).
Similarly, to the VOSviewer, the CiteSpace also performs visual analytic functions of science mapping, as reported in Chen (2006). In this study, burst detection in CiteSpace was used to provide evidence of which keywords have frequently been cited within RDP research. The burst detection in CiteSpace is based on Kleinberg’s algorithm (Kleinberg, 2002).
2.2.1 Keywords co-occurrence analysis
Keywords represent a published document’s content or the core theme of a research paper, (Cobo et al., 2011; Van Eck & Waltman, 2014; Shrivastava, & Mahajan, 2016). According to Su and Lee (2010), keyword analysis offers an opportunity to ascertain the central theme of particular research topics (Chen et al., 2021; Van Eck and Waltman, 2014; Hosseini et al., 2018: van Eck et al., 2010). To compute the visualization of the keywords, all keywords were used rather than the author’s keywords. According to Hosseini et al. (2018), using all keywords for mapping-based studies produces a large number of terms that are not solely dependent on authors’ experience and knowledge in choosing appropriate research keywords. All of our keywords have been widely used in several science mapping-based studies (Ravikumar et al., 2015; Williams et al., 2016; Zhang et al., 2017; Fridell et al., 2020). A total of 2,578 keywords were obtained from the WOS database using the fractional counting methodology. Regarding the “minimum number of occurrences” for a keyword to be included in the network, a value of 9 was selected, and the inclusion criterion was met by 103 of the 2,578 keywords.
2.2.2 Outlets direct citation analysis
Outlets’ direct citation analysis can be significant to researchers in identifying the best journals for their research publication (Hosseini et al., 2018). Furthermore, according to Guidry et al. (2004) and Darko et al. (2019), performing outlets’ direct citation analysis assists the journal editors in making adjustments to the goals and objectives of their journals, and assists institutions/libraries in optimizing the resources allocation for investing in journals. A total of 181 sources were obtained from the WOS database using the fractional counting methodology. The “minimum number of documents of a source” and the “minimum number of citations of a source” were set to 5 and 60, respectively. Of the 180 sources found, 20 items met the threshold and were added to the resultant network for the outlet’s direct citation analysis in RDP research.
2.2.3 Document citation networks in RDP
Document citation analysis helps to reveal the intuition of the structure of a scientific knowledge domain. The concept of citation has been used as a research method to measure the degree of relationship between documents and the impact of the publication. Therefore, citation analysis of documents was computed to analyze the citations of documents in the RDP research domain. A total of 522 documents were reported using the fractional counting methodology. The minimum number of citations of the documents” was set to 70. Of the 522 documents found, 18 items met the threshold and were added to the resultant network.
2.2.4 Co-authorship analysis
Co-authorship networks can evaluate scientific collaboration networks. The minimum number of documents of an author and citations were set to 7 and 40, respectively. Of the 1,366 authors, only 15 authors met the threshold and were added to the resultant network.
2.2.5 Active countries in the RDP research
The significance of determining the most active countries in the RDP research domain is to enhance future collaboration, specialties, and expertise, and can promote the exchange of technologies and innovation among countries, as reported in Wuni et al. (2019). The resultant network was generated by setting the minimum number of documents and the citation of a country to 11 and 20, respectively. Of the 51 countries of RDP research discourse, 12 met the threshold.
3 Results and discussion
3.1 Publication trend of RDP research
The RDP literature data retrieved from WOS revealed that RDP research began in 1991, based on our search keywords, as seen in Figure 2. The first study was conducted by Van Diggelen et al. (1991), who analyzed the hydro-ecology of the fen system at Leiper Posse in eastern Germany. Poddubny and Galat conducted the second study, which was published in the Regulated Rivers: Research & Management journal. Poddubny and Galat’s (1995) research, which was titled “Habitat associations of upper Volga River fishes: effects of reservoirs”, recommended that the restoration of degraded bog areas or flood plains can enhance the diversity and productivity of fishes in the upper Volga River. This indicates that RDP research has been around since 1991. Figure 2 shows the publication trend of RDP from 1991 to 2021. The number of documents published increased from 1 document in 1991 to 61 documents in 2021. Figure 2 shows an exponential increase of published documents over the reported period (1991–2021), which may probably be due to the world recognition of the significance of RDP research in the enhancement of ecosystem services. Examples of ecosystem services indicators that have been reported to enhance after the restoration of degraded peatland from 1991 to 2021 include atmospheric CO₂ sequestration (Waddington and Warner, 2001), vegetation (Tuittila et al., 2000), organic carbon (Wallage et al., 2006), porewater (Meissner et al., 2003) and so on. A steady and tremendous increase in RDP research was observed from 2012 to 2015, with a sharp decrease in 2016. Unexpectedly, there was an increase in published documents from 2019 to 2021.
The rising importance of RDP research agrees with Andersen et al.’s (2017) and Waddington et al.’s (2010) findings that most countries have degraded more than half of their original peatland coverage for agriculture and energy use, resulting in greenhouse gas emissions. Therefore, RDP research aims to decrease greenhouse gas emissions and enhance on-farm biodiversity conservation and other environmental-related issues. For example, Chapman et al. (2012) emphasized that integrating RDP as mitigation measures for reducing greenhouse gas (GHG) in Scotland could provide up to 2.7 Mt CO2-eq savings per year. A similar observation was made in Ireland that restoring degraded peatland through rewetting could reduce CO2 emissions by enhancing C sequestration, as Wilson et al. (2012) and Wilson et al. (2013) reported.
An overview of the RPD is presented in Table 1. As observed in Table 1, the total number of citations increased from 1991 to 2021. However, a slight fluctuation of citations per published document is seen with the highest total times cited observed in 2021. Therefore, based on search keywords in this study, it can be deduced that since RDP started in 1991, there have been 522 published documents, achieving total times cited of 10,480 in the RDP research field.
3.2 Structure of the body of knowledge in RDP: Research outlet, keywords co-occurrence analysis, and document citation analysis
3.2.1 Keywords co-occurrence analysis
The keywords co-occurrence network analysis helps to group the keywords into different clusters. Four clusters were obtained entailing 74 nodes, 2,730 links and a total link strength of 1,242.50, as seen in Figure 3, depicting the major keywords and their inter-relatedness of the current RDP research. The colors of each node indicate the different clusters to which the terms belong. A term was assigned to each cluster to provide in-depth interpretation and understanding of the central research theme within the RDP.
3.2.1.1 Cluster #1
Keywords explaining peatland drainage impact are classified into cluster one, represented in yellow, containing drainage, climate change, hydrology, water, hydraulic conductivity, and fire (Figure 3). The analysis of all keywords revealed that the keywords in cluster one are linked to the central research theme “impact of drainage on peatland ecosystem services,” which suggests that peatland drainage is a major threat to peatland degradation.
Peatland formation results from flooding or waterlogged conditions, which results in the inhibition of organic materials decompositions, specifically plant materials, due to the oxygen diffusion being impeded by the flooding conditions (Dise, 2009). As a result, peatland drainage is being done by constructing drainage canals to lower the groundwater table, which allows peatland conversion to various land uses such as agriculture, plantation, forestry, and mining (Jaenicke et al., 2010; Rydin et al., 2013). However, the depletion of the groundwater leads to peat oxidation, consolidation, and shrinkage resulting in peat subsidence, carbon emissions, and increased fire hazards, all of which exacerbate climate change (Hooijer et al., 2012; Dohong et al., 2017).
The peatland drainage issues are closely tied to climate change consequences, which is a major environmental phenomenon (Prévost et al., 1999; Waddington and Price, 2000; Holden et al., 2004; Joosten, 2009; Hooijer et al., 2012). Therefore, there is no doubt that RDP research has been focused on how drainage affects climate change, water chemistry, and hydrology, serving as the justification for the RDP according to the keywords seen in cluster 1. In cluster one, drainage has the highest keyword occurrence and strong links to climate change and other keywords, which implies that drainage significantly impacts climate change.
Notwithstanding the less coverage of peatland worldwide, it plays a significant role in mediating fluxes of carbon dioxide (CO2), methane (CH4) and nitrous oxide (N2O) between soil and the atmosphere (Martikainen et al., 1993). Peatland drainage stimulates soil aeration and oxygen concentrations, facilitating the growth and activity of aerobic microbes resulting in soil organic carbon decomposition, and thereby reversing the carbon flux into a net CO2 emission into the atmosphere (Nykänen et al., 1998; Blodau, 2002; Smith, & Conen, 2004; Ojanen et al., 2013). The aeration created by the drainage stimulates the nitrification process, increasing the emission of N2O, especially in the minerotrophic peatlands (Aerts & Ludwig 1997; Gao et al., 2014; Hribljan et al., 2014; Wang et al., 2017; Liimatainen et al., 2018). Mikaloff Fletcher and Schaefer (2019) predicted that the emission effects from drained peatlands in 2020–2100 entail 12%–41% of the remaining GHG emission budget. Also, runoff from drained peat through the drainage channels has known to cause degradation of water catchments and their downstream quality (Skaggs et al., 1994; Prévost et al., 1999; Piirainen et al., 2013; Kaila et al., 2016; Nieminen et al., 2017a; Nieminen et al., 2017b). Peatland drainage results in higher DOC concentrations in surface and pore water and increases DOC export into nearby streams (Strack et al., 2008). Marttila et al. (2018) observed high concentrations of nutrients and suspended sediment, mainly dissolved nitrogen and phosphorus, were found in headwater streams in catchments containing drained peatland, which agrees with similar studies reported by Mattsson et al. (2003) and Nieminen et al. (2017b).
In addition, the biogeochemical properties of peatland that have been affected by drainage are organic matter decomposition and fractions, soil respiration, soil enzymes, elemental concentration, microbial degradation, peat humification, and so on (Moore and Basiliko, 2006; Olde Venterink et al., 2009; Macrae et al., 2013; Hulatt et al., 2014; Brown et al., 2015; Krüger et al., 2015; Harris et al., 2020; Xu et al., 2021). In addition, studies on the impact of peatland drainage on the vegetation cover have been conducted by Ramchunder et al. (2009) and Haapalehto et al. (2011). For example, Stewart & Lance (1991) reported a complete disappearance of Sphagnum capillifolium on 20 years of drained raised bog. Wilson et al. (2010) studied the effect of drainage on the plant species abundance within a Welsh blanket peatland. They reported a less abundance of peat-forming plant species, specifically Eriophorum angustifolium.
3.2.1.2 Cluster #2
The second cluster (red portion of Figure 3) focuses on the impact of peatland restoration on GHG emissions, specifically N2O, CO2, and CH4 emissions. Despite peatlands covering about 3% of the Earth’s surface, they store ∼644 Gt of C and 8–15 Gt N of the terrestrial biosphere (Turetsky et al., 2015; Leifeld and Menichetti, 2018; Ahmad et al., 2020). Currently, drainage accounts for ∼10% of global peatlands degradation, transforming them from a net sink to a net source of GHG (Knox et al., 2015; Leifeld and Menichetti, 2018).
The consequences of drainage to stimulate GHG emissions into the atmosphere facilitated the need for peatland protection and restoration as proximate mitigation measures (Wilson et al., 2015). Dohong et al. (2018) review the techniques for effective tropical peatland restoration. According to Dohong et al. (2018), hydrological restoration through peatland rewetting has effectively reduced GHG emissions from degraded peatland. According to Strack and Zuback (2013), rewetting of degraded peatland reduced CO2 emission through the reduction of peat mineralization, as well as negligible N2O emissions as a result of lowering the availability of mineral nitrogen in saturated conditions.
The hydrological restoration of degraded peatland involves a process that is known as rewetting/reflooding (O‘Brien et al., 2007). Rewetting is the process whereby deliberately introduced ditches or gullies are blocked to reduce the surface runoff outflow or allow water levels within the peat to return to their natural state (Ahmad et al., 2020). The significance of peatland rewetting is to enhance the peat’s hydrological properties by raising the groundwater table so that the hydrological properties of the drained peatland are recovered and stabilized as close as possible to its pre-logging and pre-drainage hydrological conditions (Page et al., 2009a; Panda et al., 2012).
Several studies observed that rewetting of drained peatland stimulates CO2-C sink functions immediately (Tuittila et al., 1999; Zeng & Gao, 2016; Cui et al., 2017). This happens because rewetting reduces the diffusive oxygen supply in the peatland, thereby creating an anaerobic environment, which in turn inhibits the organic matter and the litter decomposition, resulting in soil organic matter accumulation and the formation of peat layer (partially decomposed organic matter) (Herbst et al., 2011; Kim et al., 2011). However, the rewetting of degraded peatland stimulates the CH4 gases because the resulting anoxic conditions created by the rewetting are conducive to methanogenesis. Vanselow-Algan et al. (2015), Hahn et al. (2015) reported a methane emission of 148 g CH4 m−2 yr−1 and 260 g CH4 m−2 yr−1, respectively exceeding the default IPCC emission factor for rewetted drained peatland in the temperate region (29 g CH4 m−2 yr−1). Kandel et al. (2019) observed an increase in CH4 emission after 12 years of rewetting compared to the undrained site. They attributed their findings to the decomposition of above-ground biomass under inundated soil surface conditions.
Therefore, restoration management intended to reduce CO2 and CH4 emissions should prevent on-site above-ground biomass deposition combined with site inundation (Kandel et al., 2019). N2O emission reduction from rewetted peatlands is due to the raising of the groundwater table, thereby inhibiting nitrification and the denitrification process (Oktarita et al., 2017; Pärn et al., 2018). However, the resulting GHG fluxes from rewetted peatland may differ due to temperature, vegetation cover, growing season length, microbial community composition, biogeochemistry, previous land-use history, prevailing biomass species, and site (Renou-Wilson et al., 2014; Wrage-Mönnig et al., 2018).
3.2.1.3 Cluster #3
Cluster 3 (blue) is associated with peatland restoration and biogeochemical properties, specifically DOC. The keywords that are found in cluster 3 are phosphorus, nitrogen, carbon, water quality, water table, decomposition, dissolved organic carbon, and drain blocking. Degraded peatland is known to have high nutrient content because the lower water tables of the drained peatland expose deeper peat to oxic conditions, resulting in the high decomposition of the organic matter/peat layers and nutrient mineralization in the peat matrix. The drained peatlands contribute to the loss of carbon through the aquatic fluxes of carbon (e.g., DOC) into downstream water bodies, as reported in Limpens et al. (2008).
Restoration of the degraded peatland will result in the inhibitory effects of low nutrient concentration and DOC transport. Hence, making RDP research target DOC due to its consequences (D’Andrilli et al., 2010; Tfaily et al., 2013). DOC transport from degraded peatland to nearby catchment causes organic and inorganic micropollutants, and enhances bacterial regrowth within water distribution systems (Holden, 2005; Gough et al., 2016). Haapalehto et al. (2014) reported long-term decreases in DOC and nutrient leaching but temporary increases in N and P for the first 5 years of degraded peatland restoration. In addition, major nutrient such as phosphorus and nitrogen content has been extensively studied in a restored peatland (Moore et al., 2005; Nieminen et al., 2017a; Munir et al., 2017; Salmon et al., 2021).
3.2.1.4 Cluster #4
Cluster 4, represented in green, is related to peatland restoration and species richness, having keywords such as biodiversity, conservations, sphagnum, vegetation, plant, and so on. Since co-occurrence keywords are essential in reflecting and defining research contents, cluster 4, as seen in Figure 3, revealed degraded peatland restoration on the vegetation development. Peatland vegetation supports numerous invertebrates, birds, and bryophytes (Warner and Asada, 2006). Therefore, vegetation development on drained peatland has been an indicator to measure the success of peatland restoration (Richert et al., 2000; Tuittila et al., 2000; Van Dijk et al., 2007) because the vegetation response plays a significant role in the carbon and GHG budget of a peatland (Peacock et al., 2013). The vegetation development on restored sites depends on the water table, plant propagules, temperature, and the nutrient status of the site (Campbell and Rochefort, 2003; Strack et al., 2014).
Blocking drained ditches in drained peatland is frequently used to increase the water table (WT) to enhance the establishment of peat-forming plant species. Some peat-forming plant species that have been reported to have re-established after restoring degraded peatland are Eriophorum vaginatum, Sphagnum cuspidatum, and Sphagnum auriculatum, Calluna vulgaris, Vaccinium myrtillus, Erica tetralix, and Empetrum nigrum (Komulainen et al., 1998; Lavoie et al., 2005b). Peacock et al. (2013) reported that ditch blocking increased plant species richness in restored peatland; whereas Eriophorum vaginatum and Eriophorum cuspidatum were the primary colonizing species, with additional colonization by Eriophorum angustifolium, algae, and other sphagnum species.
Table 2 shows the top 25 most active keywords in the RDP research domain based on the numerical statistics of the variations in the node sizes and total link strength (co-occurrence connection or links) of the keywords. Out of 2,578 all keywords, the top five (5) most active keywords used in the RDP research domain are restoration, vegetation, peatland, drainage, and dynamics. Furthermore, co-occurrence keywords analysis was performed using cite space to investigate the topics receiving significant attention in the RDP research domain (Figure 4).
Figure 4 shows the burst time of most co-occurring keywords or research hotspots in the RDP research domain with the strongest citation bursts analysis using CiteSpace from 1991 to 2021. Citation burst illustrates evidence of which keywords have frequently been cited within the literature of a research theme/area, specifically growing topics or topics associated with surges in citations (Chen et al., 2014). Using the WOS dataset, 68 keywords had citation bursts, as seen in Figure 4, illustrating the top 25 keywords with the strongest citation bursts. The blue lines in the figure denote the time interval, whereas the red lines represent the time interval during which a subject was found to have a burst, indicating a citation burst event. The citation burst ranges from 5.42 to 2.33. The top five keywords having the strongest citation burst were diversity (burst strength, 5.42; 2014–2016), peatland (burst strength, 3.79; 2013–2016), land use (burst strength, 3.63; 2015–2016), water (burst strength, 3.27; 2006–2012), and drain blocking (burst strength, 3.22; 2014–2018). Furthermore, soil respiration (burst strength, 2.93; 2019–2021) is the most current burst, representing the emerging trend within the RDP research domain.
3.2.2 Research outlet: Outlets direct citation analysis
Figure 5 displays the network of the landmark research outlets in the RDP, consisting of 190 links and a total link strength of 3,264. Three clusters were formed, with different colors (i.e., red, green, and yellow) assigned to differentiate between journal clusters. Cluster 1 (blue) entails research outlets publishing articles focusing on the hydrology of peatland in RDP research. The research outlets found in cluster 1 are Science of the Total Environment, Hydrological Processes, Journal of Hydrology and Water Resources Research and so on. Cluster 2 (green) comprises research outlets Restoration Ecology, Biological Conversation, Applied Vegetation Science, Ecology and Evolution, and so on, which focus on vegetation and ecology RDP research. Cluster 3 (red) is composed of Mires and Peat, Wetlands Ecology and Management, Ecosystem, Ecological Engineering, Biogeoscience, and so on.
The top 20 research outlets with their quantitative measurement are presented in Table 3, with Mires and Peat recording the highest number of articles (40) in the RDP research domain. Mires and Peat is a peer-reviewed internet journal that publishes articles on mires, peatlands and peat-related research. It is published jointly by the International Peatland Society (IPS) and the International Mire Conservation Group (IMCG) (http://mires-and-peat.net/).
3.2.3 Document citation networks in RDP research
The resultant network of the document citation network analysis of RDP research comprises 101 links and 187.50 total links as displayed in Figure 6. From Figure 6, the top three cited articles include Leifeld and Menichetti (2018) “The underappreciated potential of peatlands in global climate change mitigation strategies.” This article reveals that restoration of drained peatland drastically reduces the current annual GHG emission, which is 3.4 times less nitrogen costly, involving a much smaller land area demand than improving mineral soil carbon sequestration for mitigation of GHG emissions peat coverage areas. A review article titled “Hydrological processes in abandoned and restored peatlands: An overview of management approaches” which was written by Price et al. (2003), obtained a citation of 183, making them the second most cited article in the RDP research domain. Price et al. (2003) revealed that hydrological management increases hydrological functions, such as hydraulic conductivity, water retention capacity, vegetation development, and the specific yield of abandoned land peatland. Finally, the third most cited article was “Greenhouse gas balances of managed peatlands in the Nordic countries: Present knowledge and gaps” which was conducted by Maljanen et al. (2010). Another observation made from Table 4 suggests that the top three influential documents based on the citation index in the RDP research domain were literature review articles. A different trend was observed when evaluating the links among the top 13 articles. It was found that 22, 60, 80 and 60 of the 522 articles have cited Price et al. (2003), Maljanen et al. (2010)Leified et al. (2012), Knox et al. (2015), respectively. By analyzing the impact of rewetting on drained peatland, Komulainen et al. (1999) found that 2 years of rewetting stimulated carbon sequestration such that rewetted minerotrophic fen obtained CO2-C balance ranged from 162 to 283 g m−2, with dense E. vaginatum vegetation and a high-water table, while rewetted bog had CO2-C balance varied from 54 to 101 g m−2 having a high-water table and mire vegetation. Wuni et al. (2019) emphasized that the influence of articles can be assessed by their total citations, normalized citations, and links with other articles. Therefore, using the total citation approach, Table 4 summarizes the top 13 most influential research articles, with key findings, links, and citations.
3.3 Co-authorship and active countries in the RDP research
3.3.1 Co-authorship analysis
Scientific collaboration between researchers and institutions enhances knowledge exchange, innovation, and joint funding application (Hosseini et al., 2018). Only two clusters of productive and collaborative researchers were revealed, consisting of 105 links and a total link of 2,136, as illustrated in Supplementary Figure S1. Researchers such as Baird, Andy J., Peacock, Mike, Green, Sophie M., and Evans, Chris D. are found in cluster one (red). Cluster two (red) includes researchers such as Holden, Joseph and Evans, Martin G. Researchers such as Evans, Martin G and Holden, Joseph tend to collaborate more often in the RDP research (Evans et al., 1999; Holden et al., 2006). Supplementary Figure S1 suggests that Holden, Joseph; Kotiaho, Janne S., Vasander, Harri and Tahvanainen, Teemu are the top collaboratives researchers within the RDP research discourse. Using the citation index to measure the productivity of the top collaborative co-authors, Vasander, Harri and Holden, Joseph emerge as the top-cited authors within the RDP research domain, as seen in Supplementary Table S1.
3.3.2 Active countries in the RDP research
Supplementary Figure S2 illustrates the most active countries in the RDP research discourse, with larger nodes indicating a high number of publications. Cluster one (blue) involves the countries of the United Kingdom, especially England, Scotland, and Wales, with England possessing the higher nodes. Cluster two (green) consists of Germany, Netherland, Poland, and Sweden, with Germany obtaining the higher nodes. The higher node obtained by Germany is not surprising because they have degraded more than 85% of its original peatlands, and hence restoration research options are now implemented to restore the degraded peatland to its original state. Cluster three (red) consists of five countries, with the highest nodes obtained by Finland. Based on this analysis, it can be revealed that about 26% (51 out of 195) of all countries are involved in RDP research. Supplementary Figure S2 reveals that RDP is conducted in countries with extensive peatland coverage. Regarding the strength of links, the strongest links were between the following pairs: England-Wales; England-Scotland; England-Finland; Germany-USA; and Canada-USA.
Table 5 shows the top 12 influential countries based on the number of articles, with England (96) obtaining the higher number of articles. The top three countries are England (96), Germany (88), and Finland (68). However, an inconsistent pattern is observed when ranking based on citations. Under this, Finland (2053), England (2005), and Germany (1,498) are the top influential countries in the RDP research domain. A slightly different ranking pattern is observed when using the average citation index, such that Sweden (37), Finland (30) and Canada (28) are the influential countries in the research domain.
4 Conclusion
This study reconnoiters the status and the global trends of RDP research using scientometric analysis. RDP research has tremendously increased in the last decade, resulting in numerous literature reviews being published. Nevertheless, this current study presents the first scientometric review of RDP research using 522 documents (research articles and literature review) retrieved from WOS database. The structure of the body of knowledge in RDP research was evaluated using the research outlet, keywords co-occurrence analysis, and document citation analysis. Specifically, the scientometric analysis of the RDP dataset discovered the following conclusions:
• Annual exponential growth in the number of publications in RDP research was observed.
• Four research themes were identified with the co-occurrence of keywords analysis, namely: (i) impact of drainage on peatland ecosystem services; (ii) impact of peatland restoration on GHG emissions; (iii) peatland restoration and biogeochemical properties; and (iv) peatland restoration and plant species richness.
• The top three keywords in RDP research having the strongest citation burst were diversity (burst strength, 5.42; 2014–2016), peatland (burst strength, 3.79; 2010–2013), and land use (burst strength, 3.27; 2014–2018).
• The most contributing research outlets of RDP research are Mires and Peat and the Science of the Total Environment.
• England and Germany are the most active countries in RDP research.
• It was found that 22, 60, 80, and 60 of the 522 articles have cited Price et al. (2003), Maljanen et al. (2010), Leified et al. (2012), Knox et al. (2015), respectively.
The current scientometric analysis is limited to certain keywords, TS (topic) = (‘drained peatland restoration’ OR ‘drained bog restoration’ OR ‘drained mire restoration’ OR degraded peatland restoration’ OR ‘degraded bog restoration’ OR ‘drained peatland reclamation’ OR ‘drained bog restoration’ OR ‘degraded peatland reclamation’ OR ‘degraded bog reclamation’ OR ‘drained mire restoration’ OR ‘degraded mire reclamation’ OR ‘degraded fen restoration’ OR ‘drained fen reclamation’) and the selected documents were limited to review and research articles only. In addition, only one database (WOS) was used for the extraction of the dataset, which may suffer from the intrinsic limitations of WOS’s coverage of publications. Due to these, this study might not fully capture the whole available literature on RDP. However, scientometric analysis of RDP research provides instinctive graphics for a research hotspot in RDP, and valuable information based on a comprehensive analysis of the research theme and future trends (Liu et al., 2019).
Author contributions
Conceptualization, SA; Methodology, SA; Software, SA; Validation, SA; Formal analysis, SA; Investigation, DM; Resources, DM and FT; Data curation, SA; Writing—original draft preparation, SA; Writing—review and editing, MG, FT, and DM; Visualization, SA; Supervision, MG, FT, and DM; Project administration, MG, FT, and DM; Funding acquisition, FT. All authors have read and agreed to the published version of the manuscript.
Funding
This research is supported by the Carbon Farming and Group Project Design for Peatlands under Agricultural Management project. The project is funded by European Innovation Partnerships from The Department of Agriculture, Food and the Marine (DAFM).
Conflict of interest
Authors DM and MG were employed by the company Green Restoration Ireland Cooperative Society Ltd.
The remaining authors declare that the research was conducted in the absence of any commercial or financial relationships that could be construed as a potential conflict of interest.
Publisher’s note
All claims expressed in this article are solely those of the authors and do not necessarily represent those of their affiliated organizations, or those of the publisher, the editors and the reviewers. Any product that may be evaluated in this article, or claim that may be made by its manufacturer, is not guaranteed or endorsed by the publisher.
Supplementary material
The Supplementary Material for this article can be found online at: https://www.frontiersin.org/articles/10.3389/fenvs.2022.942788/full#supplementary-material
SUPPLEMENTARY FIGURE S1 | Density Map of Co-Authorship Network.
SUPPLEMENTARY FIGURE S2 | Network of most contributing countries in the RDP research.
References
Adesiji, A. R., Mohammed, T. A., Nik Daud, N. N., Saari, M., Gbadebo, A. O., and Jacdonmi, I. (2015). Impacts of land-use change on peatland degradation: a review. Ethiop. J. Environ. Stud. Manag. 8, 225. doi:10.4314/ejesm.v8i2.11
Aerts, R., and Ludwig, F. (1997). Water-table changes and nutritional status affect trace gas emissions from laboratory columns of peatland soils. Soil Biol. Biochem. 29, 1691–1698. doi:10.1016/s0038-0717(97)00074-6
Ahmad, S., Liu, H., Günther, A., Couwenberg, J., and Lennartz, B. (2020). Long-term rewetting of degraded peatlands restores hydrological buffer function. Sci. Total Environ. 749, 141571. doi:10.1016/j.scitotenv.2020.141571
Andersen, R., Farrell, C., Graf, M., Muller, F., Calvar, E., Frankard, P., et al. (2017). An overview of the progress and challenges of peatland restoration in Western Europe. Restor. Ecol. 25 (2), 271–282. doi:10.1111/rec.12415
Anshari, G. Z., Afifudin, M., Nuriman, M., Gusmayanti, E., Arianie, L., Susana, R., et al. (2010). Drainage and land use impacts on changes in selected peat properties and peat degradation in West Kalimantan Province, Indonesia. Biogeosciences 7 (3), 3403–3419. doi:10.5194/bg-7-3403-2010
Baker, H. K., Kumar, S., and Pattnaik, D. (2021). Twenty-five years of the journal of corporate finance: A scientometric analysis. J. Corp. Finance 66, 101572. doi:10.1016/j.jcorpfin.2020.101572
Ballhorn, U., Siegert, F., Mason, M., and Limin, S. (2009). Derivation of burn scar depths and estimation of carbon emissions with LIDAR in Indonesian peatlands. Proc. Natl. Acad. Sci. U. S. A. 106 (50), 21213–21218. doi:10.1073/pnas.0906457106
Bhiry, N., Payette, S., and Robert, É. C. (2007). Peatland development at the arctic tree line (Québec, Canada) influenced by flooding and permafrost. Quat. Res. 67 (3), 426–437. doi:10.1016/j.yqres.2006.11.009
Biancalani, R., and Avagyan, A. (2014). Towards climate-responsible peatlands management. Mitigation of climate change in agriculture series (MICCA). Rome, Italy: Food and Agriculture Organization of the United Nations, 9.
Blodau, C. (2002). Carbon cycling in peatlands A review of processes and controls. Environ. Rev. 10 (2), 111–134. doi:10.1139/a02-004
Bonn, A., Reed, M. S., Evans, C. D., Joosten, H., Bain, C., Farmer, J., et al. (2014). Investing in nature: Developing ecosystem service markets for peatland restoration. Ecosyst. Serv. 9, 54–65. doi:10.1016/j.ecoser.2014.06.011
Bonn, A., Allott, T., Evans, M., Joosten, H., and Stoneman, R. (2016). “Peatland restoration and ecosystem services: an introduction,” in Peatland restoration and ecosystem services: Science, policy and practice. Cambridge, UK: Cambridge University Press, 1–16.
Bridgham, S. D., Pastor, J., Updegraff, K., Malterer, T. J., Johnson, K., Harth, C., et al. (1999). Ecosystem control over temperature and energy flux in northern peatlands. Ecol. Appl. 9 (4), 1345–1358. doi:10.1890/1051-0761(1999)009[1345:ecotae]2.0.co;2
Brooks, T. M., Mittermeier, R. A., da Fonseca, G. A., Gerlach, J., Hoffmann, M., Lamoreux, J. F., et al. (2006). Global biodiversity conservation priorities. science 313, 58–61. doi:10.1126/science.1127609
Brown, L. E., Holden, J., Palmer, S. M., Johnston, K., Ramchunder, S. J., and Grayson, R. (2015). Effects of fire on the hydrology, biogeochemistry, and ecology of peatland river systems. Freshw. Sci. 34 (4), 1406–1425. doi:10.1086/683426
Bubier, J. L., Moore, T. R., Bellisario, L., Comer, N. T., and Crill, P. M. (1995). Ecological controls on methane emissions from a northern peatland complex in the zone of discontinuous permafrost, Manitoba, Canada. Glob. Biogeochem. Cycles 9 (4), 455–470. doi:10.1029/95gb02379
Burton, R. G. O., and Hodgson, J. M. (1987). “Lowland peat in England and Wales,” in Soil survey technical monograph No.15 (UK: Harpenden), 146.
Bussell, J., Jones, D., Healey, J., and Pullin, A. (2010). How do draining and re-wetting affect carbon stores and greenhouse gas fluxes in peatland soils. CEE Rev. 08-012.
Campbell, D. R., Rochefort, L., and Lavoie, C. (2003). Determining the immigration potential of plants colonizing disturbed environments: The case of milled peatlands in Quebec. J. Appl. Ecol. 40 (1), 78–91. doi:10.1046/j.1365-2664.2003.00782.x
Chapman, S., Artz, R., and Donnelly, D. (2012). Carbon savings from peat restoration. Clim. Change Enquiry (1205–02), 1–17.
Chen, C. (2006). CiteSpace II: Detecting and visualizing emerging trends and transient patterns in scientific literature. J. Am. Soc. Inf. Sci. Technol. 57 (3), 359–377. doi:10.1002/asi.20317
Chen, H., Yang, G., Peng, C., Zhang, Y., Zhu, D., Zhu, Q., et al. (2014). The carbon stock of alpine peatlands on the Qinghai–Tibetan Plateau during the Holocene and their future fate. Quater. Sci. Rev. 95, 151–158. doi:10.1016/j.quascirev.2014.05.003
Chen, K., Wang, J., Yu, B., Wu, H., and Zhang, J. (2021). Critical evaluation of construction and demolition waste and associated environmental impacts: A scientometric analysis. J. Clean. Prod. 287, 125071. doi:10.1016/j.jclepro.2020.125071
Chimner, R. A., Cooper, D. J., Wurster, F. C., and Rochefort, L. (2017). An overview of peatland restoration in north America: Where are we after 25 years? Restor. Ecol. 25 (2), 283–292. doi:10.1111/rec.12434
Cobo, M. J., López‐Herrera, A. G., Herrera‐Viedma, E., and Herrera, F. (2011). Science mapping software tools: Review, analysis, and cooperative study among tools. J. Am. Soc. Inf. Sci. Technol. 62 (7), 1382–1402. doi:10.1002/asi.21525
Couwenberg, J., Thiele, A., Tanneberger, F., Augustin, J., Bärisch, S., Dubovik, D., et al. (2011). Assessing greenhouse gas emissions from peatlands using vegetation as a proxy. Hydrobiologia 674 (1), 67–89. doi:10.1007/s10750-011-0729-x
Crum, H., and Planisek, S. (1992). A focus on peatlands and peat mosses. Ann Arbor, MI: University of Michigan Press.
Cui, L., Kang, X., Li, W., Hao, Y., Zhang, Y., Wang, J., et al. (2017). Rewetting decreases carbon emissions from the Zoige alpine peatland on the Tibetan Plateau. Sustainability 9 (6), 948. doi:10.3390/su9060948
D’Andrilli, J., Chanton, J. P., Glaser, P. H., and Cooper, W. T. (2010). Characterization of dissolved organic matter in northern peatland soil porewaters by ultra high resolution mass spectrometry. Org. Geochem. 41 (8), 791–799. doi:10.1016/j.orggeochem.2010.05.009
Darko, A., Chan, A. P., Adabre, M. A., Edwards, D. J., Hosseini, M. R., and Ameyaw, E. E. (2020). Artificial intelligence in the AEC industry: Scientometric analysis and visualization of research activities. Automation Constr. 112, 103081. doi:10.1016/j.autcon.2020.103081
Darko, A., Chan, A. P., Huo, X., and Owusu-Manu, D. G. (2019). A scientometric analysis and visualization of global green building research. Build. Environ. 149, 501–511. doi:10.1016/j.buildenv.2018.12.059
Dise, N. B. (2009). Peatland response to global change. Science 326 (5954), 810–811. doi:10.1126/science.1174268
Dohong, A., Aziz, A. A., and Dargusch, P. (2017). A review of the drivers of tropical peatland degradation in South-East Asia. Land use policy 69, 349–360. doi:10.1016/j.landusepol.2017.09.035
Dohong, A., Abdul Aziz, A., and Dargusch, P. (2018). A review of techniques for effective tropical peatland restoration. Wetlands 38 (2), 275–292. doi:10.1007/s13157-018-1017-6
Elisha, I. L., and Viljoen, A. (2021). Trends in rooibos tea (aspalathus linearis) research (1994–2018): A scientometric assessment. South Afr. J. Bot. 137, 159–170. doi:10.1016/j.sajb.2020.10.004
Evans, M. G., Burt, T. P., Holden, J., and Adamson, J. K. (1999). Runoff generation and water table fluctuations in blanket peat: Evidence from UK data spanning the dry summer of 1995. J. Hydrology 221 (3-4), 141–160. doi:10.1016/s0022-1694(99)00085-2
Fenner, N., and Freeman, C. (2011). Drought-induced carbon loss in peatlands. Nat. Geosci. 4, 895–900. doi:10.1038/ngeo1323
Flores, R. M. (2014). Origin of coal as gas source and reservoir rocks. Coal and coalbed gas. Amsterdam: Elsevier, 97–165.
Flores-Moreno, H., Reich, P. B., Lind, E. M., Sullivan, L. L., Seabloom, E. W., Yahdjian, L., et al. (2016). Climate modifies response of non-native and native species richness to nutrient enrichment. Phil. Trans. R. Soc. B 371 (1694), 20150273. doi:10.1098/rstb.2015.0273
Fridell, M., Edwin, S., Von Schreeb, J., and Saulnier, D. D. (2020). Health system resilience: What are we talking about? A scoping review mapping characteristics and keywords. Int. J. Health Policy Manag. 9 (1), 6–16. doi:10.15171/ijhpm.2019.71
Gao, Y., Chen, H., and Zeng, X. (2014). Effects of nitrogen and sulfur deposition on CH4 and N2O fluxes in high-altitude peatland soil under different water tables in the Tibetan Plateau. Soil Sci. Plant Nutrit. 60 (3), 404–410. doi:10.1080/00380768.2014.893812
Gough, R., Holliman, P. J., Fenner, N., Peacock, M., and Freeman, C. (2016). Influence of water table depth on pore water chemistry and trihalomethane formation potential in peatlands. water Environ. Res. 88 (2), 107–117. doi:10.2175/106143015x14362865227878
Graniero, P. A., and Price, J. S. (1999). The importance of topographic factors on the distribution of bog and heath in a Newfoundland blanket bog complex. Catena 36 (3), 233–254. doi:10.1016/s0341-8162(99)00008-9
Guidry, J. A., Guidry Hollier, B. N., Johnson, L., Tanner, J. R., and Veltsos, C. (2004). Surveying the cites: A ranking of marketing journals using citation analysis. Mark. Educ. Rev. 14 (1), 45–59. doi:10.1080/10528008.2004.11488853
Haapalehto, T., Kotiaho, J. S., Matilainen, R., and Tahvanainen, T. (2014). The effects of long-term drainage and subsequent Restoration on water table level and pore water chemistry in boreal peatlands. J. Hydrology 519, 1493–1505. doi:10.1016/j.jhydrol.2014.09.013
Haapalehto, T. O., Vasander, H., Jauhiainen, S., Tahvanainen, T., and Kotiaho, J. S. (2011). The effects of peatland restoration on water‐table depth, elemental concentrations, and vegetation: 10 years of changes. Restor. Ecol. 19 (5), 587–598. doi:10.1111/j.1526-100x.2010.00704.x
Hahn, J., Köhler, S., Glatzel, S., and Jurasinski, G. (2015). Methane exchange in a coastal fen in the first year after flooding-a systems shift. PloS one 10 (10), e0140657. doi:10.1371/journal.pone.0140657
Hammersley, M. (2001). On ‘systematic’reviews of research literatures: A ‘narrative’response to Evans & benefield. Br. Educ. Res. J. 27 (5), 543–554. doi:10.1080/01411920120095726
Harris, L. I., Moore, T. R., Roulet, N. T., and Pinsonneault, A. J. (2020). Limited effect of drainage on peat properties, porewater chemistry, and peat decomposition proxies in a boreal peatland. Biogeochemistry 151 (1), 43–62. doi:10.1007/s10533-020-00707-1
Harrison, M. E., Ottay, J. B., D’Arcy, L. J., Cheyne, S. M., Belcher, C., Cole, L., et al. (2020). Tropical forest and peatland conservation in Indonesia: Challenges and directions. People Nat. 2 (1), 4–28. doi:10.1002/pan3.10060
Herbst, M., Friborg, T., Ringgaard, R., and Soegaard, H. (2011). Interpreting the variations in atmospheric methane fluxes observed above a restored wetland. Agric. For. Meteorology 151 (7), 841–853. doi:10.1016/j.agrformet.2011.02.002
Holden, J., Chapman, P. J., and Labadz, J. C. (2004). Artificial drainage of peatlands: Hydrological and hydrochemical process and wetland restoration. Prog. Phys. Geogr. Earth Environ. 28 (1), 95–123. doi:10.1191/0309133304pp403ra
Holden, J., Evans, M. G., Burt, T. P., and Horton, M. (2006). Impact of land drainage on peatland hydrology. J. Environ. Qual. 35 (5), 1764–1778. doi:10.2134/jeq2005.0477
Holden, J., Burt, T. P., and Cox, N. J. (2001). Macroporosity and infiltration in blanket peat: The implications of tension disc infiltrometer measurements. Hydrol. Process. 15 (2), 289–303. doi:10.1002/hyp.93
Holden, J. (2005). Peatland hydrology and carbon release: Why small-scale process matters. Phil. Trans. R. Soc. A 363 (1837), 2891–2913. doi:10.1098/rsta.2005.1671
Hooijer, A., Silvius, M., Wosten, H., and Page, E. S. (2006). PEAT-CO2: Assessment of CO2 emissions from drained peatlands in SE Asia. Delft Hydraulics Report Q3943/2006. (36p.).
Hooijer, A., Page, S., Jauhiainen, J., Lee, W. A., Lu, X. X., Idris, A., et al. (2012). Subsidence and carbon loss in drained tropical peatlands. Biogeosciences 9 (3), 1053–1071. doi:10.5194/bg-9-1053-2012
Hooijer, A., Page, S., Navratil, P., Vernimmen, R., van der Vat, M., Tansey, K., et al. (2014). Carbon emissions from drained and degraded peatland in Indonesia and emission factors for measurement, reporting and verification (MRV) of peatland greenhouse gas emissions–a summary of KFCP research results for practitioners. Jakarta, Indonesia: IAFCP.
Hosseini, M. R., Martek, I., Zavadskas, E. K., Aibinu, A. A., Arashpour, M., and Chileshe, N. (2018). Critical evaluation of off-site construction research: A scientometric analysis. Automation Constr. 87, 235–247. doi:10.1016/j.autcon.2017.12.002
Hribljan, J. A., Kane, E. S., Pypker, T. G., and Chimner, R. A. (2014). The effect of long‐term water table manipulations on dissolved organic carbon dynamics in a poor fen peatland. J. Geophys. Res. Biogeosci. 119, 577–595. doi:10.1002/2013jg002527
Hulatt, C. J., Kaartokallio, H., Asmala, E., Autio, R., Stedmon, C. A., Sonninen, E., et al. (2014). Bioavailability and radiocarbon age of fluvial dissolved organic matter (DOM) from a northern peatland-dominated catchment: Effect of land-use change. Aquat. Sci. 76 (3), 393–404. doi:10.1007/s00027-014-0342-y
Jaenicke, J., Wösten, H., Budiman, A., and Siegert, F. (2010). Planning hydrological restoration of peatlands in Indonesia to mitigate carbon dioxide emissions. Mitig. Adapt. Strateg. Glob. Chang. 15 (3), 223–239. doi:10.1007/s11027-010-9214-5
Jauhiainen, J., Limin, S., Silvennoinen, H., and Vasander, H. (2008). Carbon dioxide and methane fluxes in drained tropical peat before and after hydrological restoration. Ecology 89 (12), 3503–3514. doi:10.1890/07-2038.1
Joosten, H., and Clarke, D. (2002). Wise use of mires and peatlands. International Mire Conservation Group and International Peat Society, 304.
Joosten, H. (2009). The global peatland CO2 picture: Peatland status and drainage related emissions in all countries of the world.
Kaila, A., Asam, Z., Koskinen, M., Uusitalo, R., Smolander, A., Kiikkilä, O., et al. (2016). Impact of re-wetting of forestry-drained peatlands on water quality—A laboratory approach assessing the release of P, N, Fe, and dissolved organic carbon. Water Air Soil Pollut. 227 (8), 1–15. doi:10.1007/s11270-016-2994-9
Kandel, T. P., Lærke, P. E., Hoffmann, C. C., and Elsgaard, L. (2019). Complete annual CO2, CH4, and N2O balance of a temperate riparian wetland 12 years after rewetting. Ecol. Eng. 127, 527–535. doi:10.1016/j.ecoleng.2017.12.019
Kim, D. G., Vargas, R., Bond-Lamberty, B., and Turetsky, M. R. (2011). Effects of soil rewetting and thawing on soil gas fluxes: A review of current literature and suggestions for future research. Biogeosciences 8 (5), 2459–2483. doi:10.5194/bg-9-2459-2012
Kleinberg, J. (2002). An impossibility theorem for clustering. Adv. Neural Inform. Process. Syst. 15.
Knox, S. H., Sturtevant, C., Matthes, J. H., Koteen, L., Verfaillie, J., and Baldocchi, D. (2015). Agricultural peatland restoration: Effects of land‐use change on greenhouse gas (CO2 and CH4) fluxes in the sacramento‐san joaquin delta. Glob. Chang. Biol. 21 (2), 750–765. doi:10.1111/gcb.12745
Komulainen, V. M., Nykänen, H., Martikainen, P. J., and Laine, J. (1998). Short-term effect of Restoration on vegetation change and methane emissions from peatlands drained for forestry in southern Finland. Can. J. For. Res. 28 (3), 402–411. doi:10.1139/x98-011
Komulainen, V. M., Tuittila, E. S., Vasander, H., and Laine, J. (1999). Restoration of drained peatlands in southern Finland: Initial effects on vegetation change and CO2 balance. J. Appl. Ecol. 36 (5), 634–648. doi:10.1046/j.1365-2664.1999.00430.x
Könönen, M., Heinonsalo, J., Laiho, R., Kusin, K., Limin, S., and Vasander, H. (2018). Deforested and drained tropical peatland sites show poorer peat substrate quality and lower microbial biomass and activity than unmanaged swamp forest. Soil Biol. Biochem. 123, 229–241. doi:10.1016/j.soilbio.2018.04.028
Krüger, J. P., Leifeld, J., Glatzel, S., Szidat, S., and Alewell, C. (2015). Biogeochemical indicators of peatland degradation–a case study of a temperate bog in northern Germany. Biogeosciences 12 (10), 2861–2871. doi:10.5194/bg-12-2861-2015
Lamers, L. P., Van Diggelen, J. M., Op den Camp, H. J., Visser, E. J., Lucassen, E. C., Vile, M. A., et al. (2012). Microbial transformations of nitrogen, sulfur, and iron dictate vegetation composition in wetlands: A review. Front. Microbiol. 3, 156. doi:10.3389/fmicb.2012.00156
Lavoie, C., Marcoux, K., Saint-Louis, A., and Price, J. S. (2005a). The dynamics of a cotton-grass (Eriophorum vaginatum L.) cover expansion in a vacuum-mined peatland, southern Québec, Canada. Wetlands 25 (1), 64–75. doi:10.1672/0277-5212(2005)025[0064:tdoace]2.0.co;2
Lavoie, M., Paré, D., Fenton, N., Groot, A., and Taylor, K. (2005b). Paludification and management of forested peatlands in Canada: A literature review. Environ. Rev. 13 (2), 21–50. doi:10.1139/a05-006
Leifeld, J., and Menichetti, L. (2018). The underappreciated potential of peatlands in global climate change mitigation strategies. Nat. Commun. 9 (1), 1071–1077. doi:10.1038/s41467-018-03406-6
Leifeld, J., Steffens, M., and Galego‐Sala, A. (2012). Sensitivity of peatland carbon loss to organic matter quality. Geophys. Res. Lett. 39 (14). doi:10.1029/2012GL051856
Li, J., Goerlandt, F., and Reniers, G. (2021). An overview of scientometric mapping for the safety science community: Methods, tools, and framework. Saf. Sci. 134, 105093. doi:10.1016/j.ssci.2020.105093
Liimatainen, M., Voigt, C., Martikainen, P. J., Hytönen, J., Regina, K., Óskarsson, H., et al. (2018). Factors controlling nitrous oxide emissions from managed northern peat soils with low carbon to nitrogen ratio. Soil Biol. Biochem. 122, 186–195. doi:10.1016/j.soilbio.2018.04.006
Limpens, J., erendse, F., Blodau, C., Canadell, J. G., Freeman, C., Holden, J., et al. (2008). Peatlands and the carbon cycle: From local processes to global implications—a synthesis. Biogeosciences 5, 1475–1491. doi:10.5194/bg-5-1475-2008
Liu, Z., Lu, Y., and Peh, L. C. (2019). A review and scientometric analysis of global building information modeling (BIM) research in the architecture, engineering and construction (AEC) industry. Buildings 9 (10), 210. doi:10.3390/buildings9100210
Macrae, M. L., Devito, K. J., Strack, M., and Waddington, J. M. (2013). Effect of water table drawdown on peatland nutrient dynamics: Implications for climate change. Biogeochemistry 112 (1), 661–676. doi:10.1007/s10533-012-9730-3
Maftu’ah, E., Fahmi, A., and Hayati, A. (2019)). Changes in degraded peat land characteristic using FTIR-spectrocopy. IOP Conf. Ser. Earth Environ. Sci. 393, 012091. doi:10.1088/1755-1315/393/1/012091
Maljanen, M., Hytönen, J., and Martikainen, P. J. (2010). Cold-season nitrous oxide dynamics in a drained boreal peatland differ depending on land-use practice. Canadian J. Forest Res. 40 (3), 565–572.
Markoulli, M. P., Lee, C. I., Byington, E., and Felps, W. A. (2017). Mapping human resource management: Reviewing the field and charting future directions. Hum. Resour. Manag. Rev. 27 (3), 367–396. doi:10.1016/j.hrmr.2016.10.001
Martinez, S., del Mar Delgado, M., Marin, R. M., and Alvarez, S. (2019). Science mapping on the environmental footprint: A scientometric analysis-based review. Ecol. Indic. 106, 105543. doi:10.1016/j.ecolind.2019.105543
Martikainen, P. J., Nykänen, H., Crill, P., and Silvola, J. (1993). Effect of a lowered water table on nitrous oxide fluxes from northern peatlands. Nature 366 (6450), 51–53. doi:10.1038/366051a0
Martikainen, P. J., Nykänen, H., Alm, J., and Silvola, J. (1995). Change in fluxes of carbon dioxide, methane and nitrous oxide due to forest drainage of mire sites of different trophy. Plant Soil 168 (1), 571–577. doi:10.2307/42939899
Marttila, H., Karjalainen, S. M., Kuoppala, M., Nieminen, M. L., Ronkanen, A. K., Kløve, B., et al. (2018). Elevated nutrient concentrations in headwaters affected by drained peatland. Sci. Total Environ. 643, 1304–1313. doi:10.1016/j.scitotenv.2018.06.278
Matějková, Š., and Šimon, T. (2012). Application of FTIR spectroscopy for evaluation of hydrophobic/hydrophilic organic components in arable soil. Plant Soil Environ. 58 (4), 192–195. doi:10.17221/317/2011-pse
Mattsson, T., Finér, L., Kortelainen, P., and Sallantaus, T. (2003). Brook water quality and background leaching from unmanaged forested catchments in Finland. Water, Air, Soil Pollut. 147 (1), 275–298. doi:10.1023/a:1024525328220
Meissner, R., Rupp, H., and Leinweber, P. (2003). Re-wetting of fen soils and changes in water quality-experimental results and further research needs. J. Water Land Dev. 7, 75–91.
Miettinen, J., and Liew, S. C. (2010). Status of peatland degradation and development in Sumatra and Kalimantan. Ambio 39 (5), 394–401. doi:10.1007/s13280-010-0051-2
Mikaloff Fletcher, S. E., and Schaefer, H. (2019). Rising methane: A new climate challenge. Science 364, 932–933. doi:10.1126/science.aax1828
Miller, J., Anderson, H., Ray, D., and Anderson, A. (1996). Impact of some initial forestry practices on the drainage waters from blanket peatlands. Forestry 69 (3), 193–203. doi:10.1093/forestry/69.3.193
Minayeva, T. Y., and Sirin, A. A. (2012). Peatland biodiversity and climate change. Biol. Bull. Rev. 2 (2), 164–175. doi:10.1134/s207908641202003x
Minkkinen, K., and Laine, J. (1998). Effect of forest drainage on the peat bulk density of pine mires in Finland. Can. J. For. Res. 28, 178–186. doi:10.1139/x97-206
Mishra, S., Page, S. E., Cobb, A. R., Lee, J. S. H., Jovani‐Sancho, A. J., Sjögersten, S., et al. (2021). Degradation of Southeast Asian tropical peatlands and integrated strategies for their better management and restoration. J. Appl. Ecol. 58 (7), 1370–1387. doi:10.1111/1365-2664.13905
Moore, T., and Basiliko, N. (2006). “Decomposition in boreal peatlands,” in Boreal peatland ecosystems (Berlin, Heidelberg: Springer), 125–143.
Moore, T. R., Trofymow, J. A., Siltanen, M., Prescott, C., and Group, C. W. (2005). Patterns of decomposition and carbon, nitrogen, and phosphorus dynamics of litter in upland forest and peatland sites in central Canada. Can. J. For. Res. 35 (1), 133–142. doi:10.1139/x04-149
Munir, T. M., Khadka, B., Xu, B., and Strack, M. (2017). Mineral nitrogen and phosphorus pools affected by water table lowering and warming in a boreal forested peatland. Ecohydrology 10 (8), e1893. doi:10.1002/eco.1893
Nikolenko, S. I., Koltcov, S., and Koltsova, O. (2017). Topic modelling for qualitative studies. J. Inform. Sci. 43 (1), 88–102. doi:10.1177/0165551515617393
Nieminen, M., Sallantaus, T., Ukonmaanaho, L., Nieminen, T. M., and Sarkkola, S. (2017a). Nitrogen and phosphorus concentrations in discharge from drained peatland forests are increasing. Sci. Total Environ. 609, 974–981. doi:10.1016/j.scitotenv.2017.07.210
Nieminen, M., Sarkkola, S., and Laurén, A. (2017b). Impacts of forest harvesting on nutrient, sediment and dissolved organic carbon exports from drained peatlands: A literature review, synthesis and suggestions for the future. For. Ecol. Manag. 392, 13–20. doi:10.1016/j.foreco.2017.02.046
Nykänen, H., Alm, J., Silvola, J., Tolonen, K., and Martikainen, P. J. (1998). Methane fluxes on boreal peatlands of different fertility and the effect of long‐term experimental lowering of the water table on flux rates. Glob. Biogeochem. Cycles 12 (1), 53–69. doi:10.1029/97gb02732
O’Brien, H., Labadz, J. C., and Butcher, D. P. (2007). Review of blanket bog management and restoration. Nottingham Trent University research rep. (BD1241) to Defra. London.
Ojanen, P., Minkkinen, K., and Penttilä, T. (2013). The current greenhouse gas impact of forestry-drained boreal peatlands. For. Ecol. Manag. 289, 201–208. doi:10.1016/j.foreco.2012.10.008
Oktarita, S., Hergoualc’h, K., Anwar, S., and Verchot, L. V. (2017). Substantial N 2 O emissions from peat decomposition and N fertilization in an oil palm plantation exacerbated by hotspots. Environ. Res. Lett. 12 (10), 104007. doi:10.1088/1748-9326/aa80f1
Olde Venterink, H., Kardel, I., Kotowski, W., Peeters, W., and Wassen, M. J. (2009). Long-term effects of drainage and hay-removal on nutrient dynamics and limitation in the Biebrza mires, Poland. Biogeochemistry 93 (3), 235–252. doi:10.1007/s10533-009-9300-5
Oraee, M., Hosseini, M. R., Papadonikolaki, E., Palliyaguru, R., and Arashpour, M. (2017). Collaboration in BIM-based construction networks: A bibliometric-qualitative literature review. Int. J. Proj. Manag. 35 (7), 1288–1301. doi:10.1016/j.ijproman.2017.07.001
Parish, F., and Looi, C. C. (1999). “Wetlands, biodiversity and climate change,” in Options and needs for enhanced linkage between the ramsar convention on wetlands, convention on biological diversity andUN framework convention on climate change (Global Environment Network).
Parish, F., Sirin, A., Charman, D., Joosten, H., Minayeva, T., Silvius, M., et al. (2008). Assessment on peatlands, biodiversity and climate change: Main report. Wageningen: Global Environment Centre, Kuala Lumpur and Wetlands International.
Pärn, J., Verhoeven, J. T., Butterbach-Bahl, K., Dise, N. B., Ullah, S., Aasa, A., et al. (2018). Nitrogen-rich organic soils under warm well-drained conditions are global nitrous oxide emission hotspots. Nat. Commun. 9 (1), 1135–1138. doi:10.1038/s41467-018-03540-1
Peacock, M., Evans, C. D., Fenner, N., and Freeman, C. (2013). Natural revegetation of bog pools after peatland restoration involving ditch blocking—the influence of pool depth and implications for carbon cycling. Ecol. Eng. 57, 297–301. doi:10.1016/j.ecoleng.2013.04.055
Perianes-Rodriguez, A., Waltman, L., and Van Eck, N. J. (2016). Constructing bibliometric networks: A comparison between full and fractional counting. J. Inf. 10 (4), 1178–1195. doi:10.1016/j.joi.2016.10.006
Piirainen, S., Domisch, T., Moilanen, M., and Nieminen, M. (2013). Long-term effects of ash fertilization on runoff water quality from drained peatland forests. For. Ecol. Manag. 287, 53–66. doi:10.1016/j.foreco.2012.09.014
Poddubny, A. G., and Galat, D. L. (1995). Habitat associations of upper Volga River fishes: Effects of reservoirs. Regul. Rivers Res. Mgmt. 11 (1), 67–84. doi:10.1002/rrr.3450110107
Prévost, M., Plamondon, A. P., and Belleau, P. (1999). Effects of drainage of a forested peatland on water quality and quantity. J. Hydrology 214 (1-4), 130–143. doi:10.1016/s0022-1694(98)00281-9
Price, J. S. (1996). Hydrology and microclimate of a partly restored cutover bog. Québec. Hydrol. Proc. 10, 1263–1272. doi:10.1002/(SICI)1099-1085(199610)10:10<1263::AID-HYP458>3.0.CO;2–1.
Price, J. S., Heathwaite, A. L., and Baird, A. J. (2003). Hydrological processes in abandoned and restored peatlands: An overview of management approaches. Wetl. Ecol. Manag. 11 (1–2), 65–83. doi:10.1023/A:1022046409485
Ramchunder, S. J., Brown, L. E., and Holden, J. (2009). Environmental effects of drainage, drain-blocking and prescribed vegetation burning in UK upland peatlands. Prog. Phys. Geogr. Earth Environ. 33 (1), 49–79. doi:10.1177/0309133309105245
Ravikumar, S., Agrahari, A., and Singh, S. N. (2015). Mapping the intellectual structure of scientometrics: A co-word analysis of the journal scientometrics (2005–2010). Scientometrics 102 (1), 929–955. doi:10.1007/s11192-014-1402-8
Renou-Wilson, F., Barry, C., Müller, C., and Wilson, D. (2014). The impacts of drainage, nutrient status and management practice on the full carbon balance of grasslands on organic soils in a maritime temperate zone. Biogeosciences 11 (16), 4361–4379. doi:10.5194/bg-11-4361-2014
Richert, M., Dietrich, O., Koppisch, D., and Roth, S. (2000). The influence of rewetting on vegetation development and decomposition in a degraded fen. Restor. Ecol. 8 (2), 186–195. doi:10.1046/j.1526-100x.2000.80026.x
Rieley, J. O., Wüst, R. A. J., Jauhiainen, J., Page, S. E., Wösten, J. H. M., Hooijer, A., et al. (2008). “Tropical peatlands: Carbon stores, carbon gas emissions and contribution to climate change processes,” in Peatlands and climate change (Finland: International Peat Society), 148–181.
Rydin, H., Jeglum, J. K., and Bennett, K. D. (2013). The biology of peatlands, 2e. Oxford University Press.
Salmah, Z., Spoor, G., Zahari, A. B., and Welch, D. N. (1991). “Importance of water management in peat soil at farm level, level,” in Proc. Of the inter. Trop. Peatland, May 6–10, 1991, Kuching, Sarawak, Malaysia. Editor B. Y. Aminuddinet al. (MARDI-Dep. Agric.), 228–238.
Salmon, V. G., Brice, D. J., Bridgham, S., Childs, J., Graham, J., Griffiths, N. A., et al. (2021). Nitrogen and phosphorus cycling in an ombrotrophic peatland: A benchmark for assessing change. Plant Soil 466 (1), 649–674. doi:10.1007/s11104-021-05065-x
Şenel, E. (2019). Evolution of homeopathy: A scientometric analysis of global homeopathy literature between 1975 and 2017. Complementary Ther. Clin. Pract. 34, 165–173. doi:10.1016/j.ctcp.2018.11.018
Shrivastava, R., and Mahajan, P. (2016). Artificial intelligence research in India: A scientometric analysis. Sci. Technol. Libr. 35 (2), 136–151. doi:10.1080/0194262x.2016.1181023
Sirin, A., Minayeva, T., Vozbrannaya, A., and Bartalev, S. (2011). How to avoid peat fires? Sci. Russ. 2, 13–21.
Sjörs, H., and Gunnarsson, U. (2002). Calcium and pH in north and central Swedish mire waters. J. Ecol. 90, 650–657. doi:10.1046/j.1365-2745.2002.00701.x
Sjörs, H. (1980). Peat on earth: multiple use or conservation? Ambio 9 (6), 303–308. http://www.jstor.org/stable/4312610
Sjörs, H., and Sjors, H. (1950). On the relation between vegetation and electrolytes in north Swedish mire waters. Oikos 2 (2), 241–258. doi:10.2307/3564795
Skaggs, R. W., Breve, M. A., and Gilliam, J. W. (1994). Hydrologic and water quality impacts of agricultural drainage. Crit. Rev. Environ. Sci. Technol. 24 (1), 1–32. doi:10.1080/10643389409388459
Smith, K. A., and Conen, F. (2004). Impacts of land management on fluxes of trace greenhouse gases. Soil use Manag. 20 (2), 255–263. doi:10.1111/j.1475-2743.2004.tb00366.x
Spitzer, K., and Danks, H. V. (2006). Insect biodiversity of boreal peat bogs. Annu. Rev. Entomol. 51, 137–161. doi:10.1146/annurev.ento.51.110104.151036
Stewart, A. J., and Lance, A. N. (1991). Effects of moor-draining on the hydrology and vegetation of northern Pennine blanket bog. J. Appl. Ecol. 28, 1105–1117. doi:10.2307/2404228
Strack, M., Waddington, J. M., Bourbonniere, R. A., Buckton, E. L., Shaw, K., Whittington, P., et al. (2008). Effect of water table drawdown on peatland dissolved organic carbon export and dynamics. Hydrol. Process. 22 (17), 3373–3385. doi:10.1002/hyp.6931
Strack, M., and Zuback, Y. C. A. (2013). Annual carbon balance of a peatland 10 yr following restoration. Biogeosciences 10 (5), 2885–2896. doi:10.5194/bg-10-2885-2013
Strack, M., Keith, A. M., and Xu, B. (2014). Growing season carbon dioxide and methane exchange at a restored peatland on the Western Boreal Plain. Ecol. Eng. 64, 231–239. doi:10.1016/j.ecoleng.2013.12.013
Su, H. N., and Lee, P. C. (2010). Mapping knowledge structure by keyword co-occurrence: A first look at journal papers in technology foresight. Scientometrics 85 (1), 65–79. doi:10.1007/s11192-010-0259-8
Tanneberger, F., and Wichtmann, W. (2011). Carbon credits from peatland rewetting: Climate, biodiversity, land use. Stuttgart: Schweizerbart Science Publishers.
Tarnocai, C., and Stolbovoy, V. (2006). Northern peatlands: Their characteristics, development and sensitivity to climate change. Dev. earth Surf. Process. 9, 17–51. doi:10.1016/S0928-2025(06)09002-X
Tarnocai, C. (2006). The effect of climate change on carbon in Canadian peatlands. Glob. Planet. Change 53 (4), 222–232. doi:10.1016/j.gloplacha.2006.03.012
Tfaily, M. M., Hamdan, R., Corbett, J. E., Chanton, J. P., Glaser, P. H., and Cooper, W. T. (2013). Investigating dissolved organic matter decomposition in northern peatlands using complimentary analytical techniques. Geochimica Cosmochimica Acta 112, 116–129. doi:10.1016/j.gca.2013.03.002
Tiemeyer, B., Frings, J., Kahle, P., Köhne, S., and Lennartz, B. (2007). A comprehensive study of nutrient losses, soil properties and groundwater concentrations in a degraded peatland used as an intensive meadow - implications for re-wetting. J. Hydrol. X. 345, 80–101. doi:10.1016/j.jhydrol.2007.08.002
Tuittila, E. S., Komulainen, V. M., Vasander, H., and Laine, J. (1999). Restored cut-away peatland as a sink for atmospheric CO2. Oecologia 120 (4), 563–574. doi:10.1007/s004420050891
Tuittila, E. S., Vasander, H., and Laine, J. (2000). Impact of rewetting on the vegetation of a cut‐away peatland. Appl. Veg. Sci. 3 (2), 205–212. doi:10.2307/1478999
Turetsky, M. R., Benscoter, B., Page, S., Rein, G., Van Der Werf, G. R., and Watts, A. (2015). Global vulnerability of peatlands to fire and carbon loss. Nat. Geosci. 8 (1), 11–14. doi:10.1038/ngeo2325
Valat, B., Jouany, C., and Riviere, L. M. (1991). Characterization of the wetting properties of air-dried peats and composts. Soil Sci. 173 (5), 100–107. doi:10.1097/00010694-199108000-00006
Van Diggelen, R., Grootjans, A. P., Kemmers, R. H., Kooijman, A. M., Succow, M., De Vries, N. P. J., et al. (1991). Hydro‐ecological analysis of the fen system Lieper Posse, eastern Germany. J. Veg. Sci. 2 (4), 465–476. doi:10.2307/3236028
Van Dijk, J., Stroetenga, M., Van Bodegom, P. M., and Aerts, R. (2007). The contribution of rewetting to vegetation restoration of degraded peat meadows. Appl. Veg. Sci. 10 (3), 315–324. doi:10.1111/j.1654-109x.2007.tb00430.x
Van Eck, N. J., and Waltman, L. (2014). “Visualising bibliometric networks,” in Measuring scholarly impact (Cham: Springer), 285–320.
Van Eck, N. J., and Waltman, L. (2020). VOSviewer manual: Manual for VOSviewer version 1.6. 15. Leiden: Centre for Science and Technology Studies (CWTS) of Leiden University. https://www.vosviewer.com/documentation/Manual_VOSviewer_1.6.13.pdf
van Eck, N., Waltman, L., Noyons, E., and Buter, R. (2010). Automatic term identification for bibliometric mapping. Scientometrics 82 (3), 581–596. doi:10.1007/s11192-010-0173-0
Vanselow-Algan, M., Schmidt, S. R., Greven, M., Fiencke, C., Kutzbach, L., and Pfeiffer, E. M. (2015). High methane emissions dominated annual greenhouse gas balances 30 years after bog rewetting. Biogeosciences 12 (14), 4361–4371. doi:10.5194/bg-12-4361-2015
Vasander, H., Tuittila, E. S., Lode, E., Lundin, L., Ilomets, M., Sallantaus, T., et al. (2003). Status and restoration of peatlands in northern Europe. Wetl. Ecol. Manag. 11 (1), 51–63. doi:10.1023/a:1022061622602
Waddington, J. M., Strack, M., and Greenwood, M. J. (2010). Toward restoring the net carbon sink function of degraded peatlands: Short‐term response in CO2 exchange to ecosystem‐scale Restoration. J. Geophys. Res. 115 (G1), G01008. doi:10.1029/2009jg001090
Waddington, J. M., and Price, J. S. (2000). Effect of peatland drainage, harvesting, and Restoration on atmospheric water and carbon exchange. Phys. Geogr. 21 (5), 433–451. doi:10.1080/02723646.2000.10642719
Waddington, J. M., and Warner, K. (2001). Atmospheric CO2 sequestration in restored mined peatlands. Ecoscience 8 (3), 359–368. doi:10.1080/11956860.2001.11682664
Wallage, Z. E., Holden, J., and McDonald, A. T. (2006). Drain blocking: An effective treatment for reducing dissolved organic carbon loss and water discolouration in a drained peatland. Sci. total Environ. 367 (2-3), 811–821. doi:10.1016/j.scitotenv.2006.02.010
Wang, H., Yu, L., Zhang, Z., Liu, W., Chen, L., Cao, G., et al. (2017). Molecular mechanisms of water table lowering and nitrogen deposition in affecting greenhouse gas emissions from a Tibetan alpine wetland. Glob. Chang. Biol. 23, 815–829. doi:10.1111/gcb.13467
Warner, B. G., and Asada, T. (2006). Biological diversity of peatlands in Canada. Aquatic Sci. 68 (3), 240–253. doi:10.1007/s00027-006-0853-2
Williams, R., Runco, M. A., and Berlow, E. (2016). Mapping the themes, impact, and cohesion of creativity research over the last 25 years. Creativity Res. J. 28 (4), 385–394. doi:10.1080/10400419.2016.1230358
Wilson, D., Renou-Wilson, F., Farrell, C., Bullock, C., and Müller, C. (2012). “Carbon restore – The potential of Irish peatlands for carbon uptake and storage,” in Climate change research programme (Johnstown Castle, Co. Wexford, Ireland: prepared for the Environmental Protection Agency). Report Series No.17.
Wilson, J. D., Anderson, R., Bailey, S., Chetcuti, J., Cowie, N. R., Hancock, M. H., et al. (2014). Modelling edge effects of mature forest plantations on peatland waders informs landscape-scale conservation. J. Appl. Ecol. 51, 204–213. doi:10.1111/1365-2664.12173
Wilson, D., Dixon, S. D., Artz, R. R. E., Smith, T. E. L., Evans, C. D., Owen, H. J. F., et al. (2015). Derivation of greenhouse gas emission factors for peatlands managed for extraction in the Republic of Ireland and the United Kingdom. Biogeosciences 12 (18), 5291–5308. doi:10.5194/bg-12-5291-2015
Wilson, L., Wilson, J., Holden, J., Johnstone, I., Armstrong, A., and Morris, M. (2010). Recovery of water tables in Welsh blanket bog after drain blocking: discharge rates, time scales and the influence of local conditions. J. Hydrol. 391 (3–4), 377–386. doi:10.1016/j.jhydrol.2010.07.042
Wösten, J. H. M., Clymans, E., Page, S. E., Rieley, J. O., and Limin, S. H. (2008). Peat–water interrelationships in a tropical peatland ecosystem in Southeast Asia. Catena 73 (2), 212–224. doi:10.1016/j.catena.2007.07.010
Wrage-Mönnig, N., Horn, M. A., Well, R., Müller, C., Velthof, G., and Oenema, O. (2018). The role of nitrifier denitrification in the production of nitrous oxide revisited. Soil Biol. Biochem. 123, A3–A16. doi:10.1016/j.soilbio.2018.03.020
Wuni, I. Y., Shen, G. Q., and Osei-Kyei, R. (2019). Scientometric review of global research trends on green buildings in construction journals from 1992 to 2018. Energy Build. 190, 69–85. doi:10.1016/j.enbuild.2019.02.010
Xu, J., Morris, P. J., Liu, J., and Holden, J. (2018). PEATMAP: Refining estimates of global peatland distribution based on a meta-analysis. Catena 160, 134–140. doi:10.1016/j.catena.2017.09.010
Xu, Z., Wang, S., Wang, Z., Dong, Y., Zhang, Y., Liu, S., et al. (2021). Effect of drainage on microbial enzyme activities and communities dependent on depth in peatland soil. Biogeochemistry 155 (3), 323–341. doi:10.1007/s10533-021-00828-1
Yu, D., and Liao, H. (2016). Visualization and quantitative research on intuitionistic fuzzy studies. J. Intelligent Fuzzy Syst. 30 (6), 3653–3663. doi:10.3233/ifs-162111
Yuwati, T. W., Rachmanadi, D., Turjaman, M., Indrajaya, Y., Nugroho, H. Y. S. H., Qirom, M. A., et al. (2021). Restoration of degraded tropical peatland in Indonesia: A review. Land 10 (11), 1170. doi:10.3390/land10111170
Zeng, X., and Gao, Y. (2016). Short-term effects of drying and rewetting on CO2 and CH4 emissions from high-altitude peatlands on the Tibetan Plateau. Atmosphere 7 (11), 148. doi:10.3390/atmos7110148
Zhang, Y., Huang, K., Yu, Y., and Yang, B. (2017). Mapping of water footprint research: A bibliometric analysis during 2006–2015. J. Clean. Prod. 149, 70–79.
Zhang, B., Ahmad, W., Ahmad, A., Aslam, F., and Joyklad, P. (2021). A scientometric analysis approach to analyse the present research on recycled aggregate concrete. J. Build. Eng. 46, 103679. doi:10.1016/j.jobe.2021.103679
Keywords: peatland restoration, degraded peatland, scientometric, publication trend analysis, greenhouse gas, drainage
Citation: Apori SO, Mcmillan D, Giltrap M and Tian F (2022) Mapping the restoration of degraded peatland as a research area: A scientometric review. Front. Environ. Sci. 10:942788. doi: 10.3389/fenvs.2022.942788
Received: 13 May 2022; Accepted: 04 July 2022;
Published: 04 October 2022.
Edited by:
Chuanyu Gao, Northeast Institute of Geography and Agroecology (CAS), ChinaReviewed by:
Liang Yang, Northeast Institute of Geography and Agroecology (CAS), ChinaMiriam Groß-Schmölders, University of Basel, Switzerland
Copyright © 2022 Apori, Mcmillan, Giltrap and Tian. This is an open-access article distributed under the terms of the Creative Commons Attribution License (CC BY). The use, distribution or reproduction in other forums is permitted, provided the original author(s) and the copyright owner(s) are credited and that the original publication in this journal is cited, in accordance with accepted academic practice. No use, distribution or reproduction is permitted which does not comply with these terms.
*Correspondence: Samuel Obeng Apori, ZDIxMTI1MTkyQG15dHVkdWJsaW4uaWUmI3gwMjAwYTs=; Furong Tian, ZnVyb25nLnRpYW5AdHVkdWJsaW4uaWU=