- 1Water Quality and Citizen Science Laboratory, Department of Aquatic System, Faculty of Environmental Sciences, EULA Center, University of Concepcion, Chile, Concepcion, Chile
- 2Postgraduate Program, Department of Urban and Regional Planning and Geo-Information Management, Faculty of Geo-Information Science and Earth Observation ITC, University of Twente, Enschede, Netherlands
It is becoming increasingly clear that the threat to the use of natural landscapes and persistent droughts are affecting the water quality of rural communities. The socio-ecological fit emerges as an aid to advance in the search for solutions to environmental problems in rural communities. Consequently, between May 2016 and September 2021, an interdisciplinary study of community-based water quality monitoring (CWQM) was implemented at a coastal catchment in the Ñuble region, Central Chile. The objective of the study was to involve the participation of local communities in initiatives to control the water quality of various parameters [pH, total iron (FeT), alkalinity as CaCO3, hardness (Ca2+ and Mg2+), sodium (Na+) and potassium (K+), water temperature, sulfate (SO42−) chloride (Cl−), and nitrate (NO3-)] in groundwater, share local knowledge, and apply co-creative measures for improving water conservation. In situ chemical data were also analyzed in the laboratory to check data quality. The PHREEQC hydrogeochemical model is used to characterize hydrogeochemical parameters of groundwater and to know about hydrogeochemical processes related to water quality. High levels of NO3− and FeT are recorded in 75% of the groundwater samples, mainly when water scarcity is higher during the summer. According to Chilean standards, nitrate levels exceed the maximum permissible limits for drinking water. A type of mainly Ca (Mg)–HCO3− carbonated water was recorded with Ca2+, Na+, Mg2+, and HCO3− as dominant ions. This study demonstrated the opportunity of a rural community group to help fill spatial data gaps in water quality monitoring and reassess water quality protection, especially considering the decrease in water availability in the region. Therefore, the community together with researchers can respond with an early alert system to water quality to collaborate with the implementation of rural drinking established on a community-based water quality monitoring. Moreover, to prioritize the level of urgency of rural water, minimize water pollution and improve the perception of the water and the resilience of their social–ecological system.
Introduction
Citizen science is defined as collaborative science between citizens and professional scientists, in order to investigate a topic that is relevant to the local community (Cohn, 2008; Buytaert et al., 2016; Vohland et al., 2021). It corresponds to the participation of amateur volunteers from local communities in scientific research (Paul et al., 2018). In this way, the interests of both the scientific and local community are aligned to achieve a common goal, such as the sustainability of a local resource (Perevochtchikova et al., 2016). There is a huge increase in acceptance globally in recent decades (Bonney et al., 2014). Therefore, citizen science may be a resource with a huge potential, mainly because it can fill knowledge gaps in the territory, can increase environmental awareness to provoke a behavioral change, facilitate traditional monitoring incorporating cost-effective alternative help, and can provide incentive corporate funding and support (Haklay, 2013). Currently, various citizen science programs, where non-scientists participate in the design, collection, or interpretation of data, have been successfully implemented in several fields of study, such as ecology, ornithology, and astronomy (Buytaert et al., 2014; Shupe, 2017). Examples include websites iNaturalist, eBird, ZooUniverse, and CitSci among others. This enables the exploration of scientific questions about greater scales and includes locations without available data (Loss et al., 2015; Reed et al., 2018; Kirchhoff et al., 2021). However, in certain disciplines such as geology, hydrology, and environmental chemistry, there has been a more gradual integration of citizen science, mainly because the technical experience is needed for effective sampling. This requires participant training within a research project. It has been shown that through these collaborations, academics are able to increase the scope of their research and obtain better information on spatial and temporal variability (Weyhenmeyer et al., 2017). Over this decade, bottom-up approaches have been conducted in implementing community-based water quality monitoring in response to local concerns regarding environmental issues (Bonney et al., 2014; Alender, 2016; Jollymore et al., 2017; Enqvist et al., 2020; Hegarty et al., 2021). Different ways to perform citizen science participation such as community-based water quality monitoring is possible through contributive, collaborative, and co-creative initiatives (Bonney et al., 2009). Table 1 summarizes different community-based water quality monitoring initiatives over the past decade carried out using the citizen science methodology, mainly in Mexico, Colombia, the United States, and European countries such as the Netherlands, the United Kingdom, and France. These studies involve interested volunteers to be trained in the monitoring of basic water quality parameters, data collection, and interpretation of the data. Training the volunteers develops their capacity to carry out independent monitoring and assigns the implementation of these tools to volunteers in rural areas, where water quality is generally difficult to monitor for non-specialized people (Baalbaki et al., 2019). However, the benefits of these citizen science trainings include changes in attitude, behavior, and increased awareness about relevant issues within a community (Roetman and Daniels, 2011). Therefore, ensuring that the data obtained is valuable and reliable remains a challenge that requires the dedication of both the scientist and the scientific volunteers. In Chile, there is still a relatively low citizen participation in subjects related to hydrology and environmental chemistry topics, although projects such as “citizen pluviometers” have been developed, that encouraged the installation of rain gauges at home for sampling and uploading data onto an internet platform (DGA, 2004). Furthermore, community-based water quality monitoring has been applied to investigate the accelerated increase in nutrients in Lake Panguipulli, in southern Chile (Amsteins, 2013). Community-based water quality monitoring initiatives have also been implemented in rural coastal communities of Central Chile to investigate water quality and availability (Rivas et al., 2020), but still there are specific initiatives that are scarce, relative to other areas in the world (Sherbinin et al., 2021). However, the majority of research projects stem from contributive proposals from academia, rather than collaborative and co-creative from local initiatives. Overcoming this hurdle, so that the community takes a lead in these initiatives, could transform the interactions between communities and their local environment, and help fulfill the United Nations (UN) Sustainable Development Goals (SDGs). In this way, citizen science projects highlight the importance of good water stewardship within an ecosystem, facilitating harmonization and shared areas of interest between communities and researchers. There are opportunities in Central Chile to develop community-based water quality monitoring over various rural locations, exposed to different land uses, involving citizens that are keen to understand and attend issues around the water quality problems. Particular consideration is given to the coexistence of various land uses such as industrial activities, irrigation and agriculture, and urban and rural populations. On the other hand, an increase over the past two decades in forest plantations of non-native tree species such as pine and eucalyptus is likely to continue. These issues, together with a prolonged water shortage (Garreaud et al., 2018; Boisier et al., 2018), are accentuated in rural communities without drinking water (Aldunce et al., 2017). At present, the Boca Itata area, located in the coastal area of the Ñuble region of Central Chile, is home to rural communities dedicated to subsistence farming and they make up a rural area with lack of drinking water. Groundwater is important for both irrigation and consumption. In this area, the lack of drinking water prevents the development of water conservation strategies, as communities receive weekly provisions of drinking water from water supply trucks (Municipality of Trehuaco, 2018). Furthermore, wells are located near subsistence farming areas, it is likely those agrochemicals such as nitrate and phosphate, enter the surrounding water sources. Therefore, community-based water quality monitoring can help to identify the chemistry of the water sources based on the lithology of the study place and look for potential solutions if they know what type of water is present. Moreover, systematically generated data from citizens can lead to more thorough analysis and the application of hydrogeochemical modeling that allows prediction of water composition and the resulting mass transfers.
To estimate water composition and weathering reactions to know water quality, inverse modeling can be used as an important tool (Plummer, 1992). Inverse modeling efforts to account for the chemical changes that strike as water evolves along a flow path (Plummer et al., 1994). Inverse mass balance modeling efforts to assessing sets of mole transfers of phases that account for changes in water chemistry between one or a mixture of initial water compositions and final water compositions (Parkhurst and Appelo, 1999a). In this way, it is possible to determine the reactions that lead to the conversion of minerals and explain the changes in water chemistry (Parkhurst and Appelo, 1999b; Parkhurst and Appelo, 2013). By using the lithology of a place and knowing the solutes present in the water through the PHREEQC model, it is possible to understand and characterize historical community-based water quality monitoring data and evaluate the geochemical processes that generated water quality changes. PHREEQC has been widely used for hydrogeochemical analysis of groundwater (Naderi Peikan and Jalali, 2016; Mahaqi et al., 2018; Abdelshafy et al., 2019). Naderi Peikan and Jalali (2016) explained how the water chemistry changed along the flow path. Li et al. (2010) carried out the analysis of the hydrochemical evolution of groundwater and the mechanisms of its formation, identifying variation in terms of its composition passing from the Ca–HCO3− and Mg–Cl type to the Mg–Cl–HCO3− type.
Consequently, this study was focused on the concern of the community to know their water quality composition and to find a topic that ensures future drinking water. Therefore, the objective of this co-creative study was to evaluate the water quality (pH, total iron (FeT), alkalinity as HCO3−, hardness (Ca2+ and Mg2+), temperature, sulfate (SO42−) chloride (Cl−), and nitrate (NO3−) and hydrogeochemical processes through the community-based water quality monitoring and geochemical modeling. This study was carried out in the rural area of the Itata Valley, Boca Itata in the Ñuble Region of Central Chile.
In order to carry out this, together with the community, basic information was generated which was used in a geochemical model (PHREEQC) to understand the changes in chemical quality and the origin of these changes. In this way, to initiate the formation of a network within the community that promotes issues around water security.
Materials and Methods
Study Area
The study area is located within a catchment of approximately 50 km2, in the coastal area of Boca Itata, located in the Itata Valley in the Ñuble region of Central Chile (Figure 1). Out of a community of approximately 150 habitants, about 20–25 locals meet monthly at the Boca Itata Neighborhood Meeting Center to discuss their community issues. The concerns from the community were related to the lack of sampling stations in the area and the presence of a duct from a pulp and paper plant, part of the local forestry industry. This duct crosses a road within the community and generates disbelief in the content of the water, due to its potential fractures. Hence, an important reason for the community is to know and understand the water type and quality.
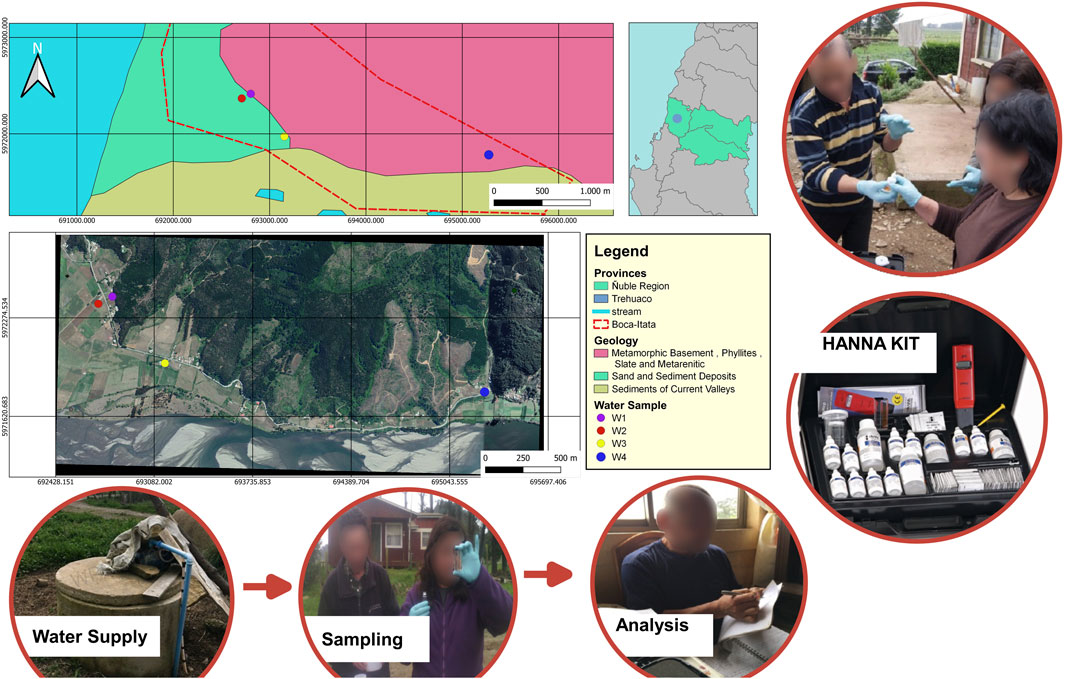
FIGURE 1. Location of groundwater sampling sites (w) in Boca Itata, Trehuaco, Ñuble region in Central Chile.
Taking into consideration the typical Mediterranean temperate climate of Central Chile, the study area has an average annual temperature and a rainfall of 14.1°C and 1,550 mm, respectively (CR2, 2017). According to the soil types in the Biobio region (Stolpe, 2006), these correspond to the Cobquecura series, characterized by sedimentary soils with a silty clay loam texture, a physiography of marine terraces, dissected by streams, a smooth and undulating topography, transitionary forest vegetation, and a moderate permeability and good water retention. The main material in the area is myceous marine sediment. According to the FAO-UNESCO system, alfisol is the predominant soil type in the area (Luzio et al., 2003). The morphology of the catchment corresponds to rounded hills, and gentle slopes interspersed with valleys and plains, such as the Itata River Valley. Between the town of Trehuaco and the mouth of the Itata River, the river flows between lateral river terraces extending toward the river mouth. At the river mouth, there is a wide and dynamic sand bar (Aqualogy Medioambiente, 2013). The area has a pluvio-nival regime, with an average monthly flow between 760 m3/s during the winter and 22 m3/s during the summer (DGA, 2004). Drinking water is delivered to the Boca Itata community once or twice a week by water supply trucks. The average water consumption, per person, is 0.02 m3/s. The community predominantly subsists on traditional subsistence farming, including potatoes, artichokes, onions, and other vegetables, and artisanal fishing, including rock cod (Eleginops maclovinus), mullet (Mugil cephalus), shellfish, and edible algae.
Related to the lithology of this area, there are intrusive rocks from the CPg formation, mainly granite formation, mainly granites, granodiorites, tonalites, hornblende diorites, and biotite, locally of muscovite. In addition, metamorphic rocks of the Pz4b formation are found, characterized by the content of shales, phyllites, and metarenstones with low-gradient metamorphism from the early Carboniferous (Aquaterra Ingenieros Limitada, 2011). Munizaga et al. (1973) presented the main minerals for this area such as quartz (SiO2), biotite K(Mg,Fe)3 (AlSi3·O10) (OH,F)2, muscovite (KAl3·Si3·O10·(OH)2), filite (Al2·O3-4SiO2-H2), chlorite (ClO2−), and andalucite (Al2 SiO5). It is also possible to identify several types of clays such as kaolinite, hornblende, montmorillonite, and illite (SERNAGEOMIN, 2003).
Data Generation
From May 2016 to September 2021, water quality workshops to explain water quality concepts and water management importance were held at the Boca Itata Neighborhood Meeting Center, and physicochemical variables from water samples of groundwater (from wells) were taken in four stations within the catchment (Figure 1), selected according to the interests of the community. During previous workshops, the Boca Itata community had the interest to know water quality of their wells, regarding the concern about the growing industrial impact on the water consumed by the inhabitants. According to this interest, a study approach was approved by the Boca Itata Neighborhood Council (INC), and all water-sampling activities carried out on/in their lands were executed in accordance with the INC. A total of four workshops were held with the community, and a verbal informed consent was obtained from all participants to act in the water sampling and in the workshops. The first workshop aimed to identify sites considered by the community as unsafe for consumption and irrigation, identifying potential sources of pollution according to the location of an industrial wastewater pipeline and agricultural farming areas. A second workshop trained a group of 20 adults representing the families of the community (older than 45 years old) on different physicochemical parameters present in the water. All water-sampling activities from their lands were carried out in accordance with the community neighborhood and only water from wells was sampled during wet (winter and spring) and dry seasons (summer and autumn). Subsequently, the remaining workshops were held to increase awareness in the community of relevant sampling parameters and water quality results and management.
A mobile laboratory was used (from the brand Hanna), for the community, to determine different physicochemical parameters, such as pH, alkalinity as CaCO3−, hardness (Ca2+ and Mg2+), temperature, sulfate, (SO42−) chloride (Cl−), and nitrate (NO3−). Physicochemical parameters (Table 1) were selected according to the ease of sampling, sampling duration, and ensuring low-moderate sensitivity. These major ions were selected due to their importance to explain the basin geochemistry of the water. These ions are constituents that contribute to the amount of total and dissolved solids in waters. Water samples were taken from the community, with the support of the researchers, once every 3 months. A total of 546 samples were taken during the studied period during wet and dry seasons. Before sampling, the local representative (President of the Neighborhood Board) of the community agreed on the sampling details (timing) and the relevant neighbors were advised, that is, the owners of the well. The laboratory analysis for different parameters was made to compare with in situ results. Nitrates were measured using a UV-Vis spectrometer, total iron through ICP-OES spectrophotometry, and major ions were analyzed by ionic chromatography. Total arsenic (AsT) was included in the laboratory analysis and was measured by atomic spectrophotometry. These analyses were performed through the methodology recommended in Standard Methods (1999) (APHA, 1998).
Hydrogeochemical Analysis and Modeling
The quality of the results of chemical analysis was verified by calculating the ion balance. This calculation considers the sum of cations and anions expressed in units of milliequivalent/liter (meq/L).
The hydrogeochemical analysis of groundwater was carried out through diagrams such as Piper, which provides a convenient method to classify and compare types of water by knowing the ionic composition of the different water samples (Mahaqi et al., 2018). Piper diagrams have a triangular shape that represents the proportion of major ions.
Through the information obtained from the data, the inverse modeling was carried out using the PHREEQC program, where the geochemical reactions that led to a certain water quality must be identified. In addition, it is necessary to identify the equilibrium conditions for the concentrations of chemical species in solution and geochemical processes (precipitation/dilution) through the mineral saturation indices of the solid phases, among others. The SI of the mineral phases is calculated as follows (Parkhurst and Appelo, 2013):
where IAP is the product of ionic activity of the chemical species dissociated in solution, and Ksp denotes the equilibrium solubility product for the chemical substances involved at the same temperature. IS < 0 y IS > 0 indicate states of under-saturation (dissolution) and super-saturation (precipitation) for groundwater with respect to the specific mineral phase, respectively. PHREEQC reproduces a list of scenarios that consider the most plausible combinations of geochemical processes that are likely to occur in a water ecosystem. For instance, the way to identify whether calcite dissolution/precipitation is relevant or not consists of solving the inverse problem under few scenarios.
To carry out the inverse modeling it is necessary to consider the following from Kuldip et al. (2011):
(1) To know the chemistry of the initial and final point and meet the flow trend.
(2) The hydrochemistry of the groundwaters is not influenced by diffusion.
(3) The chemical composition of the analyzed point prevails during a considered time, the chemical species dissolved in the aqueous phase are in homogeneous equilibrium, although the solution is not in equilibrium with other phases present, such as the different species of chemicals that constitute a soil or any other solid phase that is in contact with the original aqueous phase forming a heterogeneous equilibrium.
(4) The mineral phases used in the modeling are or were present in the aquifer.
(5) To develop the model, it is necessary to have the physicochemical parameters, the identification of the minerals present in the study area, the codes to develop the model and to have the Notepad+ platform, which is the text editor where the model is written and developed. This editor also allows obtaining the output files with the data produced and performed by the PHREEQC program.
In general, data were reviewed by following the regulations established under the applicable Chilean Laws, such as NCh1333, which applies to recreational water use and irrigation. Data analysis was carried out by the researchers, using independent t tests to identify variances between the data collected in the field (Murno, 2005). Statistical t tests were performed based on community vs. scientific data. The R program was used to carry out data analysis. Comparing the volunteer data with data collected and analyzed in the laboratory studies validated the data.
Results
Participation of the Community in Obtaining Data
Initially, all the participants, 25 families represented, who attended the first workshop questioned whether the water quality from local wells was adequate for drinking or cooking. A total of 84% of participants believed that the well water was contaminated with chemicals from industry and forestry activities in the area. Only 16% of participants were satisfied with the water delivered by the water supply trucks. A fixed number of four volunteers show up as water quality monitors to represent the community to carry out the sampling throughout the duration of the project. These monitors play an important role in the study because they transmit the information generated in each water quality monitoring. During the study period, the researchers shared information with the volunteers on the environment and water stewardship and were available to answer questions related to the water quality in the local area. There was a good participation of the community in this meeting, including the owners of wells and a representative of the municipality. A biannual meeting was held to share the results of field sampling by the community and researchers.
Geochemical Trends From Water Quality Monitoring
Table 2 presents the concentrations of seven physicochemical parameters for water quality reported by community volunteers and researchers. Water temperature values varied between 10.2 and 20.3 (average, 14.4 ± 2.2°C), and hydrogen ion concentration (pH) indicator of acidity or basicity of a solution showed basic groundwater, with neutral to moderately basic pH ranging from 6.2 to 8.2 (average 7.1 ± 0.4). The physicochemical parameters obtained by the community were corroborated with the in situ measurements and in the laboratory taken by the researchers. In addition, an independent t-test was used to show no significant difference (p > 0.05) in the average pH concentration from well data ([pH] =6.91 ± 0.46 mg/L) compared to data taken by the researchers ([pH] = 6.97 ± 0.30 mg/L). Some significant differences were obtained with the laboratory analysis for some ions such as SO42−, Cl−, and FeT. Nitrate results indicate a difference between the samples taken by volunteers and researchers, mainly because the range limit for the nitrate kit reaches 50 mg/L but was lower than the concentrations reported by the researchers (mean [NO3−] = 99.3 ± 0.07 mg/L).
The studied physicochemical parameters by the community, based on the hydrochemistry of the well samples, showed that the major ion types in the general dominance of anions were in the order of HCO3− > Ca2+ + Mg2+ > NO3− > Cl− > SO42−. Most of the waters are bicarbonate type and less percentage is classified as bicarbonate–calcic–magnesic, with Ca2+ ranging 3.3–39 mg/L HCO3− as 35–250 mg/L. Relative to Cl− values ranging from 7.3 mg/L to 50.3 mg/L where the minimum value was found at W4 and the maximum concentration at W3. Mg2+ varies between 2.7 mg/L and 26.2 mg/L, the minimum concentration was presented at point W4 and the maximum concentration at point W2. The average presented by this ion is 14.4 ± 7.2 mg/L. K+ concentrations ranging from 0.28 mg/L to 34.75 mg/L with the smallest at W2 and the largest at W4. K+ concentrations are mostly low, reaching an average of 5.5 ± 10.5 mg/L (Table 3). Alkalinity showed concentrations from 35 mg/L to 250 mg/L, with the lowest concentration during spring and the highest concentration during winter. On average, alkalinity reached concentrations of 119.6 ± 55.4 mg/L, which is within the normal ranges of alkalinity, since if there are concentrations lower than 10 mg/L this could result in water being very corrosive. Related to the correlation coefficient, NO3− is significantly correlated with alkalinity as HCO3− (r2 = 0.48; p = 0.03) and Cl− (r2 = 0.45; p = 0.04).
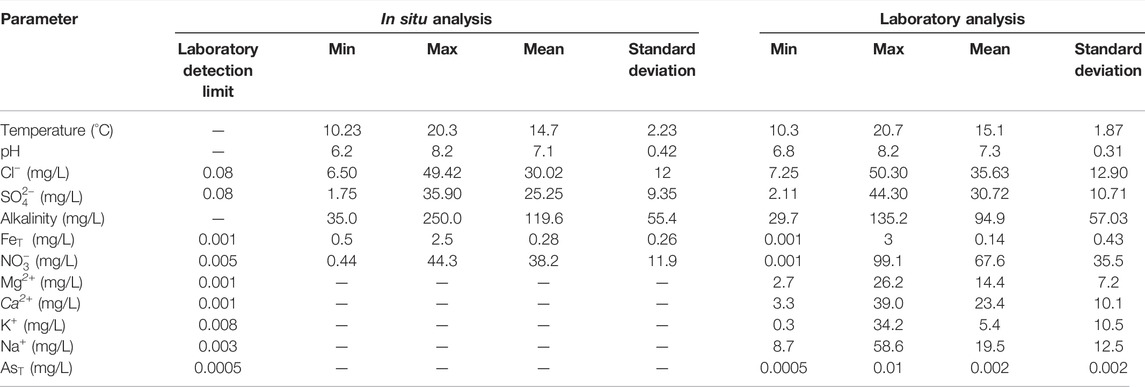
TABLE 3. Average physicochemical parameters for data sampled from well water recorded by volunteers and researchers from the Boca Itata community.
The association between hardness (Ca2+ + Mg2+) versus (HCO3− + SO42−) shows that points follow the line that suggests the Ca2+ and Mg2+ chemistry is mainly explained by calcite and gypsum weathering processes in the system (Figure 2). From the saturation index, the dominant geochemical process is the dissolution of carbonate minerals (e.g. calcite, halite, and dolomite were oversaturated) in the system, which contributes the Ca2+, Mg2+, and HCO3− to the groundwater. Goethite and hematite minerals were supersaturated, indicating precipitation (Table 4). There are higher concentrations of HCO3−, for which there is an increase in alkalinity, which allows maintaining the balance of the system in the event of sudden changes. In all the wells is presented supersaturated water with respect to silica.
From Geochemical Data to PHREEQC Model Predictions
Based on the saturation indices (Table 4), it is possible to determine one of the main processes that generate chemical changes in the water, is the dissolution of carbonates such as calcite and dolomite. These present negative saturation indices, which implies that these minerals are saturated, and this is in accordance with what was found with citizen volunteers, that from point W3 to the other points there is an increase in the presence of ions such as Ca2+, Mg2+, and HCO3−, which come mainly from these minerals.
As a result of the inverse hydrogeochemical modeling from point W3 to W2, the transfer of moles that developed between the water and the rock present in the study area was obtained. Based on this, it was determined that the molar transfer values with a positive value indicate dissolution. On the other hand, a negative value indicates precipitation. Modeling results show that halite, calcite, gypsum, biotite, and Fe(OH)3 are saturated to oversaturated with respect to carbonate oversaturation could be due to an input excess of Ca2+ and Mg2+ ions from silicate weathering processes. Carbonate compounds are found dissolved in water, which is aligned with the water composition of the water in the area, together with the precipitation of minerals that contain silicates such as chlorite and illite, thus contributing cations and silica.
In the case of the hydrogeochemical modeling from point W1 to W2, the model has five scenarios. In all models, the dissolution of halite, gypsum, goethite, and calcite and a precipitation of montmorillonite, chlorite, and muscovite. All this is related to what was previously analyzed, since the sampled points are close and have similar trajectories. The solubility of goethite (9.80E+02 mmol/L) was higher than that of calcite, (1.26E−01 mmol/L) and gypsum (2.49E−02 mmol/L), as observed in Table 5. To select the model that is more representative of the hydrogeochemical processes that occur in the groundwater of the area, it is necessary to take into account the analysis of the hydrogeochemistry of the water, the minerals present, and the conditions found in the water (Li et al., 2010). Based on this, the models follow a good representation of the processes that may be occurring in the water, such as the case of halite, gypsum, and calcite. In the five models, this is found in the dissolved form, for which within the W1 and W2 route there should be an increase in these minerals such as Ca2+, Cl−, SO42−, HCO3−, and Na2+. This is consistent with the hydrogeochemical analysis. In addition, this model represents most of the minerals found in the area and due to their presence, mostly in dissolved form, explains the increase in the concentrations of major ions such as FeT, Ca2+, and HCO3− among others.
Scientific Diffusion to the Community
Since 2016, people from Boca Itata have carried out community-based water quality monitoring of the groundwater sources that are used daily for consumption and irrigation. This initiative to monitor water quality arises as a response from the community itself to the concern of knowing the quality of the water they use. By not having rural potable water treatment or monitoring systems, it produced a growing uncertainty among neighbors of the water risks. Hence, this co-creative approach is considered as a base to the community members. It is also the same community with constant scientific support that has identified the main question problems and detected the sampling points of interest for the community. This allows the community to become a central part of the investigation development. On the other hand, scientists supported the application of geochemical modeling with PHREEQC of the data generated by the community and interpreted the results of the study for the community.
Once the results over time were obtained, it was disseminated to the community, through citizen science conferences, regional newspapers, as well as face-to-face conversations. Face-to-face meetings with the president of the neighborhood council allowed showing the parameters that were above the permitted levels, and their corresponding implications. In addition to the origin of the main solutes recorded over time, they were obtained through geochemical modeling. Outstanding solutes such as nitrate (NO3−) were well above the limits allowed in Chilean regulations, exceeding 50 mg/L. This type of groundwater anomaly is not related to the lithology of the area, and it was explained to the community that it rather has a human origin because of agricultural activities they carry out. These anthropogenic activities, that take place in the area such as agriculture, livestock, and the use of septic tanks, produces levels of ammonia and nitrate that may remain available in the surrounding environment.
In the absence of natural sources of nitrogen, the values of NO3− in the groundwater should not exceed 10 mg/L. The dissolved FeT also registered some stations with higher levels than those allowed by the regulations (0.3 mg/L. An increase in this parameter brings with it, aesthetic problems in the water or in the water supply systems. However, the alkalinity present in the area provided a buffer that allowed to balance sudden changes in the pH of the water. In this way, fouling and acidification problems are avoided. The hardness of the water was high, which was corroborated by the volunteers.
Discussion
This study considers community-based water monitoring to highlight how data collection using simple field sampling techniques can be used in geochemical modeling to promote rural water management. This citizen science study supports the notion that volunteer involvement in the project can foster community motivation and self-identification, raise awareness of the importance of clean water, and certainly reduce uncertainty about water issues in the community (Jordan et al., 2015; Gray et al., 2017). In this way, a direct link can be formed between the benefits provided by continuous measurements of water quality by the community of people and the concern of the local government to cover the environmental points that it cannot measure regularly. The monitoring of water quality with different parameters carried out by the community can become a regular practice to obtain an early warning system of solute levels and control the measured parameters for future rural drinking water system installation for the community. In this study, it was identified that one point was systematically of better quality and served to identify it as a potential point to suggest to the local government the installation of rural drinking water. Hence, they become significant actions for the conservation of the water quality of their territory.
Considering the current scenario of lower water availability, it is also highly necessary to evaluate the quality of the available water for human consumption. A decade ago, there was a greater availability of surface water (Garreaud et al., 2018), and groundwater availability was less of an issue. However, there is now an ongoing situation related to a lack of groundwater in Central Chile, enhanced by the mega-drought present in the country (CR2, 2015). Rural areas, that are more directly dependent on groundwater, are most affected by water scarcity. Therefore, it is necessary to be focused on successful practices within rural communities, thus that they understand the problem of decreasing water availability and the resulting consequences on water quality. Through this study it was identified that an initiative that emerged from the community-based group and contributed positively to social–ecological fit, refers to the inability of the institutions to have a greater spatial coverage of water quality in rural communities to adequately align with water quality dynamics (Enqvist et al., 2020).
However, the results indicate an overall good physicochemical water quality, but with higher nitrate levels, that exceed (>90 mg/L), in most wells an exception of well 4, the maximum limits established in the World Health Organization (WHOS) standards with a maximum of 50 mg/L (Ward et al., 2018). This type of groundwater anomaly is not related to the lithology of the site. However, anthropogenic activities developed in the area such as agriculture, livestock, and the use of septic tanks produce levels of ammonium and nitrate that can remain available in the surrounding environment. In the absence of natural sources of nitrogen, the values of NO3− should not exceed 10 mg/L. The Chilean standard NCh1333 does not include nitrate, however, according to the Chilean Drinking Water Standard 409/1, nitrate–nitrogen should not exceed 10 mg/L, given its adverse health effects (Herrera-Apablaza, 2018). In the case of iron, it showed ranges between 0.23 and 2.2 mg/L, which is high for the Chilean standard of human consumption (NCH 409/1), especially in well 3. The increase in this parameter brings with it aesthetic problems in the water or problems in the water supply systems. However, water alkalinity was a good buffer, with values reaching 150 mg/L CaCO3. Low alkalinity causes a greater sensitivity to contamination, which allowed to balance sudden changes in the pH of the water, thus allowing to avoiding fouling and acidification problems. Previous studies have indicated that borates, silicates, and phosphates may also contribute to alkalinity, and in this area (between the mid to deep part of the basin) there have been previous reports of high boron (0.75–1.10 mg/L) and silicate (3–10.5 mg/L) concentrations (CIREN, 2003; Yevenes et al., 2018).
Based on the Piper diagram, it was indicated that most samples present a type of water Ca (Mg)–
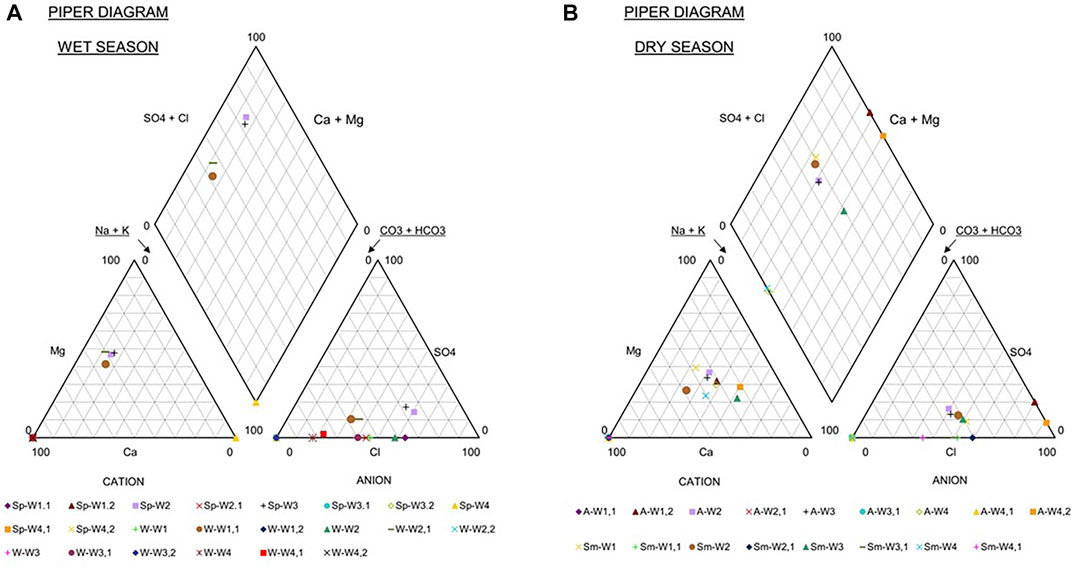
FIGURE 3. Seasonal hydrochemistry from the community-based water monitoring through the Piper diagram (A) during the wet season that included winter and spring (B) dry season summer and autumn.
By analyzing the inverse modeling, the influence of technology in the water chemistry of Boca Itata is recorded, where the dissolution of carbonate minerals plays a fundamental role in the composition of the water, since minerals such as calcite, dolomite, gypsum, and halite were found in the trajectory in dissolved form, producing an increase in the concentration of higher ions. Other factors that influence the chemical composition of the water are the precipitation of compounds containing Fe2+, which intensifies the high levels of this ion. According to this analysis carried out with inverse modeling, together with the geochemistry of the area, it was determined that from all stations analyzed, the point W4 would present optimal general chemical conditions for the implementation of a rural drinking water system (RDWS), given the need of the community. This is the point where the composition of the water presents better chemical conditions and presents no great changes in the geology of the area and anthropogenic activity.
In this study, it is worth highlighting the importance of the rural community of Boca Itata, who, due to their concerns, sought and were part of the development of a citizen science project, such as community monitoring of water quality (Figure 4). In order to determine the quality of the water they use daily, an essential natural resource for people and that in many parts of our country there are still communities that do not have the necessary support to carry out these investigations, mainly due to budget limitations and scientific knowledge. This type of initiative is a contribution to the generation of knowledge and the participation of people, which brings with it, greater confidence and involvement with issues as important as water quality. This type of initiative can be implemented in a simple way through social organizations, neighborhood associations, or interested groups and can allow the creation of a surveillance system, where the participants themselves identify possible problems in the water and can alert the community to these cases, in order to be able to search for the origin of the problem and find solutions with the help of researchers, scientists, or state agencies in charge of these issues (Aravena et al., 1989; Aqualogy Medioambiente, 2013; Herrera and Custodio, 2014; Santibañez, 2016; Yevenes, 2016; Yevenes, 2018; Brintrup et al., 2019).
The results were disseminated through scientific conferences, regional newspapers, as well as face-to-face conversations, which are the baseline for future research on community participation. Similarly, effective communication with the local community was essential to obtain satisfactory results. The researchers were interested in building trust with the community through the sharing of the results, and discussing potential solutions to improve the current situation, such as those reported in other studies (Jordan et al., 2015; Perevochtchikova et al., 2016; Baalbaki et al., 2019). This initiative in Central Chile provides the opportunity to build relationships between academia and local communities, generating valuable information and diminishing the uncertainty about consumed water content for local communities, in addition to allowing the community to act as a self-identified community with this resource. This initiative has encouraged local citizens to get involved in data analysis, as well as considering potential corrective measures in order to prepare for the installation of rural drinking/potable water (RD/PW) in the local area. Local demand for water is increasing, considering both drinking water and monocultures from the forestry industry (Hurlbert et al., 2009). Furthermore, this increase in water use is further aggravated considering current climate change projections, which estimate a reduction in the basin flow of up to 50% over the next few decades in Chile (MME, 2017). This is in addition to an increase in the frequency, intensity, and duration of drought-related phenomena (Tarhule, 2017). These threats are enhanced by the absence of a well-defined and consistent water resource plan, along with disaggregated and poorly coordinated governmental sectors (Montaña et al., 2016; Urquiza et al., 2019). Furthermore, the community faces strategic difficulties to obtain rural drinking/potable water (RD/PW), considering its geographical location and future supervision. Potentially dangerous chemicals are more readily concentrated in groundwater compared to surface water, mainly due to the longer residence times for groundwater (Burri et al., 2019). The results indicated that the prevalence of septic tanks in the area, combined with the absence of sewage treatment, might cause groundwater contamination, as well as the proximity of the study area to the coast.
However, community-based efforts can provide important complementary research to water quality government sampling stations, and even raise public awareness of the water paradigm shift so that the rural population learns the responsible management of water to reduce pollution prior to consumption. The challenge is to apply adaptive solutions so that there is more frequent control of water quality in rural areas.
Conclusion
This study demonstrates that community organizations offer an alternative methodology to rural water stewardship through a citizen science approach. Moreover, key information from areas where the data are not available to study water quality. This experience gives an example of a pilot project for an early alert system of water quality built from the community and that could be replicated throughout neighboring communities, local and regional governments. This would create a type of the rural community water network, which is an appealing model to those responsible for regional water management. Among the seven physicochemical water quality parameters sampled, iron and nitrate in wells presented the greatest variation between the summer and winter seasons. Trained volunteers within the community were committed and available to participate in the study, despite having to also fulfill other activities in the rural area, mainly because the water quality for them is overly concerning.
Community results were supported with reliable, repeatable, and accurate data on water quality and chemical concentrations in the water. In this way, it was possible to identify variations in indicators of water pollution, and trained volunteers can notify these changes to relevant authorities. However, there were issues regarding the measurements of sensitive parameters in the field. Future studies should focus on resolving this issue. At this stage, it was not possible to measure the impact of the industry, as only basic parameters were considered in this first approach to community monitoring. Ultimately, the success of the study was mainly due to the mutual efforts made by the community and academia.
Data Availability Statement
The datasets presented in this article are not readily available because author can send the data once the article is published. Requests to access the datasets should be directed to bXlldmVuZXNAdWRlYy5jbA==.
Ethics Statement
Ethics review and approval/written informed consent were not required as per local legislation and institutional requirements.
Author Contributions
MY and RB designed the study. MY, HP, and RB performed the analysis. MY wrote the manuscript. All the authors reviewed and edited the manuscript.
Conflict of Interest
The authors declare that the research was conducted in the absence of any commercial or financial relationships that could be construed as a potential conflict of interest.
Publisher’s Note
All claims expressed in this article are solely those of the authors and do not necessarily represent those of their affiliated organizations, or those of the publisher, the editors, and the reviewers. Any product that may be evaluated in this article, or claim that may be made by its manufacturer, is not guaranteed or endorsed by the publisher.
Acknowledgments
The authors thank the community of Boca Itata for their long-standing willingness to want to conserve their water, especially the President of the Neighborhood Board until 2021, Omar Gutiérrez, and his family for their continued support.
References
Abdelshafy, M., Saber, M., Abdelhaleem, A., Abdelrazek, S. M., and Seleem, E. M. (2019). Hydrogeochemical Processes and Evaluation of Groundwater Aquifer at Sohag City, Egypt. Sci. Afr. 6, E00196. doi:10.1016/j.sciaf.2019.e00196
Aldunce, P., Araya, D., Sapiain, R., Ramos, I., Lillo, G., Urquiza, A., et al. (2017). Local Perception of Drought Impacts in a Changing Climate: The Mega-Drought in Central Chile. Sustainability 9, 2053. doi:10.3390/su9112053
Alender, B. (2016). Understanding Volunteer Motivations to Participate in Citizen Science Projects: A Deeper Look at Water Quality Monitoring. J. Sci. Commun. 15. doi:10.22323/2.15030204
Amsteins, O. K. P. (2013). Monitoreo ambiental participativo y Ciencia Ciudadana en el Lago Panguipulli: análisis de caso y propuestas para su conservación, Región de Los Ríos, Chile. Ingeniero en Conservación de Recursos Naturales, Universidad Austral de Chile. Valdivia, Chile,
APHA (1998). Standard Methods for the Examination of Water and Waste Water. American Public Health Association.
Aqualogy Medioambiente, C (2013). Modelación Hidrogeológica cuenca Itata-bajo, Región del Biobío. Dirección general de Aguas. Informe final SIT320.
Appelo, C. A. J., and Postma, D. (1993). Geochemistry, Groundwater and Pollution. Rotterdam: Balkema.
Aravena, R., Suzuki, O., and Pollastri, A. (1989). Coastal Fog and its Relation to Groundwater in the IV Region of Northern Chile. Chem. Geol. Isot. Geosci. Sect. 79, 83–91. doi:10.1016/0168-9622(89)90008-0
Aquaterra Ingenieros Limitada. (2011). Estudio Hidrogeológico Cuencas Biobío e Itata, tomo I informe final Ministerio de Obras Públicas Dirección General de Agua. https://snia.mop.gob.cl/sad/SUB5348v1.pdf.
Baalbaki, R., Ahmad, S. H., Kays, W., Talhouk, S. N., Saliba, N. A., and Al-Hindi, M. (2019). Citizen Science in Lebanona - a Case Study for Groundwater Quality Monitoring, 6. Royal Society Open Science. doi:10.1098/rsos.181871
Bartelt-Hunt, S., Kolok, A., and Ali, J. (2018). Citizen science as a quantitative tool for temporal and spatial water quality assessment. Abstracts of Papers of the American Chemical Society, 1155. WASHINGTON, DC, USA: AMER CHEMICAL SOC.
Boisier, J. P., Alvarez-Garreton, C., Cordero, R. R., Damiani, A., Gallardo, L., Garreaud, R. D., et al. (2018). Anthropogenic Drying in Central-Southern Chile Evidenced by Long-Term Observations and Climate Model Simulations. Elem. Sci. Anthropocene 6, 74. doi:10.1525/elementa.328
Bonney, R., Cooper, C. B., Dickinson, J., Kelling, S., Phillips, T., Rosenberg, K. V., et al. (2009). Citizen Science: A Developing Tool for Expanding Science Knowledge and Scientific Literacy. BioScience 59, 977–984. doi:10.1525/bio.2009.59.11.9
Bonney, R., Shirk, J. L., Phillips, T. B., Wiggins, A., Ballard, H. L., Miller-Rushing, A. J., et al. (2014). Next Steps for Citizen Science. Science 343, 1436–1437. doi:10.1126/science.1251554
Bos, J. S., Nanayakkara, L., Hurlbert, M., and Finlay, K. (2019). Citizen Science for Saskatchewan Lakes: a Pilot Project. Lake Reserv. Manag. 35, 77–89. doi:10.1080/10402381.2018.1538172
Brintrup, K., Amigo, C., Fernández, J., Hernández, A., Pérez, F., Félez-Bernal, J., et al. (2019). Comparison of organic matter in intermittent and perennial rivers of mediterranean chile with the support of citizen science, 92. Revista chilena de historia natural. doi:10.1186/s40693-019-0083-3
Burgos, A., Páez, R., Carmona, E., and Rivas, H. (2013). A Systems Approach to Modeling Community-Based Environmental Monitoring: A Case of Participatory Water Quality Monitoring in Rural Mexico. Environ. Monit. Assess. 185, 10297–10316. doi:10.1007/s10661-013-3333-x
Burri, N. M., Weatherl, R., Moeck, C., and Schirmer, M. (2019). A Review of Threats to Groundwater Quality in the Anthropocene. Sci. Total Environ. 684, 136–154. doi:10.1016/j.scitotenv.2019.05.236
Buytaert, W., Dewulf, A., De Bièvre, B., Clark, J., and Hannah David, M. (2016). Citizen Science for Water Resources Management: Toward Polycentric Monitoring and Governance? J. Water Resour. Plan. Manag. 142, 01816002. doi:10.1061/(asce)wr.1943-5452.0000641
Buytaert, W., Zulkafli, Z., Grainger, S., Acosta, L., Alemie, T. C., Bastiaensen, J., et al. (2014). Citizen Science in Hydrology and Water Resources: Opportunities for Knowledge Generation, Ecosystem Service Management, and Sustainable Development. Front. Earth Sci. 2. doi:10.3389/feart.2014.00026
CIREN (2003). Centro de informacion de recursos naturales [Online]. Available at: http://bibliotecadigital.ciren.cl/handle/123456789/9342 (Accessed 06 17, 2019).
Cohn, J. P. (2008). Citizen Science: Can Volunteers Do Real Research? BioScience 58, 192–197. doi:10.1641/b580303
CR2 Center For Climate And Resilience Research (2017). Available at: aHR0cDovL2V4cGxvcmFkb3IuY3IyLmNs (Accessed 11 11, 2018).
CR2 Center For Climate And Resilience Research (2015). The 2010-2015 mega-drought: a lesson for the future.
Dga Dirección General De Aguas, C.-I. (2004). Diagnóstico y clasificación de los cursos y cuerpos de agua según objetivos de calidad. Preparado por Cade- Idepe, Consultores en Ingeniería para el Ministerio de Obras Públicas, Dirección General de Aguas. Ministerio de Obras Públicas, Dirección General de Aguas.
Enqvist, J. P., Tengö, M., and Bodin, ö. (2020). Are Bottom-Up Approaches Good for Promoting Social-Ecological Fit in Urban Landscapes? Ambio 49, 49–61. doi:10.1007/s13280-019-01163-4
Garreaud, R. D., Alvarez-garreton, C., Barichivich, J., Boisier, J. P., Christie, D., Galleguillos, M., et al. (2018). The 2010–2015 Megadrought in Central Chile: Impacts on Regional Hydroclimate and Vegetation. Hydrol. Earth Syst. Sci. 21, 6307–6327.
Gray, S., Jordan, R., Crall, A., Newman, G., Hmelo-silver, C., Huang, J., et al. (2017). Combining Participatory Modelling and Citizen Science to Support Volunteer Conservation Action. Biol. Conserv. 208, 76–86. doi:10.1016/j.biocon.2016.07.037
Haklay, M. (2013). Citizen Science and Volunteered Geographic Information: Overview and Typology of Participation, Crowdsourcing Geogr. Knowl., 105–122. doi:10.1007/978-94-007-4587-2_7
Hegarty, S., Hayes, A., Regan, F., Bishop, I., and Clinton, R. (2021). Using Citizen Science to Understand River Water Quality while Filling Data Gaps to Meet United Nations Sustainable Development Goal 6 Objectives. Sci. Total Environ. 783, 146953. doi:10.1016/j.scitotenv.2021.146953
Herrera Apablaza, V., Gutiérrez roa, N., Córdova Molina, S., Luque Marín, J., Idelfonso Carpanchay, M., Flores Riveras, A., et al. (2018). Calidad del agua subterránea para el riego en el Oasis de Pica, norte de Chile. Idesia (Arica) 36, 181–191.
Herrera, C., and Custodio, E. (2014). Origin of Waters from Coast of Chile, Small Springs Located at the Northern in the Vicinity of Antofagasta. Andean Geol. 41, 314–341. doi:10.5027/andgeov41n2-a03
Hurlbert, M., Corkal, D. R., and Diaz, H. Government and Civil Society: Adaptive Water Management in the South Saskatchewan River Basin. 2009. 181–210.
Jollymore, A., Haines, M. J., Satterfield, T., and Johnson, M. S. (2017). Citizen Science for Water Quality Monitoring: Data Implications of Citizen Perspectives. J. Environ. Manag. 200, 456–467. doi:10.1016/j.jenvman.2017.05.083
Jordan, R., Crall, A., Gray, S., Phillips, T., and Mellor, D. (2015). Citizen Science as a Distinct Field of Inquiry. BioScience 65, 208–211. doi:10.1093/biosci/biu217
Kirchhoff, C., Callaghan, C. T., Keith, D. A., Indiarto, D., Taseski, G., Ooi, M. K. J., et al. (2021). Rapidly Mapping Fire Effects on Biodiversity at a Large-Scale Using Citizen Science. Sci. Total Environ. 755, 142348. doi:10.1016/j.scitotenv.2020.142348
Kuldip, S., Hundal, H. S., and Dhanwinder, S. (2011). Geochemistry and Assessment of Hydrogeochemical Processes in Groundwater in the Southern Part of Bathinda District of Punjab, Northwest India. Environ. Earth Sci. 64 (7), 1823–1833. doi:10.1007/s12665-011-0989-9
Li, P.-Y., Qian, H, Wu, J.-H., and Ding, J. (2010). Geochemical Modeling of Groundwater in Southern Plain Area of Pengyang County, Ningxia, China. Water Sci. Eng. 3, 282–291.
Loss, S. R., Loss, S. S., Will, T., and Marra, P. P. (2015). Linking Place-Based Citizen Science with Large-Scale Conservation Research: A Case Study of Bird-Building Collisions and the Role of Professional Scientists. Biol. Conserv. 184, 439–445. doi:10.1016/j.biocon.2015.02.023
Luzio, W., Sadzawka, A., Besoain, E., and Lara, P. (2003). Influencia de materiales volcánicos en la génesis de suelos arcillosos. Rev. Cienc. Suelo Nutr. Vegetal 3, 37–52.
Mahaqi, A., Moheghi, M. M., Mehiqi, M., and Moheghy, M. A. (2018). Hydrogeochemical Characteristics and Groundwater Quality Assessment for Drinking and Irrigation Purposes in the Mazar-I-Sharif City, North Afghanistan. Appl. Water Sci. 8, 133. doi:10.1007/s13201-018-0768-9
Martínez, D. E., and Osterrieth, M. (2013). Geoquimica De La Silice Disuelta En El Acuifero Pampeano En La Vertiente Sudoriental De Tandilia. Revista facultad de Ingeniería Universidad de Antioquia. IMPRENTA UNIV ANTIOQUIA ed. Medellin.
MME Ministry Of Environment (2017). Diagnostico y clasificacion de los cursos y cuerpos de agua segun objetivos de calidad Ministerio de Obras publicas Dirección General de aguas.
Montaña, E., Diaz, H. P., and Hurlbert, M. (2016). Development, Local Livelihoods, and Vulnerabilities to Global Environmental Change in the South American Dry Andes. Reg. Environ. Change 16, 2215–2228. doi:10.1007/s10113-015-0888-9
Municipalidad De Trehuaco (2018). Available: https://www.trehuaco.com/Wordpress/mas-de-80-familias-de-trehuaco-fueron-beneficiadas-con-estanques-para-la-acumulacion-de-agua/ (Accessed 04 12, 2018).
Munizaga, F., Aguirre, l., and Herve, F. (1973). Rb/Sr Ages of Rocks from the Chilean Metamorphic Basement. Earth Planet. Sci. Lett. 18, 87–92. doi:10.1016/0012-821x(73)90038-1
Munro, B. (2005). Statistical Methods for Health Care Research. Philadelphia: Lippincott Williams & Wilkins.
Naderi peikam, E., and Jalali, M. (2016). Application of Inverse Geochemical Modelling for Predicting Surface Water Chemistry in Ekbatan Watershed, Hamedan, Western Iran. Hydrological Sci. J. 61, 1124–1134. doi:10.1080/02626667.2015.1016947
Parkhurst, D., and appelo, T. (2013). Parkhurst DL, Appelo CAJ (2013) Description of Input and Examples for PHREEQC Version 3—a Computer Program for Speciation, Batch-Reaction, One-Dimensional Transport, and Inverse Geochemical Calculations. U. S. Geol. Surv. Tech. Methods. book 6, chap A43, p 497. Available only at http://pubs.usgs.gov/tm/06/a43.
Parkhurst, D. L., and Appelo, C. (1999a). A Computer Program for Speciation, Batch-Reaction, One-Dimensional Transport, and Inverse Geochemical Calculations. USGS report.
Parkhurst, D. L., and Appelo, C. A. J. (1999b). User's Guide to PHREEQC (Version 2): A Computer Program for Speciation, Batch-Reaction, One-Dimensional Transport, and Inverse Geochemical Calculations. Water-Resources Investigations Report.
Paul, J. D., Buytaert, W., Allen, S., Ballesteros-Cánovas, J. A., Bhusal, J., Cieslik, K., et al. (2018). Citizen Science for Hydrological Risk Reduction and Resilience Building. WIREs Water 5, e1262. doi:10.1002/wat2.1262
Peckenham, J. M., Thornton, T., and Peckenham, P. (2012). Validation of Student Generated Data for Assessment of Groundwater Quality. J. Sci. Educ. Technol. 21, 287–294. doi:10.1007/s10956-011-9317-0
Perevochtchikova, M., and Sandoval-Romero, G. E. (2016). Monitoreo comunitario participativo del agua en la periferia suroeste de la Ciudad de México. Investigaciones geográficas. doi:10.14350/rig.60063
Plummer, I. N., Busenberg, E., Glynn, P. D., and Blum, A. E. (1992). Dissolution of Aragonite-Strontianite Solid Solutions in Nonstoichiometric Sr (HCO3)2−Ca (HCO3)2−CO2-H2O Solutions. Geochimica Cosmochimica Acta 56, 3045–3072. doi:10.1016/0016-7037(92)90289-u
Plummer, l. N., Prestemon, E. C., and Parkhurst, D. L. (1994). An Interactive Code (NETPATH) for Modeling Net Geochemical Reactions along a Flow Path, Version 2.0. Water-Resources Investig. Rep. 94, 4169.
Reed, C. C., Winters, J. M., Hart, S. C., Hutchinson, R., Chandler, M., Venicx, G., et al. (2018). Building Flux Capacity: Citizen Scientists Increase Resolution of Soil Greenhouse Gas Fluxes. PLOS ONE 13, e0198997. doi:10.1371/journal.pone.0198997
Rivas, Y., Rivera, D., Gallardo, R., Lagos, E., Yevenes, M., Zambrano, F., et al. (2020). Water Availability, Quality, and use in Rural Communities of the Chilean Coastal Range. J. Soil Water Conserv. 75 (1), 75–90. doi:10.2489/jswc.75.1.75
Roa García, C. E., and Brown, S. (2009). Assessing Water Use and Quality through Youth Participatory Research in a Rural Andean Watershed. J. Environ. Manage 90, 3040–3047.
Roetman, P., and Daniels, C. (2011). The Benefits of Citizen Science in Research, Education and Community Engagement.
Ruppen, D., Chituri, O. A., Meck, M. L., Pfenninger, N., and Wehrli, B. (2021). Community-Based Monitoring Detects Sources and Risks of Mining-Related Water Pollution in Zimbabwe. Front. Environ. Sci. 9. doi:10.3389/fenvs.2021.754540
Santibañez, Q. (2016). El cambio climático y los recursos hídricos de Chile. Santiago: Ministerio de Agricultura. [Online]. Ministerio de Agricultura. Available: http://www.odepa.cl/wp-content/files_mf/1482956610RecursosHidricosCambioClimatico.pdf (Accessed 06 12, 2019).
SERNAGEOMIN, . (2003). Mapa Geológico de Chile: Versión digital. Geológica Digital, Servicio Nacional de Geología y Minería. https://portalgeominbeta.sernageomin.cl.
Sherbinin, A., Bowser, A., Chuang, T-R., Cooper, C., Danielsen, F., Edmunds, R., et al. (2021). The Critical Importance of Citizen Science Data. Front. Clim. 3, 20. doi:10.3389/fclim.2021.650760
Shupe, S. M. (2017). High Resolution Stream Water Quality Assessment in the Vancouver, British Columbia Region: a Citizen Science Study. Sci. Total Environ. 603-604, 745–759. doi:10.1016/j.scitotenv.2017.02.195
Stolpe, N. (2006). Descripciones De Los Principales Suelos De La Viii Región De Chile, Universidad de Concepción.
Tarhule, A. (2017). The Future of Water: Prospects and Challenges for Water Management in the 21st Century.
Thornhill, I., Chautard, A., and Loiselle, S. (2018). Monitoring Biological and Chemical Trends in Temperate Still Waters Using Citizen Science. Water 10, 839. doi:10.3390/w10070839
Urquiza, A., Amigo, C., Cortes Oggero, J., Billi, M., and Labraña, J. (2019). Gobernanza policéntrica y problemas ambientales en el siglo XXI: desafíos de coordinación social para la distribución de recursos hídricos en Chile, Persona y Sociedad. 33(1):133. doi:10.53689/pys.v33i1.258
Vohland, K., Land-Zandstra, A., Ceccaroni, L., Lemmens, R., Perelló, J., Ponti, M., et al. (2021). The Science of Citizen Science. Springer Nature.
Ward, M., Jones, R., Brender, J., De Kok, T., Weyer, P., Nolan, B., et al. (2018). Drinking Water Nitrate and Human Health: An Updated Review. Ijerph 15 (7), 1557. doi:10.3390/ijerph15071557
Weyhenmeyer, G. A., Mackay, M., Stockwell, J. D., Thiery, W., Grossart, H.-P., Augusto-Silva, P. B., et al. (2017). Citizen Science Shows Systematic Changes in the Temperature Difference between Air and Inland Waters with Global Warming. Sci. Rep. 7, 43890. doi:10.1038/srep43890
Yevenes, M. A., Figueroa, R., and Parra, O. (2018). Seasonal Drought Effects on the Water Quality of the Biobío River, Central Chile. Environ. Sci. Pollut. Res. 25, 13844–13856. doi:10.1007/s11356-018-1415-6
Yevenes, M. (2018). Monitoreo comunitario de la calidad del agua en la microcuenca Boca Itata, Trehuaco, Región del Ñuble, Chile. International G-WADI Seminar- Citizen Science for Water and Drought Management. Available at: https://en.unesco.org/climwar/citizen-science/water-drought-management.
Keywords: citizen science, water quality, community-based monitoring, PHREEQC model, rural communities
Citation: Yevenes MA, Pereira H and Bermudez R (2022) Citizen Science as a Co-Creative Measure to Water Quality: Chemical Data and Local Participation in a Rural Territory. Front. Environ. Sci. 10:940778. doi: 10.3389/fenvs.2022.940778
Received: 10 May 2022; Accepted: 17 June 2022;
Published: 21 September 2022.
Edited by:
Ramanathan Alagappan, Jawaharlal Nehru University, IndiaReviewed by:
Thilagavathi Rajendiran, Annamalai University, IndiaLinda Kay Silka, University of Maine, United States
Copyright © 2022 Yevenes, Pereira and Bermudez. This is an open-access article distributed under the terms of the Creative Commons Attribution License (CC BY). The use, distribution or reproduction in other forums is permitted, provided the original author(s) and the copyright owner(s) are credited and that the original publication in this journal is cited, in accordance with accepted academic practice. No use, distribution or reproduction is permitted which does not comply with these terms.
*Correspondence: Mariela A. Yevenes, bXlldmVuZXNAdWRlYy5jbA==