- 1ICAR Research Complex for NEH Region, Manipur Centre, Lamphelpat, India
- 2ICAR- Indian Institute of Farming System Research, Modipuram, Meerut, India
- 3Division of Agronomy, ICAR- Indian Agricultural Research Institute, New Delhi, India
Soil quality restoration and crop productivity maximization are the global challenge to feed the galloping population. The task is much more daunting in a risk-prone, fragile, and low productive hilly region due to the depletion of supporting and regulating ecosystem services. A five-year long-term (2012–2017) field experiment was conducted to stabilize the yield and soil quality through legume green manuring and crop residue recycling in intensified cropping systems in the Eastern Himalayan region of India. Four treatments involving three green manures [green gram (Vigna radiata); cowpea (Vigna unguiculata); Sesbania (Sesbania aculeata) along with control (no-green manure)], three cropping systems [groundnut (Arachis hypogaea)—pea (Pisum sativum); maize (Zea mays)—pea, and maize + groundnut–pea] and two levels of residue management practices [residue removal and residue retention] were evaluated in three times replicated split–split plot design. Among the green manure options, Sesbania exerted a significant positive impact on the soil organic carbon (SOC) stock, available micro- (Fe, Mn, Zn, and Cu), and macronutrients (N, P and K) in surface (0–0.15 m) and subsurface (0.15–0.45 m) soils. The improvement in soil enzymatic activities (acid phosphatase, alkaline phosphatase, dehydrogenase, beta-glucosidase, and aryl sulfatase activity) (p < 0.05) in Sesbania-treated soil was +28.1% to +38.9% in surface and +18.3% to +27.3% in subsurface soils over non-green manure. Sesbania-treated soils also exhibited higher soil quality index (SQI) and stratification ratio (SR) of available soil nutrients and enzymes over non-green manured soils. Among the cropping systems, groundnut intercropped with maize followed by peas (MGP) with in situ residue retention increased (p < 0.05) the available soil macro- and micronutrients, SOC stock, soil enzymes, SR, and SQI in comparison to other cropping systems. Sesbania green manuring and residue retention improved the yield sustainability by +19% and +11% in the MGP system over non-green manuring and residue removal, respectively. Therefore, Sesbania green manuring in the MGP cropping system along with residue retention is recommended for stabilizing the soil quality through enhancing supporting and regulating ecosystem services and maintaining long-term productivity in the fragile Eastern Himalayan ecosystem of India.
Introduction
Land degradation, irrational population growth, and urbanization are threatening the food security and ecosystem services of agroecology in many countries (Yadav et al., 2021). Factors associated with land degradation are mainly on account of over reliance of agri-food production systems on agrochemicals, accelerating the losses of regulating and supporting ecosystem services and deteriorating the environmental sustainability, which foster the challenges for achieving United Nations sustainable development goals (SDGs). Yield stagnation has become a major hurdle for food security and zero hunger (SDG–2). Similarly, reduction in soil ecosystem services and fertility is mainly caused by nutrient mining, soil erosion, imbalanced fertilization, poor nutrient recycling (residue recycling), lack of soil restoration practices, mono-cropping systems, etc. (Singh et al., 2021). It has become a challenge to address SDG–3 (good health and well-being) and SDG–15 (life on land). Eastern Himalayan Region of India, a habitat for ∼50 million populace, is suffering from poor farm productivity due to the tremendous pressure of land degradation (Singh et al., 2021). Large-scale adoption of mono-cropping generally results in yield stagnation (Babu et al., 2020; Ansari et al., 2022a), low farm income, and poor resource utilization (Sarkar et al., 2018). Therefore, soil quality stabilization has emerged as a major challenge to sustain soil fertility without reduction in crop productivity (Babu et al., 2020). In the hilly ecosystem of the North Eastern Himalayan (NEH) region, where the annual rainfall is high, cultivation along the steep slopes often results in the loss of soil organic carbon (SOC), nutrients, and soil binding agents. This causes a decline in soil aggregation and structural degeneration leading to soil erosion and environmental degradation (Choudhury et al., 2022).
Aluminum-induced soil acidity, intermittent moisture stress and soil degradation in the upland, and reduction of soil productive capacity are some of the major notable causes of low crop production in the Indian Himalayas (Meena et al., 2018). In acid soils, the reduction of H+ by organic anions to H2O and CO2 during the mineralization of organic manure increases the soil pH (Buragohain et al., 2017). Because of oxidation, Fe and Mn consume protons generated during the breakdown of organic matter and reduction of organic compounds may cause the soil pH to rise (Meena et al., 2018). Green manuring using legumes is easily decomposable and offers the extra benefit of fixing atmospheric N. Soil carbon storage, nutrient availability, biological aspects of soil, and yield stability could be possible only through sustainable management practices. Maintaining agronomic productivity and SOC levels is difficult in intensive cereal-based monoculture with minimal nutritional supplementation and poor soil management practices (Ansari et al., 2021; Ravisankar et al., 2022). In many parts of the world, the sequential cereal-based cropping systems with pulses in sole or inter-cropping considerably increase soil quality and productivity (Wander et al., 2019). Crop residue recycling and green manuring are used to improve and sustain the soil through long-term stabilization of SOC stock, soil biological properties including soil microbial biomass carbon (SMBC) and soil enzymatic activities, and soil chemical properties (macro- and micronutrients) (Yuan and Yue, 2012).
Therefore, the integration of fast-growing legumes with high nitrogen-fixing fecundity in cereal-based cropping systems as green manure has been considered a substitute and sustainable approach for restoring soil fertility and system productivity. Incorporation of crops at the active vegetative stage in soil could amplify the soil nutrient supplying capacity to the succeeding crops (Yadav et al., 2021). Nutrient pumping from deeper soil horizons to the furrow soil layer improves the crop yield after cultivation or incorporation of green manure crops (Stagnari et al., 2017). Green manures improved ecosystem services like soil physical properties, water holding capacity, which helps in reducing the soil moisture loss, improving soil organic matter and microbial activity, and also results in cooling effect (Melero et al., 2006). Green manuring also increased the quantity and quality of soil organic matter, thereby a suitable alternative to restore the quality of poor/degraded soils (Meena et al., 2018). The fast-growing, deep-rooted, high nitrogen-fixing, and low lignin-containing crops like Sesbania and cowpea are efficient in capturing and recycling nutrients (Das et al., 2021).
Hence, there is a need to explore the spatial and temporal intensification in existing cropping systems with fast growing legumes without compromising the land productivity and food security. Due to population pressure and land scarcity, small and marginal growers do not prefer the cultivation of green manures; hence, there is a need to sandwich short duration crops within the existing cropping systems. The probability of integrating green manures into the system becomes necessary when nutrient mining imbalances the soil quality stabilization due to inadequate management practices. The potential niche available in these cropping systems would be degraded due to the non-incorporation of legumes in the cereal rotation system (Amede and Taboge, 2007; Das et al., 2018). Therefore, biomass recycling becomes important to regulate and stabilize the soil quality with the improvement of soil biological (soil enzymatic activities), physical, and chemical properties (macro- and micronutrients).
Storage and stabilization of soil carbon, nutrients, and soil biological properties in subsurface soil are equally important as in surface soil. The presence of more than 60% of the maize vegetative roots in the 0.2–0.6 m soil layer indicated that subsurface soil interacts greatly with ground vegetation (Zhang et al., 2014). As a result, it is critical to understand the impact of long-term vegetation regeneration on changes in SOC stock and available macro- and micronutrients in the subsoil. In this respect, the stratification ratio (SR) is a very important criterion to evaluate the stabilization of soil properties in the subsoil. SR is the ratio of a surface layer property to a deeper layer property. Good soil quality is indicated by high SR values. The SR is extensively used as a farmland soil quality indicator (Peregrina et al., 2014).
However, systematic research on green manuring coupled with crop residue retention and its effect on ecosystem service restoration viz., SOC storage, soil enzymatic activities, and available nutrients in surface and subsurface soils, is limited especially in the regions where soil acidity is the major phenomenon in farming. We hypothesized that green manuring along with crop residue retention in groundnut intercropped with maize systems could improve the crop productivity as a result of improvement in ecosystem services. Further, studies on SRs are lacking, and evidence for using SRs to determine soil quality in the subsoil under manuring and residue recycling is needed. Therefore, to test the hypothesis, a field experiment was executed for five consecutive years (2012–2017) to assess the effect of legume green manuring and crop residue recycling in three different cropping systems involving combination cereal and legumes. We studied their effect on the vertical distribution of SOC storage, soil microbial enzymes, and nutrients and developed the soil quality stabilization indices like soil quality index (SQI) and SR. The findings of the study will help researchers and policy planners in the designing of ecosystem supportive policy for improving agronomic productivity while stabilizing soil quality in fragile hill ecosystem of the Eastern Himalayas.
Materials and methods
Description of the site, soil characteristics, and weather
The study site was in the Himalayan foothills of the Indian State of Manipur located in North Eastern Himalayan (NEH) region. Soils of these regions are mainly formed from sedimentary rocks, with parent materials from the Disang (Eocene) and Barail (Oligocene) groups of sandstone and shale (Ansari et al., 2022b). The sloping hill agriculture limits the cultivable land to 2.23 M ha (<12% of the total geographical area of Manipur) confined only to the intermontane valleys. Soil quality and crop productivity of hill ecosystem of NEH region are declining due to the burning of vegetation under jhum/shifting cultivation and it has become a challenge to fulfill the food requirement of the burgeoning population (Ansari et al., 2020). Rainfed paddy-based crop intensification in the lowlands was popularized to meet immediate food demand from limited farm lands. In the hill ecosystem, sole rainfed cereals (primarily rice and maize) are grown as mono-cropping throughout the rainy season (April to October), with only a minor periodic replenishment of plant nutrients from external organic or inorganic sources, typifying the region’s subsistence farming (Ansari et al., 2021).
Field study was conducted with fixed plots for five continuous years (2012–2017) at Langol Hill Research Farm (24ο49’ N latitude, 93ο55’ E longitude, and 786 m above MSL altitude) of the ICAR (Indian Council of Agricultural Research) Research Complex for NEH Region, Manipur Centre, Imphal, India. The climate of the study site is subtropical humid with the average minimum and maximum temperatures during the 5 years’ study period ranging between 14.8°C and 16.2°C and 26.8°C and 28.5°C, respectively. The mean monthly minimum and maximum relative humidities varied between 57.8–64% and 84.2–90.3%, respectively (Supplementary Figure S1). During the experimental period, the study area received an average annual rainfall of 1,545.5 mm. The soil of the experimental site was sandy clay loam in texture. Depth-wise information of different soil parameters (0–0.45 m) at the initiation of the study is given in Supplementary Table S1.
Treatment details and experimental design
The field experiment was conducted with three green manuring crops, viz., 1) GGM: green gram (Vigna radiata); 2) CGM: cowpea (Vigna unguiculata); 3) SGM: Sesbania (Sesbania aculeata); 4) control (NGM: no green manuring); and three cropping systems viz., 1) [groundnut (Arachis hypogaea)—pea (Pisum sativum) (GP); 2) maize (Zea mays)—pea (MP) and 3) maize + groundnut—pea (MGP)] involving two levels of residue management practices [residue removal (R-) and residue retention (R+)]. Green manuring, cropping systems, and residue management treatments were imposed as main plots, sub plots, and sub–sub plots, respectively, in a split–split plot design and replicated thrice. The dimensions of the main plots, sub plots, and sub–sub plots for each replication were 50.4 m × 12.6 m, 4.2 m × 12.6 m, and 4.2 m × 6.3 m, respectively.
Crop management
The main Kharif crops, i.e., maize (cv. HQPM–5) and groundnut (cv. ICGS–76), were manually sown as sole and intercrops during the month of June every year. Field for succeeding main crops (maize/groundnut) was prepared by two ploughings with power tiller followed by one planking. In residue retention plots, all the above-ground crop residues were retained in the field. For this, maize, groundnut, and pea crops were harvested, and above-ground residues were retained in the field after collecting their economic part. In the residue removal plots, residues of maize, groundnut, and pea were removed completely. Before sowing of the main crop in each of three cropping systems, three green manuring crops were sown in the respective plots by broadcasting after single ploughing and planking during the first week of April (65–70 days before sowing of main crops) with the pre-monsoon showers. Green manuring crops were chopped and incorporated 60 days after sowing and before field preparation for succeeding main crops in the respective plots. To compare the effect of green manuring on cropping system performance, and soil properties (soil nutrients and enzymes), one treatment without any green manuring crop was also maintained.
A spacing (row to row and plant to plant) of 60 cm × 30 cm for maize and 30 cm × 10 cm for groundnut was adopted under sole cropping. In maize + groundnut intercropping, one row of groundnut was sown between two rows of maize (60 cm) with 10 cm plant to plant distance. Maize and groundnut were harvested during the last week of October till the first week of November. During the winter season, pea ‘‘Rachna’’ was sown under zero tillage in all the plots during the 2nd week of November and harvested during the 3rd week of February. The recommended dose of fertilizers (RDF) [20–40–40 kg N—P2O5—K2O ha−1] was applied as a basal dose in groundnut. In sole cropping of maize and intercropping (maize + groundnut), the RDF was applied at 100–60–40 kg N–P2O5–K2O ha−1. In maize (sole and intercropping), 50% nitrogen and full dose of phosphorus and potassic fertilizers were applied as basal, and the remaining nitrogen (50%) was top-dressed in two equal splits at 30 and 55 days after sowing. The source of fertilizer N, P2O5, and K2O was urea (46% N), single super phosphate (16% P2O5), and muriate of potash (60% K2O), respectively. The experiment was conducted under rainfed conditions.
Soil sampling and analysis
Initial soil properties of experimental field were assessed before the commencement of the experiment (Supplementary Table S1). After completion of the experiment, soil samples were taken from three different depths (L1: 0–0.15 m, L2: 0.15–0.30 m, and L3: 0.30–0.45 m) using a bucket augur during the post-monsoon period (March 2017) from each plot. Three random spots in each plot were sampled, which were then composited to provide a representative soil sample. For the SMBC determination, fresh soil from each sample was kept at 4°C. For examination, the remaining soils were air-dried, crushed, and passed through a 2.0 mm mesh sieve. After oven drying at 105 ± 1°C, soil bulk density (ρb) was determined in situ using the core method (5.15 cm height and 4.7 cm diameter) (Blake and Hartge, 1986). Soil pH was determined using a 1:2.5 soil: water suspension (pH meter; Eutech pH 700-Eutech Instruments, Singapore). The available N (Av. N) was determined using the KMnO4 oxidation method (Subbiah and Asija, 1956), and the SOC content was determined using the K2Cr2O7 wet oxidation method (Walkley and Black, 1934). The available P (Av. P) was extracted using the Bray-1 reagent. The available K (Av. K) in soil extracted with neutral normal NH4OAc solution was determined using a flame photometer (ESICO-1382, India). The chloroform fumigation extraction method (Vance et al., 1987) was used to determine the SMBC. Modified universal buffer (MUB, pH 6.5 and 11) and p-nitrophenyl phosphate disodium salt (0.025 M) as a substrate were used to estimate the acid (ACP) and alkaline phosphatase (ALK) activity (Tabatabai and Bremmer, 1969). The activity of the β-glucosidase enzyme (GLU) was determined by using the Eivazi and Tabatabai’s (1988) method. Arylsulfatase activity (ARY) was measured with sodium acetate buffer and p-nitrophenyl sulfate (0.025 M) as a substrate (Tabatabai and Bremner, 1970). Soil dehydrogenase activity (DHY) was estimated by the method of Casida et al. (1964). A UV-VIS spectrophotometer (Spectroquant ® Prove 300, Germany) was used to measure the soil enzymatic activities. Lindsay and Norvell (1978) approach was used to estimate the available micronutrients (Fe, Mn, Zn, Cu) in soil. In a 10 g soil sample, 20 ml of 0.005 mol L−1 DTPA (diethylenetriamine pentaacetic acid) + 0.1 mol L−1 TEA (triethanolamine) + 0.01 mol L−1 CaCl2 (at pH 7.30) were added (Sarkar et al., 2018; Choudhury et al., 2021). The solution was centrifuged and filtered through Whatman No. 42 filter paper after being shaken for 2 hours at room temperature. DTPA-extractable micronutrient (available Fe, Mn, Zn, and Cu) concentrations were then determined using an atomic absorption spectrophotometer (AAS) on clear aliquots (Model Perkin Elmer A Analyst 200). For the determination of the total micro- and macro (P, K)-nutrients in plant tissues, 1 g of plant samples was ashed in a muffle furnace at 550°C for 3 h and subsequently extracted with 2 N HCl. The extract, after suitable dilution, was analyzed for Fe, Mn, Zn, and Cu using AAS; P (Vanadomolybdate yellow color method; Jackson, 1973) and K (Flame photometer). The total nitrogen concentration of plant tissues was determined by micro-Kjeldahl digestion and distillation (Nelson and Sommers, 1973). The total macro- (N, P, K) and micro (Fe, Mn, Zn, Cu)-nutrients added through green manuring/crop residues (maize/groundnut/pea) during 5 years (2012–2017) of the respective treatments are presented in Figure.1.
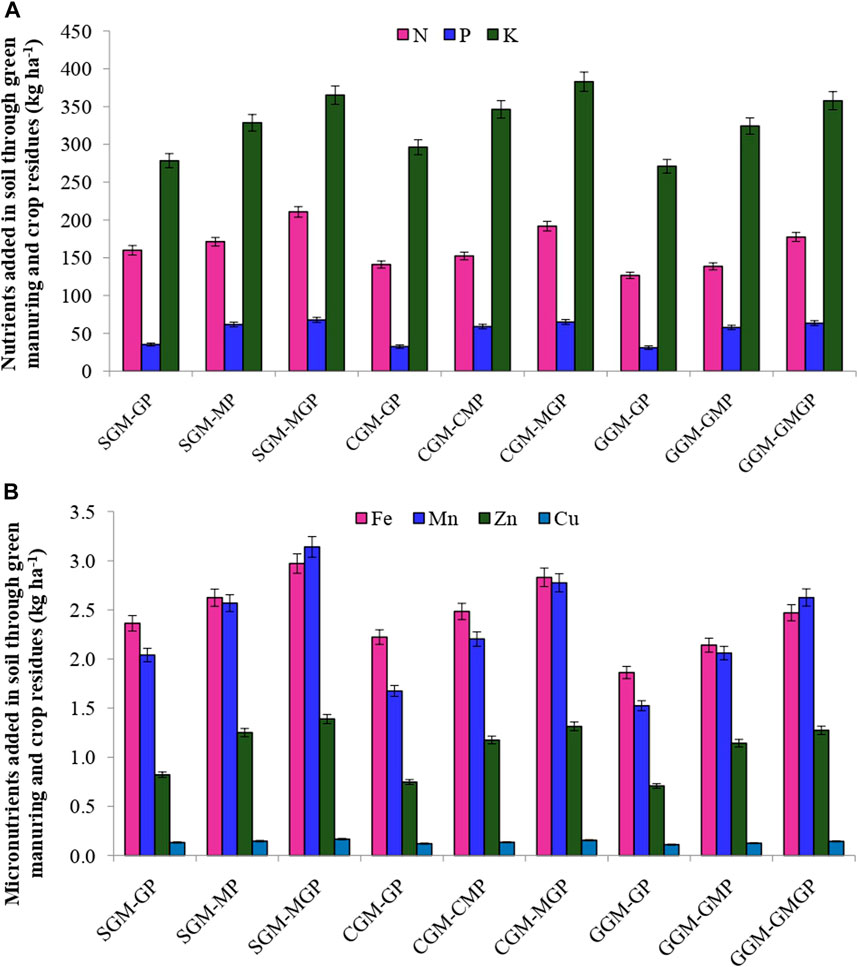
FIGURE 1. Nutrient input (A) macronutrients (N, P, K: Available nitrogen, phosphorus, and potassium, respectively), (B) micronutrients (Fe, Mn, Zn, Cu; DTPA-extractable soil micronutrients iron, manganese, zinc, and copper, years of experimentation. GGm: greengram green manuring, CGM: cowpea green manuring, SGM: sesbania green manuring, GP: groundnut–pea, MP: maize + groundnut–pea. Vertical bar (both way) represents the standard error of mean (p<0.05).
Soil quality index
The SQI was calculated for each farming system using the approach given by Banerjee et al. (2015). To summarize, all of the soil indicators studied (physical, chemical, and biological) were treated with principal component analysis (PCA) to reduce dimensionality while maintaining the most variation in the dataset. The largest variability was explained by the first PC, and the remainder of the residual variability was explained by the remaining PCs. Based on factor loading values, the essential underlying variables for each PC were discovered. Under each PC, the variables with absolute values less than 20% of the maximum weighted factor were kept. The correlation matrix was used to verify the inter-linkage of the extracted variables under respective PCs, and the most prominent variables from each PC were chosen for SQI development. After homothetic translation of each value within a mutual scale ranging from 0.1 to 1.0, the weighted addition for the final computation of SQI for each cropping system was computed individually.
Stratification ratio
SR is the value of a soil parameter at the overlying layer divided by the value at the underneath layer (L1/L2 and L1/L3) (Qua et al., 2020). The SR allows comparing the wide range of diversity of soils on the same assessment scale through internal normalization procedure by accounting for inherent soil differences. The SR of soil nutrients and soil enzymes are efficient indicators of soil quality, and its increase is related to the rate and amount of soil improvement through their addition (Franzluebbers, 2002).
Computation of sustainability index
Sustainability yield index was computed by using Eq. 1
where y, σ, and ymax represent the average yield of a treatment over the years, standard deviation, and observed maximum yield over the years.
Data analysis
Using the PROC GLM procedure in SAS version 9.2, the data generated from soil analysis and measuring grain yield were processed for analysis of variance (ANOVA) in a split–split plot design to test the statistical significance among the treatments (green manuring, cropping system, and residue management). LSD of the mean was computed based on Duncan’s multiple range test (DMRT) (p < 0.05) by using SAS 9.2 (SAS Institute, Cary, North Carolina, United States ). In DMRT, the values in a column that are followed by a similar letter in lowercase are not significantly different at the p < 0.05 level of significance. Principal component analysis (PCA) was used to reduce dimensionality while maintaining the most variation in the examined dataset by analyzing data on soil quality indicators (SOC stock, macro- and micro-nutrients, and soil enzymes) from various treatments. Variables with factor loadings and PCs with multiple eigen values were judged to be variables that best described system attributes. Therefore, PCs with eigen values more than 1.0 were considered for further analysis as these PCs were considered more informative than the others (Kaiser, 1960). The first PC explained maximum variability, and the rest of the PCs explained the remaining lion share for the residual variability (Supplementary Table S2). The NCSS 2020 programme was used to create the 3D surface plots.
Results
Soil enzymatic activities
Green manuring had a significant positive effect on soil enzymatic activities. Maximum and significant improvement of the enzymatic activities was recorded under SGM compared to that in other green manures and NGM treatment. SGM harbored 28.3%–38.9%, 16.4%–27.5%, and 18.2%–27.9% more enzymatic activities in 0–0.15 m, 0.15–0.30 m, and 0.30–0.45 m soil layers, respectively, as compared to NGM treatment (Table 1). As shown in Table 1, soil enzymes acid phosphatase (ACP), alkaline phosphatase (ALK), dehydrogenase (DHY), beta-glucosidase (GLU), and arylsulfatase (ARY) activities were improved by 16.0%–28.3%, 16.0%–28.3%, 20.6%–31.3%, 12.5%–28.1%, and 19.7%–38.9%, respectively, in 0–0.15 m soil layer over NGM treatment. Higher enzymatic activities were recorded in the topsoil layer (0–0.15 m) than in subsurface soil (0.15–0.45 m). The relative increase in the topsoil layer being almost twice as compared to the subsurface soil layer (Table 1).
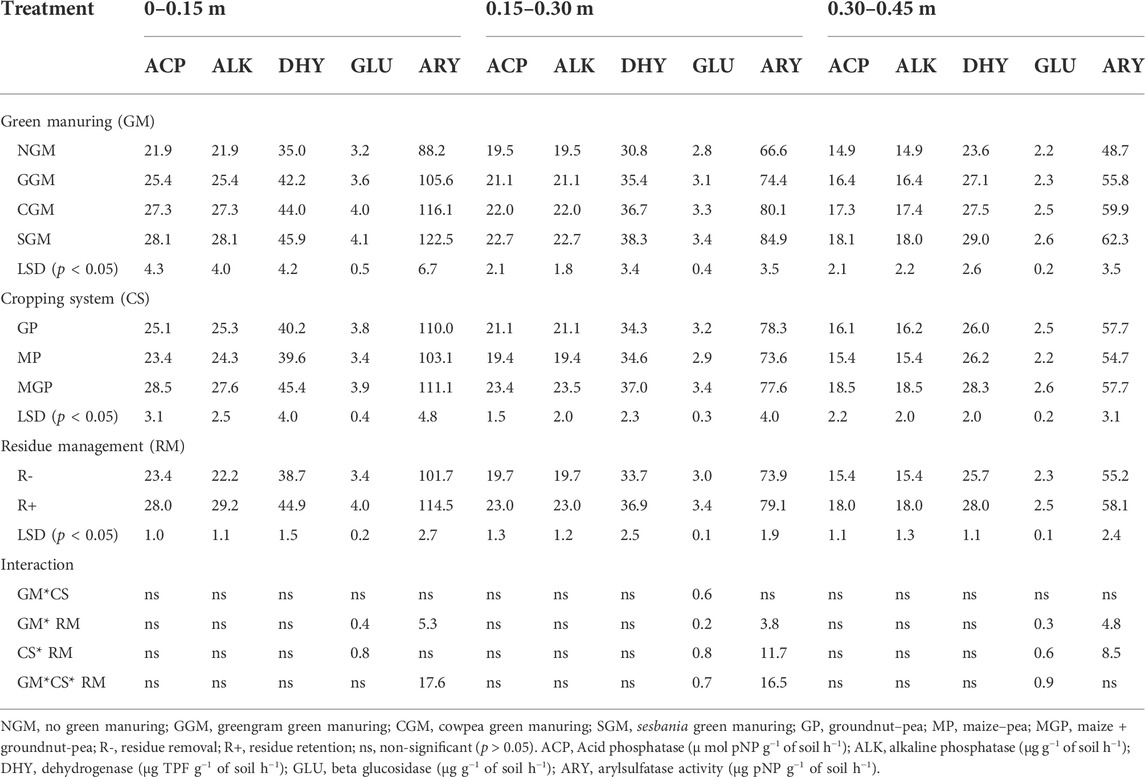
TABLE 1. Effect of green manuring, cropping systems, and residue management on soil enzymes in different soil depths after 5 years of experimentation.
MGP cropping system significantly improved the soil enzymes acid phosphatase, alkaline phosphatase, dehydrogenase, beta-glucosidase, and arylsulfatase activity by 13.5%–21.8%, 9.1%–13.6%, 12.9%–14.6%, 2.6%–14.7%, and 1–7.8%, respectively, in surface (0–0.15 m) soil layer compared to GP and MP systems. On an average, the highest soil enzymes in the subsurface (0.15–0.45 m) soil layer were also recorded in the MGP (ACP: 21.0 µ mol pNP g−1 of soil h−1, ALK: 21.0 μg g−1 of soil h−1, DHY: 32.7 µg TPF g−1 of soil h−1, GLU: 3.0 μg g−1 of soil h−1 and ARY: 67.7 μg pNP g−1 of soil h−1) cropping system. The enzymatic activities decreased in the subsurface soil layer, as compared to the surface soil layer (Table 1).
The residue retention-mediated soil enzymatic activities (ACP, ALK, DHY, GLU, and ARY) were significantly (p < 0.05) higher in the surface (12.6%–31.5%) and subsurface soils (6.1%–16.8%) (Table 1).
Nutrient storage
The highest macronutrient (available N, P, and K) storage was recorded in SGM in the surface layer as compared to other green manuring treatments. The similar trend was also found in the subsurface layer under SGM over the remaining green manures. Green manuring (Sesbania, cowpea, and greengram) increased the available N, P, and K significantly by 16.6%–29.9%, 15.4%–31.5%, and 16.3%–26.0%, respectively, in 0–0.15 m soil layer as compared to NGM. Similarly, macronutrients (available N, P, and K) also increased significantly by 12.4%–22.7%, 9.9%–24.8%, and 11.1%–19.4% in 0.15–0.30 m soil layer as well as by 11.3%–21.5%, 10.4%–23.9%, and 13.6%–20.8% in 0.30–0.45 m soil layer compared to non-green manuring (Table 2). SGM highly improved the micronutrient (available Fe, Mn, Zn, and Cu) storage in the surface soil layer (0–0.15 m) as compared to the other green manuring tested. These changes manifested the highest in the top soil layers as compared to the subsurface soil layers. The available Fe, Mn, Zn, and Cu storage increased by 11.8%–27.1%, 8.45–25.3, 21.1–36.8, and 9.5%–28.8%, respectively, in 0–0.15 m soil layer over the NGM treatment. However, it decreased in the subsequent soil layers (0.15–0.30 and 0.30–0.45 m) compared to the topsoil layer (0–0.15 m) (Table 3).
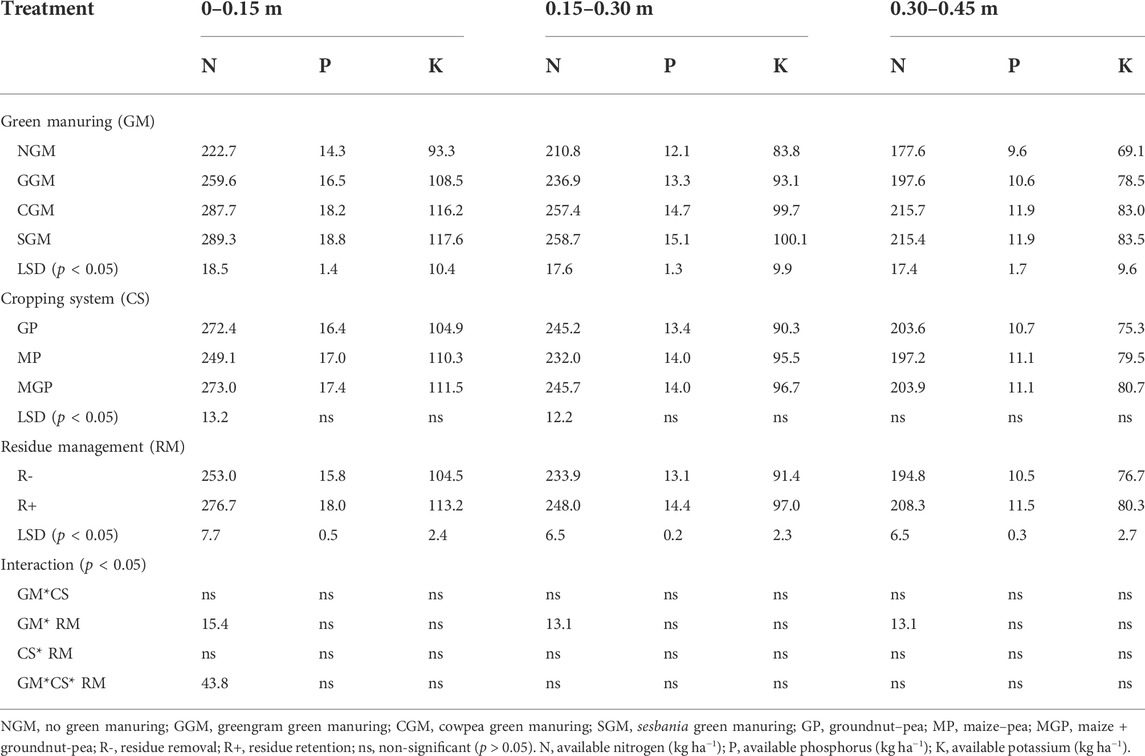
TABLE 2. Effect of green manuring, cropping systems, and residue management on the nutrient storage of available N, P, and K in different soil depths after 5 years of experimentation.
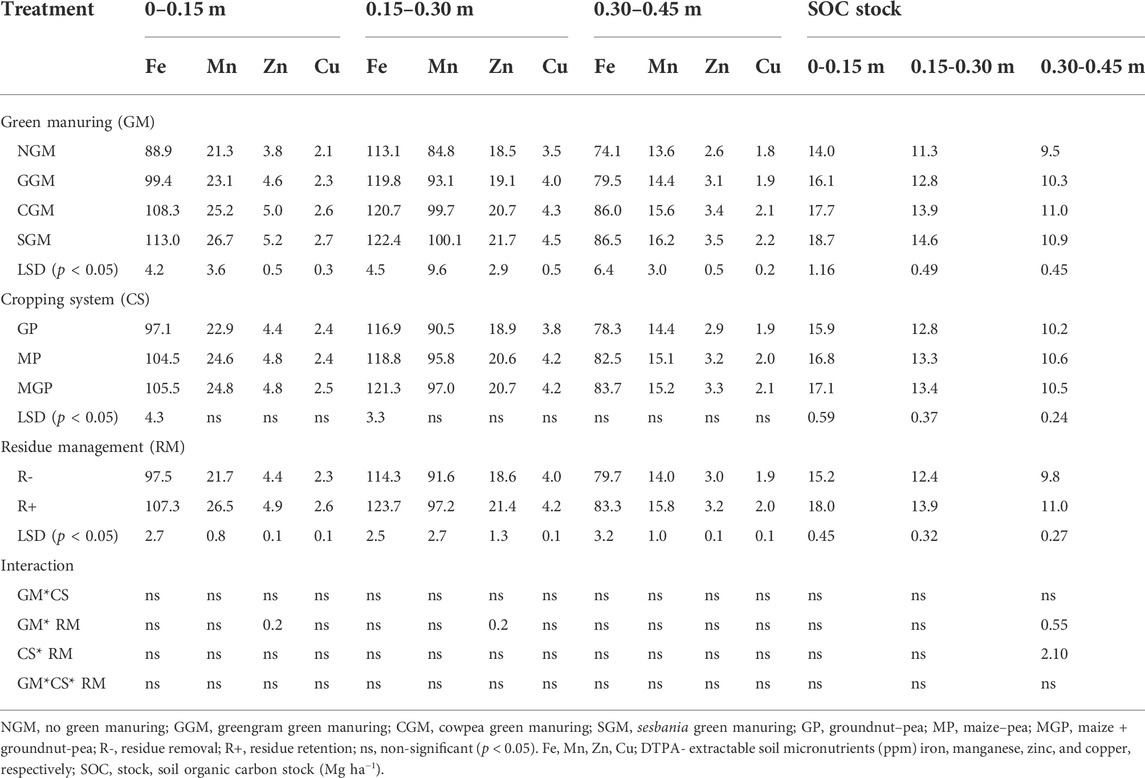
TABLE 3. Effect of green manuring, cropping systems, and residue management on micronutrients and soil organic C storage in different soil depths after 5 years of experimentation.
Averaged over 5 years, the maize + groundnut–pea and groundnut–pea cropping system increased the available N by 9.4%–9.6% and 5.7%–5.9% in 0–0.15 and 0.15–0.30 m soil layers, respectively, over the maize–pea cropping system, while the non-significant difference was recorded in 0.30–0.45 m soil layer. There was no significant improvement of available P and K in all three soil layers (Table 2). The available Fe concentration increased significantly more in the surface (0–0.15 m) soil layer in maize + groundnut–pea and maize–pea (by 8.7% and 7.1%, respectively) than groundnut–pea cropping system, while the maize + groundnut–pea system significantly influenced the available Fe concentration only in 0.15–0.30 m soil layers (by 3.8%) over groundnut–pea system. The remaining micronutrients (available Mn, Zn, and Cu) did not significantly differ due to the cropping systems in the surface and subsurface (0.15–0.45 m) soil layers (Table 3).
Residue retention resulted in significantly higher (p < 0.05) available N, P, and K concentrations in surface soil (0–0.15 m) by 9.4%, 13.9%, and 8.3%, respectively, over residue removal. Notably, residue retention had also higher (p < 0.05) available N, P, and K concentrations in subsurface soil (0.15–0.45 m: 6.5%, 9.7%, 5.4%, respectively) as compared to residue removal after 5 years of experimentation. Similarly, residue retention had significantly higher micronutrient concentration (available Fe, Mn, Zn, and Cu) over residue removal and increased by 10.1%, 22.1%, 11.4%, and 13.0% in surface soils and by 6.4%, 9.5%, 10.9%, and 5.1% in subsurface soils. The surface soil (0–0.15 m) had significantly higher available micronutrient concentration than the subsoil layers, and it decreased linearly with an increase in soil depth (Table 3).
SOC stock
The highest SOC stock was recorded in SGM (18.7, 14.6, and 10.9 Mg ha−1, respectively, in L1, L2, and L3 layers) compared to the remaining green manuring practices. Green manuring compared with no green manuring significantly increased the SOC stock by 15%–33.6%, 13.3%–29.2%, and 8.4%–14.7%, respectively, in L1, L2, and L3 soil layers (Table 3). MGP and MP increased the SOC stock by 5.4%–7.5%, 3.8%–4.7%, and 2.9%–3.8% in the L1, L2, and L3 soil layers over the GP cropping system, respectively. SOC stock was increased by 18.4% to 12.1% in surface and subsurface soils, respectively, due to residue retention over residue removal (Table 3).
Stratification ratio
Among the green manuring treatments, the highest SR1 was recorded in Sesbania followed by cowpea and greengram. Notably, the SR2 of available N, P, and K between the surface (L1) to bottom-most layer (L3) was higher in Sesbania followed by cowpea and greengram (Figure 2). The highest SR of micronutrients (SR1: 1.14–1.24) and SR2 (1.28–1.67) was recorded in Sesbania green manuring followed by cowpea > green gram green manuring treatment (Figure 2). Green manuring (Sesbania > cowpea > greengram) increased (p < 0.05) the SR1 and SR2 on an average by 8.71>8.14>8.71% and 6.15>7.44>8.32%, respectively, over the NGM treatment. However, across the green manuring treatments (Sesbania > cowpea > greengram), ARY was highly (SR1: 1.42 to 1.45, SR2: 1.91–1.99) stratified enzyme than the ALK > ACP > DHY > GLU (Figure 2). SR in the cropping system did not differ significantly; however, higher SR1 and SR2 of available macro- and micronutrients as well as soil enzymes were recorded in the MGP cropping system (Supplementary Figure S2).
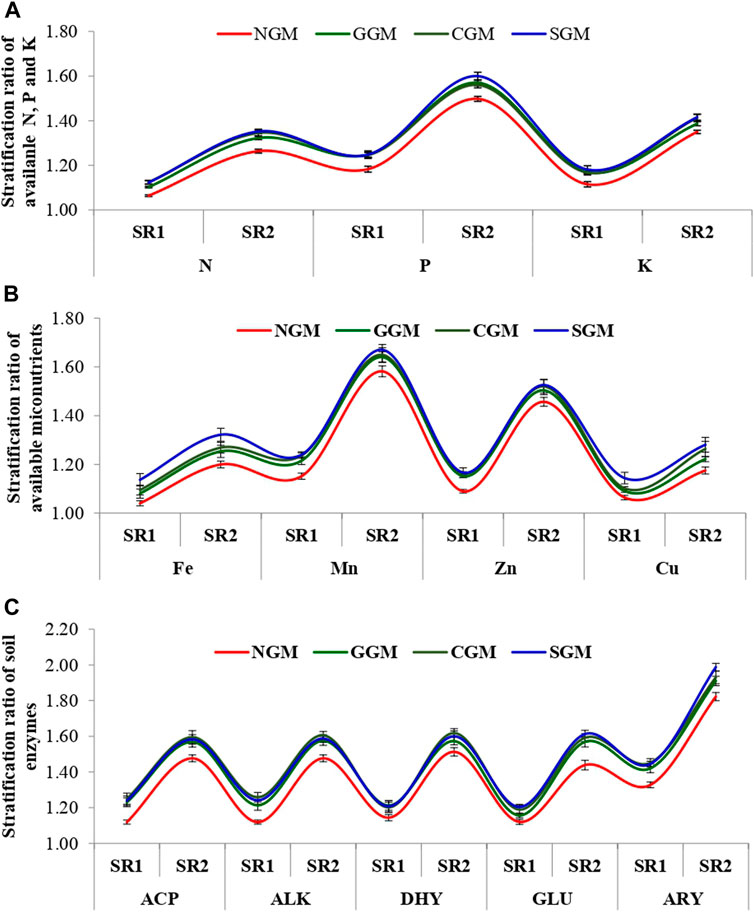
FIGURE 2. Stratification rations of (A) available N, P, and K (B). Micronutrients Fe, Mn, Zn, and Cu (C). Soil enzymes as influenced by green manuring after five years of experimentation. NGM: No green manuring, GGM: greengram green manuring, CGM: cowpea green manuring, SGM: sesbania green manuring, R-: residue stratification ratio (ratio of 0.15–0.30 and 0.30–0.45 m soil depth) ACP: Acid phosphates (ug TPF g-1 of soil h-1), ALK: Alkaline phosphates (ug g-1 of soil h-1), DHY: Dehydrogenase (ug TPF g-1 of soil h-1), GLU: beta glucosidase (ug g-1 of soil h-1), ARY: arylsulfatase activity (ug pNp g-1 of soil h-1) vertical bar (both way) represents the standard error of mean (p < 0.05).
The SR was significantly (p < 0.05) influenced by residue retention. The estimated SR1 of available N (+3.1%), P (+3.7%), and K (+2.0%) were higher (p < 0.05) in residue retention than in the residue removal treatment. Similarly, the estimated SR2 of available N, P, and K were higher (1.33, 1.59, and 1.41) in residue retention as compared to residue removal (1.31, 1.53, and 1.37), respectively (Figure 3). The SR1 estimated for micronutrients (available Fe, Mn, Zn, and Cu) were higher (+3.6% to +6.2%) in residue retention than in the residue removal. SR2 of available micronutrients increased most for the Mn (+8.7%) followed by Cu > Fe > Zn in residue retention than in the residue removal treatment. Similarly, SR1 and SR2 of soil enzymes (ACP, ALK, DHY, GLU, and ARY) increased by 2.2%–6.1% and 1.8%–8.2%, respectively, in residue retention than in the residue removal treatment (Figure 3).
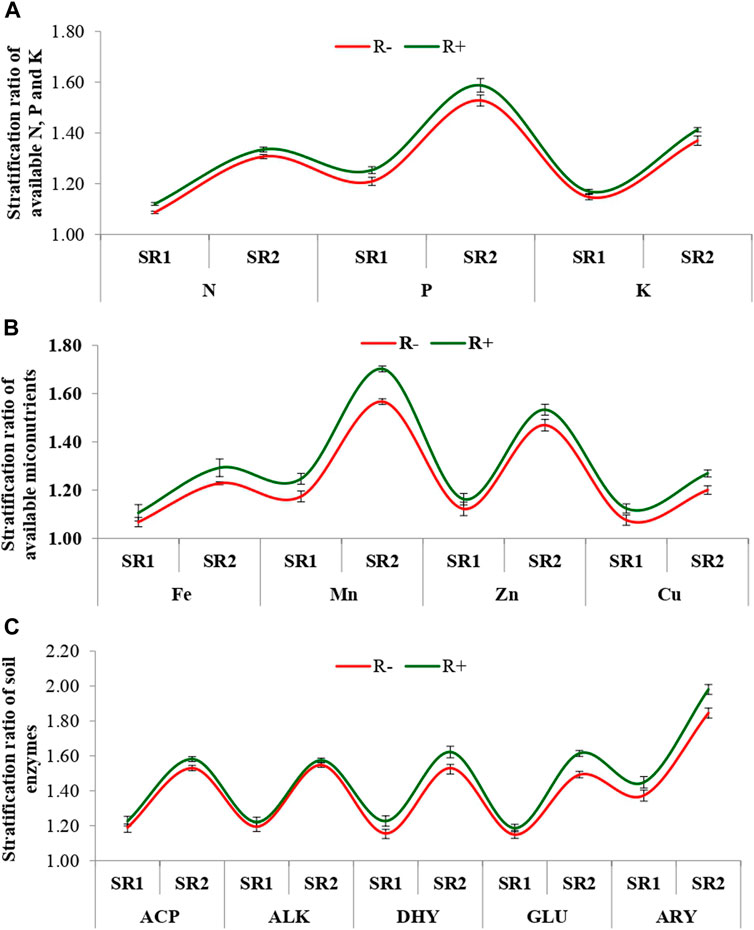
FIGURE 3. Stratification ratios of (A). Available N, P, and K (B). Micronutrients Fe, Mn, Zn, and Cu (C). Soil enzymes as influenced by residue management after five years of experimentation. NGM: No green manuring, GGM: greengram green manuring, CGM: cowpea green manuring, SGM: sesbania green manuring, R-: residue removal, R+: residue retention SRI: stratification ratio (ratio of 0–0.15 and 0.15–0.30 m soil depth), SR2: stratification ratio (ratio of 0–0.15 and 0.15–0.30 m soil depth). ACP: Acid phosphates (u mol pNP g-1), GLU: beta glucosidase (ug g-1 of h-1), ARY: arylsulfatase activity (ug pNP g-1 of soil h-1). Vertical bar (both way ) represents the standard error of mean (p<0.05).
Soil quality index
For deriving SQI, available macronutrients (N, P, and K), micronutrients (Fe, Mn, Zn, and Cu), and soil enzyme (ACP, ALK, DHY, GLU, and ARY) datasets were subjected to PCA analysis. In Figure 4 and Supplementary Table S2, the first and second PCs explained 76.48% and 8.82% of variability with eigen values of 11.47 and 1.32, respectively. Therefore, the eigen values of two PCs were ≥1, which explained 85.30% of the cumulative variability. The highly weighted factors were explained (>0.8) with all the variables in the first principal component (PC-1) except GLU, which has less than 0.8 factor loading (0.714). SOC stock has shown a strong positive correlation with other soil quality indicators and it was retained in PC-1 as minimum dataset (MDS). In the PC-2, GLU (0.539) was selected with the highest loading factor. Thus, SOC and GLU were selected as MDS for the calculation of SQI. The maximum improvement of the SQI was recorded under SGM in surface (SQIs: 0.547) and subsurface (SQIss: 0.525) followed by CGM and GGM as compared to no green manuring treatment (SQIs: 0.403 and SQIss: 0.422). The maximum SQI in surface (SQIs: 0.521) and subsurface (SQIss: 0.500) soil was recorded in the MGP cropping system as compared to MP and GP systems. Similarly, residue retention significantly increased the SQI by 15.7% and 8.1%, respectively, in surface and subsurface soil over residue removal treatment (Figure 5).
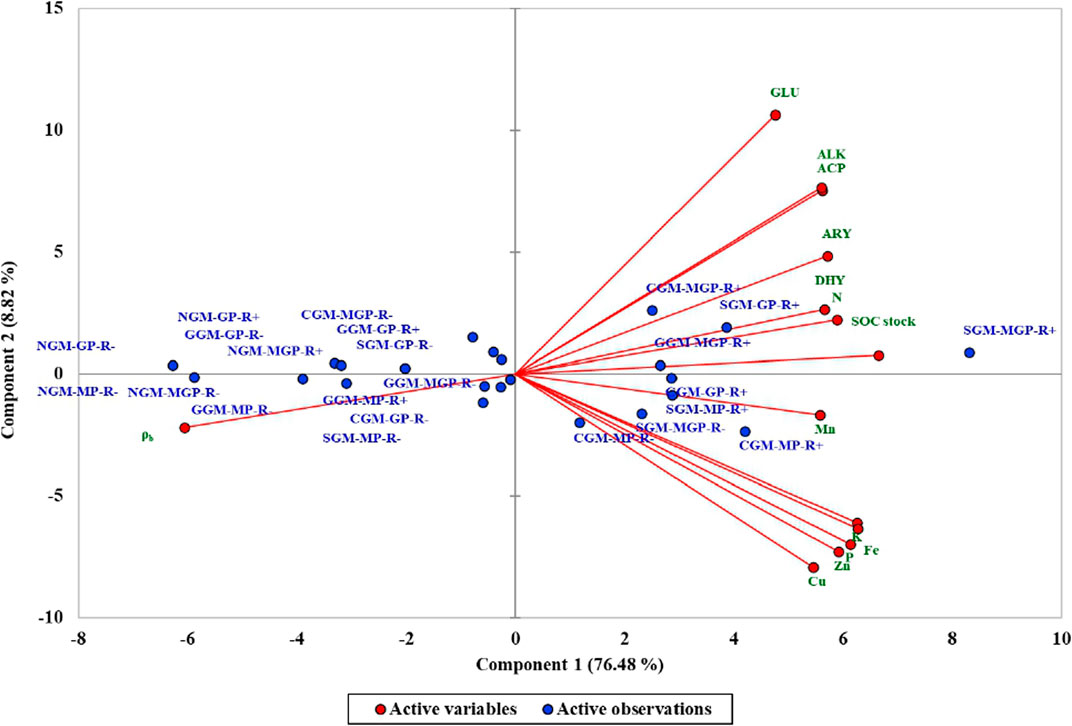
FIGURE 4. Principal component analysis (PCA). of system productivity and soil variables for treatment combinations of green manuring, cropping system, and residue management practices. NGM: No green manuring, GGM: greengram green manuring, CGM: cowpea green manuring, SGM: sesbania green manuring, GP: groundnut–pea, MP: maize–pea, MGP: maize + groundnut–pea, R-: residue removal, R+: Available phosphorus, K: Available potassium, Fe, Mn, Zn, Cu: DTPA-extractable soil micronutrients iron, manganese, zinc, and copper, respectively. ACP: Acid phosphates (u mol pNP g-1 of soil h-1), ALK: Alkaline phosphates (ug g-1 of soil h-1), DHY: Dehydrogenase (ug TPF g-1 of soil h-1) GLU: beta glucosidase (ug g-1 of soil h-1), ARY: arylsulfatase activity (ug pNP g-1 of soilh-1); SOC stock: soil organic carbon stock.
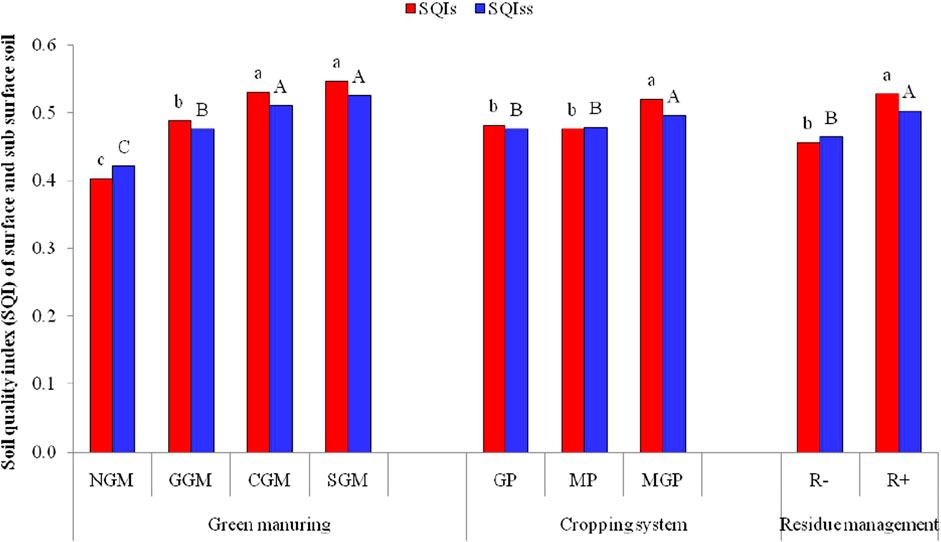
FIGURE 5. Soil quality index of surface (0–0.15 m) and subsurface soil (0.15–0.45 m) as influenced by green manuring, cropping systems, and residue management Green manuring, cropping system, and residue management followed by different letters in surface (a–c) and subsurface (A–C) soil is significantly different at (p < 0 .05). (DMRT: Duncan’s Multiple Range Test). NGM: No green manuring, GGM: greengram green manuring, CGM: cowpea green manuring, SGM: sesbania green manuring, GP: groundnut–pea, MP: maize–pea, MGP: maize + groundnut-pea, R-: residue removal, R+: residue retention, SQIs: Soil quality index of surface soil, SQIss: Soil quality index of subsurface soil. Different lowercase letters in the same color columns (for each main factor are significantly different at p < 0.05).
The surface plot analysis revealed a strong positive relationship between SQIs and SQIss with maize grain yield in sole cropping (r =0.9612** and 0.9902**, p < 0.01) as well as intercropping (r =0.8673 and 0.8461, p < 0.01); pod yield of groundnut in sole cropping (r =0.8991** and 0.8893**, p < 0.01) as well as intercropping (r =0.8797**, p < 0.01) (Figure 6). Grain yield of pea was also strongly correlated with SQIs and SQIss (r =0.8739** and 0.8732, p < 0.01). The favorable relationship between soil parameters and grain/pod yield has a significant impact on maize/groundnut/pea system productivity.
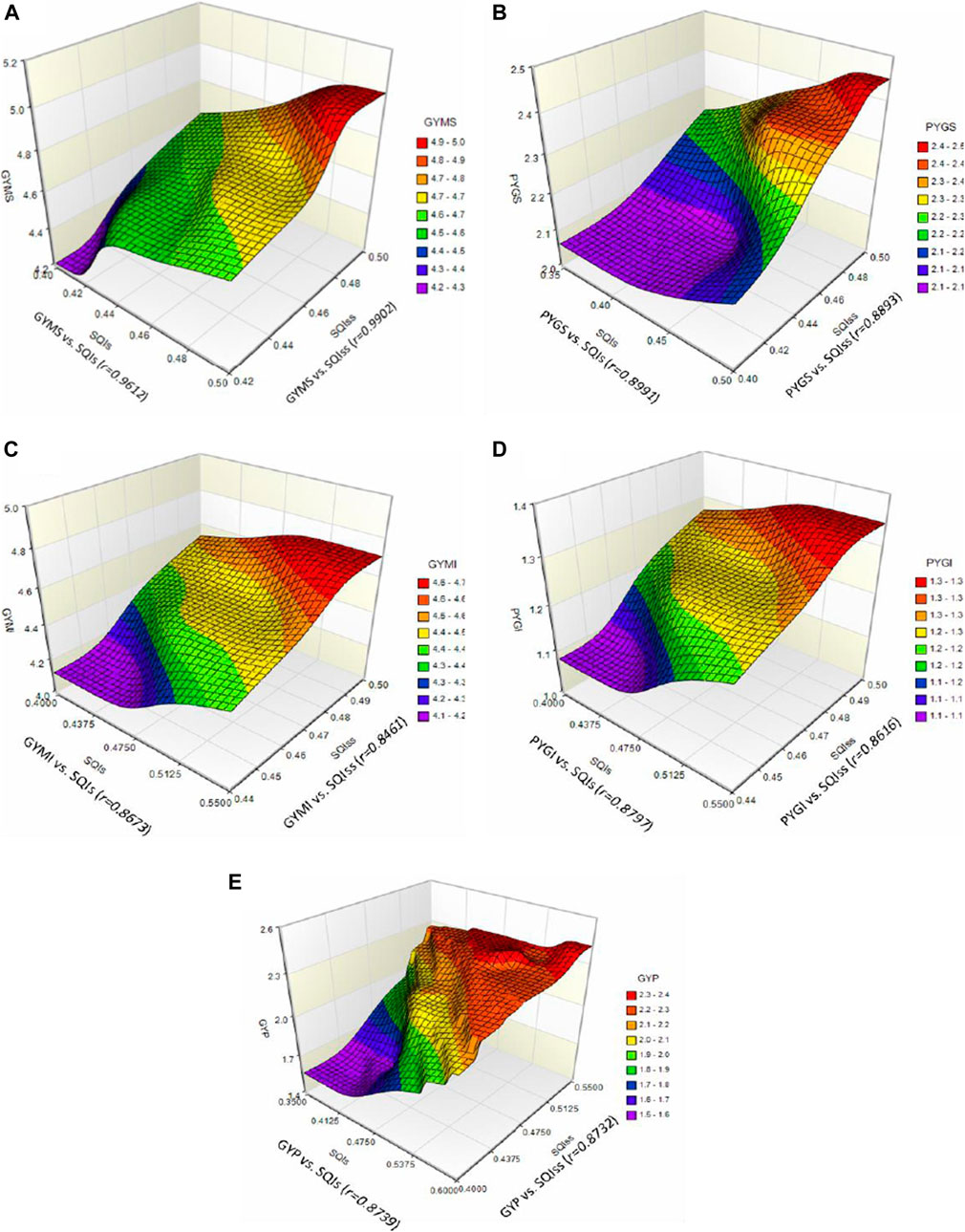
FIGURE 6. 3D surface plots of relationship (r) between soil quality index of soil (SQIs, 0–0.15 m) and subsurface soil (SQIss, 0.15–0.45 m) with mean maize/groundnut yield after five years of experimentation, (A) grain yield of maize sole crop (GYMS, Mg ha-1), (B) pod yield of groundnut sole group (PYGS, Mg ha-1), (C) grain yield of intercropped maize (GYMI, Mg ha-1), (D) pod yield of intercropped groundnut (PYGI, Mg ha-1), (E) grain yield of pea (GYP, Mg ha-1).
Sustainability yield index
Among the green manuring treatments, SYI was significantly (p < 0.05) higher in Sesbania green manuring (0.80) followed by cowpea (0.74) and green gram green manuring (0.73) as compared to non-green manuring (0.67) treatment. Similarly, groundnut intercropped with maize followed by pea cropping system observed the highest (p < 0.05) SYI (0.787) followed by groundnut–pea (0.74) and maize–pea (0.67) cropping systems. Residue retention resulted in significantly higher (p < 0.05) SYI (0.78) as compared to residue removal (0.70) treatment (Figure 7).
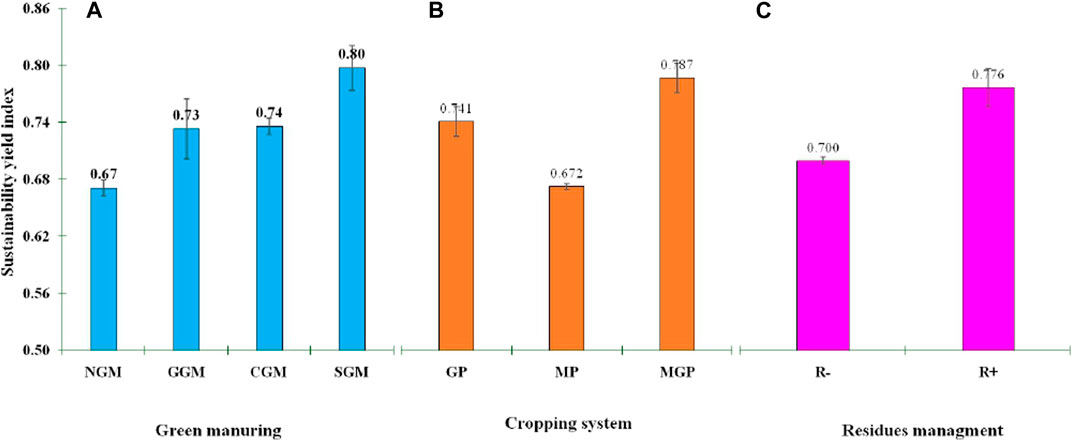
FIGURE 7. Sustainability yield index as influenced by (A) green manuring, (B) cropping systems, (C) residue management NGM: No green manuring, GGM: greengram manuring, CGM: cowpea green manuring, SGM: sesbania green manuring, GP: groundnut–pea, MP: maize–pea, MGP: maize + Groundnut–pea, R-: residue removal, R+: residue retention. The vertical bars represent p<0.05 significant level.
Discussion
Soil enzymes, nutrients, and C stock
Ecologically viable agricultural management practices are crucial in maintaining supporting and regulating ecosystem services (Baveye et al., 2016; Singh et al., 2021). Soil quality is a set of specific soil parameters that are important for long-term agricultural production and ecosystem health (vegetation and soil) (Karlen et al., 2001). In tropical and subtropical soils, biomass addition, either through green manuring (leguminous) or crop residue retention in the soil, is known to build up SOC accumulation and improve soil biological function (enzymatic activities) (Lungmuana, et al., 2019). Our findings showed that using Sesbania green manure along with residues retained from maize + groundnut followed by pea cropping system resulted in the highest overall biomass accumulation (22.84 Mg ha−1 annum−1) and supplied a significant quantity of C to the soil (>9.58 Mg ha−1 annum−1). Because of its quick growth behavior, Sesbania aculeata and maize along with legumes (groundnut and pea) in the cropping system are known to have increased shoot and root biomass, resulting in a substantial (p < 0.05) increase in SOC stock (Ansari et al., 2022b). Soil nutrients and enzymes are greatly affected by several factors like land-use patterns, type of vegetation, crop residue recycling, green manuring, and soil management practices (Huang et al., 2016). Intensive crop cultivation without proper nutrient application and input addition to the soil reduces SOC stock and nutrient concentrations and imposes adverse effects on the soil physico-chemical and biological properties (Choudhury et al., 2021). In our findings, it is reported that the consecutive 5 years of Sesbania green manuring in the maize + groundnut-pea cropping system added +210.7, +67.8, +365.2, +3.0, +3.14, +1.39, and +0.17 kg ha−1 N, P, K, Fe, Mn, Zn, and Cu, respectively (Figure 1), in an experimental plot. The highest concentration of macro- and micronutrients in surface and subsurface soil under the MGP cropping system coupled with SGM may be attributed to nutrient addition and recycling ultimately improving soil nutrient (macro- and micro) concentrations. The addition of biomass either through green manuring with leguminous crops or residue retention is known to increase SOC build-up, and nutrient concentration in the soils (Nath et al., 2019; Choudhury et al., 2021). Stabilization of soil C and nutrients will help to reduce the CO2 emission to the atmosphere and can address several SDGs such as climate action (SDG-13), life on land (SDG-15) through maintaining soil microbial diversity, which is an essential component of growing crops for human and livestock on the Earth. Sesbania is a leguminous crop that has a narrow C:N ratio (23.5:1.0), thereby enhancing the residue decomposition and release of nutrients and harbors potential enzymatic activities (Babu et al., 2020; Yadav et al., 2021). The retention of moisture and ambient temperature in the surface soil due to crop cover in Sesbania incorporated plots may have created a favorable environment for increased microbial decomposition, resulting in faster decomposition and nutrient release. Ma et al. (2021) concluded in their meta-analysis on the effect of green manure on nutrient availability that among different kinds of green manures, leguminous green manure significantly increased nitrate and hydrolysable nitrogen, whereas non-legume green manure significantly improved soil potassium. Amede et al. (2021) obtained an 18–26% increased wheat yield from the plots green manured with vetch and lupin over the fertilizer treatments due to the improved soil water status, improved P availability, notably increased exchangeable K, Ca, and Mg, and increased pH by about 0.5 units. The increase in soil C relies on the balance between the addition of C inputs (root exudates, root biomass, crop residues) and C losses (respiration by soil biota, erosion, etc.). It is also influenced by the amount of residue accumulated, the quality of residue, and the rate of decomposition (Chen et al., 2020; Almagro et al., 2021). The incorporation of green manure biomass significantly improves the soil enzymatic activities (Sürücü et al., 2014). The inclusion of nutrient-rich leguminous crops and their incorporation as green manure biomass into the soil under cereal-based cropping systems safeguard nutrients available to subsequent crops, improve the carrying capacity, and make the system viable and sustainable (Ansari et al., 2021). Ma et al. (2021) conducted a meta-analysis of the effect of green manure in China and concluded that green manure significantly improves soil quality by reducing bulk density (approximately by 5.6%) and improving soil enzymatic activities (14–39%). This study notably suggests that the incorporation of Sesbania as green manure in the MGP cropping system along with crop residue retention could increase the nutrient concentration in soil and harbor more soil enzymatic activity.
Vertical nutrient distribution, soil enzymes, and stratification ratio
Sesbania aculeata has fast growth behavior, and it is well known for the higher shoot and root biomass, resulting in a significant (p < 0.05) improvement in nutrient content and enzymatic activities in surface and subsurface soil (Ansari et al., 2021). Ansari et al. (2016) reported that maize roots were found in 1:3 ratio in the surface (0–0.20 m) and subsurface (0.20–0.40 m) soils, respectively. The significant amount of biomass of Sesbania aculeata and residue retention of the MGP cropping system improved the SOC stock, soil available nutrients (macro and micro), and soil enzymatic activities in the surface followed by subsurface soil as compared to no green manuring with residual removal. In another study, Hirte et al. (2018) reported that 186 g m−2 root biomass might have stronger influence on soil properties. The fine roots were significantly higher in the subsurface layer as compared to that in the surface layers, which could be explained by the decrease in root diameter and length as the soil depth increased (Zhang et al., 2021). The higher carbon accumulated from higher biomass in surface soil provides the higher energy source for microbes, which improves the enzymatic activities (Das et al., 2021). Higher enzymatic activities indicate the good quality of soil, which is directly related to soil carbon and biomass accumulation (Meetei et al., 2020). The vertical distribution of soil enzymes was also affected due to the quality and quantity of biomass accumulation. Reduction in soil enzymatic activities in the subsurface layer could be due to a decrease in C input deposition from the land use-mediated addition of plant residues, such as root biomass (Meetei et al., 2020). However, a continuous drop in soil C stocks in deeper soil depths could explain the decrease in soil enzymatic activities and nutrients as soil depth increased (Lungmuana et al., 2019).
In this study, higher SR of nutrients (macro: N, P, K and micro: Fe, Mn, Zn, Cu) and soil enzymes (ACP, ALK, DHY, GLU, and ARY) were observed with SGM > CGM > GGM > NGM. Similarly, residue retention enhanced the SR of nutrients and enzymes as compared to residue removal in the MGP cropping system, which might be attributed to higher biomass and residue-mediated nutrient concentration in soil (Qua et al., 2020). Higher SR of nutrients and soil enzymes improves soil health and indicates that the soil is free from the degradation process (Franzluebbers, 2002). In the sloping uplands of Manipur, India’s Eastern Himalayan area, Ansari et al. (2022a) confirmed that degraded soils from unsustainable land-use activities (e.g. hill slope agriculture) produced a reduction in SR, whereas stable land-use techniques (e.g., agroforestry or woody horticulture) improved SRs. The aforesaid findings were validated in the MGP cropping system, which included green manuring and crop residue retention and gave higher nutrient sequestration and harbored more enzymatic activities than other treatments. Almost a two fold increase in SR1 and SR2 corroborated the constant decrease in available nutrients (micro and macro) and enzymatic activities from the surface (L1) to the subsoil (L3). Previous studies have also affirmed that the soil nutrients and enzymatic activities decline at varying rates depending on soil depth (Lungmuana et al., 2019). In the studied region, Ansari et al. (2022a) found a substantial increase in SR along with a depth of up to 1.0 m in both cultivated (e.g., upland and lowland agriculture, horticulture, and agroforestry) and uncultivated (dense forest) land uses. Thus, our findings notably reported that the use of green manuring is important in maize/groundnut cropping systems in the subtropical regions for stabilizing soil quality indicators (soil nutrients and enzymatic activities), which is critical for maintaining long-term crop productivity.
Soil quality index
Biomass accumulation through green manuring and crop residue retention in cropping systems is one of the most significant and easily implemented strategies for enhancing, regulating, and supporting ecosystem services and combating soil degradation (Lal, 2017). Soil micro-climate-mediated biomass accumulation creates a favorable soil environment, which improves the nutrient utilization efficiency and leads to higher crop productivity (Lal, 2017; Ansari et al., 2022b). However, an intensive cropping system with inadequate agro-technique and residue removal could lead to soil quality deterioration as well as poor responsive soils (Singh et al., 2021). In 5 years of study, we notably observed that the soil quality indicators like SOC stock, available macro- (N, P, and K) and micro (Fe, Mn, Zn, and Cu)-nutrients as well as enzymatic activities (ACP, ALK, DHY, GLU, and ARY) improved significantly (p < 0.05) in the MGP cropping systems with SGM and residue retention as compared to other cropping systems with residue removal. Across the cropping systems, SYI significantly correlated with available macronutrients (r = +0.65–0.781), available micronutrients (r= +0.782–0.824), and soil enzymatic activities (r = +0.0.570–0.791) (Supplementary Table S3). In this study, soil carbon, nutrients, and potential soil enzyme status improved significantly in MGP cropping system. SGM and crop residue retention resulted in the improvement of SQI. Green manuring and residue retention resulted in the substantial reduction in dependency on fertilizers through improvement in nutrient availability and modified biomass-mediated enzyme activities (Shahid et al., 2013; Nath et al., 2019). Further, higher SQI in surface and subsurface soil suggests for the adoption of MGP with SGM and residue retention in this region as well as similar ecosystems elsewhere.
Yield sustainability
The SYI is used to measure long-term sustainability in terms of yield. Its value varies from zero to unity. Higher values indicate that treatments give constantly higher yields across the years. Higher SYI and system productivity provide the opportunity to achieve the SDGs and especially good health and well-being. Averaged over the years, Sesbania green manuring treatment gave a 24.2% higher system productivity in terms of maize equivalent yield (MEY) over no-green manuring treatment (Figure 7). The continuous application of SGM treatment reflected significant (p < 0.05) improvement in the SYI (+18.8%) as compared to that of NGM. Similarly, the maize + groundnut–pea cropping system recorded +16.4 and +39.6% higher system productivities over the groundnut–pea and maize–pea cropping system, respectively. Consequently, the MGP cropping system recorded higher SYI (6.8%–17.6%) as compared to the other two systems. Continuous crop residue retention increased the system productivity by 11.3% averaged over 5 years and consequently recorded higher SYI (+11%) over residue removal. No-green manuring and removal of crop residues from maize + groundnut–pea cropping system resulted in a decline in system productivity and SYI over 5 years of experimentation.
Conclusion
Stabilized soil carbon and nutrient (macro and micro) management efficiency and ecosystem services, as well as maintaining higher yield sustainability and soil quality through sustainable agriculture management practices, are the key aims for sustainable agricultural production. These improvements may contribute a little toward achieving of SDGs viz., reduction of poverty, ensure zero hunger, good health and well-being, climate action, and life on land. Continuous five-year SGM incorporation, MGP cropping system, and residue retention increased the SYI by 19%, 17.0%, and 11.0%, respectively, over NGM, MP, and residue removal. Similarly, the same treatment increased the SQI by 35.7%, 9.5%, and 15.8% in the surface and by 24.4%, 4.4%, and 8.0% in subsurface soils, respectively. Higher available soil macro- and micronutrient content and soil enzymatic activities have resulted from the increase in their SR, SQI, and SYI under groundnut intercropped with maize followed by the pea cropping system. Soil SR and SQI along with SYI have emerged as major sustainability indicators. Augmenting these indicators in the long-term production system is of paramount significance. Hence, a multi-indicator-based approach including crop management under resource conservation along with green manuring, residue retention, and cropping intensification with the improvement of enzymatic activities, availability of macro- and micronutrients, and land productive capacity.
Data availability statement
The datasets presented in this study can be found in online repositories. The names of the repository/repositories and accession number(s) can be found in the article/Supplementary Material.
Author contributions
Conceptualization, investigation, monitoring, data curation, and writing of original and final draft: MA; review and writing, editing: SB and JC; review and writing, funding acquisition and review: NR and ASP.
Acknowledgments
The authors acknowledge the physical and, scientific equipment facilities and financial support received from the Director, ICAR Research Complex for NEH Region, Meghalaya, India under the ICAR Institute project (Project code: IXX09777). The authors are gratefully acknowledged to the Director, ICAR-IIFSR, Modipuram and All India Network Programme on Organic Farming (AI-NPOF) for financial assistance in publication of research outcome. The authors express their gratitude to the project staff and technical officer for their assistance during the project.
Conflict of interest
The authors declare that the research was conducted in the absence of any commercial or financial relationships that could be construed as a potential conflict of interest.
Publisher’s note
All claims expressed in this article are solely those of the authors and do not necessarily represent those of their affiliated organizations, or those of the publisher, the editors, and the reviewers. Any product that may be evaluated in this article, or claim that may be made by its manufacturer, is not guaranteed or endorsed by the publisher.
Supplementary material
The Supplementary Material for this article can be found online at: https://www.frontiersin.org/articles/10.3389/fenvs.2022.940349/full#supplementary-material
References
Almagro, M., Ruiz-Navarro, R. N., Díaz-Pereira, D. P., Albaladejo, A., and Martínez-Mena, M. M. (2021). Plant residue chemical quality modulates the soil microbial response related to decomposition and soil organic carbon and nitrogen stabilization in a rainfed Mediterranean agroecosystem. Soil Biol. Biochem. 156, 108198. doi:10.1016/j.soilbio.2021.108198
Amede, T., Legesse, G., Agegnehu, G., Gashaw, T., Degefu, T., Desta, G., et al. (2021). Short term fallow and partitioning effects of green manures on wheat systems in East African highlands. Field Crops Res. 269, 108175. doi:10.1016/j.fcr.2021.108175
Amede, T., and Taboge, E. (2007). “Optimizing soil fertility gradients in the enset systems of the Ethiopian highlands: Trade-offs and local innovations,” in Advances in integrated soil fertility management in Sub-Saharan Africa: Challenges and Opportunities (Dordrecht: Springer), 289–297. Avaliable At: http://hdl.handle.net/10625/38152.
Ansari, M. A., Choudhary, B. U., Prakash, N., Kumar, B., Jat, S. L., Mishra, D., et al. (2016). Genotypic variations in phenology, productivity and heat-use efficiency of rainfed maize (Zea mays) in acid soils of north eastern Himalayan region. Indian J. Agric. Sci. 86 (6), 796–802. Avaliable At: https://epubs.icar.org.in/ejournal.
Ansari, M. A., Choudhury, B. U., Layek, J., Das, A., Lal, R., and Mishra, V. K. (2022b). Green manuring and crop residue management: Effect on soil organic carbon stock, aggregation, and system productivity in the foothills of eastern himalaya (India). Soil Tillage Res. 218, 105318. doi:10.1016/j.still.2022.105318
Ansari, M. A., Choudhury, B. U., MandalJat, S. S. L., and Jat, C. B. (2022a). Converting primary forests to cultivated lands: Long-term effects on the vertical distribution of soil carbon and biological activity in the foothills of Eastern Himalaya. J. Environ. Manage. 301, 113886. doi:10.1016/j.jenvman.2021.113886
Ansari, M. A., Choudhury, B. U., Roy, S. S., Sharma, S. K., Singh, I. M., and SinghPrakash, A. L. N. (2020). Performance of groundnut (Arachis hypogaea) cultivars for higher productivity in hilly ecosystem. Indian J. Agric. Sci. 90 (4), 818–822. Avaliable At: https://epubs.icar.org.in/ejournal.
Ansari, M. A., Saha, S., Das, A., Lal, R., Das, B., Choudhury, B. U., et al. (2021). Energy and carbon budgeting of traditional land use change with groundnut-based cropping system for environmental quality, resilient soil health and farmers income in eastern Indian Himalayas. J. Environ. Manage. 293, 112892. doi:10.1016/j.jenvman.2021.112892
Babu, S., Mohapatra, K. P., Das, A., Yadav, G. S., Tahasildar, M., Singh, R., et al. (2020). Designing energy-efficient, economically sustainable and environmentally safe cropping system for the rainfed maize–fallow land of the Eastern Himalayas. Sci. Total Environ. 722, 137874. doi:10.1016/j.scitotenv.2020.137874
Banerjee, V., Krishnan, P., Das, B., Verma, A. P. S., and Varghese, E. (2015). Crop status index as an indicator of wheat crop growth condition under abiotic stress situations. Field Crops Res. 18, 16–31. doi:10.1016/j.fcr.2015.06.009
Baveye, P. C., Baveye, J., and Gowdy, J. (2016). Soil ecosystem services and natural capital: Critical appraisal of research on uncertain ground. Front. Environ. Sci. 4, 1–49. doi:10.3389/fenvs.2016.00041
Blake, G. R., and Hartge, K. H. (1986). “Bulk density,” in Methods of soil analysis, Part I: Physical and mineralogical methods. Editor A. Klute. second edn. (Madison WI: American Society of Agronomy).
Buragohain, S., Sharma, B., Nath, J. D., Gogaoi, N., Meena, R. S., and Lal, R. (2017). Impact of ten years of bio-fertilizer use on soil quality and rice yield on an inceptisol in Assam, India. Soil Res. 56 (1), 49–58. doi:10.1071/SR17001
Casida, L. E., Klein, D. A., and Santoro, T. (1964). Soil dehydrogenase activity. Soil Sci. 98, 371–376. doi:10.1097/00010694-196412000-00004
Chen, A., Wang, Z., Lin, Y., Wang, X., Li, Y., Zhang, Y., et al. (2020). Temporal variation of soil organic carbon pools along a chronosequence of reforested land in Southwest China. Catena 194, 104650. doi:10.1016/j.catena.2020.104650
Choudhury, B. U., Ansari, M. A., Chakraborty, M., and Meetei, T. T. (2021). Effect of land-use change along altitudinal gradients on soil micronutrients in the mountain ecosystem of Indian (Eastern) Himalaya. Sci. Rep. 11, 14279. doi:10.1038/s41598-021-93788-3
Choudhury, B. U., Nengzouzam, G., Ansari, M. A., and Islam, A. (2022). Causes and consequences of soil erosion in northeastern Himalaya, India. Cur. Sci. 122 (7), 772–789. doi:10.18520/cs/v122/i7/772-789
Das, A., Devi, M. T., Babu, S., Ansari, M. A., Layek, J., Bhowmick, S. N., et al. (2018). “Cereal-legume cropping system in Indian himalayan region for food and environmental sustainability,” in Legumes for soil health and sustainable management. Editors (Springer Nature Singapore Pte Ltd). doi:10.1007/978-981-13-0253-4_2
Das, A., Layek, J., Yadav, G. S., Lal, R., Saha, S., Singh, R., et al. (2021). “Managing soil organic carbon in croplands of the eastern Himalayas, India,” in Soil organic matter and feeding the future. Editor R. Lal. doi:10.1201/9781003102762-12
Eivazi, F., and Tabatabai, M. A. (1988). Glucosidases and galactosidases in soils. Soil Biol. biochem. 20, 601–606. doi:10.1016/0038-0717(88)90141-1
Franzluebbers, A. J. (2002). Soil organic matter stratification ratio as an indicator of soil quality. Soil Tillage Res. 66 (2), 95–106. doi:10.1016/S0167-1987(02)0001810.1016/s0167-1987(02)00018-1
Hirte, J., Leifeld, J., Abiven, S., and Mayer, J. (2018). Maize and wheat root biomass, vertical distribution, and size class as affected by fertilization intensity in two long-term field trials. Field Crops Res. 216, 197–208. doi:10.1016/j.fcr.2017.11.023
Huang, K., Yangjian, Z., Juntao, Z., Yaojie, L., Jiaxing, Z., and Jing, Z. (2016). The influences of climate change and human activities on vegetation dynamics in the Qinghai-Tibet Plateau. Remote Sens. 8 (10), 876. doi:10.3390/rs8100876
Kaiser, H. F. (1960). The application of electronic computers to factor analysis. Educ. Psychol. Meas. 20, 141–151. doi:10.1177/001316446002000116
Karlen, D. L., Andrews, S. S., and Doran, J. W. (2001). Soil quality: Current concepts and applications. Adv. Agron. 74, 1–40. doi:10.1016/s0065-2113(01)74029-1
Lal, R. (2017). Improving soil health and human protein nutrition by pulses-based cropping systems. Adv. Agron. 145, 167–204. doi:10.1016/bs.agron.2017.05.003
Lindsay, W. L., and Norvell, W. A. (1978). Development of a DTPA soil test for zinc, iron, manganese and copper. Soil Sci. Soc. Am. J. 42, 421–428. doi:10.2136/sssaj1978.03615995004200030009x
Lungmuana, S., Singh, S. B., Choudhury, B. U., VanthawmlianaSaha, S., and Hnamte, V. (2019). Transforming jhum to plantations: Effect on soil microbiological and biochemical properties in the foot hills of North Eastern Himalayas, India. Catena 177, 84–91. doi:10.1016/j.catena.2019.02.008
Ma, D., Lina, Y., Wenliang, J., Xiankun, L., Xiaoxiao, L., Xiping, D., et al. (2021). Meta-analysis of green manure effects on soil properties and crop yield in northern China. Field Crops Res. 266, 108146. doi:10.1016/j.fcr.2021.108146
Meena, B. L., Fagodiya, R. K., Prajapat, K., Dotaniya, M. L., Kaledhonkar, M. J., Sharma, P. C., et al. (2018). “Legume green manuring: An option for soil sustainability,” in Legumes for soil health and sustainable management (Singapore: Springer), 387–408. doi:10.1007/978-981-13-0253-4_12
Meetei, T. T., Choudhury, B. U., Mohapatra, K. P., Singh, N. U., Das, A., and Devi, Y. B. (2020). Effect of 25 years old agroforestry practices on soil quality attributes in the northeastern Himalayan region of India. Int. J. Chem. Sci. 8 (1), 2371–2379. doi:10.22271/chemi.2020.v8.i1aj.8623
Melero, S., Juan, C. R. P., Jua, F. H., and Engracia, M. (2006). Chemical and biochemical properties in a silty loam soil under conventional and organic management. Soil Tillage Res. 90 (1-2), 162–170. doi:10.1016/j.still.2005.08.016
Nath, C. P., Hazra, K. K., Kumar, N., Praharaj, C. S., Singh, S. S., Singh, U., et al. (2019). Including grain legume in rice-wheat cropping system improves soil organic carbon pools over time. Ecol. Eng. 129, 144–153. doi:10.1016/j.ecoleng.2019.02.004
Nelson, D. W., and Sommers, L. E. (1973). Determination of total nitrogen in plant material. Agron. J. 65, 109–112. doi:10.2134/agronj1973.00021962006500010033x
Peregrina, F., Pérez-Álvarez, E. P., and García-Escudero, E. (2014). Soil microbiological properties and its stratification ratios for soil quality assessment under different cover crop management systems in a semiarid vineyard. J. Plant. Nutr. Soil Sci. 177 (4), 548–559. doi:10.1002/jpln.201300371
Qua, Q., Xub, H., Xuea, S., and Liua, G. (2020). Stratification ratio of rhizosphere soil microbial index as an indicator of soil microbial activity over conversion of cropland to forest. Catena 195, 104761. doi:10.1016/j.catena.2020.104761
Ravisankar, N., Ansari, M. A., Shamim, M., Prusty, A. K., Singh, R., Panwar, A. S., et al. (2022). Sustainable livelihood security of small farmers improved through resilient farming systems in the Semi-Arid Region of India. Land Degrad. Dev., 1–13. doi:10.1002/ldr.4358
Sarkar, D., Baishya, L. K., Meitei, C. B., Naorem, G. C., Thokchom, R. C., Singh, J., et al. (2018). Can sustainability of maize-mustard cropping system be achieved through balanced nutrient management? Field Crops Res. 225, 9–21. doi:10.1016/j.fcr.2018.05.018
Shahid, M., Nayak, A. K., Shukla, A. K., Tripathi, R., Kumar, A., Mohanty, S., et al. (2013). Long-term effects of fertilizer and manure applications on soil quality and yields in a sub-humid tropical rice-rice system. Soil Use Manag. 29, 322–332. doi:10.1111/sum.12050
Singh, R., Babu, S., Avasthe, R. K., Meena, R. S., Yadav, G. S., Das, A., et al. (2021). Conservation tillage and organic nutrients management improve soil properties, productivity, and economics of the maize-vegetable pea system in the Eastern Himalayas. Land Degrad. Dev. 32 (16), 4637–4654. doi:10.1002/ldr.4066
Stagnari, F., Maggio, A., Galieni, A., and Pisante, M. (2017). Multiple benefits of legumes for agriculture sustainability: An overview. Chem. Biol. Technol. Agric. 4 (2), 1–13. doi:10.1186/s40538-016-0085-1
Subbiah, B. V., and Asija, C. L. (1956). A rapid procedure for estimation of available nitrogen in soils. Curr. Sci. 25, 259–260. Avaliable At: https://www.scienceopen.com/document?vid=6fc73f08-2bbf-4ac7-93e5-7f405a35961f.
Sürücü, A., Mehmetm, A. Ö., Betül, B., and Rıdvan, K. (2014). Effects of green manuring on soil enzyme activity. Fresenius Environ. Bull. 23 (9), 2126–2132.
Tabatabai, M. A., and Bremmer, J. M. (1969). Use of p–nitrophenyl phosphate for assay of soil phosphatase activity. Soil Biol. biochem. 1, 301–307. doi:10.1016/0038-717(69)90012-110.1016/0038-0717(69)90012-1
Tabatabai, M. A., and Bremner, J. M. (1970). Arylsulfatase activity in soils. Soil Sci. Soc. Am. Proc. 34, 225–229. doi:10.2136/sssaj1970.03615995003400020016x
Vance, E. D., Brookes, P. C., and Jenkinson, D. S. (1987). An extraction method for measuring soil microbial biomass C. Soil Biol. biochem. 19, 703–707. doi:10.1016/0038-0717(87)90052-6
Walkley, A., and Black, I. A. (1934). Estimation of soil organic carbon by the chromic acid titration method. Soil Sci. 37, 29–38. doi:10.1097/00010694-193401000-00003
Wander, M. M., Cihacek, L.,J., Coyne, M., Drijber, R. A., Grossman, J. M., Gutknecht, J. L. M., et al. (2019). Developments in agricultural soil quality and health: Reflections by the research committee on soil organic matter management. Front. Environ. Sci. 7, 109. doi:10.3389/fenvs.2019.00109
Yadav, G. S., Das, A., Kandpal, B. K., Babu, S., Lal, R., Datta, M., et al. (2021). The food-energy-water-carbon nexus in a maize-maize-mustard cropping sequence of the Indian Himalayas: An impact of tillage-cum-live mulching. Renew. Sustain. Energy Rev. 151, 111602. doi:10.1016/j.rser.2021.111602
Yuan, B. C., and Yue, D. X. (2012). Soil microbial and enzymatic activities across a chronosequence of Chinese pine plantation development on the loess plateau of China. Pedosphere 22 (1), 1–12. doi:10.1016/S1002-0160(11)60186-0
Zhang, C., Chen, L., and Jiang, J. (2014). Vertical root distribution and root cohesion of typical tree species on the Loess Plateau, China. J. Arid. Land. 6 (5), 601–611. doi:10.1007/s40333-014-0004-x
Keywords: crop residue management, nutrient storage, soil enzymatic activities, stratification ratio, soil quality index, sustainability index
Citation: Ansari MA, Babu S, Choudhary J, Ravisankar N and Panwar AS (2022) Soil quality restoration and yield stabilization in acidic soils of northeastern Himalayas: Five years impact of green manuring and crop residue management. Front. Environ. Sci. 10:940349. doi: 10.3389/fenvs.2022.940349
Received: 10 May 2022; Accepted: 25 July 2022;
Published: 06 September 2022.
Edited by:
Thiru Selvan, Tripura University, IndiaReviewed by:
Mengyang You, Chinese Academy of Sciences (CAS), ChinaSanjeev Kumar Chaudhary, Regional Horticultural Research Sub Station Bhaderwah, India
Copyright © 2022 Ansari, Babu, Choudhary, Ravisankar and Panwar. This is an open-access article distributed under the terms of the Creative Commons Attribution License (CC BY). The use, distribution or reproduction in other forums is permitted, provided the original author(s) and the copyright owner(s) are credited and that the original publication in this journal is cited, in accordance with accepted academic practice. No use, distribution or reproduction is permitted which does not comply with these terms.
*Correspondence: Meraj A. Ansari, bWVyYWppYXJpQGdtYWlsLmNvbQ==; Subhash Babu, c3ViaGlhcmlAZ21haWwuY29t