- 1School of Earth and Environment, Anhui University of Science and Technology, Huainan, China
- 2Engineering Laboratory of Anhui Province for Comprehensive Utilization of Water and Soil Resources and Construction of Ecological Protection in Mining Area with High Groundwater Level, Anhui University of Science and Technology, Huainan, China
- 3State Key Laboratory of Crop Stress Adaptation and Improvement, School of Life Sciences, Henan University, Kaifeng, China
- 4Key Laboratory of Tobacco Biology and Processing, Ministry of Agriculture and Rural Affairs, Tobacco Research Institute, Chinese Academy of Agricultural Sciences, Qingdao, China
Revegetation is an important restoration approach after reclamation in coal mining subsidence area. However, few studies have paid attention to the impacts of different vegetation species on soil fertility and bacterial community composition in the reclamation area filled with fly ash for a long time. In this study, soil fertility and bacterial community composition were investigated in a non-subsidence area (FCK) and a coal mining subsidence reclamation area restored vegetation with woody plants (pagoda trees, FS; peach trees, FP) and herbaceous plants (wheat-maize rotation, FW) for 20 years. Results showed that topsoil and subsoil nutrients including available K and alkali-hydrolyzable N, soil organic matter, and total carbon in the non-subsidence site were significantly higher than those in reclamation sites. Topsoil fertility indices (SFI) in reclamation sites were lower than that in non-subsidence site, and soil fertility index in FW was higher than that in FS and FP. Moreover, the SFI in topsoil (from −0.24 to 2.08) was significantly higher than that in subsoil (from −1.12 to −0.39). 16S rRNA gene sequencing analysis showed Proteobacteria, Acidobacteria and Actinobacteria were the dominant bacterial phyla in all sites, but the subsoil bacterial alpha indices (Shannon and Simpson) in the non-subsidence site were higher than those in reclamation sites. Principal coordinates analysis and non-metric multidimensional scaling analysis showed that the bacterial community composition in reclamation sites was significantly different from that in the non-subsidence site, and they were similar in the soil with wheat-maize rotation model between non-subsidence and reclamation sites. Co-occurrence network analysis noted that the network of dominant bacterial operational taxonomic units in the subsoil was more complex than that in the topsoil. Redundancy analysis suggested soil pH in topsoil and subsoil was an important driving factor for soil bacteria community composition. Overall, the reclamation site with the wheat-maize rotation model had higher soil fertility and bacterial community composition was similar to that of the non-subsidence site, indicating revegetation with the wheat-maize rotation model is a good reclamation approach to improve soil fertility in coal mining subsidence area.
Introduction
With the development of industry, demands for coal resources are increasing in most countries over the world, especially in developing countries (Feng et al., 2019). For instance, coal consumption in China accounted for 56.8% of the total energy consumption in 2020 (Hu et al., 2022), and coal consumption will continue to grow by 3.4% in the next 20 years (Yu et al., 2022). The extensive exploitation of coal resources has caused serious environmental and social problems including land subsidence, land damage, and poor harvest (Wang et al., 2020; Ma et al., 2021). In high groundwater level areas, coal mining always resulted in land subsidence and changed terrestrial to aquatic ecosystems (Ma et al., 2021). Such dramatic environmental changes have destroyed agricultural lands and threatened the safe production of crops and the survival of local residents. Therefore, it is of great significance to repair the coal mining subsidence area.
Previous literature reviews have noted that fly ash, coal gangue, and other solid wastes can be used as filling materials in the coal mining subsidence areas and recover with surface soil above the filling materials (Ram and Masto, 2014; Hemalatha and Ramaswamy, 2017; Hu, 2019). Fly ash as a by-product of the coal-fired power station was widely used to fill the subsidence area (Hu, 2019). It can also provide lots of essential nutrients (K, Si, Fe, etc.) for plants (Ram and Masto, 2014), and its filling was beneficial for soil carbon sequestration and biodiversity conservation (Pandey, 2013). However, the filling substrates were significantly different from the subsoil and cannot effectively retain soil moisture and fertility, which may result in poor fertility and low soil productivity (Ma et al., 2019). For example, Duo and Hu (2018) reported that the soil quality significantly decreased after reclamation, and the crop yield was only 80.5% of that in the non-subsidence area after 5 years of reclamation (Duo and Hu, 2018). Consequently, the long-term changes in soil quality should be paid more attention in reclamation area filled with solid wastes/amendments.
Additionally, revegetation in reclamation area can effectively improve soil fertility and restore soil ecosystem functions (Zhou et al., 2020). It is essential to establish a self-sustaining revegetation ecosystem by selecting suitable plant species in the light of the condition of the subsidence areas (Kohler et al., 2016; Qu et al., 2017; Li and Liber, 2018). Numerous plants including woody plants (Acacia catechu, Dalbergia sissoo, Pongamia pinnata and Terminalia bellirica, etc.) (Srivastava et al., 2014) and herbaceous plants (grass, wheat, maize, Ricinus communis, Vigna radiata, Saccharum munja, etc.) (Pandey et al., 2012; Pandey, 2013; Yang et al., 2020) have been reported to revegetate in coal mining subsidence areas. However, little information is available on the impacts of vegetation species on soil microbial community composition in reclamation areas filled with fly ash. Soil microbial communities are critical to organic matter decomposition, nutrient cycling, long-term ecosystem stability and revegetation (Lehmann et al., 2011; Chen et al., 2017; Sun et al., 2019), and it is considered as an important indicator for the ecological restoration effect in coal mining subsidence area (Xu et al., 2019; Yuan et al., 2021). Natural succession of reclamation areas is a slow biogeochemical process and needs a long term before reaching a stable state (Ma et al., 2021). However, most studies about ecological restoration concentrated on a relatively short time (1–15 years) (Liu et al., 2018; Ma et al., 2019; Wang et al., 2020), and few studies were available on the evaluation of soil fertility and microbiome composition in reclamation areas.
In this study, a coal mining subsidence area filled with fly ash in eastern China was selected as the study area and soil samples were collected in four sites planted with woody plants (pagoda tree and peach tree) and herbaceous plants (wheat-maize rotation model) for 20 years. Here, we aim to 1) investigate soil physicochemical properties and fertility in reclamation areas; 2) evaluate the impacts of reclamation on soil bacterial community composition and biodiversity.
Materials and Methods
Site Description
This study was conducted in a coal mining subsidence area (∼100 ha, 116°85′-116°88′ E, 33°97′-33°98′ N) filled with fly ash in the eastern Huaibei city, Anhui Province, China (Supplementary Figure S1). The climate is characterized with a warm temperate semi-humid monsoon climate. The average annual precipitation and average annual temperature are 850 mm and 15.9°C in this area, respectively. Since high groundwater level existed above the coal bed, resulting in surface subsidence and land damage after long-term coal mining. In 2000, the surface soil (40 cm) was stripped and filled with fly ash in the subsidence area, and then covered the surface soil above the fly ash. During the next 20 years, most reclamation area was adopted a wheat-maize rotation model with two crops per year (FW), and part of the area was planted with pagoda trees (Sophora japonica Linn) (FS) and peach trees (Prunus persica L.) (FP), respectively. In addition, a non-subsidence site with a wheat-maize rotation model was also selected as the background area (FCK), which was 2.50 km to the reclamation area.
Sample Collection and Physicochemical Analysis
In November 2020, topsoil (0–20 cm) and subsoil (20–40 cm) samples were collected in each area. All soil samples were passed through a 2 mm sieve. Half of the soil samples were dried at room temperature for chemical analysis, and the other samples were stored at −40°C for bioanalysis.
Soil pH was analyzed in a soil-to-water ratio of 1:2.50 (w/v) by pH electrode (PHS-3C, Shanghai, China). Soil organic matter (SOM), available phosphorus (AP), total phosphorus (TP), alkali-hydrolyzable nitrogen (AN), available potassium (AK) and total potassium (TK) were measured according to Lu (2000). Detailed information about the analysis methods is provided in Supplementary Text S1. An elemental analyzer (Vario Macro Cube, Elementar Company, Germany) was used to determine soil total nitrogen (TN) and total carbon (TC) concentrations (Wang et al., 2021).
Soil Biological Analysis
Soil whole DNA was extracted and purified according to the method of Cui H. et al. (2018). The concentration and purity of DNA were checked using NanoDrop One (Thermo Fisher Scientific, MA, United States). The standard bacterial primer set (515F and 806R) combined with barcode sequences (Caporaso et al., 2011) were used to amplify 16S rRNA genes (Cui H. et al., 2018). The Illumina Hiseq2500 platform was used for PE250 sequencing of the constructed amplicon library. Detailed information about the analysis methods is provided in Supplementary Text S2.
To clarify the associations among the soil key operational taxonomic units (OTUs), we selected the top 30 OTUs with the highest relative abundance in all sites (Hill et al., 2016). Spearman’s correlation coefficient was chosen, and the threshold value of the correlation coefficient was 0.4. The network was analyzed by the tool of the Wekemo Bioincloud (https://www.bioincloud.tech). To evaluate the contributions of microbial populations to the overall community structure, the bipartite networks created in Cytoscape (3.8.2) were used to describe the associations between the OTUs and different sites (Supplementary Text S3) (Smoot et al., 2011; Jiao et al., 2017).
Statistical Analysis
The igraph and vegan in R (4.1.0) were used for statistical analysis The factor analysis was used to calculate the soil fertility index (SFI) (Supplementary Text S4) (Li et al., 2014; Guo et al., 2018). The relationships between soil physicochemical properties and microbial alpha diversity indexes were detected by Pearson correlation analysis. The phylum to genus-level abundance information was extracted by R to visualize using Origin 2021. Richness of OTUs, Chao1, ACE, Shannon, Simpson, and goods coverage indices were applied to characterize the bacterial alpha diversity. Principal coordinates analysis (PCoA) and non-metric multidimensional scaling (NMDS) were performed in the R software. The relationship between environmental parameters and bacteria was analyzed by redundancy analysis (RDA) which was performed by Lingbo MicroClass cloud application (http://www.cloud.biomicroclass.com/cloudplatform/softpage/CCA). The linear discriminant analysis (LDA) effect size (LEfSe) method (http://www.cloud.biomicroclass.com/CloudPlatform/SoftPage/LF2) was performed to identify multiple taxonomical levels of potential biomarkers (LDA score threshold >3.0, factor Kruskal–Wallis and Wilcoxon test with an alpha value of 0.05), the analysis strategy of multi-group classification analysis was used one against one (Chen et al., 2020).
Results and Discussion
Soil Physicochemical Properties and Fertility
Soil physicochemical properties affected by different vegetation species were shown in Table 1. Topsoil was alkalinity in this area and its pH ranged from 8.14 to 8.69. Soil pH in FS and FP (wood land) were 0.11–0.53 units higher than those of FCK and FW (wheat field), suggesting that vegetation species influence soil pH, which was consistent with the findings of Deng et al. (2019) and Luo et al. (2022). Generally, the concentrations of WC, AK, TN, AN, SOM, and TC in FCK were the highest, and followed by FW (Table 1). For example, in the topsoil, WC in FCK was 0.98 1.26 and 0.62 times higher than that in FS, FP, and FW, respectively, and 0.64, 0.98 and 0.41 times higher for SOM. These results suggested filling reclamation caused soil water loss and fertility degradation, which also have been reported in previous study (Qu et al., 2018). However, concentrations of plant nutrients (including AP, SOM, and TC) of topsoil in FW were significantly higher than that in FS and FP (p < 0.05, Table 1), which suggested the wheat-maize rotation model was a good approach for the enhancement of soil fertility.
Similar to topsoil, most subsoil plant nutrients concentrations (including AK, TK, TN, AN, SOM, and TC) in FCK were higher than those in the other sites (Table 1). Moreover, subsoil pH (8.35–8.69) was higher than that of topsoil (8.05–8.57), and a similar trend was found for soil moisture content. In contrast to soil pH, contents of AK, TN, AN, and SOM in topsoil were higher than those in the subsoil. For example, topsoil SOM in FCK, FS, FP, and FW were 0.69, 0.32, 0.38, and 0.79 times higher than those of subsoil, respectively. In addition, the principal component analysis showed the eigenvalues of PC1 and PC2 were 3.61 and 1.47, respectively (Supplementary Table S1). The variance contribution rates were 51.6 and 21.0%, which can explain 72.6% of the total variance contribution. The score matrix of the PCA analysis showed that the loadings of TN, AK, and AN in PC1 exceeded 0.87 (Supplementary Table S2), indicating that these factors in PC1 play a significant role in soil fertility changes. SFI in the topsoil of FCK (2.08) was significantly greater than those of FS (−0.24) and FP (−0.56) (p < 0.05) (Supplementary Table S3), but there was no noticeable difference between FCK and FW (1.58) (p > 0.05, Supplementary Table S3). These results suggested that the soil fertility level in reclamation areas with woody plants was lower than that in the non-subsidence area with agricultural crops, and they also noted that the wheat-maize rotation model is an effective method to improve the soil fertility in the reclamation area.
Correlation analysis showed AK, AN, SOM, and TC were positively correlated with each other (p < 0.05, Supplementary Figure S2), especially for the positive correlation between TC and TN (p < 0.01). It may be due to the addition of nitrogen fertilizers that can promote plant growth and carbon accumulation (Qi et al., 2020). Moreover, we found the C/N ratios (Supplementary Figure S3) in the topsoil of wood land (33.33–33.78, FS and FT) were higher than those in the agricultural field (23.47–23.98, FCK and FW), which was also reported by Gao et al. (2014). This may be due to the intensive cultivation and agricultural management practices in agricultural fields resulting in the mineralization and loss of SOM to the atmosphere (Lal, 2002; Gao et al., 2014).
Soil Bacterial Community Composition
In all sites, 360,000 high-quality sequences and 7,141 different OTUs were obtained. The abundance of the dominant bacterial phyla/classes between reclamation and non-subsidence areas was shown in Figure 1 and Supplementary Figure S4. Proteobacteria, Acidobacteria, and Actinobacteria were the most dominant bacterial phyla in the topsoil and subsoil, but their percentages were significantly different between topsoil and subsoil, suggesting the bacterial community composition was significantly different. For example, Proteobacteria in the topsoil of FCK accounted for 27.5%, which was 0.09 times lower than that in the subsoil of FW, and Acidobacteria in the subsoil of FS was higher compared to that in the topsoil of FS. Moreover, Proteobacteria in the topsoil of FW accounted for 40.1%, which was 0.46, 0.68 and 0.73 times higher than that in FCK, FS and FP, respectively. Similar to Wang et al. (2017), Gammaproteobacteria and Alphaproteobacteria were the dominant bacterial classes in the topsoil of the reclamation area, and accounted for 13.3 and 9.24% in all sites (Figure 1; Supplementary Table S4). Previous studies noted Gammaproteobacteria and Alphaproteobacteria participated in the process of nitrogen fixation (Madigan et al., 1984; Wolińska et al., 2020), thus their existence with large amounts in the reclamation area favored the enhancement of soil quality. In addition, the highest relative abundance of bacterial phylum and classes of FW was higher than that of FCK, FP and FS, indicating that the wheat-maize rotation model is a good selection to reconstruct the bacterial community in the reclamation area.
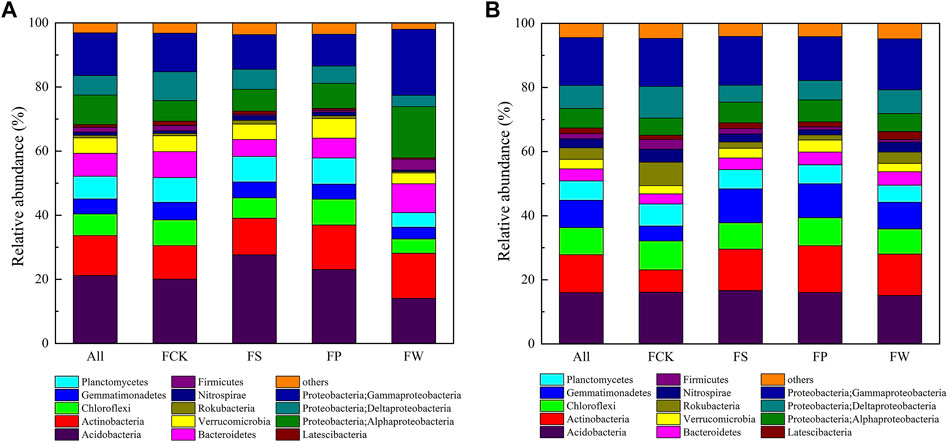
FIGURE 1. Relative abundances of dominant bacterial phyla/classes in topsoil (A) and subsoil (B) among different sites. Relative abundances are based on the proportional frequencies of 16S rDNA sequences that could be classified at the phyla/classes level. Note: All, the whole soil samples; FCK, non-subsidence site (wheat-maize rotation); FS, reclamation site with pagoda trees; FP, reclamation site with peach trees; FW, reclamation site with wheat-maize rotation.
The bacterial richness in the reclamation sites (FS, FP and FW) for the topsoil were not significantly different from that in the non-subsidence site (FCK) (Table 2). There were no significant variations in the Chao1 (5,038–5,255) and ACE (4,934–5,195) indices of topsoil and subsoil among the four sites yet. These results indicated topsoil bacterial richness indices in the reclamation sites had reached a steady state within 20 years compared to the non-subsidence site. Previous studies have also reported soil bacterial communities reached a steady state within 15 and 20 years in reclamation areas (Li et al., 2018; Zhao et al., 2019). Moreover, Shannon and Simpson indices in the topsoil of non-subsidence site (FCK) were significantly higher than those in reclamation sites (Table 2), suggesting that different bacterial diversity existed between non-subsidence and reclamation sites. For example, Shannon indices in the topsoil of FK (non-subsidence site) were higher by 0.41, 0.14 and 0.29 than those in FS, FP and FW (reclamation sites) (p < 0.05). However, Shannon and Simpson indices for the subsoil had no significant differences between non-subsidence (FK) and reclamation sites (FS, FP and FW).
As shown in Figure 2, PCoA and NMDS analysis noted that microbial composition in woody plant revegetated sites (FS, FP) clustered together in the topsoil and subsoil, and a similar result was found by the cluster analysis at the phylum level (Supplementary Figure S4). Because woody plants had a robust root system and considerable amounts of exudates and mucilage which can strongly absorb soil nutrients (Yang et al., 2020), resulting in an substantial impact on the soil microbial community. These findings suggested that vegetation species significantly influenced soil bacterial community composition (Kohler et al., 2016). Moreover, the PCoA and NMDS (Figure 2) showed the soil bacterial community composition in the non-subsidence site was significantly differentiated from reclamation sites, indicating that reclamation changes the soil environment and influences the soil microbial community structure. It may be attributed to soil fertility in FCK being higher than that in the reclamation sites. In agreement with our study, Xu et al. (2020) also reported that soil organic carbon, inorganic nitrogen and other nutrients were the key factors affecting soil microbial diversity. Moreover, the PCoA and NMDS analysis indicated bacterial community composition in FW was the closest to the site of FCK (Figure 2) compared to other reclamation sites, thus the bacterial community composition in the two sites was similar and which may be due to the same wheat-maize rotation cultivation model. In addition, PCoA and NMDS analysis markedly separated topsoil and subsoil into two clusters (Figure 2), which indicated that the bacterial community composition in topsoil was different from that in the subsoil at each site, and it was in agreement with the result in Figure 1.
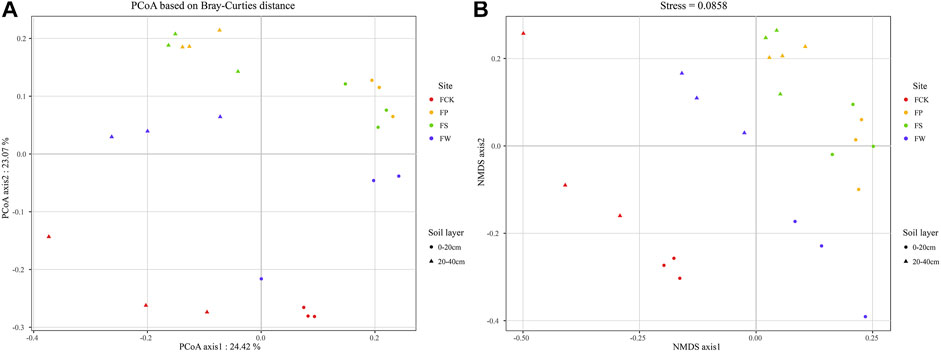
FIGURE 2. Principal coordinates analysis (PCoA) ordination (A) and non-metric multidimensional scaling (NMDS) ordination (B) based on Bray-Curtis distance at OTU level showing the effects of different vegetation species on bacterial communities among different sites. The circles represent topsoil. The triangles represent subsoil. The colors of the nodes represent different sites. Note: Site abbreviations are defined in Figure 1.
Identification of Biomarkers and Co-Occurrence Networks
LEfSe was used to identify the different biomarkers. As shown in Supplementary Figure S5, more bacterial taxa (93 clades, 15 phyla, 25 classes, 39 orders, and 27 families) in the topsoil were identified than that in the subsoil (78 clades, 12 phyla, 24 classes, 28 orders, and 25 families), which indicated that soil bacteria in the topsoil were more sensitive to the environmental disturbance than those in the subsoil. These biomarkers can be regarded as key microbial taxa shaping the metabolic threshold of the microbial community (Cui Y. et al., 2018). Chen et al. (2020) also noted biomarkers vary depending on the different environments and the relative abundance of core microorganisms. Moreover, the results of LDA revealed 37, 27, 13 and 16 bacterial phylotypes in the topsoil for FCK, FS, FP, and FW (Supplementary Figure S6A), and 27, 10, 30 and 11 bacterial phylotypes in the subsoil for FCK, FS, FP, and FW (Supplementary Figure S6B), respectively. The taxa and quantities of biomarkers were different between the non-subsidence and reclamation sites, which may be caused by the various soil quality and vegetation species.
To describe the interactions among microbial communities, we performed a network analysis of the top 30 OTUs in abundance (Figure 3) and matched them in the NCBI database (Supplementary Table S5, S6). In the topsoil, OTU31 had 9 significant positive correlations and 1 significant negative correlation with other OTUs (p < 0.05), and OTU126 and OTU23 had 13 and 11 significant positive correlations, and 2 and 4 significant negative correlations with other OTUs (p < 0.05) in the subsoil (Figure 3). The above three OTUs all significantly correlated with the top 30 OTUs, thus they can be recognized as keystone taxa and play important roles in the soil reclamation process (Ma et al., 2020). Moreover, the network of dominant bacterial OTUs in the subsoil was more complex than that in the topsoil (Figure 3), suggesting the dominant OTU in the subsoil were more closely connected than those in the topsoil.
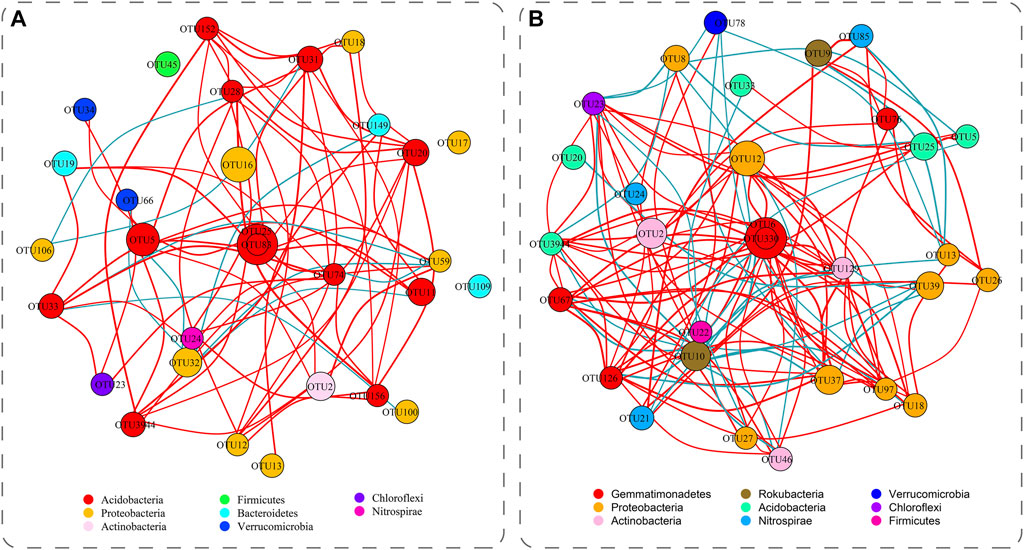
FIGURE 3. The network of dominant bacterial OTUs of topsoil (A) and subsoil (B) in all sites. The nodes in the network represent the top 30 OTUs. The size of each node is proportional to the relative abundance. The red edge represents the positive correlation between OTUs, the blue edge represents the negative correlation. The colors of the nodes represent different soil bacteria phyla. Note: Site abbreviations are defined in Figure 1.
Nowadays, rare microorganisms have attracted more attention for their important ecological role in maintaining microbial diversity and mediating biogeochemical processes (Sauret et al., 2014; Jiao et al., 2017). To analyze the connection between rare microorganisms and different sites. The contribution of microbial taxa to the community structure and the associations between genus-level OTUs and sites were evaluated by the bipartite association network (Supplementary Figure S7). Similar to Cao et al. (2021), we found the number of OTUs classified as genera in bacterial communities was at a low level (less than 15%). We screened a total of 587 nodes and 4315 edges in all sites, and they can be divided into 10 clusters and several keystone taxa according to the relationship between bacteria and sites. In co-occurrence network analysis, intermediate centrality represents the influence potential of an individual on the interaction between other nodes of the network (Greenblum et al., 2012). The core cluster was consisted by 451 nodes, and it accounted for 76.5% of the total nodes. The bipartite network analysis noted that most of OTUs were cross combinations, meaning similar taxa were presented among different sites, but their abundances were significantly different among all sites. In addition, several OTUs were connected to only a few sites, which may have specific environmental significance. For example, 27F-1492R_uncultured delta proteobacterium was a common genus of FCK and FW in the topsoil, which may be a keystone taxon in wheat-maize rotation mode.
Impacts of Soil Physicochemical Properties on Soil Microbial Community
Figure 4 showed the relationship between the top ten bacterial phyla and soil physicochemical properties. The first and second axes of RDA explained 89.9 and 6.44% of the variation in the bacterial community at the OTU level in the topsoil, while 65.8 and 16.2% were explained in the subsoil. (Figure 4). The total variation in bacterial community explained by the two axes exceeded 80% in both soil layers. Bacterial composition in all sites was significantly influenced by pH (p = 0.044) in the topsoil, and was significantly influenced by pH (p = 0.003), WC (p = 0.001), AK (p = 0.010) and TK (p = 0.003) in the subsoil (Supplementary Table S7). Moreover, Acidobacteria in the topsoil was positively correlated with pH, and Actinobacteria and Bacteroidetes were positively correlated with SOM, TN, TP, and AP (Figure 4). For the subsoil, Rokubacteria was positively correlated with pH, while Chloroflexi and Acidobacteria were positively correlated with AN, SOM, AP, TC, AK, and TN. These results suggested soil pH was a key driving factor for bacterial community structure (Ma et al., 2020; Ma et al., 2021), and further confirmed that soil nutrients can significantly influence soil bacterial communities in different reclamation areas (Wan and He, 2020).
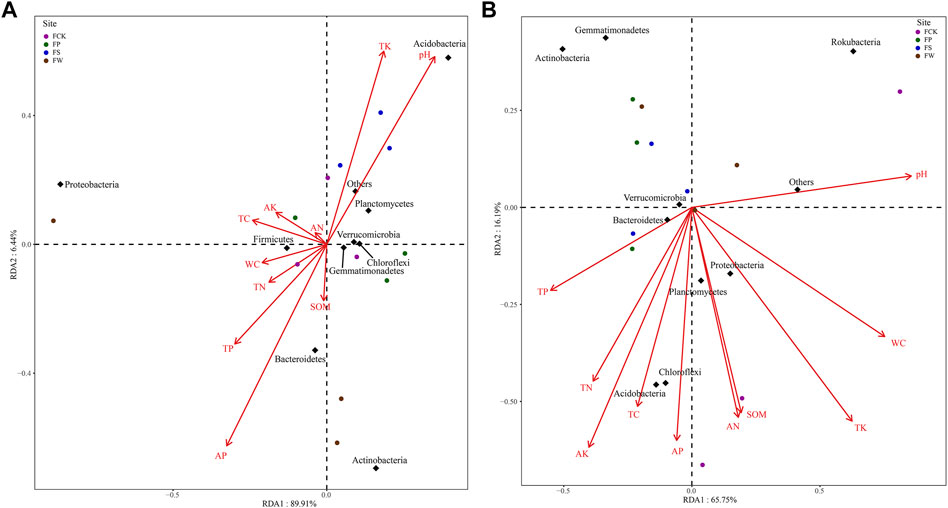
FIGURE 4. Redundancy analysis (RDA) based on the bacterial community composition and soil characteristics in topsoil (A) and subsoil (B) among different sites. The red arrows represent soil physicochemical properties. Arrow length indicates the strength of correlation with a given ordination axis. The circles represent soil samples from different sites. The diamonds represent the major soil bacteria phylum. Note: Site abbreviations are defined in Figure 1. Soil physicochemical property abbreviations are defined in Table 1.
In the topsoil, WC was positively correlated with the Shannon and Simpson indices (p < 0.05), but WC was negatively correlated with the Richness of OTUs (p < 0.05) in the subsoil (Table 3). For subsoil, soil pH was markedly negatively correlated with and Richness of OTUs (p < 0.05). Moreover, AN and SOM in the topsoil were positively correlated with alpha diversity indices, this may be because carbon and nitrogen sources are essential nutrients for microbial survival, and they are also important driving factors for the evolution of soil bacterial diversity (Liu et al., 2020). Interestingly, we found that the correlations between alpha-diversity indices and physicochemical properties (TP, AP, SOM, and pH) in the topsoil overall differed from those in the subsoil, which indicated that soil microorganisms in different soil layers were sensitive to the changes in soil physicochemical properties.
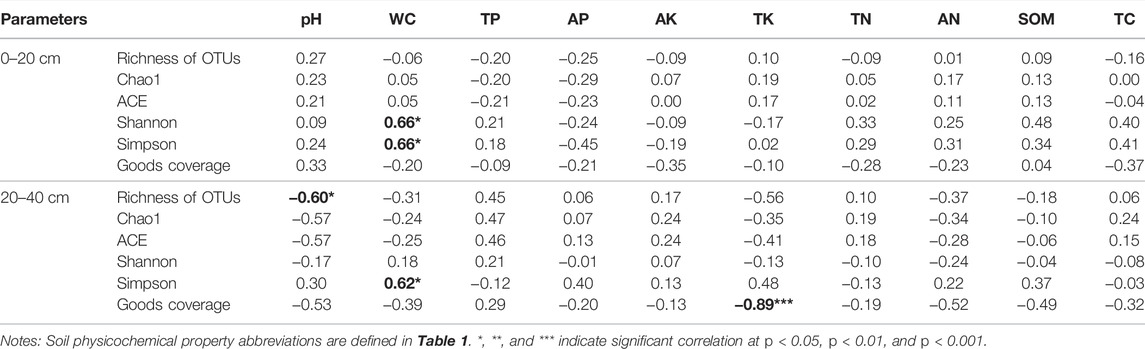
TABLE 3. Pearson’s correlation between alpha diversity indices of bacterial community and soil physicochemical properties.
Conclusion
In this study, we found soil fertility in the non-subsidence site was significantly higher than that in reclamation sites, and topsoil SFI was higher than that in the subsoil. Furthermore, topsoil SFI in FW was higher than that in FS and FP. Proteobacteria, Acidobacteria and Actinobacteria were the dominant bacterial phyla in all sites, and topsoil bacterial Shannon and Simpson indices in FCK were significantly higher than those in reclamation sites. Soil bacterial community in reclamation sites was different from that in non-subsidence site based on the PCoA and NMDS analysis, and the bacterial community composition in FW was more similar to FCK compared with FS and FP. Moreover, co-occurrence network analysis showed topsoil had a more complex network of dominant OTUs compared with that of subsoil. Our study suggested that soil pH was an important driving factor for soil bacterial community composition. Results of this study suggested soil fertility in reclamation sites with a wheat-maize rotation model was higher and it had similar bacterial community composition to that in the non-subsidence site. Therefore, the wheat-maize rotation model is a good choice to improve soil fertility and soil bacterial community composition in coal mining subsidence area filled with fly ash.
Data Availability Statement
The raw data supporting the conclusions of this article will be made available by the authors, without undue reservation.
Author Contributions
Conceptualization: YW, YF, YS, and XZ. Methodology: YW, YF, YS, and XZ. Formal analysis: QW, SZ, and YS. Funding acquisition: YF, XZ, and YW. Project administration: YF, XZ, and YW. Resources: YW and YF. Supervision: XZ, YF, and YW. Validation: XZ, YW, and YF. Investigation: YW, YF, QW, SZ, and XZ. Visualization: YF, QW, SZ, YS, and YW. Writing—original draft: YW, YF, SZ, YS, and XZ Writing—review and editing: YW, YF, YS, and XZ.
Funding
This research was supported by the Natural Science Foundation of Universities of Anhui Province (KJ2020ZD35), the Graduate Innovation Fund Project of Anhui University of Science and Technology (2021CX2011), the Technology R&D Project of Huaibei Mining Group (2020-113 and 2022-103), the National Natural Science Foundation of China (31901195), and the Shandong Provincial Natural Science Foundation (ZR2019BD062).
Conflict of Interest
The authors declare that this study received funding from Huibei Mining (Group) Co., Ltd. The funder was not involved in the study design, collection, analysis, interpretation of data, the writing of this article or the decision to submit it for publication.
Publisher’s Note
All claims expressed in this article are solely those of the authors and do not necessarily represent those of their affiliated organizations, or those of the publisher, the editors and the reviewers. Any product that may be evaluated in this article, or claim that may be made by its manufacturer, is not guaranteed or endorsed by the publisher.
Supplementary Material
The Supplementary Material for this article can be found online at: https://www.frontiersin.org/articles/10.3389/fenvs.2022.937688/full#supplementary-material
References
Cao, M., Cui, L., Sun, H., Zhang, X., Zheng, X., and Jiang, J. (2021). Effects of Spartina Alterniflora Invasion on Soil Microbial Community Structure and Ecological Functions. Microorganisms 9 (1), 138. doi:10.3390/microorganisms9010138
Caporaso, J. G., Lauber, C. L., Walters, W. A., Berg-Lyons, D., Lozupone, C. A., Turnbaugh, P. J., et al. (2011). Global Patterns of 16S rRNA Diversity at a Depth of Millions of Sequences per Sample. Proc. Natl. Acad. Sci. U.S.A. 108, 4516–4522. doi:10.1073/pnas.1000080107
Chen, J., Nan, J., Xu, D., Mo, L., Zheng, Y., Chao, L., et al. (2020). Response Differences between Soil Fungal and Bacterial Communities under Opencast Coal Mining Disturbance Conditions. Catena 194, 104779. doi:10.1016/j.catena.2020.104779
Chen, Y.-L., Deng, Y., Ding, J.-Z., Hu, H.-W., Xu, T.-L., Li, F., et al. (2017). Distinct Microbial Communities in the Active and Permafrost Layers on the Tibetan Plateau. Mol. Ecol. 26 (23), 6608–6620. doi:10.1111/mec.14396
Cui, H., Shi, Y., Zhou, J., Chu, H., Cang, L., and Zhou, D. (2018a). Effect of Different Grain Sizes of Hydroxyapatite on Soil Heavy Metal Bioavailability and Microbial Community Composition. Agric. Ecosyst. Environ. 267, 165–173. doi:10.1016/j.agee.2018.08.017
Cui, Y., Fang, L., Guo, X., Wang, X., Wang, Y., Li, P., et al. (2018b). Responses of Soil Microbial Communities to Nutrient Limitation in the Desert-Grassland Ecological Transition Zone. Sci. Total Environ. 642, 45–55. doi:10.1016/j.scitotenv.2018.06.033
Deng, J., Yin, Y., Luo, J., Zhu, W., and Zhou, Y. (2019). Different Revegetation Types Alter Soil Physical-Chemical Characteristics and Fungal Community in the Baishilazi Nature Reserve. PeerJ 6, e6251. doi:10.7717/peerj.6251
Duo, L., and Hu, Z. (2018). Soil Quality Change after Reclaiming Subsidence Land with Yellow River Sediments. Sustainability 10 (11), 4310. doi:10.3390/su10114310
Feng, Y., Wang, J., Bai, Z., and Reading, L. (2019). Effects of Surface Coal Mining and Land Reclamation on Soil Properties: A Review. Earth Sci. Rev. 191, 12–25. doi:10.1016/j.earscirev.2019.02.015
Gao, Y., He, N., Yu, G., Chen, W., and Wang, Q. (2014). Long-Term Effects of Different Land Use Types on C, N, and P Stoichiometry and Storage in Subtropical Ecosystems: A Case Study in China. Ecol. Eng. 67, 171–181. doi:10.1016/j.ecoleng.2014.03.013
Greenblum, S., Turnbaugh, P. J., and Borenstein, E. (2012). Metagenomic Systems Biology of the Human Gut Microbiome Reveals Topological Shifts Associated with Obesity and Inflammatory Bowel Disease. Proc. Natl. Acad. Sci. U.S.A. 109 (2), 594–599. doi:10.1073/pnas.1116053109
Guo, X.-M., Zhao, T.-Q., Chang, W.-K., Xiao, C.-Y., and He, Y.-X. (2018). Evaluating the Effect of Coal Mining Subsidence on the Agricultural Soil Quality Using Principal Component Analysis. Chil. J. Agric. Res. 78 (2), 173–182. doi:10.4067/S0718-58392018000200173
Hemalatha, T., and Ramaswamy, A. (2017). A Review on Fly Ash Characteristics - Towards Promoting High Volume Utilization in Developing Sustainable Concrete. J. Clean. Prod. 147, 546–559. doi:10.1016/j.jclepro.2017.01.114
Hill, R., Saetnan, E. R., Scullion, J., Gwynn-Jones, D., Ostle, N., and Edwards, A. (2016). Temporal and Spatial Influences Incur Reconfiguration of Arctic Heathland Soil Bacterial Community Structure. Environ. Microbiol. 18 (6), 1942–1953. doi:10.1111/1462-2920.13017
Hu, Y., Zhang, R., Qie, X., and Liu, H. (2022). Evaluation of China’s Coal Resource Energy Carbon Emission Efficiency in the Whole Life Cycle. China Environ. Sci. (in Chinese). doi:10.19674/j.cnki.issn1000-6923.20220218.002
Hu, Z. (2019). The 30 years’ Land Reclamation and Ecological Restoration in China: Review, Rethinking and Prospect. Coal Sci. Technol. 47 (1), 25–35. (in Chinese). doi:10.13199/j.cnki.cst.2019.01.004
Jiao, S., Zhang, Z., Yang, F., Lin, Y., Chen, W., and Wei, G. (2017). Temporal Dynamics of Microbial Communities in Microcosms in Response to Pollutants. Mol. Ecol. 26 (3), 923–936. doi:10.1111/mec.13978
Kohler, J., Caravaca, F., Azcón, R., Díaz, G., and Roldán, A. (2016). Suitability of the Microbial Community Composition and Function in a Semiarid Mine Soil for Assessing Phytomanagement Practices Based on Mycorrhizal Inoculation and Amendment Addition. J. Environ. Manage. 169, 236–246. doi:10.1016/j.jenvman.2015.12.037
Lal, R. (2002). Soil Carbon Dynamics in Cropland and Rangeland. Environ. Pollut. 116, 353–362. doi:10.1016/s0269-7491(01)00211-1
Lehmann, J., Rillig, M. C., Thies, J., Masiello, C. A., Hockaday, W. C., and Crowley, D. (2011). Biochar Effects on Soil Biota - A Review. Soil Biol. Biochem. 43 (9), 1812–1836. doi:10.1016/j.soilbio.2011.04.022
Li, J. P., Xu, M. F., Su, Z. Y., Sun, Y. D., and Hu, Y. Q. (2014). Soil Fertility Quality Assessment under Different Vegetation Restoration Patterns. Acta Eco. Sin. 34 (9), 2297–2307. (in Chinese). doi:10.5846/stxb201306111672
Li, J., Xin, Z., Yan, J., Li, H., Chen, J., and Ding, G. (2018). Physicochemical and Microbiological Assessment of Soil Quality on a Chronosequence of a Mine Reclamation Site. Eur. J. Soil Sci. 69 (6), 1056–1067. doi:10.1111/ejss.12714
Li, S., and Liber, K. (2018). Influence of Different Revegetation Choices on Plant Community and Soil Development Nine Years after Initial Planting on a Reclaimed Coal Gob Pile in the Shanxi Mining Area, China. Sci. Total Environ. 618, 1314–1323. doi:10.1016/j.scitotenv.2017.09.252
Liu, T., Wu, X., Li, H., Alharbi, H., Wang, J., Dang, P., et al. (2020). Soil Organic Matter, Nitrogen and pH Driven Change in Bacterial Community Following Forest Conversion. For. Ecol. Manag. 477, 118473. doi:10.1016/j.foreco.2020.118473
Liu, Z., Chen, M., Li, Y., Huang, Y., Fan, B., Lv, W., et al. (2018). Different Effects of Reclamation Methods on Macrobenthos Community Structure in the Yangtze Estuary, China. Mar. Pollut. Bull. 127, 429–436. doi:10.1016/j.marpolbul.2017.12.038
Lu, R. K. (2000). Agricultural Chemical Analysis of Soil. Beijing: China Agriculture Press. (in Chinese).
Luo, Y., Zheng, Z., Wu, P., and Wu, Y. (2022). Effect of Different Direct Revegetation Strategies on the Mobility of Heavy Metals in Artificial Zinc Smelting Waste Slag: Implications for Phytoremediation. Chemosphere 286, 131678. doi:10.1016/j.chemosphere.2021.131678
Ma, J., Gonzalez‐Ollauri, A., Zhang, Q., Xiao, D., and Chen, F. (2021). Ecological Network Analysis to Assess the Restoration Success of Disturbed Mine Soil in Zoucheng, China. Land Degrad. Dev. 32 (18), 5393–5411. doi:10.1002/ldr.4116
Ma, J., Lu, Y., Chen, F., Li, X., Xiao, D., and Wang, H. (2020). Molecular Ecological Network Complexity Drives Stand Resilience of Soil Bacteria to Mining Disturbances Among Typical Damaged Ecosystems in China. Microorganisms 8 (3), 433. doi:10.3390/microorganisms8030433
Ma, K., Zhang, Y., Ruan, M., Guo, J., and Chai, T. (2019). Land Subsidence in a Coal Mining Area Reduced Soil Fertility and Led to Soil Degradation in Arid and Semi-Arid Regions. Int. J. Environ. Res. Public Health 16 (20), 3929. doi:10.3390/ijerph16203929
Madigan, M., Cox, S. S., and Stegeman, R. A. (1984). Nitrogen Fixation and Nitrogenase Activities in Members of the Family Rhodospirillaceae. J. Bacteriol. 157 (1), 73–78. doi:10.1128/jb.157.1.73-78.1984
Pandey, V. C., Singh, K., Singh, R. P., and Singh, B. (2012). Naturally Growing Saccharum Munja L. on the Fly Ash Lagoons: A Potential Ecological Engineer for the Revegetation and Stabilization. Ecol. Eng. 40, 95–99. doi:10.1016/j.ecoleng.2011.12.019
Pandey, V. C. (2013). Suitability of Ricinus Communis L. Cultivation for Phytoremediation of Fly Ash Disposal Sites. Ecol. Eng. 57, 336–341. doi:10.1016/j.ecoleng.2013.04.054
Qi, L., Zhou, P., Yang, L., and Gao, M. (2020). Effects of Land Reclamation on the Physical, Chemical, and Microbial Quantity and Enzyme Activity Properties of Degraded Agricultural Soils. J. Soils Sediments 20 (2), 973–981. doi:10.1007/s11368-019-02432-1
Qu, J.-F., Hou, Y.-L., Ge, M.-Y., Wang, K., Liu, S., Zhang, S.-L., et al. (2017). Carbon Dynamics of Reclaimed Coal Mine Soil under Agricultural Use: A Chronosequence Study in the Dongtan Mining Area, Shandong Province, China. Sustainability 9 (4), 629. doi:10.3390/su9040629
Qu, J. F., Tan, M., Hou, Y. L., Ge, M. Y., Wang, A. N., Wang, K., et al. (2018). Effects of the Stability of Reclaimed Soil Aggregates on Organic Carbon in Coal Mining Subsidence Areas. Appl. Eng. Agric. 34 (5), 843–854. doi:10.13031/aea.12829
Ram, L. C., and Masto, R. E. (2014). Fly Ash for Soil Amelioration: A Review on the Influence of Ash Blending with Inorganic and Organic Amendments. Earth Sci. Rev. 128, 52–74. doi:10.1016/j.earscirev.2013.10.003
Sauret, C., Séverin, T., Vétion, G., Guigue, C., Goutx, M., Pujo-Pay, M., et al. (2014). 'Rare Biosphere' Bacteria as Key Phenanthrene Degraders in Coastal Seawaters. Environ. Pollut. 194, 246–253. doi:10.1016/j.envpol.2014.07.024
Smoot, M. E., Ono, K., Ruscheinski, J., Wang, P.-L., and Ideker, T. (2011). Cytoscape 2.8: New Features for Data Integration and Network Visualization. Bioinformatics 27 (3), 431–432. doi:10.1093/bioinformatics/btq675
Srivastava, N. K., Ram, L. C., and Masto, R. E. (2014). Reclamation of Overburden and Lowland in Coal Mining Area with Fly Ash and Selective Plantation: A Sustainable Ecological Approach. Ecol. Eng. 71, 479–489. doi:10.1016/j.ecoleng.2014.07.062
Sun, S., Sun, H., Zhang, D., Zhang, J., Cai, Z., Qin, G., et al. (2019). Response of Soil Microbes to Vegetation Restoration in Coal Mining Subsidence Areas at Huaibei Coal Mine, China. Int. J. Environ. Res. Public Health 16 (10), 1757. doi:10.3390/ijerph16101757
Wan, P., and He, R. (2020). Soil Microbial Community Characteristics under Different Vegetation Types at the National Nature Reserve of Xiaolongshan Mountains, Northwest China. Ecol. Inf. 55, 101020. doi:10.1016/j.ecoinf.2019.101020
Wang, K., Zhang, J., Yi, Q., Wang, X., and Yi, Y. (2021). Metals in Sediments and Their Accumulation in Zostera Japonica in Different Sediment Habitats of the Yellow River Estuary. J. Soils Sediments 21 (3), 1539–1549. doi:10.1007/s11368-021-02875-5
Wang, L., Li, J., Yang, F., E, Y., Raza, W., Huang, Q., et al. (2017). Application of Bioorganic Fertilizer Significantly Increased Apple Yields and Shaped Bacterial Community Structure in Orchard Soil. Microb. Ecol. 73 (2), 404–416. doi:10.1007/s00248-016-0849-y
Wang, X., Li, Y., Wei, Y., Meng, H., Cao, Y., Lead, J. R., et al. (2020). Effects of Fertilization and Reclamation Time on Soil Bacterial Communities in Coal Mining Subsidence Areas. Sci. Total Environ. 739, 139882. doi:10.1016/j.scitotenv.2020.139882
Wolińska, A., Włodarczyk, K., Kuźniar, A., Marzec-Grządziel, A., Grządziel, J., Gałązka, A., et al. (2020). Soil Microbial Community Profiling and Bacterial Metabolic Activity of Technosols as an Effect of Soil Properties Following Land Reclamation: A Case Study from the Abandoned Iron Sulphide and Uranium Mine in Rudki (South-Central Poland). Agronomy 10 (11), 1795. doi:10.3390/agronomy10111795
Xu, M., Gao, D., Fu, S., Lu, X., Wu, S., Han, X., et al. (2020). Long-Term Effects of Vegetation and Soil on the Microbial Communities Following Afforestation of Farmland with Robinia Pseudoacacia Plantations. Geoderma 367, 114263. doi:10.1016/j.geoderma.2020.114263
Xu, Z., Zhang, Y., Yang, J., Liu, F., Bi, R., Zhu, H., et al. (2019). Effect of Underground Coal Mining on the Regional Soil Organic Carbon Pool in Farmland in a Mining Subsidence Area. Sustainability 11 (18), 4961. doi:10.3390/su11184961
Yang, Y., Cheng, H., Liu, L., Dou, Y., and An, S. (2020). Comparison of Soil Microbial Community between Planted Woodland and Natural Grass Vegetation on the Loess Plateau. For. Ecol. Manag. 460, 117817. doi:10.1016/j.foreco.2019.117817
Yu, X., Cui, Y., Chen, Y., Chang, I.-S., and Wu, J. (2022). The Drivers of Collaborative Innovation of the Comprehensive Utilization Technologies of Coal Fly Ash in China: A Network Analysis. Environ. Sci. Pollut. Res.. doi:10.1007/s11356-022-19816-5
Yuan, C., Li, F., Yuan, Z., Li, G., and Liang, X. (2021). Response of Bacterial Communities to Mining Activity in the Alpine Area of the Tianshan Mountain Region, China. Environ. Sci. Pollut. Res. 28 (13), 15806–15818. doi:10.1007/s11356-020-11744-6
Zhao, Y., Zhang, F., Yang, L., Wang, D., and Wang, W. (2019). Response of Soil Bacterial Community Structure to Different Reclamation Years of Abandoned Salinized Farmland in Arid China. Arch. Microbiol. 201 (9), 1219–1232. doi:10.1007/s00203-019-01689-x
Zhou, W.-H., Wang, Y.-T., Lian, Z.-H., Yang, T.-T., Zeng, Q.-W., Feng, S.-W., et al. (2020). Revegetation Approach and Plant Identity Unequally Affect Structure, Ecological Network and Function of Soil Microbial Community in a Highly Acidified Mine Tailings Pond. Sci. Total Environ. 744, 140793. doi:10.1016/j.scitotenv.2020.140793
Keywords: soil bacterial community composition, reclamation, coal mining subsidence area, revegetation, bacterial alpha diversity
Citation: Wang Y, Fan Y, Wang Q, Zhang S, Shi Y and Zheng X (2022) Response of Soil Fertility and Bacterial Community Composition to Vegetation Species in a Coal Mining Subsidence Area: A Survey After 20-Year Reclamation. Front. Environ. Sci. 10:937688. doi: 10.3389/fenvs.2022.937688
Received: 06 May 2022; Accepted: 16 May 2022;
Published: 04 July 2022.
Edited by:
Zhu Li, Institute of Soil Science (CAS), ChinaReviewed by:
Yi Cheng, Nanjing Normal University, ChinaChao Ma, Anhui Agricultural University, China
Copyright © 2022 Wang, Fan, Wang, Zhang, Shi and Zheng. This is an open-access article distributed under the terms of the Creative Commons Attribution License (CC BY). The use, distribution or reproduction in other forums is permitted, provided the original author(s) and the copyright owner(s) are credited and that the original publication in this journal is cited, in accordance with accepted academic practice. No use, distribution or reproduction is permitted which does not comply with these terms.
*Correspondence: Yuchao Fan, ahfanyuchao@126.com; Xuebo Zheng, zhengxuebo@caas.cn