- 1School of Business Administration, Zhongnan University of Economics and Law, Wuhan, China
- 2Waseda Institute for Advanced Study, Waseda University, Tokyo, Japan
- 3Institute for Future Initiatives, The University of Tokyo, Tokyo, Japan
The rapid expansion of electric vehicles (EVs) in China will induce a potential imbalance in the demand and supply of critical metals, which emphasized the importance of recycling critical metals. Evaluating their stock and recycling potential is essential to the electrification transformation in the automobile industry and provides references to the overall national resource strategy. In this study, we identified the critical metals in the electrification transformation of the automobile industry, estimated the stock of critical metals from 2022 to 2050 under multiple scenarios in China, and assessed the recycling potential of critical metals in EVs as well as their economic value. The results show that China’s passenger vehicles will reach 547.5–623.8 million in 2050. According to China’s current energy conservation and emission reduction policies, fuel vehicle (FV) ownership will peak in 2042, at 488.2 million. If strict energy conservation and emission reduction policies are adopted, electric vehicle ownership will increase from 148.3 million to 293.9 million by 2050, leading to a rapid increase in the stock of critical metals. The total stock of key critical metals under the scenario with stringent policies will be 29.27 million tons in 2050, 10.55 million higher compared to the scenario with no ban on fuel vehicles. Based on our results, the recycling of critical metals can be an effective option for the inadequate critical metal supply, especially given the policy context of increasingly expanding EV ownership in the near-term future.
1 Introduction
China remains the largest automobile manufacturing country and automobile market in the world since 2009. China’s annual vehicle production has accounted for over 30% percent of global vehicle production. In 2021, China’s vehicle production and sales reached 26.08 million and 26.28 million, with a year-on-year increase of 3.4 and 3.8%, respectively. The production and sales of new energy vehicles (NEVs) were 3.55 million and 3.52 million, respectively, with a year-on-year increase of 160%. The NEV market has a great potential for development in the future. The automobile industry is one of the world’s largest consumers of raw materials (Serrenho et al., 2017). In recent years, more and more new materials have been used for automobile components such as engines, sensors, and electronic equipment to make safer, more complex vehicles (Ortego et al., 2020; Sharma and Pandey, 2020). The manufacturing of NEVs consumes many critical metals, including scarce supplies of Li, Co, rare earth, and others. Specifically in China, serious resource shortages may occur for several key minerals given the carbon neutrality goal by 2050, such as Cr, Cu, Mn, Ag, Te, Ga, and Co (Wei et al., 2022). The possible imbalances in the supply and demand of those critical metals call for a more detailed assessment in the near and long run.
The definition, demand, and supply of critical metals (as shown in Figure 1) in the automobile industry, especially NEVs should be clarified first. The critical metals refer to those metal resources or raw materials that have a high supply risk and an important impact on the national economy, social welfare, and the ecological environment and development strategies (Ge and Liu, 2020; Li et al., 2022). They are of great significance to national security and the development of emerging industries. However, regarding the boundary, it differs among countries. Table 1 summarized critical metal development strategies in different countries in recent years and shows the differences in the definition boundaries.
Regarding the demand of critical metals, in the context of an accelerating sustainable transformation, the development of relevant strategic industries such as NEVs, high-end equipment, next-generation information technology, and energy conservation and environmental protection would induce great demand for the critical metals (Grandell et al., 2016; Fishman and Graedel, 2019). For example, Valero et al. (2018) pointed out that the development of global wind energy, solar photovoltaic, solar thermal energy, and other industries from 2016 to 2050 will increase the demand for 31 critical minerals. Deetman et al. (2018) pointed out that the development of power production and electronic equipment will increase the global total demand for Nd and Ta by about 2–3.2 times in 2050. Zhou et al. (2019) assessed the increase in demand for 12 critical metals caused by the development of solar and wind energy in China.
Moreover, the transformation from fuel vehicles (FVs) to electric vehicles (EVs) has led to great changes in the demand for critical metals (International Energy Agency, 2021). Scholars have discussed critical metals for the development of automobile electrification in many aspects. Simon et al. (2015) analyzed EVs in Europe and pointed out that they are continuously increasing, the shortage of Li and Ni reserves could intensify around 2025, and the demand for Co and Mn will be far lower than the current level. Liu et al. (2021) analyzed the material flow changes of Li batteries in China and found the development of EVs after 2015 is the main reason for the increase in Li-battery consumption. Wang et al. (2018) assessed the global supply risk of 23 critical raw materials required by NEVs. Li X. Y.et al. (2019) based on the sales data of China’s NEVs, pointed out that from 2018 to 2030, the maximum total demand for rare earth will reach 315,000 tons, accounting for 22% of the world output in the same period, while the demand for the commonly used elements (Nd, Dy, Ce, Pr, and La) will account for 99% of the total demand.
The large demand for critical metals has brought supply pressure. Habib et al. (2020) pointed out that with the development of electrification in the future, the reserves of Co, Li, and Ni will face great pressure. As critical metals are mostly mined in the form of symbiotic or associated ore, the capacity expansion is slow and the development cost is too high (Ali et al., 2017). Recycling is regarded as a powerful measure to relieve the supply pressure of critical metals (Zuo and Wang., 2020). Many scholars analyzed the recycling potential of critical metals in vehicles (Yano et al., 2016; Xu et al., 2016). Xu et al. (2016) pointed out that recycling Nd and Dy could meet 23% of the production need for EV batteries in Japan. In addition, Ahmadi et al. (2014) also pointed out that by recycling Li batteries, carbon dioxide can be reduced by 56%. Critical metals recycling can reduce the pressure on resources and the environment, which is conducive to sustainable development and carbon emission reduction (Cheng et al., 2021). To sum up, most of the existing studies focus on developed countries to analyze the definition, demand, and supply risks of critical metals. The analysis of medium and long-term stock and recovery potential of Critical metals in the process of automobile electrification transformation in developing countries is not sufficient.
The acceleration of automobile electrification transformation has led to the rapid growth of the consumption of critical metals. EV is an important dimension of green development and low-carbon transformation in the global automobile industry, however, with huge consumption of critical metals. As the world’s largest automobile market, China’s rapid expansion of EVs in the short term will bring great pressure on the demand for critical metals and provide great recycling potential. Accurately evaluating the change and recovery potential of medium and long-term critical metal inventory is of great significance to the sustainable development of the automobile industry. Therefore, the objective of this study is to clarify the relationship between electrification transformation, fuel ban policy, and critical metal supply risk in China’s automobile industry, predict the trend of medium and long-term critical metal inventory and recovery potential, and put forward policy suggestions to reduce the imbalance between supply and demand of Critical metal minerals. This study provides a reference for the sustainable supply of critical metals required for the electrification transformation and sustainable development of the automobile industry.
The methodologies for evaluating the passenger vehicle ownership (Section 2.1), critical metal stock (Section 2.2), recycled critical metals from FVs and EVs (Section 2.3), together with the scenario designs (Section 2.4) are listed in Chapter 2, followed with the corresponding results in Chapter 3. We also discussed the strategic risk, resource governance, and uncertainties in Chapter 4. The conclusions are listed in Chapter 5.
2 Methodology
Long-term predictions of stock and flows can effectively reveal the maximum recycling potential and supply risk of critical metals. This study identified the critical metals in the electrification transformation of the automobile industry, estimated the stock of critical metals from vehicles from 2022 to 2050 under multiple scenarios, and discussed the economic value and maximum recycling potential of critical metals in EVs. The critical metal stock model constructed in this paper can be applied to the stock analysis of other industries and different materials. The estimation results obtained based on the model can provide a scientific basis for promoting the recycling of material resources. The steps of this study are shown in Figure 2.
Specifically, 1) combined with literature and policy analysis, this study selects the critical metals in the electrification transformation of China’s automobile industry as the research object. 2) Referring to the experience of developed countries and the actual situation of China, setting four scenarios: the saturation value of passenger vehicle ownership per thousand people (high or low) and fuel ban policy (planned or unplanned), using the Gompertz function to predict passenger vehicle ownership. 3) Combined the above four scenarios, predicting the stock changes of critical metal stocks of different vehicles (FV and EV) from 2022 to 2050 in China’s automobile industry. 4) Combined with the previous research results (Li et al., 2021), the economic value and maximum recovery potential of critical metals in EV under different recovery levels (high and low) are discussed.
2.1 Passenger vehicle ownership prediction model
The number of passenger vehicles owned is jointly determined by the population and the passenger vehicle ownership per thousand people. It can be formulated as:
where
The critical metal stock is closely related to the number of vehicle ownership. The methods for estimating the critical metal stock based on vehicle ownership are numerous. For the long-term prediction of regional vehicle ownership, saturation level modeling, such as regression models is usually used. The correlation between vehicle ownership and main driving factors is established by the regression model. Compared with developed countries, China is in the primary stage of automobile popularization. In the initial stage, income growth is one of the most important driving forces for the growth of vehicle ownership (Dargay et al., 2007; Li Y. et al., 2019). According to the experience of developed countries (Huo & Wang, 2012), the development trend of vehicle ownership per thousand people can be an S-shaped “slow–urgent–slow” development process, namely the slow growth in the initial stage, rapid growth in the medium term, slow growth in the later stage, and entering the saturation stage. Therefore, according to the characteristics of changes in vehicle ownership, we used the S-curve model to predict passenger vehicle ownership. Commonly used S-shaped curves include the Richards, logistic, and Gompertz functions (Weiner, 1990). Due to the short development time of China’s automobile industry, the availability of data should be considered in the selection of methods. The Richards function involves many parameters, and the logistic function is most suitable for an S-shaped curve with a symmetrical inflection point, while an S-shaped curve with right deflection is well fitted by the Gompertz model (Lee & Shaw, 1996). Compared with the Richards function, the Gompertz function uses simple data and is more suitable for predicting the growth of vehicle ownership in developing countries such as China. Compared with the logistic function, the Gompertz model shows an upward state in the later stage of growth, and other explanatory variables can be added to the model to integrate the development of the automobile industry into the national macroeconomic development system, which is more in line with the changes in China’s passenger vehicle ownership. The forecast of the Gompertz curve is flexible and the parameter and saturation value can be adjusted according to the policies, which can be more suitable for medium and long-term prediction at the national level. Therefore, this paper uses the Gompertz model to predict the number of passenger vehicles per thousand people in China in the future. The formula is as follows:
where
Regarding the Estimation of population, the incidence rate is a virtual variable in population prediction, such as survival and mortality rates, incidence and non-incidence rates, and gender ratio. At this time, it is no longer feasible to study such problems with a linear regression model; a logistic regression model can analyze multiple independent variables, including discrete and continuous variables, at the same time, and can effectively analyze the interactions between independent variables, providing a quantitative description of the relationships between multiple independent and dependent variables. The logistic model has the characteristics of limited growth and monotonic increase, which is in line with the population growth model. However, the defect of this model is that population growth generally shows an upward trend in the short term (30–50 years). This study assumes that China’s population growth will continue to grow from 2020 to 2050, so it can be predicted by logistic regression model.
According to the research needs, combined with the logistic curve model equation, the following population prediction formula can be obtained:
where
2.2 Critical metal stock model for passenger vehicles
In this paper, the bottom-up method is used to calculate the material stock of passenger vehicles, which is determined according to the weight of each critical metal per vehicle. The formula is as follows:
where
2.3 Estimation of recycled critical metals and their economic value
The total weight of recycled critical metals from end-of-life passenger vehicles,
where
The estimation of the economic value of the
where
2.4 Scenario design
2.4.1 Total passenger vehicle ownership
This paper refers to passenger vehicle ownership per thousand people in countries with a developed automobile industry when setting the saturation value. Taking the US, United Kingdom, France, and Japan as reference objects, the average value of the growth rate of passenger vehicle ownership per thousand people fluctuated by within 2% (±) for more than four consecutive years, and this is taken as the saturation value of the country. Calculating the data from 1960 to 2012, the following saturation values can be obtained: 513 vehicles per thousand people in the US, 472 vehicles per thousand people in the United Kingdom, 433 vehicles per thousand people in France, and 446 vehicles per thousand people in Japan, with an average value of the four countries of 466 vehicles per thousand people (Li et al., 2021). As China’s auto market has not yet entered the saturation stage and the level of domestic economic development is uneven, referring to the historical data of the above countries and combined with the characteristics of China, this paper sets the saturation value of passenger vehicle ownership in two scenarios: 1) under the low saturation (LS) level of passenger vehicle ownership, the saturation value per thousand people is 350, and 2) under the high saturation (HS) level of passenger vehicle ownership, the saturation value is 400.
2.4.2 Ownership of EVs
In recent years, in order to reduce carbon emissions, some countries began to vigorously promote vehicle electrification (Kromer and Heywood, 2007) and proposed banning the sale of new FVs. Table 2 shows the timeline for the implementation of bans on the sale of FVs.
During 2016–2018, many countries proposed a ban on FV sales. The earliest implementation time was in Rome, Italy, in 2024. Other countries focus on the period from 2030 to 2040. In China, Hainan first proposed to ban the sale of FVs from 2030 at a press conference for the Information Office of the State Council. Banning the sale of FVs will inevitably change the proportion of BEVs. To better understand the impact of these bans, this paper analyzes the potential outcomes in terms of changes in material stocks and carbon emissions, with 2035 taken as the time point for the implementation of bans.
As the ratio of EV ownership to vehicle ownership and the ratio of new energy passenger vehicle ownership to passenger vehicle ownership are similar (China Automobile Industry Association, 2021), in this paper we estimate the proportion of EV ownership to obtain the proportion of new energy passenger vehicle ownership. According to the predictions of the China Automobile Association, the sales volume of EVs in China will reach 50% in 2035. By considering the relationship between the proportions of EV sales and EV ownership from 2015 to 2020, this paper infers such a relationship for the future. It is found that the average ratio of the proportion of EV ownership to the proportion of sales in the past 6 years is 0.27. Based on this, two scenarios are set: 1) In the case of NBS (no plan to ban sales of FVs), the proportion of EVs in 2035 will be 13.7%, and 2) in the case of SBS (plan to ban sales of FVs), the proportion of EVs in 2035 will be 27.39%. The proportion of EV ownership from 2021 to 2050 was fitted by a logarithmic function.
Thus, the overall situation can be divided into four scenarios:
1) HS-NBS: high saturation of passenger vehicle ownership and no plan to ban sales of FVs
2) HS-SBS: low saturation of passenger vehicle ownership and plan to ban sales of FVs
3) LS-NBS: low saturation of passenger vehicle ownership and no plan to ban sales of FVs
4) LS-SBS: low saturation of passenger vehicle ownership and plan to ban sales of FVs
When analyzing the recycling potential, we also adopted 2 levels of recycling rate (high recycling rate for HR and low for LR) from Li et al. (2021).
2.5 Data source
The population, per capita GDP, and passenger vehicle ownership data in China from 1990 to 2020 used in this paper are from the China Statistical Yearbook (2021). The data of EV ownership and sales volume from 2015 to 2020 are from the public data of the Ministry of Public Security (Ministry of Public Security of the People’s Republic of China, 2016) and the China Automobile Industry Yearbook (2021).
According to the research, among the critical minerals specified by the state, Ni, Cr, Co, Li, and REE are very important metals in the development of EVs (Wang et al., 2018; Zhou, et al., 2019). In addition, Mn is often studied as a critical material for automobiles (Olivetti et al., 2017). Among rare earth elements, Ce, Eu, La, Nd, PR, Dy, Gd, TB, and Y are commonly used in EVs (Field et al., 2017). Among them, Ni, Co, Li, and REE are the critical metals in great demand in the development of EVs, while Mn and Cr are the critical metals in great demand in both EVs and FV. At the same time, Ni, Co, Li, Cr, and Mn are characterized by low reserves and high external dependence in China (Ministry of Land and Resources of the People’s Republic of China, 2016). Therefore, Ni, Cr, Co, Li, Pt, Mn, and rare earth elements (Ce, Eu, La, Nd, Pr, Dy, Gd, Tb, and Y) were selected as the critical metals in this paper. The specific parameters and sources are shown in Table 3.
Regarding the representative type of EV, at present, BEV and HEV are the main EVs in China. According to the current development of these two vehicles, BEV ownership has accounted for more than 80% of EV ownership in the past 6 years (China Automobile Industry Association, 2021). In addition, according to national policies, at the end of 2018, the China Development and Reform Commission issued the regulations on investment management in the automotive industry, which mentioned that future PHEV investment projects will be approved and filed in accordance with the requirements of FV projects (National Development and Reform Commission, 2018). In addition, relevant subsidy policies for PHEV have been gradually abolished. It can be seen that HEV, as a transitional product, will gradually withdraw from the market, and BEV will further expand in the future. In addition, in terms of the selection of critical metals. This paper selects metals that are indispensable in the development of EV and are currently highly dependent on imports from China, such as Li, Ni, Co. According to the research of Ortego et al. (2020), the single-vehicle metal composition of Li, Ni, and Co in PHEV is 2,242g, 16,049.57g, and 2,712g, which is far lower than 7,709g, 55,724g and 9,330 g of BEV. With the further increase of BEV’s market share in EV, the demand for these critical metals will further expand. In order to cover such critical metals, BEV is selected as the representative type of EV in this paper.
Regarding the unit price of recycled resources, we considered the unit price of both primary resource market and secondary resource market from Shanghai Nonferrous Metals Industry Association (2022).
3 Result
3.1 Passenger vehicle ownership in China
3.1.1 GDP per capital forecast
This paper posits that China’s economy will grow steadily up to 2050. By fitting the time series data from 1978 to 2020 (constant prices in 1978), the prediction function of GDP per capita in the future is as follows:
where variable x ranges from 1 to 73, representing the years 1978–2050.
Figure 3 shows China’s GDP per capita from 2021 to 2050 predicted according to GDP per capita from 1978 to 2020.
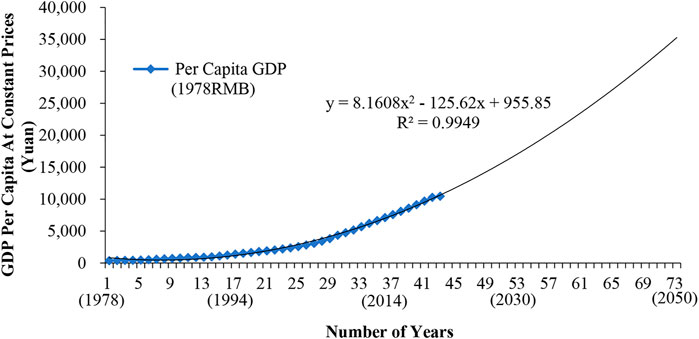
FIGURE 3. Prediction of China’s GDP per capita (constant prices in 1978). This study assumes that the GDP per capita will continue to grow within the forecast range.
According to the forecast, the GDP per capita in 2035 will be 17,222 yuan. In 2050, it will be 35,274 yuan, which is almost three times the value in 2020.
3.1.2 Population forecast
This paper uses the actual population data of China from 1949 to 2020 by MATLAB programming fitting and predicts the population from 2021 to 2050. The fitting between predicted and actual data is better. According to the fitting data,
The fitting result of Equation 8 is shown in Figure 4.
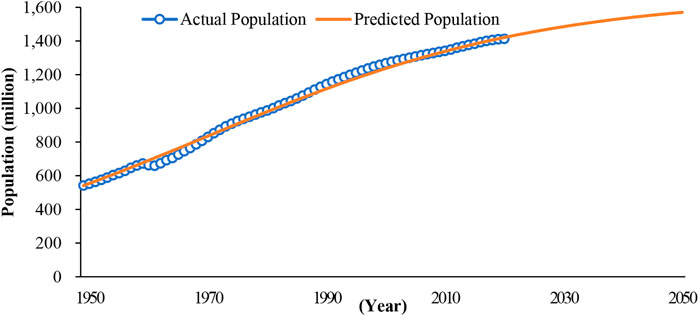
FIGURE 4. Population trends in China. This study will predict that China’s population will continue to grow.
The population in 2035 and 2050 will be 1,511.45 million and 1,570.32 million, and the growth rates from 2021 to 2035 and 2036 to 2050 will be 5.82 and 3.57%, respectively. Although China’s population will continue to grow in the future, the rate will slow down.
3.1.3 Prediction of passenger vehicle ownership per thousand people
This paper considers the ownership of passenger vehicles by setting LS and HS scenarios. Table 4 shows the estimation of passenger vehicle ownership per thousand people from 2025 to 2050 under LS and HS scenarios.
3.1.4 Prediction of passenger vehicle ownership
Table 5 shows the overall change in passenger vehicle ownership under LS and HS scenarios from 2025 to 2050.
Under the LS scenario, China’s passenger vehicle ownership will reach 436.03 million in 2030, increase by 84.53 million in 2040 to reach 520.56 million, and reach 547.50 million in 2050, an increase of 2.05 times compared with 2021. The number of passenger vehicles will reach 623.77 million under the HS scenario in 2050, which is 76.27 million more than that of the LS scenario.
Based on two ownership scenarios, HS and LS, and adding the plan to ban sales of FVs for analysis, four EV and FV ownership scenarios of HS-NBS, HS-SBS, LS-NBS, and LS-SBS can be obtained. Figure 5 shows the changes in EV and FV ownership from 2022 to 2050 under the four scenarios.
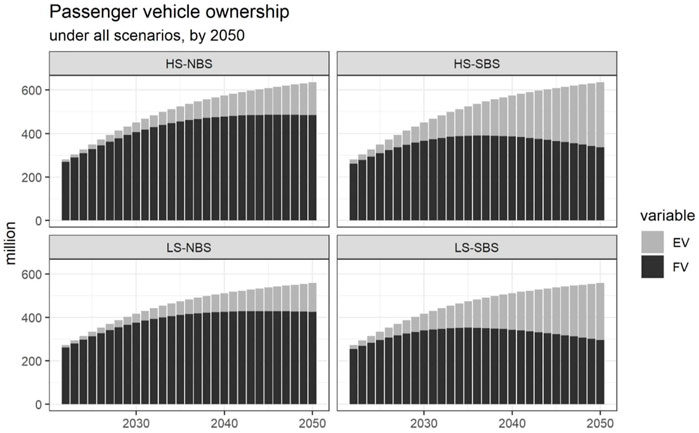
FIGURE 5. Estimation of EV and FV ownership under four scenarios from 2022 to 2050 in China. Under the four scenarios, passenger vehicle ownership continued to rise. The growth rate of the two scenarios under HS is higher than that under LS. FV ownership will reach its peak at different time points under the four scenarios.
Under the HS-NBS scenario, EV ownership will reach 46.48 million, 98.53 million, and 148.27 million in 2030, 2040, and 2050, respectively, while FV ownership will also increase, reaching 475.50 million in 2050. Under HS-SBS, the growth rate of EV ownership is 16.79%, higher than the 13.30% for HS-NBS. Moreover, the peak value of FV advances from 2042 to 2035 for HS-NBS, and peak ownership is reduced from 488.17 million to 402.65 million. EV and FV ownership are slightly lower in the LS scenario than the HS scenario, but the FV peak value will be reached sooner: 364 million in 2034 under LS-SBS vs 432.96 million in 2040 under LS-NBS.
3.2 Critical metal stock for passenger vehicles
Table 6 shows the changes in total stocks of critical metals under the four scenarios from 2022 to 2050.
Under the HS-NBS scenario, the total stock of critical metals will reach 18.72 million tons in 2050. Under HS-SBS, the total stock of critical metals will increase rapidly, reaching 29.27 million tons in 2050, showing banning FV sales has a great impact on the stock of critical metals.
The change of a single metal under the four scenarios is similar to that of the total stock. Next, we take a single scenario as an example to analyze the stock of each critical metal. Figure 6 shows the changes in the stock of critical metals under the HS-NBS scenario from 2022 to 2050.
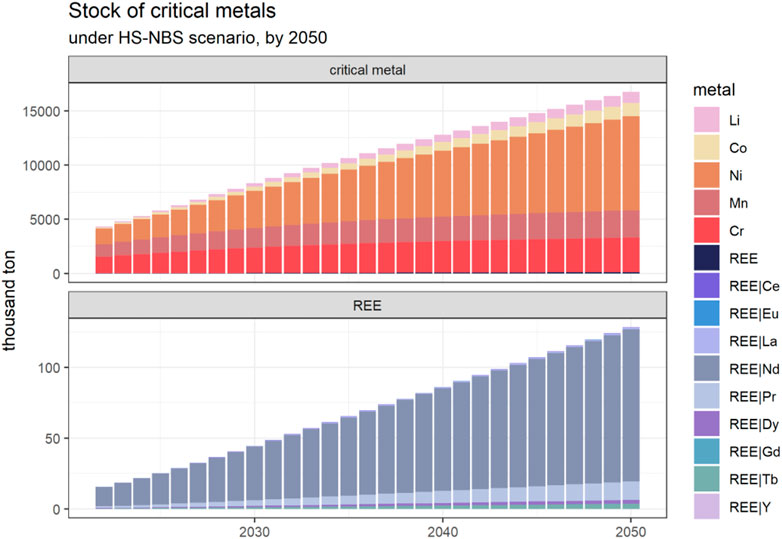
FIGURE 6. Stock of critical metals under HS-NBS scenario from 2022 to 2050 in China (thousand tons): total metal stock and the details in REE. Under the HS-NBS scenario, the stock of each critical metal continues to increase. Since the total REE stock is small, each REE metal details will be shown separately.
The stock of Li will reach 360.27 thousand, 761.81 thousand, and 1,145.20 thousand tons in 2030, 2040, and 2050, respectively. Similarly, the stock of Co will increase by 76.80 thousand, 161.38 thousand, and 241.97 thousand tons, respectively, compared with 2021, reaching 437.07 thousand, 923.19 thousand, and 1,387.17 thousand tons. The stocks of Mn and Cr will be larger than those of Li and Co, reaching 2,822.26 thousand and 3,540.85 thousand tons, respectively in 2050. Ni is the critical metal with the largest stock. Its stock will be greater in 2030 than in 2050, reaching 9,685.26 thousand tons which is 8.46 times the amount of Li in that year. Due to the diversity and small stock of REEs, nine REEs are analyzed separately. The total stock of REE will be 50.53 thousand, 98.70 thousand, and 143.30 thousand tons in 2030, 2040, and 2050 respectively. Among them, Nd will account for the largest proportion, at 84.77, 84.10, and 83.78% of total REE in 2030, 2040, and 2050, respectively. Next are Dy and Tb, with stocks of 3.01 thousand and 4.00 thousand tons, respectively, in 2050. Eu and Gd account for very little REE, with a stock of only 34.15 and 25.44 tons in 2050.
3.3 Estimation of recycling critical metals and their economic value
According to the above analysis, EVs will be widely used in the future, and the supply pressure on critical metals will be huge. On the other hand, EVs will become one of the largest consumers of critical metals, and recycling can be an important source of those critical metals. Therefore, we analyzed the recycling potential of critical metals from EVs. This paper uses the prediction data of the theoretical scrap quantity of electric passenger vehicles in previous research (Li et al., 2021), assuming the scrap electric passenger vehicles are fully recycled. The recycling potential of scrap electric passenger vehicles is shown in Table 7.
According to the current research, the potential recycling rate of Li, Co, Ni, Mn, and Cr is more than 90%, while the recycling rate of REE is low due to the large-scale mining and the need for mature technical support during recycling (Li X. Y. et al., 2019). The lowest recycling rate among REEs is for La, at 17%. Figure 7 shows the recycling quantity of each metal under the HR scenario from 2022 to 2030.
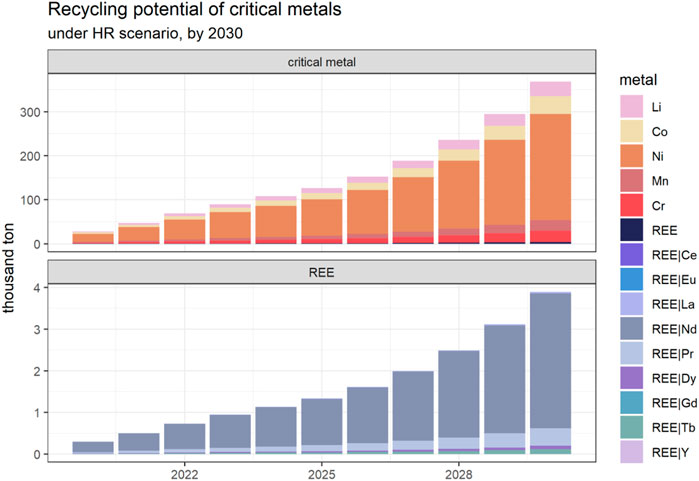
FIGURE 7. Recycling potential of critical metals under HR scenario from 2020 to 2030 in China (thousand tons): total metal recycling and the details in REE. The recycling potential of critical metals in EOL EVa is increasing year by year. HR scenario results based on Li et al. (2021).
Under the HR scenario, the recycling potential of Li, Co, Mn, and Cr in 2030 is 48.03 thousand, 57.51 thousand, 34.45 thousand, and 35.59 thousand tons, respectively, and the recycling potential of Ni is the highest at 361.77 thousand tons. The overall recycling potential of REEs is not high, only 4.56 thousand tons. Looking at the nine REEs separately, Nd has the highest recycling potential, reaching 2,558.65 tons in 2030, while for GD it is only 0.07 tons.
The results of Section 3.2 show that under the scenario proposed in this study, the policy banning sales of FVs has a greater impact on the supply and demand of critical metals in the future than the ownership of passenger vehicles. In the future, EVs will become one of the largest consumers of critical metals, so they also represent the greatest potential for critical metal recycling in the future. According to the above description, this paper supposes that when an EV owner chooses not to replace the battery and directly scraps the vehicle after 8 years of use, the service life of the EV is consistent with that of the power battery; then the stock of critical metals of EVs in 2030 can be regarded as accumulated from 2022, and the scrap volume corresponds to 2022–2030.
Figure 8 shows the uses the sum of the theoretical amount of critical metals recycled from EOL EVs from 2022 to 2030 to compare with the critical metal stock of EVs in 2030.
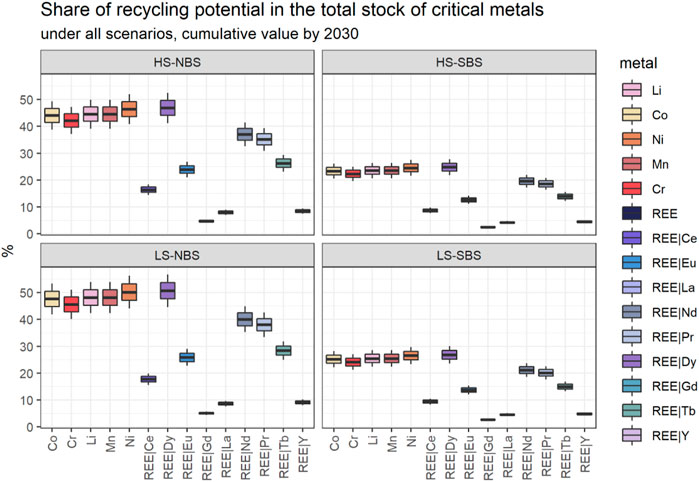
FIGURE 8. Share of recycling potential in the total stock of critical metals in China. Cumulative recycling of critical metals is the sum of theoretical recycling of critical metals of all EOL EVs from 2022 to 2030, and stock is critical metals of EVs in 2030.
It can be observed that under NBS, the recycling potential of Li, Co, Ni, Mn, and Cr in 2030 can cover 49.89, 49.37, 52.00, 49.89, and 47.27% of the stock, respectively. The recycling potential of most REE metals is low; the proportion of Dy covering its stock is the highest, at 41.24%. Gd and La can only cover 4.12 and 7.01% of their stock, respectively. Under the SBS scenario, the recycling potential of all critical metals decreases significantly, with Li, Co, Ni, Mn, and Cr decreasing to 26.38, 26.11, 27.49, 26.38, and 25.00%, respectively.
Next, we take CO, Ni, Li, and Mn as the object to analyze the recycling value of major critical metals, shown in Table 8.
For critical metals with high prices, the recovery rate has a great impact on the recycling income. For example, in 2030, under the HR scenario, the recycling income from the recycling of Li is 31.46 billion yuan, 10.73 billion yuan more than that of the LR scenario, showing a great economic value. Therefore, recycling critical metals with higher prices can relieve the economic pressure due to the resource imports.
To sum up, this study identifies the critical metals in the electrification transformation of the automobile industry, analyzes the stock changes of critical metals in the automobile industry from 2022 to 2050 under different passenger vehicle ownership saturation levels (LS and HS) and different energy-saving and emission reduction policies (NBS and SBS), and discusses the economic value and maximum recycling potential of critical metals in EV. The results of this study show that from 2022 to 2050, with the increase in income and population, China’s car ownership will increase rapidly, and the number of passenger vehicles in China will reach 547.50–623.77 million in 2050. According to China’s current energy conservation and emission reduction policies, China’s fuel vehicle (FV) ownership will reach a peak in 2042, with 488.17 million. If strict energy conservation and emission reduction policies are adopted, the ownership of FVs will be advanced to 2035, with the ownership of 402.65 million. Under any scenario, FV ownership will show a decreasing trend in the future. The policy banning FV sales will not only rapidly increase EV ownership but also accelerate the FV peaking. The rapid growth of EVs has accelerated the demand for critical metals. Therefore, long-term predictions of critical metals stock can effectively reveal the supply risk of critical metals. In this paper, we have identified 14 kinds of critical metals in the electrification transformation of China’s automotive industry and estimated the stocks of critical metals under four scenarios from 2022 to 2050. The stock of all critical metals will increase rapidly in the future. Recycling can alleviate the pressure from meeting the supply and demand of critical metals. The stock of Ni will be the highest, which will be 9,685.26 thousand tons in 2050 under the HS-NBS scenario. Recycling can alleviate the pressure from meeting the demand of critical metals with the supply. We have discussed the recycling potential and economic value of critical metals in EVs. For critical metals with a high recycling rate, such as Li, Mn, and Ni, the resource reuse chain can be improved by establishing recycling channels and formulating relevant policies. For metals with a low recycling rate, such as REEs, the demand pressure can be relieved by developing alternative and recycling technologies. The results of this study show the policy banning sales of FVs will expand the gap between theoretical recycling and stock, thus increasing the pressure on the demand for critical metals. Therefore, considering the scarcity of some critical metals, it is necessary to implement a flexible policy for the process of automobile electrification. In order to alleviate the pressure of supply and demand of critical metals, countries have planned the layout in advance according to their own metal scarcity, developing alternative metals and improving recycling technology and recycling channels. The results of this study can provide data basis for material flow analysis in China’s automotive industry. It can also be extended to the analysis of material stock and flow in different geographical regions and other industries. The estimation results based on this model can provide a scientific basis for promoting the recycling of material resources.
4 Discussion
4.1 The strategic risk assessment of critical metals
Global value chains are becoming more integrated and raising questions about trade, technological changes, economic growth, and environmental issues (Umer et al., 2022). The environmental sustainability contests facing the world’s most pressing problems, including biodiversity loss, climate change, and increasing natural resources demand (Irfan et al., 2022a; Rehman et al., 2021). Natural resources play a critical part in animating economic expansion and maintaining technical standards (Tang et al., 2022; Irfan et al., 2022b; Islam et al., 2021). Countries with abundant natural resources also have higher wealth sources (Mingting et al., 2022), which will bring more economic advantages (Muhammad and Khan, 2021). Although the environmental impact of globalization is usually negative (Zhang et al., 2022), free markets can also expand access to new technology and increase feedstock supply (Irfan M. et al., 2022). Therefore, the strategic risk assessment of critical metals in the process of automobile electrification should be analyzed from two perspectives. First, from a global perspective, materials with a relatively low recovery rate and great difficulty in recovery for electrical transformation-oriented estimation of critical metals are more important because their recovery cannot alleviate the pressure of future demand. Therefore, material mining and storage should be strengthened. For materials with a high recovery rate and little recovery difficulty, effective recovery channels should be established to realize the multiplier effect of material reuse through resource recycling. In addition, the recovery potential can be improved as the recovery and alternative technologies mature. At present, pyrometallurgical/hydrometallurgical recovery technologies are relatively mature. In the future, high-efficiency composite technology could be used to improve the recovery rate and the efficiency of comprehensive resource utilization. Second, from the national perspective, due to differences in the geographical distribution of critical metals and the development levels of different countries, the strategic risks of critical metals are also different. For example, in 2020, China’s Li resource reserves ranked fourth in the world. With the development of EVs, the consumption demand for Li resources is also growing rapidly. China has become the world’s largest consumer of Li mineral resources. In 2020, the total consumption of metal Li is 52,000 t in China, accounting for 61.4% of the total global consumption. However, China’s current technological constraints lead to the slow process of Li resource mining and industrialization, and 70% of Li resource still depends on imports (Qu et al., 2021). Import dependence is an important factor in assessing supply risk. It helps to assess areas where appropriate intervention is needed to avoid a shift to a predominantly import-dependent scenario.
4.2 Resource governance implication
The accelerated transformation of automobile electrification has led to a rapid increase in the demand for critical metals. Affected by multiple factors, such as industrial cycles, the coronavirus, and international tension, there are potential risks in the supply of critical metals. It is of great significance for the sustainable development of the automobile industry to accurately assess the changes in the stocks of critical metals in the medium and long term and to determine the risks in the supply-demand relationship of critical metals that may be caused by the development of EVs. Based on the importance of recycling to the supply of critical metals, a more perfect industry recycling chain needs to be established. For critical metals with low recycling potential, such as REEs, there is a large gap in cumulative recovery and stock. Although China is currently rich in REEs, very important metals in the electrification of the global automobile industry, it also needs to strengthen its metal reserves and layout in advance to alleviate the demand pressure by developing alternative metals and improving recycling technology. Therefore, different countries and regions should formulate more flexible energy conservation and emission reduction policies according to the scarcity of critical metals to effectively alleviate the pressure created by their supply. For critical metals with relatively high recycling potential, such as Li, Ni, and Co, more effective recycling channels should be established to improve the comprehensive utilization efficiency of renewable resources.
4.3 Uncertainties
The first type of uncertainty lies in the parameter recycling rate. The recycling rate in this paper refers to the potential recycling rate, which is the possible recycling degree in the future. Different recycling rate choices will affect the results. Taking the metal Dy as an example, with the development of technology, the potential recycling rate of Dy is high, which can reach 100% (Ciacci, et al., 2015; Zuo and Wang, 2020). However, according to the current recycling technology, the recycling rate of Dy is only 15% (Junne et al., 2020).
We compared the recycling of Dy with the mentioned two recycling rates under LR and HR recovery scenarios. Under the HR scenario, the recycling of 100% Dy will reach 122.83 tons in 2030, while the recycling of 15% Dy will only be 18.42 tons. From 2022 to 2030, the cumulative recycling of 100% Dy accounted for 56.81% of the Dy stock in 2030 under the LS-NBS scenario, while the cumulative recycling of 15% Dy accounted for only 8.52% of the stock. The uncertainty brought by the recycling rate of one specific metal might be huge, also indicating that Dy has great recycling potential with the improvement of recycling technology.
The unit prices of critical metals are also with large fluctuations, which brought uncertainties to our results. If focusing on the primary resource market, the unit prices of Li, Co and Ni are relatively high, especially Li, which reached 2.97 million yuan/ton in June 2022. Most uncertainties come from these four types of metals. Figure 9 shows the uncertainties from the recycling potential parameters and from unit price parameters.
Taking Li as an example, if we adopt the unit price of Li in 2021 as the unit price parameter, the total potential economic value of Li in 2030 may range from 29.78billion to 151.21billion yuan. If focusing on the secondary resource marking, still, taking the example of Li, based on the unit price of the recycled Ni in the 5 months from March 2022 to July 2022, the total potential economic value of Li in 2030 may range from 36.43 billion to 74.13 billion.
5 Conclusion
In this study, we set the saturation level of passenger vehicle ownership per thousand people (LS and HS, low and high saturation of passenger vehicles), forecasted passenger vehicle ownership in China from 2022 to 2050 under different saturation levels, and analyzed the impact of policies banning the sale of FVs (NBS and SBS, without and with the ban on the sales of FVs) on EV ownership in the future.
Under the two saturation scenarios, LS and HS, the number of passenger vehicles in China will reach 547.50 million and 623.77 million, respectively, in 2050. During the period from 2022 to 2050, FV ownership will reach a peak regardless of whether sales of FVs are banned or not, but the FV peak will occur significantly earlier under the SBS scenario. A strict energy conservation and emission reduction policy will advance the peak of FV ownership by 7 years, and EV ownership will also increase from 148.27 million to 293.90 million in 2050.
On this basis, the trend of the stock of critical metals from 2022 to 2050 under four scenarios was analyzed. Under the four scenarios, the stock of critical metals shows an upward trend year by year, among which that of HS-SBS is the largest. Under the HS-NBS scenario, the stock of critical metals such as Li, Co, and Ni will reach 1,145.20 thousand, 1,387.17 thousand, and 9,685.26 thousand tons, respectively, in 2050. The implementation of policies strictly banning the sale of FVs will lead to a rapid rise in the stock of critical metals. Under the HS-SBS scenario, the stock of critical metals such as Li, Co, and Ni will reach 2,267.18 thousand, 2,744.71 thousand, and 17,364.44 thousand tons, respectively. The results show that the massive popularization of EVs in the future will bring huge demand pressure on critical metals.
On the other hand, EVs, the largest consumers of critical metals, also represent the maximum potential for recycling critical metals in the future. This study thus further discusses the economic value of and maximum recovery potential for critical metal recycled from EOL EVs. It is found that recycling critical metals are an effective means to avoid the risk of a reduced critical metal supply. At the same time, the economic benefit brought by the metals with higher recycling prices would also be significant.
This paper can be further improved in the following two aspects. First, due to the availability of data, this study only analyzes the major critical metals with greater supply risk in the future automotive electrification transformation process. For example, as one of the critical materials, platinum group metals have not been included in the analysis object in this paper, as China’s vigorous promotion of EVs will reduce the use of platinum group metals in the future. As the next step, we will investigate the supply risk and recovery potential of platinum group metals considering such adjustments in policies and regulations. Second, this study is based on the current material composition, which could be changed with the technical development. Traditional fuel vehicles will be gradually withdrawn from the market between 2030 and 2040 in many developed automobile industrial countries. The supply of critical metals will become a bottleneck problem restricting the electrification transformation of the global automobile industry in the future. We also consider expanding the research from China to more countries in the future, especially focusing on the advanced automotive technical standards and policies of developed countries, to verify the applicability of this study.
Data availability statement
The raw data supporting the conclusions of this article will be made available by the authors, without undue reservation.
Author contributions
LY and JY conceived of the study. LYh, LY, HS, and SL conducted the empirical analysis. LY and JY produced figures. LY, LYh, HS, SL, and JY wrote the manuscript, which is edited and approved by all the authors.
Funding
This research received funding from the National Natural Science Foundation of China (No. 71804195), Grants-in-Aid for Scientific Research of Japan (JSPS KAKENHI, No. 22K18070), and the China Ministry of Education, Humanities and Social Sciences Research Youth Fund Project (Research on Driving Mechanism and Path Optimization of High-Quality Development of Renewable Resources Industry from the Perspective of Value Co-creation).
Conflict of interest
The authors declare that the research was conducted in the absence of any commercial or financial relationships that could be construed as a potential conflict of interest.
Publisher’s note
All claims expressed in this article are solely those of the authors and do not necessarily represent those of their affiliated organizations, or those of the publisher, the editors and the reviewers. Any product that may be evaluated in this article, or claim that may be made by its manufacturer, is not guaranteed or endorsed by the publisher.
References
Ahmadi, L., Yip, A., Fowler, M., Young, S. B., and Fraser, R. A. (2014). Environmental Feasibility of Re-use of Electric Vehicle Batteries. Sustain. Energy Technol. Assessments 6, 64–74. doi:10.1016/j.seta.2014.01.006
Ali, S. H., Giurco, D., Arndt, N., Nickless, E., Brown, G., Demetriades, A., et al. (2017). Erratum: Corrigendum: Mineral Supply for Sustainable Development Requires Resource Governance. Nature 547, 246. doi:10.1038/nature22982
Australian Department of Industry, Science, Energy and Resources (2019). Australian Trade and Investment Commission.Australia's Critical Minerals Strategy, Commonwealth of Australia.
Chen, X., and Zhou, T. (2014). Hydrometallurgical process for the recovery of metal values from spent lithium-ion batteries in citric acid media. Waste Manag. Res. 32 (11), 1083–1093. doi:10.1177/0734242X14557380
Cheng, J. H., Yi, J. H., and andWu, Q. S. (2021). Carbon Neutrality, Development of Strategic Emerging Industries and Management of Key Mineral Resources. China Popul. Resour. Environ. 31, 135–142.
China Automobile Industry Association (2021). Available at http://www.caam.org.cn/.
China Automobile Industry Yearbook (2021). China Automobile Industry Yearbook. Available at https://www.hnsaf.com/displaynews.html?id=4033378932966208.
China Statistical Yearbook (2021). China Statistical Yearbook. Available at http://www.stats.gov.cn/tjsj/ndsj/.
Ciacci, L., Graedel, T. E., Reck, B. K., and Nassar, N. T. (2015). Lost by Design. Environ. Sci. Technol. 49, 9443–9451. doi:10.1021/es505515z
Council on Energy, Environment and Water (2016). Critical Non-fuel Mineral Resources for India’s Manufacturing Sector: A Vision for 2030.
Dargay, J., Gately, D., and Sommer, M. (2007). Vehicle Ownership and Income Growth, Worldwide: 1960‐2030. Energy J. 28, 143–170. doi:10.5547/issn0195-6574-ej-vol28-no4-7
Deetman, S., Pauliuk, S., Vuuren, D. P. V., Voet, E. V. D., and Tukker, A. (2018). Scenarios for Demand Growth of Metals in Electricity Generation Technologies, Cars, and Electronic Appliances. Environ. Sci. Technol. 52 (8), 4950–4959. doi:10.1021/acs.est.7b05549
Energy and transportation innovation center (2019). A Study on China’s Timetable for Phasing-Out Traditional Ice-Vehicles.
Field, F. R., Wallington, T. J., Everson, M. P., and Kirchain, R. E. (2017). Strategic Materials in the Automobile: A Comprehensive Assessment of Strategic and Minor Metals Use in Passenger Cars and Light Trucks. Environ. Sci. Technol. 51 (24), 14436–14444. doi:10.1021/acs.est.6b06063
Fishman, T., and Graedel, T. E. (2019). Impact of the Establishment of US Offshore Wind Power on Neodymium Flows. Nat. Sustain 2, 332–338. doi:10.1038/s41893-019-0252-z
Ge, J. P., and Liu, J. Q. (2020). International Comparison of Critical Mineral Strategies: Historical Evolution and Tool Selection. Resour. Sci. 42, 1464–1476. doi:10.18402/resci.2020.08.03
Grandell, L., Lehtila, A., Kivinen, M., Koljonen, T., Kihlman, S., and Lauri, L. S. (2016). Role of Critical Metals in the Future Markets of Clean Energy Technologies. Renew. Energy 95, 53–62. doi:10.1016/j.renene.2016.03.102
Greim, P., Asfaw, S. A., and Breyer, C. (2020). Assessment of Lithium Criticality in the Global Energy Transition and Addressing Policy Gaps in Transportation. Nat. Commun. 11, 4570. doi:10.1038/s41467-020-18402-y
Habib, K., Hansdottir, S. T., and Habib, H. (2020). Corrigendum to <’Critical Metals for Electromobility: Global Demand Scenarios for Passenger Vehicles, 2015–2050’> <[Resources, Conservation and Recycling 154 (2020) 1–12]>. Resour. Conserv. Recycl. 160, 104932. doi:10.1016/j.resconrec.2020.104932
Huo, H., and Wang, M. (2012). Modeling future vehicle sales and stock in China. Energy Policy 43, 17–29. doi:10.1016/j.enpol.2011.09.063
Irfan, K., Abdulrasheed, Z., Jinjun, Z., Vishal, D., and Sanjeet, S. (2022b). A Study of Trilemma Energy Balance, Clean Energy Transitions, and Economic Expansion in the Midst of Environmental Sustainability: New Insights from Three Trilemma Leadership. Energy 248, 123619. doi:10.1016/j.energy.2022.123619
Irfan, K., Abdulrasheed, Z., Vishal, D., and Sanjeet, S. (2022a). World Energy Trilemma and Transformative Energy Developments as Determinants of Economic Growth amid Environmental Sustainability. Energy Econ. 108, 105884. doi:10.1016/j.eneco.2022.105884
Irfan, M., Elavarasan, R. M., Ahmad, M., Mohsin, M., Dagar, V., and Hao, Y. (2022c). Prioritizing and Overcoming Biomass Energy Barriers: Application of Ahp and G-Topsis Approaches. Technol. Forecast. Soc. Change 177, 121524. doi:10.1016/j.techfore.2022.121524
Islam, M. M., Khan, M. K., Tareque, M., Jehan, N., and Dagar, V. (2021). Impact of Globalization, Foreign Direct Investment, and Energy Consumption on CO2 Emissions in Bangladesh: Does Institutional Quality Matter? Environ. Sci. Pollut. Res. 28, 48851–48871. doi:10.1007/s11356-021-13441-4
Junne, T., Wulff, N., Breyer, C., and Naegler, T. (2020). Critical Materials in Global Low-Carbon Energy Scenarios: the Case for Neodymium, Dysprosium, Lithium, and Cobalt. Energy 211, 118532. doi:10.1016/j.energy.2020.118532
Kromer, M. A., and Heywood, J. B. (2007). Electric Powertrains: Opportunities and Challenges in the U.S. Light-DutyVehicle Fleet. Cambridge, MA, USA: Massachusetts Institute of Technology, 157. Available online http://web.mit.edu/sloan-auto-lab/research/beforeh2/files/kromer_electric_powertrains.pdf.
Lee, C. K., and Shaw, M. S. (1996). “Constrained Diffusion Models for the Prediction of Multi‐Class Motor Vehicle Ownership. Volume 1: Travel Behavior,” in Proceedings of the World Transport Research World Conference on Transport Research, Sydney, Australia, 30 August 1996.
Li, W. Q., Li, J. W., Xie, G. Q., Zhang, X. F., and Liu, H. (2022). Current Situation, Research Content and Resource Strategy Analysis of Key Minerals in China. Earth Sci. Front. 29 (1), 13. doi:10.13745/j.esf.sf.202
Li, X. Y., Ge, J. P., Chen, W. Q., and Wang, P. (2019). Scenarios of Rare Earth Elements Demand Driven by Automotive Electrification in China: 2018–2030. Resour. Conserv. Recycl. 145, 322–331. doi:10.1016/j.resconrec.2019.02.003
Li, Y., Huang, S. Y., Liu, Y. H., and Ju, Y. Y. (2021). Recycling Potential of Plastic Resources from End-Of-Life Passenger Vehicles in China. Int. J. Environ. Res. Public Health 18, 10285. doi:10.3390/ijerph181910285
Li, Y., Miao, L., Chen, Y., and Hu, Y. (2019). Exploration of Sustainable Urban Transportation Development in China through the Forecast of Private Vehicle Ownership. Sustain. Switz. 11 (16), 4259. doi:10.3390/su11164259
Liu, W. Q., Liu, W., Li, X. X., Liu, Y. Y., Ogunmoroti, A. E., Li, M. Y., et al. (2021). Dynamic Material Flow Analysis of Critical Metals for Lithium-Ion Battery System in China from 2000-2018. Resour. Conserv. Recycl. 164, 105122. doi:10.1016/j.resconrec.2020.105122
Mashael, K., Marco, R., and Allan, H. (2021). A dynamic material flow analysis of lithium-ion battery metals for electric vehicles and grid storage in the UK: Assessing the impact of shared mobility and end-of-life strategies. Resour. Conserv. Recycl. 170, 105582. doi:10.1016/j.resconrec.2021.105412
Mingting, X., Muhammad, I., Asif, R., and Vishal, D. (2022). Forest and Mineral Volatility and Economic Performance: Evidence from Frequency Domain Causality Approach for Global Data. Resour. Policy 76, 102685. doi:10.1016/j.resourpol.2022.102685
Ministry of Land and Resources of the People's Republic of China (2016). National Mineral Resources Planning.
Ministry of Public Security of the People's Republic of China (2016). Ministry of Public Security of the People's Republic of China. Available at https://www.mps.gov.cn/.
Muhammad, B., and Khan, M. K. (2021). Foreign Direct Investment Inflow, Economic Growth, Energy Consumption, Globalization, and Carbon Dioxide Emission Around the World. Environ. Sci. Pollut. Res. 28, 55643–55654. doi:10.1007/s11356-021-14857-8
National Development and Reform Commission (2018). Regulations on Investment Management of Automobile Industr. Available at http://www.gov.cn/gongbao/content/2019/content_5377111.htm.
Olivetti, E. A., Ceder, G., Gaustad, G. G., and Fu, X. (2017). Lithium-Ion Battery Supply Chain Considerations: Analysis of Potential Bottlenecks in Critical Metals. Joule 1 (2), 229–243. doi:10.1016/j.joule.2017.08.019
Ortego, A., Iglesias-Mbil, M., Calvo, G., Valero, A., Valero, A., and Villacampa, M. (2020). Assessment of Strategic Raw Materials in the Automobile Sector. Resour. Conserv. Recycl. 121, 104968. doi:10.1016/j.resconrec.2020.104968
Qu, J. Z., Zhang, Y. S., Zhang, Y., and Fan, X. L. (2021). Safety Evaluation of Lithium Resources Supply in China under the New Situation. China Min. Mag. 30, 1–7.
Rehman, A., Ma, H., Ozturk, I., Murshed, M., and Dagar, V. (2021). The Dynamic Impacts of Co2 Emissions from Different Sources on pakistan's Economic Progress: a Roadmap to Sustainable Development. Environ. Dev. Sustain. 23, 17857–17880. doi:10.1007/s10668-021-01418-9
Serrenho, A. C., Norman, J. B., and Allwood, J. M. (2017). The Impact of Reducing Car Weight on Global Emissions: The Future Fleet in Great Britain. Phil. Trans. R. Soc. A 375, 20160364. doi:10.1098/rsta.2016.0364
Shanghai Nonferrous Metals Industry Association (2022). Shanghai Nonferrous Metals Network. https://www.smm.cn/.
Sharma, L., and Pandey, S. (2020). Recovery of Resources from End-Of-Life Passenger Cars in the Informal Sector in India. Sustain. Prod. Consum. 24, 1–11. doi:10.1016/j.spc.2020.06.005
Simon, B., Ziemann, S., and Weil, M. (2015). Potential Metal Requirement of Active Materials in Lithium-Ion Battery Cells of Electric Vehicles, and its Impact on Reserves: Focus on Europe. Resour. Conserv. Recycl. 104, 300–310. doi:10.1016/j.resconrec.2015.07.011
Tang, C., Irfan, M., Razzaq, A., and Dagar, V. (2022). Natural Resources and Financial Development: Role of Business Regulations in Testing the Resource-Curse Hypothesis in Asean Countries. Resour. Policy 76, 102612. doi:10.1016/j.resourpol.2022.102612
Umer, S., Mara, M., Vishal, D., Sudeshna, G., and Buhari, D. (2022). Exploring the Role of Export Product Quality and Economic Complexity for Economic Progress of Developed Economies: Does Institutional Quality Matter? Struct. Chang. Econ. Dyn. 62, 40–51. doi:10.1016/j.strueco.2022.04.003
US Department of Commerce (2019). A Federal Strategy to Ensure Secure and Reliable Supplies of Critical Minerals.
Valero, A., Calvo, G., and Ortego, A. (2018). Material Bottlenecks in the Future Development of Green Technologies. Renew. Sustain. Energy Rev. 93 (10), 178–200. doi:10.1016/j.rser.2018.05.041
Wang, C., Sun, J., Zuo, L. S., and Song, H. L. (2018). Risk Assessment of Global Supply of Key Raw Materials for New Energy Vehicles. Forum Sci. Technol. China 4, 11.
Wei, W., Ge, Z., Geng, Y., Jiang, M., Chen, Z., and Wu, W. (2022). Toward Carbon Neutrality: Uncovering Constraints on Critical Minerals in the Chinese Power System. Fundam. Res. 2 (3), 367–374. doi:10.1016/j.fmre.2022.02.006
Weiner, J. (1990). Asymmetric Competition in Plant Populations. Trends Ecol. Evol. 5, 360–364. doi:10.1016/0169‐5347(90)90095‐U
Xu, G., Yano, J ., and Sakai, S. I . (2016). Scenario Analysis for Recovery of Rare Earth Elements from End-Of-Life Vehicles. J. Mat. Cycles Waste Manag. 18 (3), 469–482. doi:10.1007/s10163-016-0487-y
Yano, J., Muroi, T., and Sakai, S. (2016). Rare Earth Element Recovery Potentials from End-Of-Life Hybrid Electric Vehicle Components in 2010–2030. J. Mat. Cycles Waste Manag. 18, 655–664. doi:10.1007/s10163-015-0360-4
Zhang, C., Khan, I., Dagar, V., Saeed, A., and Zafar, M. W. (2022). Environmental Impact of Information and Communication Technology: Unveiling the Role of Education in Developing Countries. Technol. Forecast. Soc. Change 178, 121570. doi:10.1016/j.techfore.2022.121570
Zhou, Y., Li, J., Wang, G., Chen, S., Xing, W., and Li, T. (2019). Assessing the Short-To Medium-Term Supply Risks of Clean Energy Minerals for china. J. Clean. Prod. 215 (4), 217–225. doi:10.1016/j.jclepro.2019.01.064
Keywords: critical metals, passenger vehicle, electric vehicle (EV), recycling potential, scenario analysis
Citation: Li Y, Liu Y, Huang S, Sun L and Ju Y (2022) Estimation of critical metal stock and recycling potential in China’s automobile industry. Front. Environ. Sci. 10:937541. doi: 10.3389/fenvs.2022.937541
Received: 06 May 2022; Accepted: 27 July 2022;
Published: 29 August 2022.
Edited by:
Wendong Wei, Shanghai Jiao Tong University, ChinaReviewed by:
Abhilash, National Metallurgical Laboratory (CSIR), IndiaShijiang Xiao, Shanghai Jiao Tong University, China
Copyright © 2022 Li, Liu, Huang, Sun and Ju. This is an open-access article distributed under the terms of the Creative Commons Attribution License (CC BY). The use, distribution or reproduction in other forums is permitted, provided the original author(s) and the copyright owner(s) are credited and that the original publication in this journal is cited, in accordance with accepted academic practice. No use, distribution or reproduction is permitted which does not comply with these terms.
*Correspondence: Yiyi Ju, anV5aXlpQGFvbmkud2FzZWRhLmpw