- 1Central South University of Forestry and Technology, Changsha, China
- 2Hunan Lutou Forest Ecosystem National Positioning Observation and Research Station, Yueyang, China
- 3National Engineering Laboratory of Forestry Ecological Application Technology in Southern China, Changsha, China
Canopy conductance was an important index to measure the process of water exchange between canopy and atmosphere interface of forest ecosystem, as well as to judge the status of water use or the degree of drought stress. Therefore, the accurate estimation of forest canopy conductance was of great significance for the research of water-use efficiency. In the past, canopy conductance was measured on vegetation types in a single-point region, but there were few studies on systematic comparison in different climate zones. Based on the data sets of EC flux and conventional meteorological elements from the eddy covariance (EC) flux observation station during 2003–2010 in three typical climate zones (temperate continental monsoon climate zone, south subtropical monsoon climate zone, and mid-subtropical monsoon climate zone), Penman-Monteith model was used to calculate forest canopy conductance in different climate regions and analyze the dynamic changes of canopy conductance in different time scales. At the same time, combined with environmental factors including temperature, net radiation, soil water content, and vapor pressure deficit to explore their driving ability on the canopy conductivity of forest ecosystem in different climate regions, we finally explored the mechanism driving the canopy conductivity of forest ecosystem under different climates. The results showed that: 1) the driving ability of environmental factors in different climate regions to the canopy conductance was different, and the contribution rate of soil water content to the canopy conductance in subtropical monsoon climate zone was the largest. It was 36.01%, and the contribution rate of vapor pressure deficit to the canopy conductance in mid-subtropical monsoon climate region was the largest. It was 29.4% and the contribution rate of temperature to the canopy conductance in temperate monsoon climate region was the largest; it was 28.14%. 2). Temperature was an important factor limiting and driving canopy conductance, and there was a synergistic effect between water and temperature, which jointly drove the change in canopy conductance. 3) Environmental factors in different climate regions had threshold for the synergistic driving effect of canopy conductance. When the factors were within the appropriate threshold, the factors had a strong promoting effect on canopy conductance.
1 Introduction
1.1 Overview of Canopy Conductance
Canopy was the core place of water vapor exchange between plant and atmosphere in soil-plant-atmosphere continuum (SPAC) (Evaristo et al., 2015), and the canopy–atmospheric water vapor output process could be divided into canopy water vapor export process and near-leaf turbulent water vapor output process. In the process of canopy water vapor output, the resistance of water vapor inside the canopy when it diffused outward through the stomatal layer and boundary layer is called canopy resistance, which was usually quantified by canopy conductivity (Wang et al., 2021). Canopy conductance as an indicator of stomatal regulation at ecosystem scale had been widely concerned (De Boer et al., 2011; Deng and Ding, 2015; Bai et al., 2017), and dynamic changes reflected stomatal behavior and water regulation mode of plants, which was the overall index of material and energy exchange in the plant canopy (Hu et al., 2019), and had been widely used in the study of plant stomatal behavior regulation (Chen et al., 2021).
1.2 Effects of Environmental Factors on Canopy Conductance at Different Scales
Canopy conductance was easily affected by different environmental conditions which was an important biological factor indicating the response of ecosystem to environmental factors (Igarashi et al., 2015). Changes in canopy conductance and control mechanisms had been applied to different ecosystems, such as poplar plantations (Song et al., 2021), tropical rainforest (Yoshifuji et al., 2020), farmland shelterbelt (Fu et al., 2016), and temperate deciduous forests (Wehr et al., 2017) conduct research. In addition to tree species, the reason for the difference in canopy conductance was that the regional environment also played a particularly important role in driving the change in canopy conductance (Saito et al., 2017). It was found that canopy conductance had different responses to the changes of vapor pressure deficit (VPD), net radiation (Rn), air temperature (T), and soil water content (SWC) at different scales (Komatsu et al., 2012). In addition to tree species, the cause may be related to regional environment (Saito et al., 2017). On long time scales, soil moisture was an important factor affecting canopy conductance. Soil water content was the controller of canopy conductance on both seasonal and interannual scales (Harris et al., 2004). When soil moisture was higher, stomatal sensitivity to various environmental factors was relatively small, and canopy stomatal conductance was high (Kumagai et al., 2008). When soil water was subjected to drought stress, with the increase of stress degree, stomatal sensitivity to vapor pressure deficit and solar radiation increases, and canopy conductance was inhibited (Mo et al., 2014). Therefore, by exploring the change in canopy conductance, we can judge the soil water status or the degree of drought stress, which played an important role in the forest ecosystem to better adapt to the changing environmental conditions under global climate change (Richardson et al., 2013). On the daily scale, vapor pressure deficit was the main dominant factor in environmental variables (Pataki et al., 1998.) As an important factor affecting canopy conductance, vapor pressure deficit can significantly affect the sensitivity of stomatal conductance to transpiration, photosynthesis, and other physiological processes, and had an important impact on evapotranspiration and water-use efficiency of forest ecosystems (Wang et al., 2016; Rodriguez-Dominguez et al., 2019). At high vapor pressure deficit, stomatal conductance decreased and transpiration increased in most species (Grossiord et al., 2020). Radiation was another important factor that regulates the canopy conductance. Increasing solar radiation could induce pore opening to some extent, resulting in increased tree transpiration, while under high solar radiation, pores were partially closed, causing reduced stomatal conductance (Martin et al., 1997; She et al., 2013). In addition, studies have found that high temperatures can lead to lower stomatal conductivity in the tree canopy (Gillner et al., 2017). It was found that temperature more often regulates canopy conductance on seasonal and interannual scales (Yoshida et al., 2010). Therefore, it is crucial to explore the response of canopy conductance to environmental factors at different time scales to better understand the adaptation of ecosystems to climate change. Although there were many studies on canopy conductance of different vegetation types, there were few studies on the characteristics of canopy conductance in the macro perspective, such as the climate areas where different vegetation types were located, and there were few studies on the relative contribution rate of various environmental factors driving the change in canopy stomatal conductance and the impact on the change process of canopy stomatal conductance in different thresholds.
1.3 Study Area Selection
Exploring the changes of canopy stomatal conductivity and its response to environmental variables under different environmental conditions not only could deepen the understanding of the environmental control mechanism of plant stomatal behavior but also be of great significance to judge the water-use relationship of plants and select the stomatal regulation mode of tree species. To achieve this goal, long-term observations were indispensable. The National Changbai Mountain temperate mixed forest (CBS), Qianyanzhou subtropical plantation conifer (QYZ), and Dinghushan subtropical mixed forest (DHS) Critical Zone Observatory were distributed in the main forest areas and climate zones in China; the three sites located in different climate zones were all located in eastern China and belong to the monsoon climate zone, and the hydrothermal conditions had obvious latitudinal gradient characteristics from north to south. Therefore, the selection of these three climate stations could form a fully representative long-term forest flux observation network in the monsoon climate region of eastern China.
1.4 Aims and Objectives
This study was based on the carbon water flux and conventional meteorological element observation dataset of flux observation station from 2003 to 2010 (Wu et al., 2021; Li et al., 2021; Dai et al., 2021). Combined with environmental factors such as temperature (T), net radiation (Rn), soil water content (SWC), and vapor pressure deficit (VPD), we explored the variation characteristics of canopy conductance in different climate regions on different time scales and correlation with various environmental factors, and quantitatively analyzed the contribution of environmental factors in different climate regions to canopy conductance variation and forest water vapor exchange.
The aim of this study was to explore the main controlling factors of canopy conductance change in forest ecosystem in different climate areas, and reveal the environmental driving mechanism of canopy conductance change of forest ecosystem in different climate areas.
The results of our study will hopefully provide a theoretical basis for the study of water and heat exchange in forest ecosystems in different monsoon climate regions in eastern part of China.
2 Materials and Methods
2.1 Study Area
The distribution, location, and climate area of the research sites are shown in Figure 1. The data of China’s climate zoning came from the resource and environmental science and data center of the Chinese Academy of Sciences. Dinghushan National Nature Reserve (23°09′21″–23°11′30″N, 112°30 ′39″–112°33′41″E) was located in Dinghu District, Zhaoqing City, Guangdong Province, with an altitude of 100–700 m. The region has south subtropical monsoon climate (Li et al., 2021). The vegetation was mainly subtropical zonal vegetation, which was abundant on the Tropic of Cancer, mainly composed of Castanopis chinensis, Schim Asuperba, Cryptocarya Chinensis, and other communities (Li et al., 2021). The eddy covariance (EC) flux observation system of Qianyanzhou (115°04′E, 26°43′N) is located at Qianyanzhou Station, Guanxi Town, Taihe County, Jiangxi Province, with an average altitude of 110.8 m. The station area has subtropical monsoon climate (Xu et al., 2021). The station area belongs to the subtropical evergreen broad-leaved forest area. The existing forests were mainly artificial forests built around 1985, and the main tree species were Pinus Massoniana, P. elliottii, and Cunninghamia Lanceolata (Wen et al., 2005). Changbai Mountain Nature Reserve is located in the southeast of Jilin Province (41°41′49″–42°25′18″N, 127°42′5″–128°16′ 48″E). It belongs to temperate continental monsoon climate, with distinct seasonal alternations (Wang et al., 2016). The temperate broad-leaved Korean pine forest in Changbai Mountain is the main part of mountain forest system in northeast China (Wang et al., 2016).
2.2 Data Observation and Processing
2.2.1 Observation of Eddy Covariance Flux and Micrometeorological Elements
Each platform was equipped with micro meteorological observation tower and open eddy covariance (EC) flux observation system (OPEC). A set of microclimate gradient observation system was installed on the observation tower to simultaneously observe conventional meteorological elements such as wind speed, temperature, humidity, precipitation, light, and effective radiation, as well as CO2 and water and heat flux at the canopy–atmosphere interface. The main observation devices were vorticity correlation system by ultrasonic anemometer and OPEC system by ultrasonic anemometer (CAST3, Campbell Scientific Inc., Logan, Utah, United States), and open-loop CO2/H2O infrared gas analyzer (Li7500, Licor Inc., Lincoln, Nebraska) was used to measure three-dimensional wind speed and CO2/H2O concentration pulse (Alistair et al., 2017), sensible/latent heat flux (Li-7500A, Li-COR), air temperature, relative humidity (HMP45C, VAISALA, Finlang), and wind speed WS (A100R, Campbell, United States), effective radiation (Li-190SB, Licor), soil water content (CS615-L, Campbell Scientific Inc., Logan, Utah, United States), and air temperature/humidity (HMP45C, CR23X, Campbell) (Wu et al., 2021; Li et al., 2021; Dai et al., 2021).
2.2.2 The Data Collection
The flux observation data used in this paper were from the continuous flux observation data of three EC flux observation stations (DHS, QYZ, CBS) in recent 8 years (2003–2010) of China terrestrial ecosystem flux observation network (ChinaFLUX). The flux station adopts a unified open circuit vorticity correlation system and observation technology to observe the fluxes of CO2, water and energy between vegetation and atmosphere for a long time. In order to ensure better comparability between ecosystem carbon exchange data obtained by different observation stations, instruments, data collectors, and data storage formats of vorticity-related systems at all flux observation stations of ChinaFLUX adopt unified standards (Yu et al., 2006). The dataset was downloaded from the National Science and Technology Basic Conditions Platform - National Ecological Science Data Center (http://www.nesdc.org.cn), and for a small number of missing records, linear interpolation or the correlation between meteorological elements and the internal relationship of physical quantities were used for interpolation. Table 1 provides the basic information of each research and observation station.
2.3 Calculation of Vapor Pressure Deficit
Vapor pressure deficit (VPD/kPa) refers to the difference between the saturated vapor pressure and the actual vapor pressure at a certain air temperature (Yu et al., 2006). It is one of the important driving factors of evapotranspiration and one of the most important climatic factors for simulating plant water and carbon fluxes in ecological models. VPD could be estimated from atmospheric relative humidity (RH/%) temperature (T/◦C) (Endler et al., 1999).
Calculation formula:
2.4 Calculation of the Aerodynamic Resistance
Aerodynamic resistance was caused by the turbulent motion in the turbulent layer above the canopy, which was regarded as the irregular fluctuation motion of an independent vortex. The aerodynamic conductivity could be calculated according to Monin–Obukov formula (Granier et al., 2000).
Z0 is the surface roughness (about 0.1 h, h was forest average tree height), d is the zero plane displacement (about 0.75 h); K is the von Karman’s constant (0.4) and u (m/s) is the wind speed at height Z (m).
2.5 Calculation of Canopy Conductance
In the flux tower, vorticity correlation technology was used to measure latent and sensible heat fluxes of the two stands, and instruments were installed for meteorological observation, including air temperature (Ta/◦C), relative humidity (RH/%). H is the sensible heat flux (W/M2), and
The canopy conductivity gs (mm/s) was calculated using Penman–Monteith equations (Xu et al., 2018):
Δ is the function slope of saturated vapor pressure and temperature (Pa/K); Cp is the specific heat of dry air at constant pressure (J/(kgK)) and the value in this study was 1,005;
2.6 The Data Analysis
Based on the calculated canopy conductance, this study analyzes the changes in canopy conductance in different climate regions on different time scales, combined with environmental factors such as temperature, net radiation, soil water content, and vapor pressure deficit. SPSS Statistics 23 was used to analyze the correlation between canopy conductance change and environmental factors in different climate regions. OriginPro2018 software (OriginLab, Northampton, MA, United States) was used to fit the generalized linear regression, logarithm, and logical model of canopy conductance to each factor. Finally, the well-fitting function with higher value and lower p-value was selected, and the contribution rate of each factor to driving canopy conductance was calculated. In this study, we used the factor contribution rate to clarify the driving ability of environmental factors on canopy conductance, and quantitatively analyzed the contribution of environmental factors in different climate regions to the change of forest ecosystem canopy conductance and forest carbon and water exchange.
3 Results
3.1 Characteristics of Meteorological Factors
The interannual variation characteristics of environmental factors in different climate zones are shown in Figure 2. As can be seen from the figure, canopy temperature has a parabolic seasonal characteristics with an obvious peak value. The temperature rises gradually from growing season every year, reaches the maximum in June to July, and then in non-growing season begins to decline. The temperature of Dinghushan was higher than Qianyanzhou and Changbai Mountain. The temperature of Changbai Mountain was lower. The highest temperature appeared in July 2008, and the temperature was 20.1°C, and the temperature fluctuates greatly. Net radiation had a similar trend with temperature, and net radiation in temperate climate zone was significantly lower than that in Subtropical climate zone, which accorded with the law of latitudinal gradient hydrothermal change. In the non-growing season, the change and the value were small, and reached the maximum value in summer, but Dinghushan had a lag response to this, and the variation of soil bulk water content also had obvious seasonality, which varies with the change of precipitation with a small range. However, there were also seasonal droughts with mismatched rainfall and temperature, resulting in low soil water content.
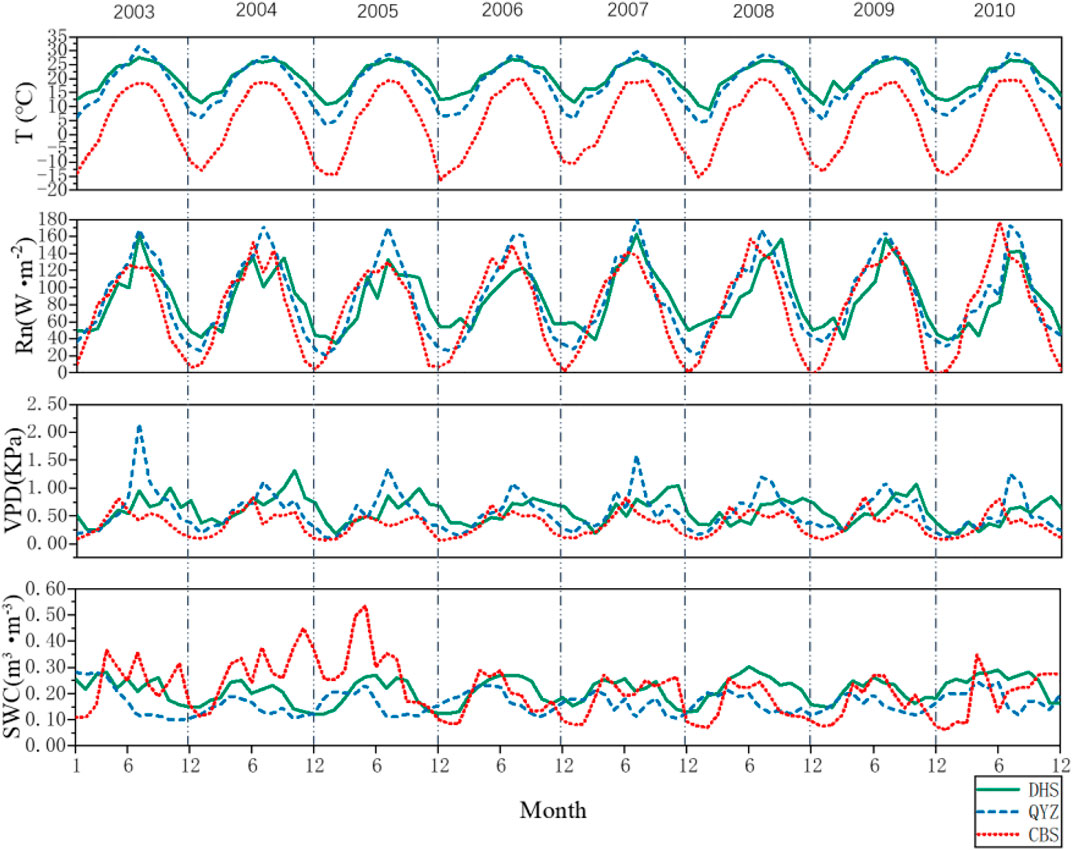
FIGURE 2. Variation characteristics of environmental factors. (DHS: Dinghushan; QYZ: Qianyanzhou; CBS: Changbai Mountain)
3.2 Characteristics of Canopy Conductance in Different Climate Zones
3.2.1 Variation Characteristics of Canopy Conductance
There were significant differences in the interannual variation characteristics of canopy conductance in different climate types, which reflected the response of canopy conductance to environmental factors in different climate regions. As shown in Figure 3, on the whole, the seasonal variation of subtropical canopy conductance was obvious, reaching the peak in spring and the minimum in winter. In 2003 and 2007, the seasonal variation pattern of canopy conductance in Qianyanzhou did not follow the general law, and decreased in July. The vegetation phenology and climate conditions with four distinct seasons of Changbai Mountain area made the canopy conductance of temperate coniferous and broad-leaved mixed forest ecosystem in Changbai Mountain and showed obvious seasonal changes. The peak value of canopy conductance in Changbai Mountain was from July to August, and the change in canopy conductance was more active in summer, followed by spring and autumn, and the weakest in winter.
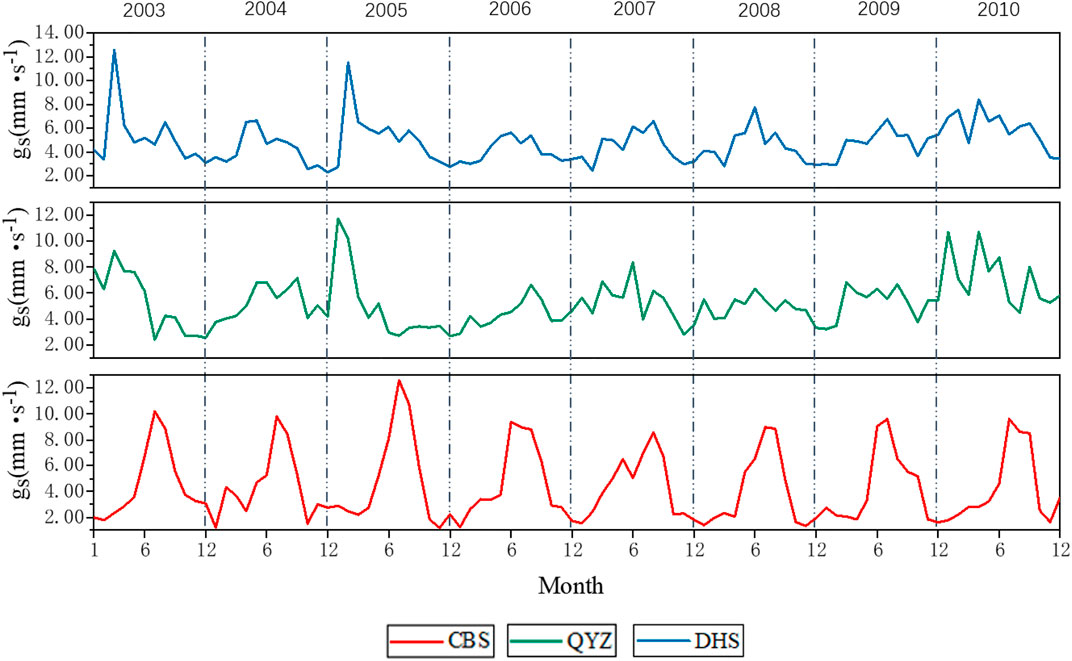
FIGURE 3. Interannual variation characteristics of canopy conductance. (DHS: Dinghushan; QYZ: Qianyanzhou; CBS: Changbai Mountain)
3.2.2 Characteristics of Monthly Canopy Conductance
The variation in average monthly canopy conductance in different climatic regions is shown in Figure 4, which shows that the canopy conductance value increased gradually from May to September, while it decreased gradually from October to next April. However, in the subtropical climate area, the canopy conductance did not reach the peak in July, and the value of canopy conductance was small. The variation dynamics of canopy conductance in Changbai Mountain was consistent with the seasonal variation and the variation trend of meteorological factors. Specifically, the canopy conductance began to increase gradually in spring, and it showed that the canopy conductance gradually increases in spring, fluctuates, and then decreases after reaching the maximum in summer, and returns to a lower level in winter. The mean variance of monthly canopy conductance was small and the change was stable, that is, the coupling degree between the change of canopy conductance and the change of environmental factors was high in temperate monsoon climate area. However, the subtropical monsoon climate region showed an obvious changing trend similar to but not completely corresponding to the seasonal change, which was also related to the seasonal growth change of leaves and the influence of forest’s underlying surface. The variation degree of the average value of monthly canopy conductance in the subtropical monsoon climate region was also high, especially in Qianyanzhou, and the trend showed a fluctuating decline.
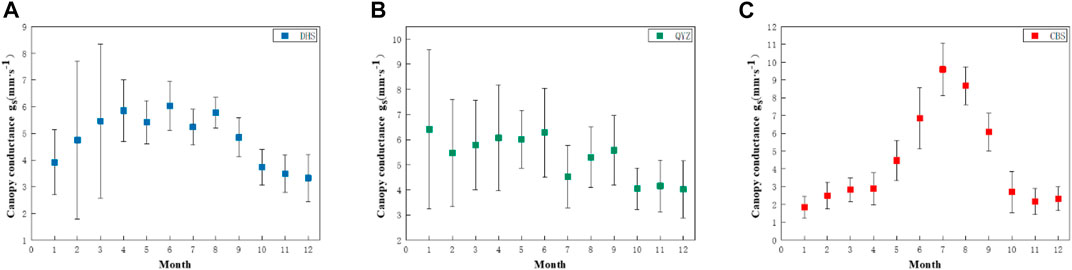
FIGURE 4. Multiyear monthly canopy conductance averages with standard variations. (A): Dinghushan; (B) Qianyanzhou; (C) Changbai Mountain).
3.2.3 Characteristics of Annual Canopy Conductance
In the variation of annual average canopy conductance (Figure 5), the annual average canopy conductance in subtropical monsoon climate area was higher than that in the temperate monsoon climate area. The average canopy conductance of Dinghushan was 4.82 mm/s−1, that of Qianyanzhou was 5.3 mm/s−1, and that of Changbai Mountain was 4.41 mm/s−1. The variation rate of interannual canopy conductance in subtropical zone was also higher than that in temperate monsoon zone, and the interannual variability of canopy conductance in Dinghushan, Qianyanzhou, and Changbai Mountain was 12.24, 13.94, and 5.51%, respectively. The average annual variation of canopy conductance increases with passing years on the whole. Global warming may lead to an overall trend of temperature rise. Climate change will affect forest canopy transpiration and increase the transpiration rate of forest ecosystem.
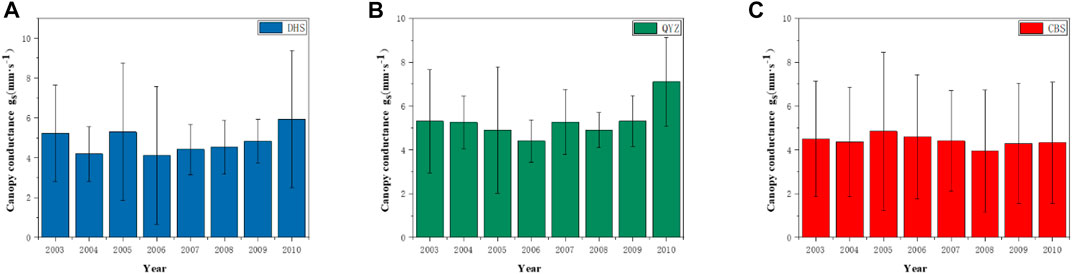
FIGURE 5. Annual canopy conductance averages with standard variations. (A): Dinghushan; (B) Qianyanzhou; (C) Changbai Mountain).
3.3 Correlation Analysis Between Canopy Conductance and Environmental Factors
Correlation analysis was conducted between canopy conductance values and environmental factors such as temperature (Ta), net radiation (Rn), vapor pressure deficit (VPD), and soil water content (SWC) (as shown in Figure 6) in different climate zones. The results showed that net radiation and temperature had different responses to canopy conductance in different climate regions. Although the change of canopy conductance in subtropical monsoon climate region had a positive correlation with the response of net radiation and temperature, the correlation coefficient was small. The response of a single factor to canopy conductance was weakened, and in 2003, 2004, and 2006, the change in canopy conductance was inconsistent with the changing trend of net radiation and temperature. They decreased with the increase in net radiation. Similarly, the temperature also had such a changing trend. In the temperate monsoon climate area, the canopy conductance increased with the increase of net radiation and temperature, and there was a significant correlation between it and the change of canopy conductance, R2 was 0.715 and 0.780. Therefore, the change of canopy conductance in the temperate monsoon climate was sensitive to the change of temperature and net radiation, that is, net radiation and temperature play an important role in the change of forest canopy conductance in the temperate monsoon climate area.
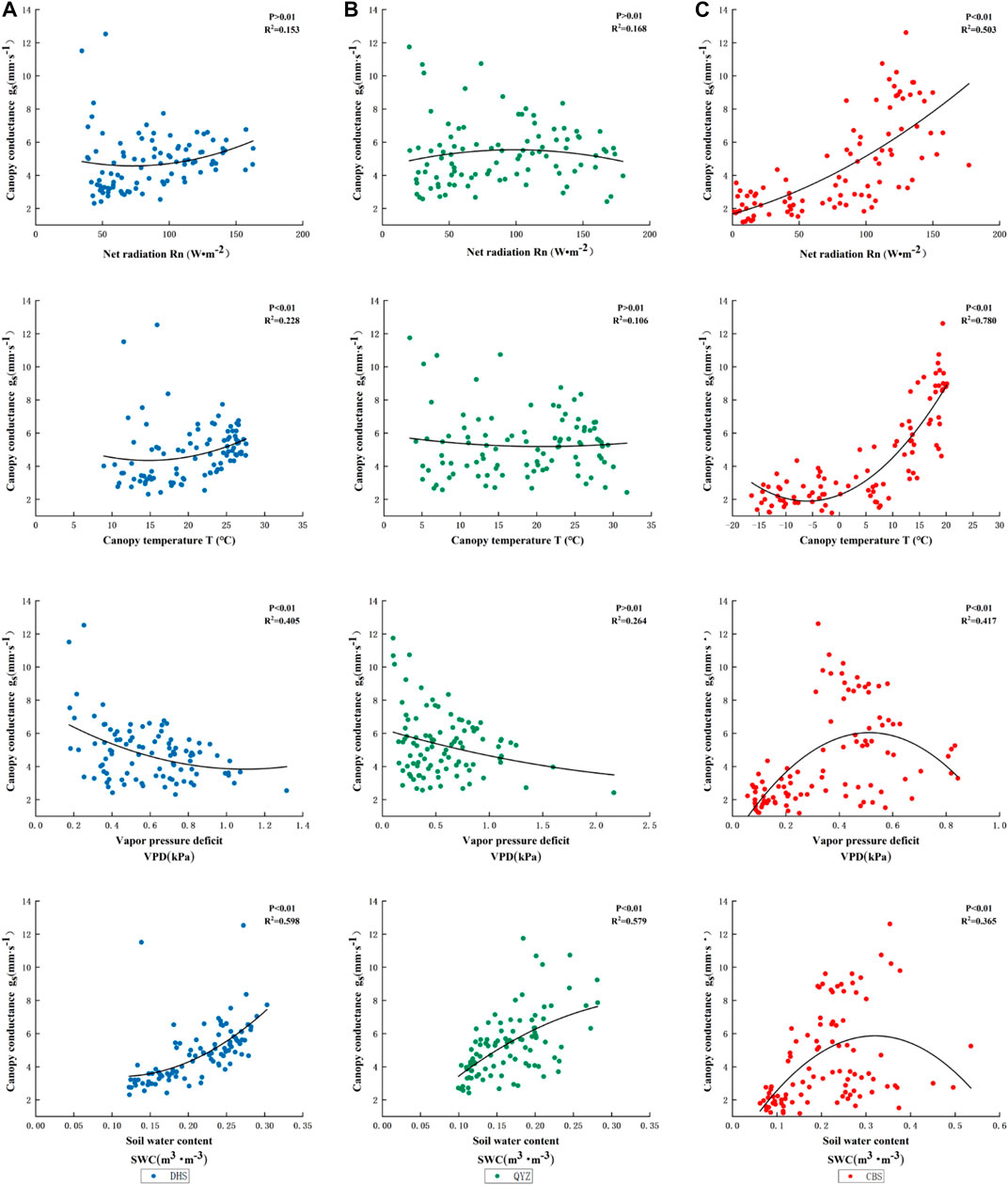
FIGURE 6. Correlation between canopy conductance and environmental factors. (A): Dinghushan; (B) Qianyanzhou; (C) Changbai Mountain).
The canopy conductance also had a strong correlation with the vapor pressure deficit, and the canopy conductance of subtropical forest also decreased with the increase in vapor pressure deficit. Therefore, the vapor pressure deficit was an important factor affecting the change in subtropical canopy conductance. R2 of Dinghushan, Qianyanzhou, and Changbai Mountain were 0.405, 0.264, and 0.417,respectively, p < 0.01, while in Changbai Mountain, under the obvious climate conditions of hydrothermal synchronization, when the vapor pressure deficit was low, with the increase in temperature and the increase in vapor pressure in the substomatal cavity, water escaped from the plant leaves, strengthened the transpiration rate, and increased the canopy conductance. When the vapor pressure deficit was higher than 0.5 kPa, the canopy conductance decreased gradually.
Soil water content was a physical quantity indicating the degree of soil dryness and wetness in a certain depth of soil layer, and soil humidity determines the water supply of vegetation. Canopy conductance in different climatic areas was more sensitive to soil water content, R2 was 0.598, 0.579, and 0.365, p < 0.01. With the increase of soil water content, the canopy conductance in subtropical monsoon climate area obviously increased in logarithmic function. The influence of the change of soil water content in Changbai Mountain on canopy conductance was different from that in subtropical monsoon area. The change of soil water content in Changbai Mountain had a certain threshold. When the soil water content was lower than 0.3, the canopy conductance increased with the increase in water content, but when the soil water content was higher than 0.3, the canopy conductance decreased gradually with the increase in water content.
3.4 Driving Ability of Environmental Factors to Canopy Conductance Change
3.4.1 Estimation of Contribution Rate of Environmental Factors to Canopy Conductance Change
In order to deeply study the driving force of various environmental factors on canopy conductance under different climate types, this study used the factor contribution rate to clarify the driving ability of environmental factors on canopy conductance. The contribution rate of various environmental factors of different climate types to driving the change of canopy conductance is shown in Figure 7. The factor with the strongest driving force of Dinghushan environmental factors on canopy conductance was soil water content, with a contribution rate of 36.01%, followed by temperature. In terms of net radiation and vapor pressure deficit, the strongest driving force of Qianyanzhou environmental factors on canopy conductance was vapor pressure deficit, with a contribution rate of 29.4%, followed by temperature, net radiation, and soil water content. The strongest driving factor of environmental factors on canopy conductance in Changbai Mountain was temperature, with a contribution rate of 28.14%, followed by net radiation, vapor pressure deficit, and soil water content, that is, soil water content was the first driving factor of canopy conductance change in subtropical monsoon climate area, and vapor pressure deficit was the first driving factor of canopy conductance change in subtropical monsoon climate area, The first driving factor of canopy conductance change in temperate monsoon climate area was temperature.
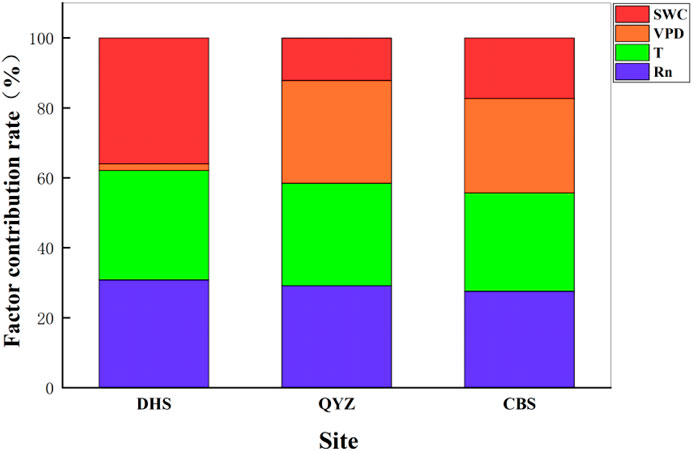
FIGURE 7. Contribution of different forest environmental factors to canopy conductance change. (DHS: Dinghushan; QYZ: Qianyanzhou; CBS: Changbai Mountain).
3.4.2 Synergistic Driving Effect Among Environmental Factors
The driving effect of various factors on canopy conductance was not invariable, and the change in canopy conductance was not only driven by a single factor but also driven by other factors. Therefore, we selected the environmental factors with the highest and second highest contribution rate to the change in canopy conductance in different climate regions, and conducted a three-dimensional two-factor analysis (as shown in Figure 8) to explore the synergistic driving ability of factors in different climate regions on the change in canopy conductance. In this study, when the canopy conductance value in the climate area was greater than 4 mm s−1, it indicated that the canopy conductance was high.
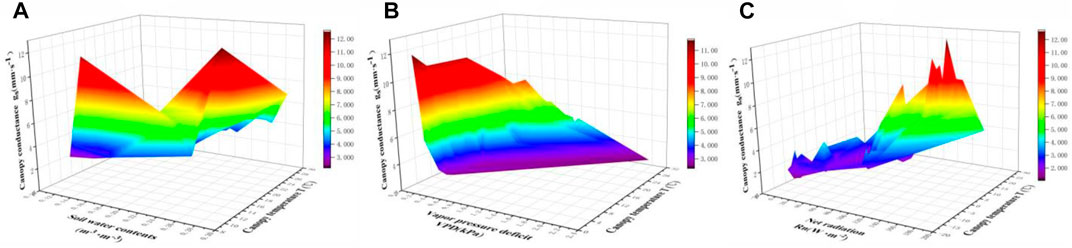
FIGURE 8. Three dimensional two factor driven analysis. (A): Dinghushan; (B) Qianyanzhou; (C) Changbai Mountain).
Soil water content was the factor driving the change in canopy conductance in the South subtropical monsoon climate zone, and temperature was the factor contributing the second; when soil water content was 0.12–0.3 m3 m−3, and the temperature was 13–24°C, it had a strong synergistic driving effect on the canopy conductance in the south subtropical monsoon climate zone. However, with the increase in water content and temperature, the canopy conductance increased. When the temperature was too low, plants would be subjected to low-temperature stress and the canopy conductance decreased.
Vapor pressure deficit is the environmental factor with the largest driving contribution to the change in canopy conductance in the mid-subtropical monsoon climate area, and there was a significant negative correlation between it and the change of canopy conductance. The vapor pressure deficit will affect the opening of stomata and the change of canopy conductance. Compared with the south subtropical climate area, the suitable threshold of tree species for temperature was wide in the mid-ssubtropical climate area, and the suitable temperature threshold was 5–28°C. With the increase in temperature, the canopy conductance increased, but when the temperature exceeded the threshold range, it had a strong inhibitory effect on the canopy conductance, and the canopy conductance value was low. Therefore, when the vapor pressure deficit was 0.2–1.5 kPa and the temperature was 5–28°C, it had a strong synergistic driving effect on the canopy conductance in the mid-subtropical monsoon climate area.
Temperature contributed greatly to driving changes of canopy conductance in monsoon climate region. Changbai Mountain belongs to the temperate monsoon climate zone, and the heat conditions on the canopy conductance change driving effect was greater than the water condition, and the optimum temperature range was narrow and for 5–20°C, the suitable threshold of net radiation was 80–180 W m−2. To the temperate monsoon climate zone, canopy conductance synergy drive effect was stronger, as with the increase in temperature and net radiation, canopy conductance exponential function to increase, but the different climate zones environmental factors driving action had a certain threshold, when factor in climate zones outside environmental factors suitable threshold value, lower canopy conductance instead. Therefore, temperature in different climatic regions was an important factor limiting and driving canopy conductance, and there was a strong synergistic driving effect among the factors, thus jointly affecting the change in canopy conductance.
4 Discussion
4.1 Differences of Canopy Conductance in Different Climatic Regions
There were significant differences in canopy conductance at different time scales in different climatic regions. On the whole, canopy conductance increased gradually in rainy season and decreased gradually in dry season. In the temperate monsoon climate zone, the seasonal variation is obvious, so the change of canopy conductance also conforms to the trend of gradually increasing from spring, fluctuating and decreasing after reaching the maximum in summer, and finally recovering to a lower level in winter. However, the subtropical regions of China had abundant annual rainfall but frequent seasonal droughts (Xu et al., 2021). In summer, high temperature and insufficient precipitation result in mismatch between rainfall and temperature, and seasonal droughts often occur. If drought stress occurs, for example, when Qianyanzhou drought occurred in summer (2003 and 2007), the seasonal pattern of canopy conductance did not follow the general law and decreased in July, which is consistent with the results obtained by M. Xu et al. (Xu et al., 2021). If the seasonal rainfall decreases, the leaf stomata will close, and the transpiration rate will show a downward trend, that is, the stomata tended to close under water stress, resulting in the decrease of canopy conductance. The peak value of canopy conductance in Changbai Mountain was generally from July to August, which is consistent with the research results of Xiaofei Lu’s study (Lu, 2018). When the temperature increased, the increase of the vapor pressure in the lower stomatal cavity was greater than that of the air vapor pressure, so the increase of the vapor pressure deficit marked the water escape in the leaves of plants and strengthened the transpiration rate.
The canopy conductance in different climatic regions had an overall trend of increasing with the passing of the year on the annual scale, which was related to the overall rising trend of global warming temperature. Climate change will affect the canopy transpiration of forest ecosystem and increase the plant transpiration rate. Compared with the temperate monsoon climate area, the growth trend of annual average canopy conductance in the subtropical monsoon climate area was more obvious. The three stations in different climate areas were located in the east of China and belong to the monsoon affected area. The hydrothermal conditions had obvious latitudinal gradient characteristics from north to south. Therefore, with the decrease in latitude, the temperature increased and the forest structure was complex, which would affect the plant transpiration rate.
The difference of canopy conductance was also related to the structural characteristics of leaves and xyloids of tree species. The annual canopy conductance of Dinghushan was lower than that of Qianyanzhou, which was also related to the main vegetation of Dinghushan, this being evergreen broad-leaved forest, and the increase in canopy leaf area will hinder air flow, so the canopy conductance will be affected. In Qianyanzhou, coniferous species such as Masson pine and Cunninghamia lanceolata were the main species, and their stomata were round and large in diameter, while in Dinghushan, there were more broad-leaved species such as Schima superba and Castanea, and the broad-leaved stomata were slender and small (Zhang et al., 2017). During the day, coniferous forest showed a stronger stomatal sensitivity, and stomatal conductance fluctuated greatly. In addition, the xylem structure of coniferous forest was non-porous wood, which could maintain higher water transport efficiency under high vapor pressure deficit conditions (Peters et al., 2010).
4.2 Correlation Between Canopy Conductance and Environmental Factors
Canopy conductance had a corresponding response to the changes in various environmental factors, and the main driving factors of canopy conductance were different with different vegetation types. For example, in the subtropical monsoon climate zone, the response of canopy conductance to net radiation and temperature was weak, and the change of canopy conductance in 2003, 2004, and 2006 was inconsistent with the changed trend of net radiation and temperature. In case of high-temperature stress, the high-temperature stress will cause photoinhibition, and the transpiration between canopy will be weakened, thus affecting canopy conductance. Or when the net radiation is too strong, leaves lose more water, trees will adjust stomata to maintain their own water, so stomata opening threshold become smaller, the canopy conductance decreases. However, temperature and net radiation were the important factors affecting the change of canopy conductance in the temperate monsoon climate zone, which showed that with the increase in temperature and net radiation, the stomata of vegetation opened to exchange matter and energy, and the canopy conductance increased.
The canopy conductance in subtropical climate area increased significantly as the soil water content increased. In the dry season, less rainfall and low soil humidity will lead to soil drought, less vegetation water content, weak forest transpiration, and low canopy conductance. However, with the change of seasons and the advent of rainy season, the rainfall increased, the temperature increased, the transpiration became stronger, and the canopy conductance also increased. The temperate monsoon climate region had different response to canopy conductance. Although the rainfall in the subtropical monsoon region was more than temperate monsoon region, the higher temperature in the subtropical region promotes the increase of evaporation. Therefore, the soil water content promoted the increase of canopy conductivity within an appropriate threshold. When the soil water content was more than 0.3, the temperate monsoon climate region will have the phenomenon of soil overwetting due to thermal conditions, and the soil overwetting will reduce the soil aeration, affect the activities of soil microorganisms, inhibit the respiration, growth, and other life activities of vegetation roots, and weaken the transpiration of plants, thus affecting the change of canopy conductance, that is, higher soil water content cannot promote the maintenance of large opening or infinite increase of plant stomatal conductance.
The same was true for the vapor pressure deficit. Vapor pressure deficit was easily affected by hydrothermal conditions. When the vapor pressure deficit was low, with its increases, the vapor pressure in the lower stomatal cavity increased, which made the water escape from the plant leaves, strengthened the transpiration rate, increased the transpiration rate of the forest ecosystem, and the canopy conductance gradually increased. When high temperature stress occurred in summer, the vapor pressure deficit increased, resulting in the closure of some stomata and the decrease of canopy conductance. When high-temperature stress was easy to occur in summer, the vapor pressure deficit increased, resulting in the closure of some stomata, and when the vapor pressure deficit was higher than 0.5, the canopy conductance no longer increased with the increase of the vapor pressure deficit. The vapor pressure deficit was the key factor limiting the opening and closing of pores. Therefore, an appropriate vapor pressure deficit ensured the appropriate threshold of pore opening and maintained a large degree of pore opening to ensure the efficient flow of water vapor.
4.3 Synergistic Driving Effect Among Environmental Factors
In this study, the factor contribution rate was used to clarify the driving ability of environmental factors on canopy conductance in different monsoon climate regions. The results showed that in different climate regions, the driving ability of environmental factors on canopy conductance change was different, but temperature was an important factor limiting and driving canopy conductance, and there was a synergistic effect between water and temperature factors to jointly drive the change of canopy conductance. The driving ability of heat factor on the change of canopy conductance in temperate climate area was greater than that of water factor. There was a synergistic driving effect between temperature and net radiation, which jointly affected the change of canopy conductance. The first factor driving the change of canopy conductance in the South subtropical monsoon climate area was soil water content. There was a significant correlation between the change of canopy conductance and the change of soil water content, and the synergistic driving effect was obvious. When the value of temperature or soil water content was not within the threshold that was conducive to driving the increase of canopy conductance, as the factor changed, its driving effect on canopy conductance would be inhibited. During the study period, Qianyanzhou was subjected to more drought stress, resulting in increased evapotranspiration and increased vapor pressure deficit. In order to reduce water dissipation, plants will close some stomata and reduce canopy conductance. Therefore, the first factor driving the change of canopy conductance in the mid-subtropical monsoon climate area was vapor pressure deficit; temperature was still a synergistic factor, which drove the change of canopy conductance together. When the temperature was at a threshold favorable to drive the change in canopy conductance, the canopy conductance also increased with increasing temperature. But when the temperature was above or below this threshold, the driving effect of temperature on canopy conductance was suppressed. Therefore, the change of the canopy conductance in each climate area did not depend on only one main factor, but there are significant synergistic driving phenomena among all factors, which jointly affect the change of canopy conductance.
5 Conclusions
1) The driving ability of environmental factors in different climate regions to the canopy conductance was different. The contribution rate of soil water content to the canopy conductance in South subtropical monsoon climate region was the largest, it was 36.01%, and the contribution rate of vapor pressure deficit to the canopy conductance in mid-subtropical monsoon climate region was the largest, and it was 29.4%, and the contribution rate of temperature to the canopy conductance in temperate monsoon climate region was the largest, it being 28.14%.
2) For different climate regions, temperature was an important factor limiting and driving canopy conductance, and there was a synergistic effect between moisture and temperature factors to jointly drive the change of canopy conductance. In the south tropical monsoon climate region, the synergistic effect of soil water content and temperature on canopy conductance was stronger. In the mid-subtropical monsoon climate region, vapor pressure deficit and temperature played a synergistic role in driving canopy conductance. In the temperate monsoon climate region, the net radiation and temperature synergistically drove the change of canopy conductance.
3) The synergistic driving effects of environmental factors in different climatic regions all had a certain threshold. When the soil water content was 0.12–0.3 m3 m−3 and the temperature was 13–24°C, there was a strong positive synergistic driving effect on the canopy conductance in the subtropical monsoon climate zone. In the mid-subtropical monsoon climate zone, when the water vapor pressure deficit was 0.2–1.5 kPa and the temperature was 5–28°C, there was a strong synergistic driving effect on the canopy conductance, which showed that the canopy conductance increased with the increase of temperature. When the temperature was 5–20°C and the net radiation was 80–180 w•m−2, the temperature and net radiation had a stronger effect on the canopy conductance in the temperate monsoon climate zone.
5.1 Permission to Reuse and Copyright
The author agreed that figures, tables, and images will be published under a Creative Commons CC-BY license and permission must be obtained for use of copyrighted material from other sources (including re-published/adapted/modified/partial figures and images from the internet). It was the responsibility of the authors to acquire the licenses, to follow any citation instructions requested by third-party rights holders, and cover any supplementary charges.
Data Availability Statement
Publicly available datasets were analyzed in this study. These data can be found here: http://www.cnern.org.cn/.
Author Contributions
R-QW (First Author) contributed to writing the whole manuscript, count the final data and analyze the results, and complete the first draft. J-BJ (Corresponding Author) contributed to conception and design of the study, provided funding support, and supervised project schedule. W-DY (corresponding author) contributed to comment on article structure and content revisions, provided funding support, and supervised the progress of the manuscript. LH contributed to the manuscript and performed the data collation and calculation. Y-FW validated the results of the paper and revised the article. YC performed the statistical analysis and used software to present more intuitive results. All authors agree to be accountable for the content of the work.
Funding
This research was funded by the key research and development program in Hunan province (2020NK2022) and Three Gorges Follow-up Project of Ministry of Water Resources (HY110161A0012022).
Conflict of Interest
The authors declare that the research was conducted in the absence of any commercial or financial relationships that could be construed as a potential conflict of interest.
Publisher’s Note
All claims expressed in this article are solely those of the authors and do not necessarily represent those of their affiliated organizations, or those of the publisher, the editors, and the reviewers. Any product that may be evaluated in this article, or claim that may be made by its manufacturer, is not guaranteed or endorsed by the publisher.
Acknowledgments
The authors gratefully acknowledge all the grants for this research and the teachers and colleague who helped them to finalize the study.
References
Bai, Y., Li, X., Liu, S., and Wang, P. (2017). Modelling Diurnal and Seasonal Hysteresis Phenomena of Canopy Conductance in an Oasis Forest Ecosystem. Agric. For. Meteorology 246, 98–110. doi:10.1016/j.agrformet.2017.06.006
Bai, Y. J., Zhu, Y. J., Ma, J. Y., Liu, P., Yang, R. Z., Li, C., et al. (2020). Seasonal Variation in Canopy Conductance of Urban Green Land and its Environmental Control. Chin. J. Ecol. 39 (1), 120–129. doi:10.13292/j.1000-4890.202001.028
Chen, S.-N., Chen, Z.-S. -N., and Zhang, Z.-Q. (2021). Canopy Stomatal Conductance Characteristics of Pinus Tabulaeformis and Acer Truncatum and Their Responses to Environmental Factors in the Mountain Area of Beijing. Chin. J. Plant Ecol. 45 (12), 1329–1340. doi:10.17521/cjpe.2021.0198
Dai, X. Q., Wang, H. M., Xu, M. J., Yang, F., Wen, X., Chen, Z., et al. (2021). An Observation Dataset of Carbon and Water Fluxes of Artificial Coniferous Forestsin Qianyanzhou(2003-2010). Chin. Sci. data 6 (01), 36. Online edition in English and Chinese. doi:10.11922/csdata.2020.0036.zh
De Boer, H. J., Lammertsma, E. I., Wagner-Cremer, F., Dilcher, D. L., Wassen, M. J., and Dekker, S. C. (2011). Climate Forcing Due to Optimization of Maximal Leaf Conductance in Subtropical Vegetation under Rising CO2. Proc. Natl. Acad. Sci. U.S.A. 108 (10), 4041–4046. doi:10.1073/pnas.1100555108
Deng, J., Yao, J., Zheng, X., and Gao, G. (2021). Transpiration and Canopy Stomatal Conductance Dynamics of Mongolian Pine Plantations in Semiarid Deserts, Northern China. Agric. Water Manag. 249 (11b), 106806–113938. doi:10.1016/j.agwat.2021.106806
Endler, J. A., Campbell, A. G. S., and Norman, J. M. (1999). An Introduction to Environmental Biophysics. Gaylon S. Campbell , John M. Norman. Q. Rev. Biol. 74, 488–489. doi:10.1086/394187
Evaristo, J., Jasechko, S., and Mcdonnell, J. J. (2015). Global Separation of Plant Transpiration from Groundwater and Streamflow. Nature 525 (7567), 91–94. doi:10.1038/nature14983
Fu, S., Sun, L., and Luo, Y. (2016). Canopy Conductance and Stand Transpiration of Populus Simonii Carr in Response to Soil and Atmospheric Water Deficits in Farmland Shelterbelt, Northwest China. Agroforest Syst. 91 (6), 1165–1180. doi:10.1007/s10457-016-0002-4
Gillner, S., Korn, S., Hofmann, M., and Roloff, A. (2017). Contrasting Strategies for Tree Species to Cope with Heat and Dry Conditions at Urban Sites. Urban Ecosyst. 20, 853–865. doi:10.1007/s11252-016-0636-z
Granier, A., Biron, P., and Lemoine, D. (2000). Water Balance, Transpiration and Canopy Conductance in Two Beech Stands. Agric. For. Meteorology 100 (4), 291–308. doi:10.1016/S0168-1923(99)00151-3
Grossiord, C., Buckley, T. N., Cernusak, L. A., Novick, K. A., Poulter, B., Siegwolf, R. T. W., et al. (2020). Plant Responses to Rising Vapor Pressure Deficit. New Phytol. 226, 1550–1566. doi:10.1111/nph.16485
Harris, P. P., Huntingford, C., Cox, P. M., Gash, J. H. C., and Malhi, Y. (2004). Effect of Soil Moisture on Canopy Conductance of Amazonian Rainforest. Agric. For. Meteorology 122 (3-4), 215–227. doi:10.1016/j.agrformet.2003.09.006
Hu, Y., Duman, T., Vanderklein, D., Zhao, P., and Schäfer, K. V. (2019). A Stomatal Optimization Approach Improves the Estimation of Carbon Assimilation from Sap Flow Measurements. Agric. For. Meteorology 279, 107735. doi:10.1016/j.agrformet.2019.107735
Igarashi, Y., Kumagai, T. o., Yoshifuji, N., Sato, T., Tanaka, N., Tanaka, K., et al. (2015). Environmental Control of Canopy Stomatal Conductance in a Tropical Deciduous Forest in Northern Thailand. Agric. For. Meteorology 202, 1–10. doi:10.1016/j.agrformet.2014.11.013
Komatsu, H., Onozawa, Y., Kume, T., Tsuruta, K., Shinohara, Y., and Otsuki, K. (2012). Canopy Conductance for a Moso Bamboo (Phyllostachys Pubescens) Forest in Western Japan. Agric. For. Meteorology 156, 111–120. doi:10.1016/j.agrformet.2012.01.004
Kumagai, T. o., Tateishi, M., Shimizu, T., and Otsuki, K. (2008). Transpiration and Canopy Conductance at Two Slope Positions in a Japanese Cedar Forest Watershed. Agric. For. Meteorology 148, 1444–1455. doi:10.1016/j.agrformet.2008.04.010
Li, Y. L., Yan, J. H., Meng, Z., Huang, J. Q., Zhang, L. M., Chen, Z., et al. (2021). An Observation Dataset of Carbon and Water Fluxes in a Mixed Coniferous Broad-Leaved Forest at Dinghushan, Southern China (2003-2010). Chinese Sci. Data 6 (01). doi:10.11922/csdata.2020.0046.zh
Lu, X. F. (2018). Response of Carbon-Water Flux to Climate Change in Temperateconiferous and Broad-Leaved Mixed Forests in Changbai Mountain. Shenyang City, China: Shenyang Agricultural University.
Martin, T. A., Brown, K. J., Cermák, J., Ceulemans, R., Kucera, J., Meinzer, F. C., et al. (1997). Crown Conductance and Tree and Stand Transpiration in a Second-Growth Abies Amabilis Forest. Can. J. For. Res. 27, 797–808. doi:10.1139/x97-006
Mo Kangle, 莫., Chen Lixin, 陈., Zhou Jie, 周., Fang Xianrui, 方., Kang Manchun, 康., and Zhang Zhiqiang, 张. (2014). Transpiration Responses of a Poplar Plantation to the Environmental Conditions on a Floodplain in Northern China. Acta Eco Sin. 34 (20), 5812–5822. doi:10.5846/stxb201301280172
Pataki, D. E., Oren, R., Katul, G., and Sigmon, J. (1998). Canopy Conductance of Pinus Taeda, Liquidambar Styraciflua and Quercus Phellos under Varying Atmospheric and Soil Water Conditions. Tree Physiol. 18 (5), 307–315. doi:10.1093/treephys/18.5.307
Peters, E. B., Mcfadden, J. P., and Montgomery, R. A. (2010). Biological and Environmental Controls on Tree Transpiration in a Suburban Landscape. J. Geophys. Res. 115 (G4), 1266. doi:10.1029/2009jg001266
Richardson, A. D., Keenan, T. F., Migliavacca, M., Ryu, Y., Sonnentag, O., and Toomey, M. (2013). Climate Change, Phenology, and Phenological Control of Vegetation Feedbacks to the Climate System. Agric. For. Meteorology 169, 156–173. doi:10.1016/j.agrformet.2012.09.012
Rodriguez-Dominguez, C. M., Hernandez-Santana, V., Buckley, T. N., Fernández, J. E., and Diaz-Espejo, A. (2019). Sensitivity of Olive Leaf Turgor to Air Vapour Pressure Deficit Correlates with Diurnal Maximum Stomatal Conductance. Agric. For. Meteorology 272-273, 156–165. doi:10.1016/j.agrformet.2019.04.006
Rogers, A., Medlyn, B. E., Dukes, J. S., Bonan, G., Caemmerer, S., Dietze, M. C., et al. (2017). A Roadmap for Improving the Representation of Photosynthesis in Earth System Models. New Phytol. 213, 22–42. doi:10.1111/nph.14283
Saito, T., Kumagai, T. o., Tateishi, M., Kobayashi, N., Otsuki, K., and Giambelluca, T. W. (2017). Differences in Seasonality and Temperature Dependency of Stand Transpiration and Canopy Conductance between Japanese Cypress (Hinoki) and Japanese Cedar (Sugi) in a Plantation. Hydrol. Process. 31 (10), 1952–1965. doi:10.1002/hyp.11162
She, D., Xia, Y., Shao, M., Peng, S., and Yu, S. (2013). Transpiration and Canopy Conductance of Caragana Korshinskii Trees in Response to Soil Moisture in Sand Land of China. Agroforest Syst. 87, 667–678. doi:10.1007/s10457-012-9587-4
Song, L., Zhu, J., Zhang, T., Wang, K., Wang, G., and Liu, J. (2021). Higher Canopy Transpiration Rates Induced Dieback in Poplar (Populus × Xiaozhuanica) Plantations in a Semiarid Sandy Region of Northeast China. Agric. Water Manag. 243, 106414. doi:10.1016/j.agwat.2020.106414
Wang, S. Y., Zhang, X. X., Zhu, T., Yang, W., and Zhao, J. Y. (2016). Assessment of Ecological Environment Quality in the Changbai Mountain NatureReserve Based on Remote Sensing Technology. Prog. Gogr. 35 (10), 1269–1278. doi:10.18306/dlkxjz.2016.10.010
Wang, Z. Y., Yan, W. D., Yan, C. Z., Luo, Z., and Jia, J. (2021). Simulation and Environmental Response of Canopy-Atmospheric transpiration.Conductance of Platycladus Orientalis Forests in the Beijing Mountain Area. Acta Ecol. Sin. 41 (6), 11. doi:10.5846/stxb201910202197
Wehr, R., Commane, R., Munger, J. W., McManus, J. B., Nelson, D. D., Zahniser, M. S., et al. (2017). Dynamics of Canopy Stomatal Conductance, Transpiration, and Evaporation in a Temperate Deciduous Forest, Validated by Carbonyl Sulfide Uptake. Biogeosciences 14 (2), 389–401. doi:10.5194/bg-14-389-2017
Wen, X. F., Yu, G. R., Sun, X. M., and Liu, Y. F. (2005). Turbulence Flux Measurement above the Overstory of a Subtropical Pinus Plantation over the Hilly Region in Southeastern China. Sci. China(Series D:Earth Sci. 48 (S1), 63–73. doi:10.1360/05zd0006
Wu, J. B., Guan, D. X., Wang, A. Z., Yuan, F. H., Diao, H. Y., Yu, G. R., et al. (2021). A Dataset of Carbon and Water Flux Observation in a Broad-Leaved Red Pine Forestin Changbai Mountain(2003-2010). Sci. Data Bank. 6 (01), 41. Chinese science data: Online edition in English and Chinese. doi:10.11922/csdata.2020.0041.zh
Xu, S., Yu, Z., Zhang, K., Ji, X., Yang, C., and Sudicky, E. A. (2018). Simulating Canopy Conductance of the Haloxylon Ammodendron Shrubland in an Arid Inland River Basin of Northwest China. Agric. For. Meteorology 249, 22–34. doi:10.1016/j.agrformet.2017.11.015
Xu, M., Hu, J., Zhang, T., Wang, H., Zhu, X., Wang, J., et al. (2021). Specific Responses of Canopy Conductance to Environmental Factors in a Coniferous Plantation in Subtropical China. Ecol. Indic. 131, 108168. doi:10.1016/j.ecolind.2021.108168
Yoshida, M., Ohta, T., Kotani, A., and Maximov, T. (2010). Environmental Factors Controlling Forest Evapotranspiration and Surface Conductance on a Multi-Temporal Scale in Growing Seasons of a Siberian Larch Forest. J. Hydrology 395 (3-4), 180–189. doi:10.1016/j.jhydrol.2010.10.023
Yoshifuji, N., Kumagai, T. o., Ichie, T., Kume, T., Tateishi, M., Inoue, Y., et al. (2020). Limited Stomatal Regulation of the Largest-Size Class of Dryobalanops Aromatica in a Bornean Tropical Rainforest in Response to Artificial Soil Moisture Reduction. J. Plant Res. 133 (2), 175–191. doi:10.1007/s10265-019-01161-3
Yu, G.-R., Wen, X.-F., Sun, X.-M., Tanner, B. D., Lee, X., and Chen, J.-Y. (2006). Overview of ChinaFLUX and Evaluation of its Eddy Covariance Measurement. Agric. For. Meteorology 137 (3-4), 125–137. doi:10.1016/j.agrformet.2006.02.011
Keywords: canopy conductivity, Penman-Monteith model, driving mechanism, environmental factors, monsoon climate zones
Citation: Wu R-Q, Jia J-B, Yan W-D, Hu L, Wang Y-F and Chen Y (2022) Characteristics of Canopy Conductance and Environmental Driving Mechanism in Three Monsoon Climate Regions of China. Front. Environ. Sci. 10:935926. doi: 10.3389/fenvs.2022.935926
Received: 04 May 2022; Accepted: 30 May 2022;
Published: 11 July 2022.
Edited by:
Jifeng Deng, Shenyang Agricultural University, ChinaReviewed by:
Wenping Deng, Jiangxi Agricultural University, ChinaGuodong Jia, Beijing Forestry University, China
Copyright © 2022 Wu, Jia, Yan, Hu, Wang and Chen. This is an open-access article distributed under the terms of the Creative Commons Attribution License (CC BY). The use, distribution or reproduction in other forums is permitted, provided the original author(s) and the copyright owner(s) are credited and that the original publication in this journal is cited, in accordance with accepted academic practice. No use, distribution or reproduction is permitted which does not comply with these terms.
*Correspondence: Jian-Bo Jia, amlhamlhbmJvQGNzdWZ0LmVkdS5jbg==; Wen-De Yan, Y3NmdXl3ZEBob3RtYWlsLmNvbQ==