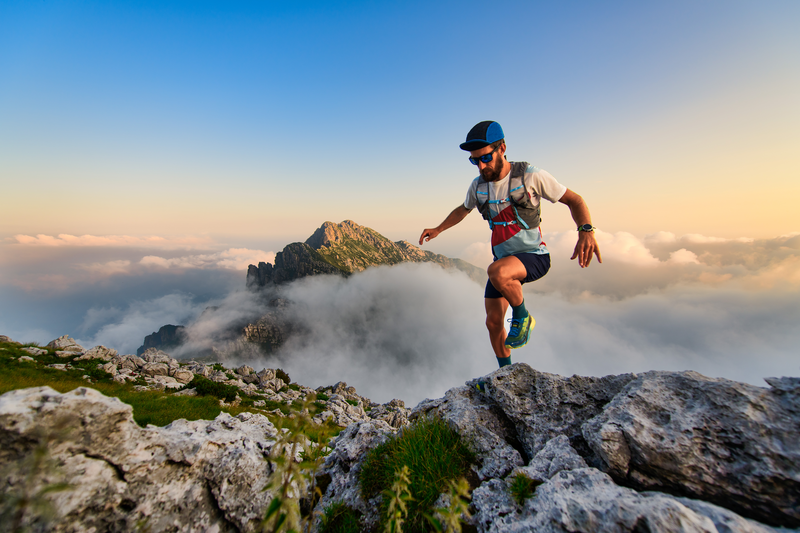
95% of researchers rate our articles as excellent or good
Learn more about the work of our research integrity team to safeguard the quality of each article we publish.
Find out more
REVIEW article
Front. Environ. Sci. , 19 August 2022
Sec. Soil Processes
Volume 10 - 2022 | https://doi.org/10.3389/fenvs.2022.935785
This article is part of the Research Topic Land Degradation Pattern and Ecosystem Services View all 12 articles
One billion hectares of land worldwide is affected by several kinds of salinity and associated problems. The soil quality (SQ) in salt-affected soil (SAS) is impaired because of the presence of excess electrolytes, disproportionate Na and Ca in soil solution and exchange phase, rhythmic changes in the hydrological cycle, decreasing soil organic matter, poor vegetative cover, low soil biological activity, and crop residue return. Sodic and saline–sodic soils have the potential to provide alkaline reactions and soil physical constraints to regulate the soil attributes affecting SQ. Because of high spatial variability and rapid temporal changes, selection of simple, robust, low cost, and high-throughput master indicators for assessing SQ is very essential for monitoring the aggradation or degradation of SAS. Therefore, screening the master indicators for developing a minimum dataset for SQ assessment of SAS is an important issue for sustainable management of soil in these agro-ecologies. We captured the SQ indicators for SAS from several ecosystems of different countries and discussed the problems of parameterization for assessing SQ. Improved SQ for optimum soil functioning is needed for confirming agricultural productivity and food security around the globe. This review describes the causes and drivers for sodification/salinization and mechanism-oriented rehabilitation options such as the application of mineral gypsum, flue-gas–desulfurized gypsum, elemental S, acidified biochar, polymer, salt tolerance mechanisms, and other agro-techniques for improving the quality of SAS. Based on the SQ assessment, a suite of site-specific soil management practices are advocated for the greening of SAS and prosperity.
The presence and abundance of soluble and precipitated salts are the primary cause of soil salinization, halting ecosystem functions and limiting crop growth and production (Seleiman et al., 2022). Rehabilitation of salt-affected soil (SAS) is a principal agenda in the present plans of developing countries and countries of arid–semi-arid regions to meet the food–feed–bioenergy need of the expanding population. Shortage of freshwater, erratic rain, increase in temperature, the incidence of drought, rise in evapotranspiration requirement of the crops, sea-level rise, intrusion of brackish water, ingression of sea–water, extension of irrigated farming in the canal command area, and dependency on wastewater for irrigation causes increase the salt load in the farmland (Al-Suhaibani et al., 2021; Alkharabsheh et al., 2021; Badawy et al., 2021). Furthermore, inappropriate drainage networks increase the risk of salinization/alkalization and cause deterioration of soil health (Kamra, 2021).
Based on the nature and stoichiometric dominance of electrolytes, SAS is categorized into saline, sodic, and saline–sodic soils. Saline soil has an electrolytic conductivity of the aqueous soil saturated paste extract (ECe) higher than 4.0 dS m−1 at 25°C and the pH of the saturation paste (pHs) less than 8.2 and ESP (exchangeable sodium percent) less than 15 or/and sodium adsorption ratio (SARe) of the same saturated paste extract <13.0 mmol1/2 L−1/2 (Abrol et al., 1998). Soil salinization occurs because of the existence of water-soluble salts in the soil; a rising flux of saline underground water; prolonged irrigation with saline, brackish, or wastewater; and drainage congestion and intrusion of saline seawater in the coastal areas (Singh, 1998; Eswar et al., 2021). The sodic soils are usually known as “alkali soils” carrying a disproportionately larger quantity of Na+ than Ca2+ and Mg2+ in the soil solution and exchange phase. The soil reaction (pHs) in this type of soil >8.2, ESP of greater than 15 or/and sodium adsorption ratio (SARe) of the saturated paste extract >13.0 mmol1/2 L−1/2 and variable electrolyte concentration. These soils are categorized as “saline–sodic” having pHs of >8.5, ESP of >15%, SARe>13 mmol1/2 L−1/2, and ECe of >4 dS m−1 at 25°C. Irrigation with sodic water (presence of carbonates and bicarbonates of Na) and a shallow sodic water table led to soil sodification (Choudhary et al., 2011; Sheoran et al., 2021). The unfavorable influence of different types of salts on soil properties creates limitations for crop production (Taha et al., 2020; Ding et al., 2021; Taha et al., 2021; Zain et al., 2021). Therefore, reclamation and strategic management are requisite for crop production (Rai et al., 2021c; Hopmans et al., 2021).
Soil health or soil quality is largely defined as “fitness for use” or “capacity of the soil to function” (Karlen et al., 1997). A modest attempt had been made for assessing the soil quality of agricultural soil (Masto et al., 2008a; Martins et al., 2017; Basak et al., 2021a). But only a few groups had attempted a comprehensive assessment of soil quality of salt-affected soils. Some discrete studies identified key soil quality indicators for addressing soil quality of saline and sodic soil (Mahajan et al., 2016, 2021b; Sione et al., 2017; Vasu et al., 2018; Yu et al., 2018). It is a well-established fact that reclamation and management of salt-affected soils are associated with a decline in ESP, ECe, pHs, and improved crop yield (Rai et al., 2022; Sheoran et al., 2022). But, the reclamation and management strategies in SAS had an influence on all soil processes influencing the physical, chemical, biological, and biochemical properties of soil (Mahajan et al., 2021b; Zhao et al., 2021). The relative response of different soil attributes depends upon the intrinsic soil properties, geological settings, hydrological cycle, and other pedogenic forces driving salinization and sodication (Jobbágy and Jackson, 2004). The nature and amount of the amendment needs are also governed by these factors. The amendments needed for bringing the same amount of change in soil quality to perform the desired function in vertisols are different from those of inceptisols at the same level of pH and ESP in the semi-arid region of the Indian Deccan Plateaus (Pal et al., 2006). Therefore, linking all the management and reclamation needs with the change in soil quality desired is an important aspect of the management of these soils. In the absence of a wholesome soil quality index, the imbalance in fertilization and other management practices not only reduces the use efficiency of applied nutrients but also increases the cost of cultivation. Overuse of some nutrients also acts as a non-point source of pollution in different components of the ecosystem. Soil salinity and use of poor quality groundwater (saline/sodic with high RSC; residual sodium carbonate) further aggravate the agrarian distress. Therefore, orienting the crop management decisions while keeping soil quality or soil health in focus is the first and foremost step needed to manage soils more efficiently and ensure profitability. Therefore, this study aimed to provide an overview of the global extent of salt-affected soils, strategies for successful rehabilitation, and approaches for soil quality assessment followed worldwide. The mechanism for salinity tolerance and agronomic measures for the management and their impact on soil quality are critically discussed. Furthermore, issues related to parameterization in salt-affected soils for developing a minimum dataset for describing the changes in soil attributes after the reclamation of salt-affected soils, soil quality assessment, and future research needs are also highlighted.
This review was developed based on the published information on the extent, distribution, characteristics, development, rehabilitation strategies, salt-tolerance mechanisms in crop plants, and soil quality assessment as per the requirement of the hypothesis of the review. The information available from Google Scholar, Crossref, Google Scholar profile, Pubmed, ScienceDirect.com, Springer.com, Wiley Online Library, CSIRO Publishing, FAO reports, and CSSRI database was retrieved using relevant keywords. Based on the comprehensive review of the available literature (n = 161), present state and key issues associated with the soil quality assessment of salt-affected soil were developed as envisaged in the hypotheses and objective of this review.
Soluble salts of different nature and quantities are present in salt-affected soils. These salts are formed with a combination of cations (Na+, Ca2+, Mg2+, and K+) and anions [Cl−, SO42–, CO32–, HCO3−, and SiOx–n as and minor quantity of (H)xPO4n and NO3−]. Considering the stoichiometric dominance of electrolytes and their nature, SAS are classified into saline, sodic, and saline–sodic.
Accumulation of soluble minerals from weathering of rocks and minerals, aeolian deposits, and evaporation of seawater in geological areas with drainage congestion are the reasons for soil salinization (Gupta and Mathur, 2011). Scarcity of rainfall, shift in precipitation patterns, high air temperature which led to intense evaporation, and shortage in surface irrigation water resources are the reasons for secondary salt accumulation in irrigated agriculture. Faulty irrigation management through border irrigation in the canal command area, imperfect drainage system, and excessive use of underground saline/alkali water without proper management also hastened soil salinity development (Cai et al., 2010). An extensive irrigation network had been developed in Indo-Gangetic Plain in India and Pakistan (Fishman, 2018; Hayat et al., 2020), China (Xu et al., 2013), Australia (Ranatunga et al., 2010), Egypt (Abdel-Fattah et al., 2020), and United States (Hansen et al., 2018) for creating the habitation settlement, crop productivity assurance, and increasing the livelihood security. Irrigation in some regions without giving due consideration to irrigability classification caused accumulation of the appreciable quantity of electrolytes (Na+, Ca2+, and Mg2+ salts) present in irrigation water in the root zone. Some proportions of electrolytes maintain soil permeability or prevent aggregate failure, loss in soil permeability, and drainage congestion (Quirk, 2001; Bennett et al., 2019). The poor vegetation cover and excess use of irrigation water and agricultural input alters the equilibrium of the underground water and causes exposure of soils to salts. Rise in mean air temperature causes an increase in evaporation demand as well as change and shifting in rainfall distribution. This phenomenon, in turn, increases/decreases water availability, drainage congestion or occurrence of flood, and land subsidence and soil erosion at coastal lines (Brammer, 2014). Sea level rise because of the melting of glacial snow and thermal expansion of seas are responsible for the inundation of adjacent coastlines (Eswar et al., 2021). The sea-level rise and terrestrial ingress of brackish water is the primary threat to global coastal ecosystems (Dasgupta et al., 2015). Heavy storm and/or surges influence the temporary inundation of low-lying coastal lines with brackish water. The recurring intrusion of seawater by land-use change from paddy rice to brackish aquaculture or shrimp farming is also responsible for soil salinization, build-up of acidity, and losses of organic C content, which increases the vulnerability of the rice ecosystem in coastal areas (Ali, 2006; Blankespoor et al., 2017). The climate change also drives soil salinization by promoting upward salt flux because of the increased temperature of the earth and seawater and changes in rainfall patterns (Wasko and Sharma, 2017; Seleiman and Kheir, 2018).
Carbonate salts supplied through weathering of alkaline alumino–silicate minerals, shallow groundwater table, large evaporation demand, and aeolian deposition favor the build-up of these salts (Jobbágy et al., 2017). The Indus basin of India (Qadir et al., 2018; Sheoran et al., 2021), the vertisols (Shirale et al., 2018) of the Indian Deccan plateau, irrigated farming areas in Australia, Central Asia with Iraq, Iran, and the Aral Sea Basin (Raiesi and Kabiri, 2016), Nile and Niger valley in Egypt and Africa (Abdel-Fattah et al., 2020), and the irrigated basin of Argentina (Sione et al., 2017) showed the deposition of Na salts and prognosis of sodication (Figure 1). Sodic soil remains dispersed and deflocculated because of failure in aggregation. Clogging of pore space restricts air entry and lowers hydraulic conductivity (Bennett et al., 2019). Presence of Na+ and CO32–/HCO3− and poor microbial activity under alkaline soil pH conditions limits carbon supply and promotes its loss because of increased organic matter mobility (Datta et al., 2019; Basak et al., 2021a Deb et al., 2020). The precipitation of Ca2+ as amorphous CaCO3 aggravated the Na+-induced toxicity and nutritional deficiency of Ca2+ (Rai et al., 2021b). The disintegration of rocks is the primary source of CaCO3 in the soil having poor aeration. The soluble Ca2+ and Mg2+ in the soil–water environment supplied from biochemical weathering and exchange process supply and prolonged water trajectory make sure the system is saturated with Ca2+/Mg2+ upon HCO3− (Jobbágy et al., 2017). Contrarily, in short trajectories, the chance of HCO3− saturation may arise because of chemical weathering of calcareous rocks. Moreover, Na+ selectivity in the sodic environment reinstates Ca in the solution and results in precipitation of CaCO3. In an extreme alkaline condition (pH ∼12.0), the hydrolysis of Na2CO3 (Bajwa and Swarup, 2012) and precipitation out of Ca2+ in CaCO3 favor the existence of OH− in soil–water solution. Therefore, a rise in the soil pH in calcareous sodic soils than in non-calcareous sodic soil is rational because of the spontaneous release of OH−. In one way, the presence of exchangeable Na and in another way the nonexistence of a good quantity of neutral soluble salts lead to development of extremely higher pH (Basak et al., 2015).
FIGURE 1. Salinity and sodicity: problem and threat (adopted from Falloon and Betts, 2010; Raiesi and Kabiri, 2016; Rengasamy, 2016; Liu et al., 2017; Sione et al., 2017; Mandal U. K. et al., 2019; Neubauer et al., 2019; Abdel-Fattah et al., 2020; Hayat et al., 2020; Mahajan et al., 2021a).
A significant land area (∼932.2 Mha) of the Earth is affected with salinity (Rengasamy, 2006). The faulty irrigation practices further aggravated this problem, and 34.2 Mha of total irrigated area is affected by salinity (Mateo-Sagasta and Burke, 2011; Aquastat, 2016). Because of the dynamic nature of soil salinity/sodicity, more than hundred countries are affected by SAS distributed on all the continents. Central and southeastern Asian countries (China, India, Pakistan, Iran, and Iraq) and other Western countries (United States), a major part of Australia, Argentina, and some areas of Brazil from the Southern Hemisphere; Italy and Spain from Europe are the main spots of global soil salinization (Ghassemi et al., 1995; Aquastat, 2016). Soil salinization is extensively reported in some important river basins, namely, Aral Sea Basin in Central Asia, Indo-Gangetic Basin in India, Indus Basin in Pakistan, Euphrates Basin in Syria and Iraq, Yellow River Basin in China, San Joaquin Valley in California, and Murray–Darling Basin in Australia, and these are reported to have severe problems of salinization (Chang and Silva, 2014; Qadir et al., 2014). Salinity- and sodicity-induced land degradation and faulty agricultural practices promote the buildup of salinity and increase sodification and reduce crop productivity. Salinization dents nearly of US$ 31 million annual loss in agricultural productivity (FAO, 2015). The yield losses of major agricultural crops, namely, wheat, rice, sugarcane, and cotton are about 40, 45, 48, and 63 percent on SAS in the Indo-Gangetic Plain of India, respectively (Sharma et al., 2015). These losses in wheat and rice crops are 20–43 and 36–69 percent from SAS in the Indus Basin of Pakistan, respectively (Murtaza, 2013). Furthermore, in the United States, Egypt, Uzbekistan, and Turkmenistan, the crop yield losses are 10, 30, 40, and 40 percent, respectively (Pitman et al., 2004). The monetary loss of agricultural production in India and Australia are ∼2.0 and 1.3 billion US$, respectively. Long-time irrigation with high alkali water resulted in the build-up of sodicity with 14–16% loss in the grain yield of wheat and rice (Sheoran et al., 2021). The effect of salinity and presence of exchangeable Mg cause a yield loss of cotton in the Aral Sea Basin of southern Kazakhstan (Vyshpolsky et al., 2010). Depending on the type of land degradations and their intensity, the cultivated crop/cropping system followed, irrigation water quality, extension and congestion of the irrigation/drainage network, and available amendment use/management options available, and the saline areas of Kazakhstan, India, and Pakistan reported a wide variation in yield losses from 6 to 71, 40–63, and 36–69%, respectively. Because of inflation adjustment, the cost of salt-induced land degradation was US$ 441 ha−1 in 2013 (Qadir et al., 2014), and the aggregated total annual economic loss was US$ 30 billion (Shahid et al., 2018) at a global scale. Both yield penalty and need for additional investment of higher input multifaceted the economic costs for salt-affected lands. The crop yield losses are particularly detrimental at the farm level but often underestimated at the macro level (Mandal et al., 2018). India loses annually around 16.8 Mt of agricultural production because of salinity and associative constraints (Mandal S. et al., 2019). Five percent of the Earth’s land area is salt-affected, and 75% of the cultivable area under irrigated agriculture is affected by salinity (Hopmans et al., 2021). For feeding the nine billion people by 2050, it is anticipated that crop production can be met by greening SAS soil with appropriate agro-techniques.
Plants face primarily the osmotic stress when exposed to salinity (electrical conductivity, ECe) and suboptimal soil water potential. Under these conditions, plants generate different osmolytes such as proline, simple carbohydrates, polyols, amino acids, and quaternary ammonium compounds such as betaine and glycine to increase the osmotic balance at the cytoplasmic scale and try to maintain cell turgidity (Seleiman et al., 2022). In this way, the plant metabolic activities are sustained, and their growth and productivity are maintained. By accumulating the simple and soluble carbohydrates, plants enhance their osmotic potential and alleviate osmotic stress (Sharp et al., 1990). In addition to water stress, roots also experienced toxicity of Na+ in severe salinity; therefore, plants need acclimatization under such conditions. When Na+ is absorbed by roots, the plants can either exclude Na+ from the cytoplasm or move it to the inactive metabolic sites such as vacuoles. The K+/Na+ antiporter present at the vacuolar surface plays a major role in separating Na+ and consequently lowering the Na+ concentration in the cytoplasm (Liang et al., 2018). The tolerant plants generate an antioxidant mechanism by biosynthesis of various enzymes, namely, superoxide dismutase and catalase. Several reports proved that the antioxidant guard mechanism copes with the oxidative damage during salinity stress in crops (Noctor and Foyer, 1998).
Huge spatial and temporal variations of soil are a challenge for estimating some soil properties of SAS for soil quality assessment. Alkalinity usually affect soil physical properties with poor soil water permeability. Massive soil structure and hard crust on the surface layer hinder seedling emergence and poor plant establishment. Greater osmotic potential of saline soils because of the abundance of soluble and quasi-soluble electrolytes largely affect soil–plant water relation, and plants face severe water stress due to physiological unavailability of water (Wong et al., 2010). Impaired biogeochemical cycling of essential nutrients, namely, excess losses of N, mysterious behavior of soil P (Sundha et al., 2017), and an antagonism relation between Cl− and H2PO4−, Cl− and NO3−, Cl−, and SO42– and Na+ and K+ (Rai et al., 2021b; Sundha et al., 2022) are the major issues associated with the nutrition of plant under SAS. Furthermore, increased ionic strength also affects nutrient elements present on soil colloids. Deficiency of Ca and excess of Na affect K nutrition; the toxic appearance of HCO3− and CO32– in alkali soil also decreases the solubility and availability of Zn and Fe. Many sodic areas also exhibit severe soil erosion, which promotes the loss of soil organic matter (Wong et al., 2010; Datta et al., 2019; Deb et al., 2021; Basak et al., 2021b). The extended periods of submergence because of poor soil permeability also affect soil biological properties (Wong et al., 2010). The researcher mostly specified most of the time to analyze physical features and climatic factors associated with specific salt-affected soils. Therefore, special attention is needed while selecting analytical techniques (Table 1) for estimating physical, chemical, and biological attributes of these soils (USSL, 1954; Bhargava, 2003; Basak, 2014).
TABLE 1. Critical issues associated with quality data generation at a usual soil science laboratory for analyses of parameters of salt-affected soil.
Soil quality is measured for making an inventory of the health status of soils and assessing the impacts of human perturbations on them. Such assessment should be carried out with respect to undisturbed pristine or virgin sites. However, it is difficult to find such sites (as a reference level) for comparison to capture possible degradation or aggradation caused by anthropogenic perturbation. Therefore, a relative assessment of the quality of soils with varying anthropogenic stresses is performed for screening and subsequent adoption of less/non-damaging types for upkeeping health (Basak et al., 2021a). Screening of the indicator constitutes an important part of soil quality assessment. Indicators are of two types—one, inherent indicators—these are native and quasi-permanent and undergo little changes; and the other, dynamic indicators—these reflect/capture the signatures of perturbations to which soils are subjected. They are selected based on several principles, as indicated in Figure 2 (Mandal et al., 2016).
FIGURE 2. Criteria for soil quality indicator selection modified from Mandal et al. (2016)
After generating the database on identified indicators for soils subjected to different management practices, a critical statement needs to be made as to the aggrading or degrading influence of the practices on their (soils) quality (Masto et al., 2008b). Often, it is difficult to assess the soil quality using individual indicators, unless the databases are judged for their influence on the functional goal of soil. Accordingly, the databases are screened through several parametric and nonparametric statistical tests based on their influence on goal variables to formulate a minimum dataset (MDS) of indicators (Masto et al., 2008b). Subsequently, the databases for different indicators screened are validated with goal variables or with expected functions through statistical tools, namely, simple correlation, multiple regression, principal component analysis (PCA), and discriminant analysis (DA) to indicate their (indicators) representation in the variability of goal functions. The whole process of the formulation of MDS has elegantly been described by earlier researchers (Andrews and Carroll, 2001; Andrews et al., 2004; Basak et al., 2016) (Figure 3). Many researchers in India also screened out master indicators for different soil types and cropping systems for assessment of soil health as a function of biological productivity/sustainable yield only (Table 2); few of them have assessed soil quality using management goals, namely, productivity, environmental protection, specify targeted soil threats, and/or ecosystem services by capturing identified distinguishing minimum datasets of indicators (Bhaduri et al., 2014; Bhaduri and Purakayastha, 2014; Bünemann et al., 2018). These indicators varied significantly even within a soil type or production system. Similarly, variation was apparent in screened master indicators for salt-affected soil across the globe (Table 2). Therefore, the selection of methods for measuring indicators assumes significance to minimize the variation within a soil type or production system and measure soil functionality and their integration to capture informative soil quality indices (Lehmann et al., 2020). A few desirable features of methods for measuring indicators are 1) high throughput, 2) ease of use, 3) storage, 4) potential reference material, 5) achievability, 6) deployment status, 7) sample collection, 8) international comparisons, 9) how much soil, 10) infrastructure, 11) cost—hardware/labor, and 12) multi-parametric in nature among the screened indicators even for a similar production system raised at different geographical sites (Mandal et al., 2016). One of the major tasks for researchers is to work on finding commonality in indicators for different soil types and production systems. This will facilitate development of easy and inexpensive methods for common master indicators and their inclusion in routine analysis in soil testing laboratories for assessment of soil health. Studies assessing the quality of soils to meet other ecosystem services have hardly been conducted in India, although its role and capability for providing water security (NO3, As, or F pollution), waste recycling, biodiversity, environmental protection, and climate change abatement are in urgent demand for assessment. A modest beginning can be made with the existing long-term experiments where along with biological productivity, biological diversity, carbon budgeting, and crop quality may be included as goal variables (Bhaduri et al., 2014).
FIGURE 3. Schematic diagram showing all the processes, namely, indicator selection, minimum dataset formation, interpretation, and integration involved for calculation of soil quality index (modified from Andrews et al., 2004)
TABLE 2. Soil quality assessment in salt-affected soils; figures in parenthesis are the number of attributes used.
Screened soil quality indicators for a production system highlighting the aggradation or degradation effect through multidimensional trends (positive, negative, or no change) and intensity (degree) are converted into an index. These indicators are combined together (Figure 3) into a combinable index (soil quality index) for getting a unique value for describing aggradations or degradation in soils owing to specific management practices. To combine the indicators, sometimes different weights are given to them based on personal judgment or values of the coefficients of determinations of multiple regression, principal component analysis (PCA), and discriminant analysis (DA) associated with those indicators during screening through different statistical methods in the form of a weighted additive or simple additive (Andrews and Carroll, 2001; de Lima et al., 2008; Mukherjee and Lal, 2014). The soil quality index (SQI) is developed for soils under different management practices and cropping systems in a large number of long-term fertility experiments in India by several researchers (Basak et al., 2021a; Figure 4). Recently, Soni et al. (2021) assessed the SQI for saline soils under tillage, mulching, and deficit saline water irrigation.
Sodic soil reclamation was initiated by applying inorganic substances, namely, gypsum, phosphogypsum, fly ash, inorganic sulfur, sulfuric acids, pyrites, aluminum chloride, sugar industry byproduct press mud and acid formers, and bioaugmented substances with gypsum (CSSRI, 2006; Hafez et al., 2015; Rai et al., 2021a). If irrigation water is safe for soil and crops, then soil sodicity reclamation is principally a single-time investment for sustaining production (Rai et al., 2021b). Usually, 3–4 years are required after the adoption of reclamation technology for rice-based cropping systems to reach their productivity potential because of the gradual replacement of a large amount of Na+ from soil colloids by Ca2+ during the progress of reclamation (Abrol and Bhumbla, 1979; Zhang et al., 2021). When sodicity is a consequence of water-born alkalinity, then recurring applications of amendments are advocated to overcome water-born sodicity (residual alkalinity) and maintain soil quality and sustainable crop yield (Singh et al. 2019; Sheoran et al., 2021). The application of organic materials such as city waste compost, gypsum enrich compost, sulfur-rich compost, and the byproduct of sugarcane industry press mud in conjunction with gypsum improves crop yield by decreasing soil pH and ESP because of decrease in the precipitation of Ca and greater removal of Na in drainage waters (Grigg et al., 2006; Choudhary et al., 2011; Sundha et al., 2020). Calcareous sodic soils are recommended for reclamation through the application of elemental sulfur (Ganjegunte et al., 2018). On-farm and participatory research experiments conducted in the CSSRI and other national laboratories reported improvement in soil quality upon reclamation (Supplementary Table S1). The rice–wheat system in the sodic area (pH1:2 8.7) of western India maintained optimum yield by addition of 100 and 150 percent of the recommended doses of fertilizer (RDF) with necessary improvement in pH1:2, EC1:2, SOC, Olsen’s P, NH4OAc extractable K, and DTPA extractable Zn and Mn (Swarup and Yaduvanshi, 2000). Application of N @120 and P @ 26 kg ha−1 + FYM/press mud/gypsum improved SOC and sustained wheat yield in sodic soil (pH1:2) when irrigated with sodic water (RSC 8.5 me L−1; SAR of 8.8) in sodic areas of northwestern India (Yaduvanshi and Sharma 2008; Yaduvanshi and Swarup, 2005). Reclamation of soil declined soil pH1:2 and SARe and improved SOC, and increased the uptake of KMnO4–N, Olsen’s P, and NH4OAc extractable K. The results from the participatory experiments carried out at 10 locations in the central Indo-Gangetic plain showed a reduced level of sodicity (as decrement in ESP, SARe, and soil pH1:2 and EC1:2Singh) and increased SOC and improved soil porosity, void ratio, and saturated hydraulic conductivity because of green manuring in the rice–wheat cropping system (Singh et al., 2014), although the phosphogypsum (PG) application is questionable because of the presence of heavy metals (Mishra et al., 2019). However, the application of PG was associated with declined soil pH1:2, EC1:2, ESP, and SARe and improved soil fertility (increased Olsen’s P, NH4OAc extractable K, and DTPA extractable Fe, Mn, Zn, Cu etc.), soil physical quality (reducing water-dispersible clay and bulk density; and improving infiltration, aggregate ratio, mean weight diameter and aggregate associated C), and improved rice yield (Nayak et al., 2013). Recently, Yu et al. (2014) claimed the neutralization of soil sodicity and improvement of soil physical properties such as an increase in hydraulic conductivity and soil porosity reduce by the application of flue gas desulphurization gypsum (FGDG). Acidified biochar produced from rice straw and dicer wood chips facilitate the losses of Na and reduce the EC and SARe (Sadegh-Zadeh et al., 2018).
Reduce tillage, deficit irrigation, mulching, adoption of low water requiring crops, and cultivation of salt-tolerant varieties for water-saving and minimizing salt load in applied irrigation events are strategies for productive utilization of saline soils (Singh, 2009; Li et al., 2019; Seleiman et al., 2019). Deficit irrigation (DI) with available saline water is adopted to check frequent drought and sustain crop production (Jiang et al., 2012; Nagaz et al., 2012). Paddy straw mulch and efficient water management had the potential for restoring soil health, checking evaporation, and improving crop productivity (Purakayastha et al., 2019; Soni et al., 2021) (Supplementary Table S2). These practices permit water to move more quickly into and through the soil profile, increasing salt leaching and inhibiting salt build-up in surface soil (Grigg et al., 2006; Yuan et al., 2019; Zhang et al., 2021). Therefore, the DI and mulching synergized with the climate and soil of a geographical area can be a nature-based solution working within the limits of the existing natural resources (Visser et al., 2019; Soni et al., 2021). Zero tillage is also effective in increasing soil fertility, building soil organic carbon, restoring soil structure, and leading to a reduction in soil evaporation and salinity (Wang et al., 2014). Several researchers report that salinity has an undesirable effect on soil microbial biomass C, N, and basal soil respiration (BSR), whereas it has a positive effect on metabolic quotient (qCO2) (Tripathi et al., 2006; Egamberdieva et al., 2011; Iwai et al., 2012; Mahajan et al., 2016). Salinity-induced stress might have reduced the positive respiratory activities of the microorganisms (Tripathi et al., 2007; Iwai et al., 2012). The toxic effect of the dominant cation Na+ slows down the growth of soil microorganisms and MBC rather than the C input (Rietz and Haynes, 2003; Egamberdieva et al., 2011; Mavi and Marschner, 2013). In general, larger soil enzyme activities were noted with low electrolyte concentration and vice versa. Organic amendments in association with microflora increase mineralization, with an associated increase in CO2 release and, consequently, soil aeration, apparently due to increased enzymatic activities. Such an increase in mineralization improves the soil fertility and crop productivity in saline soil. Leaching accomplished by ponding water in the well-leveled field with good quality water (annual rain, river, or underground) is the reasonable choice to remove excess salts and electrolytes in soils below the root zone in the soil profile. The amount of salts leached from soils depends on the amount and quality of irrigation water and soil texture. Surface flushing with water is advocated to wash surface-deposited salts (Nayak et al., 2008). This practice is recommended for soil with low permeability, and soil is prone to crust formation. Scarping of loaded salts is followed to manage marginal landholding affected by salinity; however, recurring removal of salts is recommended to attain desired change in soil salinity for productive use. Proper leveling, zero (minimum) tillage, mulching, conjunctive use of saline water in cyclic or mixing mode with good quality water, light and frequent application of saline irrigation for reduction of cumulative water deficit, irrigating with best available water at sensitive crop stages (germination and seeding emerging stages), pre-sowing irrigation for Kharif (summer) crop, improving water use efficiency practice by pressurized drip/sprinkler irrigation which facilitates the washing of root zone salinity, and sustaining crop production are the promising options for fruitful utilization of SAS and saline water (Soni et al., 2021; Garg et al., 2022; Rai et al., 2022). Exogenous application of plant growth regulators (salicylic acid, thio-urea, and potassium nitrate) alleviated sodicity stress of both soil and water-born sodicity by triggering physiological parameters of the rice (Singh et al., 2022). The use of bio-drainage is advocated to manage waterlogged saline soils by physiological transpiration of water (Dagar et al., 2016).
Underground or surface drainage is a long-term solution for lowering the water table and leaching of salts and providing a favorable salt balance in surface soil. A perforated corrugated PVC pipe protected with synthetic filter consistently established in the proper plan below the rooting depth to reduce poor quality water table and remove excess salts and water by gravitational action or pressurized pump. Ingress of brackish water and seawater tides can be checked by building tall and well-made earthen banks. Building of pond and water harvesting unit conserves seasonal rain and utilization of it for irrigation of dry season crops and leaching of salts. The farm pond technology delivers the scope for crop diversification, round-the-year cropping, and integrated farming because of improved irrigation facilities, checking of salinity, and improved drainage conditions (Chinchmalatpure et al., 2015).
Soil salinity and sodicity are the major issues impacting crop production on ∼1 billion hectares of land distributed in arid and semi-arid regions worldwide. These soils are broadly categorized into saline, sodic, and saline–sodic depending upon the dominance of soluble (chloride/sulfate) and/or alkaline salts (carbonate/bicarbonates) of Na, K, Ca, and Mg. The disproportionate presences of salts in these soils reduce the choice of the crop and incur the annual productivity loss equivalent to 31 million US dollars. Application of Ca-bearing salts, pyrites, and other organic amendments and engineering and agronomic approaches are successful in the rehabilitation of these soils. The success of rehabilitation of SAS can be further enhanced by selecting crop varieties capable of withstanding osmotic stress, water deficit, and toxicities of specific ions. The soil quality indices developed for different stages of the rehabilitation of salt-affected soils act as a tool for monitoring the real-time progress of the management process adopted in these soils. The screening of the inherent and dynamic indicators capturing the signature of the soil perturbations constitutes an important part of soil quality assessment. Different studies had identified the bulk density, hydraulic conductivity, pH, electrical conductivity, exchangeable sodium percent, microbial biomass C, and soil enzymes, which are the master indicators for soil quality assessment. The spatial and temporal variations of salt-affected areas are the major problems for the selection of master indicators of soil. A developed soil quality index for the SAS soil will enable the functionaries to assess the real-time impact of management options for different soil functions. It also enables the farmers to select site-specific and goal-specific management plans for improved resource use efficiency, reduced environmental impact, increased productivity, and farm economy. The soil quality–based management plan can be a tool for policy planners for monitoring the progress of land reclamation, soil health management, and environmental protection. Recent development in material science has the potential to develop some formulations such as polymers, nano-materials, acidified biochars, elemental sulfur, sulfuric acid generator, and flue-gas-desulphurization gypsum (FGDG), having the potential for replacing gypsum as an amendment. In the future, scientifically managed city waste compost can also supplement the sodic soil reclamation program. Moreover, future application of these alternatives for sustainable and profitable use in salt-affected soils would not be practicable unless efficacy and efficiency in sodic soil reclamation are established and low-cost methods for the production are not devised.
NB: conceptualization, framework development, and preparation of the manuscript; AKR: conceptualization, framework development, and preparation of the draft; PS: collection and organization of the database, preparation of the manuscript; RM: collection and organization of the database, preparation of the manuscript; SB: collection and organization of the database, review and editing; RKY: resources, review, and editing; PCS: resources, review, and editing.
This work was supported by the Indian Council of Agricultural Research, New Delhi (India).
This work is a part of the CSSRI project “Developing soil quality indices for sodic soil under different reclamation strategies (NRMACSSRISIL201801700969)”. The authors thank the Director, CSSRI, Karnal (Haryana) for the financial support.
The authors declare that the research was conducted in the absence of any commercial or financial relationships that could be construed as a potential conflict of interest.
All claims expressed in this article are solely those of the authors and do not necessarily represent those of their affiliated organizations, or those of the publisher, the editors, and the reviewers. Any product that may be evaluated in this article, or claim that may be made by its manufacturer, is not guaranteed or endorsed by the publisher.
The Supplementary Material for this article can be found online at: https://www.frontiersin.org/articles/10.3389/fenvs.2022.935785/full#supplementary-material
Abdel-Fattah, M. K., Abd-Elmabod, S. K., Aldosari, A. A., Elrys, A. S., and Mohamed, E. S. (2020). Multivariate analysis for assessing irrigation water quality: A case study of the bahr mouise canal, eastern nile delta. Water 12, 2537. doi:10.3390/w12092537
Abrol, I. P., and Bhumbla, D. R. (1979). Crop responses to differential gypsum applications in a highly sodic soil and the tolerance of several crops to exchangeable sodium under field conditions. Soil Sci. 127, 79–85. doi:10.1097/00010694-197902000-00004
Abrol, I. P., Yadav, J. S. P., and Massoud, F. I. (1998). Salt-affected soils and their management. FAO: Rome, Italy.
Al-Suhaibani, N., Seleiman, M. F., El-Hendawy, S., Abdella, K., Alotaibi, M., Alderfasi, A., et al. (2021). Integrative effects of treated wastewater and synthetic fertilizers on productivity, energy characteristics, and elements uptake of potential energy crops in an arid agro-ecosystem. Agronomy 11, 2250. doi:10.3390/agronomy11112250
Ali, A. M. S. (2006). Rice to shrimp: Land use/land cover changes and soil degradation in Southwestern Bangladesh. Land Use Policy 23, 421–435. doi:10.1016/j.landusepol.2005.02.001
Alkharabsheh, H. M., Seleiman, M. F., Hewedy, O. A., Battaglia, M. L., Jalal, R. S., Alhammad, B. A., et al. (2021). Field crop responses and management strategies to mitigate soil salinity in modern agriculture: A review. Agronomy 11, 2299. doi:10.3390/agronomy11112299
Andrews, S. S., and Carroll, C. R. (2001). Designing a soil quality assessment tool for sustainable agroecosystem management. Ecol. Appl. 11, 1573–1585. doi:10.1890/1051-0761(2001)011[1573:DASQAT]2.0.CO;2
Andrews, S. S., Karlen, D. L., and Cambardella, C. A. (2004). The soil management assessment framework. Soil Sci. Soc. Am. J. 68, 1945–1962. doi:10.2136/sssaj2004.1945
Aquastat (2016). FAO’s information system on water and agriculture. Rome, Italy: Food and Agricultural Organization (FAO).
Badawy, S. A., Zayed, B. A., Bassiouni, S. M. A., Mahdi, A. H. A., Majrashi, A., Ali, E. F., et al. (2021). Influence of nano silicon and nano selenium on root characters, growth, ion selectivity, yield, and yield components of Rice (Oryza sativa L.) under salinity conditions, Plants (Basel). Plants 10, 1657. doi:10.3390/plants10081657
Bajwa, M. S., and Swarup, A. (2012). “Soil salinity and alkalinity,” in Fundamentals of soil science. N. N. Goswami, R. K. Rattan, G. Dev, G. Narayansamy, and D. K. Das. New Delhi, 329–339.
Barman, A., Sheoran, P., Yadav, R. K., Abhishek, R., Sharma, R., Prajapat, K., et al. (2021). Soil spatial variability characterization: Delineating index-based management zones in salt-affected agroecosystem of India. J. Environ. Manag. 296, 113243. doi:10.1016/j.jenvman.2021.113243
Basak, N., Chaudhuri, S. K., and Sharma, D. K. (2015). Influence of water quality on exchange phase-solution phase behavior of texturally different salt-affected soils. J. Indian Socie. Soil Scie. 64, 365. doi:10.5958/0974-0228.2015.00048.1
Basak, N., Datta, A., Mitran, T., Roy, S. S., Saha, B., Biswas, S., et al. (2016). Assessing soil-quality indices for subtropical rice-based cropping systems in India. Soil Res. 54, 20. doi:10.1071/SR14245
Basak, N., Mandal, B., Rai, A. K., and Basak, P. (2021a). Soil quality and productivity improvement: Indian story. Proc. Indian Natl. Sci. Acad. 87, 2–10. doi:10.1007/s43538-021-00007-8
Basak, N., Sheoran, P., Sharma, R., Yadav, R. K., Singh, R. K., Kumar, S., et al. (2021b). Gypsum and pressmud amelioration improve soil organic carbon storage and stability in sodic agroecosystems. Land Degrad. Dev. 32, 4430–4444. doi:10.1002/ldr.4047
Bennett, J. M., Marchuk, A., Marchuk, S., and Raine, S. R. (2019). Towards predicting the soil-specific threshold electrolyte concentration of soil as a reduction in saturated hydraulic conductivity: The role of clay net negative charge. Geoderma 337, 122–131. doi:10.1016/j.geoderma.2018.08.030
Bhaduri, D., and Purakayastha, T. J. (2014). Long-term tillage, water and nutrient management in rice–wheat cropping system: Assessment and response of soil quality. Soil Tillage Res. 144, 83–95. doi:10.1016/j.still.2014.07.007
Bhaduri, D., Purakayastha, T. J., Patra, A. K., and Chakraborty, D. (2014). Evaluating soil quality under a long-term integrated tillage–water–nutrient experiment with intensive rice–wheat rotation in a semi-arid Inceptisol, India. Environ. Monit. Assess. 186, 2535–2547. doi:10.1007/s10661-013-3558-8
Bhargava, G. P. (2003). Training manual for undertaking studies on Genesis of sodic/alkali soils. Karnal 132001: Central Soil salinity Resarch Institute.
Blankespoor, B., Dasgupta, S., and Lange, G.-M. (2017). Mangroves as a protection from storm surges in a changing climate. Ambio 46, 478–491. doi:10.1007/s13280-016-0838-x
Brammer, H. (2014). Bangladesh’s dynamic coastal regions and sea-level rise. Clim. Risk Manag. 1, 51–62. doi:10.1016/j.crm.2013.10.001
Bünemann, E. K., Bongiorno, G., Bai, Z., Creamer, R. E., De Deyn, G., de Goede, R., et al. (2018). Soil quality – a critical review. Soil Biol. Biochem. 120, 105–125. doi:10.1016/j.soilbio.2018.01.030
Cai, X., Sharma, B. R., Matin, M. A., Sharma, D., and Gunasinghe, S. (2010). An assessment of crop water productivity in the Indus and ganges river basins: Current status and scope for Improvement 140. IMWI Research Report. Columbo, Srilanka.
Chang, A. C., and Silva, D. B. (2014). “Prologue BT - salinity and drainage in san Joaquin Valley, California: Science, technology, and policy,” in, eds. A. C. Chang, and D. Brawer Silva (Dordrecht: Springer Netherlands), 1–6. doi:10.1007/978-94-007-6851-2_1
Chinchmalatpure, A. R., Ali, S., Kulshrestra, N., Singh, R. K., Bundela, D. S., Kumar, P., et al. (2015). Intellectual property management and commercialization of ICAR-CSSRI technologies for management of salt-affected and waterlogged soils of India. Haryana: ICAR-Central Soil Salinity Research Institute.
Choudhary, O. P., Ghuman, B. S., Bijay-Singh, , Thuy, N., and Buresh, R. J. (2011). Effects of long-term use of sodic water irrigation, amendments and crop residues on soil properties and crop yields in rice–wheat cropping system in a calcareous soil. Field Crops Res. 121, 363–372. doi:10.1016/j.fcr.2011.01.004
CSSRI (2006). CSSRI: A journey to excellence (1969-2006). Karnal 132 001, India: Central Soil Salinity Research Institute.
Dagar, J. C., Lal, K., Ram, J., Kumar, M., Chaudhari, S. K., Yadav, R. K., et al. (2016). Eucalyptus geometry in agroforestry on waterlogged saline soils influences plant and soil traits in North-West India. Agric. Ecosyst. Environ. 233, 33–42. doi:10.1016/j.agee.2016.08.025
Dasgupta, S., Hossain, M. M., Huq, M., and Wheeler, D. (2015). Climate change and soil salinity: The case of coastal Bangladesh. Ambio 44, 815–826. doi:10.1007/s13280-015-0681-5
Datta, A., Setia, R., Barman, A., Guo, Y., and Basak, N. (2019). “Carbon dynamics in salt-affected soils,” in Research developments in saline agriculture. Editors J. Dagar, and R. Yadav (Singapore: Springer).
de Lima, A. C. R., Hoogmoed, W., and Brussaard, L. (2008). Soil quality assessment in rice production systems: Establishing a minimum data set. J. Environ. Qual. 37, 623–630. doi:10.2134/jeq2006.0280
Deb, S., Mandal, B., and Bhadoria, P. B. S. (2020). Influence of sea water ingression on carbon sequestration in soils under coastal agro-ecosystems of eastern India. Agric. Res. 9, 622–630. doi:10.1007/s40003-020-00456-5
Ding, Z., Kheir, A. M. S., Ali, O. A. M., Hafez, E. M., ElShamey, E. A., Zhou, Z., et al. (2021). A vermicompost and deep tillage system to improve saline-sodic soil quality and wheat productivity. J. Environ. Manag. 277, 111388. doi:10.1016/j.jenvman.2020.111388
Egamberdieva, D., Kucharova, Z., Davranov, K., Berg, G., Makarova, N., Azarova, T., et al. (2011). Bacteria able to control foot and root rot and to promote growth of cucumber in salinated soils. Biol. Fertil. Soils 47, 197–205. doi:10.1007/s00374-010-0523-3
Eswar, D., Karuppusamy, R., and Chellamuthu, S. (2021). Drivers of soil salinity and their correlation with climate change. Curr. Opin. Environ. Sustain. 50, 310–318. doi:10.1016/j.cosust.2020.10.015
Falloon, P., and Betts, R. (2010). Climate impacts on European agriculture and water management in the context of adaptation and mitigation—the importance of an integrated approach. Sci. Total Environ. 408, 5667–5687. doi:10.1016/j.scitotenv.2009.05.002
FAO, I. (2015). Status of the world’s soil resources – main report. Rome, Italy: Food and Agriculture Organization of the United Nations and Intergovernmental Technical Panel on Soils.
Fishman, R. (2018). Groundwater depletion limits the scope for adaptation to increased rainfall variability in India. Clim. Change 147, 195–209. doi:10.1007/s10584-018-2146-x
Ganjegunte, G. K., Clark, J. A., Parajulee, M. N., Enciso, J., and Kumar, S. (2018). Salinity management in pima cotton fields using sulfur burner. Agrosyst. Geosci. Environ. 1, 1–10. doi:10.2134/age2018.04.0006
Garg, N., Choudhary, O. P., Thaman, S., Sharma, V., Singh, H., Vashistha, M., et al. (2022). Effects of irrigation water quality and NPK-fertigation levels on plant growth, yield and tuber size of potatoes in a sandy loam alluvial soil of semi-arid region of Indian Punjab. Agric. Water Manag. 266, 107604. doi:10.1016/j.agwat.2022.107604
Ghassemi, F., Jakeman, A. J., and Nix, H. A. (1995). Stalinization of land and water resources: Human causes, extent, management and case studies. Wallingford, UK: CABI.
Grigg, A. H., Sheridan, G. J., Pearce, A. B., and Mulligan, D. R. (2006). The effect of organic mulch amendments on the physical and chemical properties and revegetation success of a saline-sodic minespoil from central Queensland, Australia. Soil Res. 44, 97. doi:10.1071/sr05047
Gupta, R. N., and Mathur, A. (2011). “Origin, distribution and classificatinof the salt-affeted soils of India,” in Bulletin of the Indian society of soil science. Editors R. Rattan, and A. K. Singh (New Delhi, India: Indian Society of Soil Science), 1–28.
Hafez, E. M., Hassan, W. H. A. E., Gaafar, I. A., and Seleiman, M. F. (2015). Effect of gypsum application and irrigation intervals on clay saline-sodic soil characterization, rice water use efficiency, growth, and yield. J. Agric. Sci. (Tor). 7, 208. doi:10.5539/jas.v7n12p208
Hansen, J. A., Jurgens, B. C., and Fram, M. S. (2018). Quantifying anthropogenic contributions to century-scale groundwater salinity changes, San Joaquin Valley, California, USA. Sci. Total Environ. 642, 125–136. doi:10.1016/j.scitotenv.2018.05.333
Hayat, K., Bundschuh, J., Jan, F., Menhas, S., Hayat, S., Haq, F., et al. (2020). Combating soil salinity with combining saline agriculture and phytomanagement with salt-accumulating plants. Crit. Rev. Environ. Sci. Technol. 50, 1085–1115. doi:10.1080/10643389.2019.1646087
Hopmans, J. W., Qureshi, A. S., Kisekka, I., Munns, R., Grattan, S. R., Rengasamy, P., et al. (2021). “Chapter One - critical knowledge gaps and research priorities in global soil salinity,” in, ed. A. Sparks (Academic Press), 1–191. doi:10.1016/bs.agron.2021.03.001
Iwai, C. B., Oo, A. N., and Topark-ngarm, B. (2012). Soil property and microbial activity in natural salt affected soils in an alternating wet–dry tropical climate. Geoderma 190, 144–152. doi:10.1016/j.geoderma.2012.05.001
Jiang, J., Huo, Z., Feng, S., and Zhang, C. (2012). Effect of irrigation amount and water salinity on water consumption and water productivity of spring wheat in Northwest China. Field Crops Res. 137, 78–88. doi:10.1016/j.fcr.2012.08.019
Jobbágy, E. G., and Jackson, R. B. (2004). Groundwater use and salinization with grassland afforestation. Glob. Chang. Biol. 10, 1299–1312. doi:10.1111/j.1365-2486.2004.00806.x
Jobbágy, E. G., Tóth, T., Nosetto, M. D., and Earman, S. (2017). On the fundamental causes of high environmental alkalinity (ph ≥ 9): An assessment of its drivers and global distribution. Land Degrad. Dev. 28, 1973–1981. doi:10.1002/ldr.2718
Kamra, S. K. (2021). “Subsurface drainage for saline and waterlogged alluvial soils,” in Managing salt affected soils for sustainable agricultue. Editors P. S. Minhas, R. K. Yadav, and P. C. Sharma (New Delhi, India: Indian Council of Agricultural Research ICAR), 346–366.
Karlen, D. L., Mausbach, M. J., Doran, J. W., Cline, R. G., Harris, R. F., Schuman, G. E., et al. (1997). Soil quality: A concept, definition, and framework for evaluation (A guest editorial). Soil Sci. Soc. Am. J. 61, 4–10. doi:10.2136/sssaj1997.03615995006100010001x
Lehmann, J., Bossio, D. A., Kögel-Knabner, I., and Rillig, M. C. (2020). The concept and future prospects of soil health. Nat. Rev. Earth Environ. 1, 544–553. doi:10.1038/s43017-020-0080-8
Li, J., Gao, Y., Zhang, X., Tian, P., Li, J., Tian, Y., et al. (2019). Comprehensive comparison of different saline water irrigation strategies for tomato production: Soil properties, plant growth, fruit yield and fruit quality. Agric. Water Manag. 213, 521–533. doi:10.1016/j.agwat.2018.11.003
Liang, W., Ma, X., Wan, P., and Liu, L. (2018). Plant salt-tolerance mechanism: A review. Biochem. Biophysical Res. Commun. 495, 286–291. doi:10.1016/j.bbrc.2017.11.043
Liu, X., Ren, Y., Gao, C., Yan, Z., and Li, Q. (2017). Compensation effect of winter wheat grain yield reduction under straw mulching in wide-precision planting in the North China Plain. Sci. Rep. 7, 213. doi:10.1038/s41598-017-00391-6
Mahajan, G. R., Das, B., Manivannan, S., Manjunath, B. L., Verma, R. R., Desai, S., et al. (2021a). Soil and water conservation measures improve soil carbon sequestration and soil quality under cashews. Int. J. Sediment Res. 36, 190–206. doi:10.1016/j.ijsrc.2020.07.009
Mahajan, G. R., Das, B., Morajkar, S., Desai, A., Murgaokar, D., Patel, K. P., et al. (2021b). Comparison of soil quality indexing methods for salt-affected soils of Indian coastal region. Environ. Earth Sci. 80, 725. doi:10.1007/s12665-021-09922-x
Mahajan, G. R., Manjunath, B. L., Latare, A. M., D’Souza, R., Vishwakarma, S., Singh, N. P., et al. (2016). Microbial and enzyme activities and carbon stock in unique coastal acid saline soils of Goa. Proc. Natl. Acad. Sci. India Sect. B. Biol. Sci. 86, 961–971. doi:10.1007/s40011-015-0552-7
Majumder, B., Mandal, B., Bandyopadhyay, P. K., Gangopadhyay, A., Mani, P. K., Kundu, A. L., et al. (2008). Organic amendments influence soil organic carbon pools and rice-wheat productivity. Soil Sci. Soc. Am. J. 72, 775–785. doi:10.2136/sssaj2006.0378
Mandal, B., Basak, N., Singha, R. S., and Biswas, S. (2016). “Soil health measurement techniques,” in Soil health: Concept (New Delhi: Status and Monitoringonitoring), 1–98.
Mandal, S., Mandal, U. K., Lama, T. D., Burman, D., and Sharma, P. C. (2019). Economic analysis of farm-level agricultural risks in coastal region of West Bengal in India. J. Soil Salin. Water Qual. 11, 269–279.
Mandal, U. K., Burman, D., Bhardwaj, A. K., Nayak, D. B., Samui, A., Mullick, S., et al. (2019). Waterlogging and coastal salinity management through land shaping and cropping intensification in climatically vulnerable Indian Sundarbans. Agric. Water Manag. 216, 12–26. doi:10.1016/j.agwat.2019.01.012
Mandal, S., Raju, R., Kumar, A., Kumar, P., and Sharma, P. C. (2018). Current status of research, technology response and policy needs of salt-affected soils in India -A review. J. Indian Soc. Coast. Agric. Res. 36, 40–53.
Martins, A. P., Denardin, L. G. de O., Borin, J. B. M., Carlos, F. S., Barros, T., Ozório, D. V. B., et al. (2017). Short-term impacts on soil-quality assessment in alternative land uses of traditional paddy fields in southern Brazil. Land Degrad. Dev. 28, 534–542. doi:10.1002/ldr.2640
Masto, R. E., Chhonkar, P. K., Purakayastha, T. J., Patra, A. K., and Singh, D. (2008a). Soil quality indices for evaluation of long-term land use and soil management practices in semi-arid sub-tropical India. Land Degrad. Dev. 19, 516–529. doi:10.1002/ldr.857
Masto, R. E., Chhonkar, P. K., Singh, D., and Patra, A. K. (2008b). Alternative soil quality indices for evaluating the effect of intensive cropping, fertilisation and manuring for 31 years in the semi-arid soils of India. Environ. Monit. Assess. 136, 419–435. doi:10.1007/s10661-007-9697-z
Mateo-Sagasta, J., and Burke, J. (2011). Agriculture and water quality interactions: A global overview” (solaw background thematic report-T R08. Rome, Italy: Food and Agricultural Organization (FAO).
Mavi, M. S., and Marschner, P. (2013). Salinity affects the response of soil microbial activity and biomass to addition of carbon and nitrogen. Soil Res. 51, 68. doi:10.1071/SR12191
Meena, M. D., Joshi, P. K., Narjary, B., Sheoran, P., Jat, H. S., Chinchmalatpure, A. R., et al. (2016). Effects of municipal solid waste compost, rice-straw compost and mineral fertilisers on biological and chemical properties of a saline soil and yields in a mustard–pearl millet cropping system. Soil Res. 54, 958. doi:10.1071/SR15342
Mishra, V. K., Jha, S. K., Damodaran, T., Singh, Y. P., Srivastava, S., Sharma, D. K., et al. (2019). Feasibility of coal combustion fly ash alone and in combination with gypsum and green manure for reclamation of degraded sodic soils of the indo-gangetic plains: A mechanism evaluation. Land Degrad. Dev. 30, 1300–1312. doi:10.1002/ldr.3308
Mitran, T., Basak, N., Mani, P. K., Tamang, A., Singh, D. K., Biswas, S., et al. (2021). Improving crop productivity and soil quality through soil management practices in coastal saline agro-ecosystem. J. Soil Sci. Plant Nutr. 21, 3514–3529. doi:10.1007/s42729-021-00624-8
Mukherjee, A., and Lal, R. (2014). Comparison of soil quality index using three methods. Plos One 9 (8), e105981. doi:10.1371/journal.pone.0105981
Murtaza, G. (2013). Economic aspects of growing rice and wheat crops on salt-affected soils in the Indus Basin of Pakistan. Faisalabad, Pakistan: Institute of Soil and Environmental Sciences, University of Agriculture. (unpublished data).
Nagaz, K., Masmoudi, M. M., and Mechlia, N. Ben (2012). Impacts of irrigation regimes with saline water on carrot productivity and soil salinity. J. Saudi Soc. Agric. Sci. 11, 19–27. doi:10.1016/j.jssas.2011.06.001
Nayak, A. K., Mishra, V. K., Sharma, D. K., Jha, S. K., Singh, C. S., Shahabuddin, M., et al. (2013). Efficiency of phosphogypsum and mined gypsum in reclamation and productivity of rice–wheat cropping system in sodic soil. Commun. Soil Sci. Plant Analysis 44, 909–921. doi:10.1080/00103624.2012.747601
Nayak, A. K., Sharma, D. K., Mishra, V. K., Minhas, P. S., and Verma, C. L. (2008). Reclamation of saline-sodic soil under a rice–wheat system by horizontal surface flushing. Soil Use Manag. 24, 337–343. doi:10.1111/j.1475-2743.2008.00162.x
Neubauer, S. C., Piehler, M. F., Smyth, A. R., and Franklin, R. B. (2019). Saltwater intrusion modifies microbial community structure and decreases denitrification in tidal freshwater marshes. Ecosystems 22, 912–928. doi:10.1007/s10021-018-0312-7
Noctor, G., and Foyer, C. H. (1998). Ascorbate and glutathione: Keeping active oxygen under control. Annu. Rev. Plant Physiol. Plant Mol. Biol. 49, 249–279. doi:10.1146/annurev.arplant.49.1.249
Oades, J. M. (1988). The retention of organic matter in soils. Biogeochemistry 5, 35–70. doi:10.1007/BF02180317
Pal, D. K., Bhattacharyya, T., Ray, S. K., Chandran, P., Srivastava, P., Durge, S. L., et al. (2006). Significance of soil modifiers (Ca-zeolites and gypsum) in naturally degraded Vertisols of the Peninsular India in redefining the sodic soils. Geoderma 136, 210–228. doi:10.1016/j.geoderma.2006.03.020
Pitman, A. J., Narisma, G. T., Pielke, ., R. A., and Holbrook, N. J. (2004). Impact of land cover change on the climate of southwest Western Australia. J. Geophys. Res. 109, D18109. doi:10.1029/2003JD004347
Purakayastha, T. J., Pathak, H., Kumari, S., Biswas, S., Chakrabarty, B., Padaria, R. N., et al. (2019). Soil health card development for efficient soil management in Haryana, India. Soil Tillage Res. 191, 294–305. doi:10.1016/j.still.2018.12.024
Qadir, M., Quillérou, E., Nangia, V., Murtaza, G., Singh, M., Thomas, R. J., et al. (2014). Economics of salt-induced land degradation and restoration. Nat. Resour. Forum 38, 282–295. doi:10.1111/1477-8947.12054
Qadir, M., Schubert, S., Oster, J. D., Sposito, G., Minhas, P. S., Cheraghi, S. A. M., et al. (2018). High-magnesium waters and soils: Emerging environmental and food security constraints. Sci. Total Environ. 642, 1108–1117. doi:10.1016/j.scitotenv.2018.06.090
Quirk, J. P. (2001). The significance of the threshold and turbidity concentrations in relation to sodicity and microstructure. Soil Res. 39, 1185. doi:10.1071/SR00050
Rai, A. K., Basak, N., Soni, P. G., Kumar, S., Sundha, P., Narjary, B., et al. (2022). Bioenergy sorghum as balancing feedback loop for intensification of cropping system in salt-affected soils of the semi–arid region: Energetics, biomass quality and soil properties. Eur. J. Agron. 134, 126452. doi:10.1016/j.eja.2021.126452
Rai, A. K., Basak, N., and Sundha, P. (2021a). “Alternate amendments for reclamation of alkali soils,” in Managing salt-affected soil for sustainable agriculture. Editors P. C. S. P. S. Minhas, and R. K. Yadav (New Delhi, India: New Delhi: Indian Council of Agricultural Research ICAR), 332–345.
Rai, A. K., Basak, N., and Sundha, P. (2021b). “Chemistry of salt-affected soils,” in Managing salt-affected soils for sustainable agriculture. Editors P. C. S. P. S. Minhas, and R. K. Yadav (New Delhi: Indian Council of Agricultural Research ICAR).
Rai, A. K., Basak, N., and Sundha, P. (2021c). “Saline and sodic ecosystems in the changing world,” in Soil science: Fundamentals to recent advances. Editors B. A. A. Rakshit, S. Singh, and P. Abhilash (Singapore: Springer), 175–190. doi:10.1007/978-981-16-0917-6_9
Raiesi, F., and Kabiri, V. (2016). Identification of soil quality indicators for assessing the effect of different tillage practices through a soil quality index in a semi-arid environment. Ecol. Indic. 71, 198–207. doi:10.1016/j.ecolind.2016.06.061
Ranatunga, K., Nation, E. R., and Barodien, G. (2010). Potential use of saline groundwater for irrigation in the Murray hydrogeological basin of Australia. Environ. Model. Softw. 25, 1188–1196. doi:10.1016/j.envsoft.2010.03.028
Rengasamy, P. (2016). Soil chemistry factors confounding crop salinity tolerance—A review. Agronomy 6, 53. doi:10.3390/agronomy6040053
Rengasamy, P. (2006). World salinization with emphasis on Australia. J. Exp. Bot. 57, 1017–1023. doi:10.1093/jxb/erj108
USSL (1954). “Diagnosis and improvement of saline and alkali soils,”. Editor L. A. Richards (Washington, DC: USDA Handbook).
Rietz, D. N., and Haynes, R. J. (2003). Effects of irrigation-induced salinity and sodicity on soil microbial activity. Soil Biol. Biochem. 35, 845–854. doi:10.1016/S0038-0717(03)00125-1
Sadegh-Zadeh, F., Parichehreh, M., Jalili, B., and Bahmanyar, M. A. (2018). Rehabilitation of calcareous saline-sodic soil by means of biochars and acidified biochars. Land Degrad. Dev. 29, 3262–3271. doi:10.1002/ldr.3079
Sanyal, S. K., Poonia, S. R., and Baruah, T. C. (2012). Soil colloids and ion exchange in soil. New DelhiNew Delhi: Indian Society of Soil Science India.
Seleiman, M. F., Aslam, M. T., Alhammad, B. A., Hassan, M. U., Maqbool, R., Chattha, M. U., et al. (2022). Salinity stress in wheat: Effects, mechanisms and management strategies. Phyt. J. Exp. Bot. 91. doi:10.32604/phyton.2022.017365
Seleiman, M. F., Kheir, A. M. S., Al-Dhumri, S., Alghamdi, A. G., Omar, E.-S. H., Aboelsoud, H. M., et al. (2019). Exploring optimal tillage improved soil characteristics and productivity of wheat irrigated with different water qualities. Agronomy 9, 233. doi:10.3390/agronomy9050233
Seleiman, M. F., and Kheir, A. M. S. (2018). Saline soil properties, quality and productivity of wheat grown with bagasse ash and thiourea in different climatic zones. Chemosphere 193, 538–546. doi:10.1016/j.chemosphere.2017.11.053
Shahid, S. A., Zaman, M., and Heng, L. (2018). Soil salinity: Historical perspectives and a world overview of the problem BT - guideline for salinity assessment, mitigation and adaptation using nuclear and related techniques. Cham: Springer International Publishing.
Sharma, D. K., ThimmappaChinchmalatpure, K. A. R., Mandal, A. K., Yadav, R. K., Chaudhury, S. K., Kumar, S., et al. (2015). Assessment of production and monetary losses from salt-affected soils in India. Haryana, India: Technical Bulletin. Karnal.
Sharp, R. E., Hsiao, T. C., and Silk, W. K. (1990). Growth of the maize primary root at low water potentials 1: II. Role of growth and deposition of hexose and potassium in osmotic adjustment. Plant Physiol. 93, 1337–1346. doi:10.1104/pp.93.4.1337
Sheoran, P., Basak, N., Kumar, A., Yadav, R. K., Singh, R., Sharma, R., et al. (2021). Ameliorants and salt tolerant varieties improve rice-wheat production in soils undergoing sodification with alkali water irrigation in Indo–Gangetic Plains of India. Agric. Water Manag. 243, 106492. doi:10.1016/j.agwat.2020.106492
Sheoran, P., Sharma, R., Kumar, A., Singh, R. K., Barman, A., Prajapat, K., et al. (2022). Climate resilient integrated soil–crop management (CRISCM) for salt affected wheat agri–food production systems. Sci. Total Environ. 837, 155843. doi:10.1016/j.scitotenv.2022.155843
Shirale, A. O., Kharche, V. K., Wakode, R. R., Meena, B. P., Das, H., Gore, R. P., et al. (2018). Influence of gypsum and organic amendments on soil properties and crop productivity in degraded black soils of central India. Commun. Soil Sci. Plant Analysis 49, 2418–2428. doi:10.1080/00103624.2018.1510952
Singh, R., Yaduvanshi, N. P. S., Singh, K. N., Kumar, S., Mishra, V. K., Singh, Y. P., et al. (2014). Bio-chemical amelioration effects on physico-chemical dynamics of sodic soils under rice (Oryza sativa) – wheat (Triticum aestivum) cropping system. Indian J. Agric. Sci. 84, 349–355.
Singh, A., Bali, A., Kumar, A., Yadav, R. K., and Minhas, P. S. (2022). Foliar spraying of potassium nitrate, salicylic acid, and thio-urea effects on growth, physiological processes, and yield of sodicity-stressed paddy (Oryza sativa l.) with alkali water irrigation. J. Plant Growth Regul. 41, 1989–1998. doi:10.1007/s00344-022-10575-8
Singh, G. (2009). Salinity-related desertification and management strategies: Indian experience. Land Degrad. Dev. 20, 367–385. doi:10.1002/ldr.933
Singh, O. P. (1998). “Salinity and waterlogging problems in irrigation commands,” in, eds. N. K. Tyagi, and P. S. Minhas (Karnal (Haryana), India.: Agricultural salinity management in India. Centreal Soil Salinity Research Institute).
Singh, R. K., Singh, A., and Sharma, P. C. (2019). Successful adaptations in salt affected agroecosystems of India. Karnal (Haryana), India: ICAR-Central Soil Salinity Research Institute.
Singh, R., Yaduvanshi, N. P. S., Singh, K. N., Kumar, S., Mishra, V. K., Singh, Y. P., et al. (2014). Bio-chemical amelioration effects on physico-chemical dynamics of sodic soils under rice (Oryza sativa) –wheat (Triticum aestivum) cropping system. Indian J. Agric. Sci. 84, 349–355.
Singh, R. K., Singh, A., and Sharma, P. C. (2019). Successful adaptations in salt affected agroecosystems of India. Karnal (Haryana), India: ICAR-Central Soil Salinity Research Institute.
Sione, S. M. J., Wilson, M. G., Lado, M., and González, A. P. (2017). Evaluation of soil degradation produced by rice crop systems in a Vertisol, using a soil quality index. Catena 150, 79–86. doi:10.1016/j.catena.2016.11.011
Soni, P. G., Basak, N., Rai, A. K., Sundha, P., Narjary, B., Kumar, P., et al. (2021). Deficit saline water irrigation under reduced tillage and residue mulch improves soil health in sorghum-wheat cropping system in semi-arid region. Sci. Rep. 11, 1880. doi:10.1038/s41598-020-80364-4
Sundha, P., Basak, N., Rai, A. K., Yadav, R. K., Sharma, P. C., Sharma, D. K., et al. (2020). Can conjunctive use of gypsum, city waste composts and marginal quality water rehabilitate saline-sodic soils? Soil Tillage Res. 200, 104608. doi:10.1016/j.still.2020.104608
Sundha, P., Basak, N., Rai, A., Yadav, R., Sharma, D., and Sharma, P. (2017). N and P Release pattern in saline-sodic soil amended with gypsum and municipal solid waste compost. J. Soil Salin. Water Qual. 9, 145–155.
Sundha, P., Rai, A. K., Basak, N., Yadav, R. K., and Sharma, P. C. (2022). P solubility and release kinetics in the leachate of saline–sodic soil: Effect of reclamation strategies and water quality. Soil Tillage Res. 222, 105440. doi:10.1016/j.still.2022.105440
Swarup, A., and Yaduvanshi, N. P. S. (2000). Effects of integrated nutrient management on soil properties and yield of rice in alkali soils. J. Indian Soc. Soil Sci. 48, 279–282.
Taha, S. R., Seleiman, M. F., Alhammad, B. A., Alkahtani, J., Alwahibi, M. S., Mahdi, A. H. A., et al. (2021). Activated yeast extract enhances growth, anatomical structure, and productivity of lupinus termis l. plants under actual salinity conditions. Agronomy 11, 74. doi:10.3390/agronomy11010074
Taha, S. R., Seleiman, M. F., Alotaibi, M., Alhammad, B. A., Rady, M. M., Mahdi, H. A., et al. (2020). Exogenous potassium treatments elevate salt tolerance and performances of Glycine max L. by boosting antioxidant defense system under actual saline field conditions. Agronomy 10, 1741. doi:10.3390/agronomy10111741
Tripathi, S., Chakraborty, A., Chakrabarti, K., and Bandyopadhyay, B. K. (2007). Enzyme activities and microbial biomass in coastal soils of India. Soil Biol. Biochem. 39, 2840–2848. doi:10.1016/j.soilbio.2007.05.027
Tripathi, S., Kumari, S., Chakraborty, A., Gupta, A., Chakrabarti, K., Bandyapadhyay, B. K., et al. (2006). Microbial biomass and its activities in salt-affected coastal soils. Biol. Fertil. Soils 42, 273–277. doi:10.1007/s00374-005-0037-6
Tucker, B. M. (1985). The partitioning of exchangeable magnesium, calcium and sodium in relation to their effects on the dispersion of Australian clay subsoils. Soil Res. 23, 405. doi:10.1071/SR9850405
Vance, E. D., Brookes, P. C., and Jenkinson, D. S. (1987). Microbial biomass measurements in forest soils: The use of the chloroform fumigation-incubation method in strongly acid soils. Soil Biol. Biochem. 19, 697–702. doi:10.1016/0038-0717(87)90051-4
Vasu, D., Tiwary, P., Chandran, P., Singh, S. K., Ray, S. K., Butte, P., et al. (2018). A conceptual model of natural land degradation based on regressive pedogenesis in semiarid tropical environments. Land Degrad. Dev. 29, 2554–2567. doi:10.1002/ldr.3000
Visser, S., Keesstra, S., Maas, G., de Cleen, M., and Molenaar, C. (2019). Soil as a basis to create enabling conditions for transitions towards sustainable land management as a key to achieve the SDGs by 2030. Sustainability 11, 6792. doi:10.3390/su11236792
Vyshpolsky, F., Mukhamedjanov, K., Bekbaev, U., Ibatullin, S., Yuldashev, T., Noble, A. D., et al. (2010). Optimizing the rate and timing of phosphogypsum application to magnesium-affected soils for crop yield and water productivity enhancement. Agric. Water Manag. 97, 1277–1286. doi:10.1016/j.agwat.2010.02.020
Wang, Q., Lu, C., Li, H., He, J., Sarker, K. K., Rasaily, R. G., et al. (2014). The effects of no-tillage with subsoiling on soil properties and maize yield: 12-Year experiment on alkaline soils of northeast China. Soil Tillage Res. 137, 43–49. doi:10.1016/j.still.2013.11.006
Wasko, C., and Sharma, A. (2017). Global assessment of flood and storm extremes with increased temperatures. Sci. Rep. 7, 7945. doi:10.1038/s41598-017-08481-1
Wong, V. N. L., Greene, R. S. B., Dalal, R. C., and Murphy, B. W. (2010). Soil carbon dynamics in saline and sodic soils: A review. Soil Use Manag. 26, 2–11. doi:10.1111/j.1475-2743.2009.00251.x
Xu, X., Huang, G., Sun, C., Pereira, L. S., Ramos, T. B., Huang, Q., et al. (2013). Assessing the effects of water table depth on water use, soil salinity and wheat yield: Searching for a target depth for irrigated areas in the upper Yellow River basin. Agric. Water Manag. 125, 46–60. doi:10.1016/j.agwat.2013.04.004
Yaduvanshi, N. P. S., and Swarup, A. (2005). Effect of continuous use of sodic irrigation water with and without gypsum, farmyard manure, pressmud and fertilizer on soil properties and yields of rice and wheat in a long term experiment. Nutr. Cycl. Agroecosyst. 73, 111–118. doi:10.1007/s10705-005-3361-1
Yaduvanshi, N. P. S., and Sharma, D. R. (2008). Tillage and residual organic manures/chemical amendment effects on soil organic matter and yield of wheat under sodic water irrigation. Soil Tillage Res. 98, 11–16. doi:10.1016/j.still.2007.09.010
Yu, H., Yang, P., Lin, H., Ren, S., and He, X. (2014). Effects of sodic soil reclamation using flue gas desulphurization gypsum on soil pore characteristics, bulk density, and saturated hydraulic conductivity. Soil Sci. Soc. Am. J. 78, 1201–1213. doi:10.2136/sssaj2013.08.0352
Yu, P., Liu, S., Zhang, L., Li, Q., and Zhou, D. (2018). Selecting the minimum data set and quantitative soil quality indexing of alkaline soils under different land uses in northeastern China. Sci. Total Environ. 616, 564–571. doi:10.1016/j.scitotenv.2017.10.301
Yuan, C., Feng, S., Huo, Z., and Ji, Q. (2019). Effects of deficit irrigation with saline water on soil water-salt distribution and water use efficiency of maize for seed production in arid Northwest China. Agric. Water Manag. 212, 424–432. doi:10.1016/j.agwat.2018.09.019
Zain, D., Seleiman, M., Khan, I., Chattha, M. U., Ali, E. F., Alhammad, B., et al. (2021). Glycine-betaine induced salinity tolerance in maize by regulating the physiological attributes, antioxidant defense system and ionic homeostasis. Not. Bot. Horti Agrobot. Cluj. Napoca. 49, 12248. doi:10.15835/nbha49112248
Zhang, W., Zhao, Y., Wang, S., Li, Y., Zhuo, Y., Liu, J., et al. (2021). Soil salinity and sodicity changes after a one-time application of flue gas desulphurization gypsum to paddy fields. Land Degrad. Dev. 32, 4193–4202. doi:10.1002/ldr.4025
Keywords: amendments, soil quality, soil quality indicators, salt-affected soil, salt tolerance mechanism, sustainable management
Citation: Basak N, Rai AK, Sundha P, Meena RL, Bedwal S, Yadav RK and Sharma PC (2022) Assessing soil quality for rehabilitation of salt-affected agroecosystem: A comprehensive review. Front. Environ. Sci. 10:935785. doi: 10.3389/fenvs.2022.935785
Received: 04 May 2022; Accepted: 11 July 2022;
Published: 19 August 2022.
Edited by:
Donatella Valente, University of Salento, ItalyReviewed by:
Mahmoud F. Seleiman, King Saud University, Saudi ArabiaCopyright © 2022 Basak, Rai, Sundha, Meena, Bedwal, Yadav and Sharma. This is an open-access article distributed under the terms of the Creative Commons Attribution License (CC BY). The use, distribution or reproduction in other forums is permitted, provided the original author(s) and the copyright owner(s) are credited and that the original publication in this journal is cited, in accordance with accepted academic practice. No use, distribution or reproduction is permitted which does not comply with these terms.
*Correspondence: Nirmalendu Basak, bmlybWFsZW5kdS5iYXNha0BpY2FyLmdvdi5pbg==, bmlybWFsZW5kdWJhc2FrQHJlZGlmZm1haWwuY29t; Arvind Kumar Rai, cmFpYXJ2aW5ka3VtYXJAZ21haWwuY29t, YWsucmFpQGljYXIuZ292Lmlu; Parul Sundha, cGFydWwuc3VuZGhhQGljYXIuZ292Lmlu
Disclaimer: All claims expressed in this article are solely those of the authors and do not necessarily represent those of their affiliated organizations, or those of the publisher, the editors and the reviewers. Any product that may be evaluated in this article or claim that may be made by its manufacturer is not guaranteed or endorsed by the publisher.
Research integrity at Frontiers
Learn more about the work of our research integrity team to safeguard the quality of each article we publish.