- 1College of Environmental Science and Engineering, Yangzhou University, Yangzhou, China
- 2Soil Fertilizer Technical Guidance Station, Nantong, China
- 3People’s Government of Zhenyu Town, Nantong, China
- 4Duke Kunshan University, Suzhou, China
- 5Jiangsu Collaborative Innovation Center for Solid Organic Waste Resource Utilization, Nanjing, China
Rice-wheat rotation has long been the most typical cropping system along the mid-lower reaches of the Yangtse River in China, and the full amount returning of wheat straw before rice season is widely practiced in the rotation system. However, massive incorporation of fresh wheat straw may activate Cd in the soil solid phase and cause more labile Cd entering soil solution in Cd-contaminated soil during the flooded rice season. An incubation experiment was conducted under flooded conditions to investigate the solubility and speciation of Cd and its variation and driving factors with an ongoing decomposition phase in simulated Cd-contaminated paddy soil treated with wheat straw addition. The results indicated that wheat straw addition enhanced Cd solubility, and soluble Cd concentration increased with the ongoing decomposition phase. The observed significant positive correlation (r = 0.648, p < 0.01, n = 77) between soluble Cd concentration and DOC content in soil leachate was gradually strengthened with ongoing decomposition. Wheat straw addition decreased the exchangeable fraction of Cd and increased the residual fraction during the first 5 days of decomposition, while the exchangeable Cd increased and the residual Cd decreased after 10 days of decomposition. The exchangeable Cd increased and the residual Cd decreased gradually with ongoing decomposition. Correlation analysis showed that the exchangeable and residual fractions were sensitive to DOC content. Nitrogen fertilizer input further activated Cd by promoting wheat straw decomposition. In summary, wheat straw addition would activate Cd by increasing Cd solubility and promoting the translation from immobile fraction to labile fraction in Cd-contaminated paddy soil under flooded conditions.
Introduction
Soil heavy metal contamination can deteriorate the quality of water bodies and crop products and has become a great threat to the health of animals and human beings (Nabulo et al., 2010; Li et al., 2014; Kumar et al., 2019). In China, fast industrialization, urbanization, and intensive agriculture over the past several decades have caused wide and serious heavy metal pollution in agricultural soil (Dong et al., 2010; Yang et al., 2018). According to the National Soil Pollution Survey Bulletin (2014), the exceedance rate of sample points surveyed on a national scale was 16.1%, among which cadmium (Cd) was the prominent contaminant, and the exceedance rate of sample points reached 7.0% (Sun et al., 2021). Compared with other heavy metals, Cd can transfer and accumulate in the soil-plant system more easily, and its hazard in contaminated soil mainly depends on its mobility and availability (Khodaverdiloo et al., 2020; Yuan et al., 2021), which in turn is significantly influenced by organic matter (Antoniadis and Alloway, 2002; Strobel et al., 2005). Organic matter itself can effectively adsorb Cd due to its strong sorption ability of functional groups on heavy metals (Weng et al., 2002; Gao et al., 2018) or be regarded as a carrier for Cd, thereby enhancing its migration and transformation in soil (Richard et al., 2008; Spaccini et al., 2008). Moreover, organic matter addition can indirectly influence Cd activity by altering soil pH and DOC (Yuan et al., 2019; Yuan et al., 2021).
High-yielding crop production has led to an enormous generation of crop straw in China (Xia et al., 2014). In order to recycle the straw resources and reduce its adverse effects (e.g., open-field burning of crop straw) on the environment, the Ministry of Agriculture and Rural Affairs of China proposed the “Fertilizer Use Zero-Growth Action Plan by 2020” in 2015, in which further increase of the nationwide proportion of crop straw incorporation to 60% by 2020 was required. Currently, the full amount returning of crop straw is practiced in the areas with a higher level of mechanization. Straw returning can elevate soil fertility by improving soil structure, returning considerable nutrients to the soil, and increasing soil organic matter (Yan and Gong, 2010; Sharma and Garg, 2018). Nevertheless, straw returning in large amounts would inevitably affect the mobility and availability of Cd in Cd-contaminated soil. Although there have been many studies that provided general information on the mobility and availability of Cd in straw-treated contaminated soil, their results are inconsistent or even contrasting. For example, some studies show that crop straw addition can reduce Cd mobility and availability via enhancing the Cd adsorption in the soil solid phase by the organic matter ligands (Mohamed et al., 2010; Yuan et al., 2019). In contrast, some studies reported that the mobility and availability of Cd increased in straw-treated soil owing to the release of low-molecular-weight organic matter (mainly DOC), as it can complex metals previously bound to soil particles (Khan et al., 2006; Bai et al., 2013; Wang et al., 2015). In addition, some studies show that straw incorporation has an insignificant influence on Cd mobility or availability (Feng et al., 2018; Nie et al., 2019). The inconsistent results were mainly attributed to the difference in crop straw materials and straw decomposing environment.
Rice-wheat rotation has long been the most typical cropping system along the mid-lower reaches of the Yangtse River in China, and the full amount returning of wheat straw before rice season is widely practiced in the rotation system. The area under rice cultivation in this region accounts for about half of the total in China. Nearly 1/3 of the surveyed 187 administrative regions with rice cultivation in China had Cd contamination (Liu et al., 2016). More importantly, the hazard of Cd might be more prominent in paddy soil as rice can accumulate Cd from contaminated soil more efficiently than other crops (Maret and Moulis, 2013). Studies carried out in 2008 and 2018 showed that 10% of the rice samples collected from Chinese rice markets contained >200 μg kg−1 Cd (Zhen et al., 2008; Chen et al., 2018). In addition, long-term flooding and nitrogen fertilizer input in rice season will inevitably affect the decomposition of preceding wheat straw and further complicate the migration and transformation of Cd in Cd-contaminated paddy soil. However, the temporal variation of mobility, availability, and speciation of Cd influenced by wheat straw incorporation and its driving factors during the wheat straw decomposition phase remains unclear in Cd-contaminated paddy soil.
In this study, simulated Cd-contaminated paddy soil was treated with different wheat straw addition rates that were cultured under different decomposition periods. The main objectives of this study were to 1) evaluate the mobility and speciation distribution of Cd under different straw addition rates in Cd-contaminated soil under flooded conditions; 2) investigate the variation of the above Cd properties during the straw decomposition phase; and 3) explore the driving factors closely related to the migration and transformation of Cd in Cd-contaminated soil treated with wheat straw.
Materials and Methods
Experimental Materials
The soil used was sampled from the upper layer of the soil (0–20 cm) in the experimental field of Yangzhou University (32°20′33″ N, 119°23′43″ E), Jiangsu Province, China, and belongs to Xiashu Loess. The soil was crushed and sieved (2 mm) after naturally air-dried. The straw used was collected from the wheat plant experimental field of Yangzhou University. The fresh wheat plant was dried at 105 C for 15 min and then oven-dried at 80 C. The oven-dried wheat plant was comminuted and then passed through 2 mm mesh. The initial pH, total Cd concentration of soil, and Cd concentration of wheat straw are 7.50, 4.74 mg kg−1, and 0.24 mg kg−1, respectively.
Experimental Design
The sieved soil was evenly mixed with CdCl2 solution and aged stably at room temperature (about 25°C) for 3 months to simulate Cd-contaminated soil. Deionized water was replenished by weighing every 3 days during the aging stabilization period to maintain a 40% field saturation in the soil. Total and available Cd concentrations in this Cd-contaminated soil were 78.63 mg kg−1 and 31.12 mg kg−1. Then, one hundred grams of Cd-contaminated soil was put into a 300-ml plastic cup. The treatments of wheat straw addition were conducted by mixing the sieved wheat straw uniformly with soils in plastic cups at the dry weight rates of 0, 2.5, 5, 10, and 20 g kg−1 (defined as S0, S1, S2, S3, and S4), respectively. In order to investigate the effect of nitrogen fertilizer input on Cd dissolution in Cd-contaminated soil treated with wheat straw, additional treatment with 10 g kg−1 wheat straw addition rate and 0.06 g ammonium nitrate was set and defined as SN. Eighteen plastic cups were set up for each treatment to ensure three replicates per sampling period. Then 150 ml deionized water was added to the plastic cup to simulate the flooding condition of paddy soil. The plastic cups were incubated in a constant temperature incubator with temperature controlled at 25–28°C. During the incubation period, the mixture of Cd-contaminated soil and wheat straw was stirred irregularly, and the lost water was replenished according to the change in the weight of the plastic cup.
Soil Analysis
The mixture in the plastic cup was collected by destructive sampling and placed in a centrifuge tube at 5, 10, 20, 40, 60, and 80 days, respectively and centrifuged for 10 min (3,000 rpm). The supernatant in the centrifuge tube passed through a 0.45 μm filter membrane for soluble Cd, pH, and DOC analysis. The remaining soil in the centrifuge tube was naturally air-dried, crushed, and sieved (1 mm) for Cd fraction analysis. Soluble Cd, pH, and DOC in soil leachate were detected by ICP-AES (Model iCAP 6300, Thermo Fisher Scientific Inc., United States), pH meter (Model IQ150, Spectrum, United States), and total organic carbon (TOC) analyzer (Model TOC-L, Shimadzu, Japan), respectively. Soil available Cd concentration was analyzed by the DTPA extraction method (Bao 2000). Geochemical fractions of Cd in the remaining soil were divided into four parts: exchangeable fraction (EX), reducible fraction (RG), oxidizable fraction (OXI), and residual fraction (RES). Sequential extraction for Cd fractions was described in our previous study (Bai et al., 2016). Furthermore, certified reference materials (CRMs) were used to ensure the reliability and accuracy of test results.
Statistical Analysis
SPSS 13.0 software (SPSS Inc., United States) was used to analyze the data using analysis of variance (ANOVA). The differences between treatments were detected by the least significant difference (LSD) method at the 5% level. The relationships between pH, DOC, and soluble Cd and geochemical fractions of Cd were established through regression analysis, and the significance of the regression was judged according to the probability (p) value for the corresponding linear model.
Results
pH and DOC in Soil Leachate
Wheat straw addition significantly increased pH in soil leachate in all treatments (Figure 1). With increasing wheat straw addition rates, the pH in soil leachate increased during the initial 60 days of decomposition, followed by a gradual decrease during the subsequent 20 days of decomposition (at 80 days). Compared with S0 (without wheat straw addition), the average increase of pH units in 20 g kg−1 treatment (S4) was 0.64, 0.55, 0.94, 0.96, 0.44, and 0.35 at 5, 10, 20, 40, 60, and 80 days, respectively. The pH in soil leachate collected from soils treated with high straw inputs (S3 and S4) remained relatively stable during the first 40 days and then slightly decreased during the subsequent 40 days of decomposition. There was no significant change in pH under nitrogen addition.
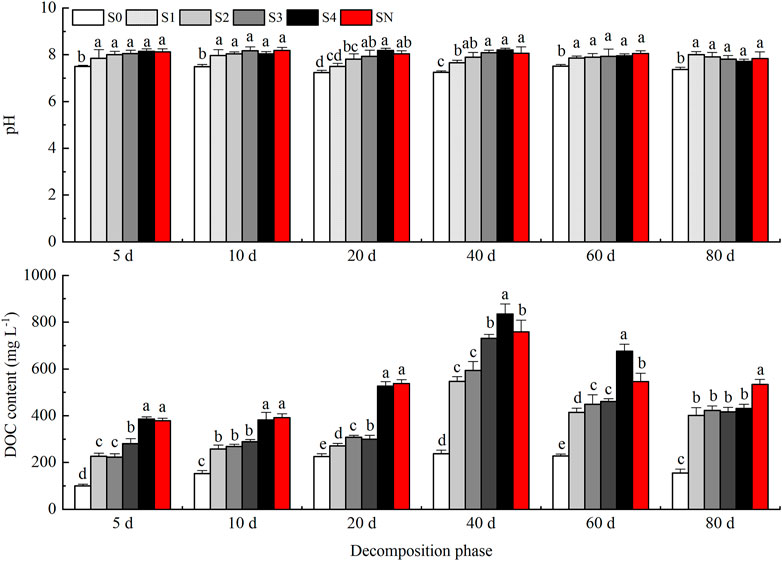
FIGURE 1. pH and DOC content in soil leachate in response to wheat straw addition. The vertical bars denote standard errors. Columns with different letters represent significant difference (p < 0.05).
Additions of wheat straw significantly increased DOC content in soil leachate and its concentration increased with increasing wheat straw addition rates (Figure 1). The maximum increments in DOC contents were 283.0% (5 days), 150.3% (10 days), 134.2% (20 days), 251.9% (40 days), 197.0% (60 days), and 178.1% (80 days), respectively, compared with S0. DOC contents in all treatments increased substantially to the maximum value after flooding for 40 days, followed by a gradual decrease during the subsequent 40 days of decomposition. DOC contents at 40 days decomposition were 135.9% (for S0), 141.3% (for S1), 165.5% (for S2), 160.3% (for S3), and 116.7% (for S4) higher than those at the first 5 days of decomposition phase. Wheat straw addition combined with nitrogen fertilizer (SN) further elevated DOC content in soil leachate. Compared with S3, the average increment in DOC content in SN reached 33.2% during wheat straw decomposition.
Distribution of Available Cd in Soil Leachate and Remaining Soil
With increasing rates of wheat straw added, Cd concentrations in soil leachate increased as a whole (Figure 2). Soluble Cd in treatments with wheat straw added was significantly higher than those in soil without wheat straw addition except for S1 and S3 treatments at the first 5 days of the decomposition phase. Soluble Cd concentration in S4 were 124.1% (5 days), 187.6% (10 days), 140.8% (20 days), 94.8% (40 days), 135.2% (60 days), and 209.1% (80 days) higher than those in S0. Soluble Cd concentrations in S0 rose gradually to the maximum value after flooding for 59 days (at 60 days) and then decreased during the subsequent 20 days of decomposition. While soluble Cd concentrations in straw-treated treatments increased substantially with ongoing decomposition, the maximum values in soluble Cd concentration after 80 days of decomposition for S1, S2, S3, and S4 reached 51.5, 62.5, 62.2, and 81.28 μg L−1, respectively. Compared with S3, SN further elevated soluble Cd concentration in soil leachate, and the increases were 32.4%, 7.5%, 57.3%, 70.0%, 35.2%, and 22.2% at 5, 10, 20, 40, 60, and 80 days, respectively.
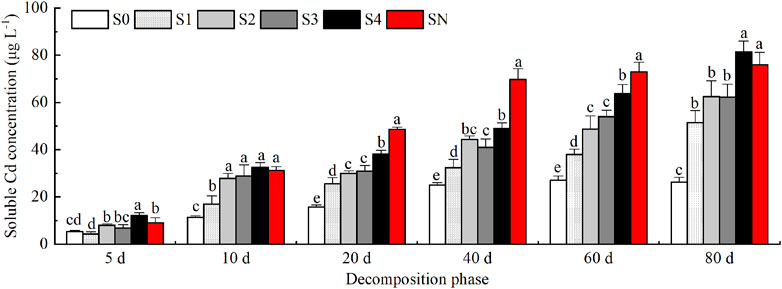
FIGURE 2. Soluble Cd concentration in soil leachate in response to wheat straw addition. The vertical bars denote standard errors. Columns with different letters represent significant difference (p < 0.05).
Figure 3 shows soluble Cd in soil leachate in S0 accounted for only 0.05%, 0.13%, 0.18%, 0.37%, 0.43%, and 0.38% of available Cd in the mixture at 5, 10, 20, 40, 60, and 80 days, respectively. While wheat straw addition significantly increased the proportion of soluble Cd in soil leachate, the proportion increased substantially with ongoing decomposition (Figure 3). The maximum proportions of soluble Cd reached 0.12% (5 days), 0.64% (10 days), 0.66% (20 days), 1.18% (40 days), 2.07% (60 days), and 2.82% (80 days), respectively. Compared with S3, the average increment in the proportion of soluble Cd in soil leachate in SN reached 70.0% during wheat straw decomposition.
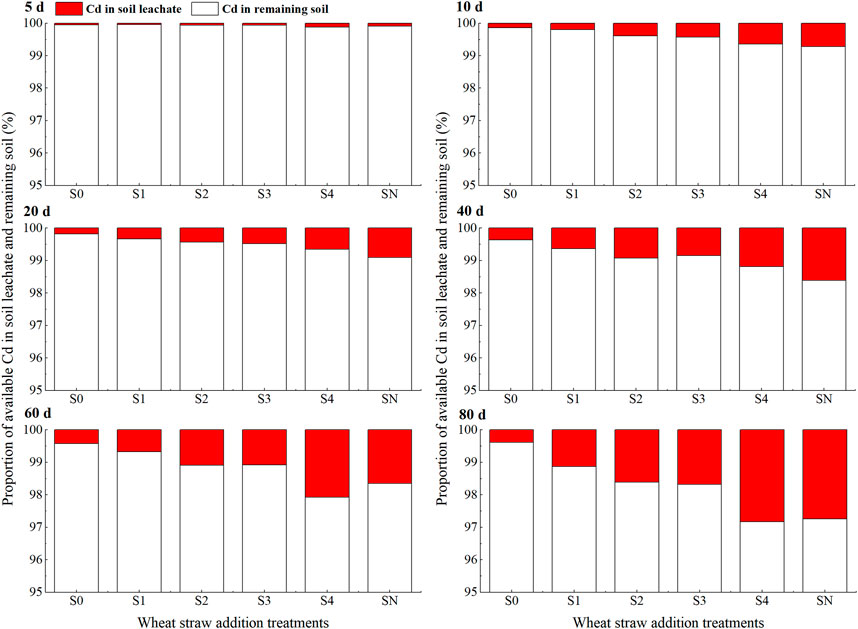
FIGURE 3. Effect of wheat straw addition on the distribution of available Cd in soil leachate and remaining soil.
Cd Speciation in Remaining Soil
Wheat straw addition initially decreased the exchangeable fraction during the first 5 days of decomposition and then increased the exchangeable fraction after 10 days of decomposition compared to S0 (Figure 4). The proportion of exchangeable fraction at 10, 20, 40, 60, and 80 days increased from 28.0%, 30.8%, 29.5%, 37.6%, and 44.4% in S0 to 30.1%, 33.3%, 41.0%, 46.0%, and 54.8% in S4, respectively. In contrast to exchangeable fraction, wheat straw addition initially increased the residual fraction during the early decomposition phase (5 and 10 days) and then decreased the residual fraction after 20 days of decomposition. Compared with S0, the proportion of residual fraction in S4 decreased by 9.7% (20 days), 26.6% (40 days), 30.9% (60 days), and 32.5% (80 days), respectively. The fractions of reducible and oxidizable showed no significant change in response to wheat straw addition. Compared with S3, SN further increased the proportions of exchangeable, reducible, and oxidizable fractions by 11.7%, 26.1%, and 19.7% and decreased the proportion of residual fraction by 21.0% throughout the decomposition phase, respectively.
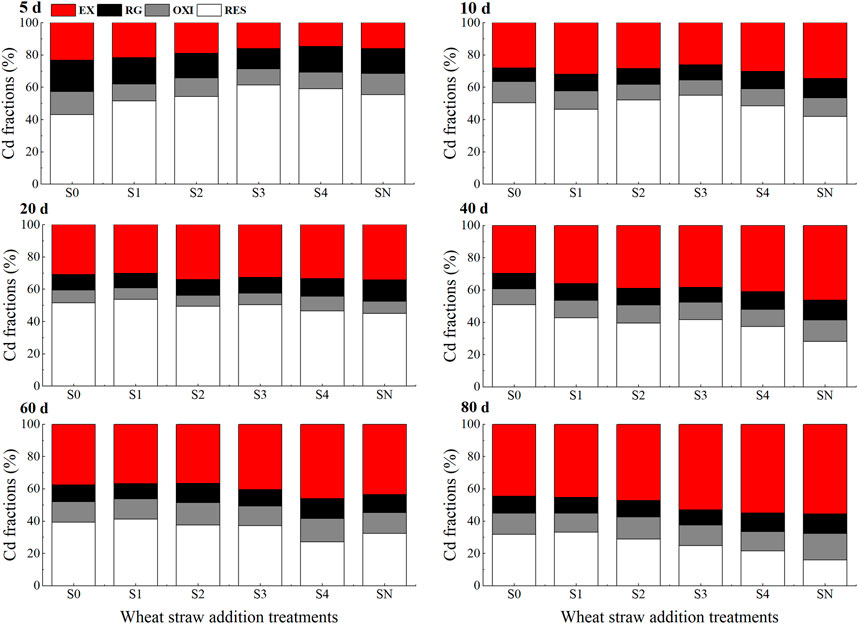
FIGURE 4. Effect of wheat straw addition on geochemical fractions of Cd in remaining soil. EX, exchangeable fraction; RG, reducible fraction; OXI, oxidizable fraction; RES, residual fraction.
The exchangeable fraction increased gradually with ongoing decomposition (Figure 4). Compared with the first 5 days of decomposition, the proportion of exchangeable fraction at 80 days increased by 91.8% (S0), 109.9% (S1), 150.2% (S2), 231.6% (S3), and 273.4% (S4), respectively. By contrast, the residual Cd decreased gradually with ongoing decomposition. The decrements in the proportion of residual fraction for S1, S2, S3, and S4 reached 35.5%, 46.7%, 59.8%, and 63.7% from 5 days to 80 days of decomposition, respectively, which were higher than 26.2% in control soil. The reducible fraction decreased markedly during the first 10 days of decomposition and then remained relatively stable. The oxidizable fraction decreased over the first 20 days of decomposition and then rose gradually to the initial values during the subsequent 60 days of decomposition.
Discussions
Wheat straw addition increased DOC in soil leachate, and its content increased with increasing straw addition rates. This result indicated the dissolution and decomposition of organic matter in wheat straw (Bai et al., 2013). A similar result was reported that straw addition led to an increase in DOC content (Kalbitz et al., 2000; Kalbitz, 2003). In this study, DOC content increased substantially after flooding for 40 days, followed by a gradual decrease during the subsequent 40 days of decomposition, which is consistent with the study that DOC content in wheat straw-treated soil nearly linearly increased (0–30 days) and decreased during the subsequent decomposition phase (30–90 days) (Gao et al., 2018). This result is mainly because the rapid decomposition of wheat straw released a large amount of DOC in the early and middle stages, which was consumed by microorganisms as a preferential substrate with ongoing decomposition (Chen et al., 2010). The pH in soil leachate increased after wheat straw addition, which might be attributed to the degradation and ammonification of organic nitrogen and the proton consumption caused by the decarboxylation of organic anions during straw decomposition (Yan et al., 1996). With ongoing decomposition, the accumulation of various organic acids released by cellulose degradation under flooded conditions and the nitrification might result in a pH decrease in soil leachate (Yan et al., 1996; Shan et al., 2008).
The addition of wheat straw in Cd-contaminated soil elevated soluble Cd concentration in soil leachate, and its concentration increased with ongoing decomposition. Although organic matter itself can directly influence Cd mobility through its higher adsorption capacity for heavy metals (Yuan et al., 2021), the adsorption capacity of fresh wheat straw which is mainly composed of cellulose is very weak for metals (Bai et al., 2013). Therefore, the increase in soluble Cd concentration in soil leachate might be attributed to the indirect effects of wheat straw addition on DOC content and soil pH (Gao et al., 2018; Yuan et al., 2019). In this study, the soluble Cd concentration correlated positively with DOC content (Table 1), with a linear equation of y = 0.065x + 6.7178 (r = 0.537, p < 0.01), which indicated that soluble Cd concentration could be increased by about 6.5 μg L−1 for each 100 mg L−1 increase in DOC content. Correlation between soluble Cd concentration and DOC content in soil leachate were all significant and positive in each decomposition period and were gradually strengthened with ongoing decomposition (Figure 5). The previous results indicated that DOC might be the driving factor influencing Cd solubility in Cd-contaminated soil treated with wheat straw under flooded conditions. Some studies also reported that DOC plays a significant role in mediating metal's solubility and mobility due to its stronger complexation ability (Weng et al., 2002; Gao et al., 2018). The release of low-molecular-weight organic matter (a major constituent of DOC) from biodegradation of wheat straw increased DOC content in soil leachate, which combined Cd and reduced the previously adsorption of Cd on soil surfaces, thus enhancing Cd solubility (Antoniadis and Alloway, 2002; Bai et al., 2018). This conclusion was further supported by the data obtained in which the proportion of available Cd decreased in the remaining soil treated with wheat straw in this study. Although there was no significant correlation between pH and soluble Cd concentration in this study mainly because pH remained relatively stable during the first 40 days (Figure 1), pH is still an important factor affecting Cd activity in Cd-contaminated soil. Some studies reported that one unit increase of soil pH can lead to 3–5 times elevation of Cd adsorption by soils (Sauvé et al., 2000; Degryse et al., 2009). In contrast, the decrease in pH will activate the insoluble Cd in soil, resulting in an increase in Cd mobility and availability (Alvarenga et al., 2009; Laird et al., 2010). Therefore, facing the decreasing trend of DOC after 40 days of decomposition, the continuous increase in soluble Cd concentration in soil leachate might be partly due to the decrease in pH during this period.
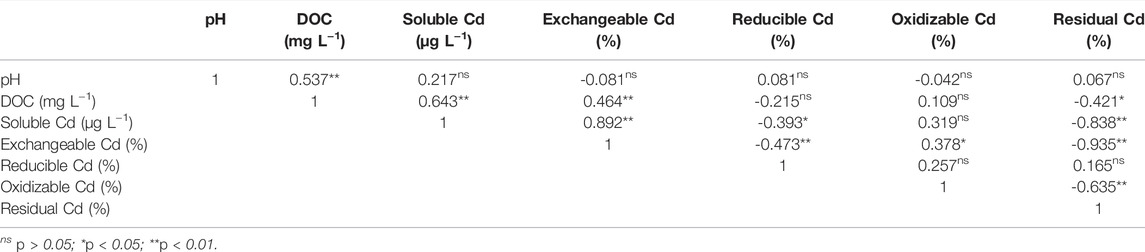
TABLE 1. Correlations between pH, DOC, and soluble Cd concentrations in soil leachate and Cd speciation in remaining soil.
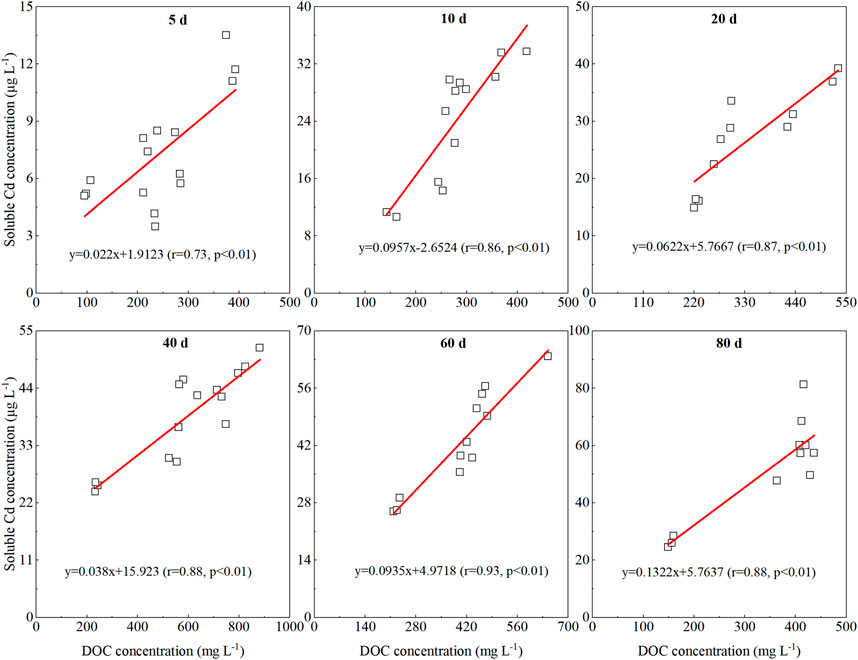
FIGURE 5. Correlations between soluble Cd concentration and DOC content in soil leachate at different decomposition phases of wheat straw (5, 10, 20, 40, 60, and 80 days).
The distribution of Cd speciation can reflect its mobility and availability more effectively (Kidd et al., 2007). In this study, wheat straw addition led to the redistribution of exchangeable, reducible, oxidizable, and residual fractions in soil. Studies have found that crop straw incorporation reduced metals’ mobility by transforming them from the easily accessible fraction into less mobile fractions (Ok et al., 2011; Xu et al., 2016). For example, Xiao et al. (2019) reported that crop straw reduced the proportion of metals in acid-soluble and reducible fractions and increased their persistence in oxidizable fraction. Similar results were found in this study that wheat straw addition decreased the exchangeable and reducible fractions of Cd, while increasing the residual fraction during the beginning of the decomposition phase (5 days). Crop straw incorporation rapidly reduces soil redox potential under flooded soil, which leads to the rapid dissolution and activation of iron oxide closely related to Cd activity (Mukwaturi and Lin, 2015), resulting in stronger adsorption of exchangeable Cd on the soil surface (Hu et al., 2004). Therefore, the weakly adsorbed Cd rapidly transformed into strongly adsorbed Cd through reabsorption or co-precipitation at the early decomposition phase of straw incorporation (Chefetz et al., 1998; Kashem and Singh, 2006). However, the soluble organic matter (mainly DOC) released with the ongoing decomposition of wheat straw has more active sites than soil and solid organic matter (Chefetz et al., 1998; Lu et al., 2000). The hydrophilic component or low molecular weight component of soluble organic matter in flooded soil has a strong complexation ability with Cd, which can form a chelate with Cd and lead to the increase of Cd solubility, and also reduce the adsorption of Cd (Hesterberg et al., 1993; Maes et al., 2003). Therefore, the exchangeable fraction increased and the residual fraction decreased in Cd-contaminated soil after 10 days of decomposition in this study. Furthermore, the increase in exchangeable fraction and the decrease in residual fraction were observed with ongoing decomposition in this study, and the highest increment or decrement in corresponding fractions were all observed in the highest wheat straw addition rate. Correlation analysis shows that DOC content correlated positively with exchangeable Cd (r = 0.464, p < 0.01) and negatively with residual Cd (r = -0.421, p < 0.05) and had no significant correlation with reducible and oxidizable fractions (Table 1). This result further indicates that the redistribution of Cd fractions was mainly attributed to the increase in DOC content, which activates Cd through promoting the release of strongly adsorbed Cd (residual fraction of Cd) in Cd-contaminated soil. However, further experimental studies are required to explore the interaction mechanism between DOC and a residual fraction of Cd in Cd-contaminated soil.
Wheat straw addition combined with nitrogen fertilizer further elevated soluble Cd concentration in soil leachate and promoted the transformation from residual fraction to exchangeable fraction in Cd-contaminated soil under flooded conditions. This result indicates that nitrogen fertilizer input might further amplify the environmental hazard of Cd by promoting the decomposition of returned wheat straw to release more DOC in Cd-contaminated soil, which further confirmed the significant role of DOC in mediating Cd solubility and mobility. Therefore, the current measures of the full amount returning of wheat straw in the mid-lower reaches of the Yangtse River in China should be adjusted to appropriately reduce the proportion of wheat straw incorporation in order to reduce Cd hazard in Cd-contaminated paddy soil. In addition, either wheat straw addition or nitrogen fertilizer input can promote the solubility of Cd and increase soluble Cd concentration in soil leachate, thus increasing the Cd hazard in Cd-contaminated soil under flooded conditions. Therefore, appropriate water management measures, such as intermittent irrigation and shallow irrigation, can be considered in the early stage of the rice growth season to prevent the dissolution of Cd in the soil solid phase from affecting the surrounding environment of Cd-contaminated paddy soil with wheat straw incorporation.
Conclusion
Wheat straw addition increased pH and DOC content in soil leachate. With the ongoing decomposition of wheat straw, pH remained relatively stable and DOC content increased substantially during the first 40 days, and then pH and DOC all decreased during the subsequent 40 days of decomposition. The soluble Cd concentration in soil leachate was elevated by wheat straw addition, and its concentration was correlated positively with DOC content. The exchangeable fraction of Cd decreased during the first 5 days of decomposition and increased after 10 days of decomposition in Cd-contaminated soil treated with wheat straw. By contrast, wheat straw addition increased the residual fraction of Cd at the first 5 days of decomposition and decreased its proportion after 10 days of decomposition. The exchangeable fraction increased gradually and the residual fraction decreased gradually with ongoing decomposition, and these two fractions were significantly positively correlated and negatively correlated with DOC content, respectively. Wheat straw addition combined with nitrogen fertilizer further elevated soluble Cd concentration and promoted the transformation from residual fraction to exchangeable fraction (National Soil Pollution Survey Bulletin, 2014).
Data Availability Statement
The raw data supporting the conclusions of this article are available upon request from the corresponding author.
Author Contributions
WZ: Writing original draft. SY and YC: Data and Methodology. GH and XZ: Investigation. YL, CG, and YB: Methodology. YS: Review and Editing. All authors read and approved the final manuscript.
Funding
This work was supported by the National Natural Science Foundation of China (No. 31872179), the China Postdoctoral Science Foundation (No. 2021M692722), the Natural Science Foundation of Jiangsu Province (No. BK20210793), the Research Fund for Jiangsu Agricultural Industry Technology System [No. JATS (2020)311], and the Key Laboratory of Organic Geochemistry, GIGCAS (No. SKLOG202118).
Conflict of Interest
The authors declare that the research was conducted in the absence of any commercial or financial relationships that could be construed as a potential conflict of interest.
Publisher’s Note
All claims expressed in this article are solely those of the authors and do not necessarily represent those of their affiliated organizations, or those of the publisher, the editors, and the reviewers. Any product that may be evaluated in this article, or claim that may be made by its manufacturer, is not guaranteed or endorsed by the publisher.
References
Alvarenga, P., Gonçalves, A. P., Fernandes, R. M., de Varennes, A., Vallini, G., Duarte, E., et al. (2009). Organic Residues as Immobilizing Agents in Aided Phytostabilization: (I) Effects on Soil Chemical Characteristics. Chemosphere 74 (10), 1292–1300. doi:10.1016/j.chemosphere.2008.11.063
Antoniadis, V., and Alloway, B. J. (2002). The Role of Dissolved Organic Carbon in the Mobility of Cd, Ni and Zn in Sewage Sludge-Amended Soils. Environ. Pollut. 117, 515–521. doi:10.1016/s0269-7491(01)00172-5
Bai, Y., Gu, C., Tao, T., Chen, G., and Shan, Y. (2013). Straw Incorporation Increases Solubility and Uptake of Cadmium by Rice Plants. Acta Agric. Scand. Sect. B - Soil & Plant Sci. 63 (3), 193–199. doi:10.1080/09064710.2012.743582
Bai, Y.-C., Zuo, W.-G., Zhao, H.-T., Mei, L.-J., Gu, C.-H., Guan, Y.-X., et al. (2016). Distribution of Heavy Metals in Maize and Mudflat Saline Soil Amended by Sewage Sludge. J. Soils Sediments 17 (6), 1565–1578. doi:10.1007/s11368-016-1630-z
Bai, Y., Yan, Y., Zuo, W., Gu, C., Guan, Y., Wang, X., et al. (2018). Distribution of Cadmium, Copper, Lead, and Zinc in Mudflat Salt-Soils Amended with Sewage Sludge. Land Degrad. Dev. 29 (4), 1120–1129. doi:10.1002/ldr.2914
Bao, S. (2000). Soil and Agro-Chemistry Analysis. 3rd edn. Beijing, China: China agriculstural press.
Chefetz, B., Chen, Y., Hadar, Y., and Hatcher, P. G. (1998). Characterization of Dissolved Organic Matter Extracted from Composted Municipal Solid Waste. Soil Sci. Soc. Am. J. 62 (2), 326–332. doi:10.2136/sssaj1998.03615995006200020005x
Chen, B., Zhu, Y.-G., Chen, B., and Zhu, Y. (2006). Humic Acids Increase the Phytoavailability of Cd and Pb to Wheat Plants Cultivated in Freshly Spiked, Contaminated Soil (7 Pp). J. Soils Sediments 6 (4), 236–242. doi:10.1065/jss2006.08.178
Chen, H.-L., Zhou, J.-M., and Xiao, B.-H. (2010). Characterization of Dissolved Organic Matter Derived from Rice Straw at Different Stages of Decay. J. Soils Sediments 10 (5), 915–922. doi:10.1007/s11368-010-0210-x
Chen, H., Tang, Z., Wang, P., and Zhao, F.-J. (2018). Geographical Variations of Cadmium and Arsenic Concentrations and Arsenic Speciation in Chinese Rice. Environ. Pollut. 238, 482–490. doi:10.1016/j.envpol.2018.03.048
Degryse, F., Smolders, E., and Parker, D. R. (2009). Partitioning of Metals (Cd, Co, Cu, Ni, Pb, Zn) in Soils: Concepts, Methodologies, Prediction and Applications - a Review. Eur. J. Soil Sci. 60 (4), 590–612. doi:10.1111/j.1365-2389.2009.01142.x
Dong, W. Q. Y., Cui, Y., and Liu, X. (2010). Instances of Soil and Crop Heavy Metal Contamination in China. Soil Sediment Contam. Int. J. 10 (5), 497–510. doi:10.1080/20015891109392
Feng, W., Guo, Z., Shi, L., Xiao, X., Han, X., Ran, H., et al. (2018). Distribution and Accumulation of Cadmium in Paddy Soil and Rice Affected by Pollutant Sources Control and Improvement Measures. Environ. Sci. 39 (1), 399–405. (in Chinese). doi:10.13227/j.hjkx.201706233
Gao, J., Lv, J., Wu, H., Dai, Y., and Nasir, M. (2018). Impacts of Wheat Straw Addition on Dissolved Organic Matter Characteristics in Cadmium-Contaminated Soils: Insights from Fluorescence Spectroscopy and Environmental Implications. Chemosphere 193, 1027–1035. doi:10.1016/j.chemosphere.2017.11.112
Hesterberg, D., Bril, J., and del Castilho, P. (1993). Thermodynamic Modeling of Zinc, Cadmium, and Copper Solubilities in a Manured, Acidic Loamy-Sand Topsoil. J. Environ. Qual. 22 (4), 681–688. doi:10.2134/jeq1993.00472425002200040008x
Hu, N., Li, Z., Huang, B., and Tao, C. (2004). Chemical Forms of Heavy Metals in Sewage-Irrigated Paddy Soil in Guixi City. J. Agro-Environ. Sci. 23 (4), 683–686. (in Chinese).
Kalbitz, K. (2003). Changes in Properties of Soil-Derived Dissolved Organic Matter Induced by Biodegradation. Soil Biol. Biochem. 35 (8), 1129–1142. doi:10.1016/s0038-0717(03)00165-2
Kalbitz, K., Solinger, S., Park, J.-H., Michalzik, B., and Matzner, E. (2000). Controls on the Dynamics of Dissolved Organic Matter in Soils: a Review. Soil Sci. 165, 277–304. doi:10.1097/00010694-200004000-00001
Kashem, M. A., and Singh, B. R. (2006). Transformations in Solid Phase Species of Metals as Affected by Flooding and Organic Matter. Commun. Soil Sci. Plant Analysis 35 (9-10), 1435–1456. doi:10.1081/css-120037556
Khodaverdiloo, H., Han, F. X., Hamzenejad Taghlidabad, R., Karimi, A., Moradi, N., and Kazery, J. A. (2020). Potentially Toxic Element Contamination of Arid and Semi-arid Soils and its Phytoremediation. Arid Land Res. Manag. 34 (4), 361–391. doi:10.1080/15324982.2020.1746707
Kidd, P. S., Domínguez-Rodríguez, M. J., Díez, J., and Monterroso, C. (2007). Bioavailability and Plant Accumulation of Heavy Metals and Phosphorus in Agricultural Soils Amended by Long-Term Application of Sewage Sludge. Chemosphere 66 (8), 1458–1467. doi:10.1016/j.chemosphere.2006.09.007
Kumar, V., Sharma, A., Kaur, P., Singh Sidhu, G. P., Bali, A. S., Bhardwaj, R., et al. (2019). Pollution Assessment of Heavy Metals in Soils of India and Ecological Risk Assessment: A State-Of-The-Art. Chemosphere 216, 449–462. doi:10.1016/j.chemosphere.2018.10.066
Laird, D. A., Fleming, P., Davis, D. D., Horton, R., Wang, B., and Karlen, D. L. (2010). Impact of Biochar Amendments on the Quality of a Typical Midwestern Agricultural Soil. Geoderma 158 (3-4), 443–449. doi:10.1016/j.geoderma.2010.05.013
Li, Z., Ma, Z., van der Kuijp, T. J., Yuan, Z., and Huang, L. (2014). A Review of Soil Heavy Metal Pollution from Mines in China: Pollution and Health Risk Assessment. Sci. Total Environ. 468-469, 843–853. doi:10.1016/j.scitotenv.2013.08.090
Liu, X., Tian, G., Jiang, D., Zhang, C., and Kong, L. (2016). Cadmium (Cd) Distribution and Contamination in Chinese Paddy Soils on National Scale. Environ. Sci. Pollut. Res. 23 (18), 17941–17952. doi:10.1007/s11356-016-6968-7
Lu, Y., Wassmann, R., Neue, H. U., and Huang, C. (2000). Dissolved Organic Carbon and Methane Emissions from a Rice Paddy Fertilized with Ammonium and Nitrate. J. Environ. Qual. 29 (6), 1733–1740. doi:10.2134/jeq2000.00472425002900060002x
Maes, A., Vanthuyne, M., Cauwenberg, P., and Engels, B. (2003). Metal Partitioning in a Sulfidic Canal Sediment: Metal Solubility as a Function of pH Combined with EDTA Extraction in Anoxic Conditions. Sci. Total Environ. 312 (1-3), 181–193. doi:10.1016/s0048-9697(03)00191-8
Maret, W., and Moulis, J.-M. (2013). “The Bioinorganic Chemistry of Cadmium in the Context of its Toxicity,” in Cadmium: From Toxicity to Essentiality. Editors A. Sigel, H. Sigel, and R. Sigel (Dordrecht: Springer), 1–29. doi:10.1007/978-94-007-5179-8_1
Mohamed, I., Ahamadou, B., Li, M., Gong, C., Cai, P., Liang, W., et al. (2010). Fractionation of Copper and Cadmium and Their Binding with Soil Organic Matter in a Contaminated Soil Amended with Organic Materials. J. Soils Sediments 10 (6), 973–982. doi:10.1007/s11368-010-0199-1
Mukwaturi, M., and Lin, C. (2015). Mobilization of Heavy Metals from Urban Contaminated Soils under Water Inundation Conditions. J. Hazard. Mater. 285, 445–452. doi:10.1016/j.jhazmat.2014.10.020
Nabulo, G., Young, S. D., and Black, C. R. (2010). Assessing Risk to Human Health from Tropical Leafy Vegetables Grown on Contaminated Urban Soils. Sci. Total Environ. 408 (22), 5338–5351. doi:10.1016/j.scitotenv.2010.06.034
National Soil Pollution Survey Bulletin (2014). Ministry of Ecology and Environment of the People's Republic of China. Available at: https://www.mee.gov.cn/gkml/sthjbgw/qt/201404/W020140417558995804588.pdf.
Nie, X., Duan, X., Zhang, M., Zhang, Z., Liu, D., Zhang, F., et al. (2019). Cadmium Accumulation, Availability, and Rice Uptake in Soils Receiving Long-Term Applications of Chemical Fertilizers and Crop Straw Return. Environ. Sci. Pollut. Res. 26 (30), 31243–31253. doi:10.1007/s11356-019-05998-y
Ok, Y. S., Usman, A. R. A., Lee, S. S., Abd El-Azeem, S. A. M., Choi, B., Hashimoto, Y., et al. (2011). Effects of Rapeseed Residue on Lead and Cadmium Availability and Uptake by Rice Plants in Heavy Metal Contaminated Paddy Soil. Chemosphere 85 (4), 677–682. doi:10.1016/j.chemosphere.2011.06.073
Richard, C., Guyot, G., Trubetskaya, O., Trubetskoj, O., Grigatti, M., and Cavani, L. (2008). Fluorescence Analysis of Humic-like Substances Extracted from Composts: Influence of Composting Time and Fractionation. Environ. Chem. Lett. 7 (1), 61–65. doi:10.1007/s10311-008-0136-3
Sauvé, S., Hendershot, W., and Allen, H. E. (2000). Solid-solution Partitioning of Metals in Contaminated Soils: Dependence on pH, Total Metal Burden, and Organic Matter. Environ. Sci. Technol. 34, 1125–1131. doi:10.1021/es9907764
Shan, Y., Cai, Z., Han, Y., Johnson, S. E., and Buresh, R. J. (2008). Organic Acid Accumulation under Flooded Soil Conditions in Relation to the Incorporation of Wheat and Rice Straws with Different C:N Ratios. Soil Sci. Plant Nutr. 54 (1), 46–56. doi:10.1111/j.1747-0765.2007.00218.x
Sharma, K., and Garg, V. K. (2018). Comparative Analysis of Vermicompost Quality Produced from Rice Straw and Paper Waste Employing Earthworm Eisenia fetida (Sav.). Bioresour. Technol. 250, 708–715. doi:10.1016/j.biortech.2017.11.101
Spaccini, R., Baiano, S., Gigliotti, G., and Piccolo, A. (2008). Molecular Characterization of a Compost and its Water-Soluble Fractions. J. Agric. Food Chem. 56 (3), 1017–1024. doi:10.1021/jf0716679
Strobel, B. W., Borggaard, O. K., Hansen, H. C. B., Andersen, M. K., and Raulund-Rasmussen, K. (2005). Dissolved Organic Carbon and Decreasing pH Mobilize Cadmium and Copper in Soil. Eur J Soil Sci. 56 (2), 189–196. doi:10.1111/j.1365-2389.2004.00661.x
Sun, T., Xu, Y., Sun, Y., Wang, L., Liang, X., and Zheng, S. (2021). Cd Immobilization and Soil Quality under Fe-Modified Biochar in Weakly Alkaline Soil. Chemosphere 280, 130606. doi:10.1016/j.chemosphere.2021.130606
Wang, S., Huang, D.-Y., Zhu, Q.-H., Zhu, H.-H., Liu, S.-L., Luo, Z.-C., et al. (2015). Speciation and Phytoavailability of Cadmium in Soil Treated with Cadmium-Contaminated Rice Straw. Environ. Sci. Pollut. Res. 22 (4), 2679–2686. doi:10.1007/s11356-014-3515-2
Weng, L., Temminghoff, E. J. M., Lofts, S., Tipping, E., and Van Riemsdijk, W. H. (2002). Complexation with Dissolved Organic Matter and Solubility Control of Heavy Metals in a Sandy Soil. Environ. Sci. Technol. 36, 4804–4810. doi:10.1021/es0200084
Xia, L., Wang, S., and Yan, X. (2014). Effects of Long-Term Straw Incorporation on the Net Global Warming Potential and the Net Economic Benefit in a Rice-Wheat Cropping System in China. Agric. Ecosyst. Environ. 197, 118–127. doi:10.1016/j.agee.2014.08.001
Xiao, R., Wang, P., Mi, S., Ali, A., Liu, X., Li, Y., et al. (2019). Effects of Crop Straw and its Derived Biochar on the Mobility and Bioavailability in Cd and Zn in Two Smelter-Contaminated Alkaline Soils. Ecotoxicol. Environ. Saf. 181, 155–163. doi:10.1016/j.ecoenv.2019.06.005
Xu, P., Sun, C.-X., Ye, X.-Z., Xiao, W.-D., Zhang, Q., and Wang, Q. (2016). The Effect of Biochar and Crop Straws on Heavy Metal Bioavailability and Plant Accumulation in a Cd and Pb Polluted Soil. Ecotoxicol. Environ. Saf. 132, 94–100. doi:10.1016/j.ecoenv.2016.05.031
Yan, F., Schubert, S., and Mengel, K. (1996). Soil pH Increase Due to Biological Decarboxylation of Organic Anions. Soil Biol. biochem. 28 (4-5), 617–624. doi:10.1016/0038-0717(95)00180-8
Yan, X., and Gong, W. (2010). The Role of Chemical and Organic Fertilizers on Yield, Yield Variability and Carbon Sequestration- Results of a 19-year Experiment. Plant Soil 331 (1-2), 471–480. doi:10.1007/s11104-009-0268-7
Yang, Q., Li, Z., Lu, X., Duan, Q., Huang, L., and Bi, J. (2018). A Review of Soil Heavy Metal Pollution from Industrial and Agricultural Regions in China: Pollution and Risk Assessment. Sci. Total Environ. 642, 690–700. doi:10.1016/j.scitotenv.2018.06.068
Yuan, C., Li, F., Cao, W., Yang, Z., Hu, M., and Sun, W. (2019). Cadmium Solubility in Paddy Soil Amended with Organic Matter, Sulfate, and Iron Oxide in Alternative Watering Conditions. J. Hazard. Mater. 378, 120672. doi:10.1016/j.jhazmat.2019.05.065
Yuan, C., Li, Q., Sun, Z., and Sun, H. (2021). Effects of Natural Organic Matter on Cadmium Mobility in Paddy Soil: A Review. J. Environ. Sci. 104, 204–215. doi:10.1016/j.jes.2020.11.016
Keywords: straw resources, Cd contamination, paddy soil, DOC, Cd solubility
Citation: Zuo W, Yi S, Chen Y, Huang G, Zhu X, Li Y, Gu C, Bai Y and Shan Y (2022) Utilization of Straw Resources May Affect the Speciation of Cd and Its Solubility in Cd-Contaminated Paddy Soil. Front. Environ. Sci. 10:933653. doi: 10.3389/fenvs.2022.933653
Received: 01 May 2022; Accepted: 08 June 2022;
Published: 07 July 2022.
Edited by:
Jiashuo Li, Shandong University, ChinaReviewed by:
Haiying Lu, Nanjing Forestry University, ChinaPengfu Hou, Jiangsu Academy of Agricultural Sciences, China
Zhenhua Zhang, University of Western Australia, Australia
Copyright © 2022 Zuo, Yi, Chen, Huang, Zhu, Li, Gu, Bai and Shan. This is an open-access article distributed under the terms of the Creative Commons Attribution License (CC BY). The use, distribution or reproduction in other forums is permitted, provided the original author(s) and the copyright owner(s) are credited and that the original publication in this journal is cited, in accordance with accepted academic practice. No use, distribution or reproduction is permitted which does not comply with these terms.
*Correspondence: Yuhua Shan, c2hhbnl1aHVhQG91dGxvb2suY29t