- 1College of Geographical Sciences and Planning, Ningxia University, Yinchuan, China
- 2China-Arab Joint International Research Laboratory for Featured Resources and Environmental Governance in Arid Regions, Yinchuan, China
- 3Engineering Research Center of Wolfberry in National Forestry and Grassland Administration, Yinchuan, China
- 4Henan Institute of Metrology, Zhengzhou, China
Goji berries (Lycium barbarum L.), widely planted in arid to semi-arid regions, are a functional resource characterized by a homology of medicine and food. Changing extensive water and fertilizer management practices to drip fertigation is one of the most cost-effective ways to achieve the sustainable development of the Goji berry industry. This study explores the effects of different fertilizer combinations on the soil organic carbon pool and L. barbarum yield under drip fertigation in Ningxia, northwestern China. A two-year field experiment (2017–2019) was conducted using different levels of drip nitrogen (40, 60, and 80 mg L−1) and phosphorus (10, 20, and 30 mg L−1) fertigation. Compared with traditional manual fertilization (control), soil organic carbon contents in the 0–20, 20–40, and 40–60 cm layers increased by 33.6–144.4, 39.6–136.8, and 14.0–73.6%, respectively, across all fertigation treatments. With increasing levels of fertigation, the easily oxidizable organic carbon content increased most prominently in the 0–20 cm soil layer and reached the highest value (538 mg kg−1) under treatment with 60 mg L−1 nitrogen plus 10 mg L−1 phosphorus. The microbial biomass carbon contents in the 20–60 cm soil layer was markedly higher under treatment with 60 mg L−1 nitrogen plus 30 mg L−1 phosphorus compared with other treatments. Fertigation increased the soil carbon pool management index and L. barbarum yield. The highest two-year average yield (13,890 kg ha−1) was obtained under treatment with 60 mg L−1 nitrogen plus 30 mg L−1 phosphorus. These findings suggest that drip fertigation with 60 mg L−1 nitrogen plus 30 mg L−1 phosphorus is the optimal practice for carbon sequestration and sustainable production of L. barbarum in arid regions.
Introduction
Soil organic carbon (SOC) is one of the most important carbon pools in the global carbon cycle. The use of rational management practices, such as irrigation, can increase the carbon pool in global agricultural soil by approximately 0.4–0.9 Pg C year−1, with a cumulative increase of 24–43 Pg C over a 50-year period (IPCC, 2007; Qi et al., 2014; Danso et al., 2015). According to its function, turnover time, and chemical attributes, the SOC pool can be divided into labile, slow, and inert fractions. Of these, labile organic carbon (LOC), including dissolved organic carbon (DOC), microbial biomass carbon (MBC), and easily oxidizable organic carbon (EOC), results in most of the dynamic changes in the SOC pool (Demessie et al., 2011; Wang et al., 2014). Although LOC accounts for only a small portion of SOC, it can reflect subtle changes in soil quality before responses of SOC to changes in agricultural management (Wang et al., 2017). As a sensitive indicator, LOC not only responds quickly to cultivation practices, but also plays a vital role in regulating the transformation of soil nutrients (Weil and Magdoff, 2004; Wang et al., 2017). Therefore, it is pivotal to closely monitor the dynamics of soil LOC fractions under agricultural management practices.
Goji berries in the genus Lycium (family Solanaceae) are a superior characteristic crop planted widely in the arid to semi-arid regions of Eurasia, Africa, and North and South America (Ili et al., 2020). In Ningxia (an arid region of northwestern China), Goji berry cultivation has become one of the major sources of income. Lycium barbarum L. is a unique green woody vegetable commonly found in Ningxia, with a homology of medicine and food. It is distinctly different from traditional Goji berries (Lycium chinense Mill.) in terms of cultivation techniques and nutrient requirements (Huang et al., 2003; Lai et al., 2010). Ningxia Province has the least water resources in all of China, with limited amounts of rainfall, surface water, and groundwater. During the L. barbarum growing season, irrigation is therefore essential, although it is often excessive, resulting in the unnecessary waste of water resources. Additionally, extensive fertilizer management practices are adopted in most areas of Ningxia, although neither the timing or rate of fertilization nor the type of fertilizer matches the nutrient requirements of L. barbarum. These irrational management practices not only cause a serious waste of resources, but also increase the risk of environmental pollution (Li X. H. et al., 2016; Wang, 2016; Wang et al., 2016). In order to achieve sustainable production in the Goji berry industry, cost-effective management practices therefore need to be applied, with the goal of improving soil quality, increasing crop productivity, and enhancing agricultural economic benefits.
Realizing the efficient utilization and protection of water resources is the common key foundation for China to promote the development of low-carbon technologies and environmental protection, cope with global climate change, and achieve the strategic goals of “carbon neutrality.” Drip fertigation is a new agricultural technology that integrates irrigation and fertilization to enhance water and fertilizer use efficiency, improve crop yield and quality, and increase economic profits. Drip fertigation has been found to reduce water usage by 40% compared with traditional flood irrigation and by 30% compared with spray irrigation (Danso et al., 2015; EI-Metwally et al., 2021). Furthermore, compared with traditional fertilization, drip fertigation has been found to increase both the yield and nitrogen use efficiency in tomatoes (Xing et al., 2015), while a positive role in terms of soil LOC content was also revealed, resulting in overall effects on soil water distribution and the carbon cycle (Singh and Singh, 1993; Qi et al., 2014). Given its advantages, drip fertigation has therefore become an important agricultural management practice with widespread applications, especially in semi-arid and arid regions (Zhang et al., 2009).
At present, drip fertigation is being gradually introduced into Goji berry cultivation in Ningxia. However, responses of the soil LOC pool to major changes in the fertilization approach in L. barbarum fields remain unknown. Additionally, a prerequisite to improving the fertilizer use efficiency and crop yield is the selection of an appropriate rate of fertilization based on the local soil characteristics and specific fertilizer demands. Many studies have document different fertilization rates based on the cultivation of traditional Goji berries or other herbaceous leafy vegetables (Kang et al., 2018), whereas few reports have documented the optimal water and fertilizer management practices for L. barbarum cultivation. In particular, the optimal rates of fertilization for drip fertigation in L. barbarum fields are unclear.
From the perspective of soil–crop systems, this study investigates the effects of different fertilizer combinations on the SOC pool (especially LOC fractions) and L. barbarum yield under drip fertigation. The fertilizer demands of L. barbarum grown in the arid region of Ningxia are also clarified during a two-year field experiment. The objectives of this study are to 1) evaluate the effects of different fertigation treatments on SOC sequestration and determine the responses of soil LOC fractions to major changes in the fertilization approach in L. barbarum fields and 2) explore the effects of different fertilizer combinations on Goji berry yield and identify the optimal fertilization rate for L. barbarum cultivation under drip fertigation. The results could be useful for rational agricultural management and sustainable production of L. barbarum in Ningxia and other arid regions.
Materials and Methods
Field Site Description
This study was conducted at the Goji Berry Demonstration Base (35°25′ N, 106°10′ E) of the Research Center of Engineering Technology for Goji Berry, State Forestry Administration of China. The study area is located in an inland arid region located in northwestern China. It has a warm temperate continental monsoon climate with large diurnal temperature variation. The mean annual temperature, precipitation, and evaporation are 8.5°C, ∼180 mm, and ∼1883 mm, respectively. The relative humidity is between 45 and 60%, the frost-free period lasts for 160–170 days, and the average annual sunshine duration ranges from 2,800 to 3,000 h. The soil in the study area is classified as aeolian sandy soil according to the US Department of Agriculture Textural Classification System. The basic properties of the 0–60 cm soil profile before the experiment are summarized in Table 1.
Experimental Design
The field experiment was commenced in March 2017 and lasted for 2 years. Six-year-old Goji berry plants (L. barbarum cv. Ningqi-9) showing consistent growth were selected from an area planted with spacing of 70 × 20 cm and density of 70,000 plants ha−1. Based on the growth requirements of L. barbarum and the nutrient status of local soil (rich in potassium, Table 1), the experiment used a 3 × 3 full factorial design with three levels of drip nitrogen fertigation (40, 60, and 80 mg L−1; N1–N3, respectively) and three levels of drip phosphorus fertigation (10, 20, and 30 mg L−1; P1–P3, respectively). Traditional manual fertilization with nitrogen, phosphorus, and potassium (1,402.5, 292.5, and 132 kg ha−1, respectively) was used as a control (CK). The fertilization rates used in the experiment were based on those previously reported for Solanacae crops, fruit, and vegetables in developed countries (e.g., Israel) (Hagin and Sneh, 2003; Wang et al., 2011) in combination with the soil characteristics in the study area. A total of 10 treatments were arranged in a randomized complete design, with three replicates per treatment. The plants of each treatment were grown in 20 × 5 m plots with five rows each. In the control treatment, Urea (N 46%), KH2PO4 (K2O 34%, P2O5 52%), and KNO3 (K2O 46%, N 14%) were divided evenly into five portions based on the annual fertilization rates and applied by broadcasting. One portion was applied in late March 2017 and the other four portions were applied as top-dressing once in mid-May, June, July, and August during the harvest stage, respectively. Annual fertilization rates of nitrogen, phosphorus, and potassium were 1,402.5, 292.5, and 132 kg ha−1, respectively. The same fertilization procedure was followed throughout the experiment.
Drip irrigation was carried out in each plot in consistent time periods and using a consistent volume of water. Irrigation was started after the soil thawed in late March and ended before the soil froze in November. In the control treatment, chemical fertilizers were applied by broadcasting. In the fertigation treatment, fertilizer solutions were formulated based on the designed nitrogen and phosphorus concentrations, with a potassium concentration of 40 mg L−1. The fertigation device consisted of a water source, a water meter, a hydraulic proportional fertilization pump, a drip irrigation pipe, and a water transmission and distribution pipeline. The proportional fertilization pump had an inlet and outlet diameter of 25 mm, a flow rate of 20–2500 L h−1, and a water pressure of 0.02–0.3 MPa. The built-in drip irrigation pipes had a diameter of 16 mm and wall thickness of 0.20 mm with a working pressure of 50–100 kPa, dripper spacing of 0.30 m, and rated flow of 2.0 L h−1. The fertilizer solutions were applied with water through the proportional fertilizer applicator throughout the growing season. Fertigation was initiated when the soil water content dropped to 80% of the field water-holding capacity, with average daily irrigation of 6 mm. Field management practices, such as weeding and pruning, were consistent across treatments.
Soil Sampling and Analysis
Soil sampling was conducted in all plots after harvest in September 2019. In each plot, soil samples (five each) were taken randomly from depths of 0–20, 20–40, and 40–60 cm. The samples from each depth were mixed thoroughly as composite samples and then transported in an ice box to the laboratory. Each sample was divided into two parts: one part was passed through 1-mm and 0.15-mm sieves after air-drying and manual removal of gravel and plant root residues for analysis of SOC and LOC contents, respectively; the other was passed through a 2-mm sieve and refrigerated for analysis of DOC, MBC, and EOC contents. The basic physicochemical properties of the soil samples were determined following standard testing methods (Bao, 2000). The SOC content was determined using the K2Cr2O7 oxidation–external heating method (Xiong et al., 2016) Soil DOC was extracted by mixing the soil sample with distilled water (1:5, w/v) and then shaking for 1 h on a shaker (25°C, 250 rpm) (Li et al., 2017). The carbon concentration in the extract was measured using a total organic carbon analyzer (Vario TOC; Elementar, Langenselbold, Germany). Furthermore, the MBC and EOC contents were determined using the chloroform fumigation–extraction method (Wang et al., 2010) and KMnO4 oxidation method (Li et al., 2019), respectively.
After stumping in spring, new shoots germinated at the base of the L. barbarum plants. Leaf buds were collected when these new shoots reached 15–20 cm long. The sampling region was free of lignification and the sample length was 8–10 cm. Sampling was conducted once every 5 to 7 days on average at fixed points (three points per plot). The area from which all plants were sampled was 10 m long. After collection, the samples were mixed and weighed to estimate the yield of L. barbarum.
Data Analyses
Carbon efficiency, as a sensitive indicator of carbon quality, was used to estimate carbon availability, which was then used to evaluate soil organic matter and soil fertility (Singh and Singh, 1993) as follows:
The carbon pool index (CPI) was used to indicate changes in SOC content caused by each experimental treatment, while the carbon pool activity (CPA) was used to indicate soil carbon instability. The carbon pool activity index (CPAI) was used to indicate carbon loss and its impact on carbon stability, while the carbon pool management index (CPMI) was used to evaluate changes in soil LOC fractions (Shen and Cao, 2000):
The sensitivity index (SI) was then used to indicate the sensitivity of soil LOC fractions in response to changes in soil management practices (Liang et al., 2012):
Statistical analyses were performed using SPSS 22.0 (IBM Corp., Armonk, NY, United States). Two-way analysis of variance, followed by the least significant difference test, was used to compare means between different treatments, and Pearson’s correlation coefficients were used to evaluate the relationship between Goji berry yield and soil LOC fractions. A p-value of less than 0.05 was considered statistically significant.
Results
Changes in Soil Organic Carbon Content Under Drip Fertigation
Drip fertigation with differing fertilizer combinations had differential effects on the SOC contents of each soil layer (Table 2). In the 0–60 cm soil profile, the SOC content tended to decrease with increasing soil depth; meanwhile, compared with the control (1.78–2.77 g kg−1), the SOC content in each layer increased under fertigation treatment (2.32–5.02 g kg−1). In the 10–20, 20–40, and 40–60 cm soil layers, the SOC contents under fertigation treatment increased by 33.6–144.4, 39.6–136.8, and 14.0–73.6%, respectively.
In the 0–20 cm soil layer, when a medium level (60 mg L−1) of nitrogen was applied, the SOC content showed an overall upward trend with increasing phosphorus application, and significant differences were detected between treatments (p < 0.05). When medium and high levels (10 and 20 mg L−1) of phosphorus were applied, a significant increase in SOC content was observed with increasing nitrogen application. The highest SOC content was observed under N3P3 treatment (6.77 g kg−1), with a considerable increase of 144.4% compared with the control. In the 20–60 cm soil layers, there was an evident plow pan effect on SOC across treatments compared with the 0–20 cm soil layer, with an overall downward trend with increasing soil depth. No significant differences were detected between treatments in terms of SOC content under increasing phosphorus application at the same nitrogen level and vice versa. However, the interaction between nitrogen and phosphorus had a significant effect on SOC content in the 0–40 cm soil layers (p < 0.05).
Contents of Soil Labile Organic Carbon Fractions Under Drip Fertigation
Overall, the EOC content decreased first and then increased with increasing soil depth, while the DOC and MBC contents gradually decreased toward deeper soil depths across all treatments (Table 2). In the 0–20 cm soil layer, when the same level of nitrogen was applied, the EOC contents gradually decreased with increasing phosphorus application, although values remained higher than the control except under N1P3 treatment. Under medium and low levels of phosphorus fertigation, no significant differences in soil EOC content were observed between different nitrogen levels. However, under high phosphorus fertigation, significant increases in the EOC content were observed with increasing nitrogen application (p < 0.05). Under a medium level of nitrogen fertigation, soil DOC contents increased significantly with increasing phosphorus application, while under high nitrogen fertigation, DOC contents decreased significantly with increasing phosphorus application, although the values under all three treatments were considerably higher than those of the control (p < 0.05). Under medium and low levels of nitrogen fertigation, the MBC contents increased significantly with increasing phosphorus application (p < 0.05), and was highest under N2P3 treatment, with a substantial increase of 428.6% compared with the control (p < 0.05).
Compared with the control, an increase in EOC, DOC, and MBC contents was observed in each soil layer under fertigation treatment (Table 2). In the 0–60 cm soil profile, the mean EOC content under fertigation treatment ranged from 328 to 406 mg kg−1, while the mean value of the control ranged from 203 to 219 mg kg−1. In general, the EOC content in each soil layer showed an overall upward trend with increasing levels of nitrogen and phosphorus, and this was most evident in the 0–20 cm soil layer. The EOC content was significantly higher under N2P1 treatment (538 mg kg−1) than all the remaining treatments, with an increase of 101.74% compared with the control (p < 0.05). The increase in DOC content in the 0–20 cm soil layer was most pronounced under N1P1 treatment, with an increase of 53.35% compared with the control. In the 40–60 cm soil layer, except under N2P2 and N2P3 treatments, the DOC content decreased compared with the control. The MBC content also decreased significantly with increasing soil depth under each treatment. In the 20–40 and 40–60 cm soil layers, the MBC contents under N2P3 treatment (76 and 33 mg kg−1, respectively) were significantly higher than those under the remaining treatments, with increases of 349.2 and 199.1% compared with the control, respectively (p < 0.05). The interaction between nitrogen and phosphorus had a significant effect on the DOC and MBC contents (p < 0.05), but not on the EOC content in the 0–60 cm soil profile.
Efficiency of Soil Labile Organic Carbon Fractions Under Drip Fertigation
The effects of each fertilizer combination on the efficiency of soil LOC under drip fertigation are shown in Figure 1. Overall, the efficiencies of soil EOC and DOC gradually increased with increasing soil depth, while the efficiency of soil MBC tended to decrease with deepening soil depth across all treatments. In the 0–20 cm soil layer, under high levels of nitrogen and phosphorus fertigation, the efficiency of soil EOC did not differ significantly between treatments compared with the control (Figure 1A). However, under medium and high levels of fertigation, the efficiency of soil DOC decreased remarkably under fertigation treatment compared with the control (p < 0.05, Figure 1B). Except under N2P3 and N3P1 treatment, the efficiency of soil MBC did not change significantly under fertigation treatment compared with the control (Figure 1C).
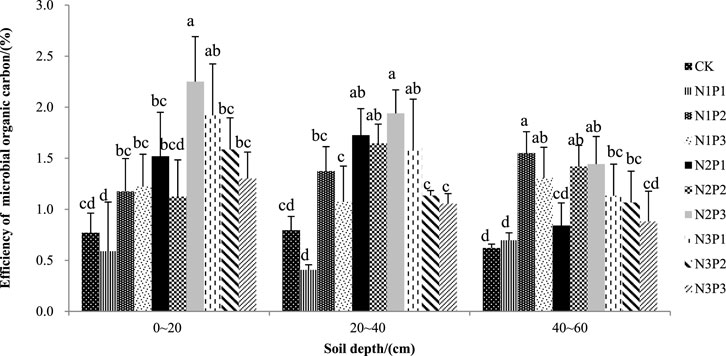
FIGURE 1. The efficiency of soil labile organic carbon under different fertigation treatments (N1–N3: 40, 60, and 80 mg L−1, respectively; P1–P3: 10, 20, and 30 mg L−1, respectively). (A) Efficiency of easily oxidized organic carbon; (B) Efficiency of dissolved organic carbon; (C) Efficiency of microbial organic carbon. Data are the mean ± standard error (n = 3). Different lowercase letters above the column indicate significant difference among treatments at the 0.05 significance level.
In the 20–40 cm soil layer, except under N3P1 treatment, there were no significant differences in the efficiency of soil EOC between the fertigation treatment and control. However, a significant difference in the efficiency of soil DOC was observed (p < 0.05). Under a medium level of fertigation, the efficiency of soil MBC increased significantly compared with the control, and the highest value was observed under N2P3 treatment. In the 40–60 cm soil layer, the efficiency of soil EOC generally increased under fertigation treatment compared with the control. The highest efficiency of soil EOC was achieved under N2P3 treatment, followed by N3P1 treatment, with increases of 91.0 and 65.7%, respectively, compared with the control. Under low levels of fertigation, the efficiency of soil DOC significantly differed from the control, while the efficiency of soil MBC increased by 11.7–132.3%. Overall, N2P3 and N1P2 treatment resulted in notable increases in the efficiency of soil LOC compared with the control.
Stability of Soil Labile Organic Carbon (LOC) Fractions Under Drip Fertigation
Compared with the control, the CPI values of total carbon in each soil layer were all greater than 1 under fertigation treatment (Table 3). In the 0–20 cm soil layer, CPI was highest under N3P3 treatment (2.44), while in the 20–60 cm soil layers, the highest CPIs were obtained under N3P2 treatment (2.37 and 1.74).
Similarly, the CPMI values of soil LOC tended to increase under fertigation treatment compared with the control. In the 0–20 cm soil layer, the CPMI was highest under N2P1 treatment (206), while in the 20–40 cm soil layer, CPMI values were in the order of N3P1 > N3P3 > N3P2 > N1P1 > N2P3 > N1P3 > N2P1 > N1P2 > N2P2 > control, with highest values under N3P1 treatment (257). In the 40–60 cm soil layer, the CPMI values tended to increase first and then decrease with increasing nitrogen and phosphorus levels under all treatments, but values remained significantly higher than those of the control, and the highest CPMI was observed under N2P3 treatment (276).
Sensitivity of Soil Labile Organic Carbon Fractions Under Drip Fertigation
The SI values of the soil LOC fractions (EOC, DOC, and MBC) under each treatment are presented in Figure 2. In general, the SI values of soil DOC and MBC tended to decrease gradually with increasing soil depth, while those of soil EOC showed no clear trend in the soil profile. The SI values of EOC were higher than those of DOC in the 20–40 cm and 40–60 cm soil layers, and except under N1P1 treatment, the SI values of MBC were higher than those of EOC and DOC in the entire 0–60 cm soil profile, with the highest value obtained under N2P3 treatment (348).
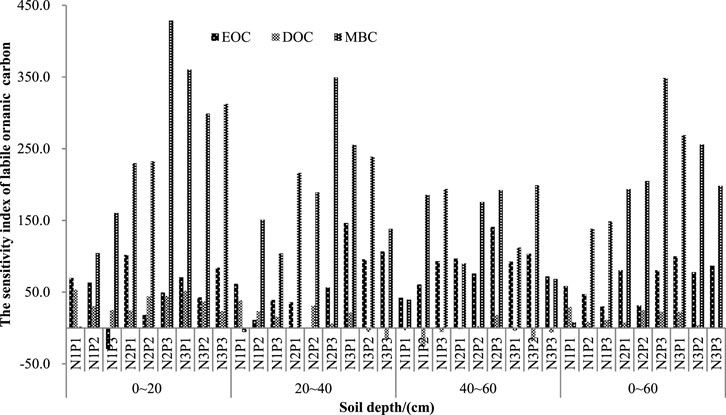
FIGURE 2. The sensitivity index of soil labile organic carbon under different fertigation treatments (N1–N3: 40, 60, and 80 mg L−1, respectively; P1–P3: 10, 20, and 30 mg L−1, respectively). EOC, easily oxidized organic carbon; DOC, dissolved organic carbon; MBC, microbial biomass carbon.
Crop Yield and Economic Profit Under Drip Fertigation
The effects of drip fertigation on L. barbarum yield differed between fertilizer treatments (Table 4). In 2018, neither the nitrogen level nor the phosphorus level had any significant effect on yield, and the highest yield was obtained under N2P3 treatment (14,326 kg ha−1). After the two-year field experiment, both the nitrogen and phosphorus levels exhibited significant effects on L. barbarum yield (p < 0.01). Under medium and low levels of nitrogen fertigation, the yield differed significantly between treatments with a high level of phosphorus (P3). Similarly, under medium and low levels of phosphorus fertigation, the yield differed significantly between treatments with a high level of nitrogen (N3). Of the 10 treatments, yield was highest under N2P3 followed by N3P3 treatment, with increases of 43.0 and 35.9%, respectively, compared with the control. The two-year average yield of L. barbarum from 2018 to 2019 increased under fertigation treatment compared with the control, and was highest under N2P3 treatment (14,110 kg ha−1) followed by N3P1 treatment (13,577 kg ha−1), with increases of 33.2 and 28.2%, respectively. Furthermore, Pearson’s correlation analysis revealed that L. barbarum yield was significantly correlated with the SOC (r = 0.73, p < 0.01) and EOC (r = 0.88, p < 0.05) contents.
Compared with the control, fertilizer input was largely reduced under fertigation treatment by 27.4–53.0% (Table 5). The output values of L. barbarum also increased substantially under fertigation treatment, especially with a high level of phosphorus application combined with low, medium, or high levels of nitrogen (N1P3, N2P3, and N3P3; 32.9, 42.9 and 35.8% increases, respectively). Overall, the output efficiency of L. barbarum was highest under N2P3 treatment.
Discussion
Effects of Different Fertilizer Combinations on Soil Organic Carbon Under Drip Fertigation
As an important component of the soil, SOC plays an essential role in soil fertility and agricultural productivity (Su et al., 2006). Meanwhile, as a vital carbon pool of terrestrial ecosystems, SOC plays a crucial role in balancing the global carbon cycle (Preethi et al., 2013; Qi et al., 2014; Kov et al., 2018). Some studies in the past have explored the effects of irrigation on SOC content and crop yield. Gillabel et al. (2007) found that irrigation can increase SOC content in the 0–20 cm soil layer by about 25% in semi-arid farmland. In addition, Qian et al. (2010) showed that irrigation has been identified as the management practice with the greatest potential for carbon sequestration, which can increase crop yields by increasing soil carbon input. A previous study revealed a positive correlation between the SOC content and carbon storage under fertigation (Hu et al., 2010). However, due to differing experimental conditions, no unified conclusion has yet been reached with regards to the effects of nitrogen fertilization on the soil carbon pool, especially the LOC fractions, in farmland.
Mockeviciene and Respsiene. (2021) suggested that long-term application of single chemical fertilizers was disadvantageous in maintaining and improving SOC. Furthermore, although nitrogen fertilization can promote the growth of plant roots, it also reduces the soil carbon/nitrogen ratio, thereby increasing the degradation of original organic carbon and fresh organic carbon, causing a reduction in the SOC content. In the present study, compared with traditional fertilization (control), drip fertigation caused an increase in the SOC content of the L. barbarum plots, while the degree of increase was dependent on the levels of nitrogen and phosphorus applied. A similar finding was reported by Lin (2019). The cumulative biological carbon sequestration was 7.36–22.41% higher than that of other irrigation modes. Therefore, open-field drip irrigation was considered to be an appropriate water-saving irrigation method to enhance carbon sequestration and emission reduction for farmland (Qiu et al., 2010). Our result also indicates that drip fertigation with nitrogen and phosphorus is more effective than traditional fertilization in increasing the SOC pool in the potassium-rich soil planted with L. barbarum.
Because the SOC content tended to decrease with increasing soil depth, the effects of different fertilizer combinations were more pronounced in the 0–20 and 20–40 cm soil layers in the L. barbarum plots. The increase in SOC under drip fertigation is thought to be the result of the following mechanisms.
During fertigation, the fertilizer solution, which contains relatively low nutrient concentrations, irrigates the roots in small amounts multiple times, thereby improving nutrient utilization by the plants, reducing nutrient losses, and substantially increasing the fertilizer use efficiency. Accordingly, these factors help optimize the water, air, and heat conditions for soil rhizosphere microorganisms and crop root activity, to some extent facilitating microbial proliferation and organic matter decomposition, thus contributing to the accumulation of SOC.
Effects of Different Fertilizer Combinations on Soil Labile Organic Carbon Under Drip Fertigation
Soil LOC is composed of EOC, DOC, and MBC (Zhang et al., 2009). Melero et al. (2009) previously reported that EOC is the most sensitive and reliable indicator of the effects of short- and long-term agricultural management practices on soil quality. In the present study, compared with the control, drip fertigation caused an increase in the EOC contents of different soil layers in the L. barbarum plots. Meanwhile, under different levels of nitrogen and phosphorus fertigation, the changes in soil EOC content varied with soil depth, similar to previous findings in wheat and corn fields (Aiziguli·Mulati et al., 2012). One plausible reason for this phenomenon is that soil microbial and enzymatic activities were enhanced by drip nitrogen and phosphorus fertigation, in turn accelerating the decomposition of EOC; however, the decomposition rate decreased with increasing soil depth. DOC, on the other hand, is easily affected by soil pH, microbial biomass and activity, and humidity (Zhang et al., 2016). Here, the soil DOC content was also remarkably higher under drip fertigation compared with the control. Overall, therefore, fertigation caused a considerable increase in the photosynthetic rate, promoting plant growth and increasing the input of plant litter and root exudates into the soil, thereby increasing the soil DOC content (Wu, 2012; Kennedy et al., 2013).
Soil MBC content is indicative of soil microbial activity and is closely related to soil fertility (Yan et al., 2007); however, the response of soil MBC content to nitrogen fertilization is inconsistent. For example, Yang et al. (2018) revealed a considerable increase in the soil MBC content under fertigation in sugar cane fields. In the present study, the MBC contents in each soil layer tended to increase first and then decrease with increasing levels of nitrogen and phosphorus fertigation, with highest values obtained under N2P3 treatment. This result suggests that rational application of nitrogen combined with phosphorus fertigation could increase the soil MBC content in the L. barbarum field. The application of chemical fertilizers increases the decomposition rate of SOC, reducing the carbon/nitrogen ratio of the soil and improving the soil environment, thereby enhancing soil microbial activity. However, with increasing soil depth, the positive effects of different fertigation treatments on soil MBC content were diminished, with differential changes in the three soil layers.
The CPMI of soil LOC can be used to indicate changes in, and the renewal of, soil quality, reflecting the effects of environmental conditions on SOC and LOC fractions. The level of CPMI sensitively reflects the effects of different fertilization approaches on the dynamics of the soil carbon pool (Xu et al., 2006). In the present study, different levels of nitrogen and phosphorus fertigation increased the CPI and CPMI of soil LOC in all three soil layers, although the effects varied with depth. Yue et al. (2014) also found an increase in the CPMI of the topsoil (0–20 cm) of cotton fields under drip fertigation. This increase suggests that fertigation practice plays a role in improving soil fertility. The increases in the SOC content and LOC fractions are beneficial in terms of the CPMI of organic carbon, thereby increasing the SOC pool. However, after the application of a certain proportion of fertilizers, the CPMI of soil LOC showed no further increases, and instead began to decrease. The optimal fertilization rates under drip fertigation therefore require further investigation in terms of local soil characteristics and the fertilizer demands of specific crops.
The efficiency of soil LOC refers to the ratio of soil LOC to soil SOC. A previous study revealed that the higher the efficiency of soil LOC fractions, the higher the availability of soil carbon (Li X. Y. et al., 2016). In the present study, compared with the control, both N2P3 and N1P2 treatments effectively increased the efficiencies of soil EOC, DOC, and MBC in the L. barbarum field. This effect may be attributed to the input of chemical fertilizers, which facilitate microbial activity and thereby accelerate the accumulation of LOC in the soil (Wu, 2012). Moreover, our results also revealed a general decrease in the SI values of soil DOC and MBC with increasing soil depth under all treatments. This suggests that soil DOC and MBC contents reflect the effects of nitrogen and phosphorus fertigation on the LOC fractions in the surface soil. The SI values of MBC were generally higher than those of EOC and DOC in all three soil layers, suggesting that the soil MBC content effectively reflects the effects of fertigation on SOC in the study area.
Effects of Different Fertilizer Combinations on Goji Berry Yield Under Drip Fertigation
Most farmers use excessive fertilization and irrigation, showing high soil moisture fluctuations and eutrophication (Wang et al., 2021). Drip fertigation not only enables reducing total irrigation water supply and fertilizer application rates, but also decreases nitrogen leaching and CO2 emission (Zhao et al., 2021). It is an environmentally friendly, effective modern agricultural technique that achieves high-quality, high-yielding crop production and efficient resource utilization (Yang et al., 2014). In Ningxia, farmers tend to apply excessive water and fertilizers (mainly nitrogen and phosphorus) during the production and management of L. barbarum. The average annual fertilization rates of nitrogen and phosphorus applied by broadcasting are 1,402.5 and 292.5 kg ha−1, both of which are much higher than the levels used in fertigation. The excessive use of fertilizers may lead to an unbalanced soil nutrient status, which, in turn, results in relatively low nutrient use efficiency and crop productivity (Zhang et al., 2021; Zhong et al., 2022). Indeed, compared with traditional manual fertilization, the two-year average yield of L. barbarum increased under drip fertigation, and the input-output ratio increased by an average of 108.8% in 2019. These results suggest that drip fertigation is a useful practice in Goji berry cultivation, increasing water and fertilizer use efficiency, as well as the yield and overall income.
The two-year average yield of L. barbarum reached its highest value under medium-to-high levels of nitrogen and phosphorus fertigation. Given the significant positive correlations of L. barbarum yield and SOC, EOC, and MBC contents, nitrogen and phosphorus fertigation improved the yield of L. barbarum by increasing the SOC pool in the study area. Overall, N2P3 treatment resulted in the highest yield, and this could be attributed to the higher SOC content, LOC fractions, and SI values compared with the remaining treatments. The experimental results suggest that drip fertigation with 60 mg L−1 nitrogen fertilizer plus 30 phosphorus fertilizer is the optimal management practice for cultivation of L. barbarum in the study area in terms of improving yield and reducing fertilizer costs.
Conclusion
There has been very little, if any, field investigations on the SOC distribution and sequestration potential or optimal water and fertilizer management practices for L. barbarum cultivation under drip fertigation with different fertilizer combinations. Based on the two-year experimental study, our data indicate that, compared with traditional manual fertilization, drip fertigation with different fertilizer combinations of nitrogen and phosphorus improved the SOC contents and altered their distribution in the surface and deep soil layers treatments. Especially for the LOC fractions, drip fertigation tended to increase the EOC, DOC, and MBC contents in each soil layer. Additionally, different fertigation treatments increased the changes in soil LOC and the overall yield of L. barbarum. On the whole, drip fertigation with 60 mg L−1 nitrogen fertilizer plus 30 mg L−1 phosphorus fertilizer is deemed optimal for improving organic carbon sequestration and increasing L. barbarum yield in the study area. Long-term comprehensive evaluation is now needed to verify the applicability of this sustainable management practice in the production of L. barbarum on arid land in northwestern China and other similar settings.
Data Availability Statement
The original contributions presented in the study are included in the article/supplementary material, and further inquiries can be directed to the corresponding authors.
Author Contributions
The work was designed by FW, XN, and JY; field work was carried out by XN, FW, and JY; soil physicochemical analyses was performed by FW, WL, and YL; data were analyzed by JY, FW, XN, and WL; the manuscript was drafted by FW and revised by XN and JY.
Funding
This research was financially supported by the National Natural Science Foundation of China (Grant Nos. 42067022 and 41761066), the Natural Science Foundation of Ningxia (Grant Nos. 2021AAC03528 and 2022AAC03024), and the Technology Planning Project of Administration for Market Regulation Henan Province (2020sj15).
Conflict of Interest
The authors declare that the research was conducted in the absence of any commercial or financial relationships that could be construed as a potential conflict of interest.
Publisher’s Note
All claims expressed in this article are solely those of the authors and do not necessarily represent those of their affiliated organizations, or those of the publisher, the editors and the reviewers. Any product that may be evaluated in this article, or claim that may be made by its manufacturer, is not guaranteed or endorsed by the publisher.
References
Aiziguli·Mulati, , Tong, Y. A., Yang, X. L., and Ma, H. Y. (2012). Effect of Different Fertilization on Soil Organic Carbon and its Fraction in Farmland. Chin. J. Soil Sci. 43, 1461–1466. doi:10.19336/jcnki.trtb.2012.06.032
Bao, S. D. (2000). Soil Agricultural Chemical Analysis. 3rd ed. Beijing: China Agricultural Press, 265–267.
Demessie, A., Singh, B. R., and Lal, R. (2011). Soil Carbon and Nitrogen Stocks under Plantations in Gambo District, Southern Ethiopia. J. Sustain. For. 30, 496–517. doi:10.1080/10549811.2010.550547
El–Metwally, I., Geries, L., and Saudy, H. (2021). Interactive Effect of Soil Mulching and Irrigation Regime on Yield, Irrigation Water Use Efficiency and weeds of Trickle-Irrigated Onion. Archives Agron. Soil Sci. 68, 1103–1116. doi:10.1080/03650340.2020.1869723
Gillabel, J., Denef, K., Brenner, J., Merckx, R., and Paustian, K. (2007). Carbon Sequestration and Soil Aggregation in Center-Pivot Irrigated and Dryland Cultivated Farming Systems. Soil Sci. Soc. Am. J. 71, 1020–1028. doi:10.2136/sssaj2006.0215
Hagin, J., and Sneh, M. A. (2003). Fertigation Fertilization through Irrigation. IPI Research Topics No. 23. Basel, Switzerland: International Potash Institute, 72.
Hu, C., Qiao, Y., Li, S.-L., Chen, Y.-F., and Liu, G.-J. (2010). Vertical Distribution and Storage of Soil Organic Carbon under Long-Term Fertilization. Chin. J. Eco-Agriculture 18, 689–692. doi:10.3724/SP.J.1011.2010.00689
Huang, G. J., Wang, G. Y., Zhang, D. N., He, R. C., Ma, H. S., and Hu, J. S. (2003). Introduction and Cultivation Techniques of Large Leaf Wolfberry for Vegetable. Jiangsu. J. For. Sci. Tech. 30, 34–35. doi:10.3969/j.issn.1001-7380.2003.04.01310.1023/a:1026392422995
Ilić, T., Dodevska, M., Marčetić, M., Božić, D., Kodranov, I., and Vidović, B. (2020). Chemical Characterization, Antioxidant and Antimicrobial Properties of Goji Berries Cultivated in Serbia. Foods 9, 1614. doi:10.3390/foods9111614
IPCC (2007). Climate Change 2007: Mitigation of Climate Change. Contribution of Working Group III to the Fourth Assessment Report of the Intergovernmental Panel on Climate Change. Cambridge, UK and New York: Cambridge University Press.
Kang, C., Yang, L., Wang, H., Nan, X. X., Wang, R., and Sun, Q. (2018). Effects of the Ratio of Nitrogen and Phosphorus Concentrations in Drip Fertigation on Yield and Yield Components of Chinese Wolfberry. J. Irr. Drain. 37, 26–30. doi:10.13522/j.cnki.ggps.2017.0674
Kennedy, T. L., Suddick, E. C., and Six, J. (2013). Reduced Nitrous Oxide Emissions and Increased Yields in California Tomato Cropping Systems under Drip Irrigation and Fertigation. Agric. Ecosyst. Environ. 170, 16–27. doi:10.1016/j.agee.2013.02.002
Kov, R., Camps-Arbestain, M., Calvelo Pereira, R., Suárez-Abelenda, M., Shen, Q., Garbuz, S., et al. (2018). A Farm-Scale Investigation of the Organic Matter Composition and Soil Chemistry of Andisols as Influenced by Land Use and Management. Biogeochemistry 140, 65–79. doi:10.1007/s10533-018-0473-7
Lai, Z. F., Zhang, S. P., Wu, S. J., and Lin, G. R. (2010). The Growth Characteristics and Nutritional Quality Analysis to Lycium Chinensis. Chin. J. Trop. Crops. 31, 1706–1709. doi:10.1080/00949651003724790
Li, J., Li, H., Zhang, Q., Shao, H., Gao, C., and Zhang, X. (2019). Effects of Fertilization and Straw Return Methods on the Soil Carbon Pool and CO2 Emission in a Reclaimed Mine Spoil in Shanxi Province, China. Soil Tillage Res. 195, 104361. doi:10.1016/j.still.2019.104361
Li, T. K., Guo, Z. L., Kou, C. L., Lv, J. L., Zhang, X. N., and Yang, X. L. (2017). Effects of Extraction Conditions on the Test Results of Soil Dissolved Organic Carbon. Ecol. Environ. Sci. 26, 878–1883. doi:10.16258/j.cnki.1674-5906.2017.11.00810.15244/pjoes/65360
Li, X. H., Guo, H. H., Zhu, Z. L., Dong, H. Y., Yang, L. P., and Zhang, X. J. (2016a). Effects of Different Straw Return Modes on Contents of Soil Organic Carbon and Fractions of Soil Active Carbon. Trans. Chin. Soc. Agric. Eng. 32, 130–135. doi:10.11975/j.issn.1002-6819.2016.09.018
Li, X. Y., Wang, S. D., Ke, Y., Luo, J. H., Chen, X. Q., and Zhang, X. J. (2016b). Characteristics of Soil Nutrients and Present Situation of Fertilization in the Major Wolfberry Producing Areas of Ningxia. Agr. Res. Arid. areas. 34, 113–118. doi:10.7606/j.issn.1000-7601.2016.02.18
Liang, Q., Chen, H., Gong, Y., Fan, M., Yang, H., Lal, R., et al. (2012). Effects of 15 Years of Manure and Inorganic Fertilizers on Soil Organic Carbon Fractions in a Wheat-Maize System in the North China Plain. Nutr. Cycl. Agroecosyst 92, 21–33. doi:10.1007/s10705-011-9469-6
Lin, L. M. (2019). Effects of Nitrogen Application and Plant Interaction on Decomposition of Soil Organic Matter. Lanzhou: Lanzhou University. [master's thesis].
Melero, S., López-Garrido, R., Murillo, J. M., and Moreno, F. (2009). Conservation Tillage: Short- and Long-Term Effects on Soil Carbon Fractions and Enzymatic Activities under Mediterranean Conditions. Soil Tillage Res. 104, 292–298. doi:10.1016/j.still.2009.04.001
Mockeviciene, I., Repsiene, R., Amaleviciute-Volunge, K., Karcauskiene, D., Slepetiene, A., and Lepane, V. (2021). Effect of Long-Term Application of Organic Fertilizers on Improving Organic Matter Quality in Acid Soil. Archives Agron. Soil Sci. 2021, 1–13. doi:10.1080/03650340.2021.1875130
Danso, E., Abenney-Mickson, S., Sabi, E. B., Plauborg, F., Abekoe, M., Kugblenu, Y. O., et al. (2015). Effect of Different Fertilization and Irrigation Methods on Nitrogen Uptake, Intercepted Radiation and Yield of Okra (Abelmoschus Esculentum L.) Grown in the Keta Sand Spit of Southeast Ghana. Agric. Water Manag. 147, 34–42. doi:10.1016/j.agwat.2014.07.029
Preethi, B., Poorniammal, R., Balachandar, D., Karthikeyan, S., Chendrayan, K., Bhattacharyya, P., et al. (2013). Long-term Organic Nutrient Managements Foster the Biological Properties and Carbon Sequestering Capability of a Wetland Rice Soil. Archives Agron. Soil Sci. 59, 1607–1624. doi:10.1080/03650340.2012.755260
Qi, Y. C., Guo, S. F., Dong, Y. S., Peng, Q., Jia, J. Q., Cao, C. C., et al. (2014). Advances in Research on the Effects of Irrigation on the Greenhouse Gases Emission and Soil Carbon Sequestration in Agro-Ecosystem. Sci. Agric. Sin. 47, 1764–1773. doi:10.3864/j.issn.0578-1752.2014.09.011
Qian, Y., Follett, R. F., and Kimble, J. M. (2010). Soil Organic Carbon Input from Urban Turfgrasses. Soil Sci. Soc. Am. J. 74, 366–371. doi:10.2136/sssaj2009.0075
Qiu, S. J., Ju, X. T., Ingwersen, J., Qin, Z. C., Li, L., Streck, T., et al. (2010). Changes in Soil Carbon and Nitrogen Pools after Shifting from Conventional Cereal to Greenhouse Vegetable Production. Soil Tillage Res. 107, 80–87. doi:10.1016/j.still.2010.02.006
Shen, H., and Cao, Z. H. (2000). Study on Soil C Pool Management Index of Different Farmland Ecosystems. Acta. Ecol. Sin. 20, 663–668. doi:10.11849/zrzyxb.1999.03.003
Singh, H., and Singh, K. P. (1993). Effect of Residue Placement and Chemical Fertilizer on Soil Microbial Biomass under Tropical Dryland Cultivation. Biol. Fert. Soils. 16, 275–281. doi:10.1007/BF00369304
Su, Y.-Z., Wang, F., Suo, D.-R., Zhang, Z.-H., and Du, M.-W. (2006). Long-term Effect of Fertilizer and Manure Application on Soil-Carbon Sequestration and Soil Fertility under the Wheat-Wheat-Maize Cropping System in Northwest China. Nutr. Cycl. Agroecosyst 75, 285–295. doi:10.1007/s10705-006-9034-x
Wang, D., Geng, Z. C., She, D., He, W. X., and Hou, L. (2014). Vertical Distribution of Soil Active Carbon and Soil Organic Carbon Storage under Different Forest Types in the Qinling Mountains. Ying Yong Sheng Tai Xue Bao 25, 1569–1577. doi:10.13287/j.1001-9332.20140415.014
Wang, F., Weil, R. R., and Nan, X. (2017). Total and Permanganate-Oxidizable Organic Carbon in the Corn Rooting Zone of US Coastal Plain Soils as Affected by Forage Radish Cover Crops and N Fertilizer. Soil Tillage Res. 165, 247–257. doi:10.1016/j.still.2016.08.022
Wang, H., Wang, X. D., and Zhao, S. W. (2011). Distribution of Soil Water and Available Potassium under Drip Fertigation. Chin. J. Soil Sci. 42, 27–32. doi:10.19336/j.cnki.trtb.2011.01.006
Wang, K. (2016). Study on Efficient Cultivation Technology of Water and Fertilizer Integration of Lycium Barbarum in Arid Zone of Central Ningxia. Yinchuan: Ningxia University. Master Dissertation [master's thesis]. doi:10.7666/d.Y3109180
Wang, R., Wang, W., Wang, Y. L., Wang, J. T., and Si, G. Y. (2016). Effect of Nitrogen Fertilizer Application on the Accumulation of NPK and Yield for Leaf Wolfberry. Nor. Hor. 160–163. doi:10.11937/bfyy.201611042
Wang, Y., Dannenmann, M., Lin, S., Lv, H., Li, G., Lian, X., et al. (2021). Improving Soil Respiration while Maintaining Soil C Stocks in Sunken Plastic Greenhouse Vegetable Production Systems - Advantages of Straw Application and Drip Fertigation. Agric. Ecosyst. Environ. 316, 107464. doi:10.1016/j.agee.2021.107464
Wang, Y., Ruan, H. H., Huang, L. L., and Feng, Y. Q. (2010). Soil Labile Organic Carbon of Different Land Use Types in a Reclaimed Land Area of Taihu Lake. Chin. J. Eco. 29, 741–748. doi:10.3969/j.issn.1000-2006.2010.05.024
Weil, R. R., and Magdoff, F. (2004). “Significance of Soil Organic Matter to Soil Quality and Health,” in Soil Organic Matter in Sustainable Agriculture. Editors F. Magdoff, and R. R. Weil (Boca Raton: CRC Press), 1–43. doi:10.1201/9780203496374.ch1
Wu, Y. (2012). The Effect of Tillage Systems on Organic Carbon Fractions in a Purple Paddy Soil. Chongqing: Southwest University. [master's thesis]. doi:10.5846/stxb201106300981
Xing, Y. Y., Zhang, F. C., Zhang, Y., Li, J., Qiang, S. C., and Wu, L. F. (2015). Effect of Irrigation and Fertilizer Coupling on Greenhouse Tomato Yield, Quality, Water and Nitrogen Utilization under Fertigation. Sci. Agric. Sin. 48, 713–726. doi:10.3864/j.issn.0578-1752.2015.04.09
Xiong, D., Shi, P., Zhang, X., and Zou, C. B. (2016). Effects of Grazing Exclusion on Carbon Sequestration and Plant Diversity in Grasslands of China-A Meta-Analysis. Ecol. Eng. 94, 647–655. doi:10.1016/j.ecoleng.2016.06.124
Xu, M. G., Yu, R., Sun, X. F., Liu, H., Wang, B. R., and Li, J. M. (2006). Effects of Long-Term Fertilization on Labile Organic Matter and Carbon Management Index (CMI) of the Typical Soils of China. Plant Nutr. Fert. Sci. 12, 459–465. doi:10.11674/zwyf.2006.0401
Yan, D., Wang, D., and Yang, L. (2007). Long-term Effect of Chemical Fertilizer, Straw, and Manure on Labile Organic Matter Fractions in a Paddy Soil. Biol. Fertil. Soils 44, 93–101. doi:10.1007/s00374-007-0183-0
Yang, X. H., Yan, C. M., Zhang, J. Z., and Shi, W. Q. (2014). The Analysis of Advantages and Disadvantages of Fertigation Technology and Development Strategies in China. J. Agric. 4, 76–80. doi:10.3969/j.issn.1007-7774.2014.01.019
Yang, X. Y., Jiang, D. H., Yang, K. R., Huang, Z. G., and Huang, K. (2018). Effects of Water and Fertilizer Integration on Soil Microbial Biomass Carbon, Nitrogen and Enzyme Activities in Sugarcane. Chin. J. Soil Sci. 49, 889–896. doi:10.19336/j.cnki.trtb.2018.04.19
Yue, H. J., Tao, R., and Chu, G. X. (2014). Response of Soil Organic Carbon Fraction to Different Ratios of Organic/Inorganic Fertilizer Application in Cotton Field. Xinjiang Agri. Sci. 51, 1630–1637. doi:10.6048/j.issn.1001-4330.2014.09.010
Zhang, L., Zhang, W. J., Xu, M. G., Cai, Z. J., Peng, C., Liu, B. R., et al. (2009). Effects of Long-Term Fertilization on Change of Labile Organic Carbon in Three Typical Upland Soils of China. Sci. Agric. Sin. 42, 1646–1655. doi:10.3864/j.issn.0578-1752.2009.05.018
Zhang, Q., Li, T., Yin, Y., Ying, H., Cui, Z., and Zhang, F. (2021). Targeting Hotspots to Achieve Sustainable Nitrogen Management in China's Smallholder-Dominated Cereal Production. Agronomy 11, 557. doi:10.3390/agronomy11030557
Zhang, X., Sun, N., Wu, L., Xu, M., Bingham, I. J., and Li, Z. (2016). Effects of Enhancing Soil Organic Carbon Sequestration in the Topsoil by Fertilization on Crop Productivity and Stability: Evidence from Long-Term Experiments with Wheat-Maize Cropping Systems in China. Sci. Total Environ. 562, 247–259. doi:10.1016/j.scitotenv.2016.03.193
Zhao, Y., Lv, H., Qasim, W., Wan, L., Wang, Y., Lian, X., et al. (2021). Drip Fertigation with Straw Incorporation Significantly Reduces N2O Emission and N Leaching while Maintaining High Vegetable Yields in Solar Greenhouse Production. Environ. Pollut. 273, 116521. doi:10.1016/j.envpol.2021.116521
Zhong, Y. J., Liu, K. L., Ye, C., Huang, S. S., Du, J. X., and Chen, J. Z. (2022). Differential Grain Yields and Soil Organic Carbon Levels between Maize and Rice Systems of Subtropical Red Soil in Response to Long-Term Fertilizer Treatments. Eurasian. Soil Sci. 55, 251–261. doi:10.1134/S1064229322020156
Keywords: arid, drip fertigation, labile carbon, soil management, production
Citation: Wang F, Li W, Lin Y, Nan X and Yuan J (2022) Soil Organic Carbon Pool and the Production of Goji Berry (Lycium barbarum L.) as Affected by Different Fertilizer Combinations Under Drip Fertigation. Front. Environ. Sci. 10:933124. doi: 10.3389/fenvs.2022.933124
Received: 30 April 2022; Accepted: 07 June 2022;
Published: 22 July 2022.
Edited by:
Yongsheng Wang, Institute of Geographic Sciences and Natural Resources Research (CAS), ChinaCopyright © 2022 Wang, Li, Lin, Nan and Yuan. This is an open-access article distributed under the terms of the Creative Commons Attribution License (CC BY). The use, distribution or reproduction in other forums is permitted, provided the original author(s) and the copyright owner(s) are credited and that the original publication in this journal is cited, in accordance with accepted academic practice. No use, distribution or reproduction is permitted which does not comply with these terms.
*Correspondence: Xiongxiong Nan, nanxiong0820@163.com; Jingjing Yuan, 15538851301@163.com
†These authors have contributed equally to this work