- 1School of Municipal and Environmental Engineering, Shandong Jianzhu University, Jinan, China
- 2Shandong Guochen Industrial Group Co., Ltd, Jinan, China
To improve the efficiency of nitrogen removal from pharmaceutical wastewater, wastewater from traditional Chinese medicine was treated in an anaerobic sequencing batch reactor (ASBR) combined with a modified sequencing batch biofilm reactor (SBBR). The chemical oxygen demand (COD) and total nitrogen (TN) contents were 3,750 ± 50 mg/L and 210 ± 10 mg/L, respectively. After 99 days of start-up and domestication, the COD, NH4+-N, and TN contents in the effluent were 230 ± 10 mg/L, 1 ± 0.5 mg/L, and 5 ± 3 mg/L, respectively, and the removal efficiencies reached more than 93.5, 99, and 96%, respectively. Among these results, the COD removal efficiency in traditional Chinese medicine wastewater with an ASBR reached more than 85%, and the effluent and raw water were mixed to adjust the C/N ratio in the SBBR influent. The initial operation mode of the improved SBBR was anaerobic–aerobic–anoxic. When the C/N ratio in the influent was adjusted to 5, the simultaneous nitrification and denitrification (SND) in the aerobic section was gradually enhanced, and the endogenous denitrification (ED) in the anoxic section gradually decreased. In conclusion, deep denitrification of the system was achieved only through SND, and the running time of the cycle was shortened from the initial 24 to 4.6 h. High-throughput sequencing analysis showed that the relative abundances of Bacteroidetes and Proteobacteria in the system were 39.69 and 37.34%, respectively. The content of Firmicutes with denitrification in the system was also high, accounting for 5.17%. At the genus level, the bacteria with denitrification functions in the system were mainly Thauera and unidentified_Sphingobacteriales, accounting for 5.67 and 1.66% of the system, respectively. In addition, there was heterotrophic nitrification–aerobic denitrification (HN-AD) activated in the system, including Denitratisoma, Paracoccus, and Pseudomonas. The total relative abundance of these bacteria was 0.612%. Their existence may be one of the reasons for the good effect of SND in this system.
1 Introduction
In recent years, improved living standards in China have gradually increased the recognition of traditional Chinese medicine. As a result, increased application of traditional Chinese medicine products has been observed, resulting in a corresponding enhancement in wastewater discharge. Traditional Chinese medicine wastewater is characterized by having a complex composition, being highly biodegradable, and having high organic matter and total nitrogen (TN) contents. The economic impact and regulation of TN contents in pharmaceutical wastewater have become an important and challenging topic in the area because of heightened stringent national emission standards.
Existing treatment methods of pharmaceutical wastewater include physical adsorption, chemical advanced oxidation, and biodegradation (Zou et al., 2020). Among them, the biological method is a popular approach because of its low costs and low production of secondary pollutants (Adishkumar et al., 2012). Anaerobic ammonium oxidation (ANAMMOX), sequencing batch reactors (SBRs), upflow anaerobic sludge blanket, aerobic granular sludge sequencing bioreactor (GSBR), membrane bioreactor (MBR), constructed wetland and various combined processes are the main methods used to date. An ANAMMOX process with an integrated biofilm-activated sludge system was effective for treating pharmaceutical wastewater with a high chemical oxygen demand (COD) concentration (1,000 mg/L), and TN removal loading rate and efficiency were 1.0 kg/m3/d and 75.2%, respectively (Zuo et al., 2020). Previous studies have analyzed the concentrations of pharmaceuticals and their main metabolites in the liquid and solid phases of activated sludge from SBR and MBR using liquid chromatography–tandem mass spectrometry (LC–MS/MS). It was found that microbial pollutants accumulated in bacterial cells, and prolonging the hydraulic retention time promoted the biodegradation of pollutants (Kruglova et al., 2016). Aerobic GSBR, using predominately Proteobacteria and Bacteroidetes (Zhao et al., 2015), also removed the pharmaceuticals and personal care products from wastewater. Anaerobic technology is more suitable for pharmaceutical wastewater with high COD. Because of the simple operation, low energy consumption, low sludge production, and efficient removal of organic matter, anaerobic sequencing batch reactors (ASBRs) were widely used in wastewater treatment (Cheong and Hansen, 2008; Oliveira et al., 2010). Reports have shown that when ASBRs can be used to treat beer wastewater with an organic loading rate between 1.5 and 5.0, the removal efficiency of COD reached 90% (Shao et al., 2008). The realization of efficient removal of organic matter and ammonia nitrogen has spurred researchers to adopt integrated systems of anaerobic and aerobic treatment using a combination of ASBRs and SBRs (Bolonhesi et al., 2021). Wang showed that using a combined ASBR and modified SBR process generated increased the TN and COD removal efficiencies to 95 and 90% from treated landfill leachate with influent NH4+-N and COD contents of 1,100 and 6,000 mg/L, respectively (Wang et al., 2013). Simultaneous nitrification and denitrification (SND) and endogenous denitrification (ED) shortened the reaction time and improved the utilization of carbon sources. Real time control of the nitrification time combined with an anaerobic/aerobic/anoxic (A/O/A) operation mode completely utilized the carbon sources for deep denitrification. Furthermore, the addition of fillers in an SBR acts as attachment sites for microorganisms and creates a more stable film hanging environment. When the wastewater flows through the filler, the filler and the formed biofilms intercept and treat the organic matter, promoting the suspension of particles in the wastewater and increasing the diversity of the microbial growth in the environment. Previous studies also found that modification of the sequencing batch biofilm reactor (SBBR) process can improve the nitrogen removal capacity of SND and ED and reduce the reaction time (Wang, 2021).
Herein, we propose the following: 1) to explore the combined process of ASBR and SBBR to realize the efficient removal of organic matter and TN from pharmaceutical wastewater; 2) to realize SND, shorten the reaction time, and improve the reaction efficiency through modified SBBR process; and 3) to discuss the composition of microbial communities related to nitrogen removal in the system via high-throughput sequencing.
2 Materials and Methods
2.1 Pharmaceutical Wastewater and Seed Sludge
The pharmaceutical wastewater was collected from a traditional Chinese medicine granule pharmaceutical factory in Zaozhuang City, Shandong Province, China. The COD, BOD5, SS, and TN contents were 3,750 ± 50 mg/L, 1850 ± 50 mg/L, 500 ± 100 mg/L, and 210 ± 10 mg/L, pH = 7.6–8.1, respectively. The inoculated ASBR sludge was the USASB from a pharmaceutical sewage plant in Jinan, Shandong Province, China. The MLSS at the time of inoculation was 6,500 mg/L, and the MLSS in the reactor after inoculation was 9,450 mg/L. The activated sludge inoculated in the SBBR was R-sludge from the secondary sedimentation tank of a municipal sewage plant in Jinan, Shandong Province, China. The MLSS was 5,500 mg/L when inoculated and 3,650 mg/L when inoculated into the SBBR reactor.
2.2 Equipment
ASBR and SBBR reactors were cylinders made of plexiglass (Figure 1) with an effective volume of 18 L, diameter of 25 cm, and height of 50 cm. The effluent from the ASBR entered the intermediate water tank through the connecting pipe and then entered SBBR reactor. The influent water flows to the ASBR and SBBR were controlled by a peristaltic pump. The inlet water tank and intermediate water tank were made of plexiglass, and the effective volumes were 10 L. The two reactors were equipped with a stirring device and an aeration device. The outside of the reactors was wrapped with heating belts, and the temperature control box was maintained at a temperature of 25 ± 1°C. The SBBR reactor was filled with a rope-shaped filler made of chemical fiber, and the filling ratio of approx. 20%. DO was controlled at 2–4 mg/L. The system was equipped with WTW Multi340i online tester to monitor the pH, DO, and redox potential (ORP) throughout the reaction process.
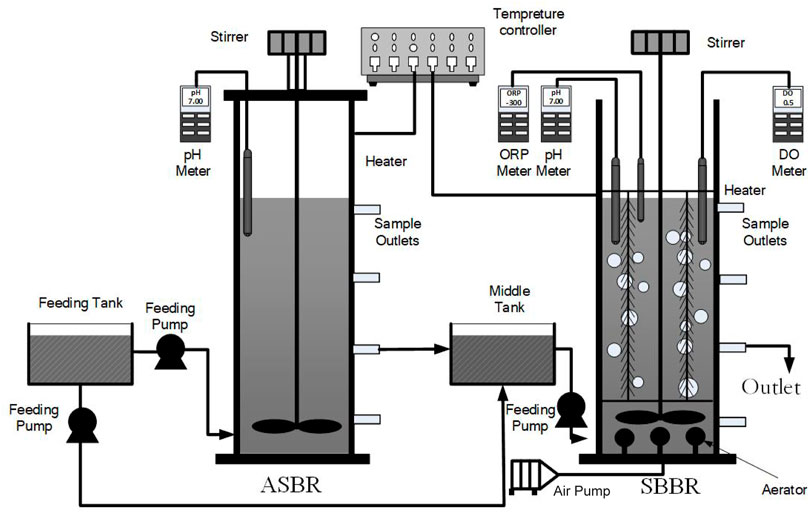
FIGURE 1. Schematic diagram of anaerobic sequencing batch reactor (ASBR) + sequencing batch biofilm reactor (SBBR) system.
2.3 Operational Conditions
The experiment was divided into two periods: the start-up period and the series operation period. During the start-up period, the ASBR and SBBR operated separately and then in series after the performance was stable. The ASBR operation was divided into stages including water inflow (10 min), stirring (determined based on the reaction endpoint in real time through the inflection point of pH value), sedimentation (30 min), drainage (20 min), and idle. The operation modes were the influent (10 min), anaerobic stirring (1 h), aeration (the endpoint of the nitrification reaction was judged in real time based on the “ammonia valley point” and “DO jump point”), anoxic stirring (the endpoint of denitrification was determined in real time based on the “nitrate knee”), sedimentation (30 min), and drainage (10 min). The ASBR and SBBR drainage ratio was 28%. During the series operation period, the inlet water from the intermediate pool was adjusted based on the ASBR inlet water flow rate, the ASBR outlet water flow rate, and the clean water flow rate to adhere to the requirements for the TN concentration and C/N ratio of the inlet water.
2.4 Analytical Methods
NH4+-N, NO2−-N, NO3−-N, TN, COD, MLSS, and MLVSS were determined using standard characterization methods (Apha, 2017). The DO, pH, oxidation-reduction potential, and temperature were monitored using a pH/Oxi 340i analyzer (WTW Company, Germany). DNA extraction and Illumina high-throughput sequencing analysis were conducted by Novogene Co., Ltd. (Beijing, China).
3 Results and Discussion
3.1 Performance of Anaerobic Sequencing Batch Reactor on Organic Matter in the Start-Up Stage
Figure 2 shows the COD removal efficiency from traditional Chinese medicine wastewater during the ASBR start-up stage. To ensure the successful start-up of the ASBR, the influent was pharmaceutical wastewater diluted with tap water in a 2:1 ratio. The COD content in the influent was 1,200 ± 50 mg/L. After 10 days of operation, the COD content in the effluent decreased to 150 ± 5 mg/L. When the COD concentration in the effluent was stable, the organic load in the influence increased. After 22 days, the inlet was switched to pharmaceutical wastewate r‒raw water. The COD concentration was 3,800 ± 50 mg/L, and the COD removal efficiency was approximately 87%. The COD of the effluent was reduced to 480 mg/L. After ASBR treatment, the organic content in the pharmaceutical wastewater was greatly reduced, which motivated the subsequent deep denitrification treatment step.
3.2 Treatment Performance of Organic Matter and Nitrogen by Sequencing Batch Biofilm Reactor
3.2.1 Performance of Chemical Oxygen Demand Removal
The pharmaceutical wastewater treatment using an SBBR was conducted for 99 days and divided into four stages according to different influent carbon–nitrogen ratios (C/N). The treatment effect is shown in Figure 3. The C/N ratio in the first stage was 3–3.2, the influent COD was 150–490 mg/L, and the corresponding influent NH4+-N concentration was 50–153 mg/L. At this stage, the effluent COD was 24–83 mg/L, and the corresponding COD removal efficiency was 83–87%. In the following three stages, the influent NH4+-N and C/N ratio showed continuous improvement, and the COD removal efficiency of effluent stabilized from 85% to approximately 75%. At the end of domestication, the COD concentration of the system’s effluent was approximately 250 mg/L.
3.2.2 Performance of NH4+-N and Total Nitrogen Removal
The goal of the SBBR domestication process was to enrich the nitrifying bacteria content to ensure nitrification of the wastewater. Figure 4 shows the observed concentration changes of NH4+-N, NO2−-N, and NO3−-N in the influent and effluent water during acclimation. In the first 50 days, the C/N ratio in the influent was controlled between 3 and 3.2, and the NH4+-N concentration in the influent was 50, 100, and 150 mg/L. The SBBR realized precise nitrification through process control and showed good nitrification ability (NH4+-N of effluent was lower than 1 mg/L). Pharmaceutical wastewater with an influent NH4+-N content of 135 mg/L was treated by MBR, and the removal efficiency was 81% (Song et al., 2022). The ammonia nitrogen removal effect of this process has obvious advantages. However, because of the low C/N ratio in the influent, the ED was very weak, and as a result, the NO3−-N content remained almost unchanged after aeration and in the final effluent. Studies have found that the key to deep denitrification in anaerobic/aerobic/anoxic operation mode is to maintain an appropriate C/N ratio (Katarzyna et al., 2008). For improving the nitrogen removal efficiency in the present system, from day 51 of the domestication period, the C/N ratio was increased to 4 by adjusting the mixing ratio of inlet and outlet water flows into the ASBR. Then, the NO3−-N contents after aeration and in the final effluent decreased. On day 60, the influent NH4+-N concentration was 150 mg/L, the NO3−-N concentration after aeration decreased to 29 mg/L, and the effluent decreased to 21 mg/L. The system removed 8 mg/L of TN via ED. The test continued to improve the NH4+-N and C/N in the influent. The influent at this stage was not diluted, and only the inlet and outlet water from the ASBR was mixed at a certain proportion. The C/N ratio in the influent increased to 4.5 between 61 and 75 days. Although the TN load in the influent increased, TN removal was more effective because of the enhancement in the C/N ratio and microbial enrichment. A negligible variation in NO3−-N content after aeration was detected, but advanced denitrification of the system was realized using ED. The NO3−-N content in the effluent was below 2 mg/L, and the effects of ED were obvious. To explore the effect of the C/N ratio on the influent of the denitrification efficiency of the system, the C/N ratio was continuously increased to 5 from day 76. As the C/N ratio increased, the SND effect significantly increased, the NO3−-N concentration after aeration decreased, and denitrification efficiency increased. After 88 days, the concentration of NO3−-N after aeration was below 2 mg/L, which was indicative of advanced denitrification of pharmaceutical wastewater. Thereafter, the denitrification capacity of the SBBR was maintained, indicating that the system could realize advanced denitrification of pharmaceutical wastewater through the utilization of SND using the appropriate C/N ratio and added fiber filler.
3.2.3 Removal Pathway of Nitrogen
During the acclimation period, TN was removed through traditional denitrification, SND, and ED processes (Figure 5). In the first stage, the C/N ratio ranged from 3 to 3.2, and because of insufficient carbon sources in the influence, the removal efficiency of TN was insignificant. TN removal mainly depended on prestirred denitrification and SND during the aeration process. The denitrification amount of ED was below 20 mg/L. The nitrification time in this stage increased with the increase of ammonia nitrogen concentration in the influent. The equilibrium NH4+-N concentration in the effluent decreased with an enrichment in the number of nitrifying bacteria. At a C/N ratio of 4, a sharp increase occurred in biofilm growth with the enhancement of the carbon source. Therefore, the amount of TN removed via traditional denitrification and SND increased, whereas the amount of ED decreased. However, when the influent C/N ratio reached 4.5, the amount of nitrogen removed by anoxic stirring in the early stage decreased significantly. By the end of the aeration process, only SND contributed to TN removal. Hence, TN removal via traditional denitrification and SND at this stage was significantly reduced compared with the previous stage, whereas nitrogen removal via ED showed a dramatic increase. In addition, enrichment of nitrifying bacteria and denitrifying bacteria at this stage not only decreased the required nitrification time but also shortened the cycle time required for complete deep denitrification from 24 to 14 h, and the denitrification efficiency increased by 41.7%. In the last stage, the influent C/N ratio increased to approximately 5. The biofilms on the fiber filler continued to grow, the strengthened system had the ability of SND, and the NO3−-N concentration after the aeration continually decreased. On day 86, the deep denitrification of pharmaceutical wastewater was realized only through aeration nitrification. The amount of SND reached the maximum and remained stable (above 200 mg/L). Since the TN content in the system reached the standard after aeration, the operation mode was also changed to influent–stirring–aeration–sedimentation–drainage. The nitrification time of this stage was slightly shorter than the previous stage and finally stabilized after approximately 3.6 h. The cycle time was significantly shorter than that in the previous stage, from the initial 14 to 4.6 h, a decrease of 67% compared with the end of the previous stage.
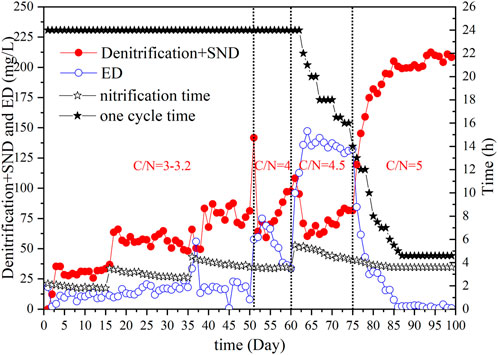
FIGURE 5. Denitrification amount by denitrification + simultaneous nitrification and denitrification and endogenous denitrification.
The TN content in the effluent at the end of aerobic treatment and the TN removal efficiency during the experiment are displayed in Figure 6. It was found that the C/N ratio had a significant impact on denitrification efficiency. The denitrification process mainly depended on predenitrification at a C/N ratio of 3–3.2. When the C/N ratio in the influent was 4, the TN concentration after aeration decreased significantly, and the ED effect increased gradually. However, at this stage, TN removal efficiency was approximately 85% and did not promote deep denitrification of wastewater. When the C/N ratio of the system inlet water was 5, deep denitrification of wastewater was observed, and the denitrification rate increased to above 98%. The main reason for the increased nitrogen removal efficiency of the system was that the increase in the influent organic matter provided sufficient internal carbon sources for denitrifying bacteria, which strengthened the ED effect. At a C/N ratio of 5.5, the TN concentration showed a continuous decrease after aeration, and the SND effect on the system increased. This was promoted by a thicker biofilm, the anoxic microenvironment of the system was more abundant, and there was a sufficient internal carbon source, which resulted in the denitrification time of the system being greatly shortened for deep denitrification of wastewater.
3.3 Comprehensive Treatment Effect of Anaerobic Sequencing Batch Reactor Combined with Sequencing Batch Biofilm Reactor
Table 1 lists the main indexes and removal efficiencies of inlet and outlet water feeds after domestication. The COD concentration in the effluent was approximately 250 mg/L after the combined action of the ASBR and SBBR processes, and the removal efficiency was approximately 93.4%. The removal efficiencies of NH4+-N and TN in the system were above 99% through real time process control and adjustment of the influent C/N ratio of SBBR, and advanced denitrification of pharmaceutical wastewater was realized.

TABLE 1. Treatment effect of anaerobic sequencing batch reactor + sequencing batch biofilm reactor system on pharmaceutical wastewater.
3.4 Diversity of Microbial Communities in Sequencing Batch Biofilm Reactor
3.4.1 Alpha Diversity
The alpha diversity analysis index of three parallel samples under 97% consistency threshold was statistically obtained, and the results are listed in Table 2. The goods coverage was 0.996, which indicated that sequencing had high coverage and could reflect the real situation of sequencing samples. Indexes of Shannon and Simpson were 6.631 and 0.964, respectively, which indicated that the species in the SBBR reactor exhibited high diversity. Chao 1 and ACE index indirectly reflected that the number of species in the sample was approximately 1,200.
The rarefaction curve (Figure 7A) tended to be flat; hence, the amount of sequencing data saturated when the amount of sequencing data was 48,730. Increasing the amount of data did not produce more new species, implying that the amount of sequencing data was reasonable. At the same time, the rarefaction curve indirectly reflected the richness of species in the sample. The number of OTUs that could be constructed by the sample when the ordinate at the flat part of the curve was 1,100. The clustering rank abundance reflected the richness and evenness of the species in the sample (Figure 7B). The width of the curve in the horizontal direction reflected the richness of the species, and the smoothness of the curve in the vertical direction illustrated the uniformity of species (Lundberg et al., 2013).
3.4.2 Microbial Community Structure and Relative Abundance
3.4.2.1 At Phylum Level
Figure 8shows the presence of 46 phyla in the sample, suggesting that the bacterial community structure in SBBR was rich. The order of relative abundance of the common phyla from large to small was as follows: Bacteroidetes, Proteobacteria, Chloroflexi, Firmicutes, Cloacimonetes, Acidobacteria, Spirochaetes, Planctomycetes, Gemmatimonadetes, and Nitrospirae. Bacteroidetes was the most abundant phylum with the highest proportion of 39.69%, followed by Proteobacteria (37.34%), Chloroflexi (6.54%), and Firmicutes (5.17%). These four kinds of phyla often appear in the activated sludge system. They have various metabolic types and could survive in aerobic and anaerobic environments, playing a key role in carbon and nitrogen removal from the system (Wang et al., 2018). Bacteroides and Chloroflexi are heterotrophic bacteria, which can effectively degrade refractory organics in wastewater. Bacteroides can also degrade particulates’ high molecular weight compounds (Díez-Vives et al., 2012). In addition, the complex polymers and sugars can be degraded by Chloroflexi (McIlroy et al., 2016), which further removes organic matter. Firmicutes are advantageous for denitrification and can produce spores that adapt to the external extreme environment so that the bacteria can adapt and recover in response to changing water quality and still decompose amino acids and proteins (Yan et al., 2021). Planctomycetes (Antwi et al., 2020) and Acidobateria (Li and Lu, 2017) can also perform denitrification. Cloacimonetes and Spirochaetes mostly exist in the anaerobic digestion period, and carbon and energy come from amino acid fermentation (Solli et al., 2014) or carbohydrate decomposition (Yan et al., 2021).
3.4.2.2 At the Genus Level
A total of 292 kinds of genera were detected via high-throughput sequencing, accounting for 47% of the total number of bacteria, and 53% of OTU were not detected. To further decipher the composition and structure of the microbial community in the SBBR system, species classification tree statistics were conducted for the top 10 genera with maximum relative abundance (Figure 9). Nitrosomonas (6.11%) had the highest abundance in the sample, which belongs to Proteobacteria, and is the most common ammonia-oxidizing bacteria (AOB). Unidentified_Nitrosomonadacea (1.51%) is also nitrifying bacteria, and the two bacteria both belong to Nitrosomonadaceae. The second most abundant genus was Thauera (5.665%), which is denitrifying bacteria that widely exists in sewage treatment plants. Unidentified_Sphingobacteriales were heterotrophic denitrifying bacteria (HDB). Large polymers such as petroleum and chloroethane and simple inorganic substances can be used as substrates and adapt to various complex extreme environments (Gabarro et al., 2013). Limnobacter is a denitrifying bacterium belonging to the phylum of Proteobacteria, which can degrade refractory pollutants such as aromatic compounds, and also play an important role in the removal of heavy metal ions (Zheng et al., 2019). Unidentified_Acidobacteria (0.644%) can reduce nitrate and nitrite. Ferruginibacter (2.955%) (Lim et al., 2009) and Candidatus_Cloacimonas (2.265%) can decompose carbohydrates to produce various organic acids, which is conducive to the subsequent denitrification community to store carbon sources (Yan et al., 2021). Novosphingobium (1.946%) usually appears in the anaerobic digestion tank and can degrade polycyclic aromatic hydrocarbons (Yuan et al., 2009). Unidentified_Saprosipraceae (0.958%) can degrade organic matter (Liang et al., 2015).
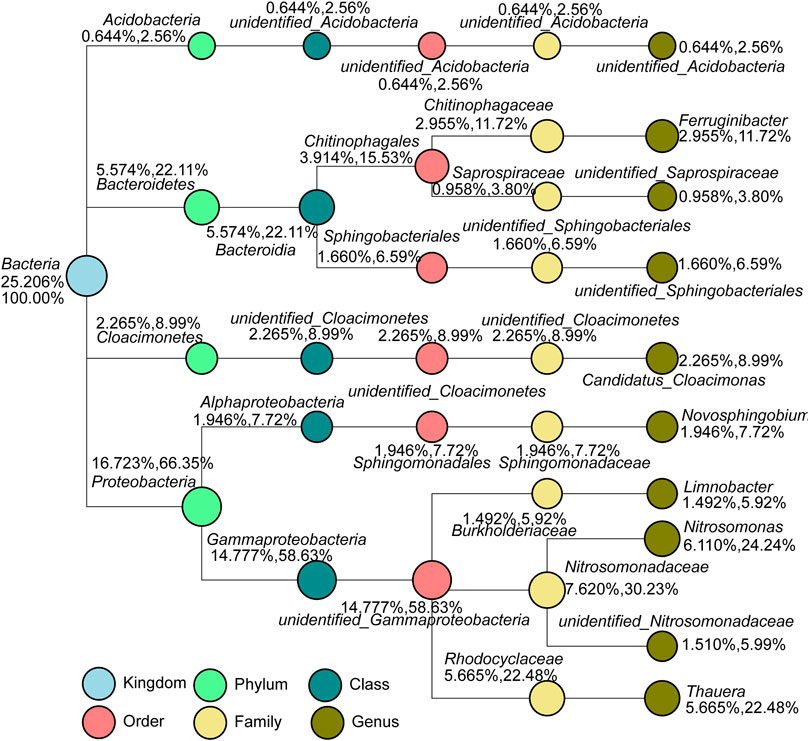
FIGURE 9. Genus level species classification tree within SBBR (Note: the two numbers below/above the taxonomic name represent the percentage of relative abundance. The former represents the percentage of the taxonomic species in the sample, and the latter indicates the percentage of the classification in the classified species selected in the sample.).
3.4.3 Diversity Analysis of Denitrifying Bacteria
3.4.3.1 Nitrifying Bacteria
In the SBBR system, two types of AOB, namely, Nitrosomonas (6.11%) and unidentified_Nitrosomonadacea (1.510%), as well as one type of nitrite-oxidizing bacteria, namely, unidentified_Nitrospiraceae (0.4857%) belonging to Nitrospirae, were detected.
3.4.3.2 Heterotrophic Denitrifies Bacteria
Eight kinds of HDB with a relative abundance of >0.5% were detected, including Thauera, Limnobacter, Steroidobacter, Rhizobiales, Hydrogenophaga, Ferruginibacter, unidentified_Sphingobacteriales, and Acidobacteria. Figure 10 shows 18 denitrifying bacteria detected in the SBBR system (Antwi et al., 2020) (Geng et al., 2014). Thauera and Azoarcus are denitrifying bacteria in Rhodocyclaceae. Hydrogenophaga is a Gram-negative bacterium, which effectively degrades nitrate (Ji et al., 2016). Rhizobiales has a coding gene nirK that can encode nitrite reductase (Saito et al., 2008).
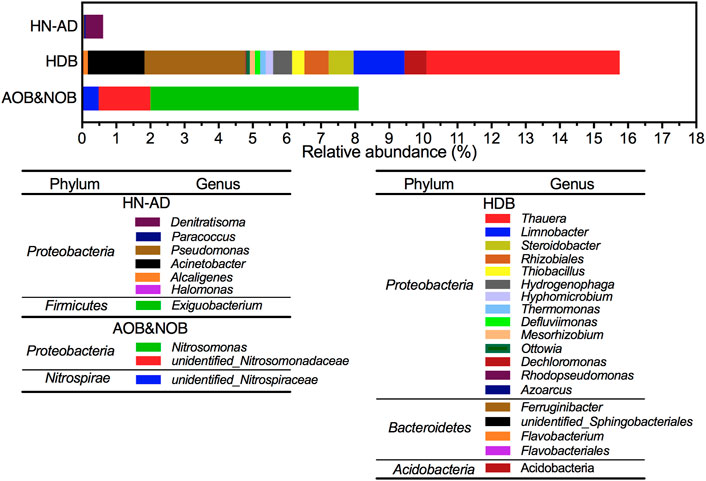
FIGURE 10. Relative abundance of heterotrophic nitrification–aerobic denitrification, heterotrophic denitrifying bacteria, and ammonia-oxidizing bacteria & nitrite-oxidizing bacteria at genus level.
3.4.3.3 Heterotrophic Nitrifying–Aerobic Denitrifying Bacteria
In addition to traditional nitrification–denitrification bacteria, heterotrophic nitrifying–aerobic denitrifying (HN-AD) bacteria also existed in the SBBR system. HN-AD bacteria can use various carbon substrates as energy and electronic sources for heterotrophic nitrification and grow faster than autotrophic nitrifying bacteria. Furthermore, HN-AD bacteria can directly use the products of heterotrophic nitrification as reactants for aerobic denitrification to convert different forms of nitrogen into N2 (Zhang et al., 2012) and to enhance the SND capacity of the SBBR system. The following were identified in the system: Denitratisoma (0.5151%) (Fahrbach et al., 2006), Paracoccus (0.0486%) (Jaffer et al., 2019), Pseudomonas (0.0219%) (Xiaoling et al., 2019), Exiguobacterium (0.0109%) (Cui et al., 2021), Acinetobacter (0.0103%) (Yang et al., 2016), Halomonas (0.0041%) (Wang et al., 2019), and Alcaligenes (0.0041%) (Joo et al., 2005), which function as heterotrophic nitrification and aerobic denitrification. Muller et al. proposed that the rate of aerobic denitrification and ammonia consumption were basically in the same order of magnitude (Muller et al., 1995). This may contribute to the denitrification of the system.
The relative abundance of the above seven HN-AD bacteria was 0.612%, and the relative abundance of HDB was 26.6 times larger, implying that HDB bacteria still played a major role in the denitrification process of the SBBR system. Many studies had shown that Proteobacteria can absorb external carbon sources and store them as internal carbon sources as PHB under anaerobic conditions. Proteobacteria was the dominant phylum of denitrifying phosphorus accumulating bacteria (DPAO) and denitrifying glycan accumulating bacteria (DGAO) which was conducive to the systematic denitrification of the wastewater (Gao et al., 2019). In this study, 75.8% of the bacteria related to nitrogen removal were Proteobacteria, including a large number of nitrifying bacteria such as Nitrosomonas and denitrifying bacteria such as Thauera, which was consistent with relevant reports (Yang et al., 2021). This may be the main reason for the strong denitrification ability of this system.
4 Conclusion
In this study, ASBR + SBBR were combined to treat pharmaceutical wastewater with a COD concentration of 3,750 ± 50 mg/L and an NH4+-N concentration of 210 ± 10 mg/L. The main conclusions were as follows:
(1) The COD, NH4+-N, and TN of the final effluent from the ASBR + SBBR combined treatment process were 230 ± 10 mg/L, 1 ± 0.5 mg/L, and 5 ± 3 mg/L, respectively, and the removal efficiencies were greater than 93.5, 99, and 96%, respectively.
(2) The C/N ratio and biofilms were the core factors determining advanced denitrification of pharmaceutical wastewater using the SBBR. Under the conditions of a C/N ratio of 5 in the influent, SBBR could be used to realize advanced denitrification only through SND. The effluent TN concentration was below 8 mg/L, TN removal efficiency was above 96%, and the reaction cycle was controlled at approximately 4.6 h.
(3) According to high-throughput sequencing analysis, Bacteroidetes, Proteobacteria, Chloroflex, and Firmicutes were the abundant phyla in the system. Nitrosomonas and Thauera were the two bacteria with the highest relative abundance at the genus level.
(4) Through the analysis of the diversity of denitrifying bacteria, it was determined that HDB and HN-AD existed in the system at the same time, and the higher SND effect of the system may be the result of the joint action of both bacteria.
Data Availability Statement
The original contributions presented in the study are included in the article/Supplementary Material, and further inquiries can be directed to the corresponding author.
Author Contributions
KW: supervision, verification, review, and editing; JJ: investigation, data collection and analysis, visualization, and writing; LM: investigation and funding acquisition; LZ: sampling and testing; GZ: sampling and testing; DW: review, resources, validation, and editing.
Funding
This work was supported by the Natural Science Foundation of Shandong Province (ZR2017BEE067), the National Natural Science Foundation of China (2019–51908334), and the National Key Research and Development Program of China (2017YFF0209903).
Conflict of Interest
LM was employed by the Shandong Guochen Industrial Group Co, Ltd.
The remaining authors declare that the research was conducted in the absence of any commercial or financial relationships that could be construed as potential conflicts of interest.
Publisher’s Note
All claims expressed in this article are solely those of the authors and do not necessarily represent those of their affiliated organizations, or those of the publisher, the editors, and the reviewers. Any product that may be evaluated in this article, or claim that may be made by its manufacturer, is not guaranteed or endorsed by the publisher.
Acknowledgments
The authors thank the editors and reviewers for their suggestions to improve this article.
References
Adishkumar, S., Sivajothi, S., and Rajesh Banu, J. (2012). Coupled Solar Photo-Fenton Process with Aerobic Sequential Batch Reactor for Treatment of Pharmaceutical Wastewater. Desalination Water Treat. 48, 89–95. doi:10.1080/19443994.2012.698799
Antwi, P., Zhang, D., Su, H., Luo, W., Quashie, F. K., Kabutey, F. T., et al. (2020). Nitrogen Removal from Landfill Leachate by Single-Stage Anammox and Partial-Nitritation Process: Effects of Microaerobic Condition on Performance and Microbial Activities. J. Water Process Eng. 38, 101572–101585. doi:10.1016/j.jwpe.2020.101572
Apha (2017). Standard Methods For The Examination Of Water And Wastewater 23th. Washington, DC, USA: American Public Health Association.
Bolonhesi, I. B. T. M., Andreani, C. L., Theodoro, J. D. P., Fazolo, A., and Lopes, D. D. (2021). Formaldehyde Biodegradation in an ASBR-SBR System: an Effective Treatment Solution for Furniture Industry Painting Booth Wastewater. Int. J. Environ. Sci. Technol. 19, 3075–3086. prepublish. doi:10.1007/s13762-021-03276-4
Cheong, D.-Y., and Hansen, C. L. (2008). Effect of Feeding Strategy on the Stability of Anaerobic Sequencing Batch Reactor Responses to Organic Loading Conditions. Bioresour. Technol. 99, 5058–5068. doi:10.1016/j.biortech.2007.08.084
Cui, Y., Cui, Y.-W., and Huang, J.-L. (2021). A Novel Halophilic Exiguobacterium Mexicanum Strain Removes Nitrogen from Saline Wastewater via Heterotrophic Nitrification and Aerobic Denitrification. Bioresour. Technol. 333, 125189. doi:10.1016/j.biortech.2021.125189
Díez-Vives, C., Gasol, J. M., and Acinas, S. G. (2012). Evaluation of Marine Bacteroidetes-Specific Primers for Microbial Diversity and Dynamics Studies. Microb. Ecol. 64, 1047–1055. doi:10.1007/s00248-012-0087-x
Fahrbach, M., Kuever, J., Meinke, R., Kämpfer, P., and Hollender, J. (2006). Denitratisoma Oestradiolicum Gen. nov., Sp. nov., a 17β-Oestradiol-Degrading, Denitrifying Betaproteobacterium. Int. J. Syst. Evol. Microbiol. 56, 1547–1552. doi:10.1099/ijs.0.63672-0
Gabarró, J., Hernández-del Amo, E., Gich, F., Ruscalleda, M., Balaguer, M. D., and Colprim, J. (2013). Nitrous Oxide Reduction Genetic Potential from the Microbial Community of an Intermittently Aerated Partial Nitritation SBR Treating Mature Landfill Leachate. Water Res. 47, 7066–7077. doi:10.1016/j.watres.2013.07.057
Gao, H., Mao, Y., Zhao, X., Liu, W.-T., Zhang, T., and Wells, G. (2019). Genome-centric Metagenomics Resolves Microbial Diversity and Prevalent Truncated Denitrification Pathways in a Denitrifying PAO-Enriched Bioprocess. Water Res. 155, 275–287. doi:10.1016/j.watres.2019.02.020
Geng, S., Pan, X.-C., Mei, R., Wang, Y.-N., Sun, J.-Q., Liu, X.-Y., et al. (2014). Ottowia Shaoguanensis Sp. nov., Isolated From Coking Wastewater. Curr. Microbiol. 68, 324–329. doi:10.1007/s00284-013-0481-8
Jaffer, Y. D., Sanath Kumar, H., Vinothkumar, R., Irfan, A. B., Ishfaq, N. M., Ganie, P. A., et al. (2019). Isolation and Characterization of Heterotrophic Nitrification-Aerobic Denitrification and Sulphur-Oxidizing Bacterium Paracoccus Saliphilus Strain SPUM from Coastal Shrimp Ponds. Aquacult Int. 27, 1513–1524. doi:10.1007/s10499-019-00407-0
Ji, B., Chen, W., Zhu, L., and Yang, K. (2016). Isolation of Aluminum-Tolerant Bacteria Capable of Nitrogen Removal in Activated Sludge. Mar. Pollut. Bull. 106, 31–34. doi:10.1016/j.marpolbul.2016.03.051
Joo, H.-S., Hirai, M., and Shoda, M. (2005). Characteristics of Ammonium Removal by Heterotrophic Nitrification-Aerobic Denitrification by Alcaligenes Faecalis No. 4. J. Biosci. Bioeng. 100, 184–191. doi:10.1263/jbb.100.184
Katarzyna, B., Irena, W.-B., and Adriana, D. (2008). Denitrification With Endogenous Carbon Source at Low C/N and its Effect on P(3HB) Accumulation. Bioresour. Technol. 99, 2410–2418. doi:10.1016/j.biortech.2007.05.008
Kruglova, A., Kråkström, M., Riska, M., Mikola, A., Rantanen, P., Vahala, R., et al. (2016). Comparative Study of Emerging Micropollutants Removal by Aerobic Activated Sludge of Large Laboratory-Scale Membrane Bioreactors and Sequencing Batch Reactors under Low-Temperature Conditions. Bioresour. Technol. 214, 81–88. doi:10.1016/j.biortech.2016.04.037
Li, E., and Lu, S. (2017). Denitrification Processes and Microbial Communities in a Sequencing Batch Reactor Treating Nanofiltration (NF) Concentrate from Coking Wastewater. Water Sci. Technol. A J. Int. Assoc. Water Pollut. Res. 76, 3289–3298. doi:10.2166/wst.2017.493
Liang, Y., Li, D., Zeng, H., Zhang, C., and Zhang, J. (2015). Rapid Start-Up and Microbial Characteristics of Partial Nitrification Granular Sludge Treating Domestic Sewage at Room Temperature. Bioresour. Technol. 196, 741–745. doi:10.1016/j.biortech.2015.08.003
Lim, J. H., Baek, S.-H., and Lee, S.-T. (2009). Ferruginibacter alkalilentus gen. nov., sp. nov. and Ferruginibacter lapsinanis sp. nov., novel members of the family 'Chitinophagaceae' in the phylum Bacteroidetes, isolated from freshwater sediment. Int. J. Syst. Evol. Microbiol. 59, 2394–2399. doi:10.1099/ijs.0.009480-0
Lundberg, D. S., Yourstone, S., Mieczkowski, P., Jones, C. D., and Dangl, J. L. (2013). Practical innovations for high-throughput amplicon sequencing. Nat. Methods 10, 999–1002. doi:10.1038/nmeth.2634
Mcilroy, S. J., Karst, S. M., Nierychlo, M., Dueholm, M. S., Albertsen, M., Kirkegaard, R. H., et al. (2016). Genomic and In Situ investigations of the novel uncultured Chloroflexi associated with 0092 morphotype filamentous bulking in activated sludge. Isme J. 10, 2223–2234. doi:10.1038/ismej.2016.14
Muller, E. B., Stouthamer, A. H., and van Verseveld, H. W. (1995). Simultaneous NH3 oxidation and N2 production at reduced O2 tensions by sewage sludge subcultured with chemolithotrophic medium. Biodegradation 6, 339–349. doi:10.1007/BF00695264
Oliveira, R. P., Ratusznei, S. M., Rodrigues, J. A. D., Zaiat, M., and Foresti, E. (2010). Interaction effects of organic load and cycle time in an AsBr applied to a personal care industry wastewater treatment. J. Environ. Manag. 91, 2499–2504. doi:10.1016/j.jenvman.2010.07.015
Saito, T., Ishii, S., Otsuka, S., Nishiyama, M., and Senoo, K. (2008). Identification of novel betaproteobacteria in a succinate-assimilating population in denitrifying rice paddy soil by using stable isotope probing. Microb. Environ. 23, 192–200. doi:10.1264/jsme2.23.192
Shao, X., Peng, D., Teng, Z., and Ju, X. (2008). Treatment of brewery wastewater using anaerobic sequencing batch reactor (ASBR). Bioresour. Technol. 99, 3182–3186. doi:10.1016/j.biortech.2007.05.050
Solli, L., Håvelsrud, O. E., Horn, S. J., and Rike, A. G. (2014). A metagenomic study of the microbial communities in four parallel biogas reactors. Biotechnol. Biofuels 7, 146. doi:10.1186/s13068-014-0146-2
Song, J., Wu, H., Yuan, H., Wu, J., Qi, W., Lu, J., et al. (2022). Reclamation of pharmaceutical wastewater by UAF, contact oxidation, and auto brush-cleaning aiding nano ZrO2 coated ceramic membrane MBR - an industrial-scale practice and functional microbial diversity. J. Water Process Eng. 46, 102563. doi:10.1016/j.jwpe.2022.102563
Wang, K. (2021). Comparative Study on Advanced Nitrogen Removal of Landfill Leachate Treated by SBR and SBBR. Water 13, 3240. doi:10.3390/w13223240
Wang, K., Wang, S., Zhu, R., Miao, L., and Peng, Y. (2013). Advanced nitrogen removal from landfill leachate without addition of external carbon using a novel system coupling ASBR and modified SBR. Bioresour. Technol. 134, 212–218. doi:10.1016/j.biortech.2013.02.017
Wang, T., Jiang, Z., Dong, W., Liang, X., Zhang, L., and Zhu, Y. (2019). Growth and nitrogen removal characteristics of Halomonas sp. B01 under high salinity. Ann. Microbiol. 69, 1425–1433. doi:10.1007/s13213-019-01526-y
Wang, X., Zhu, M., Li, F., Zhang, C., and Zhu, X. (2018). Long-term effects of multi-walled carbon nanotubes on the performance and microbial community structures of an anaerobic granular sludge system. Appl. Microbiol. Biotechnol. 102, 9351–9361. doi:10.1007/s00253-018-9273-1
Xiaoling, H., Qi, S., Tengyao, X., Meng, D., and Dongsheng, W. (2019). Removal of Nitrogen by Heterotrophic Nitrification-Aerobic Denitrification of a Novel Halotolerant Bacterium Pseudomonas mendocina TJPU04. Bioprocess and Biosystems. Bioproc. Biosyst. Eng. 42, 5. doi:10.1007/s00449-019-02088-8
Yan, H. H., Han, L., Yin, Q., Guo, X. Y., and Nian, Y. G. (2021). Corn starch processing wastewater treated by a full-scale expanded granular sludge bed reactor and comprehensive analysis of microbial community at low and high organic loading rate. IOP Conf. Ser. Earth Environ. Sci. 776, 012003. (8pp). doi:10.1088/1755-1315/776/1/012003
Yang, L., Ren, Y.-X., Zhao, S.-Q., Liang, X., and Wang, J.-P. (2016). Isolation and characterization of three heterotrophic nitrifying-aerobic denitrifying bacteria from a sequencing batch reactor. Ann. Microbiol. 66, 737–747. doi:10.1007/s13213-015-1161-7
Yang, Z., Zhou, Q., Sun, H., Jia, L., Zhao, L., and Wu, W. (2021). Metagenomic analyses of microbial structure and metabolic pathway in solid-phase denitrification systems for advanced nitrogen removal of wastewater treatment plant effluent: A pilot-scale study. Water Res. 196, 117067. doi:10.1016/j.watres.2021.117067
Yuan, J., Lai, Q., Zheng, T., and Shao, Z. (2009). Novosphingobium indicum sp. nov., a polycyclic aromatic hydrocarbon-degrading bacterium isolated from a deep-sea environment. Int. J. Syst. Evol. Microbiol. 59, 2084–2088. doi:10.1099/ijs.0.002873-0
Zhang, Q.-L., Liu, Y., Ai, G.-M., Miao, L.-L., Zheng, H.-Y., and Liu, Z.-P. (2012). The characteristics of a novel heterotrophic nitrification-aerobic denitrification bacterium, Bacillus methylotrophicus strain L7. Bioresour. Technol. 108, 35–44. doi:10.1016/j.biortech.2011.12.139
Zhao, X., Chen, Z., Wang, X., Li, J., Shen, J., and Xu, H. (2015). Remediation of pharmaceuticals and personal care products using an aerobic granular sludge sequencing bioreactor and microbial community profiling using Solexa sequencing technology analysis. Bioresour. Technol. 179, 104–112. doi:10.1016/j.biortech.2014.12.002
Zheng, M., Zhou, N., Liu, S., Dang, C., Liu, Y., He, S., et al. (2019). N2O and NO emission from a biological aerated filter treating coking wastewater: Main source and microbial community. J. Clean. Prod. 213, 365–374. doi:10.1016/j.jclepro.2018.12.182
Zou, R., Angelidaki, I., Yang, X., Tang, K., Andersen, H. R., and Zhang, Y. (2020). Degradation of pharmaceuticals from wastewater in a 20-L continuous flow bio-electro-Fenton (BEF) system. Sci. Total Environ. 727, 138684. doi:10.1016/j.scitotenv.2020.138684
Keywords: traditional Chinese medicine wastewater, ASBR, SBBR, advanced nitrogen removal, SND, microbial community structure
Citation: Wang K, Jiang J, Ma L, Zheng L, Zhang G and Wu D (2022) Study on Advanced Nitrogen Removal and Microbial Community Structure of Traditional Chinese Medicine Wastewater by a Novel System Coupling Anaerobic Sequencing Batch Reactor and Modified Sequencing Batch Biofilm Reactor . Front. Environ. Sci. 10:932657. doi: 10.3389/fenvs.2022.932657
Received: 11 May 2022; Accepted: 15 June 2022;
Published: 22 July 2022.
Edited by:
Vincenzo VaianoVincenzo Vaiano, University of Salerno, ItalyReviewed by:
Lei Miao, Huazhong University of Science and Technology, ChinaLijie Zhou, Shenzhen University, China
Copyright © 2022 Wang, Jiang, Ma, Zheng, Zhang and Wu. This is an open-access article distributed under the terms of the Creative Commons Attribution License (CC BY). The use, distribution or reproduction in other forums is permitted, provided the original author(s) and the copyright owner(s) are credited and that the original publication in this journal is cited, in accordance with accepted academic practice. No use, distribution or reproduction is permitted which does not comply with these terms.
*Correspondence: Daoji Wu, d2RqQHNkanp1LmVkdS5jbg==