- 1INCDO-INOE 2000, Research Institute for Analytical Instrumentation, Cluj-Napoca, Romania
- 2Department of Molecular Biology and Biotechnology, Faculty of Biology and Geology, Babes-Bolyai University, Cluj-Napoca, Romania
- 3Centre for Systems Biology, Biodiversity and Bioresources, Babes-Bolyai University, Cluj-Napoca, Romania
- 4National Institute of Research and Development for Biological Sciences, Institute of Biological Research, Cluj-Napoca, Romania
- 5Cluj-Napoca Department, Emil Racovita Institute of Speleology, Cluj-Napoca, Romania
In Apuseni Mountains (North-Western Romania), many of the inhabitants live in rural communities with limited or no access to the centralized and controlled water supply. This study assesses the microbiological quality of six karst spring waters from Bihor County used by rural communities as drinking water sources. Twenty-four water samples collected in January, April, June, and November 2021 were analyzed for E. coli, total coliforms, intestinal enterococci, Pseudomonas aeruginosa, and heterotrophic plate count at 37 and 22°C. Standard microbiological methods based on the membrane filter technique or pour plate method were used for the microbiological characterization of the spring waters. The study revealed that the karst springs from the studied area present microbiological contamination. The microbiological parameters for five out of the six studied spring waters exceeded the maximum limits allowed by the 98/83/EC Directive. Quantitative Microbial Risk Assessment estimated the risk of gastrointestinal illness for both adults and children due to the enteropathogenic E. coli contamination. According to the health risk evaluation model, the risk of infection/day and the risk of infection/year were high, with the maximum values of 0.24 and 1.00, respectively. The probability of illness caused by E. coli contamination of water ranged between 0.09 and 0.35 for five out of six groundwater sources. The local communities using the contaminated springs are exposed to daily and accumulated health threats. Therefore, preventive measures accompanied by continuous monitoring are necessary mainly for those water sources that are critical drinking water sources for the rural communities.
1 Introduction
Access to clean water and sanitation represent one of the 17 Sustainable Development Goals set by the United Nations, being recognized as a human right that is essential for the full enjoyment of life (United Nations, 2010). Although considerable efforts have been made to improve access to safe water, two billion people are still deprived to safely managed drinking water (World Health Organization and United Nations Children’ s Fund, 2021). Scarcity or poor quality of water affects many inhabitants, especially from developing countries, in both rural and urban areas (Singh et al., 2019; Viban et al., 2021). According to the Global Burden of Disease Study, 1.2 million people died in 2017 due to the water-related disease (GBD 2017 Risk Factor Collaborators 2018; Ritchie and Roser, 2021), most of which rely on chemically and microbiologically unmonitored, untreated, and unprotected groundwater or surface water (Boadi et al., 2020).
As the majority of the freshwater reserves are found underground, groundwater constitutes a major source of drinking water for about 50% of the world’s population (Smith et al., 2016; Velis et al., 2017; Johnson et al., 2022). Globally, about 2.5 billion people depend solely on groundwater supplies for their basic daily water needs (Grönwall and Danert, 2020). In Europe, underground aquifers cover one third of the needs of inhabitants and, in some regions, are the only available source of drinking water (Ravbar et al., 2021).
It is recognized that groundwater is less exposed to chemical and microbial contamination and more stable than surface waters, making it more suitable for drinking and a desirable resource (Dehghani et al., 2019). In contrast, karst aquifers are highly vulnerable to contamination as the karst systems have complex hydrogeological features with no or little protective cover of soil/sediment that allow the rapid infiltration of water, concentrated channel flow, and little self-purification capacity (Bakalowicz, 2005; Moldovan et al., 2019). Moreover, the karst water flow is influenced by the hydrological conditions, determining spatial and temporal changes in groundwater flow direction or catchment area (Ravbar et al., 2011). Although the seepage through the rock matrix is slow, water with contaminants flow fast through conduits and fractures and are spread far from the source, especially in the rainy season (Ghasemizadeh et al., 2012).
The demographic development, urbanization, land-use change, intensive agriculture, industrialization, and global changes constantly affect the groundwater quality (Llopis-González et al., 2014; Wu et al., 2015; Scharping and Garey, 2021). The discharge of various chemicals such as nitrates or pesticides and harmful pathogenic microorganisms into the environment can decrease water quality and food safety and increase disease transmission (Sasakova et al., 2018; Goshu et al., 2021; Kongprajug et al., 2021). Among diseases associated with consumption of contaminated water, diarrheal diseases have the highest incidence causing 485,000 deaths annually (Ezeh et al., 2014; WHO, 2019).
Microbial contaminants including Campylobacter spp., Yersinia spp., Escherichia coli, Pseudomonas aeruginosa, intestinal enterococci, Salmonella spp., Shigella spp., Bacillus spp., or Staphylococcus aureus have been previously detected in groundwater (Grisey et al., 2010; Pitkänen et al., 2011). Water contamination by pathogenic microorganisms may lead to waterborne diseases such are typhoid, paratyphoid, salmonellosis, tuberculosis, brucellosis, tularemia, leptospirosis, cholera, amoebic dysentery, schistosomiasis, infectious hepatitis, poliomyelitis, aseptic meningitis (Macler and Merkle 2000; Sasakova et al., 2018). Though the acute gastroenteric illness was the most common waterborne disease described in the outbreaks, in many cases, the causative agent was not identified (Macler and Merkle 2000). More rarely Shigella spp., hepatitis A virus, norwalk virus, Giardia lamblia, Campylobacter jejuni, and Cryptosporidium parvum were identified as disease agents (Macler and Merkle 2000). Some of these bacteria are resistant to the majority of antimicrobial compounds and can suffer horizontal gene transfer (Ozgumus et al., 2007). Generally, the outbreaks in rural areas using untreated and unmonitored spring waters are most likely underreported compared to those in urban areas using centralized water supply systems (Macler and Merkle 2000). Considering these water-related acute health risks, the management of groundwater contamination and its immediate protection are paramount. From a microbiological perspective, sustainable groundwater management requires testing appropriate microbiological indicators, regular monitoring, finding the contamination sources, and protection against pollution. According to the WHO specifications, safe drinking water must be free of pathogens (including faecal contaminants) and elevated levels of toxic substances at all times (WHO, 2017). The primary standard microbiological parameters currently used for the basic water quality assessment are E. coli, total coliforms, faecal coliforms, and intestinal enterococci (Rufino et al., 2021). These parameters typically indicate the faecal pollution of water by anthropogenic activities.
In Romania, groundwater represents 10% of the total water supply, an amount that satisfies approximately 43% of the population’s drinking water needs (Danube Water Program, 2015). In most remote rural areas, groundwater is the only drinking water source. In rural settlements from Apuseni Mountains (North-Western Romania), the potable water supply network is insufficiently developed or non-existent (Petrescu-Mag et al., 2016). Thus, the rural population has limited options to assure the daily water needs, especially for drinking, household purposes, livestock, or irrigation, and rely mostly on untreated springs or domestic well water. By consuming chemically or microbiologically contaminated water, the inhabitants are exposed to potential health risks, therefore, the quality of all drinking water sources should be monitored (Pitkänen et al., 2011; Boelee et al., 2019). So far, only a few studies have investigated the quality of the groundwater sources in the Apuseni area. Although the chemical composition and radiological risk assessment of springs has been previously reported (Roba et al., 2020; Cucoș et al., 2021; Hoaghia et al., 2021), the microbiological quality and health risk associated with the water consumption has been less explored.
The present study aimed to investigate the microbial quality of springs from the Apuseni Mountains in conjunction with the local population’s exposure to potentially contaminated water. To the best of our knowledge, our study is the first quantitative microbial risk assessment (QMRA) study carried out on springs water from Romania. The objectives of the present study were to 1) determine the concentration of the microbial indicators in the six spring waters during four seasons; 2) evaluate the microbiological quality status of the groundwater following current legislative requirements; 3) assess the human health risk associated with the consumption of spring water and identify which springs are at high risk in order to adopt a management based on prevention of contamination, protection and control of groundwater.
2 Materials and methods
2.1 Study area
Six karstic springs located in Bihor County (western part of the Apuseni Mountains) were selected for our study based on their use by the local populations (Table 1; Figure 1). GWR12 (Ferice), GWR14 (Țarina), GWR15 (Josani), GWR16 (Canton Albioara), GWR17 (Pișnița), and GWR19 (Borz) are unprotected karst springs belonging to aquifers developed in limestone, sandstone, and conglomerates. All these springs are located in rural settlements with low population density (29–62 inhabitants/km2), where the administrative territory is configured as follow: arable land 20%–40%, pastures 13%–28% and grasslands 3%–15%. The catchment areas of the springs are covered mainly by natural zones, especially broad-leaved forests, non-irrigated arable land, pastures or grasslands (Figure 1). Despite the low number of inhabitants (Table 1), vast natural territories, and limited industrial activities, degradation of surface and groundwater quality occurred. The most critical anthropogenic activities that negatively impact the water quality are deforestation, local limestone exploitation, polymetallic ores mining, poor management of natural resources processing, agriculture, poor waste and wastewater management, and household activities. The main activities of the inhabitants consist in subsistence farming, animal husbandry, wood exploitation and processing, as well as limestone and sandstone quarrying (Surd and Turnock, 2000; Hoaghia et al., 2021). The local communities use the springs for different purposes, including human and animal consumption, irrigation, washing, or bathing. These water sources are not currently under local or regional agencies monitoring program of water quality.
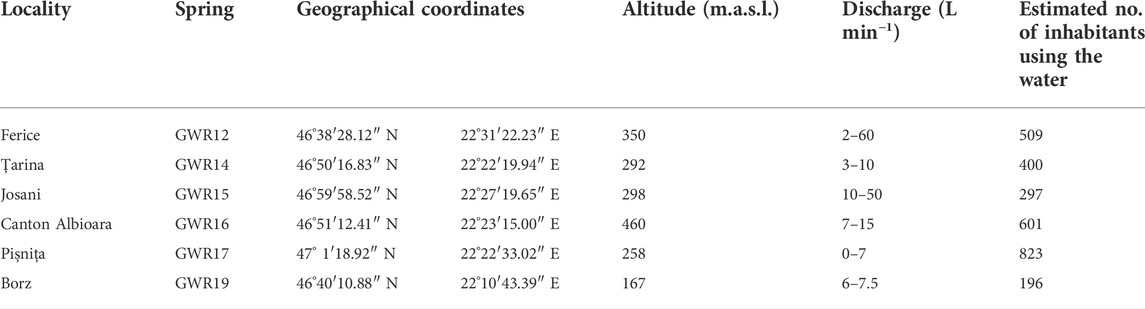
TABLE 1. Karst water springs with their geographical coordinates and the estimated number of inhabitants relying on these waters.
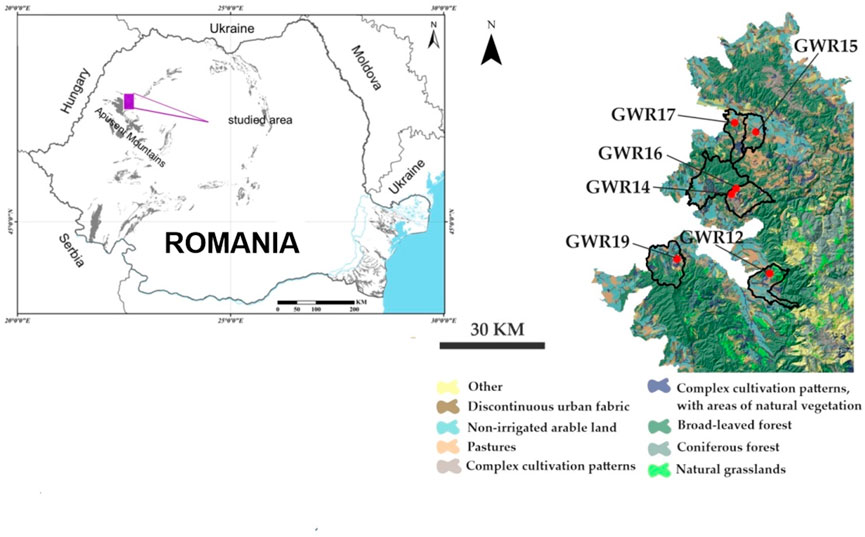
FIGURE 1. Location of the study area in Romania and a detailed map of the study area presenting the main topographic features and land uses.
2.2 Sample collection
Water samples were collected in January, April, June, and November 2021 following the ISO 19458:2006, 2006 standard. Twenty-four raw water samples were collected using 500 ml sterile screw-capped polypropylene bottles. After collection, the bottles were immediately placed in a cool box with ice packs, kept at approximately 4°C, and transported to the laboratory. All aseptic measures have been taken during the sampling campaigns to avoid any external contamination. The samples were analyzed within a maximum of 12 h. At the time of sampling, the water flow level at the springs varied widely (Table 1). The flow rate was associated with different climatic conditions, culminating with heavy rainfall and snow melting in January, moderate rainfall in April and low rainfall in June and November.
2.3 Detection and enumeration of microbial indicators from groundwater
The presence of microbial water contamination was analyzed by the determination of E. coli (EC), total coliforms (TC), intestinal enterococci (IE), Pseudomonas aeruginosa (PA), heterotrophic plate count at 22°C (HPC 22), and heterotrophic plate count at 37°C (HPC 37). Based on standard techniques for microbiological analysis, viable and cultivable microorganisms were targeted. The membrane filtration (MF) method was used to detect and quantify microbiological indicators in the selected groundwater samples (ISO 9308-1:2014, 2014; ISO 7899-2:2000, 2000; ISO 16266:2006, 2006; ISO 6222:1999, 1999). A volume of 100 ml of water and its decimal dilutions (10–1, 10–2, and 10–3) were filtered through a sterile mixed cellulose ester membrane (Schaulau, Sentmenat, Spain), with a pore size of 0.45 μm and a diameter of 47 mm. A pre-sterilized filtration unit (Sartorius, Göttingen, Germany) was used. The membrane was transferred to the surface of selective or differential media specific for each microbiological indicator tested, allowing only the target microorganism to grow. Chromogenic Coliforms Agar (CCA) (Scharlau, Sentmenat, Spain) was used for the detection and enumeration of EC and TC, Slanetz & Bartley Agar (Oxoid Ltd., Basingstoke, United Kingdom) for IE enumeration, and selective medium cetrimide nalidixic agar (CNA) (Scharlau, Sentmenat, Spain) for the isolation of PA. Agar plates were incubated aerobically at 36 ± 2°C for 21 ± 3 h (CCA) and 44 ± 4 h (Slanetz & Bartley and CN agar base). The HPC was determined by the pour plate technique using Yeast extract agar (YEA) (Scharlau, Sentmenat, Spain) according to the ISO 6222/2004 standard. Samples were incorporated in 1 and 0.1 ml of water and into the medium. The plates were incubated at 36 ± 2°C and 22 ± 2°C for 44 ± 4 h and 68 ± 4 h, respectively. The blue to blue-purple colonies were recorded as EC on the CCA medium. The pink to red colonies were classified as presumptive coliforms. For final confirmation of coliforms, pink to red colonies from each plate were transferred to nutrient agar (Oxoid Ltd., Basingstoke, United Kingdom). After 24 h of incubation, they were subjected to an oxidase test. The presumptive colonies that were oxidase negative were confirmed as TC. TC was reported as the sum of EC colonies and confirmed coliforms. Red, brown, or pink colonies grown on the Slanetz & Bartley medium were considered presumptive IE and transferred to the Bile esculin azide agar (BEAA) (Scharlau, Sentmenat, Spain) for confirmation. After 2 h of incubation at 44°C ± 0.5 h, the IE presumptive colonies hydrolyze esculin and form a brown to black compound, which diffuses into the medium. These typical brown to black colonies are considered positive reactions and are counted as IE. Colonies that produced pyocyanin on CN agar were considered PA. Other red-brown or fluorescent colonies were considered presumptive and were confirmed for their ability to produce ammonia from acetamide or by the oxidase reaction. Colonies that give a positive oxidase reaction were tested for fluorescein and ammonia production from acetamide. Individual or chain-forming colonies were counted, and after all the confirmation steps, the results were calculated, considering the dilution factor when appropriate. The results were expressed as colony-forming units (CFU) in 100 ml of water (for EC, TC, IE, PA) or in 1 ml of water (for HPC 22, HPC 37) (Masindi and Foteinis, 2021).
The microbial quality of the spring water was evaluated based on the Drinking Water Directive (98/83/EC) and World Health Organization (WHO) requirements. According to these regulations, water is considered potable if no EC, TC, or IE colony is detected in 100 ml of the water sample. For HPC, no numerical values have been set, but this regulation states that no abnormal change should be detected compared with the values obtained during routine analysis.
2.4 Quality control and quality assurance
Analytical grade chemicals and culture media were used to prepare all the reagents and reference materials. Double-distilled sterile water calibrated equipment and glassware were used for the experimental work. Special care was taken during the sample collection, transportation, and analysis to avoid other microbial contamination. Each set of analyses was conducted with a positive and negative control with specific reference strains for quality assurance purposes. Sterility tests of microaeroflora, work surfaces, sterile water, and verification of the quality performance of culture media were carried out to ensure the accuracy of the results.
2.5 Quantitative microbial risk assessment
Spring water quality may vary widely due to several variables, such as contamination, treatment operations, periodic assessment to detect microbial contamination as well as the presence of other pollutants. QMRA analysis was used to assess the human health risk due to exposure of adults and children to pathogenic microorganisms following spring water consumption. QMRA integrates the quantitative information on human exposures to pathogens (exposure assessment) and the probability that the exposures can determine infection or illness (dose-response relationship) (Seto et al., 2016; Amatobi and Agunwamba, 2022). The QMRA was conducted according to the following steps: hazard identification 1), dose-response assessment 2), exposure assessment 3), and risk characterization 4) (WHO, 2016). The pathogens and the adverse health effects were identified in the hazard identification step. In the present study, the presence of E. coli as QMRA index was used in accordance with previous approaches (Teunis et al., 2004; Haas et al., 2014; Carducci et al., 2020). Since 8% of the total E. coli is considered pathogenic, the dose was multiplied by 0.08 (WHO, 2016; Daley et al., 2019; Carducci et al., 2020). The dose-response assessment was established based on the Beta-Poisson model (Eq. 1). As a component of QMRA, the Beta-Poisson model establishes the relationship between the dose of a pathogen and the probability of adverse health effects such as infection, illness, or death occurring in the exposed population (Xie et al., 2016; Ahmed et al., 2020). The probability of infection per day (Pinf) was calculated using Eq. 1
where D represents the average dose ingested obtained by multiplying the consumed volume of water per day by the recorded average value of E. coli. The average number of ingested pathogenic E. coli was estimated by the mean concentration of bacteria and the volume of water consumed by adults and children per day. As the exposure by dermal contact and inhalation was not considered to have a major effect on the local consumer’s health, only the exposure by the ingestion of unboiled water was considered (Amatobi and Agunwamba, 2022). The α and β are the dose-response parameters for E. coli (Table 2). The risk of infection per day was calculated using the obtained values in January, April, June, and November 2021, while the risk of infection per year was calculated using the average values. The annual risk of infection (
where n is the number of days of exposure per year due to the E. coli dose, Pill is the probability of illness, and Pill/inf is the probability of illness per infection (Table 2). The estimated (
2.6 Data analysis
All water samples were analyzed in triplicate. For each spring and season, the mean value of the microbiological parameters was reported. QMRA indices were calculated based on Eqs 1–3 in Microsoft Excel 2016 MSO version 2,205 (Microsoft Corporation, Redmond, WA, United States) with the average E. coli concentration in each spring and season. For the QMRA data, mean, median, standard deviation (SD) and coefficient of variation (CV) were calculated. The CV was calculated as the ratio of the standard deviation to the mean and shows the extent of variability of data in each spring sample in relation to the mean value.
3 Results and discussion
3.1 Microbial contamination of karst springs
The seasonal microbiological results for the studied karst springs are presented in Figures 2A–E. In June and November, at least one faecal indicator was detected in 5 springs (GWR12, GWR14, GWR15, GWR16, and GWR19) except GWR17 where none of the tested indicators were present. Consequently, the water of these five springs is not suitable for drinking purposes as exceeds the acceptable limits set by Council Directive 98/83/EC, 1998 and WHO (2022). These guidelines specify that the values of the EC, TC, IE and PA (expressed as CFU) should be zero in the water samples (typically 100 ml). The mean ± SD values of the microbiological indicators found in GWR12 (EC = 123 ± 128; TC = 212 ± 120; IE = 18 ± 6.1; HPC 22 = 1,029 ± 1,224; HPC37 = 154 ± 211 CFU/100 ml), GWR14 (EC = 61 ± 60.04; TC = 105 ± 59.5; IE = 23 ± 26.3; HPC22 = 210 ± 102; HPC37 = 78 ± 79.9 CFU/100 ml), and GWR15 (EC = 17 ± 18.6; TC = 146 ± 140; IE = 28 ± 42.8; HPC22 = 1,041 ± 1,541; HPC37 = 97 ± 142 CFU/100 ml) were high. The PA bacterium was not detected in any of the studied samples.
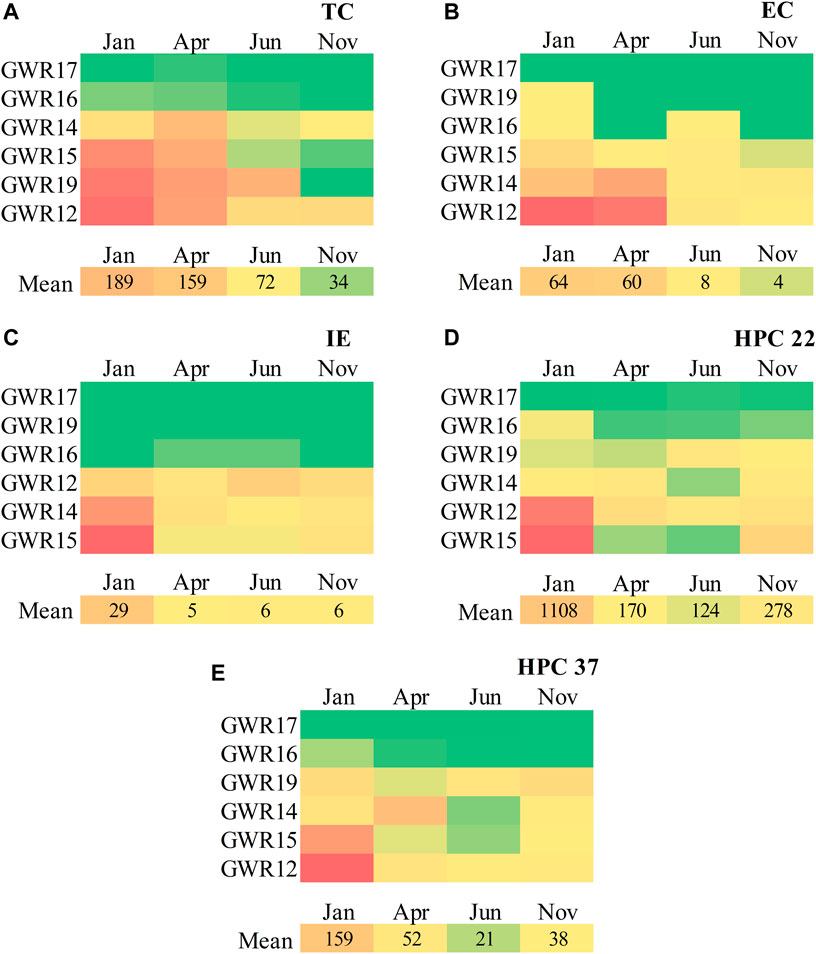
FIGURE 2. Concentration (CFU/100 ml) of microbiological indicators (A) Total coliforms (TC) (B); E. coli (EC); (C) intestinal enterococci (IE); (D) heterotrophic plate count at 22°C (HPC 22); (E) heterotrophic plate count at 37°C (HPC 37) in January, April, June, and November 2021, in the studied karst springs. The deep-slight color gradient presents the concentrations of microbiological indicators of highest (red) to intermediate (yellow) and lowest intensity (green).
3.1.1 Total coliforms
TC was detected in 83.3% of samples and ranged from 0 to 365, with mean of 113 ± 122 CFU/100 ml. The highest values was attributed to GWR12 (Figure 2A). The spring waters collected in January and April were all contaminated with coliforms, with a higher load in January. The lowest contamination frequency of TC, detected in 50% of samples, was obtained in November.
3.1.2 Escherichia coli
EC, the most reliable indicator of enteric diseases, has been detected in 62.5% of water samples from January to November 2021, with mean of 33.9 ± 69.1. Spring waters were more contaminated with EC in January (63.8 ± 95.5) and April (60.3 ± 94.7) than in June (7.5 ± 7.4) and November (4.0 ± 5.6), the highest concentration of EC (247 CFU/100 ml) being measured in GWR12 (Figure 2B). The presence of E. coli in the studied springs can be an indication that other pathogenic microorganisms can be present (Sinreich et al., 2013; Rodrigues and Cunha, 2017).
3.1.3 Intestinal enterococci
IE was detected in 58.3% of samples, with values ranging from 0 to 92, with mean of 11.5 ± 21.9 CFU/100 ml. IE bacteria were absent in all four seasons in GWR17 and GWR19, occasionally detected in GWR16, and always detected in GWR12, GWR14, and GWR15 during the study period. The highest IE contamination was recorded in January and April for GWR15 (Figure 2C).
3.1.4 Heterotrophic plate count
HPC 22 (Figure 2D) and HPC 37 (Figure 2E) showed high values of heterotrophs in January and April. The mean values for HP22 was 420 ± 843 and 67.5 ± 111 for HPC37, respectively. The maximum CFU was assigned to GWR15 (3300 CFU/100 ml) and GWR12 (2860 CFU/100 ml). The lowest concentration of microorganisms detected was obtained in June for all springs (Figures 2D,E), with HPC 22 concentrations decreasing by 9% compared to January. GWR17 had HPC values between 0 and 14 CFU/100 ml and correlated with the absence of TC, EC, and IE, has showed the lowest degree of water contamination. In the heterotrophic bacteria group, the opportunistic pathogens such as Pseudomonas spp., Klebsiella spp., Serratia spp., Aeromonas spp., Xanthomonas spp., Acinetobacter spp. Or Flavobacterium spp., are frequently present. Even if TC, EC, and IE indicators provide critical information on the microbiological quality of water, HPC results are also a valuable data on the variation of microbial load in time.
Overall, the obtained results showed microbial contamination of karst springs, with a significant decrease in June and November compared to January and April 2021. According to the European drinking water regulation (Council Directive 98/83/EC, 1998), five springs did not meet the requirements for the absence of EC, TC, and IE. Abundance pattern across samples was observed as follows: GWR12 > GWR14 > GWR15 > GWR16 > GWR19 > GWR17. The lowest level of contamination was observed in GWR17, for which only TC exceeded the allowed values for drinking water established to 0 CFU/100 ml. This overrun was recorded only in January (3 CFU/100 ml) and April (8 CFU/100 ml). For HPC 22 and HPC 37, the same Directive requires no abnormal changes in repeated tests over a period. Our data shows that HPC tends to change with the season, which can indicate a new configuration in the microbial load of the samples. Their values decrease as follows: January > April > November > June. Interestingly, the PA bacterium that is ubiquitous in the environment, was not detected in any water samples. This can be explained by its ability to adopt a viable but non-culturable state in water, or that the sampling was carried out outside the period of contamination with PA.
3.2 Microbial health risk assessment
3.2.1 Ingested dose of E. coli
The QMRA data showed high ingested doses of pathogenic E. coli, with high risks of infection and illness for five out of six springs. The presence of E. coli was not confirmed in the GWR17 samples, and thus, there were no risks of infection and illness through this enteropathogenic bacterium for the water consumers. For adults, assuming a water consumption of 2 L/day, the mean concentration of ingested E. coli decreased as follows: GWR 12 (197 ± 205) > GWR14 (97.2 ± 96.1) > GWR15 (26.8 ± 29.8) > GWR16 (3.2 ± 3.7) > GWR19 (1.6 ± 3.2).
The E. coli dose was twice as low for the children, as the water consumption was estimated to be 1 L/day (Table 3). The CV was high for the ingested dose (0.99–2.00), highlighting the strong inhomogeneity in E. coli contamination of samples during the four seasons.
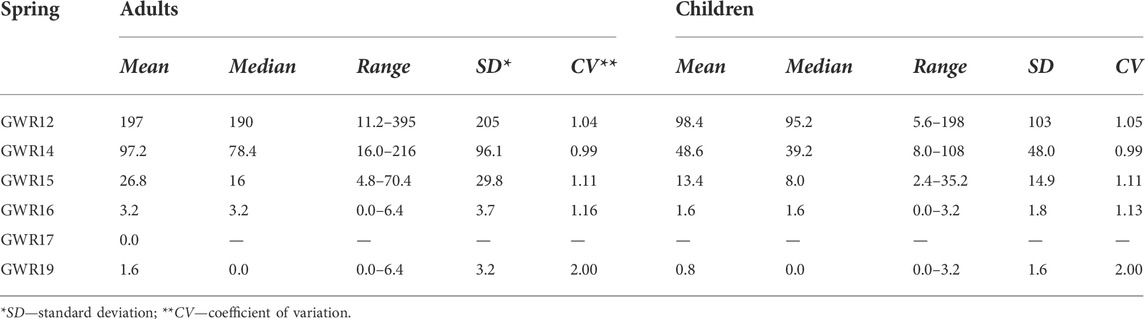
TABLE 3. The ingested dose of E. coli (ingested volume of water × mean concentration of E. coli expressed in CFU/100 ml) in relation with the water consumption by adults (2 L/person/day) and children (1 L/person/day).
3.2.2 Risk of infection
The values calculated for the risk of infection/day for adults and children due to the water consumption from the studied springs are given in Figure 3. The annual risk of infection for adults and children estimated based on the obtained results is presented in Table 4.
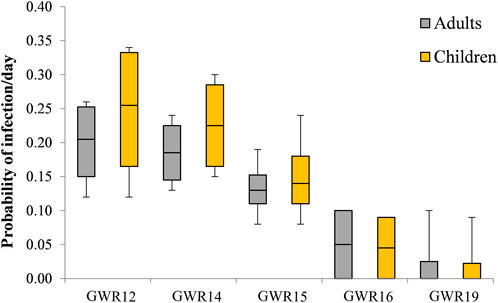
FIGURE 3. Box plots representation of the probability of infection/day for adults (grey) and children (yellow) for five out of six springs. The box reflects the inter quartile range (IQR). The black line in the box reflects the median of the four seasons. The whiskers reflect 1.5 × IQR. GWR17 had no graphic representation, as the calculated value for risk of infection/day was zero.
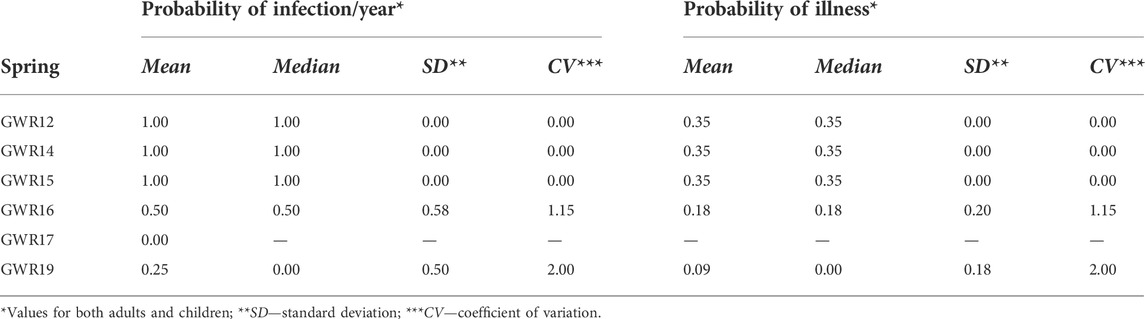
TABLE 4. Probability of infection/year and probability of illness for adults and children due to the consumption of water contaminated with pathogenic E. coli.
The mean risk of infection/day with E. coli was high for both adults and children. The water consumption from the GWR12 spring presented the highest mean infection/day value of 0.20 ± 0.07 for adults and 0.24 ± 0.11 for children. A high risk of infection per day was estimated also for GWR14 and GWR15 reaching values of 0.19 ± 0.05 and 0.13 ± 0.05 for adults, and 0.23 ± 0.08 and 0.15 ± 0.07 for children, respectively (Figure 3).
The mean risk of infection per year due to the E. coli presence ranged between 0.00 and 1.00. The GWR12, GWR14, and GWR15 springs reached a value of 1.00 ± 0.00 for the annual risk of infection for both adults and children. GWR16 and GWR19 reached values of 0.50 ± 0.58 and 0.25 ± 0.50 respectively, for both consumers’ categories.
According to the US EPA (1989) and WHO (2016), the acceptable annual risk of infection for the waterborne pathogen is 10–4. The obtained annual risk of infection values exceeded the acceptable risk for five of the studied springs indicating that health risks are probable to occur following exposure to E. coli by water consumption from unmonitored and untreated sources. At the same time, this may lead over time to significant persistence and transmission of pathogens to the environment and the possible occurrence of resistant bacteria (Coleman et al., 2013).
3.2.3 Risk of illness
The risk of illness due to E. coli infection (expressed as the probability of illness index) was estimated at 0.35 ± 0.00 for GWR12, GWR14, and GWR15, at 0.18 ± 0.20 for GWR16, and at 0.09 ± 0.18 for GWR19 (Table 4). For these last two springs (GWR16 and GWR19), the CV for the probability of illness was high (1.15–2.00), emphasizing the substantial changes in the contamination circumstances during the study period. Surprisingly, no differences in the probability of illness between adults and children were observed.
3.3 Potential microbiological contamination sources
Given the clear evidence that microbiological contamination occurred in the tested samples, understanding the springs’ particularity, the potential contamination sources and their trigger factors is essential for development of a good water management strategy. In the Apuseni Mountains, the surface karst features (e.g., ponors, dolines, blind valleys) enhance the contamination from diffuse sources such as agriculture (manure spreading), untreated discharges from households or animal husbandry, and small scale-tourism activities.
Most of the study area is covered by forest or meadows, where sheep, goats or cattle usually graze or cross over to other pasture lands. Septic tanks, animal stables, and outbuildings are also frequently found in the spring’s catchment area. Local agriculture, minimal subsistence, or semi-subsistence farms are not engaged in environmentally friendly practices. The large number of small farms combined with poor livestock management and underdeveloped sanitation, leads to nitrate and microbial contamination (Word Bank, 2018). Wildlife may also contribute to faecal contamination (Guber et al., 2015; Paruch et al., 2019). These factors are likely to explain the EC and IE occurrence in the water samples, as these bacteria usually live in the faeces of warm-blooded animals. TC, which are usually found in the warm-blooded intestine of mammals and in the environment, provides clues that other potentially pathogens may be present. Even if it was initially thought that coliforms concentration is low in groundwater (Sinton, 1982), it has been shown to be strongly correlated with depth-to-bedrock at well sites and nearby agricultural land use (Borchardt et al., 2021). The overflow of septic tanks was frequently reported as a source of groundwater contamination linked to disease outbreaks (Kumar et al., 2014; Siwila and Buumba, 2021). Due to the thin soil profiles, saturated soil, land slope, or water concentration within the epikarst zone, the microbiological pollution on the surface converges into the local aquifers enabling the release of the contaminants through the groundwater (Buckerfield et al., 2019). The vulnerability of karst aquifers to microbial contamination or other pollutants increases under certain circumstances. Thus, environmental and hydrological conditions, such as rainfall intensity and duration, permeability, humidity, and temperature of the soil, significantly influence faecal bacteria occurrence (Pandey et al., 2014).
In our case, it was assumed that the rain episodes from January and April, before sample collection, increased the abundance of faecal bacteria due to the rapid infiltration of the microorganisms in waters (Taylor et al., 2004; Ender et al., 2018). In the dry and warm season of June, the faecal bacteria population decreased.
3.4 Significance of E coli presence in the water
The QMRA data clearly indicated that the tested water is inadequate and unsafe for consumption. The consumers with the highest risk of manifesting illnesses associated with E. coli are those who consume water from GWR12, GWR14 and GWR15 (Pill = 0.35). Although GWR19 had the lowest load of contamination with E. coli and a value of Pill = 0.09, the water is not safe for drinking as the WHO requirements specifying that safe water for drinking purposes should be free of E. coli and other faecal bacteria. Moreover, detection of E. coli it is considered as a health hazard (Charles et al., 2020). As a particular finding, it can be noted that consumption of water from GWR17 does not present a real risk of illness due to the absence of E. coli. However, the assumption that GWR17 is good for drinking is questionable because the regulated criteria for drinking water acceptance involve in addition to complete set of microbiological parameters, testing several chemical parameters as well.
Consumption of/or contact with E. coli contaminated water can lead to serious acute, chronic, or sometimes fatal health consequences. E. coli typically lives in the digestive tract of humans and animals. However, an imbalance of intestinal microflora can cause disease through a massive multiplication of toxicogenic strains. E. coli O157 is one of the toxicogenic strains that can cause serious adverse health effects, such as hemorrhagic diarrhea, nausea, fever, vomiting, headaches, chills, or abdominal cramps (WHO, 2022). Generally, the effects of exposure to pathogens and especially to E. coli, through drinking water are not the same for all individuals. Prevalence of immunity and infectious agent involved play an important role in contacting the infection and developing symptomatic disease. The most vulnerable to infections and illness are children, pregnant women, elderly or immunocompromised individuals. Healthy people with a high level of immunity are less susceptible to the risk of infections caused by pathogens (Ding et al., 2017). Frequent exposure to pathogens decreases the probability or severity of illness due to acquired immunity (WHO, 2022). Acquired immunity is specific for healthy local people, which develop resistance or immunity to pathogens after infection and recovery. However, the asymptomatic infected population can contribute to the secondary spread of pathogens. According to the National Institute of Statistics (Statistical Yearbook of Romania, 2019), in 2018 in Romania, the most common infectious diseases were acute diarrheal diseases (78,286), dysentery (149), and salmonellosis (1,469), however, no statistical data mention how many of these diseases are associated with the consumption of contaminated water.
3.5 Strengths and limitation of the study
The present study results do not offer a comprehensive representation model for the spring’s features due to some limitations. The first limitation was the number of samples. Only 24 samples were taken from six springs during the year 2021. Considering the rapid changes of microbiological quality, broader approach of water sampling will be aimed in the future. By increasing the number of samples and the sampling frequency, the accuracy and confidence of the results will increase (Levy et al., 2012). Extending the microbiological monitoring downstream of the karst zone will also contribute to a better understanding extent of contamination. The second limitation was the different meteorological conditions during sampling campaigns, which significantly influenced the results of the risk scenarios. Hydrometeorological changes such as heavy rainfalls from winter and spring have made some areas difficult to access for the planned sampling campaigns. This not only caused logistical difficulties but also an assumption that the results will be strongly impacted by these events. As an understanding of this context, it can be noted that water consumption during these weather conditions can be overestimated because people consume less or no water that is visible turbid. The third limitation refers to the selection of the most appropriate microbial indicators. The E. coli and intestinal enterococci are the recommended microbial indicators for the testing routine, but many waterborne-related pathogens could be also considered, since they show little correlation with such faecal bacterial indicators, especially viruses (e.g., hepatitis A virus, hepatitis E virus), pathogenic helminths and parasitic protozoa. Generally, the number of parameters tested are limited and therefore, do not capture all microbial contamination of the samples.
Even so, the obtained results provide important knowledge on the microbiological quality of the karst springs both for consumers and authorities. The QMRA results can help the decision-makers and authorities taking urgent actions to assure access to safe drinking water in rural communities.
Based on the QMRA model, probabilities of infection and illness can be estimated for other future situations, such as: exposure to water contaminated by dermal contact or inhalation, the probability of illness for people who occasionally drink water (tourists), pregnant women, or ill people. The assessment of microbial contamination can also be extended to non-karst zones, with main focus on identifying sources of contamination and protecting the vulnerable layers of aquifers.
In order to know if springs waters contain potentially harmful microorganisms, a testing and monitoring program is needed. The locals should be constantly informed about issues affecting water quality, infectious disease outbreaks, and preventive measures that can be taken to reduce the occurrence of microbial contamination.
4 Conclusion
The microbiological characteristics of six karst springs from a rural area in the Apuseni Mountains, Romania were investigated. Local communities use these unprotected karst waters without treatment or quality evaluation. The microbiological analysis revealed high levels of faecal contamination that may pose serious health risks to the consumers. Five out of six karst springs tested exceeded the maximum limits established by the WHO and the European Directive 98/93/EC. The quantitative microbial risk assessment calculated for pathogenic E. coli indicated a high risk of infection per day and a high probability of illness for waters contaminated with this bacterium. Long-term monitoring of water quality simultaneously with public health awareness for the inhabitants is needed in the region. In addition, the prevention and reduction of groundwater contamination by the responsible agencies would minimize the risk of disease outbreaks and increase the quality of life for rural communities. Overall, the obtained data brings more insights about microbial contamination of karst groundwaters in Romania, raises awareness on those responsible, sets new research priorities; all with the aim of increasing interest in the inestimable value of groundwater.
Data availability statement
The raw data supporting the conclusion of this article will be made available by the authors, without undue reservation.
Author contributions
Conceptualization, ZS, EAL, and OTM; methodology, ZS; validation, ZS; investigation, ZS; data curation, ZS and EN; writing-original draft preparation, ZS; writing-review and editing EAL, OTM, EN, AB, ES, and ZS; visualization OTM, EN, EAL, and ZS; funding acquisition, OTM. All authors have read and agreed to the published version of the manuscript.
Funding
This research was funded by the EEA Financial Mechanism 2014-2021 under the project EEA-RO-NO-2018–0138 (GROUNDWATERISK), contract No. 4/2019 and by the Ministry of Research, Innovation and Digitization through Program 1-Development of the national research anddevelopment system, subprogram 1.2 Institutional performance—Projects that finance the RDI excellence, contract no. 18 PFE/30.12.2021. The APC was funded by the EEA Financial Mechanism 2014-2021 under the project EEA-RO-NO-2018–0138 (GROUNDWATERISK), contract No. 4/2019.
Acknowledgments
The authors are grateful to Dr. Ionuț Cornel Mirea for the help with Figure 1; Dr. Traian Brad for the April sampling campaign; and prof. Dr. Horia Leonard Banciu for his valuable suggestions during the manuscript preparation.
Conflict of interest
The authors declare that the research was conducted in the absence of any commercial or financial relationships that could be construed as a potential conflict of interest.
Publisher’s note
All claims expressed in this article are solely those of the authors and do not necessarily represent those of their affiliated organizations, or those of the publisher, the editors and the reviewers. Any product that may be evaluated in this article, or claim that may be made by its manufacturer, is not guaranteed or endorsed by the publisher.
References
Ahmed, J., Wong, L. P., Chua, Y. P., Channa, N., Mahar, R. B., Yasmin, A., et al. (2020). Quantitative microbial risk assessment of drinking water quality to predict the risk of waterborne diseases in primary-school children. Ijerph 17, 2774. doi:10.3390/ijerph17082774 | |
Amatobi, D. A., and Agunwamba, J. C. (2022). Improved quantitative microbial risk assessment (QMRA) for drinking water sources in developing countries. Appl. Water. Sci. 12, 49. doi:10.1007/s13201-022-01569-8 |
Bakalowicz, M. (2005). Karst groundwater: A challenge for new resources. Hydrogeol. J. 13, 148–160. doi:10.1007/s10040-004-0402-9 |
Boadi, N. O., Saah, S. A., Baa-Poku, F., Mensah, E. A., and Addo, M. (2020). Safety of borehole water as an alternative drinking water source. Sci. Afr. 10, e00657. doi:10.1016/j.sciaf.2020.e00657 |
Boelee, E., Geerling, G., van der Zaan, B., Blauw, A., and Vethaak, D. (2019). Water and health: From environmental pressures to integrated responses. Acta Trop. 193, 217–226. doi:10.1016/j.actatropica.2019.03.011 | |
Borchardt, M. A., Stokdyk, J. P., Kieke, B. A., Muldoon, M. A., Spencer, S. K., Firnstahl, A. D., et al. (2021). Sources and risk factors for nitrate and microbial contamination of private household wells in the fractured dolomite aquifer of Northeastern Wisconsin. Environ. Health Perspect. 129 (6), 067004. doi:10.1289/EHP7813 |
Buckerfield, S. J., Waldron, S., Quilliam, R. S., Naylor, L. A., Li, S., and Oliver, D. M. (2019). How can we improve understanding of faecal indicator dynamics in karst systems under changing climatic, population, and land use stressors? - research opportunities in SW China. Sci. Total Environ. 646, 438–447. doi:10.1016/j.scitotenv.2018.07.292 | |
Carducci, A., Federigi, I., Cioni, L., Landucci, A., Donzelli, G., Iannelli, R., et al. (2020). Approach to a water safety plan for recreational waters: Disinfection of a drainage pumping station as an unconventional point source of fecal contamination. H2Open J. 3, 1–9. doi:10.2166/h2oj.2020.017 |
Charles, K. J., Nowicki, S., and Bartram, J. K. (2020). A framework for monitoring the safety of water services: From measurements to security. NPJ Clean. Water 3, 36. doi:10.1038/s41545-020-00083-1 |
Coleman, B., Louie, M., Salvadori, M., McEwen, S. A., Neumann, N., Sibley, K., et al. (2013). Contamination of Canadian private drinking water sources with antimicrobial resistant Escherichia coli. Water Res. 47, 3026–3036. doi:10.1016/j.watres.2013.03.008 | |
Council Directive 98/83/EC (1998). Council Directive 98/83/EC of 3 November on the quality of water intended for human consumption. Official J. L 330, 05–12. Available At: https://eur-lex.europa.eu/legal-content/RO/TXT/PDF/?uri=CELEX:31998L0083&from=EN (Accessed April 20, 2022).
Cucoș, A. L., Moldovan, M. C., Burghele, B. D., Dicu, T., and Moldovan, O. T. (2021). Radiological risk assessment for karstic springs used as drinking water in rural Romania. Atmosphere 12, 1207. doi:10.3390/atmos12091207 |
Daley, K., Jamieson, R., Rainham, D., Truelstrup Hansen, L., and Harper, S. L. (2019). Screening-level microbial risk assessment of acute gastrointestinal illness attributable to wastewater treatment systems in Nunavut, Canada. Sci. Total Environ. 657, 1253–1264. doi:10.1016/j.scitotenv.2018.11.408 | |
Danube Water Program (2015). Water and wastewater services in the danube region. Available At: https://sos.danubis.org/eng/country-notes/romania/ (Accessed April 20, 2022).
Dehghani, M. H., Zarei, A., Yousefi, M., Baghal Asghari, F. B., and Haghighat, G. A. (2019). Fluoride contamination in groundwater resources in the southern Iran and its related human health risks. Dwt 153, 95–104. doi:10.5004/dwt.2019.23993 |
Ding, Z., Zhai, Y., Wu, C., Wu, H., Lu, Q., Lin, J., et al. (2017). Infectious diarrheal disease caused by contaminated well water in Chinese schools: A systematic review and meta-analysis. J. Epidemiol. 27 (6), 274–281. doi:10.1016/j.je.2016.07.006 | |
Ender, A., Goeppert, N., and Goldscheider, N. (2018). Hydrogeological controls of variable microbial water quality in a complex subtropical karst system in Northern Vietnam. Hydrogeol. J. 26, 2297–2314. doi:10.1007/s10040-018-1783-5 |
Ezeh, O. K., Agho, K. E., Dibley, M. J., Hall, J., and Page, A. N. (2014). The impact of water and sanitation on childhood mortality in Nigeria: Evidence from demographic and health surveys, 2003-2013. Ijerph 11 (9), 9256–9272. doi:10.3390/ijerph110909256 | |
GBD 2017 Risk Factor Collaborators (2018). Global, regional, and national comparative risk assessment of 84 behavioural, environmental and occupational, and metabolic risks or clusters of risks for 195 countries and territories, 1990-2017: A systematic analysis for the global burden of disease study 2017. Lancet 392, 1923–1994. doi:10.1016/S0140-6736(18)32225-6 | |
Ghasemizadeh, R., Hellweger, F., Butscher, C., Padilla, I., Vesper, D., Field, M., et al. (2012). Review: Groundwater flow and transport modeling of karst aquifers, with particular reference to the North Coast Limestone aquifer system of Puerto Rico. Hydrogeol. J. 20 (8), 1441–1461. doi:10.1007/s10040-012-0897-4 | |
Goshu, G., Koelmans, A. A., and de Klein, J. J. M. (2021). Performance of faecal indicator bacteria, microbial source tracking, and pollution risk mapping in tropical water. Environ. Pollut. 276, 116693. doi:10.1016/j.envpol.2021.116693 | |
Grisey, E., Belle, E., Dat, J., Mudry, J., and Aleya, L. (2010). Survival of pathogenic and indicator organisms in groundwater and landfill leachate through coupling bacterial enumeration with tracer tests. Desalination 261, 162–168. doi:10.1016/j.desal.2010.05.007 |
Grönwall, J., and Danert, K. (2020). Regarding groundwater and drinking water access through a human rights lens: Self-supply as a norm. Water 12, 419. doi:10.3390/w12020419 |
Guber, A. K., Fry, J., Ives, R. L., and Rose, J. B. (2015). Escherichia coli survival in, and release from, white-tailed deer feces. Appl. Environ. Microbiol. 81, 1168–1176. doi:10.1128/AEM.03295-14 | |
Haas, C. N., Rose, J. B., and Gerba, C. P. (2014). Quantitative microbial risk assessment. New York: John Wiley & Sons.
Hoaghia, M. A., Moldovan, A., Kovacs, E., Mirea, I. C., Kenesz, M., Brad, T., et al. (2021). Water quality and hydrogeochemical characteristics of some karst water sources in Apuseni Mountains, Romania. Water 13, 857. doi:10.3390/w13060857 |
Johnson, T. D., Belitz, K., Kauffman, L. J., Watson, E., and Wilson, J. T. (2022). Populations using public-supply groundwater in the conterminous U.S. 2010; Identifying the wells, hydrogeologic regions, and hydrogeologic mapping units. Sci. Total Environ. 806 (2), 150618. doi:10.1016/j.scitotenv.2021.150618 | |
Kongprajug, A., Denpetkul, T., Chyerochana, N., Mongkolsuk, S., and Sirikanchana, K. (2021). Human fecal pollution monitoring and microbial risk assessment for water reuse potential in a coastal industrial-residential mixed-use watershed. Front. Microbiol. 12, 647602. doi:10.3389/fmicb.2021.647602 | |
Kumar, A., Nirpen, L., Ranjan, A., Gulati, K., Thakur, S., and Jindal, T. (2014). Microbial groundwater contamination and effective monitoring system. Asian J. Environ. Sci. 9 (1), 37–48.
Levy, K., Nelson, K. L., Eisenberg, A., and Hubbard, J. N. (2012). Rethinking indicators of microbial drinking water quality for health studies in tropical developing countries: Case study in northern coastal Ecuador. Am. J. Trop. Med. Hyg. 86, 499–507. doi:10.4269/ajtmh.2012.11-0263 | |
Llopis-González, A., Sánchez, A. L., Requena, P., and Suárez-Varela, M. (2014). Assessment of the microbiological quality of groundwater in three regions of the Valencian community (Spain). Ijerph 11, 5527–5540. doi:10.3390/ijerph110505527 | |
Machdar, E., van der Steen, N. P., Raschid-Sally, L., and Lens, P. N. L. (2013). Application of Quantitative Microbial Risk Assessment to analyze the public health risk from poor drinking water quality in a low income area in Accra, Ghana. Sci. Total Environ. 449, 134–142. doi:10.1016/j.scitotenv.2013.01.048 | |
Macler, B. A., and Merkle, J. C. (2000). Current knowledge on groundwater microbial pathogens and their control. Hydrogeology J. 8, 29–40. doi:10.1007/PL00010972 |
Masindi, V., and Foteinis, S. (2021). Groundwater contamination in sub-Saharan Africa: Implications for groundwater protection in developing countries. Clean. Eng. Technol. 2, 100038. doi:10.1016/j.clet.2020.100038 |
Moldovan, O., T., Øvrevik Skoglund, R., Banciu, R., Dinu Cucoș, H. L., LeveiCucoș, A., Perșoiu, E. A., et al. (2019). Monitoring and risk assessment for groundwater sources in rural communities of Romania (GROUNDWATERISK). Rio 5, e48898. doi:10.3897/rio.5.e48898 |
Odiyo, J. O., Mathoni, M. M., and Makungo, R. (2020). Health risks and potential sources of contamination of groundwater used by public schools in Vhuronga 1, Limpopo province, South Africa. Ijerph 17, 6912. doi:10.3390/ijerph17186912 | |
Ozgumus, O. B., Celik-Sevim, E., Alpay-Karaoglu, S., Sandalli, C., and Sevim, A. (2007). Molecular characterization of antibiotic resistant Escherichia coli strains isolated from tap and spring waters in a coastal region in Turkey. J. Microbiol. 45 (5), 379–387. |
Pandey, P. K., Kass, P. H., Soupir, M. L., Biswas, S., and Singh, V. P. (2014). Contamination of water resources by pathogenic bacteria. Amb. Expr. 4, 51. doi:10.1186/s13568-014-0051-x | |
Paruch, L., Paruch, A. M., Eiken, H. G., and Sørheim, R. (2019). Faecal pollution affects abundance and diversity of aquatic microbial community in anthropo-zoogenically influenced lotic ecosystems. Sci. Rep. 9, 19469. doi:10.1038/s41598-019-56058-x | |
Petrescu-Mag, R. M., Petrescu, D. C., Safirescu, O. C., Hetvary, M., Oroian, I. G., and Vâju, D. (2016). Developing public policy options for access to drinking water in peripheral, disaster and polluted rural areas: A case study on environment-friendly and conventional technologies. Water 8, 80. doi:10.3390/w8030080 |
Pitkänen, T., Karinen, P., Miettinen, I. T., Lettojärvi, H., Heikkilä, A., Maunula, R., et al. (2011). Microbial contamination of groundwater at small community water supplies in Finland. Ambio 40, 377–390. doi:10.1007/s13280-010-0102-8 | |
Ravbar, N., Petrič, M., Blatnik, M., and Švara, A. (2021). A multi-methodological approach to create improved indicators for the adequate karst water source protection. Ecol. Indic. 126, 107693. doi:10.1016/j.ecolind.2021.107693 |
Ravbar, N., Engelhardt, I., and Goldscheider, N. (2011). Anomalous behaviour of specific electrical conductivity at a karst spring induced by variable catchment boundaries: The case of the podstenjšek spring, Slovenia. Hydrol. Process. 25 (13), 2130–2140. doi:10.1002/hyp.7966 |
Ritchie, H., and Roser, M. (2021). Clean water and sanitation. Available At: https://ourworldindata.org/clean-water-sanitation (Accessed July 05, 2022).
Roba, C. A., Codrea, V., Moldovan, M., Baciu, C., and Cosma, C. (2010). Radon and radium content of some cold and thermal aquifers from Bihor County (Northwestern Romania). Geofluids 10 (4), 571–585. doi:10.1111/j.1468-8123.2010.00316.x |
Rodrigues, C., and Cunha, M. Â. (2017). Assessment of the microbiological quality of recreational waters: Indicators and methods. Euro-Mediterr. J. Environ. Integr. 2, 25. doi:10.1007/s41207-017-0035-8 |
Rufino, F., Busico, G., Cuoco, E., Muscariello, L., Calabrese, S., and Tedesco, D. (2021). Geochemical characterization and health risk assessment in two diversified environmental settings (Southern Italy). Environ. Geochem. Health 44, 2083–2099. doi:10.1007/s10653-021-00930-1 | |
Sasakova, N., Gregova, G., Takacova, D., Mojzisova, J., Papajova, I., Venglovsky, J., et al. (2018). Pollution of surface and ground water by sources related to agricultural activities. Front. Sustain. Food Syst. 2, 42. doi:10.3389/fsufs.2018.00042 |
Scharping, R. J., and Garey, J. R. (2021). Relationship between aquifer biofilms and unattached microbial indicators of urban groundwater contamination. Mol. Ecol. 30 (1), 324–342. doi:10.1111/mec.15713 | |
Seto, E. Y., Konnan, J., Olivieri, A. W., Danielson, R. E., and Gray, D. M. (2016). A quantitative microbial risk assessment of wastewater treatment plant blending: Case study in san francisco bay. Environ. Sci. Water Res. Technol. 2, 134–145. doi:10.1039/C5EW00147A |
Singh, A. K., Das, S., Singh, S., Pradhan, N., Gajamer, V. G., Kumar, S., et al. (2019). Physicochemical parameters and alarming coliform count of the potable water of eastern himalayan state Sikkim: An indication of severe fecal contamination and immediate health risk. Front. Public Health 7, 174. doi:10.3389/fpubh.2019.00174 | |
Sinreich, M., Pronk, M., and Kozel, R. (2013). Microbiological monitoring and classification of karst springs. Environ. Earth. Sci. 71, 563–572. doi:10.1007/s12665-013-2508-7 |
Sinton, L. W. (1982). A groundwater quality survey of an unsewered, semi‐rural area. N. Z. J. Mar. Freshw. Res. 16, 317–326. doi:10.1080/00288330.1982.9515975 |
Siwila, S., and Buumba, C. (2021). Investigation of groundwater contamination in relation to septic systems in Kitwe West Township, Kitwe, Zambia. Water Sci. Technol. 84 (10-11), 3277–3285. doi:10.2166/wst.2021.448 | |
Smith, M., Cross, K., Paden, M., and Laban, P. (2016). Managing groundwater sustainably. Gland, Switzerland: IUCN.
ISO 16266:2006 (2006). Water quality – Detection and enumeration of Pseudomonas aeruginosa – Method by membrane filtration. Geneva: International Organization for Standardization.
ISO 19458:2006 (2006). Water quality – Sampling for microbiological analysis. Geneva: International Organization for Standardization.
ISO 6222:1999 (1999). Water quality – Enumeration of culturable micro-organisms — Colony count by inoculation in a nutrient agar culture medium. Geneva: International Organization for Standardization.
ISO 7899-2:2000 (2000). Water quality – Detection and enumeration of intestinal enterococci — Part 2: Membrane filtration method. Geneva: International Organization for Standardization.
ISO 9308-1:2014 (2014). Water quality – Enumeration of Escherichia coli and coliform bacteria – Part 1: Membrane filtration method for waters with low bacterial background flora. Geneva: International Organization for Standardization.
Statistical Yearbook of Romania (2019). Statistical yearbook of Romania. Bucharest: National Institute of Statistics. Available At: https://insse.ro/cms/files/Anuar%20arhive/serii%20de%20date/2019/anuarul_statistic_al_romaniei_2019.pdf (Accessed April 20, 2022).
Surd, V., and Turnock, D. (2000). Romania's Apuseni Mountains: Safeguarding a cultural heritage. GeoJournal 50, 285–304. doi:10.1023/a:1007170018729 |
Taylor, R., Cronin, A., Pedley, S., Barker, J., and Atkinson, T. (2004). The implications of groundwater velocity variations on microbial transport and wellhead protection â" review of field evidence. FEMS Microbiol. Ecol. 49 (1), 17–26. doi:10.1016/j.femsec.2004.02.0180123456789 | |
Teunis, P., Takumi, K., and Shinagawa, K. (2004). Dose response for infection by Escherichia coli O157:H7 from outbreak data. Risk Anal. 24 (2), 401–407. doi:10.1111/j.0272-4332.2004.00441.x | |
United Nations (2010). Resolution 64/292 the human right to water and sanitation. Available At: https://documents-dds-ny.un.org/doc/UNDOC/GEN/N09/479/35/PDF/N0947935.pdf?OpenElement (Accessed June 13, 2022).
U.S. Environmental Protection Agency (EPA) (2012). Microbial risk assessment guideline: Pathogenic organisms with focus on food and water. Available At: https://www.epa.gov/sites/production/files/2013-09/documents/mra-guideline-final.pdf (Accessed March 23, 2022).
U.S. Environmental Protection Agency (EPA) (1989). National primary drinking water regulations 40 CFR: Filtration, disinfection, turbidity, giardia lamblia, viruses, legionella, and heterotrophic bacteria. Final rule. Fed. Regist. 54, 27486.
Velis, M., Conti, K., and Biermann, F. (2017). Groundwater and human development: Synergies and trade-offs within the context of the sustainable development goals. Sustain. Sci. 12, 1007–1017. doi:10.1007/s11625-017-0490-9 | |
Viban, T. B., Herman, O-N. N., Layu, T. C., Madi, O. P., Nfor, E. N., Kingsly, M. T., et al. (2021). Risk factors contributing to microbiological contamination of boreholes and hand dug wells water in the Vina Division, Adamawa, Cameroon. AiM 11, 90–108. doi:10.4236/aim.2021.112007 |
World Bank (2018). Romania water diagnostic report: Moving toward EU compliance, inclusion, and water security. Washington, DC: World Bank. Available At: https://documents1.worldbank.org/curated/en/114311530025860150/pdf/127630-REVISED-W18010.pdf (Accessed March 10, 2022).
World Health Organization and United Nations Children’s Fund (UNICEF) (2021). Progress on household drinking water, sanitation and hygiene 2000-2020: Five years into the SDGs. World Health Organization. Available At: https://apps.who.int/iris/handle/10665/345081 (Accessed July 01, 2022).
World Health Organization (2019). Drinking-water: Key facts. Available At: https://www.who.int/news-room/fact-sheets/detail/drinking-water (Accessed April 20, 2022).
World Health Organization (2016). Quantitative microbial risk assessment: Application for water safety management. Available At: https://apps.who.int/iris/handle/10665/246195 (Accessed April 20, 2022).
World Health Organization (2017). Safely managed drinking water - thematic report on drinking water 2. Available At: https://apps.who.int/iris/handle/10665/325897 (Accessed June 10, 2022).
World Health Organization (2005). Sustainable development and healthy environments cluster. Available At: https://apps.who.int/iris/handle/10665/43403 (Accessed June 10, 2022).
World Health Organzation (2022). Guidelines for drinking-water quality: Fourth edition incorporation the first and second addenda. Available At: https://www.who.int/publications/i/item/9789240045064 (Accessed May 30, 2022).
Wu, J., Li, P., and Qian, H. (2015). Hydrochemical characterization of drinking groundwater with special reference to fluoride in an arid area of China and the control of aquifer leakage on its concentrations. Environ. Earth Sci. 73 (12), 8575–8588. doi:10.1007/s12665-015-4018-2 |
Keywords: karst spring, microbial contamination, quantitative microbial risk assessment (QMRA), rural communities, drinking water
Citation: Stupar Z, Levei EA, Neag E, Baricz A, Szekeres E and Moldovan OT (2022) Microbial water quality and health risk assessment in karst springs from Apuseni Mountains, Romania. Front. Environ. Sci. 10:931893. doi: 10.3389/fenvs.2022.931893
Received: 29 April 2022; Accepted: 30 August 2022;
Published: 16 September 2022.
Edited by:
Efthalia Chatzisymeon, University of Edinburgh, United KingdomReviewed by:
Ileana Federigi, University of Pisa, ItalyAnand Archana, Columbia University, United States
Copyright © 2022 Stupar, Levei, Neag, Baricz, Szekeres and Moldovan. This is an open-access article distributed under the terms of the Creative Commons Attribution License (CC BY). The use, distribution or reproduction in other forums is permitted, provided the original author(s) and the copyright owner(s) are credited and that the original publication in this journal is cited, in accordance with accepted academic practice. No use, distribution or reproduction is permitted which does not comply with these terms.
*Correspondence: Erika Andrea Levei, ZXJpa2EubGV2ZWlAaWNpYS5ybw==