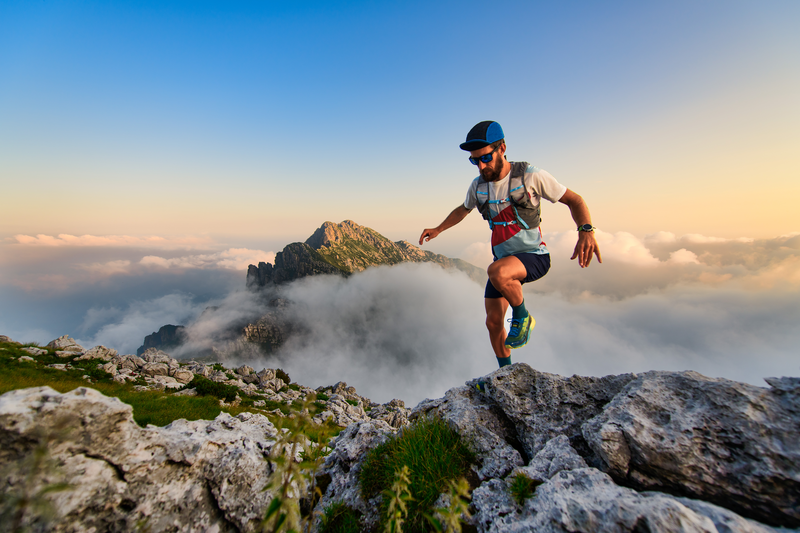
95% of researchers rate our articles as excellent or good
Learn more about the work of our research integrity team to safeguard the quality of each article we publish.
Find out more
ORIGINAL RESEARCH article
Front. Environ. Sci. , 14 July 2022
Sec. Environmental Economics and Management
Volume 10 - 2022 | https://doi.org/10.3389/fenvs.2022.930322
This article is part of the Research Topic Green Innovation and Industrial Ecosystem Reconstruction in Achieving Environmental Sustainability View all 34 articles
The transition to a low-carbon energy system is imminent under the constraints of carbon-peaking and carbon-neutral targets. Undoubtedly, coal-related carbon emissions over the past decades have had profound negative impacts on human life and the global climate. However, the main position of coal in the energy system determines that pure coal reduction strategy will inevitably lead to a systemic energy crisis. To this end, we explore the conflict formation mechanism among coal enterprises, downstream coal-fired power plants, and government in the process of strategic energy decarbonization transformation from the perspective of industrial chain, and analyze the feasible conflict states and their NASH, GMR, SMR, and SEQ equilibrium characteristics by constructing a ternary GMCR model. It is found that there are two feasible conflict states
Global warming headlines are always in the mass media, and counterintuitive seasonal climate change has erupted in many countries around the world (Howe, 2021). Various measures to reduce carbon emissions are being implemented, and energy decarbonization is an important topic in the field of energy management and environmental sustainability research (Sun et al., 2020; Savina et al., 2021; Vatalis et al., 2022; Ćorović et al., 2022). As the world’s largest developing country and carbon emitter, China is deemed vital to both global economic recovery (Verma et al., 2021) and CO2 emission reduction, especially given the current influence of multiple factors, such as the COVID-19 pandemic and China’s carbon-peaking and carbon-neutral targets (Jiang et al., 2021; Li et al., 2021). The thorny issue is that rapid economic development requires a large amount of energy supply (Gozgor et al., 2018), and the coal-based energy structure, which is determined by resource endowment, historical background, and economic development, makes it impossible for China, as the world’s second-largest economy, to fundamentally shake the main energy source of coal and electricity in the short term.
The feasibility of deep decarbonization of energy systems and their specific pathways are prerequisites for achieving the goals of carbon peaking and carbon neutrality. Numerous studies have been carried out on reducing CO2 emissions and alleviating fossil fuel dependence for sustainable energy transitions at the national level (Broto et al., 2018; Bompard et al., 2020). Furthermore, as the national top-level design continues to improve, energy restructuring studies at the provincial level are also gaining attention (Tan et al., 2016; Luo et al., 2021). The industrial CO2 emission efficiency of China’s provinces has three categories of high, medium, and low efficiency, and shows significant spatial agglomeration characteristics. Among them, the Northwest region has the greatest potential for industrial CO2 emission reduction (Zhang et al., 2016).
At a relatively microscopic level, based on institutional economics and transaction cost theory, Tan and Liu (2015) explored the boundary selection problem of coal and electricity trading from the perspective of time development and asset specialization. They provided effective strategies for trading coal and electricity enterprises at the theoretical level, technical level, and realistic level. Liu and Tan (2017) analyzed the trading characteristics between coal and power generation firms and their influencing factors from the perspective of stable matching and scale linkage, and found that coal firms are more scale efficient only when their production scale is larger than the maximum scale of coal demand that power generation firms provide for themselves. However, the pricing mechanism and price regulation of coal and electricity prices in the process of coal and electricity trading are not considered in their models. Kang and Yang (2012) analyzed the coal price between coal and power generation enterprises based on the infinite round bargaining game model and found that increasing the feed-in tariff can increase the profit of both power generation enterprises and coal enterprises, which is conducive to alleviating the coal price conflict between coal and power generation enterprises. The game model does not consider the impact of market coal price on coal used for thermal power generation, so its policy effect can only be short-lived.
From the perspective of energy consumption, the dominant factor influencing GHG emissions can be traced back to energy use (Crippa et al., 2019). At the same time, the consumption proportion of household consumption sector and transportation industry is also high (Ma et al., 2019). In general, thermal power plants are the major contributors to energy-related CO2 emissions (Muhammad, 2019). In some countries, continuous emissions monitoring systems (CEMS) are applied to measure emissions in thermal power plants, but they are costly and require continuous calibration (Cusworth et al., 2021a). As monitoring technologies continue to evolve, remote sensing and next-generation airborne visible/infrared imaging spectrometers (AVIRIS-NG) are being applied to quantify large amounts of fossil CO2 emissions (Nassar et al., 2017; Duren et al., 2019; Cusworth et al., 2021b). In reality, carbon capture and storage (CCS) is considered to be an advanced carbon emission reduction technology. Wang S. et al. (2016), Zhang X. et al. (2019), and Guo and Huang (2020) analyzed the carbon reduction investment strategies of power producers, but they did not focus on the impact of fuel price fluctuations on emission reduction investment strategies. In addition, in the analysis of government incentive policies, the issue of government incentives under the influence of fuel price risk has not been paid special attention (Zhou et al., 2014).
The existing literature is rich in exploring the trading strategies between coal companies and coal-fired power producers, as well as the investment decisions on green technologies for coal-fired power plants. However, the government’s penetration in the energy decarbonization process has been increasing since China’s carbon-peaking and carbon-neutral targets were set. In the past, the modular energy efficiency and emission reduction paths of coal, power, and polluters were not sufficient to support the achievement of the dual carbon targets, and the government’s need for a systematic solution for energy decarbonization transition has become more urgent. The factors influencing the implementation of carbon emission reduction in different types of enterprises have been diverse (Chen et al., 2018), but the prerequisite for the solution of this type of problem is to correctly deal with the conflict of interests between the various subjects involved in the process of decarbonization of the energy system. In fact, conflict occurs virtually everywhere in society and economics, and a powerful methodology called the graph model for conflict resolution (GMCR) (Kilgour et al., 1987; Fang et al., 1993) was put forward and then further associated extensions (Hipel et al., 2011; Xu et al., 2018) were designed to handle real-world conflict. The most obvious advantage of this approach is that it can fully consider the preference characteristics of different decision-makers in the conflict problem and can well reflect the conflict state evolution. In addition, unlike the classical game model, this approach also provides different definitions of stability allowing a more detailed analysis of the stable state of conflict.
Therefore, this study firstly analyzes the elements of conflict formation among government, coal enterprises, and coal-fired power plants in the process of low-carbon transition of energy system, and constructs a ternary conflict resolution graph model based on the theory of GMCR. Secondly, the conflict feasible states of government, coal enterprises, and coal-fired power plants are ranked with the help of preference statement method in GMCR theory. In addition, the stability characteristics of NASH, GMR, SMR, and SEQ for different feasible states and the evolution paths of key stable states are further analyzed. Finally, the results of the GMCR stability analysis are used to suggest targeted countermeasures for decarbonization of China's energy system.
China’s coal industry has, successively, gone through the stages of planned pricing, combination of regulation and release, and market-oriented reform. In general, from the liberalization of coal prices in 1993 to the cessation of coal price regulation by the state in 2005, coal prices have basically achieved market-oriented reforms (Zheng, 2017). A price formation mechanism dominated by the market and supplemented by the government’s macro-control is realized gradually (Wang et al., 2016; Wang, 2018; Zhang et al., 2019). However, coal prices fluctuate wildly due to numerous uncertain events. Table 1 lists China’s key policies to stabilize thermal coal prices over the years, among which the most critical and longest-running policy is coal-electricity price linkage (Bai, 2014; Li et al., 2015; Tan and Liu, 2015; Fan et al., 2018). However, Ye et al. (2018) pointed out that it has problems such as a long lag period, a high proportion of power generation companies digesting coal price fluctuations, and inadequate policy implementation. On the contrary, Zhang and Shi (2022) found that the abolition of coal-power linkage to implement electricity price marketization, although it can solve the structural contradiction of coal and electricity, will make the coal price increase bring a greater degree of industrial sector production costs and increased cost of living for residents. Objectively, the coal-power linkage policy is a transitional measure to deal with the violent fluctuations in the coal market, and its role as a coal price regulation policy itself means that coal prices are greatly influenced by the market.
Compared with the market-oriented reform process of coal, the marketization of China’s power structure is relatively low. As Table 2 shows, although market-based reforms in both the power generation and coal industries began in the 1980s, the government still holds dominant control over the power generation industry. Coal fuel accounts for 60%–70% of the cost of power generation, and the efficiency of coal consumption is determined as a priority by the level of technology and capacity scale of the generating units (Bai, 2014), so changes in coal prices affect the long-term trend of technological optimization, budget, production, and operation performance of downstream thermal power industry. In general, the degree of marketization of electricity prices lags far behind. The coal price market is the essential reason for the structural contradiction between coal and electricity (Zhang and Shi, 2022).
Global power systems are facing certain crises under the effects of decarbonization—all these negative phenomena have resulted in increased risk of violation of the power balance and insufficient supply. Geographically, thermal coal price growth remained positive in 2021, with growth rates of 4.9% and 3% in Europe and Asia, respectively (Savina et al., 2021). Power system is gradually developing into a hybrid energy system with multiple inputs and outputs (Arent et al., 2021), in which the coal-fired power generation chain has obvious deficiencies in energy supply, safe operation, and clean consumption. As presented in Figure 1A, China’s total CO2 emissions have broadly gone through three phases in the past 30 years, namely the slow growth phase (1990–1999), the rapid growth phase (2000–2013), and the oscillating and fluctuating phase (after 2014). At the same time, the share of CO2 emissions from coal to total emissions (SEC_TE) has remained above 30% for a long time. Therefore, the coal de-capacity strategy was adopted in the decarbonization transition of the energy system, as shown in Figure 1B, after years of growth, total raw coal production (TRCP) experienced a small decline in 2014, followed by a larger decline in 2015.
FIGURE 1. Time evolutionary characteristics of carbon emissions, power production and consumption, and coal production capacity in China. Source: 1) The data of total CO2 emissions and SEC_TE were obtained from CSMAR Carbon Neutrality Research Database; 2) The data of TRCP came from CHINA ENERGY STATISTICAL YEARBOOK 2020; 3) The vast majority of the data for SRCCP_CP and SP_EEC were obtained from CHINA ELECTRICITY STATISTICAL YEARBOOK 2021, except for the data from 2001 to 2004 obtained by linear interpolation.
Furthermore, Figure 1B also presents that the share of power in end-use energy consumption (SP_EEC) continues to grow, especially in 2019 for the first time above a quarter. A stable supply of electricity is vital to the security of the energy system, yet coal-fired power plants cannot operate without a stable supply of coal. From the perspective of industrial symbiosis, coal industry and electric power industry should maintain coordinated development. However, it is not the case, and in Figure 1B, the up-and-down oscillation of SRCCP_CP (share of raw coal consumed for coal-fired power generation in coal production) under the overall upward movement of SP_EEC in the last 15 years reflects, to some extent, the increased supply volatility of the coal-fired power generation chain.
In general, considering that carbon-peaking and carbon-neutral targets make the government face huge pressure to reduce carbon emissions, the government’s position in the coal power industry conflict is different from its previous role as a regulator of the price of electricity and coal. The Chinese government has to deal with the decarbonization of thermal power plants, however investment in carbon reduction technologies for power plants is affected by fluctuations in upstream coal prices. Therefore, the establishment of the government’s dual carbon goal makes it necessary to strengthen its penetration in the coal power industry conflict, and transform it into the third type of interest appealers and decision-makers, instead of only a price mediator, in the coal power industry conflict.
A ternary conflict has formed between the government, coal enterprises, and coal-fired power generation enterprises under the combined effect of the differences in the degree of marketization of the coal market and the electricity market, as well as carbon-peaking and carbon-neutral goals. As shown in Figure 2, the most straightforward option to achieve the goal of a low-carbon transformation of the energy system in the context of high-quality macroeconomic development in China is to curb the size of the coal-fired power generation industry and promote the development of green energy. However, the scale of green energy production cannot yet guarantee the safe and stable supply of the energy system after the contraction of the coal-fired power generation industry in the short term, and there are still many shortcomings in green energy itself. Even in the long run, Chai and Li (2022)use the evolutionary game model to analyze the evolutionary law between the governmental power subsidy policy and the energy structure state of power generation enterprises. It is found that the future energy structure is a combination of clean utilization of traditional energy and renewable energy in a certain proportion, rather than a hybrid transition mode of traditional energy and renewable energy. Therefore, the first element of the ternary conflict is the incongruity between the government’s energy system decarbonization reform and the secure and stable supply of the energy system.
For coal enterprises, they face the risks of capacity compression, environmental protection, and price volatility in the process of decarbonization of the energy system, and these risks are the second element in the creation of the triadic conflict.
—Production capacity pressure comes from coal mining activities, which have profound negative impacts on environment and human life (Hendryx, 2015; Grigoriou and Rothaermel, 2017), namely air pollution, climate change, resource depletion, etc. Mining of coal, not just its burning, causes occupational diseases and public health problems as well (Graber et al., 2014; Hall et al., 2019). Most of these health problems are related to inhalation of mining-related dust and chemicals (Munawer, 2018; Hendryx et al., 2020). Avoiding the above issues is possible through a package of restrictions on coal capacity in China. For example, disposal of “zombie coal enterprises” by overall withdrawal, closure and clearance, restructuring and integration, and other strategies to reduce capacity and the compression of new production capacity. Moreover, the rapid expansion of hydropower, nuclear power, solar energy, and other green energy sources at the macro level, will squeeze coal power production capacity under double carbon constraints (He et al., 2020).
—Environmental protection pressure is mainly reflected in strict environmental protection, energy consumption, and water consumption standards. In detail, coal-related environmental challenges consist of the handling of the escape of coalbed methane (CBM), soil erosion, coal gangue, mine drainage, and land subsidence (Wang et al., 2020). For coal enterprises, improving their environmental governance capabilities is a prerequisite for obtaining long-term development opportunities (Husted and de Sousa-Filho, 2017; Lemly, 2019). In detail, the environmental pressure of coal enterprises includes costs of coal consumption, exploration costs of coal resources, environmental prevention costs, environmental governance costs, environmental impact costs, costs of ecological environment damage, and environmental management and education costs (Guo et al., 2019).
—In the context of the current complex situation of international energy supply and demand, coal prices under the guidance of a market-oriented pricing mechanism often fluctuate irrationally and violently. For a long time, the Chinese government has continuously improved the coal price formation mechanism. In the period 2016– 2021, the price of coal for power generation in China has successively implemented the coal-power price linkage mechanism and the “basic price + floating up and down” dual-track system (Li, 2019). Since May 2022, based on adhering to the coal price formed by the market, clarify the reasonable range of price, strengthen the regulation of range, and guide the coal price to operate in a reasonable range (NDRC, 2022). In essence, the trade-offs and adjustments between the market and planned price formation mechanisms are the basic means of stabilizing coal prices, yet the price regulation is usually not as effective as it should be.
The third element of the ternary conflict is the operational dilemma faced by coal-fired power producers. Specifically, these include declining profits, fluctuating fuel prices, and higher energy consumption and emission standards.
— Upstream fuel price fluctuation risk: with the increase in the level of per capita electricity consumption, the amount of coal consumed in power generation is also increasing as presented in Figure 1. Coal-fired power generation does not have a substitution relationship between labor and capital in the short term, and the complementarity between coal and combustion-supporting materials is not strong (Zhang et al., 2011). Therefore, in the short term, if the price of coal rises, enterprises cannot reduce the input demand for coal by increasing labor and auxiliary materials but only by adjusting production to reduce losses, which will lead to conflict with the government’s goal of securing a stable supply of electricity.
— Supply guarantee pressure of downstream power demand: as depicted in Figure 1, in terms of electricity demand and supply, the share of electricity in China’s end-use energy consumption has tripled in the last 30 years. Among them, the proportion of coal-fired power generation in the total installed capacity has remained above 50% for a long time (Wang et al., 2018). Therefore, coal-fired power plants bear the important responsibility of a stable power supply.
— Environmental regulatory pressure: under the goals of carbon peak and carbon neutrality, the environmental protection pressure of coal-fired power plants has increased sharply, and the continuous improvement of energy consumption and emission standards has led to an increase in the operating costs of coal power companies’ generator sets.
Graph model for conflict resolution (GMCR) is a conflict modeling and analysis tool with weaker data requirements compared with classical game theory (Xu et al., 2018; Kong et al., 2019). It can analyze the strategy and preference characteristics of decision-makers based on conflict information and then explore the equilibrium characteristics of conflict feasible states with the help of different stability definitions, which provides strong support for solving the ternary conflict problem of coal-fired power generation industry chain. In detail, GMCR of the ternary conflict can be expressed as
In the market-oriented coal industry, production goals of coal enterprises are to pursue the maximization of their own interests, and their strategies can be abstracted into
Coal-fired power generation bears a high peak load demand and is strategically important for national energy security. The business goal of coal power enterprises is to ensure the supply of electricity and maximize their own benefits under the conditions of meeting their normal operation. Faced with the tight supply and soaring prices of upstream coal, coal-fired power grid prices are in a long-term loss situation. When the losses accumulate to a certain level, coal-fired power plants will take the following measures under pressure to survive: gradually stop the operation of generating units, cut the power supply, and reduce the loss. Therefore, the strategy of coal-fired power companies can be abstracted as
In the context of the double carbon constraint, government departments need to consider both the carbon emission reduction target, safe and stable supply of electricity and the set of their strategies are recorded as
Decision-makers choose one or more strategies in their strategy set (
Conflict state transfer is the process by which decision-makers move from a current conflict situation to another by changing their own strategies. The feasible states of decision-makers and their transfer characteristics in GMCR can be represented by a directed graph. In detail, Figures 3A–C show the state transfer of
Conflict state preference is the prioritization of the decision maker’s feasible states, which is often determined in practice using the method of strategy preference statement (Xu et al., 2018). Based on the characteristics of decision-makers’ conflict mechanism, conflict environment, decision-making goals, and other characteristics in the ternary conflict of coal-fired power industry chain, the order of decision-makers’ strategy preferences are declared, and the conflict feasible state ranking of different decision-makers is obtained. The strategy preference statements of
The preference scores of all feasible states of coal companies, coal-fired power plants, and governments are calculated with the help of the conflict state ranking method based on strategy preference statements (Hou and Xu, 2016), and the preference sequences of feasible states are determined accordingly. Specifically, the conflict state preference of coal enterprises is: PC={s14 ≻ s13 ≻ s17 ≻ s2 ≻ s1 ≻ s5 ≻ s8 ≻ s7 ≻ s11 ≻ s10 ≻ s9 ≻ s12 ≻ s4 ≻ s3 ≻ s6 ≻ s16 ≻ s15 ≻ s18}; The conflict state preference of coal-fired power plants is PP = { s10 ≻ s4 ≻ s16 ≻ s8 ≻ s2 ≻ s14 ≻ s12 ≻ s9 ≻ s6 ≻ s3 ≻ s18 ≻ s15 ≻ s11 ≻ s7 ≻ s5 ≻ s1 ≻ s17 ≻ s13}. The conflict states preference of government is PG = {s10 ≻ s9 ≻ s8 ≻ s7 ≻ s4 ≻ s3 ≻ s2 ≻ s1 ≻ s16 ≻ s15 ≻ s14 ≻ s13 ≻ s12 ≻ s11 ≻ s6 ≻ s5 ≻ s18 ≻ s17}.
The rational economic man hypothesis of decision-makers indicates that their decision-making goal is to pursue the maximization of their own interests, but the conflict state cannot usually be decided by any one decision-maker. Each decision-maker must consider the conflict overall situation and predict what all decision-makers may accept as the solution to the conflict and stability. Conflict equilibrium state analysis based on GMCR theory usually relies on four kinds of stability:
As presented in Table 5, presents the stability characteristics of different conflict states, where
In Figure 4, the evolution paths of conflict equilibrium states
Conflict equilibrium state
The future energy structure system tends to reduce carbon emissions under the dual carbon target constraints, and the ternary conflict mediation strategy of “coal-power-government” should fully grasp the rhythm of energy change. Objectively, the combination of strategies corresponding to the above conflicting equilibria
For the
FIGURE 5. Power generation efficiency and coal consumption of China’s power plants. Source: The data were obtained from CHINA ELECTRICITY STATISTICAL YEAR BOOK 2021.
Grasping the main contradictions in the current energy transition process according to specific national conditions and proposing a practical implementation path for low-carbon energy transition is the key to achieving the development of energy transition. Against the backdrop of the tight time frame for carbon-peaking and carbon-neutral targets, the Chinese government has outlined an ambitious blueprint for promoting climate and environmental governance and sustainable development with a series of important emission reduction strategies, demonstrating China’s strategic determination to adhere to green and low-carbon development and actively address climate change. Overall, the ternary conflict among coal enterprises, coal-fired power plants, and the government is essentially an outward manifestation of the multiple pressure shifts faced by these three parties in the process of decarbonizing the energy system in the context of China’s macro strategy for high-quality development.
Coal enterprises, coal-fired power plants, and the government are placed under a systematic analysis framework from the perspective of coal-fired power industry chain conflict to explore the formation mechanism of the above ternary conflict in the process of decarbonization transformation of energy system. Further, a conflict analysis model of the coal-fired power industry chain is constructed based on the theory of GMCR to analyze the possible coping strategies and conflict states of different decision-makers. The feasible conflict states are ranked based on the state preference statement method, and then the NASH, GMR, SMR, and SEQ equilibrium characteristics of conflict states for all the decision-makers are explored. It is found that among all feasible conflict states,
Finally, this paper provides some useful insights for future research on the transformation and upgrading of energy systems under the background of carbon-peaking and carbon-neutral targets. Future research could draw more on big data and its management and application tools to assist in determining the strategic preference characteristics of coal enterprises, coal-fired power plants, and governments, especially in identifying the pace of energy reform and conflict characteristics in different regions. In particular, it is critical to use technological innovation to promote the low-carbon transformation of the energy system to achieve the carbon reduction goal (Cai et al., 2021). For example, the energy system needs to transform from high-carbon to deep low-carbon or zero-carbon, from mechanical electromagnetic systems to power electronic devices, and from deterministic controllable continuous power sources. The decarbonization process of the energy system is radical but realistic in some respects, and further development and diffusion of green technologies facilitate more efficient decarbonization. Overall, the systematic design of optimal energy system low-carbon transition pathways is a complex process of continuous refinement.
The original contributions presented in the study are included in the article/Supplementary Material, further inquiries can be directed to the corresponding author.
YF conceived the project and wrote the manuscript; HX designed the GMCR model and edited the manuscript.
This work is supported by Social Science Foundation of Jiangsu Province (Grant No. 21GLC011), Project of Philosophy and Social Science Research in Colleges and Universities of Jiangsu Province (Grant No. 2021SJA0122).
The authors declare that the research was conducted in the absence of any commercial or financial relationships that could be construed as a potential conflict of interest.
All claims expressed in this article are solely those of the authors and do not necessarily represent those of their affiliated organizations, or those of the publisher, the editors, and the reviewers. Any product that may be evaluated in this article, or claim that may be made by its manufacturer, is not guaranteed or endorsed by the publisher.
The team of authors expresses their gratitude to the reviewers for valuable recommendations that have been considered to improve significantly the quality of this article.
The Supplementary Material for this article can be found online at: https://www.frontiersin.org/articles/10.3389/fenvs.2022.930322/full#supplementary-material
Arent, D. J., Bragg-Sitton, S. M., Miller, D. C., Tarka, T. J., Engel-Cox, J. A., Boardman, R. D., et al. (2021). Multi-input, Multi-Output Hybrid Energy Systems. Joule 5 (1), 47–58. doi:10.1016/j.joule.2020.11.004
Bai, R. (2014). Prices for Coal Used in Power Generation, Industrial Policies and Upgrading of Technological Structure of Coal-Fired Power Industry. J. Finance Econ. 40 (12), 76
Bompard, E., Botterud, A., Corgnati, S., Huang, T., Jafari, M., Leone, P., et al. (2020). An Electricity Triangle for Energy Transition: Application to Italy. Appl. Energy 277, 115525. doi:10.1016/j.apenergy.2020.115525
Cai, A., Zheng, S., Cai, L., Yang, H., and Comite, U. (2021). How Does Green Technology Innovation Affect Carbon Emissions? A Spatial Econometric Analysis of China's Provincial Panel Data. Front. Environ. Sci. 9, 813811. doi:10.3389/fenvs.2021.813811
Castán Broto, V., Baptista, I., Kirshner, J., Smith, S., and Neves Alves, S. (2018). Energy Justice and Sustainability Transitions in Mozambique. Appl. Energy 228, 645–655. doi:10.1016/j.apenergy.2018.06.057
Chai, R., and Li, G. (2022). Renewable Clean Energy and Clean Utilization of Traditional Energy: An Evolutionary Game Model of Energy Structure Transformation of Power Enterprises. Syst. Eng. 42 (1), 184
Ćorović, N., Urošević, B. G., and Katić, N. (2022). Decarbonization: Challenges for the Electricity Market Development—Serbian Market Case. Energy Rep. 8, 2200
Crippa, M., Oreggioni, G., Guizzardi, D., Muntean, M., Schaaf, E., Lo Vullo, E., et al. (2019). Fossil CO2 and GHG Emissions of All World Countries. Luxemburg: Publication Office of the European Union.
Cusworth, D. H., Duren, R. M., Thorpe, A. K., Eastwood, M. L., Green, R. O., Dennison, P. E., et al. (2021a). Quantifying Global Power Plant Carbon Dioxide Emissions with Imaging Spectroscopy. AGU Adv. 2 (2), e2020AV000350. doi:10.1029/2020av000350
Cusworth, D. H., Duren, R. M., Thorpe, A. K., Pandey, S., Maasakkers, J. D., Aben, I., et al. (2021b). Multisatellite Imaging of a Gas Well Blowout Enables Quantification of Total Methane Emissions. Geophys. Res. Lett. 48 (2), e2020GL090864. doi:10.1029/2020gl090864
Duren, R. M., Thorpe, A. K., Foster, K. T., Rafiq, T., Hopkins, F. M., Yadav, V., et al. (2019). California's Methane Super-emitters. Nature 575 (7781), 180–184. doi:10.1038/s41586-019-1720-3
Fan, J.-L., Ke, R.-Y., Yu, S., and Wei, Y.-M. (2018). Conservation, and RecyclingHow Does Coal-Electricity Price Linkage Impact on the Profit of Enterprises in China? Evidence from a Stackelberg Game Model. Resour. Conservation Recycl. 129, 383–391. doi:10.1016/j.resconrec.2016.09.016
Fang, L., Hipel, K. W., and Kilgour, D. M. (1993). Interactive Decision Making: The Graph Model for Conflict Resolution. John Wiley & Sons.
Gozgor, G., Lau, C. K. M., and Lu, Z. (2018). Energy Consumption and Economic Growth: New Evidence from the OECD Countries. Energy 153, 27–34. doi:10.1016/j.energy.2018.03.158
Graber, J. M., Stayner, L. T., Cohen, R. A., Conroy, L. M., and Attfield, M. D. (2014). Respiratory Disease Mortality Among US Coal Miners; Results after 37 Years of Follow-Up. Occup. Environ. Med. 71 (1), 30–39. doi:10.1136/oemed-2013-101597
Grigoriou, K., and Rothaermel, F. T. (2017). Organizing for Knowledge Generation: Internal Knowledge Networks and the Contingent Effect of External Knowledge Sourcing. Strat. Mgmt. J. 38 (2), 395–414. doi:10.1002/smj.2489
Guo, J.-X., and Huang, C. (2020). Feasible Roadmap for CCS Retrofit of Coal-Based Power Plants to Reduce Chinese Carbon Emissions by 2050. Appl. Energy 259, 114112. doi:10.1016/j.apenergy.2019.114112
Guo, Y., Zeng, L.-X., He, P., and Shi, J.-P. (2019). Problems and Countermeasures in Environmental Cost Accounting: A Case Study of China's Coal Industry. E3S Web Conf. 83, 01013. doi:10.1051/e3sconf/20198300001
Hall, N. B., Blackley, D. J., Halldin, C. N., and Laney, A. S. (2019). Continued Increase in Prevalence of R-type Opacities Among Underground Coal Miners in the USA. Occup. Environ. Med. 76 (7), 479–481. doi:10.1136/oemed-2019-105691
He, J., Wang, H., Tian, Z., Li, Z., Yang, X., and Zhou, L. (2020). Comprehensive Report on China's Long-Term Low-Carbon Development Strategy and Transformation Path. China Popul. Resour. Environ. 30 (11), 1
Hendryx, M. (2015). The Public Health Impacts of Surface Coal Mining. Extr. Industries Soc. 2 (4), 820–826. doi:10.1016/j.exis.2015.08.006
Hendryx, M., Zullig, K. J., and Luo, J. (2020). Impacts of Coal Use on Health. Annu. Rev. Public Health 41, 397–415. doi:10.1146/annurev-publhealth-040119-094104
Hipel, K. W., Kilgour, D. M., and Fang, L. (2011). “The Graph Model for Conflict Resolution,”in Wiley Encyclopedia of Operations Research and Management Science. Editors J. J. Cochran, L. A. Cox, P. Keskinocak, J. P. Kharoufeh, and J. C. Smith (New York: Wiley), Vol. 3 of 8, 2009–2111. doi:10.1002/9780470400531.eorms0882
Hou, Y., and Xu, H. (2016). Research on Option Prioritization for Strength of Preference Based on the Graph Model for Conflict Resolution. Chin. J. Manag. Sci. 24 (09), 64
Howe, P. D. (2021). Extreme Weather Experience and Climate Change Opinion. Curr. Opin. Behav. Sci. 42, 127–131. doi:10.1016/j.cobeha.2021.05.005
Husted, B. W., and Sousa-Filho, J. M. d. (2017). The Impact of Sustainability Governance, Country Stakeholder Orientation, and Country Risk on Environmental, Social, and Governance Performance. J. Clean. Prod. 155, 93–102. doi:10.1016/j.jclepro.2016.10.025
Jiang, P., Fan, Y. V., and Klemeš, J. J. (2021). Impacts of COVID-19 on Energy Demand and Consumption: Challenges, Lessons and Emerging Opportunities. Appl. Energy 285, 116441. doi:10.1016/j.apenergy.2021.116441
Jiang, Y., Cai, W., Wan, L., and Wang, C. (2015). An Index Decomposition Analysis of China's Interregional Embodied Carbon Flows. J. Clean. Prod. 88, 289–296. doi:10.1016/j.jclepro.2014.04.075
Kang, C. a., and Yang, T. (2012). Analysis of Coal and Electricity Price Linkage in a Bargaining Model. China coal. 38 (02), 35
Kilgour, D. M., Hipel, K. W., and Fang, L. (1987). The Graph Model for Conflicts. Automatica 23 (01), 41–55. doi:10.1016/0005-1098(87)90117-8
Kong, Y., Xu, H., and Fang, Y. (2019). Research on Conflict Stability of Decision-Makers’ Power Asymmetry Based on Matrix Representation of Solution Concepts. Control Decis. 34 (02), 298
Lejano, R. P., Kan, W. S., and Chau, C. C. (2020). The Hidden Disequities of Carbon Trading: Carbon Emissions, Air Toxics, and Environmental Justice. Front. Environ. Sci. 8, 593014. doi:10.3389/fenvs.2020.593014
Lemly, A. D. (2019). Environmental Hazard Assessment of Benga Mining's Proposed Grassy Mountain Coal Project. Environ. Sci. Policy 96, 105–113. doi:10.1016/j.envsci.2019.03.010
Li, H.-Z., Tian, X.-L., and Zou, T. (2015). Impact Analysis of Coal-Electricity Pricing Linkage Scheme in China Based on Stochastic Frontier Cost Function. Appl. Energy 151, 296–305. doi:10.1016/j.apenergy.2015.04.073
Li, K. (2019). Power Price Reform Is Imminent! the Linkage of Coal and Electricity Prices Will Be Cancelled from Next Year, and the Market-Oriented Mechanism of "base Price + Floating up and Down will be launched. Available: http://www.nbd.com.cn/articles/2019-09-26/1374889.html.
Li, Y., Lan, S., Ryberg, M., Pérez-Ramírez, J., and Wang, X. (2021). A Quantitative Roadmap for China towards Carbon Neutrality in 2060 Using Methanol and Ammonia as Energy Carriers. Iscience 24 (6), 102513. doi:10.1016/j.isci.2021.102513
Liu, P., and Tan, Z. (2017). How to Achieve Stable Match and Scale Linkage for Transaction between Power and Coal. Chin. J. Manag. Sci. 25 (01), 106
Luo, S., Hu, W., Liu, W., Xu, X., Huang, Q., Chen, Z., et al. (2021). Transition Pathways towards a Deep Decarbonization Energy System-A Case Study in Sichuan, China. Appl. Energy 302, 117507. doi:10.1016/j.apenergy.2021.117507
Ma, X., Wang, C., Dong, B., Gu, G., Chen, R., Li, Y., et al. (2019). Carbon Emissions from Energy Consumption in China: Its Measurement and Driving Factors. Sci. Total Environ. 648, 1411–1420. doi:10.1016/j.scitotenv.2018.08.183
Muhammad, B. (2019). Energy Consumption, CO2 Emissions and Economic Growth in Developed, Emerging and Middle East and North Africa Countries. Energy 179, 232–245. doi:10.1016/j.energy.2019.03.126
Munawer, M. E. (2018). Human Health and Environmental Impacts of Coal Combustion and Post-combustion Wastes. J. Sustain. Min. 17 (02), 87–96. doi:10.1016/j.jsm.2017.12.007
Nassar, R., Hill, T. G., McLinden, C. A., Wunch, D., Jones, D. B. A., and Crisp, D. (2017). Quantifying CO2 Emissions from Individual Power Plants from Space. Geophys. Res. Lett. 44 (19), 10045–10053. doi:10.1002/2017gl074702
NDRC (2022). Notice of the National Development and Reform Commission on further improving the coal market price formation mechanism [Online]. Available: https://www.ndrc.gov.cn/xwdt/tzgg/202202/t20220225_1317006.html?code=&state=123.
Savina, N., Sribna, Y., Pitel, N., Parkhomenko, L., Osipova, A., and Koval, V. (2021). “Energy Management Decarbonization Policy and its Implications for National Economies,” in IOP Conference Series: Earth and Environmental Science (IOP Publishing).
Sun, H., Edziah, B. K., Sun, C., and Kporsu, A. K. (2019). Institutional Quality, Green Innovation and Energy Efficiency. Energy policy 135, 111002. doi:10.1016/j.enpol.2019.111002
Sun, H., Mohsin, M., Alharthi, M., and Abbas, Q. (2020). Measuring Environmental Sustainability Performance of South Asia. J. Clean. Prod. 251, 119519. doi:10.1016/j.jclepro.2019.119519
Tan, X., Dong, L., Chen, D., Gu, B., and Zeng, Y. (2016). China's Regional CO2 Emissions Reduction Potential: A Study of Chongqing City. Appl. Energy 162, 1345–1354. doi:10.1016/j.apenergy.2015.06.071
Tan, Z., and Liu, P. (2015). Transaction Cost, Governance Cost and Boundary Decision: The Game between Coal Enterprises and Thermal Power Enterprises in Institutional Changes. East China Econ. Manag. 29 (01), 73
Vatalis, K. I., Avlogiaris, G., and Tsalis, T. Α. (2022). Just Transition Pathways of Energy Decarbonization under the Global Environmental Changes. J. Environ. Manag. 309, 114713. doi:10.1016/j.jenvman.2022.114713
Verma, P., Dumka, A., Bhardwaj, A., Ashok, A., Kestwal, M. C., and Kumar, P. (2021). A Statistical Analysis of Impact of COVID19 on the Global Economy and Stock Index Returns. Sn Comput. Sci. 2 (1), 27. doi:10.1007/s42979-020-00410-w
Wang, D., Nie, R., and Liu, Y. (2016a). Scenario Simulation on Chinese Coal-Electricity Price Co-movement Effects Based on Complex Network Model. Syst. Eng. 34 (08), 75
Wang, J., Wang, R., Zhu, Y., and Li, J. (2018). Life Cycle Assessment and Environmental Cost Accounting of Coal-Fired Power Generation in China. Energy Policy 115, 374–384. doi:10.1016/j.enpol.2018.01.040
Wang, S., Yang, S., and Peng, Z. (2016b). Research on the Power Producer's Carbon Abatement Investment in View of Multiple Uncertainties. J. Manag. Sci. China 19 (02), 31
Wang, Y. (2018). Energy Relationship and Price Status under Supply Side Reform--Taking Coal-Electricity Industry Chain as an Example. Mod. Econ. Res. (07), 26
Wang, Y., Lei, Y., and Wang, S. (2020). Green Mining Efficiency and Improvement Countermeasures for China's Coal Mining Industry. Front. Energy Res. 8, 18. doi:10.3389/fenrg.2020.00018
Xu, H., Hipel, K. W., Kilgour, D. M., and Fang, L. (2018). Conflict Resolution Using the Graph Model: Strategic Interactions in Competition and Cooperation. Springer.
Ye, Z., He, J., and Wang, Y. (2018). Research on the Problems and Countermeasures of Coal and Electricity Price Linkage Policy in China. China Price (01), 71
Zhang, H., and Shi, M. (2022). The Effects of Electricity Price Marketization Mechanism on Cost-An Analysis Based on Input-Output Price Model with Price Heterogeneity. Manag. Rev. 34 (01), 17
Zhang, M., Zhao, G., and Jiao, B. (2011). Research on Policy System of Coal Resource Price Formation Mechanism. Beijing: Metallurgical Industry Press.
Zhang, R., Lu, C.-C., Lee, J.-H., Feng, Y., and Chiu, Y.-H. (2019a). Dynamic Environmental Efficiency Assessment of Industrial Water Pollution. Sustainability 11 (11), 3053. doi:10.3390/su11113053
Zhang, X., Gan, D., Huang, S., and Ye, Z. (2019b). Investment Strategy of Carbon Emission Reduction of Coal-Fired Power Firms Considering Revenue Floors. J. Manag. Sci. China 22 (11), 69.
Zhang, Y.-J., Hao, J.-F., and Song, J. (2016). The CO2 Emission Efficiency, Reduction Potential and Spatial Clustering in China's Industry: Evidence from the Regional Level. Appl. Energy 174, 213–223. doi:10.1016/j.apenergy.2016.04.109
Zheng, X. (2017). Promoting the Full-Scale Liberation of Energy Price. Price Theory. Pract. (12), 17
Keywords: carbon peak, carbon neutrality, decarbonization pathway, coal-fired power industry, conflict mediation
Citation: Fang Y and Xu H (2022) Research on Decarbonization Pathway of China’s Coal-Fired Power Industry From the Perspective of Conflict Mediation. Front. Environ. Sci. 10:930322. doi: 10.3389/fenvs.2022.930322
Received: 27 April 2022; Accepted: 13 June 2022;
Published: 14 July 2022.
Edited by:
Huaping Sun, Jiangsu University, ChinaReviewed by:
Patrick Thabang Sekoai, The University of Hong Kong, Hong Kong, SAR ChinaCopyright © 2022 Fang and Xu. This is an open-access article distributed under the terms of the Creative Commons Attribution License (CC BY). The use, distribution or reproduction in other forums is permitted, provided the original author(s) and the copyright owner(s) are credited and that the original publication in this journal is cited, in accordance with accepted academic practice. No use, distribution or reproduction is permitted which does not comply with these terms.
*Correspondence: Yinhai Fang, eWluaGFpZmFuZ0BhbGl5dW4uY29t
Disclaimer: All claims expressed in this article are solely those of the authors and do not necessarily represent those of their affiliated organizations, or those of the publisher, the editors and the reviewers. Any product that may be evaluated in this article or claim that may be made by its manufacturer is not guaranteed or endorsed by the publisher.
Research integrity at Frontiers
Learn more about the work of our research integrity team to safeguard the quality of each article we publish.