- Aquatic Systems Biology Unit, TUM School of Life Sciences, Technical University of Munich, Munich, Germany
Hydropower use of rivers can exert multiple effects on aquatic species and habitats. Due to limitations of conservation projects in the main channels of hydropower-affected rivers, there has been an increasing focus on tributaries, side channels, and fish passes as target areas for conservation and restoration. However, some of these side channels require frequent dewatering for their maintenance, and the ecological effects of such measures remain largely unknown. In this study, we used two dewatering events in a side channel of the River Inn as an opportunity to assess the effects of these common measures on fish. All stranded fish were collected after the two dewatering events in remaining puddles of formerly restored bank habitats, determined to species level, and measured. The fish community was compared by electrofishing before and seven weeks after the dewatering in a subset of the same habitats. The dewatering created one to three remaining puddles in the bank habitats, covering 3% of the assessed bank habitat area. In these remaining puddles, 184 stranded fish from 12 species were found, including species strictly protected under national and international law. In relation to their relative abundance, smaller and less mobile species such as Cottus gobio were mostly affected by stranding in contrast to larger and open-water-oriented species such as Chondrostoma nasus. The dewatering also caused drying out of important nursery zones, resulting in a distinctly lower recruitment success of endangered riverine fish species in the summer following the dewatering. The evidence about the negative ecological effects documented herein should be transferred into policy measures to reduce the impairment of dewatering to a minimum and to contribute to the fulfilment to national and international legal requirements. This can be achieved by reducing the extent and frequency of periodical dewatering to a minimum, by slowing down the dewatering speed, by selecting the least critical time of the year, as well as by compensation measures.
Introduction
Hydropower is one of the most controversial forms of renewable energy with some authors questioning its environmental compatibility when balancing its “red” and “green” aspects (e.g., Seliger et al., 2016; Geist, 2021). Hydropower can affect aquatic ecosystems and aquatic species in many ways, including impaired habitat quality by the implementation and operation of the power plant, water diversions and abstractions needed for energy production, and direct injuries or mortality of aquatic animals, particularly fish (Anderson et al., 2006; Zarfl et al., 2015; Reid et al., 2019; Algera et al., 2020). Therefore, hydropower often conflicts with the international and national legislation such as the European Water Framework Directive (European Parliament, 2000) and the Habitats Directive (European Parliament, 1992), targeting the protection of highly endangered species and their habitats.
Great challenges arise when it comes to restoring habitat quality in rivers affected by hydropower production, due to the many restrictions and constraints for such action (Pander and Geist, 2018). In free-flowing rivers unaffected by hydropower exploitation, naturally fluctuating discharge, strongly changing water levels, and flow velocities permanently create areas of new habitat. Such succession is an important part of natural river functioning with evolutionary adaptions of all riverine fish to these patterns (Postel and Richter, 2012). These discharge regimes and the associated dynamic formation of diverse habitat conditions are key for the completion of the life cycles of specialized riverine fish. Unfortunately, such dynamic conditions in natural rivers are contrasting the need for a ground-based hydropower exploitation, and many natural rivers were therefore transformed into heavily modified water bodies to control their hydromorphological dynamics. In such systems, alteration of flow and sediment regimes, straightened and rip-rap protected riverbanks, as well as the disconnection with the floodplain have led to a severe loss of critical habitats for spawning and juvenile development of riverine fish species as well as other ecological and socioeconomic impacts (Bunn and Arthington, 2002; Tockner and Stanford, 2002; Aarts et al., 2004; Auerswald et al., 2019). In this context, tributaries, side channels, and fish passes got more and more into the focus of conservation practitioners to restore aquatic habitats beyond the main channel (Pander et al., 2017), with the core intention to promote population development in declining aquatic species (Moyle and Leidy, 1992; Mueller et al., 2020). However, restoration in these waters is also often more restricted than in the main channel, often being limited to small-scale instream restoration measures (Geist and Hawkins, 2016). These measures can comprise the creation of shallow bank habitats (Lorenz et al., 2013; Pander et al., 2017; Pander et al., 2021), the introduction of gravel and boulders (Barlaup et al., 2008; Pander et al., 2015), or the placement of large woody debris (Gurnell et al., 2005; Entrekin et al., 2008). It is known that these restoration measures can create valuable habitats for many aquatic species and harbor large fish diversity, often comprising relict populations of highly protected fish and macroinvertebrate species (Pander and Geist 2016; Pander et al., 2017; Meulenbroek et al., 2018; Pander et al., 2021; Nagel et al., 2022). However, tributaries, side channels, and fish passes are often also limited by hydropower-induced morphological constraints such as damming, bank protection, or maintenance-induced management (Pander et al., 2022, in press). For example, it is a common practice to completely or partly dewater side channels for maintenance reasons to overhaul turbines or to clean the channels from dumped rubbish, typically every year or every few years. This practice of reducing the water level can result in drying out of bank habitats. Since species communities in bank habitats can be particularly prone to swift water level fluctuations (Jepsen, 1997; Pander et al., 2021), it is most likely that aquatic organisms in these habitats are heavily affected by this management practice. This holds even more true when bank habitats have been previously restored with the intention to support fish population development. Despite the high ecological value of restored river banks, especially as nursery habitats for rheophilic fish species, less is known about the direct and indirect effects of regular maintenance such as the dewatering scenarios in practice. This concerns both the direct mortality of fish due to stranding and the indirect effects arising from the disturbance due to the limited accessibility and availability of shallow bank zones that are crucial habitats for the early life history of riverine fish species. In addition, dewatering of channels may also affect other aquatic organisms such as molluscs (Curley et al., 2022), crayfish, or other invertebrates (Muehlbauer et al., 2011). To date, dewatering events and fast changing water levels are only assessed regarding hydropeaking—an operational tool of hydropower production to feed peaks of energy demand and maximize energy production revenue (Venus et al., 2020). Hydropeaking can cause sudden changes in flow and is known to result in the degradation of river habitat quality (Bruder et al., 2016; Greimel et al., 2018), the alteration of stream thermal regimes (Casas-Mulet et al., 2016; Choi and Choi, 2018), the dewatering of spawning grounds (Grabowski and Isely, 2007; Casas-Mulet et al., 2015), and the stranding of fish (Puffer et al., 2015; Schmutz et al., 2015; Auer et al., 2017; Bartoň et al., 2021). Since hydropeaking-induced water level fluctuations strongly differ from dewatering during the maintenance in magnitude and frequency, such results are of limited transferability to channel dewatering for maintenance reasons that typically last several days or even months and can include much greater water level amplitudes (Auer et al., 2017).
This study aimed at understanding how the dewatering of channels as a measure of hydropower-related maintenance affects fish and their habitats, with a focus on restored shallow bank habitats. We hypothesized that 1) the common practice of quick dewatering of shallow bank habitats results in fish stranding and mortality, with smaller and less mobile species being the most strongly affected, and that 2) dewatering can subsequently affect fish community composition, particularly during the period of spawning, larval drift, and juvenile development of sensitive riverine fish species.
Material and Methods
Study Design and Site Description
To investigate the ecological effects of the common practice to dewater a side channel for maintenance reasons, two such events in the River Hammerbach (HB, Figure 1) were taken as an opportunity to assess direct and indirect effects of the dewatering on individual fish and the fish community. This also provided the opportunity of rescuing stranding specimens during the course of the investigation. During the two regular practiced dewatering events in 2016 and 2021, direct effects of this maintenance measure on fish were assessed by collecting, determining, and measuring all stranded individuals. To address the potential indirect effects on the fish community, bank habitats were assessed seasonally three times a year (in spring, summer, and autumn) by electrofishing in 2017 and seasonal prior and after the dewatering event in 2021. In this second part, the approach herein follows a BACI design (Smith et al., 1993), where habitat conditions before and after an anthropogenic disturbance (impact = dewatering) were assessed (Figure 2). The spring season in 2021 is before, and summer and autumn 2021 are after the dewatering. 2017 is thereby considered as an additional control condition in the BACI design being not affected by dewatering. In 2016, dewatering was applied by an abrupt shutdown of the discharge that was responsible for a very rapid drop in the water level in the HB. In 2021, the discharge in the HB was successively reduced with a dropping rate of 11 cm h−1.
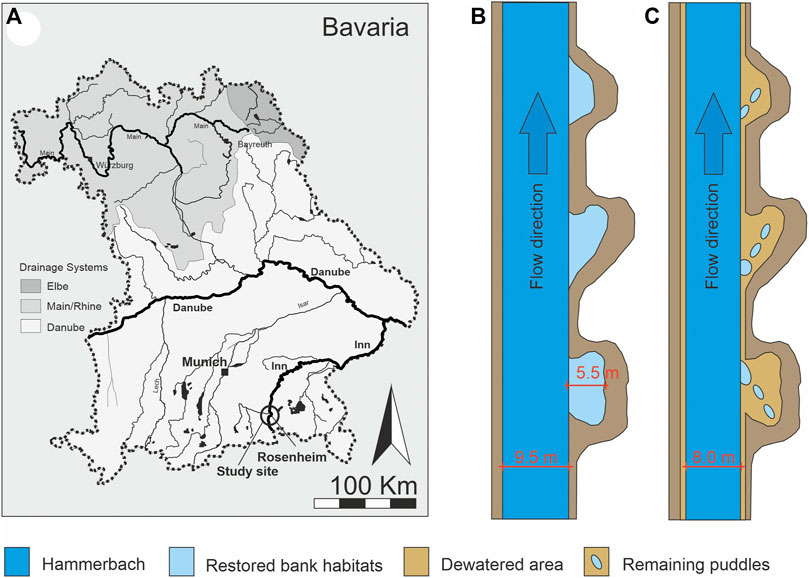
FIGURE 1. Location of the study site within Bavaria, Germany (A). Schematic top view of a principle Hammerbach stretch under the mean flow conditions (MQ) with flooded bank habitats (B) and under dewatering conditions (DQ) with partly dry habitats (C). Remaining puddles under dewatering conditions are indicated in panel (C) in light blue. River width of the Hammerbach and width of the bank habitats are given as the mean. Water levels of MQ and DQ are indicated in the side view of Figure 3.
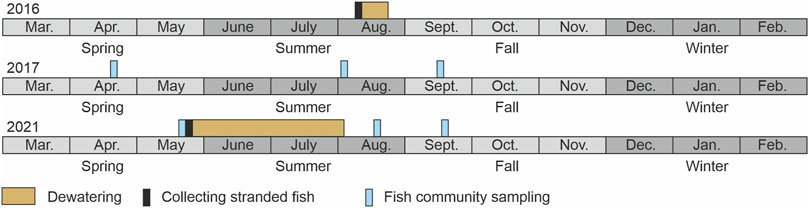
FIGURE 2. Timescale of sampling and dewatering events of 2016, 2017, and 2021 separated by month and season; abbreviation of month names corresponds to the common form. Note that the fish community sampling in 2021 contains sampling in the restored bank habitats as well as in the main channel of the Hammerbach.
The HB is a natural stream ecosystem that was heavily modified in the past. Most of its discharge generates out of the continuation of the River Mangfall channel within the upper Inn catchment in the city of Rosenheim in Bavaria, Germany (47°57′43.00″N, 12°09′22.13″E, Figure 1). The HB runs parallel to the River Inn for 15.3 km until its confluence with the River Rott, and 2.0 km further downstream with the River Inn. It is a straight channel with a trapezoidal profile and a uniform water depth during its course throughout the study area. Several hydropower plants located in the city of Rosenheim use the HB’s mean annual discharge of 8–12 m³s−1 (water gauge Rosenheim, https://www.hnd.bayern.de) for hydropower production. Due to its monotonous channel morphology comprising steep bank angles, the HB has been a subject of intensive restoration works in recent years (Pander et al., 2017). A particular focus was on the restoration of shallow bank habitats along its shoreline, and 41 shallow “bank habitats” were cut into the bank along a 3-km river course (Figure 3), intended to provide habitat for the larvae and juveniles of endangered riverine fish species. The bank habitats correspond to habitat type 1 in the study by Pander et al. (2017), described as a highly engineered bank habitat cut into a monotonous trapezoidal cross section, resulting in a steep bank angle (>45%). At the bottom of the bank, there are either boulders placed to avoid bank erosion or the complete bank is stabilized by a typical boulder rip-rap structure (boulder size 400–800 mm). The bottom of the bank habitat is covered with gravel and finer grain sizes. It is structurally enriched by single boulders as well as coarse woody debris. Shortly after their construction, these habitats were partly colonized by macrophytes (Pander et al., 2017). In addition to the creation of bank habitats, the HB was used to restore fish migration in the River Inn at the power plant Feldkirchen by supplementing an additional discharge of 0.5 m³s−1 via a technical fishpass that connects the HB upstream the power plant Feldkirchen to the River Inn. This 3-km river course including its bank habitats is the subject of this study. For maintenance reasons of the power plants located at the HB in the city of Rosenheim, regularly, every two years, the HB is shut down for a time of several weeks, and there only remains a minimal discharge of 3–4 m³ s−1 (33.3 %–37.5% of the mean annual flow). In 2016, dewatering was applied by an abrupt shut down of the discharge that was responsible for a very rapid drop in the water level in the HB. In 2021, the discharge in the HB was successively reduced with a dropping rate of 11 cm h−1.
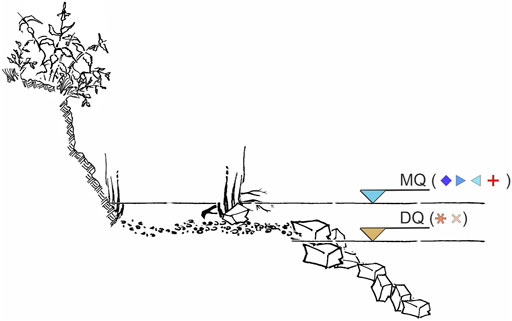
FIGURE 3. Schematic view of the assessed bank habitat type with the indication of the mean water level (MQ) and the low water level (DQ) during the dewatering. Symbols behind the depth indication MQ and DQ indicate the present fish community composition according to Figure 6.
Fish Stranding
All stranded fish were collected after the dewatering of the existing 41 bank habitats within five hours. The collection of fish started immediately when the water level dropped below the bank habitats, reaching its final low level (Figure 3). Following the sampling design, specimens were determined to the species level, measured to the nearest mm and—if alive—immediately released into the remaining flow channel of the HB. Since the water level did not drop further during fish rescue, it is impossible that once released fish stranded again and the possibility of double fish counts can thus be ruled out. All puddles in the bank habitats were consecutively screened, and at this stage, all remaining fish were caught with dip nets. In addition, all rocks larger than approximately 120 mm were turned and all the macrophyte patches were searched for hidden fish individuals. In puddles where the water depth exceeded 10 cm, a 3 kW electrofishing backpacker (Hans Grassl GmbH, Schoenau, Germany) was additionally used for a complete sampling.
Fish Community Assessment
Fish community assessment in the bank habitats was carried out seasonally in spring, summer, and autumn of 2017 and 2021, following the approach described in the study by Pander et al. (2017), where the fish community of bank habitats was assessed using a backpacking electrofishing generator. In addition, fish community assessment of the HB in 2021 followed the approach described by Pander and Geist (2010), who assessed the fish community in a pre-Alpine River in 30 m stretches using a boat-based electrofishing device. During all fishing campaigns, the same subset of nine bank habitats and HB habitats were consecutively sampled working from the downstream to upstream direction with two independently operating teams within a 5-h period. The bank habitats were sampled with a 3-kW electrofishing backpacker (Hans Grassl GmbH, Schoenau, Germany). Due to the low water depth in these habitats compared to the HB, the fishing crew worked wading along the entire bank habitat area. The fish were stunned with a single anode, collected with a dip net, and transferred into a large 80-L plastic bin before species determination. To check for the presence of mature specimens and for the fish community assessment of the HB, the channel of the HB was sampled using a boat-based 8 kW electrofishing generator (EFKO FEG 8000, EFKO-Elektrofischfanggeräte GmbH, Leutkirch, Germany). A single anode was used and stunned fish were collected with a dipnet while the boat was driving upstream. The total lengths of all specimens were measured to the nearest mm. All caught fish were immediately released after the electrofishing into the same habitats from which they were caught. The sampling stretches were not blocked with a net or similar device to hinder fish from fleeing up- or downstream due to safety issues concerning the boat-based electrofishing. All electrofishing activities were carried out under the license number 31-7562 issued by the district office of Freising, Bavaria, Germany. No mortality occurred and all fish were released in good condition.
Abiotic Habitat Variables
Ecologically relevant physicochemical variables were measured in the free-flowing section of the HB and in the bank habitats. The variables were measured in the open water of the river shortly after the electrofishing of each habitat. Temperature [°C], dissolved oxygen [mgl−1], electrical conductivity [µScm−1, corrected to 20°C], and pH were measured with a hand-held WTW® Multimeter 340i (WTW GmbH, Weilheim, Germany). In addition, turbidity [NTU] was assessed using a WTW® Turb 355 IR measuring set. Habitat surface area [m2], water depth [cm], current speed 10 cm above ground [(ms−1), Ott MFpro, Ott Hydromet GmbH, Kempten, Germany], as well as 10 cm below surface were measured at each bank habitat according to Pander et al. (2015) at nine measurement points distributed along three transects. Due to their structural habitat function (Jungwirth et al., 2003; Hauer and Lamberti, 2017) and potential function as a fish trap (as indicated in Bell et al., 2008) during the dewatering process, the presence and cover of macrophytes [(%), including helophytes and macroalgae], dead wood [%], and large boulders (structurally effective blocks between 400 and 800 mm) were mapped within each bank habitat. The percentage of dead wood was estimated in 5% steps according to Kail and Gerhard (2003). Percentages of dead wood accumulations less than 5% were estimated in 1% steps. In addition, substrate texture in percent coverage of fines (<2 mm) and gravel (>2 mm) was determined using the photographs of the bank habitats.
Data Analysis
For the univariate multiple-group comparisons of abiotic habitat variables and annual differences in fish frequencies, each dataset was tested for normal distribution (Shapiro–Wilk test) and homoscedasticity (Levene’s test). Since data did not fulfil the criteria for parametric testing, the nonparametric Kruskal–Wallis test was applied to test if the median values of abiotic habitat variables differ significantly between the bank habitats. Since previous investigations in the HB had shown that the restored river banks provide important habitats for juvenile life stages of endangered rheophilic species (Pander et al., 2017), the frequency of individuals of Squalius cephalus, Leuciscus leuciscus, Barbus barbus, Chondrostoma nasus, Alburnoides bipunctatus, and Gobio gobio was compared between sampling events using box-whisker plots and tested univariate with the Kruskal–Wallis test. A subsequent post hoc Wilcoxon test with Bonferroni correction for multiple comparisons was used to determine whether the median values of abiotic habitat variables or fish frequency differed significantly between 2017 and 2021 and seasons. Univariate statistics were carried out using statistical and graphical open-source software R (version 4.0.3, https://www.r-project.org).
To analyze the potential effects of the dewatering event on the fish community composition in the bank habitats, Bray–Curtis similarities from fish abundance data were computed in PRIMERv7. Based on the calculated Bray–Curtis resemblance matrix, an analysis of similarities (ANOSIM) was applied to test for significant differences in the fish community composition among the inter- and intra-annual pairwise comparisons of sampling events. Seasonal differences in the fish community composition, before and after the dewatering of the bank habitats, were visualized by the metric multidimensional scaling (MDS) of bootstrap averages based on the same Bray–Curtis similarities.
For all comparisons, significance was accepted at p ≤ 0.05 (= 95% probability).
Results
Abiotic Results
One day after the dewatering in 2021, 24 puddles remained in the bank habitats covering 3% of the respective bank habitat area. A total of three puddles were still connected to the main channel of the HB, while the others were completely disconnected. Important variables (Table 1) such as oxygen availability dropped in some of the puddles below 3 mgl−1 and the water depth comprised maximum values of less than 5 cm in half of the puddles. Those puddles fell completely dry during the next day. Macrophytes and deadwood in bank habitats were still present. However, the macrophytes were slumped into dense pads. After the normal water level was re-established, some of the macrophytes rose again. Macrophyte coverage remained almost constant during the sampling in summer compared to the conditions during the dewatering two month earlier in spring. Since no dead wood was displaced during dewatering or the water level rise, dead wood coverage was also constant during the fish community assessment. In addition, no differences in substratum composition between seasons could be detected. During the fish community assessment, bank habitats differed significantly (Wilcoxon test, p < 0.05) in temperature (between seasons and years), oxygen concentration (between seasons and years), electric conductivity (between seasons and years), and turbidity (between seasons and years). Water depth differed only significantly between the main channel of the HB (2.4 fold deeper) and the bank habitats in both years (Wilcoxon test, p < 0.05). A detailed description of important physicochemical variables is given in Table 2.
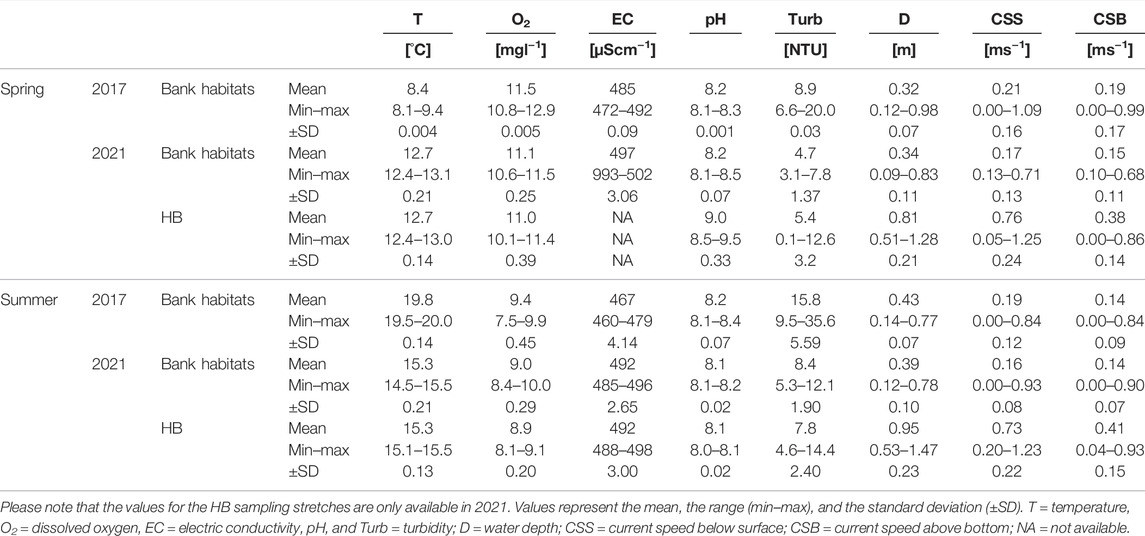
TABLE 2. Important physicochemical variables measured in the sampling stretches of the Hammerbach (HB) and the restored bank habitats in spring and summer 2017 and 2021.
Fish Stranding
During the two dewatering events in 2016 and 2021, 184 fish individuals from 12 species (out of 29 species identified during the community assessment) were detected stranded in the remaining puddles of the bank habitats with 154 individuals found in 2016 and 33 found in 2021. The most common stranded species in 2016 were S. cephalus, Cottus gobio, Rutilus rutilus, Alburnus alburnus, A. bipunctatus, Barbatula barbatula, and B. barbus. In 2021, most stranded specimens belonged to Gasterosteus aculeatus, B. barbatula, and C. gobio. No individuals of the following species, S. cephalus, R. rutilus, A. bipunctatus, and B. barbus, could be found in 2021 stranded in the bank habitats. G. aculeatus, Esox lucius, Misgurnus sp., and Eudontomyzon sp. were not found in 2016. However, except G. aculeatus, they occurred in very low frequencies (n = 1) in 2021 (Table 3).
The overall mortality of stranded fish across species was 16%. For E. lucius, Misgurnus sp., and Eudontomyzon sp., no dead individuals were detected. Mortality was mostly pronounced for A. alburnus (38.1%), B. barbatula (25.0%), R. rutilus (22.2%), B. barbus (18.8%), and C. gobio (10.0%) (Figure 4). The highest stranding risk in relation to their abundance in bank habitats was found for the small, less mobile, and highly protected species C. gobio. In addition, the stranding risk was also high for small individuals of R. rutilus and S. cephalus. The lowest stranding risk in relation to their abundance was detected for the species C. nasus, B. barbus, and L. leuciscus (Figure 5).
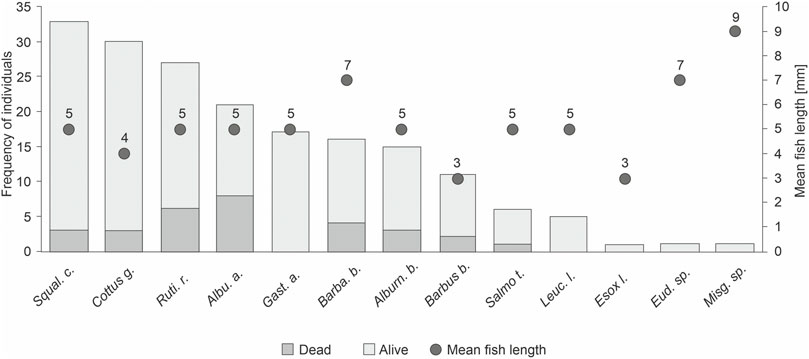
FIGURE 4. Barplots of stranded fish individuals and their mortality, combined for 2016 and 2021. Left axis displays the frequency of stranded individuals and right axis indicates the mean total length of stranded fish.
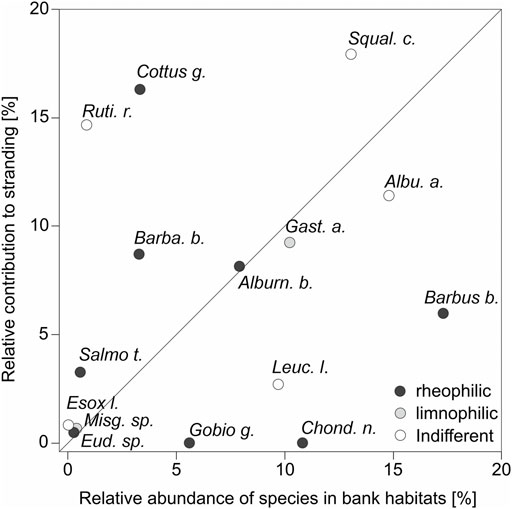
FIGURE 5. Scatterplot presenting the relative abundance of fish present in the bank habitats of the River Hammerbach versus the relative contribution of species to fish stranded during the dewatering. Shaded symbols indicate the different current preferences of species according to Zauner and Eberstaller (1999). For abbreviations of species, refer to Table 3. Due to their very low abundance and absence as stranded fish in bank habitats, the following species are not displayed in the plot: Blicca bjoerkna, Carassius gibelio, Cyprinus carpio, Leuciscus aspius, and Leuciscus idus.
Effects on Fish Community Composition
A total of 5,356 individuals from 29 species were caught during the fish community assessment. In the control (2017) and the year of dewatering (2021), fish community composition did not differ significantly (ANOSIM: R = 0.001, p = 0.41; Table 4) among the bank habitats during the spring samplings, indicating the similar baseline conditions. Even though a strong seasonal turnover form spring to summer and autumn was observed in both years (Table 4), highly significant differences in autumn and summer sampling between 2017 and 2021 were detected. These differences were particularly pronounced in summer (ANOSIM: R = 0.852, p < 0.001), directly after the dewatering event, but they were still evident in autumn, two months after the dewatering event (ANOSIM: R = 0.693, p < 0.001). No significant differences could be detected between the summer and autumn fish community in 2017 and 2021, respectively (Figure 6; Table 4).
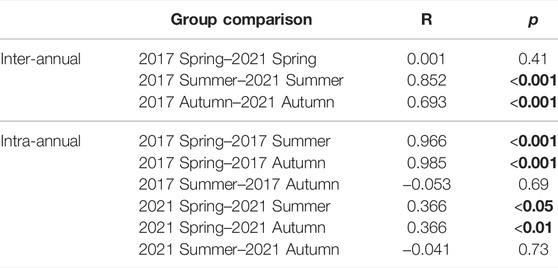
TABLE 4. Pairwise comparisons of one-way analysis of similarities (ANOSIM) regarding significant differences of fish community composition in bank habitats between different years as well as before and after the dewatering of the Hammerbach. Significant differences between comparisons are indicated in bold.
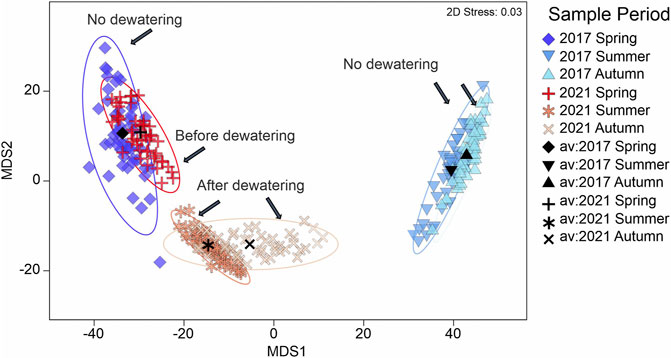
FIGURE 6. Metric multidimensional scaling (MDS) of bootstrap averages of the fish community composition in 2017 and 2021 before and after the dewatering event. Bootstrap averages are based on the pairwise Bray–Curtis similarities between the fish community composition of each sampling event. Shaded ellipses represent more than 95% of all bootstrap average points of each site, av = average.
Differences in the summer and autumn fish community between the control (2017) and the year of dewatering (2021) were already apparent at the level of detected individuals (summer 2017 = 1,783, summer 2021 = 657, autumn 2017 = 1,894, and autumn 2021 = 419), but also in strong abundance changes of certain species. Fish community changes were mainly associated with the endangered rheophilic species such as C. nasus and B. barbus, A. bipunctatus and G. gobio, and to a lesser extent also with S. cephalus and L. leuciscus (Figure 7). Mature individuals of all these six species (potential spawners) were abundant in the samples in the HB channel during the fish community assessment across all seasons.
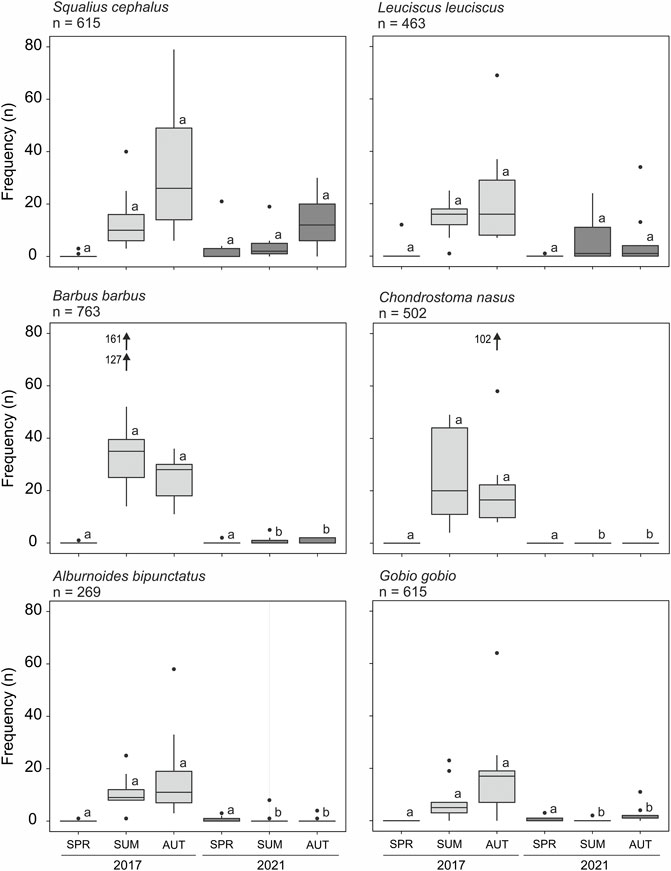
FIGURE 7. Box–whisker plots presenting individual frequencies (n) of selected species separated by season and year before (light grey boxes) and after the dewatering (dark grey boxes). Each box refers to nine replicates of the sampled bank habitats. Different small letters above boxes indicate significant differences of pairwise comparisons of sampled seasons between 2017 and 2021. SPR = spring, SUM = summer, and AUT = autumn. Box = 25% quantile, median, 75% quantile; whisker = minimum, maximum values. Black arrows indicate outliers.
Discussion
Management and restoration of aquatic ecosystems and aquatic species communities promise the greatest success when they are evidence-based (Palmer et al., 2005; Kondolf et al., 2007; Pander and Geist 2013; Geist 2015). This is particularly true for the mitigation of well-known stressors such as hydropower generation, where many of its effects on aquatic ecosystems have been intensively studied. However, even in well-known stressor-effect chains, filling of knowledge gaps such as those presented in this study can help on the way from ecological understanding into policy-making (Palmer et al., 2005; Geist and Hawkins 2016). The findings of the present study are of particular importance for an improved and less ecologically harmful maintenance management of tributaries in the hydropower-affected rivers. This is particularly important since it is well known that heavily modified water bodies and channels for hydropower generation such as the HB can greatly contribute to overall fish diversity (De Leeuw et al., 2007; Pander and Geist, 2010; Davis and Moore 2016). Such rivers do not only harbor many generalist species but also provide habitat for many highly specialized species (e.g., Pander and Geist, 2018), such as B. barbus or C. gobio which are under strict protection by national and international legislation (European Parliament 1992; Bohl et al., 2003). Legislation regarding the protection of species or habitats is strict if it is framed on European basis or transformed into national laws by the member states. For instance, it is strictly forbidden by law to kill protected animals or to destroy habitats of those by human action (European Parliament 1992). The dewatering of the HB caused both direct mortality of fish by stranding and the inaccessibility of important shallow bank habitats during the low water phase. Such areas can be crucial to fulfil critical life stages in the life cycle of many riverine fish, and with a decrease in the availability of these habitats, population effects are a logical consequence (Pander et al., 2017; Hayes et al., 2019b; Moreira et al., 2019; Stoffers et al., 2022).
The results of our study provide evidence that the dewatering of a channel for hydropower generation had multiple effects. First, it affected single individuals of fish directly by their stranding as well as by their habitats falling dry. The observed effects extended over a certain period since the dewatering also affected fish community composition months after its conductance. This was particularly evident in strong abundance declines or even complete absence of species with high conservation value such as C. nasus, B. barbus, and A. bipunctatus in samplings following the dewatering event. The intensity of fish stranding was dependent on the time of the year and dewatering speed, with more stranded fish being detected during the first very fast dewatering event in August 2016 compared to the second event in 2021. In contrast to fish stranding, community effects caused by dewatering were rather strong, most likely due to the restricted habitat availability in 2021 when the dewatering phase lasted several weeks from the middle of May to the beginning of August. This time of the year falls within the main larval drift phase of riverine fish at the River Inn (Nagel et al., 2021), and these types of bank habitats usually serve as a crucial refuge area for the development and growth of fish larvae and young-of-the-year fish (Pander et al., 2017; Stoffers et al., 2022).
As evident from the comparison of relative abundances, the risk for stranding was very different among species. Small and least mobile specimens of C. gobio, a bottom-oriented, cold stenothermic fish without a swim bladder (Utzinger et al., 1998), had an overproportioned high risk of stranding compared to the larger and open-water-oriented species such as C. nasus. Due to the timely sampling in this study, effects of predation on the stranded fish by piscivorous birds or mammals can be widely excluded which could be expected otherwise. In combination with the fact that macrophytes were slumped into dense pads making it difficult to find stranded fish (Bell et al., 2008), our data may actually underestimate real mortality associated with such dewatering events. Furthermore, in isolated puddles with no connectivity to the main channel, it is possible that a successively progressing oxygen depletion (Branco et al., 2016; Hayes et al., 2019a) would have caused much higher mortality rates than detected in the timely sampling after the dewatering in this study. It needs to be noted that partial dewatering of bank habitats can also be a natural process in free-flowing rivers not affected by hydropower. In natural rivers, this effect can contribute to the lateral nutrient exchange. However, in a natural environment, the dewatering of bank habitats is often much slower and only parts of the bank habitats are affected with the possibility for fish to move to equivalent substitute areas. This is particularly the case when the bank angles are flat and the dropping speed of the water is low. Under such conditions, a clear cutoff between bank habitats and the remaining main channel is almost impossible. The lowering of the water level for the maintenance of the hydropower system is usually realized within a very short time span of several hours. In this study, the dewatering speed did not allow all the individuals to escape into other areas or the main stem. Channels are often built as monotonous river courses with steep bank angles not comprising shallow habitats for fish as refuge as would be required. When small fish or fish larvae that are known as weak swimmers (Lechner, et al., 2014) are drifted into the main stem with faster current, they are more likely to get dislocated downstream without the potential to migrate back (Baras and Lucas, 2001; Pavlov et al., 2019). This can lead to shifts in population recruitment patterns with a particular decline of sensitive rheophilic species (Zauner and Eberstaller, 1999) as detected in this study for C. nasus, B. barbus, and A. bipunctatus in the HB. In contrast, S. cephalus, a generalist species of the HB, was able to cope better with the dewatering, potentially due to its ability to spawn several times throughout spring and summer. It can also use the bank habitats for the larval development after the dewatering ended. Species that are known to have a very limited time phase for spawning are much more susceptible to population effects when the dewatering of habitats fall within their spawning season, which may ultimately result in a loss of an entire generation. In addition, shallow areas rich of fine sediment and organic matter, like in the assessed bank habitats, can harbor highly specialized species such as lampreys or freshwater mussels. These species have a distinct life cycle that can comprise a very long phase in a very restricted habitat area. Lampreys such as Eudontomyzon sp. are known to have a sediment phase lasting five to seven years before they eventually emerge, transform to adults, and spawn (e.g., Dawson et al., 2015). In this case, population effects can even affect multiple age classes if these individuals are not able to find other substitute habitats during the dewatering phase.
The evidence about the negative ecological effects detected herein can be transferred into the policy to reduce the negative effects of dewatering to a minimum, and to contribute to the fulfilment of national and international legal requirements. At first, the usefulness of this measure should be seriously checked. If not avoidable, it should be accompanied by monitoring to determine the least harmful time window and duration for such maintenance measures. Generally, if the dewatering is not avoidable, the draining period should be kept as short as possible under the consideration of potential negative effects due to the stranding of fish and subsequently potential negative population effects due to the loss of habitat for critical life stages. To avoid stranding of fish larvae and fish, the dewatering should be carried out slowly over a time span of several days as also suggested in the study by Hayes et al. (2019a). Several general principles of flow management in relation to the reshaped channel morphologies have already been proposed, for example, in the “river in river concept” (Forseth and Harby, 2014). However, it would be very advantageous if the construction design of bank habitats prevented fish or fish larvae from being caught in the remaining puddles (Figure 8). In case fish are stranded, they should be collected and transferred into habitats where their survival is most likely. The dewatering should allow fish larvae and subsequent life stages (particularly weak swimmers) as well as lampreys and invertebrates (e.g., mollusks) to reach adequate substitute habitats during the dewatering phase. These habitats should ensure survival of these species until the dewatering ends and should allow them to migrate back. To avoid negative population effects, the dewatering should be applied outside the seasons of spawning, larval drift, and juvenile development. In this study, the main season for larval drift was spring to summer and the most dense fish population in bank habitats was detected in summer to autumn, matching previous findings of the River Inn system (Nagel et al., 2021). Consequently, the least harmful time span for dewatering in this project area would be late autumn to early spring when least fish are present in the bank habitats. However, it has to be noted that fish in overwintering habitats can also be negatively affected if those get disconnected from the main stem or fall dry. If the implementation of the abovementioned suggestions is impossible due to the many restrictions in channels and heavily modified water bodies, the responsible parties of the dewatering should compensate for the loss of species and negative effects on populations. In this context, a monitoring as mentioned previously could be used to assess the direct and indirect effects on fish and the fish community, and to determine adequate compensation. This can be achieved by a monetary habitat compensation (Venus et al., 2020) or by stocking fish eggs, larvae, or fish from the genetically adapted broodstock (Gum et al., 2009).
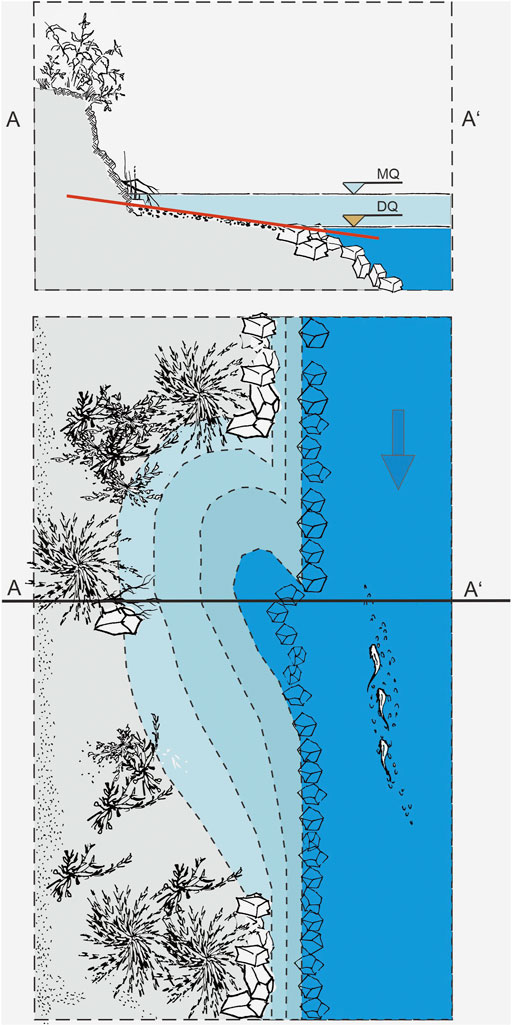
FIGURE 8. Schematic side view and top view as a suggestion of how to morphologically improve the restored bank habitats to avoid fish stranding with the indication of the mean water level (MQ) and the low water level (DQ) during the potential dewatering. Black line in top view with (A) to (A’) indicate the location of the cross section displayed in the sie view.
Data Availability Statement
The raw data supporting the conclusion of this article will be made available by the authors, without undue reservation
Ethics Statement
This study was conducted as part of a fish rescue event and thus did not require ethical review; the fish community assessment was done under license number 31-7562 issued by the district office of Freising, Bavaria, Germany (as explained in the article).
Author Contributions
All authors have read and agreed to the published version of the manuscript. Individual contributions are as follows: conceptualization: JP, CN, and JG; methodology: JP and CN; formal analysis: JP and CN; writing—original draft preparation: JP and CN; editing and artwork: JP; review: JG; project administration: JP and JG; resources: JG; funding acquisition: JG and JP.
Funding
We are grateful for the financial support of this study by Verbund Innkraftwerke GmbH, particularly to G. Loy who initiated and supported this project.
Conflict of Interest
The authors declare that the research was conducted in the absence of any commercial or financial relationships that could be construed as a potential conflict of interest.
Publisher’s Note
All claims expressed in this article are solely those of the authors and do not necessarily represent those of their affiliated organizations, or those of the publisher, the editors, and the reviewers. Any product that may be evaluated in this article, or claim that may be made by its manufacturer, is not guaranteed or endorsed by the publisher.
Acknowledgments
We would like to thank Johanna David who contributed to the sampling in the Hammerbach. We are also grateful to the local angling clubs, and all the volunteers and student assistants who helped with the electrofishing and the fish rescue.
References
Aarts, B. G. W., Van Den Brink, F. W. B., and Nienhuis, P. H. (2004). Habitat Loss as the Main Cause of the Slow Recovery of Fish Faunas of Regulated Large Rivers in Europe: The Transversal Floodplain Gradient. River Res. applic. 20 (1), 3–23. doi:10.1002/rra.720
Algera, D. A., Rytwinski, T., Taylor, J. J., Bennett, J. R., Smokorowski, K. E., Harrison, P. M., et al. (2020). What Are the Relative Risks of Mortality and Injury for Fish during Downstream Passage at Hydroelectric Dams in Temperate Regions? A Systematic Review. Environ. Evid. 9 (1), 1–36. doi:10.1186/s13750-020-0184-0
Anderson, E. P., Freeman, M. C., and Pringle, C. M. (2006). Ecological Consequences of Hydropower Development in Central America: Impacts of Small Dams and Water Diversion on Neotropical Stream Fish Assemblages. River Res. applic. 22 (4), 397–411. doi:10.1002/rra.899
Auer, S., Zeiringer, B., Führer, S., Tonolla, D., and Schmutz, S. (2017). Effects of River Bank Heterogeneity and Time of Day on Drift and Stranding of Juvenile European Grayling (Thymallus L.) Caused by Hydropeaking. Sci. Total Environ. 575, 1515–1521. doi:10.1016/j.scitotenv.2016.10.029
Auerswald, K., Moyle, P., Seibert, S. P., and Geist, J. (2019). HESS Opinions: Socio-Economic and Ecological Trade-Offs of Flood Management - Benefits of a Transdisciplinary Approach. Hydrol. Earth Syst. Sci. 23, 1035–1044. doi:10.5194/hess-23-1035-2019
Baras, E., and Lucas, M. C. (2001). Impacts of Man's Modifications of River Hydrology on the Migration of Freshwater Fishes: A Mechanistic Perspective. Int. J. Ecohydrol. Hydrobiol. 1 (3), 291–304.
Barlaup, B. T., Gabrielsen, S. E., Skoglund, H., and Wiers, T. (2008). Addition of Spawning Gravel-A Means to Restore Spawning Habitat of Atlantic Salmon (Salmo salar L.), and Anadromous and Resident Brown Trout (Salmo trutta L.) in Regulated Rivers. River Res. applic. 24, 543–550. doi:10.1002/rra.1127
Bartoň, D., Bretón, F., Blabolil, P., Souza, A. T., Vejřík, L., Sajdlová, Z., et al. (2021). Effects of Hydropeaking on the Attached Eggs of a Rheophilic Cyprinid Species. Ecohydrology 14 (4), e2280. doi:10.1002/eco.2280
Bell, E., Kramer, S., Zajanc, D., and Aspittle, J. (2008). Salmonid Fry Stranding Mortality Associated with Daily Water Level Fluctuations in Trail Bridge Reservoir, Oregon. North Am. J. Fish. Manag. 28 (5), 1515–1528. doi:10.1577/m07-026.1
Bohl, E., Kleisinger, H., and Leuner, E. (2003). Rote Liste gefährdeter Fische (Pisces) und Rundmäuler (Cyclostomata) Bayern. Bayerisches Landesamt für Umwelt, BayLfU/166/2003. Available online: https://www.sf-kemmern.de/pdf_Dateien/rote_liste.pdf (accessed on February 25, 2022).
Branco, P., Santos, J. M., Amaral, S., Romão, F., Pinheiro, A. N., and Ferreira, M. T. (2016). Potamodromous Fish Movements under Multiple Stressors: Connectivity Reduction and Oxygen Depletion. Sci. Total Environ. 572, 520–525. doi:10.1016/j.scitotenv.2016.08.070
Bruder, A., Tonolla, D., Schweizer, S. P., Vollenweider, S., Langhans, S. D., and Wüest, A. (2016). A Conceptual Framework for Hydropeaking Mitigation. Sci. Total Environ. 568, 1204–1212. doi:10.1016/j.scitotenv.2016.05.032
Bunn, S. E., and Arthington, A. H. (2002). Basic Principles and Ecological Consequences of Altered Flow Regimes for Aquatic Biodiversity. Environ. Manag. 30 (4), 492–507. doi:10.1007/s00267-002-2737-0
Casas-Mulet, R., Saltveit, S. J., and Alfredsen, K. (2015). The Survival of Atlantic Salmon (Salmo salar) Eggs during Dewatering in a River Subjected to Hydropeaking. River Res. applic. 31 (4), 433–446. doi:10.1002/rra.2827
Casas-Mulet, R., Saltveit, S. J., and Alfredsen, K. T. (2016). Hydrological and Thermal Effects of Hydropeaking on Early Life Stages of Salmonids: A Modelling Approach for Implementing Mitigation Strategies. Sci. Total Environ. 573, 1660–1672. doi:10.1016/j.scitotenv.2016.09.208
Choi, B., and Choi, S.-U. (2018). Impacts of Hydropeaking and Thermopeaking on the Downstream Habitat in the Dal River, Korea. Ecol. Inf. 43, 1–11. doi:10.1016/j.ecoinf.2017.10.016
Curley, E. A. M., Thomas, R., Adams, C. E., and Stephen, A. (2022). Adaptive Responses of Freshwater Pearl Mussels, Margaritifera , to Managed Drawdowns. Aquat. Conserv. 32 (3), 466–483. doi:10.1002/aqc.3759
Davis, A. M., and Moore, A. R. (2016). Conservation Potential of Artificial Water Bodies for Fish Communities on a Heavily Modified Agricultural Floodplain. Aquat. Conserv. Mar. Freshw. Ecosyst. 26 (6), 1184–1196. doi:10.1002/aqc.2607
Dawson, H. A., Quintella, B. R., Almeida, P. R., Treble, A. J., and Jolley, J. C. (2015). “The Ecology of Larval and Metamorphosing Lampreys,” in Lampreys: Biology, Conservation and Control. Editor M. F. Docker (Dordrecht, Netherlands: Springer), 75–137. doi:10.1007/978-94-017-9306-3_3
De Leeuw, J. J., Buijse, A. D., Haidvogl, G., Lapinska, M., Noble, R., Repecka, R., et al. (2007). Challenges in Developing Fish-Based Ecological Assessment Methods for Large Floodplain Rivers. Fish. Manage 14 (6), 483–494. doi:10.1111/j.1365-2400.2007.00576.x
Entrekin, S. A., Tank, J. L., Rosi-Marshall, E. J., Hoellein, T. J., and Lamberti, G. A. (2008). Responses in Organic Matter Accumulation and Processing to an Experimental Wood Addition in Three Headwater Streams. Freshw. Biol. 53, 1642–1657. doi:10.1111/j.1365-2427.2008.01984.x
European Parliament (1992). Council Directive 92/43/EEC on the Conservation of Natural Habitats and of Wild Fauna and Flora. Off. J. Eur. Union 206, 7–50.
European Parliament (2000). Directive 2000/60/EC of the European Parliament and of the Council Establishing a Framework for the Community Action in the Field of Water Policy. Off. J. Eur. Union 327, 1–73.
Forseth, T., Harby, A., Ugedal, O., Pulg, U., Fjeldstad, H. P., Robertsen, G., et al. (2014). Handbook for Environmental Design in Regulated Salmon Rivers. Editors T. Forseth, and A. Harby (Bergen, Norway: Nina Special Report 53, 90.
Geist, J. (2015). Seven Steps towards Improving Freshwater Conservation. Aquat. Conserv. Mar. Freshw. Ecosyst. 25, 447–453. doi:10.1002/aqc.2576
Geist, J. (2021). Editorial: Green or Red: Challenges for Fish and Freshwater Biodiversity Conservation Related to Hydropower. Aquat. Conserv. Mar. Freshw. Ecosyst. 31 (7), 1551–1558. doi:10.1002/aqc.3597
Geist, J., and Hawkins, S. J. (2016). Habitat Recovery and Restoration in Aquatic Ecosystems: Current Progress and Future Challenges. Aquat. Conserv. Mar. Freshw. Ecosyst. 26 (5), 942–962. doi:10.1002/aqc.2702
Grabowski, T. B., and Isely, J. J. (2007). Effects of Flow Fluctuations on the Spawning Habitat of a Riverine Fish. Southeast. Nat. 6 (3), 471–478. doi:10.1656/1528-7092(2007)6[471:eoffot]2.0.co;2
Greimel, F., Schülting, L., Graf, W., Bondar-Kunze, E., Auer, S., Zeiringer, B., et al. (2018). “Hydropeaking Impacts and Mitigation,” in Riverine Ecosystem Management: Science for Governing towards a Sustainable Future. Editors S. Schmutz, and J. Sendzimir (Vienna, Austria: Riverine Ecosystem Management, Aquatic Ecology Series), 8, 91–110. doi:10.1007/978-3-319-73250-3_5
Gum, B., Gross, R., and Geist, J. (2009). Conservation Genetics and Management Implications for European grayling,Thymallus thymallus: Synthesis of Phylogeography and Population Genetics. Fish. Manag. Ecol. 16, 37–51. doi:10.1111/j.1365-2400.2008.00641.x
Gurnell, A., Tockner, K., Edwards, P., and Petts, G. (2005). Effects of Deposited Wood on Biocomplexity of River Corridors. Front. Ecol. Environ. 3 (7), 377–382. doi:10.1890/1540-9295(2005)003[0377:eodwob]2.0.co;2
Hauer, F. R., and Lamberti, G. A. (2017). “Macroinvertebrates,” in Methods in Stream Ecology: Volume 1: Ecosystem Structure. Editors F. R. Hauer, and G. A. Lamberti (Vienna, Austria: Academic Press), 297–319.
Hayes, D. S., Branco, P., Santos, J. M., and Ferreira, T. (2019a). Oxygen Depletion Affects Kinematics and Shoaling Cohesion of Cyprinid Fish. Water 11, 642. doi:10.3390/w11040642
Hayes, D., Moreira, M., Boavida, I., Haslauer, M., Unfer, G., Zeiringer, B., et al. (2019b). Life Stage-specific Hydropeaking Flow Rules. Sustainability 11 (6), 1547. doi:10.3390/su11061547
Jepsen, D. B. (1997). Fish Species Diversity in Sand Bank Habitats of a Neotropical River. Environ. Biol. Fishes 49, 449–460. doi:10.1023/a:1007371132144
Jungwirth, M., Haidvogl, G., Moog, O., Muhar, S., and Schmutz, S. (2003). Angewandte Fischökologie an Fließgewässern. 1st ed. Stuttgart, Germany: UBT, 547.
Kail, J., and Gerhard, M. (2003). Totholz in Fließgewässern – eine Begriffsbestimmung. Wasser Boden 55 (1-2), 49–55.
Kondolf, G. M., Anderson, S., Lave, R., Pagano, L., Merenlender, A., and Bernhardt, E. S. (2007). Two Decades of River Restoration in California: What Can We Learn? Restor. Ecol. 15, 516–523. doi:10.1111/j.1526-100x.2007.00247.x
Lechner, A., Keckeis, H., Schludermann, E., Humphries, P., McCasker, N., and Tritthart, M. (2014). Hydraulic Forces Impact Larval Fish Drift in the Free Flowing Section of a Large European River. Ecohydrol. 7 (2), 648–658. doi:10.1002/eco.1386
Lorenz, A. W., Stoll, S., Sundermann, A., and Haase, P. (2013). Do adult and YOY Fish Benefit from River Restoration Measures? Ecol. Eng. 61, 174–181. doi:10.1016/j.ecoleng.2013.09.027
Meulenbroek, P., Drexler, S., Nagel, C., Geistler, M., and Waidbacher, H. (2018). The Importance of a Constructed Near-nature-like Danube Fish By-Pass as a Lifecycle Fish Habitat for Spawning, Nurseries, Growing and Feeding: A Long-Term View with Remarks on Management. Mar. Freshw. Res. 69, 1857–1869. doi:10.1071/mf18121
Moreira, M., Hayes, D. S., Boavida, I., Schletterer, M., Schmutz, S., and Pinheiro, A. (2019). Ecologically-based Criteria for Hydropeaking Mitigation: A Review. Sci. Total Environ. 657, 1508–1522. doi:10.1016/j.scitotenv.2018.12.107
Moyle, P. B., and Leidy, R. A. (1992). “Loss of Biodiversity in Aquatic Ecosystems: Evidence from Fish Faunas,” in Conservation Biology. Editors P. L. Fiedler, and S. K. Jain (Boston, MA: Springer). doi:10.1007/978-1-4684-6426-9_6
Muehlbauer, J. D., Doyle, M. W., and Bernhardt, E. S. (2011). Macroinvertebrate Community Responses to a Dewatering Disturbance Gradient in a Restored Stream. Hydrol. Earth Syst. Sci. 15, 1771–1783. doi:10.5194/hess-15-1771-2011
Mueller, M., Bierschenk, A. M., Bierschenk, B. M., Pander, J., and Geist, J. (2020). Effects of Multiple Stressors on the Distribution of Fish Communities in 203 Headwater Streams of Rhine, Elbe and Danube. Sci. Total. Environ. 703, 134523. doi:10.1016/j.scitotenv.2019.134523
Nagel, C., Mueller, M., Pander, J., Stoeckle, B. C., Kuehn, R., and Geist, J. (2021). Going with the Flow: Spatio-Temporal Drift Patterns of Larval Fish in a Large Alpine River. Freshw. Biol. 66, 1765–1781. doi:10.1111/fwb.13790
Nagel, C., Mizerakis, V. L., Pander, J., and Geist, J. (2022). The Overlooked Contribution of a Fish Bypass Channel to the Density and Diversity of Macroinvertebrate Drift in a Heavily Modified River System. In prep.
Palmer, M. A., Bernhardt, E. S., Allan, J. D., Lake, P. S., Alexander, G., Brooks, S., et al. (2005). Standards for Ecologically Successful River Restoration. J. Appl. Ecol. 42, 208–217. doi:10.1111/j.1365-2664.2005.01004.x
Pander, J., Casas-Mulet, R., and Geist, J. (2022). Hydropeaking Impairs Upstream Salmonid Spawning Habitats in a Restored Danube Tributary. Riv. Res. Appl. (early view). doi:10.1002/rra.3953
Pander, J., and Geist, J. (2010). Seasonal and Spatial Bank Habitat Use by Fish in Highly Altered Rivers - a Comparison of Four Different Restoration Measures. Ecol. Freshw. Fish. 19, 127–138. doi:10.1111/j.1600-0633.2009.00397.x
Pander, J., and Geist, J. (2013). Ecological Indicators for Stream Restoration Success. Ecol. Indic. 30, 106–118. doi:10.1016/j.ecolind.2013.01.039
Pander, J., and Geist, J. (2016). Can Fish Habitat Restoration for Rheophilic Species in Highly Modified Rivers Be Sustainable in the Long Run? Ecol. Eng. 88, 28–38. doi:10.1016/j.ecoleng.2015.12.006
Pander, J., and Geist, J. (2018). The Contribution of Different Restored Habitats to Fish Diversity and Population Development in a Highly Modified River: A Case Study from the River Günz. Water 10 (9), 1202. doi:10.3390/w10091202
Pander, J., Mueller, M., and Geist, J. (2015). A Comparison of Four Stream Substratum Restoration Techniques Concerning Interstitial Conditions and Downstream Effects. River Res. applic. 31 (2), 239–255. doi:10.1002/rra.2732
Pander, J., Mueller, M., Knott, J., Egg, L., and Geist, J. (2017). Is it Worth the Money? the Functionality of Engineered Shallow Stream Banks as Habitat for Juvenile Fishes in Heavily Modified Water Bodies. River Res. applic. 33, 63–72. doi:10.1002/rra.3065
Pander, J., Nagel, C., Ingermann, H., and Geist, J. (2021). Water Level Induced Changes of Habitat Quality Determine Fish Community Composition in Restored and Modified Riverbanks of a Large Alpine River. Int. Rev. Hydrobiol. 107, 1–14. doi:10.1002/iroh.202002079
Pavlov, D. S., Mikheev, V. N., and Kostin, V. V. (2019). Migrations of Fish Juveniles in Dammed Rivers: the Role of Ecological Barriers. J. Ichthyol. 59 (2), 234–245. doi:10.1134/s0032945219020140
Postel, S., and Richter, B. (2012). Rivers for Life: Managing Water for People and Nature. Washington DC, USA: Island press.
Puffer, M., Berg, O. K., Huusko, A., Vehanen, T., Forseth, T., and Einum, S. (2015). Seasonal Effects of Hydropeaking on Growth, Energetics and Movement of Juvenile Atlantic Salmon (Salmo salar). River Res. applic. 31 (9), 1101–1108. doi:10.1002/rra.2801
Reid, A. J., Carlson, A. K., Creed, I. F., Eliason, E. J., Gell, P. A., Johnson, P. T. J., et al. (2019). Emerging Threats and Persistent Conservation Challenges for Freshwater Biodiversity. Biol. Rev. 94, 849–873. doi:10.1111/brv.12480
Schmutz, S., Bakken, T. H., Friedrich, T., Greimel, F., Harby, A., Jungwirth, M., et al. (2015). Response of Fish Communities to Hydrological and Morphological Alterations in Hydropeaking Rivers of Austria. River Res. applic. 31 (8), 919–930. doi:10.1002/rra.2795
Seliger, C., Scheikl, S., Schmutz, S., Schinegger, R., Fleck, S., Neubarth, J., et al. (2016). Hy:Con: A Strategic Tool for Balancing Hydropower Development and Conservation Needs. River Res. applic. 32 (7), 1438–1449. doi:10.1002/rra.2985
Smith, E. P., Orvos, D. R., and Cairns Jr., J. (1993). Impact Assessment Using the Before-After-Control-Impact (BACI) Model: Concerns and Comments. Can. J. Fish. Aquat. Sci. 50 (3), 627–637. doi:10.1139/f93-072
Stoffers, T., Buijse, A. D., Verreth, J. A. J., and Nagelkerke, L. A. J. (2022). Environmental Requirements and Heterogeneity of Rheophilic Fish Nursery Habitats in European Lowland Rivers: Current Insights and Future Challenges. Fish. 23 (1), 162–182. doi:10.1111/faf.12606
Tockner, K., and Stanford, J. A. (2002). Riverine Flood Plains: Present State and Future Trends. Envir. Conserv. 29 (3), 308–330. doi:10.1017/s037689290200022x
Utzinger, J., Roth, C., and Peter, A. (1998). Effects of Environmental Parameters on the Distribution of Bullhead Cottus gobio with Particular Consideration of the Effects of Obstructions. J. Appl. Ecol. 35 (6), 882–892. doi:10.1111/j.1365-2664.1998.tb00006.x
Venus, T. E., Smialek, N., Pander, J., Harby, A., and Geist, J. (2020). Evaluating Cost Trade-Offs between Hydropower and Fish Passage Mitigation. Sustainability 12 (20), 8520. doi:10.3390/su12208520
Zarfl, C., Lumsdon, A. E., Berlekamp, J., Tydecks, L., and Tockner, K. (2015). A Global Boom in Hydropower Dam Construction. Aquat. Sci. 77, 161–170. doi:10.1007/s00027-014-0377-0
Zauner, G., and Eberstaller, J. (1999). Klassifizierungsschema der Österreichischen Flußfischfauna in Bezug auf Deren Lebensraumansprüche. Osterr. Fisch. 52, 198–205. Available at: https://www.ezb-fluss.at/wp-content/uploads/Klassifizierungsschema_Fische.pdf (accessed on March 29, 2022).
Keywords: channel dewatering, water diversion, hydropower operation, fish stranding, river restoration, aquatic conservation, hydropeaking, drought
Citation: Pander J, Nagel C and Geist J (2022) Effects of a Hydropower-Related Temporary Stream Dewatering on Fish Community Composition and Development: From Ecology to Policy. Front. Environ. Sci. 10:929746. doi: 10.3389/fenvs.2022.929746
Received: 27 April 2022; Accepted: 10 June 2022;
Published: 15 July 2022.
Edited by:
Riccardo Fornaroli, University of Milano-Bicocca, ItalyReviewed by:
Daniel S. Hayes, University of Natural Resources and Life Sciences Vienna, AustriaMauro Carolli, SINTEF Energy Research, Norway
Copyright © 2022 Pander, Nagel and Geist. This is an open-access article distributed under the terms of the Creative Commons Attribution License (CC BY). The use, distribution or reproduction in other forums is permitted, provided the original author(s) and the copyright owner(s) are credited and that the original publication in this journal is cited, in accordance with accepted academic practice. No use, distribution or reproduction is permitted which does not comply with these terms.
*Correspondence: Juergen Geist, Z2Vpc3RAdHVtLmRl