- 1College of Ecology and Environment, Xinjiang University, Urumqi, China
- 2Key Laboratory of Oasis Ecology, Ministry of Education, Urumqi, China
Elucidating the relationship between the variation of plant leaf functional traits and the environment is necessary for understanding the adaptation mechanism of plants and predicting changes in ecosystem structure. In this study, the leaf traits of desert plants in Ebinur Lake National Wetland Nature Reserve in Xinjiang, China were studied from the aspects of plant life forms (annuals, perennials and shrubs), phylogenetic signals, and relation to soil properties, using the principal component analysis, variance decomposition, and one-way analysis of variance. The results showed that: (1) There were significant differences in leaf carbon concentration (annuals>shrubs>perennials), leaf nitrogen concentration (shrubs ≥ perennials ≥ annuals), and leaf moisture content (perennials ≥ annuals ≥ shrubs) among the life forms, but there was no significant difference in leaf phosphorus concentration. Besides, soil nitrogen and phosphorus were significantly positively correlated with leaf carbon concentration and leaf nitrogen concentration. (2) There were significant differences in leaf carbon concentration, leaf nitrogen concentration, specific leaf area, and leaf moisture content between C3 and C4 plants, while the differences in P and leaf dry matter content were not significant. Besides, there were significant differences in leaf carbon concentration, leaf nitrogen concentration, specific leaf area, and leaf moisture content between leguminous and non-leguminous plants. Leguminous plants had higher leaf carbon concentration, leaf nitrogen concentration, and specific leaf area than non-leguminous plants, while non-leguminous plants had higher leaf moisture content than leguminous plants. (3) One way ANOVA analysis showed that taxonomy had a more significant effects on leaf carbon concentration, leaf nitrogen concentration, specific leaf area, and leaf moisture content than soil properties, and the coefficient of variation of leaf carbon concentration was greater than 50%. The phylogenetically independent contrasts analysis showed that the phylogenetic signal of all leaf traits was detected in all species and low (K value < 1, p > 0.05), indicating that the functional traits were weakly affected by phylogenetics. Therefore, desert plants in the Ebinur Lake Basin evolved to adapt to arid environments, and leaf traits showed convergent variation.
Introduction
Plant functional traits are biological characteristics that directly affect the growth and reproduction of plants (Ackerly and Cornwell, 2007). Leaf traits are important ecological indicators that can directly reflect the characteristics of plants utilizing environmental resources and the survival strategies to adapt to complex environments (Bao and Liu, 2009). Convergence refers to the tendency of organisms (two or more) with low affinities to evolve to have similar structures, functions, and phenotypes in similar habitats (usually under extreme environmental conditions) (Losos, 2011). Similar habitat conditions produce similar environmental stresses on the morphophysiological characteristics of different plants, and plants develop similar traits to compete for environmental resources and adapt to the environment, leading to the occurrence of convergent evolution, although there are many differences between their lineages (Givnish, 2017). Studies have shown that plants are dwarfed, with thick leaves in phylogenetic development in high-altitude areas (Losos, 2011), and annual succulents have small, white downy, or spiny leaves in desert areas (Griffiths and Males, 2017). Plants in karst areas generally show the characters of low specific leaf area (SLA) and leaf area (LA) and high leaf dry matter content (LDMC), indicating that plants tend to develop some traits to adapt to the drought caused by the shallow soil layer and soil water leakage in karst areas (Liu et al., 2014). Besides, studies in arid regions have shown that plants usually adapt to drought stress with thick and leathery leaves, low specific leaf area, small leaf size, increased leaf tissue density (LTD), and large specific root length (SRL) (Reich et al., 1998).
Environmental filtering can screen some species which can survive in a specific environment (Cui et al., 2022), especially in harsh environmental conditions (Botta-Dukát and Czúcz, 2016), and make their growth and abundance have convergent characteristics (Shipley et al., 2016). For example, under coastal environmental conditions, due to the influence of periodic tidal flooding, the leaf stomatal characteristics and photosynthetic function of plants up the tidal line showed convergent adaptation, while the leaf stomatal and morphological traits of plants below the tidal line showed co-evolution (Coyle et al., 2014). Besides, different plants have different levels of adaptation. For example, plants in the dune community in New Zealand have different water use patterns, thereby they can coexist, with a low competition between them. Convergence may be weakened by competition between functionally similar species, leading to differentiation. Harsh environmental conditions and physical factors may limit the survival and development of many plants. Study has found that plants in Alpine and desert have the patterns of convergence, differentiation, and diversification (Bao and Liu, 2009).
Based on convergence theory (McCoy-Sulentic et al., 2017), leaf traits are often used to elucidate the linkages between species traits, flora, habitats, and their roles in ecosystems in ecological researches (McCoy-Sulentic et al., 2017). The interspecific variation of leaf traits has the same trend in same environments at both small and large scales (Kattge et al., 2011), and this trend reinforces the plant strategy theories by illustrating the characteristics of plant traits developed in same environments (Westoby and Wright, 2006). As a basic framework for understanding species responses to ecological changes and their distribution differences, more and more researches have focused on the functional characteristics of plants.
Leaf traits of different plant taxa in different biomes have great ecological and evolutionary significance. Therefore, it is of great significance to examine the adaptation of leaf traits by phylogeny (Wang et al., 2017). The trait combination of plant species comes from trade-offs. In recent years, model studies have emerged to elucidate the leaf trait convergence and its influencing factors. However, the mechanisms underlying phylogenetic influences on leaf traits remain unclear.
Arid zones are one of the most vulnerable ecosystems, with water being the most important influencing factor (Guo et al., 2003). At present, due to global climate change and overexploitation, plants in arid desert areas are severely stressed in terms of growth, reproduction, and diversity. Ebinur Lake Basin has a typical arid desert ecosystem. Current studies mainly focus on the community characteristics, plant physiology and ecology, etc. in Ebinur Lake Basin, but there are few studies on the convergent evolution of desert plants. In this study, the desert plants with different life forms in the Ebinur Lake Basin were studied to explore their differences in functional traits and the relationship with phylogeny, aiming to clarify the changing trends of leaf traits among different desert plant species and taxa. We hypothesized that: (1) plants in the same environment might have a similar pattern of convergence in leaf morphology and physiological characteristics; and (2) the convergence pattern might be phylogenetically specific among different plant species. This study will improve our understanding of the variation of desert plant functional traits to similar environments in arid areas.
Materials and Methods
Experimental Site
The study area is located in Ebinur National Wetland Nature Reserve in Jinghe County, Bortala Mongol Autonomous Prefecture, Xinjiang, China (44°31′05″-45°09′35″N, 82°33′47″-83°53′21″E). As the lowest depression in the Junggar Basin, Ebinur Lake wetland is mainly recharged by rivers and groundwater. The Ebinur Lake Basin has a typical temperate continental climate, with dry air, little rain, and large evaporation. The average daily temperature was 6–8°C, the accumulated temperature was 3,000–3,500°C, and the average annual precipitation was 90.9 mm. The soil types are gray desert soil and aeolian sandy soil. Plants are drought tolerant, including Haloxylon ammodendron Bunge, Populus euphratica Oliv, Halimodendron halodendron Pall., Seriphidium kaschgaricum Krasch., Nitraria tangutorum Bobr., Kalidium foliatum Pall., Alhagi sparsifolia Shap., Aeluropus pungens Pungens., Apocynum venetum L., Sageretia pycnophylla Ulicina., Suaeda salsa L., Phragmites australis Cav., Calligonum mongolicum Turcz., Halimodendron halodendron Pall., Karelinia caspia Caspia., etc.
Experimental Design and Data Acquisition
In the Ebinur Lake Basin, 5 km away from the Aqikesu River, three transects (1.62 km in length) with a distance of 100 m from east to west were set in the direction perpendicular to the river. Then, plots (1.5 m × 1.5 m) were set at an interval of 40 m from north to south in each transect, and there were 120 plots in total (Figure 1). Plant samples were collected from June to July 2017 (the most vigorous growth season for plants), and the geographic coordinates and elevation of each plot and plant species in the study area were recorded (Supplementary Table S1). For the plants in each plot, a total of 20 g of complete and mature leaves were collected from each plant in the direction of south, east, north, and west. The leaf samples of the same species in each plot were bagged. Leaf samples of 17 plant genera were collected finally, but only 14 genera were used for this study duo to the low sample size of the other 3 genera (Supplementary Table S1). According to Halophytes in China (Zhao and Li, 1999), the plant samples were divided into 3 functional groups (4 annuals, 5 perennials, and 5 shrubs). Chemical analysis was conducted using the leaf samples in the lab after drying and grinding.
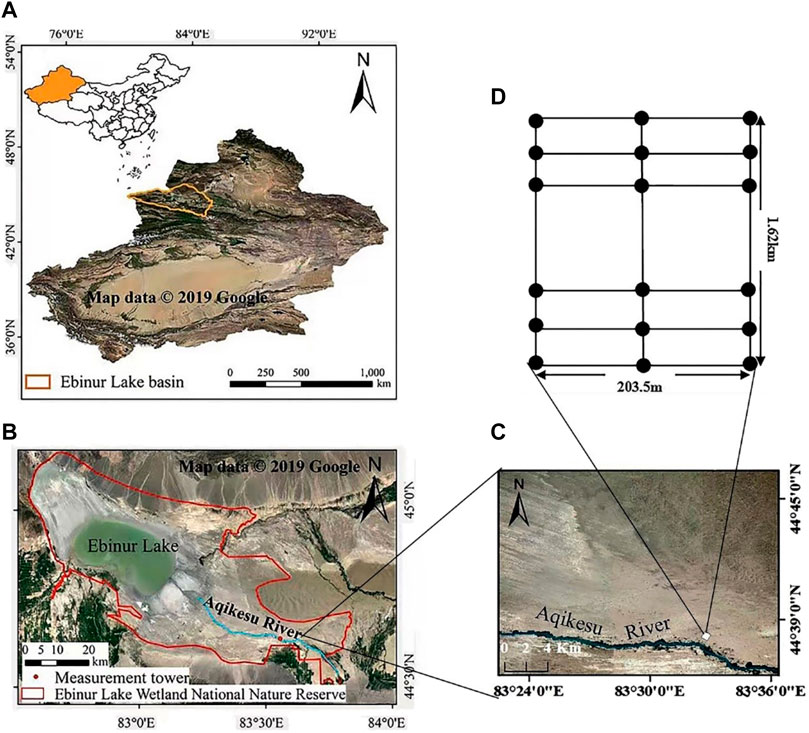
FIGURE 1. Remote sensing image of the study area. (A) Location of the Ebinur Lake Basin; (B) Location of the Aqikesu River; (C) Location of the study area; (D) Plot layout.
In this study, the leaf traits including leaf carbon concentration (LCC), leaf nitrogen concentration (LNC), leaf phosphorus concentration (LPC), specific leaf area (SLA), leaf dry matter content (LDMC), and leaf moisture content (LMC) were determined (Supplementary Table S1; Table 1).
In each plot, soils of the 0–30 cm layer were sampled using aluminum boxes (five replications), and mixed as the soil sample of the plot to measure the soil moisture content (SMC) in the lab. Besides, soils of 0–30 cm layer were also collected using shovel and packaged in ziplock bags, to determine soil properties including salt concentration (SSC), pH, organic matter concentration (SOC), total nitrogen concentration (STN), total phosphorus concentration (STP), and available phosphorus concentration (SAP) in the lab after air drying (Table 2).
Data Analysis
To identify and explain the variation in leaf traits of each species, the data were analyzed from functional group and phylogeny. Firstly, the data for each functional group (such as annuals, perennials, and shrubs) were analyzed at the species level. In addition, the differences in C, N, P, SLA, LDMC, and LMC between functional groups were explored by one-way analysis of variance (ANOVA) using SPSS software (IBM, NY, USA). Principal component analysis (PCA) was used to analyze the correlations of the above 6 traits of plant leaves. The general linear model (GLM) was used to compute the contribution of soil and plant functional groups/family to the total variance of leaf traits.
Plant classification was performed using Angiosperm Phylogeny Group III (APG-III) (Twohey et al., 2019), and the phylogenetic tree was constructed by using the online tool Phylomatic (http://www.phylodiversity.net/phylomatic/phylomatic.html). The phylogenetic signal (PS) intensity of all leaf traits was confirmed by K statistics for leaf trait identification and phylogenetic preservation analysis. The trait-related phylogenetic errors were eliminated using phylogenetic independent contrasts (PIC), and PIC was calculated using the “Picante” package and the “Vegan” package in the R software (version 4.1.3, R core team, Vienna, Austria). Averages of LCC, LNC, LPC, SLA, LDMC, and LMC were plotted on a phylogenetic tree to determine their phylogenetic patterns. The binary relationship between leaf traits of various plant species and plant life forms (annuals, perennials, and shrubs) was evaluated using linear regression analysis. Statistical analyses of phylogenetic data were performed using R software (version 4.1.3, R core team, Vienna, Austria), and other relevant statistical analyses were performed using Origin 2018 (Origin Lab, Northampton, WA, USA) and SPSS software (IBM, NY, USA). The graphs were completed by using R software (version 4.1.3, R core team, Vienna, Austria) and Origin software, and the data sorting and statistics were completed by Office software (Microsoft, USA, 2016) and SPSS software.
Results
Species Composition and Soil Properties
A total of 17 plant species belonging to 17 genera and 8 families (Amaranthaceae (7), Asteraceae (3), Poaceae (3), Apocynaceae (1), Fabaceae (1), Nitrariaceae (1), Tamaricaceae (1), Polygonaceae (1)) were recorded. Classification of functional groups of the species was as follows: (I) Annuals (5), perennials (6), and shrubs (6); (II) C3 species (9) and C4 species (8); (III) Legumes (1) and non-legumes (16). Perennials and shrubs were the dominants (Figure 2; Supplementary Table S1).
The soil in the study area was alkaline, and the coefficient of variations (CVs) of STN, SOC, and SMC were all greater than 50% (Table 3).
Patterns of Leaf Traits of Different Functional Groups
The One-way ANOVA analysis showed that the LCC and LNC in perennials and shrubs were higher than those in annuals, and there was no difference in the LPC. The SLA and LDMC in perennials were higher than that in annuals and shrubs (Figure 2; Table 4), and the LMC of annuals and shrubs was higher than that of perennials. The analysis of variance showed that there were significantly differences in the LCC, LNC, LPC, SLA, LDM, and LMC among different life forms (Table 4).
C3 plants had higher LCC, LNC, and LPC than C4 plants, while C4 plants had higher SLA, LDMC, and LMC than C3 plants. The one-way ANOVA analysis showed that there were significant differences in LCC, LNC, SLA, and LMC between C3 and C4 plants, but there was no difference in LPC and LDMC (Table 5). Leguminous plants had higher LCC, LNC, and SLA than non-leguminous plants, while non-leguminous plants had higher LMC than legumes. The ANOVA analysis showed that there were significant differences in LCC and LNC between legumes and non-leguminous plants, but there were no significant difference in LPC, SLA, LDMC, and LMC (Table 6).
Correlations Between Leaf Traits
Pearson correlation analysis showed that LCC was significantly negatively correlated with SLA and LMC. LNC was significantly positively correlated with LPC. LPC was significantly negatively correlated with SLA and LDMC and negatively correlated with LMC. SLA was significantly positively correlated with LDMC. LDMC was significantly negatively correlated with LMC (Table 6).
The PSs of functional traits were detected in all species (Table 7). However, the PSs of all leaf traits were low (K-value < 1, p > 0.05), and that of LCC was the largest (K = 0.73).
In annuals, LCC was negatively correlated with LNC (p < 0.05) and LPC (p < 0.05), and LNC was positively correlated with LPC (p < 0.05). In perennials, LCC was positively correlated with LNC (p < 0.05) and LPC (p < 0.05), and LNC was positively correlated with LPC (p < 0.05). In shrubs, LCC was negatively correlated with LNC (p < 0.05) and LPC (p > 0.05), and LNC was positively correlated with LPC (p > 0.05) (Figures 3, 4).
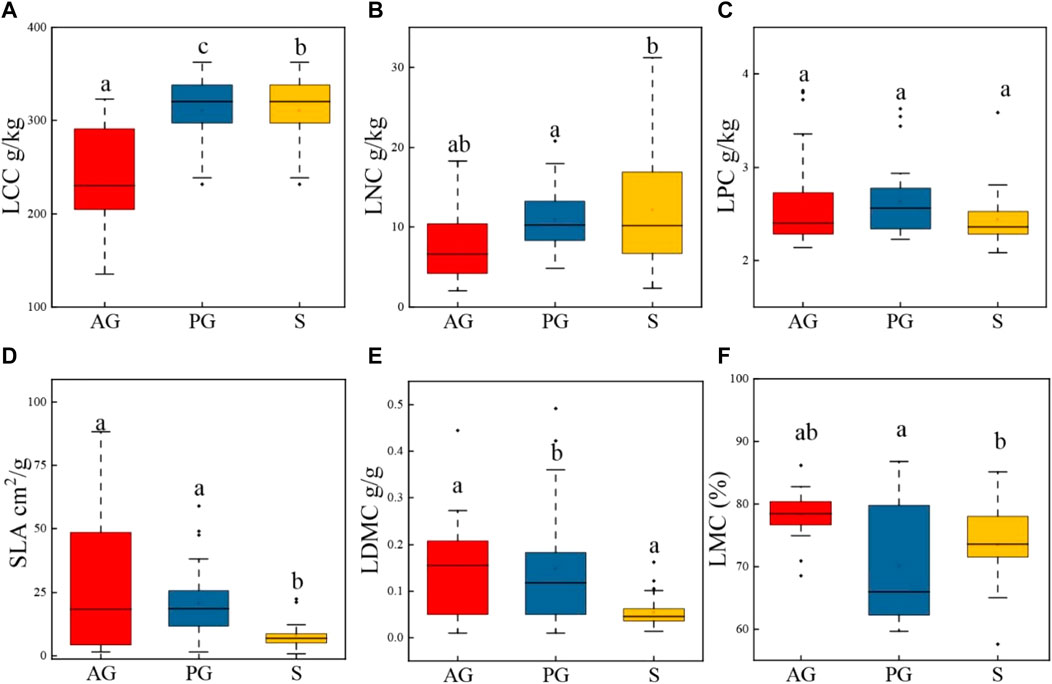
FIGURE 3. Leaf traits for plant species with different life forms (AG, PG, S). (A) Leaf carbon concentration; (B) Leaf nitrogen concentration; (C) Leaf phosphorus concentration; (D) Specific leaf area; (E) Leaf dry matter content; (F) Leaf moisture content.
Principal component analysis (PCA) showed that SLA and LDMC loaded on the PC1, C and LMC loaded on the PC2, and N and P loaded on the PC3. PC1, PC2, and PC3 explained 32.3%, 27.5%, and 19.0% of the total variation, respectively (Figure 5; Supplementary Table S2).
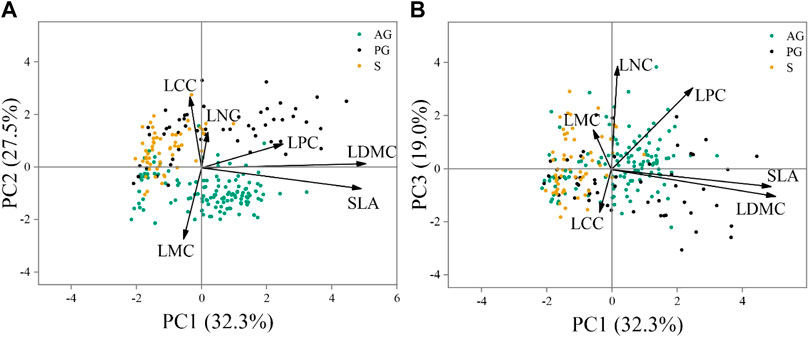
FIGURE 5. The expression of leaf traits on the three axes in principal components analysis (PCA) at the species level (N = 14). (A) Loading values of leaf traits for PC1 and PC2, (B) Loading values of leaf traits for PC 1 and PC3. Different colors show the contribution of each variable.
Relationships Between Leaf Traits and Soil Properties
Only STN and STP were significantly positively correlated with LCC and LNC (Table 8).
Pearson correlation analysis showed that SOC was significantly positively correlated with STP, SAP, SMC, pH, and soil electrical conductivity (SCE). STN was significantly positively correlated with STP and SAP. STP was significantly positively correlated with SAP, SMC, pH, and SCE. SAP was significantly positively correlated with SMC, pH, and SCE. SMC was significantly positively correlated with pH and SCE. pH was significantly positively correlated with SCE (Table 9).
Taxonomy of Leaf Traits at Species and Family Levels
The leaf traits of different species varied greatly (Supplementary Table S3). At the species level, Seriphidium kaschgaricum and Aeluropus pungens had a higher LCC, Kalidium foliatum and Nitraria tangutorum had a higher LNC, Seriphidium kaschgaricum, Suaeda salsa, and Reaumuria alternifolia had a higher LPC. Suaeda salsa and Seriphidium kaschgaricum had a higher SLA, Suaeda salsa and Seriphidium kaschgaricum had a higher LDMC, and Karelinia caspia and Lactuca tatarica had a higher LMC.
One-way analysis of variance showed that the six leaf traits varied widely among the 7 families (Supplementary Table S4). At the family level, Asteraceae had a higher LCC, Poaceae and Nitrariaceae had a higher LNC, and Asteraceae and Tamaricaceae had a higher LPC. Amaranthaceae and Asteraceae had a higher SLA. Asteraceae and Poaceae had a higher LDMC. Amaranthaceae and Nitrariaceae had a higher LMC.
Partitioning of Variance in Leaf Traits
The effects of taxonomy and soil properties on leaf traits were studied using general linear model (GLM). This model explained 13%–55% of the total variation. Among them, taxonomy explained 11%–43% of the variation, and soil properties explained 2.15%–12% of the variation. Therefore, taxonomy could better explain leaf trait variation. This is because taxonomy had a greater impact on LCC, LNC SLA, and LMC, while soil properties explained less variation in the same leaf trait (Table 10).
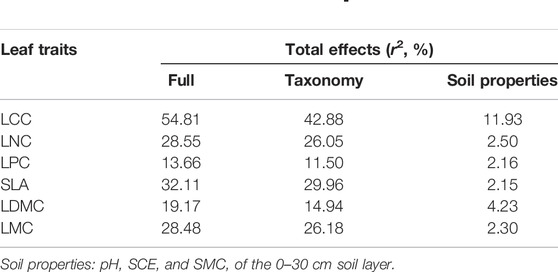
TABLE 10. General linear model (GLM) analysis of the effects of taxonomy and soil properties on leaf traits.
Discussion
Differences in Leaf Traits of Different Functional Groups
Leaf is an important functional organ for plant photosynthesis, and it is also the organ that has the most contact with the surrounding environment. Using the change of leaf traits to study the adaptability of plants to the environment has become one of the important means to study the stress resistance of plants. Studies have found that species coexistence can be better elucidated only when functional trait differences are fully considered at the species level. In this study, leaf traits were investigated for 14 genera (Supplementary Table S1). The average LCC of the 14 plant genera (Table 4) was lower than that of plants in China (Tang et al., 2018) and herbs in Yucatan, Mexico (Abdala-Roberts et al., 2018). The average LPC was significantly lower than that of global plants (Reich and Oleksyn, 2004) and plants in the terrestrial ecosystems in China (Tang et al., 2018). This indicates that the desert plants in the study area have a high degree of adaptation and tolerance to arid and barren environments. Leaf nutrient concentration plays a fundamental role in plant growth and physiological processes. During vigorous growth, plants may allocate most nutrients to leaves to improve the dry matter accumulation in the aboveground organs (Fernández-Martínez et al., 2019).
In terms of life form, perennials had the highest LCC, SLA, and LDMC, followed by shrubs and annuals. The higher LCC of perennials may be due to the difference in the ability of plants with different life forms to obtain light sources. Plants accumulate C through leaf photosynthesis, while perennials have a larger SLA (35.65 cm2 g−1) and higher photosynthetic capacity than annuals and shrubs (Chen et al., 2016). Díaz (Perez-Harguindeguy et al., 2016) found that plants with high SLA had higher photosynthetic capacity and growth rate, while plants with low SLA could better adapt to the water and nutrient insufficient environments. In this study, compared with low-height shrubs (Apocynum venetum and Alhagi sparsifolia), perennials (mainly Karelinia caspia and Phragmites australis) in the study area had stronger photosynthetic capacity and could quickly capture external resources, while slow-growing annuals had lower SLA value and tended to preserve internal resources better (Grassein et al., 2010). Similar to SLA, the analysis of LDMC also indicates the resource utilization strategies of plants at different heights with different life forms (Guo et al., 2003).
In this study, annuals had the highest LMC, followed by shrubs and perennials. In arid environments, water is the main limiting factor. As an “opportunist,” annuals can complete their seed germination and maintain their life cycle after spring rains (Zhang et al., 2006). The growth of annuals mainly depends on the environmental resources, while perennials have certain anti-interference and self-recovery capabilities (Alhamad and Alrababah, 2013). The results of this study showed that compared with plants in other types of habitats, desert plants in the study area had relatively low LPC and relatively high LNC. This indicates that P is the main limiting factor for the plants in the study area. The higher LNC may be due to that soil N is significantly positively correlated with leaf N. In this study, STN was higher than STP (Table 3). Previous studies have shown that the LPC in desert plants is higher in arid environment. Because P is very important for plants to maintain water use efficiency and growth, desert plants need to allocate a large amount of P-rich elements to meet their growth demands (Sardans and Peñuelas, 2007). This is contrary to our results. It may be due to the fact that under extreme drought conditions, plants require a large amount of N to enhance the activities of enzymes related to drought tolerance of plants to adapt to the drought environment and maintain normal growth and development (Yuan and Tang, 2010).
In the study area, C3 plants had higher LCC, LNC, and LPC than C4 plants, and C4 plants had higher SLA and LDMC than C3 plants (Table 5). Under the same environmental conditions, C3 plants with lower photosynthesis rate need more C, N, and P to maintain photosynthesis to obtain energy, which reflects that plants adapt to environmental changes by increasing or reducing nutrient cycling (Guo et al., 2009). Compared with C3 plants, C4 plants had a larger SLA and are easier to receive light to promote photosynthesis. SLA reflects the ability of plants to acquire, preserve, and use resources. A larger SLA is more conducive to the capture and utilization of light by plants (Wright et al., 2005). The LDMC of C3 plants was lower than that of C4 plants in this study. The leaf dry matter reflects the plant’s ability to retain nutrients. The larger the LDMC, the greater the leaf tissue density, the stronger the resistance and tolerance of plants (Sun et al., 2020). Previous research has shown that C4 plants are suitable to grow in the environment of high temperature and drought (Pau et al., 2013). To enhance the drought tolerance, C4 plants resist the adverse environments by accumulating more dry matter, leading to the higher LDMC compared with C3 plants.
This study showed the LCC, LNC, and SLA of legumes were higher than those of non-leguminous plants, and there was no significant difference in LPC and LMC (Table 5). The STP in desert environment was low in this study, which was insufficient for plant growth, while STN was sufficient for the growth of leguminous shrubs in this study. Legumes use soil nitrogen-fixing symbionts to exchange C into N (Rogers et al., 2009), resulting in higher LCC and LNC in legumes than in non-leguminous plants. Liu et al. showed that in addition to nitrogen fixation in legumes, plant acid phosphatase (APASE) can hydrolyze organic phosphates, thus improving P absorption by legumes (Liu et al., 2018). However, we did not find higher LPC in legumes in this study. This may be due to the different adaptation strategies of desert plants. The other reason may be that the relatively low soil P in China inhibit the uptake of P by legumes. When faced with P stress, legume nodules can redistribute P from root to nodules through internal P cycling and P retention mechanisms, rather than taking up P directly from soil (Vardien et al., 2016).
Relationship Between Leaf Traits, Phylogenetic Signals, and Soil Properties
PIC analysis showed that K < 1. This indicates that leaf traits of the desert plants in the study area were phylogenetically conserved. Whether or not phylogeny is considered, the relationship between leaf traits may be related to the chemical properties and biochemical functions (Zhang et al., 2012). It was found that the phylogenetic signal (PS) of C, N, P, SLA, LDMC, and LMC for all species was not significant. Therefore, the phylogeny may have a great influence on the functional traits (C, N, P, SLA, LDMC, and LMC), and these traits may be more phylogenetically conserved. The PS of all leaf traits were low (K < 1) in this study (Table 7). It indicates that leaf traits are predominantly influenced by climatic factors (Zhang et al., 2012). LDMC showed a significant negative correlation with LMC (Table 6). This reflects the convergence in the leaf traits of desert plants related to nutrient absorption and light capture capacity. Therefore, these leaf traits have evolutionary changes. The SLA and LDMC loaded on PC1 are of great importance for plant nutrient retention and photosynthetic efficiency (Tang et al., 2018). The LCC, LMC, LNC, and LPC loaded on PCA 2 and 3 are of great importance for plant growth, physiological processes, photosynthesis, enzyme activity, and water use (Tang et al., 2018; Ratzmann et al., 2019).
SOC, STN, and STP reflects soil fertility and productivity. Among them, SOC directly affect the productivity of ecosystems (Ouyang et al., 2017), and STN and STP are necessary for plant growth and directly affect photosynthesis and the processes associated with productivity (Liu et al., 2013). In this study, correlation analysis showed that STN and STP were significantly positively correlated with LCC and LNC (Table 8). This indicates that when STN and STP increase, the C and N in leaf may be increased. STN can significantly promote the aboveground growth of desert plants. Liu et al. (Liu et al., 2014) found that in a certain range, the higher the STN, the higher the LCC of plants. This is consistent with our study results. SAP plays an important role in promoting plant growth and development (Du et al., 2009). In this study, SAP had a significant positive correlation with SMC and pH. However, the soils in the study area are mostly calcium carbonate-rich soils, and the soil particles are coarse, leading to the vast majority of P being fixed in the soil and low levels of SAP (Ja and Li, 2011). Wang et al. (Wang, 2010) investigated soils in northern China and found that in areas with generally low soil P concentrations, plants had higher P use efficiency. Besides, study has shown that the pH value of 7.76–8.89 can improve the bioavailability of soil P (Zhou et al., 2011). The soil pH in the study area is 7.76–8.39, which promotes the absorption of P by desert plants.
Partitioning of Variance of Leaf Traits by Taxonomy
Our results showed that there were significant differences in leaf traits at species (Supplementary Table S2) and family levels (Table 10) (p < 0.05). Taxonomy and soil properties jointly explained 19.17%–54.81% of the variation of leaf traits. Soil properties explained 2.15%–12% of the variation (Table 10). Besides, it was also found that taxonomy and plant phylogeny greatly affected plant leaf traits, resulting in great differences in the degree of variation between leaf traits. Due to the different survival strategies of plants caused by factors such as arid climate, less precipitation, and barren soil, the selective absorption of nutrients by plants leads to great variation of leaf traits, reducing the competition for resources and achieving convergent development (Zhang et al., 2012).
Conclusion
In the Ebinur Lake Basin, except for C and LMC, leaf traits (N, P, SLA, and LDMC) show a convergent pattern for all plant life forms. The plants have evolved and developed different survival strategies to adapt to the arid environment. The differences in leaf traits reflect the differences of functional groups. Therefore, the plants in this study exhibit unique intrinsic characteristics. In addition, the leaf traits of desert plants tend to be consistent in adapting to the environment of the Ebinur Lake Basin, and the convergence pattern is not phylogenetically specific. All leaf traits are phylogenetically conserved (K < 1, p > 0.05). In addition, the convergence in leaf traits of the desert plants in Ebinur Lake Basin is most likely due to the environmental factors. This research may contribute to understanding the convergent adaptations and patterns of desert plants in arid regions.
Data Availability Statement
The original contributions presented in the study are included in the article/Supplementary Material, further inquiries can be directed to the corresponding author.
Author Contributions
ZL provided field data for the paper; SN wrote the paper, and JL and LG contributed to writing—review and editing. All authors have read and agreed to the published version of the manuscript.
Funding
This research was jointly funded by the National Natural Science Foundation of China (42171026), Xinjiang Uygur Autonomous Region innovation environment Construction special project—Science and technology innovation base construction project (PT2107). Thanks for the joint support of the National Natural Science Foundation of China.
Conflict of Interest
The authors declare that the research was conducted in the absence of any commercial or financial relationships that could be construed as a potential conflict of interest.
Publisher’s Note
All claims expressed in this article are solely those of the authors and do not necessarily represent those of their affiliated organizations, or those of the publisher, the editors and the reviewers. Any product that may be evaluated in this article, or claim that may be made by its manufacturer, is not guaranteed or endorsed by the publisher.
Acknowledgments
Thank my tutor professor Lv for the technical support and financial support for my thesis, and thank my senior brothers and sisters for their selfless help, which has improved my thesis writing level.
Supplementary Material
The Supplementary Material for this article can be found online at: https://www.frontiersin.org/articles/10.3389/fenvs.2022.927572/full#supplementary-material
References
Abdala-Roberts, L., Covelo, F., Parra-Tabla, V., Terán, J. C. B. M. Y., Mooney, K. A., and Moreira, X. (2018). Intra-Specific Latitudinal Clines in Leaf Carbon, Nitrogen, and Phosphorus and Their Underlying Abiotic Correlates in Ruellia Nudiflora. Sci. Rep. 8 (1), 596. doi:10.1038/s41598-017-18875-w
Ackerly, D. D., and Cornwell, W. K. (2007). A Trait-Based Approach to Community Assembly: Partitioning of Species Trait Values into within- and Among-Community Components. Ecol. Lett. 10, 135–145. doi:10.1111/j.1461-0248.2006.01006.x
Alhamad, M., and Alrababah, M. (2013). The Impacts of Biologically Induced Micro-environments on Biodiversity in a Dry Mediterranean Grassland. Trans. Botanical Soc. Edinb. 6 (2), 10. doi:10.1080/17550874.2013.773105
Bao, L., and Liu, Y. L. (2009). Comparison of Leaf Functional Traits in Different Forest Communities in M T. Dongling of Beijing. Acta Ecol. Sin. 29 (7), 3692–3703. doi:10.3321/j.issn:1000-0933.2009.07.030
Botta-Dukát, Z., and Czúcz, B. (2016). Testing the Ability of Functional Diversity Indices to Detect Trait Convergence and Divergence Using Individual-Based Simulation. Methods Ecol. Evol. 7, 114–126. doi:10.1111/2041-210x.12450
Chen, C., Wang, G. J., Zhao, Y., Zhou, G. X., Li, L., and Gao, J. Q. (2016). Seasonal Dynamics and Allometric Growth Relationships of C, N, and P Stoichiometry in the Organs of Cunninghamia Lanceolata from Huitong. Acta Ecol. Sin. 36 (23), 7614–7623. doi:10.5846/stxb201512142500
Coyle, J. R., Halliday, F. W., Lopez, B. E., Palmquist, K. A., Wilfahrt, P. A., and Hurlbert, A. H. (2014). Using Trait and Phylogenetic Diversity to Evaluate the Generality of the Stress-Dominance Hypothesis in Eastern North American Tree Communities. Ecography 37, 814–826. doi:10.1111/ecog.00473
Crosby, N. T. (1968). Determination of Ammonia by the Nessler Method in Waters Containing Hydrazine. Analyst 93 (1107), 406. doi:10.1039/an9689300406
Cui, H., Vitousek, P. M., Reed, S. C., Sun, W., Sokoya, B., Bamigboye, A. R., et al. (2022). Environmental Filtering Controls Soil Biodiversity in Wet Tropical Ecosystems. Soil Biol. Biochem. 166, 108571. (prepublish). doi:10.1016/J.SOILBIO.2022.108571
Du, Z. J., Chen, X. M., Zhang, J. B., Deng, J. B., Wang, B. R., and Huang, J. (2009). Time-spatial Variability and Influenced Factors of Available Phosphorus under Different Fertilizer Treatments in Dry Land of Red Soil. J. Nanjing Agric. Univ. 32 (4), 112–115. doi:10.3321/j.issn:1000-2030.2009.04.021
Fernández-Martínez, M., Pearse, I., Sardans, J., Sayol, F., Koenig, W. D., LaMontagne, J. M., et al. (2019). Nutrient Scarcity as a Selective Pressure for Mast Seeding. Nat. Plants 5 (12), 1222–1228. doi:10.1038/s41477-019-0549-y
Givnish, T. (2017). Convergent Evolution, Adaptive Radiation, and Species Diversification in Plants, 3. Amsterdam, Netherlands: Elsevier.
Grassein, F., Till-Bottraud, I., and Lavorel, S. (2010). Plant Resource-Use Strategies: the Importance of Phenotypic Plasticity in Response to a Productivity Gradient for Two Subalpine Species. Ann. Bot. 106 (4), 637–645. doi:10.1093/aob/mcq154
Griffiths, H., and Males, J. (2017). Succulent Plants. Curr. Biol. 27 (17), R890. doi:10.1016/j.cub.2017.03.021
Guo, W. H., Li, B., Huang, Y. M., Zhao, H. X., and Zhang, X. S. (2003). Effects of Different Water Stresses on Eco-Physiological Characteristics of Hippophae Rhamnoides Seedlings. Acta Bot. Sin. 45 (10), 1238–1244. doi:10.1023/A:1022289509702
Guo, W. D., Sang, D., Zhen, J. S., Liao, H. B., and Chen, W. R. (2009). Effects of Nitrogen Deficiency on the Gas Exchange, Chlorophyll Fluorescence and Chloroplast Ultrastructure in Fingered Citron. J. Zhejiang Univ. Agric. Life Sci. 35 (3), 307–314. doi:10.3785/j.issn.1008-9209.2009.03.012
Ja, X. Y., and Li, J. M. (2011). Study on Soil Phosphorus Availability and its Relation to the Soil Properties in 14 Soils from Different Sites in China. Soil Fertil. Sci. China 6, 76–82. doi:10.11838/sfsc.20110615
Kattge, J., Diaz, S., Lavorel, S., Prentice, I. C., Leadley, P., Bönisch, G., et al. (2011). TRY–a Global Database of Plant Traits. Glob. Change Biol. 17, 2905–2935. doi:10.1111/j.1365-2486.2011.02451.x
Liu, Z.-P., Shao, M.-A., and Wang, Y.-Q. (2013). Spatial Patterns of Soil Total Nitrogen and Soil Total Phosphorus across the Entire Loess Plateau Region of China. Geoderma 197-198, 67–78. doi:10.1016/j.geoderma.2012.12.011
Liu, A., Contador, C. A., Fan, K., and Lam, H.-M. (2018). Interaction and Regulation of Carbon, Nitrogen, and Phosphorus Metabolisms in Root Nodules of Legumes. Front. Plant Sci. 9, 1860. doi:10.3389/fpls.2018.01860
Liu, H., Guo, X. M., Tu, S. P. X, M., Li, X. M., and Hu, D. N. (2014). The Coupling Effects of Water and Fertilizer on the Soil Total Nitrogen and Yield of Camellia Oleifera Forest. Chin. J. Soil Sci. 45 (4), 897–902. doi:10.19336/j.cnki.trtb.2014.04.021
Liu, H. W., Wang, W., Zou, J., and Tao, J. P. (2014). Leaf Traits of Main Plants on Limestone Area in Zhong Liang Mountain. J. Southwest China Normal Univ. Nat. Sci. Ed. 39, 50–55. doi:10.13718/j.cnki.xsxb.2014.09.010
Liu, K. P. (2017). Evaluation of Uncertainty of Soil Organic Matter Measured by Potassium Dichromate Outside Heating Method. Constr. Des. Eng. 16, 101–102. doi:10.13616/j.cnki.gcjsysj.2017.08.146
Losos, J. B. (2011). Convergence, Adaptation, and Constraint. Evolution 65, 1827–1840. doi:10.1111/j.1558-5646.2011.01289.x
Lu, C. (2009). Comparative Study on Two Methods for Determination of Total Phosphorus in Wetland Plants. Acta Agric. Jiangxi 21 (8), 142–144.
McCoy-Sulentic, M. E., Kolb, T. E., Merritt, D. M., Palmquist, E. C., Ralston, B. E., and Sarr, D. A. (2017). Variation in Species-Level Plant Functional Traits over Wetland Indicator Status Categories. Ecol. Evol. 7, 3732–3744. doi:10.1002/ece3.2975
Murphy, J., and Riley, J. P. (1962). A Modified Single Solution Method for the Determination of Phosphate in Natural Waters. Anal. Chim. Acta 27, 31–36. doi:10.1016/s0003-2670(00)88444-5
Ouyang, S., Xiang, W., Gou, M., Lei, P., Chen, L., and Deng, X. (2017). Variations in Soil Carbon, Nitrogen, Phosphorus and Stoichiometry along Forest Succession in Southern China. Biogeosci. Discuss. 1, 1–27. doi:10.5194/bg-2017-408
Pau, S., Edwards, E. J., and Still, C. J. (2013). Improving Our Understanding of Environmental Controls on the Distribution of C3 and C4 Grasses. Glob. Change Biol. 19 (1), 184–196. doi:10.1111/gcb.12037
Perez-Harguindeguy, N., Diaz, S., Garnier, E., Lavorel, S., Poorter, H., Jaureguiberry, P., et al. (2016). New Handbook for Standardised Measurement of Plant Functional Traits Worldwide. Aust. J. Bot. 64 (8), 715. doi:10.1071/bt12225_co
Ratzmann, G., Zakharova, L., and Tietjen, B. (2019). Optimal Leaf Water Status Regulation of Plants in Drylands. Sci. Rep. 9 (1), 3768. doi:10.1038/s41598-019-40448-2
Reich, P. B., and Oleksyn, J. (2004). Global Patterns of Plant Leaf N and P in Relation to Temperature and Latitude. Proc. Natl. Acad. Sci. U.S.A. 101 (30), 11001–11006. doi:10.1073/pnas.0403588101
Reich, P. B., Walters, M. B., Ellsworth, D. S., Vose, J. M., Volin, J. C., Gresham, C., et al. (1998). Relationships of Leaf Dark Respiration to Leaf Nitrogen, Specific Leaf Area and Leaf Life-Span: Atest across Biomes and Functional Group. Oecologia 114, 471–482. doi:10.1007/s004420050471
Rogers, A., Ainsworth, E. A., and Leakey, A. D. B. (2009). Will Elevated Carbon Dioxide Concentration Amplify the Benefits of Nitrogen Fixation in Legumes? Plant Physiol. 151, 1009–1016. doi:10.1104/pp.109.144113
Saéz-Plaza, P., Navas, M. J., Wybraniec, S., Michalowski, T. T., and Asuero, A. G. (2013). An Overview of the Kjeldahl Method of Nitrogen Determination. Part II. Sample Preparation, Working Scale, Instrumental Finish, and Quality Control. Crit. Rev. Anal. Chem. 43 (4), 224–272. doi:10.1080/10408347.2012.751787
Sardans, J., and Peñuelas, J. (2007). Drought Changes Phosphorus and Potassium Accumulation Patterns in an Evergreen Mediterranean Forest. Funct. Ecol. 21 (2), 191–201. doi:10.1111/j.1365-2435.2007.01247.x
Shipley, B., De Bello, F., Cornelissen, J. H. C., Laliberté, E., Laughlin, D. C., and Reich, P. B. (2016). Reinforcing Loose Foundation Stones in Trait-Based Plant Ecology. Oecologia 180, 923–931. doi:10.1007/s00442-016-3549-x
Sun, Y. M., Tian, Q., Guo, A. X., Zuo, X. R., Lv, P., and Zhang, S. X. (2020). Effects of Water and Nitrogen Changes on Vegetation Characteristics and Leaf Traits in Horqin Sandy Land, Northern China. J. Desert Res. 40 (6), 223–232. doi:10.7522/j.issn.1000-694X.2020.00087
Tang, Z., Xu, W., Zhou, G., Bai, Y., Li, J., Tang, X., et al. (2018). Patterns of Plant Carbon, Nitrogen, and Phosphorus Concentration in Relation to Productivity in China's Terrestrial Ecosystems. Proc. Natl. Acad. Sci. U. S. A. 115 (16), 4033–4038. doi:10.1073/pnas.1700295114
Twohey, R. J., Roberts, L. M., and Studer, A. J. (2019). Leaf Stable Carbon Isotope Composition Reflects Transpiration Efficiency in Zea mays. Plant J. 97 (3), 475–484. doi:10.1111/tpj.14135
Vardien, W., Steenkamp, E. T., and Valentine, A. J. (2016). Legume Nodules from Nutrient-Poor Soils Exhibit High Plasticity of Cellular Phosphorus Recycling and Conservation during Variable Phosphorus Supply. J. Plant Physiol. 191, 73–81. doi:10.1016/j.jplph.2015.12.002
Wang, L., Wang, L., He, W., An, L., and Xu, S. (2017). Nutrient Resorption or Accumulation of Desert Plants with Contrasting Sodium Regulation Strategies. Sci. Rep. 7, 17035. doi:10.1038/s41598-017-17368-0
Wang, Y. G. (2010). Study on Correlation of Measured Values of Soil Available Phosphorus and Plant Uptake in Northern China. linfen: Shanxi Normal University.
Westoby, M., and Wright, I. J. (2006). Land-plant Ecology on the Basis of Functional Traits. Trends Ecol. Evol. 21, 261–268. doi:10.1016/j.tree.2006.02.004
Wright, I. J., Reich, P. B., Cornelissen, J. H. C., Falster, D. S., Garnier, E., Hikosaka, K., et al. (2005). Assessing the Generality of Global Leaf Trait Relationships. New Phytol. 166, 485–496. doi:10.1111/j.1469-8137.2005.01349.x
Xu, X. J., Li, F. Q., Deng, J., Fan, X., Li, R. D., Zhang, Y. B., et al. (2020). Influence Factors of Determination for Soil Available Phosphorus by Olsen Method in Sugarcane Planting Region. Sugar Crops China 42 (3), 37–42. doi:10.13570/j.cnki.scc.2020.03.007
Yuan, S., and Tang, H. (2010). Research Advances in the Eco-Physiological Characteristics of Ephemerals Adaptation to Habitats. Acta Pratacult. Sin. 19, 240–247.
Zhang, T., Tia, C. Y., Sun, Y., and Feng, G. (2006). Soil Seed Banks of Ephemerals in the Gurbantunggut Desert Area. Arid. Land Geogr. 5, 675–681. doi:10.13826/j.cnki.cn65-1103/x.2006.05.010
Zhang, S.-B., Zhang, J.-L., Slik, J. W. F., and Cao, K.-F. (2012). Leaf Element Concentrations of Terrestrial Plants across China Are Influenced by Taxonomy and the Environment. Glob. Ecol. Biogeogr. 21, 809–818. doi:10.1111/j.1466-8238.2011.00729.x
Keywords: convergence, plant functional traits, desert plants, phylogenetic signals, Ebinur Lake Basin
Citation: Nurbolat S, Guanghui L, Lamei J and Lei Z (2022) Convergent Variation in the Leaf Traits of Desert Plants in the Ebinur Lake Basin. Front. Environ. Sci. 10:927572. doi: 10.3389/fenvs.2022.927572
Received: 24 April 2022; Accepted: 06 May 2022;
Published: 03 June 2022.
Edited by:
Hongbo Ling, Xinjiang Institute of Ecology and Geography (CAS), ChinaReviewed by:
Jingzhe Wang, Shenzhen Polytechnic, ChinaKaihui Li, Xinjiang Institute of Ecology and Geography (CAS), China
Copyright © 2022 Nurbolat, Guanghui, Lamei and Lei. This is an open-access article distributed under the terms of the Creative Commons Attribution License (CC BY). The use, distribution or reproduction in other forums is permitted, provided the original author(s) and the copyright owner(s) are credited and that the original publication in this journal is cited, in accordance with accepted academic practice. No use, distribution or reproduction is permitted which does not comply with these terms.
*Correspondence: Lv Guanghui, guanghui_xju@sina.com