- 1Department of Bioengineering, School of Engineering, Vels Institute of Science, Technology & Advanced Studies (VISTAS), Chennai, India
- 2Department of Chemical Engineering, Alagappa College of Technology, Anna University, Chennai, India
This study examined the aquatic toxicity of dichloromethane (DCM) on Oreochromis mossambicus. Along with aquatic toxicity studies, in silico research was also conducted to identify ways of improving the fish’s immune system, which may help to fight the oxidative stress and neurotoxic effects of DCM. The activities of glutathione S-transferase and acetylcholinesterase enzymes were studied in samples from the brains, muscles, livers, and gills of fish treated with different concentrations of DCM (730, 760, and 790 ppm). Histopathological and hematological studies were also completed at various concentrations of DCM. Molecular docking studies of the bioactive compounds of Aloe vera against interleukin-1β (IL-1β) were conducted, and drug properties were also analyzed. The lethal concentration (LC50) of DCM in the fish was found to be 760 ppm. The hematological study revealed that tissues exposed to 760 ppm of DCM had an elevated leukocyte count, high amounts of hemoglobin, and very low platelet counts. The liver histopathological study identified cellular alterations such as necrosis, and the gills showed lamellar fusion and congestion. The compound sitosterol showed strong binding energy (-12.398 kcal/mol) against IL-1β, followed by squalene (−12.157 kcal/mol). Pharmacokinetic properties were also analyzed, with satisfactory results. Thus, the hematological and histopathological studies reveal that DCM has a potential ability to induce oxidative stress and neurotoxic effects. Hence, the phytochemicals of Aloe vera can improve the immune system to fight against the neurotoxicity of DCM, which can be further validated by in vitro and in vivo studies.
Introduction
Dichloromethane (DCM), also referred to as methylene chloride, is an organic chlorinated hydrocarbon solvent (Jaffe et al., 2019) used for diverse applications in various industries and is a volatile solvent at room temperature. DCM is extensively used in the industrial production of paints, varnishes, aerosol propellants, and refrigerants, and for extraction, manufacturing, paint stripping, degreasing, and many other industrial processes; thus, the danger of its leakage into the aquatic atmosphere is a plausible factor (Bonfiglioli et al., 2014). It is known to be soluble in compounds such as methanol, ethanol, ether, and other organic solvents, and interactions with DCM in organic solvents might increase or decrease its toxic effects. Furthermore, DCM is a stable compound (De Rooij et al., 2004), and its carcinogenic properties in mice have been previously studied (Watanabe et al., 2007). The effects of trichloroethylene, tetrachloroethylene, trichloroethane, tetrachloromethane, and chloroform in various marine organisms have been studied (Pan and Dutta, 1998), but the toxicity effects of DCM have rarely been studied in water bodies. Many studies have considered the entire aquatic system on a large scale. However, data on DCM’s acute toxicities have rarely been analyzed (Bos et al., 2006) because DCM is sparingly soluble in water and is the least toxic of all the chloromethanes (Watanabe et al., 2007; Kim and Carlson, 1986). Several studies have reported the effects of DCM exposure on mental consciousness (Mahmud and Kales, 1999) and on the inhibition of acetylcholine neurotransmitters in industrial settings (Pacheco et al., 2016). The solvent’s harmful effects can be direct or indirect, as with the release of carbon monoxide, which is a by-product of this solvent (Wirkner et al., 1997). Other possible ill effects could be due to its direct effects on the central nervous system during its oxidation to phosgene when exposed to flame (Goullé et al., 1999). These compounds are believed to form complexes with organic compounds, leading to the malfunction of affected cells. To examine the functional grade of the fish exposed to toxicants, blood parameters like hematocrit, red blood cells (RBCs), white blood cells (WBCs), and hemoglobin (Hb) serve as pathophysiological indicators of pollution in aquatic bodies (Ahmad et al., 2015). On the whole, Dichloromethane produces fatal effects in humans and other aquatic organisms at high concentrations (Raphael et al., 2002; Fechner et al., 2001; Kobayashi et al., 2008; Ball et al., 2018). In addition, there are no ongoing monitoring programs for volatile organic compounds (VOCs), in contrast to other organic pollutants such as PCBs (Sweeney et al., 2008; Toccalino et al., 2006). The present study aims to evaluate the toxic effects of DCM on Oreochromis mossambicus, which was selected for this study due to its sturdiness and availability throughout the year. In the tissues that were exposed to 760 ppm DCM, total WBC count, Hb, and packed cell volume (PCV) were higher than at 730 ppm but less than at 790 ppm of DCM, while platelet counts in tissues exposed to 760 ppm of DCM was less than in the control and in 790 ppm-exposed tissues. MCV for the tissues exposed to 760 ppm of DCM concentration was higher than in the control and in 730 ppm exposure to DCM. GST and acetylcholinesterase (AChE) activities were also studied in the exposed tissues.
The interleukin family of cytokines produces immune responses and is often involved in regulating inflammation against microbial infection, tissue injury, and immunological reactions (Liang et al., 2016). Interleukin 1 beta (IL-1β) is a pro-inflammatory cytokine that is commonly found in the macrophages, natural killer cells, monocytes, and neutrophils of animal blood. Generally, IL-1β helps leukocytes to pass through blood vessel walls to sites of infection and causes fever by affecting areas of the brain that control body temperature. In fish, IL-1β increases the number of phagocytes migrating into the peritoneal cavity, phagocytic activity, and lysozyme activity in macrophages. There is also evidence of IL-1β inducing the other pro-inflammatory cytokines such as tumor necrosis factor alpha, IL-6, IL-18, IL-34, IL-8, and cyclooxygenase-2 (Zou and Secombes, 2016). Hence, IL-1β boosts the immune system; for this reason, it was taken as the target for the molecular docking studies (PDB ID:4DEP).
Aloe vera is a succulent, evergreen perennial plant that originated in the Arabian Peninsula and is now grown in tropical, semi-tropical, and arid climatic regions around the world. It is a short-stemmed or stemless plant growing between 60 and 100 cm tall. It forms a symbiotic relationship with arbuscular mycorrhiza, a type of fungus which penetrates its roots to better access soil nutrients (Oliver Grandman, 2012). Aloe vera contains numerous vitamins, minerals, enzymes, and natural bioactive compounds; therefore, it has many medicinal properties which include emollient, purgative, antimicrobial, anti-inflammatory, antioxidant, aphrodisiac, anti-helminthic, antifungal, and antiseptic properties. Aloe vera can be used as an immune-boosting medicinal herb, as is rich in immune-boosting polysaccharides; it helps to slow inflammation and to reorder the immune response to prevent adverse health conditions (Sahu et al., 2013). Therefore, this study docked the bioactive compounds of Aloe vera against IL-1β, which regulates the inflammation process and improves the immune system of Oreochromis mossambicus, helping the immune cells to fight the side effects caused by DCM. However, the study of the DCM-treated fish for certain parameters is very limited. Therefore, the aim of the present investigation was to assess hematological changes in Oreochromis mossambicus following the exposure and subsequent withdrawal of DCM. Changes in these parameters can provide useful information about environmental conditions and risk assessment for aquatic organisms.
Materials and Methods
Ethics Statement
The study protocol was approved by the Biosafety, Animal Use and Ethics Committee of the Faculty of Veterinary Medicine, Vels University, India. Informed consent was obtained verbally from fish farmers before they participated in the study.
Rearing of Fish
Oreochromis mossambicus specimens were obtained from the Central Institute of Brackish Water Aquaculture, Muttukadu, Chennai. The fish weighed between 20 and 23 g, and their total length ranged from 10 to 12 cm. The selected healthy fish were used for experimental purposes, and no ethical approval was required for the experiment.
Chemicals and Glassware
Merck-grade chemicals were used. The DCM (99.5%), gas chromatography assay, and methanol were from Southern India Scientific Corporation (SISCO).
Fish Acclimatization
The selected healthy fish were transferred into 75 cm × 29 cm × 40 cm glass aquaria filled with 25 L brackish water. Six separate aquaria were used, and ten fishes were introduced into each. The fishes were acclimatized to the laboratory conditions for a one-week period; they received a continuous supply of oxygen and were fed regularly (every 12 h) during the acclimatization period. The tanks were cleaned periodically to avoid the accumulation of dirt and fecal matter, and dead fishes were removed to avoid pseudoreplication.
Treatment with Dichloromethane
After acclimatization, fishes were divided into four experimental groups (n = 10) and a control group (n = 10) in static chambers (25 L). Experimental groups were treated with varying concentrations of DCM (730, 760, and 790 ppm), and a control tank contained a mixture of water and methanol. Methanol was used as a binder for each of the DCM concentrations and was prepared by adding 5 ml to each concentration. This was done to increase the dissolution rate of the DCM in water to extend its availability in aqueous conditions. Observations were noted carefully, and the activities of the fish were also constantly monitored. During the treatment period, water was renewed daily, maintaining the same concentrations of VOCs, while the dead fishes in each tank were counted and removed daily. The treatment was terminated at 96 h and mortality was observed until that point. The LC50 was calculated, based on the data obtained, by regression probit analysis (M. Ramesh and M. Sarvanan, 2008). To ensure that the methanol used in the sample preparations did not affect the fish, the control tank with 10 fish was treated with 5 ml of methanol and renewed daily.
Tissue Samples
After 96 h, living fishes from each concentration were sacrificed and dissected. Their gills, livers, muscles, and brains were washed in ice-cold (0.5%) NaCl solution, blotted, weighed, and stored at -80°C until analysis. Tissues (40 mg each) were homogenized in phosphate buffer (0.1 M, pH 7.0 for AChE; 0.3 M, pH 6.5 for GST) using a mortar and pestle and then centrifuged at 10,000 rpm for 20 min at 4°C. Supernatants of tissue homogenates were used to determine the AChE and GST activities.
Sample Preparation for Histopathological Sectioning
The frozen muscles, gills, and liver tissue samples of treated (760 ppm) and control fishes were weighed (20 mg), stored in 10% formalin, and sent for analysis.
Measurement of Enzyme Activities
AChE activity was determined by the method of Ellman et al., 1961. Enzyme activity was calorimetrically determined by measuring the increase in absorbance at 412 nm in the presence of 2.6 ml of phosphate buffer (0.1 M, pH-7.0), 100 μL of 5,5’-dithiobis-2-dinitrobenzoic acid (DTNB), 400 μL of tissue homogenate, and 20 μL of acetylcholine iodide. The enzymatic reaction was quantified against the control for activity measurement, and the rate of activity was expressed as nmole/min/mg protein of tissue.
GST activity was measured according to Shah SL, 2006 using 1-chloro-2, 4-dinitrobenzene (CDNB) as the substrate. The assay mixture contained 1 ml of 0.3 M phosphate buffer at pH 6.5, 200 μL of 30 mM CDNB, 100 μL of 30 mM reduced GSH, 100 μL of tissue homogenate, and 1.5 ml of water. An increase in absorbance at 340 nm against the control showed the formation of adduct S-2 and 4-dinitrophenyl glutathione. The molar extinction coefficient used for CDNB was 9.6 Mm−1cm−1, and the rate of activity was measured in nmol/min/mg protein of tissue. Using bovine serum albumin as a standard, the protein concentration of tissue homogenates was determined using Lowry’s method.
Molecular Docking Studies
Determination of Bioactive Compounds of Aloe vera Through GC-MS Analysis and Screening of Protein-Ligand Library
The Aloe vera was subjected to gas chromatography and mass spectrometry analysis, which revealed 26 compounds in total (Arunkumar and Muthuselvam, 2009). Of these compounds, only 12 satisfied the Lipinski rule of five, in which the five main parameters are molecular mass (< 500 KDa), hydrogen bond donor (< 5), hydrogen bond acceptor (< 10), log P (< 5), and molecular refractivity (40–130). The ligand structure was retrieved from the PubChem web server (https://pubchem.ncbi.nlm.nih.gov/), which is maintained by the National Center for Biotechnology Information (NCBI) (Nirmala1 et al., 2020). The protein IL-1β was retrieved from the protein data bank (PDB ID: 4DEP) and studied. The heteroatomic molecules, water, and hydrophobic molecules were removed using a PyMol viewer, and a single chain was determined for the molecular docking studies. The ligand binding site was predicted using the CastP web server (http://sts.bioe.uic.edu/castp/index.html?3trg) (Abhishek Biswal et al., 2020).
Molecular Docking Studies and Visualization of the Protein-Ligand Complex
The molecular docking study was done using Autodock4.2 software, which is generally used to study protein-protein and protein-ligand interactions. This software is based on the Lamarckian genetic algorithm. The charges and the polar hydrogens were added, and the grid parameters were set at dimensions X: 126 Å, Y: 96 Å, Z:100 Å, and grid points for the total map were 1244219. The center grid box size was set at dimensions X: 13.556 Å, Y: 13.222 Å, and Z: 7.917 for the IL-1β protein. The default grid spacing was set to be the same for all the proteins sized 0.375 Å. The interaction of the protein with its ligand forms the basis of the drug development process. The binding energy was obtained for each ligand, and the protein-ligand complex was analyzed using Discovery Studio 3.1 (Abhishek Biswal and Pazhamalai, 2020).
Discovery Studio 3.1 is software for visualizing the 2D and 3D conformations of protein-ligand complex. This software visualizes interactions such as van der Waals interactions and conventional hydrogen bonding. It also creates surface images which show the conformation of structure-based ligand-protein docking against the targeted protein. Discovery Studio 3.1 software is maintained by the Dassault system (Abhishek Biswal and Pazhamalai, 2020; Abhishek Biswal et al., 2020)
Prediction of Drug Properties
ADMET refers to the drug properties of a ligand and is an analysis to identify the absorption, distribution, metabolism, excretion, and toxicity levels of the drug. Generally, the ADMET properties help to identify the behavior and characteristics of a drug in animals (Abhishek Biswal and Pazhamalai, 2020). The major parameters to be noted are water solubility, gastrointestinal absorption, penetration of the drug in the blood-brain barrier and central nervous system, and toxicity level in humans and rats. These properties are based on a vector-based algorithm which can easily access datasets of known inhibitors and non-inhibitors as well as substrates and non-substrates. These parameters were analyzed using the SwissADME web server (http://www.swissadme.ch/) (Abhishek Biswal et al., 2020; Nirmala1 et al., 2020).
Statistical Analysis
Statistical analyses were performed using a two-way analysis of variance (ANOVA) at a significant rate. A post-hoc test was conducted using Duncan’s multiple comparison procedure. All the data have been expressed as mean ± SD. All statistics were carried out using SPSS 16.0 version.
Results and Discussion
Water Characteristics
The physicochemical characteristics of water were analyzed by standard methods. The temperature was maintained at 28οC, and the pH was adjusted to 7.3 ± 0.6. The salinity and hardness of the water sample were determined to be 26.1 ± 1.2 ppm and 500 ± 2.4 mg/L, respectively.
Post-Treatment Behavioral Changes
DCM caused fish to exhibit a lack of coordination, such as swirling movements and breathing problems, within a half hour. Another remarkable change was the darkening of the body color and the appearance of green coloration on the gills. Wide opening of the gills and clustering around the aerators indicated breathing difficulties, while the tailfins were streaked with slight pink color. Excessive fecal matter was also released after a period of 24 h, suggesting that the fish have a mechanism to excrete the toxic compound. The number of fishes found dead was recorded, and 10 fishes that were used as controls were alive, which showed that the methanol used in this experiment did not have any effect on fishes. The mortality data for the 96 h period for each concentration is given in Figure 1, and the 96 h median lethal concentration (LC50) was found to be 760 ppm mg/L.
Glutathione S-Transferase Activity
Glutathione S-transferases are a multifaceted family of proteins involved in normal cellular metabolism, xenobiotic detoxification, and excretion of xenobiotics and their metabolites (Clayton and Clayton, 1981). They play important roles in protecting tissues from oxidative stress (Buckley et al., 1976). In the present study, there was increased GST activity in tissues exposed to lower concentrations of DCM (730 ppm), which suggests that the detoxification process was elevated. In contrast, there was a decline in GST activity in tissues exposed to higher concentrations of DCM (760 ppm, 790 ppm), which indicates that there was a decrease in the detoxification process due to over-production of reactive species, leading to depletion of GSH and inactivation of the GST enzyme (Townsend and Tew, 2003; Oh et al., 2002). The tissues that were exposed to 760 ppm showed less GST activity than the control but higher activity than tissues exposed to 790 ppm of DCM. There was a significant decrease in GST activity in tissues exposed to 790 ppm and a significant increase in GST activity in the livers and muscles of fishes exposed to 730 ppm as compared to the control, as shown in Figure 2. GST has been identified as a biomarker for assessing the environmental impact of xenobiotics generating oxidative stress, water, and sediment contamination (El-Sharkawy et al., 1994). GST activity was more pronounced in hepatic tissues than in white muscle and gill, which indicates the effective role of the liver in xenobiotic detoxification (Ellman et al., 1961). Thus, the investigation of GST activity in various fish species, considering their phylogenic rank, definite features of ecology, and structure, is important in assessing fishes’ capabilities to protect against pollutants. Gobi et al., 2018 have noted similar observations during the assessment of selenium against the GST enzyme, finding that enzyme expression increased in the gills and liver after 96 h of exposure.
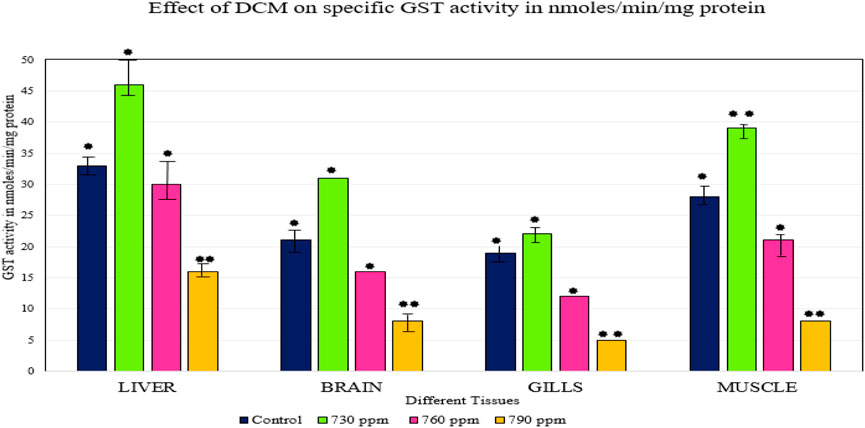
FIGURE 2. Effect of DCM on specific GST activity (nmoles/min/mg protein) in the different tissues of O. mossambicus. —p < 0.05, —p > 0.05.
Acetylcholinesterase Activity
Muscular functioning in animals is aided by AChE activity. AChE is a central target of some toxicants, which can produce certain toxic effects (Rao et al., 2005). This study examined the action of AChE in the essential organs, such as the gills, liver, muscle, and brain of Oreochromis mossambicus. AChE activity decreased in different tissues; inhibition of AChE was accompanied by an increase in acetylcholine levels in the cells. This condition can lead to an increase in catecholamines and affect the activity of enzymes involved in glycogenolysis and glycogen synthesis. Continuous stress may affect the synthesis site of AChE and decrease the levels of excess AChE. The mortality of fish may be due to inhibition of other enzymes, especially those involved in carbohydrate and protein metabolism. The inhibitory effect of AChE activity indicates that VOCs might interfere with vital process like the energy metabolism of nerve cells.
The inhibition of AChE activity in fish might be dangerous, potentially affecting the swimming activity and spatial orientation of the species (Rao et al., 2005; Roose and Brinkmann, 1998). Previous research has observed that the inhibition of AChE activity in muscle may lead to muscle paralysis, especially in fins and the respiratory apparatus, as well as hyperactivity and loss of balance (Rathbun, 1998). The inhibition of AChE activity in the brain in our study resulted in the accumulation of acetylcholine in the brain, leading to tremors, convulsions, and finally the death of the organism. There was a significant decrease in AChE activity in all tissues exposed to 730 ppm, 760 ppm, and 790 ppm DCM, compared to the control, as shown in Figure 3. Tissues exposed to 760 ppm DCM showed less AChE activity than the control, but the sample exposed to 790 ppm of DCM showed the lowest AChE activity. Gobi et al., 2018 have observed the inhibition of acetylcholine neurotransmitters in brain tissues upon exposure to high concentrations of selenium.
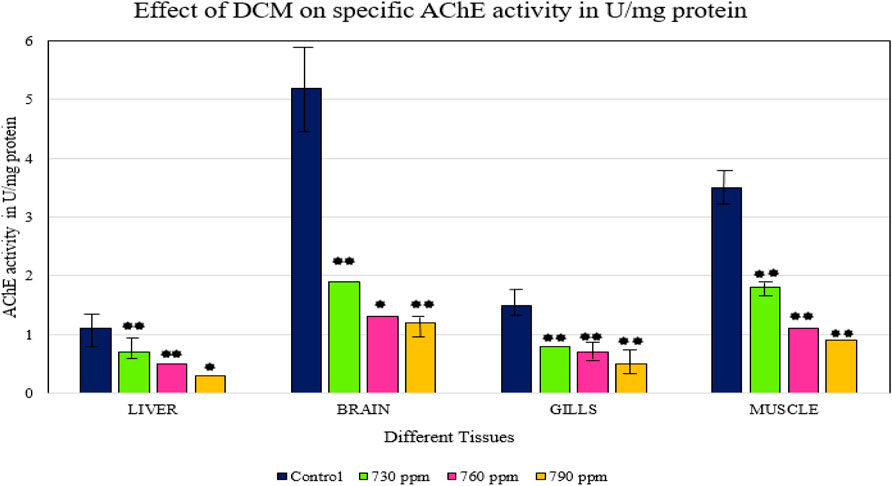
FIGURE 3. Effect of DCM on specific AChE activity (U/mg protein) in the different tissues of O. mossambicus. —p < 0.05, —p > 0.05.
Hematological Examination
Blood is an important pathophysiological indicator of the whole body; hence, hematological analysis be a quick and efficient method for evaluating water quality, based on the survival condition of the fishes (Shah et al., 2020). WBCs play the main role in cellular protection, which is based on the severity of the stress condition in all organisms. This induces higher mobilization and extravasation at the site of inflammation. A gradual decrease in RBCs and Hb reflects the anemic state of the fish, and a rise in MCV values in this study may be considered as the notable guide of RBC destruction due to endosmosis (Delpire and Gagnon, 2018). In the tissues that were exposed to 760 ppm DCM, total WBC count, Hb, and PCV control were higher than 730 ppm but less than with 790 ppm of DCM, while the platelet counts of tissues exposed to 760 ppm of DCM were lower than in the control and 790 ppm-exposed tissues. MCV for the tissues exposed to 760 ppm of DCM was higher than for the control and for 730 ppm exposure to DCM. MCH values for tissues exposed to 760 ppm DCM were higher than for 730 ppm and 790 ppm. MCHC amount for the tissues exposed to 760 ppm was slightly lower than for the control. Hematological changes in fishes exposed to different concentrations of DCM are depicted in Table 1. There was a slow decrease in RBC count while WBC count increased. A decrease in platelet count was observed as compared to control, and MCV, PCV, and MCH values showed slight fluctuations. MCHC value was almost equal to the control value in all concentrations, and there was a decrease in hemoglobin content.
Decreased hematological values indicated anemia in DCM-exposed fish, which may be due to erythropoiesis, chemosynthesis, and osmoregulatory dysfunction or due to an increased rate of erythrocyte destruction in hematopoietic organs (Fujii et al., 1999; Livingstone, 1998). The prolonged reduction in hemoglobin content is deleterious to oxygen transport; degeneration of erythrocytes can be understood as a pathological condition in fishes exposed to toxicants (Roose and Brinkmann, 1998). The decrease in hemoglobin content in the present study resulted from the rapid oxidation of hemoglobin into methemoglobin or from the release of oxygen radicals caused by the oxidative stress of DCM. In the present research, the decrease in RBC count during acute treatment might have resulted from a severe anemic state or from the hemolyzing power of the toxicant, particularly on the RBC membrane. The increase in WBCs is correlated with an increase in antibody production, which helps in the survival and recovery of fish exposed to toxicants (Balint et al., 1995). In the present study, the increase in WBC count indicates fishes’ hypersensitivity to DCM, and these changes may be due to immunological reactions that produce antibodies to cope with stress.
MCH and MCV values fluctuated and decreased during this research, and these alterations were attributed to direct or feedback responses of structural damage to RBC membranes, resulting in hemolysis and impairment of hemoglobin synthesis and stress-related release of RBC from the spleen and hypoxia, induced by exposure to the toxicant (Edwards, 1993).
Histopathological Sectioning
The histopathological sections of the slides showed deformations in all the three tissues that were tested.
Liver
The histopathological sections of control and treated livers showed marked variations, as represented in Figures 4, 5. The sections of the treated livers showed liver necrosis, which may be due to the direct toxic effects of pollutants on hepatocytes since the liver is the main site of detoxification for all types of toxins and chemicals. Oxygen deficiency because of gill degeneration may be the common cause of cellular degeneration in the liver. The vascular dilation, intracellular hemolysis, and thrombosis formation observed in blood vessels, with subsequent stasis of blood, may also be responsible for cellular degeneration and necrosis in the liver. Histopathological tests in the livers of carp exposed to hexachlorocyclohexane have revealed diffuse necrosis, just as in the present case (Monteiro et al., 2006). These findings suggest that DCM and other chlorine-containing compounds have similar effects on the liver.
Gill
After 96 h of treatment, there was a marked variation between the control and treated gill tissues. The gill arches of the control group (Figure 1) showed a normal arrangement pattern, while the treated gill sections Figures 6, 7 exhibited congestion and epithelial proliferation. (Essien et al., 2013) have found that exposing Malpighian cells to chemical irritation leads to an accumulation of cells at the leading edge of the secondary lamella, resulting in the epithelial proliferation of secondary gill lamellae. This indicates that DCM has a direct effect on gill filaments as a cytotoxic and irritating substance, resulting in the proliferation and fusion of secondary lamellae.
Moreover, gills are important not only for gaseous exchange but also for the osmoregulation and excretion of toxic waste products (Basha and Rani, 2003). Thus, any harm to the gills leads to impairment of these vital functions, revealing respiratory distress, impaired osmoregulation, and retention of toxic wastes. Histopathological studies conducted in goldfish have reported a fusion of the gill filaments due to toluene, suggesting a similar effect in gills due to other volatile organic compounds (Pandey et al., 2008). A similar effect was also seen in the gill samples of carp exposed to hexachlorocyclohexane (Cooley et al., 2001). Bhanot and Hundal, 2020 also reported similar observations of cytotoxicity in gills during the assessment of treated and un-treated polluted wastewater on Labeo rohita; they observed an increase in cytotoxicity upon an increase in the concentration of wastewater.
Muscle
The control muscles (Figure 8) showed bundles with striations as usual, while the treated muscles (Figures 8, 9) showed fiber splitting and variation in myofibril diameter. The initial stimulus of DCM can induce hyperactivity and excitability in animals, leading to a release of lactic acid and subsequent muscular fatigue. All these changes were clear as clinical signs at the initial stage of the experiment and were subsequently observed in histopathological changes to muscle (Das and Mukherjee, 2000). Similarly, one of our experiments assessed the cellular stress and histopathology undergone by Oreochromis mossambicus, with biochemical compounds being used as tools or biomarkers to analyze metal contamination. The antioxidative enzymes, cellular biomarkers, AChE, and histopathology can be used as biomarkers for monitoring metal contamination in the aquatic ecosystem. Similarly, one of the studies on Oreochromis mossambicus found that exposure to nanoparticles, such as silver nanoparticles and silicon nanoparticles, induced oxidative stress and cellular alterations, which were reflected in the histopathological studies. Nitschke et al., 1988 reported the inhalation toxicity and oncogenicity of DCM on Sprague-Dawley rats at different concentrations (i.e., 0, 50, 200, and 500 ppm) for 6 h/day and 5 days/week for 2 years. They reported a linear increase in the blood carboxyhemoglobin levels due to the exposure (Kim and Kim, 1996; Kim et al., 2007). Their histopathological studies revealed an increase in hepatocellular vacuolization when exposed to a concentration of 500 ppm. The male and female rats developed multinucleated hepatocytes and a number of spontaneous, benign mammary tumors vigorously progressing to malignant tumors when exposed to 50–200 ppm. There were no significant increases in malignancy at concentrations above 500 ppm. Rooij et al., 2004 reported the toxic effects of both acute and chronic DCM toxicity on fish at 830 μg/L in the marine environment. Thus, this study of DCM toxicity on Oreochromis mossambicus has not been reported anywhere.
Molecular Docking Studies of Aloe vera Against Interleukin-1β
The ligand binding site was predicted using the CastP web server, where about 75 amino acids were reported for the binding site. The amino acids residues include ARG9, GLU10, GLU11, LYS12, ILE13, ILE14, LEU15, VAL16, SER17, SER18, ASP23, VAL24, ARG25, PRO26, CYS27, PRO28, LEU29, ASN30, PRO31, ASN32, GLU33, GLY36, THR37, ILE38, HIS60, LYS61, GLU62, LYS63, LEU64, TRP65, PHE66, TYR77, CYS79, VAL80, VAL81, ASN83, SER85, TYR86, CYS87, LEU88, ARG89, ILE90, ILE92, SER93, ALA94, VAL97, ASN99, CYS104, TYR105, ASN106, ALA109, PHE111, VAL124, CYS125, PRO126, TYR127, MET128, GLU129, PHE130, PHE131, LYS132, ASN133, GLU134, ASN135, ASN136, GLU137, LEU138, LYS161, ASP162, ARG163, TYR183, TYR185, THR193, ARG194, and VAL195. The screening of bioactive compounds satisfying the Lipinski rule of five is given in Supplementary Table S1. Out of 26 bioactive compounds, only 12 compounds have satisfied the rule; further, the ligands were taken for molecular docking studies. The top compounds with high binding energies were sitosterol (-12.398 kcal/mol), squalene (-12.157 kcal/mol), and alpha-tocopherol (-11.993 kcal/mol). The compound sitosterol interacted with amino acids GLU11 and SER93, forming a conventional hydrogen bond with GLU129. Squalene, which ranked second with the score of -12.157 kcal/mol, exhibited only van der Waals interactions with residues such as GLU11, SER93, CYS27, GLU33, and LEU29; other interactions included pi-alkyl and alkyl interactions. When binding energy is lower, binding affinity is higher and the effect of inhibition is stronger. No formation of conventional hydrogen bonding was observed, as there were no acceptor or donor atoms present in the ligand. Alpha-tocopherol had the third-least binding energy (-11.993 kcal/mol) and formed 2 conventional hydrogen bonds with the residues SER93 and ILE13. The other interactions of alpha-tocopherol included dan der Waals, pi-alkyl, alkyl, and pi-sigma interactions with amino acid residues. The molecular docking results are given in Supplementary Table S2.
Screening of Drug Properties
Drug properties such as a compound’s absorption, distribution, metabolism, excretion, and toxicity level were analyzed for the 5 compounds with the highest binding energies (Supplementary Tables S3–S6). All the compounds reported a satisfactory level of gastrointestinal absorption (i.e., above 88%). All the compounds except for oleic acid were reported for the p-glycoprotein inhibitor. The compounds have shown a satisfactory level of blood-brain barrier permeability and CNS permeability properties. All the compounds have been reported as the substrate of CYP3A4 c, and the compounds oleic acid and eicosane were inhibitors of CYP1A2. Furthermore, the compound alpha-tocopherol has been reported for inhibiting the CYP2C19 cytochrome enzyme. None of the other compounds were CYP2D6, CYP3A4, and CYP2C9 inhibitors. None of the compounds exhibited Ames mutagenicity, and none were hERG 1 inhibitors. The compounds were safe and were not hepatotoxic in nature. Compounds such as oleic acid and eicosane were studied for their skin sensitization properties.
Conclusion
As the results suggest, the LC50 of DCM is 760 ppm, and the hematology and histopathology of the dead fishes support the research. According to research, the side effects of human consumption of intoxicated Oreochromis mossambicus are fatal, as it increases the risk of several cancers including brain cancer, bile-duct cancer, non-Hodgkin lymphoma, and multiple myeloma. Intoxicated fishes can be treated with Aloe vera to boost their immunity, causing the effect of DCM on the fish to be minimal. As the in silico work suggests, the activation of IL-1β to fight oxidative stress and neurotoxicity is done exceptionally well by the Aloe vera plant. This in silico research can be validated by in vitro and in vivo studies. Therefore, fish can be made human friendly and can avoid the above-mentioned diseases and seafood poisoning.
Data Availability Statement
The original contributions presented in the study are included in the article/Supplementary Material. Further inquiries can be directed to the corresponding author.
Ethics Statement
Ethics review and approval/written informed consent was not required as per local legislation and institutional requirements.
Author Contributions
GN conceived and designed the study, wrote the discussion, performed the experiments, and wrote and proofread the paper. AS calibrated the graphs, performed the in silico research, and wrote the molecular docking part of the manuscript.
Acknowledgments
Thanks to the Department of Bioengineering, Vels Institute of Science, Technology and Advanced Studies, Chennai.
Conflict of Interest
The authors declare that the research was conducted in the absence of any commercial or financial relationships that could be construed as a potential conflict of interest.
Publisher’s Note
All claims expressed in this article are solely those of the authors and do not necessarily represent those of their affiliated organizations, or those of the publisher, the editors and the reviewers. Any product that may be evaluated in this article, or claim that may be made by its manufacturer, is not guaranteed or endorsed by the publisher.
Supplementary Material
The Supplementary Material for this article can be found online at: https://www.frontiersin.org/articles/10.3389/fenvs.2022.913065/full#supplementary-material
References
Abhishek Biswal, R., and Pazhamalai, V. (2020). A Structure Based Molecular Docking of Acacia Catechu against Contractile Protein Plasmodium Falciparum – a Malarial Disease. Int. J. Pharm. Sci. Drug Res. November-December 12.1. doi:10.25004/IJPSDR.2020.120110
Abhishek Biswal, R., Sharma, Akshata, Aishwarya, A., and Pazhamalai, Vivek (2020). Molecular docking and ADMET studies of bioactive compounds of rhizopora mucornata AGAINST bacterial enzyme protein tyrosine phosphatase. Int. J. Pharm. Sci. Res. 11 (4). doi:10.13040/IJPSR.0975-8232
Ahmad, Y., Andrabi, S. M., Hussain, A., Hussain, G., Wani, A. R., Bannetwala, R. C., et al. (2015). Haematological Studies of Freshwater Fish, Labeo Rohita (ham.) Exposed to Heavy Metals. Int. J. Sci. Environ. Technol. 4 (6), 1513–1523.
Arunkumar, S., and Muthuselvam, M. (2009). Analysis of phytochemical constituents and antimicrobial activities of Aloe Vera L. Against clinical pathogens. World J. Agric. Sci. 5 (5), 572–576. ISSN 1817-3047, IDOSI Publications, 2009.
Balint, T., Szegletes, T., Szegletes, Z., Halasy, K., and Nemcsók, J. (1995). Biochemical and Subcellular Changes in Carp Exposed to the Organophosphorus Methidathion and the Pyrethroid Deltamethrin. Aquat. Toxicol. 33 (3-4), 279–295. doi:10.1016/0166-445x(95)00029-4
Ball, W. T., Alsing, J., Mortlock, D. J., Staehelin, J., Haigh, J. D., Peter, T., et al. (2018). Evidence for a Continuous Decline in Lower Stratospheric Ozone Offsetting Ozone Layer Recovery. Atmos. Chem. Phys. 18 (2), 1379–1394. doi:10.5194/acp-18-1379-2018
Basha, P. S., and Rani, A. U. (2003). Cadmium-induced Antioxidant Defense Mechanism in Freshwater Teleost Oreochromis mossambicus (Tilapia). Ecotoxicol. Environ. Saf. 56 (2), 218–221. doi:10.1016/s0147-6513(03)00028-9
Bhanot, R., and Hundal, S. S. (2020). Assessment of Cytotoxicity in Gills of Fish Labeo Rohita Reared in Untreated and Treated Sewage Water. Environ. Sci. Pollut. Res. 10, 59306–59316. doi:10.1007/s11356-020-10619-0
Bonfiglioli, R., Carnevali, L., Di Lello, M., and Violante, F. S. (2014). Bilateral Hearing Loss after Dichloromethane Poisoning: A Case Report. Am. J. Ind. Med. 57 (2), 254–257. doi:10.1002/ajim.22257
Bos, P. M., Zeilmaker, M. J., and van Eijkeren, J. C. (2006). Application of Physiologically Based Pharmacokinetic Modeling in Setting Acute Exposure Guideline Levels for Methylene Chloride. Toxicol. Sci. 91 (2), 576–585. doi:10.1093/toxsci/kfj176
Buckley, J. A., Whitmore, C. M., and Matsuda, R. I. (1976). Changes in Blood Chemistry and Blood Cell Morphology in Coho Salmon (Oncorhynchus kisutch) Following Exposure to Sublethal Levels of Total Residual Chlorine in Municipal Wastewater. J. Fish. Res. Bd. Can. 33 (4), 776–782. doi:10.1139/f76-095
Clayton, G. D., and Clayton, F. E. (1981). Patty's industrial hygiene and toxicology, 2A. Baffins Lane, Chichester, Sussex PO19 1DU: John Wiley & Sons.
Cooley, H. M., Fisk, A. T., Wiens, S. C., Tomy, G. T., Evans, R. E., Muir, D. C., et al. (2001). Examination of the Behavior and Liver and Thyroid Histology of Juvenile Rainbow Trout (Oncorhynchus mykiss) Exposed to High Dietary Concentrations of C10-C11-C12-And C14-Polychlorinated N-Alkanes. Aquat. Toxicol. 54 (1-2), 81–99. doi:10.1016/s0166-445x(00)00172-7
Das, B. K., and Mukherjee, S. C. (2000). A Histopathological Study of Carp (Labeo Rohita) Exposed to Hexachlorocyclohexane. Veterinarski Arh. 70 (4), 169–180.
De Rooij, C., Thompson, R. S., Garny, V., Lecloux, A., and Van Wijk, D. (2004). Dichloromethane Marine Risk Assessment with Special Reference to the OSPARCOM Region: North Sea. Environ. Monit. Assess. 97 (1-3), 3–22. doi:10.1023/b:emas.0000033040.18114.49
Delpire, E., and Gagnon, K. B. (2018). “Water Homeostasis and Cell Volume Maintenance and Regulation,” in Current topics in membranes (Cambridge, Massachusetts, United States: Academic Press), 81, 3–52.
Edwards, C. A. (1993). “The Impact of Pesticides on the Environment,” in The pesticide question (Boston, MA: Springer), 13–46.
El-Sharkawy, A. M., Abdel-Rahman, S. Z., Hassan, A. A., Gabr, M. H., El-Zoghby, S. M., El-Sewedy, S. M., et al. (1994). Biochemical Effects of Some Insecticides on the Metabolic Enzymes Regulating Glutathione Metabolism. Bull. Environ. Contam. Toxicol. 52 (4), 505–510. doi:10.1007/bf00194136
Ellman, G. L., Courtney, K. D., Andres, V., and Featherstone, R. M. (1961). A New and Rapid Colorimetric Determination of Acetylcholinesterase Activity. Biochem. Pharmacol. 7 (2), 88–95. doi:10.1016/0006-2952(61)90145-9
Essien, E. B., Abbey, B. W., Chinwe, N., and Odeghe, O. B. (2013). Physico-chemical evolution, gill mda concentration and histology of Tilapia exposed to mixed effluent in okrika river, rivers state, Nigeria. J. Environ. Earth Sci. 3 (2), 88–96.
Fechner, G., Du Chesne, A., KöhIer, H., and KohIer, H. (2001). Fatal Intoxication Due to Excessive Dichloromethane Inhalation. Forensic Sci. Int. 122 (1), 69–72. doi:10.1016/s0379-0738(01)00468-6
Fujii, Toshiko, Kawabe, Satoko, Horike, Tokushi, Horike, Toyohiro, Tagachi, Toyohiro, and Ogata, Masana (1999). Simultaneous Determination of the Urinary Metabolites of Toluene, Xylene and Styrene Using High-Performance Capillary Electrophoresis. J. Chromatogr. B Biomed. Sci. Appl. 730, 41–47. doi:10.1016/s0378-4347(99)00175-9
Gobi, N., Vaseeharan, B., Rekha, R., Vijayakumar, S., and Faggio, C. (2018). Bioaccumulation, Cytotoxicity, and Oxidative Stress of the Acute Exposure Selenium in Oreochromis mossambicus. Ecotoxicol. Environ. Saf. 162, 147–159. doi:10.1016/j.ecoenv.2018.06.070
Goullé, J. P., Lacroix, C., Vaz, E., Rouvier, P., and Proust, B. (1999). Fatal Case of Dichloromethane Poisoning. J. Anal. Toxicol. 23 (5), 380–383. doi:10.1093/jat/23.5.380
Jaffe, T. A., Boyer, E. W., Erickson, T. B., Studley, H., Hayes, B. D., Chai, P. R., et al. (2019). Acute and Delayed Toxicity from Co-ingestion of Methylene Chloride and Methanol. Toxicol. Commun. 3 (1), 79–84. doi:10.1080/24734306.2019.1685222
Kim, S. K., and Kim, Y. C. (1996). Effect of a Single Administration of Benzene, Toluene, or M-Xylene on Carboxyhaemoglobin Elevation and Metabolism of Dichloromethane in Rats. J. Appl. Toxicol. 16 (5), 437–444. doi:10.1002/(sici)1099-1263(199609)16:5<437::aid-jat371>3.0.co;2-2
Kim, S. N., Seo, J. Y., Jung, D. W., Lee, M. Y., Jung, Y. S., Kim, Y. C., et al. (2007). Induction of Hepatic CYP2E1 by a Subtoxic Dose of Acetaminophen in Rats: Increase in Dichloromethane Metabolism and Carboxyhemoglobin Elevation. Drug Metab. Dispos. 35 (10), 1754–1758. doi:10.1124/dmd.107.015545
Kim, Y. C., and Carlson, G. P. (1986). The Effect of an Unusual Workshift on Chemical Toxicity: II. Studies on the Exposure of Rats to Aniline. Toxicol. Sci. 7 (1), 144–152. doi:10.1093/toxsci/7.1.144
Kobayashi, A., Ando, A., Tagami, N., Kitagawa, M., Kawai, E., Akioka, M., et al. (2008). Severe Optic Neuropathy Caused by Dichloromethane Inhalation. J. ocular Pharmacol. Ther. 24 (6), 607–612. doi:10.1089/jop.2007.0100
Liang, Quan, Li, Weifen, Guo, Ningning, Tong, Chao, Zhou, Yingshan, Fang, Weihuan, et al. (2016). Identification and functional analysis of interleukin-1β in the Chinese soft-shelled turtle pelodiscus Sinensis. Genes (Basel) 7 (5), 18. Published online 2016 May 4. doi:10.3390/genes7050018
Livingstone, D. R. (1998). The Fate of Organic Xenobiotics in Aquatic Ecosystems: Quantitative and Qualitative Differences in Biotransformation by Invertebrates and Fish. Comp. Biochem. Physiology Part A Mol. Integr. Physiology 120, 43–49. doi:10.1016/s1095-6433(98)10008-9
Mahmud, M., and Kales, S. N. (1999). Methylene Chloride Poisoning in a Cabinet Worker. Environ. health Perspect. 107 (9), 769–772. doi:10.1289/ehp.99107769
Monteiro, D. A., De Almeida, J. A., Rantin, F. T., and Kalinin, A. L. (2006). Oxidative Stress Biomarkers in the Freshwater Characid Fish, Brycon Cephalus, Exposed to Organophosphorus Insecticide Folisuper 600 (Methyl Parathion). Comp. Biochem. Physiology Part C Toxicol. Pharmacol. 143 (2), 141–149. doi:10.1016/j.cbpc.2006.01.004
Nirmala1, G. N., Sharma, A., Dharani Dharan, .K., and Venkatraghavan, R. (2020). Effect of Essential Oil of santalum Album against Covid-19, Lung Cancer and Streptococcal Pneumonia: An insilco Approach. Solid State Technol. 63 (6).
Nitschke, K. D., Burek, J. D., Bell, T. J., Kociba, R. J., Rampy, L. W., and McKenna, M. J. (1988). Methylene Chloride: A 2-year Inhalation Toxicity and Oncogenicity Study in Rats. Fundam. Appl. Toxicol. 11 (1), 48–59. doi:10.1016/0272-0590(88)90269-2
Oh, S. J., Kim, S. K., and Kim, Y. C. (2002). Role of Glutathione in Metabolic Degradation of Dichloromethane in Rats. Toxicol. Lett. 129 (1-2), 107–114. doi:10.1016/s0378-4274(01)00523-9
Oliver, Grandman (2012). Aloe Vera Gel Research Review an Overview of its Clinical Uses and Proposed Mechanisms of Action. Nat. Med. J. 4 (9).
Pacheco, C., Magalhaes, R., Fonseca, M., Silveira, P., and Brandão, I. (2016). Accidental Intoxication by Dichloromethane at Workplace: Clinical Case and Literature Review. J. Acute Med. 6 (2), 43–45. doi:10.1016/j.jacme.2016.03.008
Pan, G., and Dutta, H. M. (1998). The Inhibition of Brain Acetylcholinesterase Activity of Juvenile Largemouth Bass Micropterus salmoides by Sublethal Concentrations of Diazinon. Environ. Res. 79, 133–137. doi:10.1006/enrs.1998.3868
Pandey, S., Parvez, S., Ansari, R. A., Ali, M., Kaur, M., Hayat, F., et al. (2008). Effects of Exposure to Multiple Trace Metals on Biochemical, Histological, and Ultrastructural Features of Gills of a Freshwater Fish, Channa punctata Bloch. Chemico-biological Interact. 174 (3), 183–192. doi:10.1016/j.cbi.2008.05.014
Ramesh, M., and Sarvanan, M. (2008). Haematological and Biochemical Responses in a Freshwater Fish Cyprinus carpio Exposed to Chlorpyrifos. Int. J. Integr. Biol. 3, 80–83.
Rao, J. V., Begum, G., Pallela, R., Usman, P. K., and Rao, R. N. (2005). Changes in Behavior and Brain Acetylcholinesterase Activity in Mosquito Fish, Gambusia affinis in Response to the Sub-lethal Exposure to Chlorpyrifos. Int. J. Environ. Res. Public Health 2 (3), 478–483. doi:10.3390/ijerph2005030013
Raphael, M., Nadiras, P., and Flacke-Vordos, N. (2002). Acute Methylene Chloride Intoxication—A Case Report on Domestic Poisoning. Eur. J. Emerg. Med. 9 (1), 57–59. doi:10.1097/00063110-200203000-00013
Rathbun, R. E. (1998). Transport, behavior, and fate of volatile organic compounds in streams: U.S. Washington, USA: Geological Survey Professional Paper 1589, 151.
Roose, Patrick, and Brinkmann, Udo A. Th (1998). Determination of Volatile Organic Compounds in Marine Biota. J. Chromatogr. A 799, 233–248. doi:10.1016/s0021-9673(97)01081-9
Sahu, Pankaj, Giri, Deen, Singh, Ritu, Pandey, Priyanka, Gupta, Sharmistha, Shrivastava, Atul, et al. (2013). Therapeutic and medicinal uses of Aloe Vera: A review. Pharmacol. Pharm. 4.
Shah, N., Khan, A., Ali, R., Marimuthu, K., Uddin, M. N., Rizwan, M., et al. (2020). Monitoring bioaccumulation (In gills and muscle tissues), hematology, and genotoxic alteration in Ctenopharyngodon Idella exposed to selected heavy metals. BioMed Res. Int. 15, 1–16. doi:10.1155/2020/6185231
Shah, S. L. (2006). Hematological Parameters in tenchTinca tinca after Short Term Exposure to Lead. J. Appl. Toxicol. 26 (3), 223–228. doi:10.1002/jat.1129
Sweeney, L. M., Saghir, S. A., and Gargas, M. L. (2008). Physiologically Based Pharmacokinetic Model Development and Simulations for Ethylene Dichloride (1, 2-dichloroethane) in Rats. Regul. Toxicol. Pharmacol. 51 (3), 311–323. doi:10.1016/j.yrtph.2008.05.002
Toccalino, P., Rowe, B. L., and Norman, J. E. (2006). Volatile organic compounds in the nation's drinking-water supply wells: What findings may mean to human health. Washington, D.C., United States: US Department of the Interior, US Geological Survey.
Townsend, D. M., and Tew, K. D. (2003). The Role of Glutathione-S-Transferase in Anti-cancer Drug Resistance. Oncogene 22 (47), 7369–7375. doi:10.1038/sj.onc.1206940
Watanabe, K., Liberman, R. G., Skipper, P. L., Tannenbaum, S. R., and Guengerich, F. P. (2007). Analysis of DNA Adducts Formed In Vivo in Rats and Mice from 1, 2-dibromoethane, 1, 2-dichloroethane, Dibromomethane, and Dichloromethane Using HPLC/accelerator Mass Spectrometry and Relevance to Risk Estimates. Chem. Res. Toxicol. 20 (11), 1594–1600. doi:10.1021/tx700125p
Wirkner, K., Damme, B., Poelchen, W., and Pankow, D. (1997). Effect of Long-Term Ethanol Pretreatment on the Metabolism of Dichloromethane to Carbon Monoxide in Rats. Toxicol. Appl. Pharmacol. 143 (1), 83–88. doi:10.1006/taap.1996.8077
Keywords: dichloromethane, histological examination, hematological, molecular docking studies, oxidative stress, neurotoxic
Citation: Nirmala GN, Sharma A and Ragunathan V (2022) Antagonistic Effect of Dichloromethane on Oreochromis mossambicus and Immune Stimulation Activity of Aloe Vera. Front. Environ. Sci. 10:913065. doi: 10.3389/fenvs.2022.913065
Received: 05 April 2022; Accepted: 21 June 2022;
Published: 29 August 2022.
Edited by:
Bechan Sharma, Allahabad University, IndiaReviewed by:
Özge Temiz, Osmaniye Korkut Ata University, TurkeyJosef Velíšek, University of South Bohemia, Czechia
Copyright © 2022 Nirmala, Sharma and Ragunathan. This is an open-access article distributed under the terms of the Creative Commons Attribution License (CC BY). The use, distribution or reproduction in other forums is permitted, provided the original author(s) and the copyright owner(s) are credited and that the original publication in this journal is cited, in accordance with accepted academic practice. No use, distribution or reproduction is permitted which does not comply with these terms.
*Correspondence: G. N. Nirmala, gnnirmala.se@velsuniv.ac.in