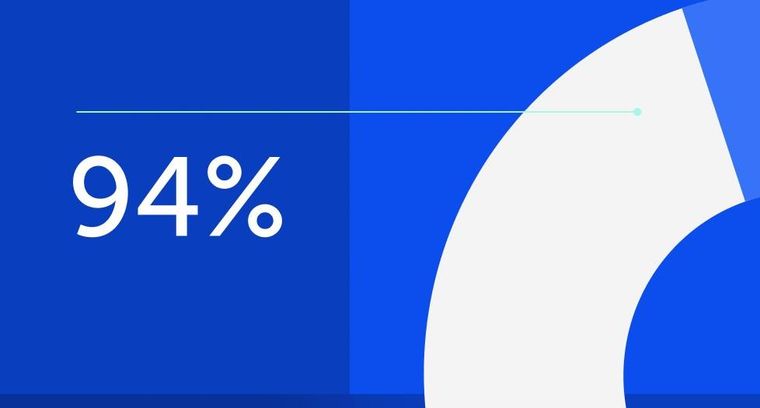
94% of researchers rate our articles as excellent or good
Learn more about the work of our research integrity team to safeguard the quality of each article we publish.
Find out more
ORIGINAL RESEARCH article
Front. Environ. Sci., 12 May 2022
Sec. Freshwater Science
Volume 10 - 2022 | https://doi.org/10.3389/fenvs.2022.908088
This article is part of the Research TopicThe Effects of Benthic-Pelagic Coupling on Shallow Lake Ecosystems: Implications for Lake ManagementView all 8 articles
Climate warming, a serious environmental problem worldwide, is considered a major threat to aquatic ecosystems. A primary feature of climate warming is elevated temperatures which in shallow aquatic ecosystems might affect competition for light and nutrient between benthic algae on the sediment surface and planktonic algae in the water. The outcomes of such competition would not only affect the distribution of primary production, but also determine the fundamental character of shallow aquatic habitats as clear water or turbid water systems. We conducted a mesocosm study to evaluate the effects of elevated temperature on competition between planktonic algae and benthic algae for light and nutrients. We found that elevated temperature increased the concentrations of total nitrogen (TN), total phosphorus (TP), and total suspended solids (TSS) in overlying water and enhanced the growth of planktonic algae (measured as chlorophyll a, Chl a), but decreased light intensity and benthic algal biomass (Chl a). Our results indicate that elevated temperature can increase the growth of planktonic algae and enhance their competitive advantage over the benthic algae in shallow lakes, thereby contributing to eutrophication and a decline in water quality. These findings shed further light on the effects of global warming on aquatic ecosystems.
Climate warming has become a serious environmental problem worldwide and is considered a major threat to the natural environment, including aquatic ecosystems (Paerl and Huisman, 2008; Jeppesen et al., 2010). A major feature of climate warming is elevated temperatures (Paerl et al., 2011). According to the Sixth Scientific Assessment Report (IPCC, 2018), global average temperatures increased 1.5°C from 1880 to 2018, and this trend continues. The currents warming rate is projected to be 0.25°C per decade until the middle of the 21st century and 0.36°C per decade in the second half of the century (Andreas-Gobiet et al., 2014). Warming will have profound impacts on ecological processes in aquatic ecosystems (Amundrud and Srivastava, 2019) by affecting primary production, water resource distribution and water quality (Daufresne et al., 2009).
Elevated temperatures can promote the growth of algae in both benthic and planktonic habitats and affect the physiology of primary producers (Butterwick et al., 2010; Liu et al., 2015; Li et al., 2016) because temperature plays a crucial role in metabolic processes, including photosynthesis, nutrient uptake and the enzymatic activity of cells (Claquin et al., 2008; Thorel et al., 2014). However, temperature also influences the chemical and physical processes of aquatic ecosystems (Takano and Hino, 2000; Ozaki et al., 2010; Liu et al., 2015) with implication for the dynamics of the aquatic ecosystems. Increased temperature promotes the release of nitrogen and phosphorus from sediments (Malmaeus et al., 2005), which may enhance planktonic algal growth and thus an increase in TSS. Increases of planktonic algae and total suspended solids (TSS) reduce the light intensity on the sediment surface (Marcus, 1998; Zhang et al., 2015; Razlutskij et al., 2021), limiting the growth of benthic algae (Takano and Hino, 2000; Butterwick et al., 2010). Thus, elevated temperature might affect the growth of both planktonic and benthic algae in aquatic systems.
In shallow lakes, benthic algae growing on sediment surfaces are often limited by light resources as a result of attenuation caused by planktonic algae in the overlying water (Vadeboncoeur et al., 2003). In systems where external nutrient loading has been reduced, internal loading becomes a limiting factor for the planktonic algae (Zhu et al., 2010), while benthic algae benefit from nutrients in the sediment. If abundant, benthic algae may reduce sediment release of nutrients and thereby further hampering growth of planktonic algae (Vadeboncoeur et al., 2003; Zhang et al., 2013; Blottière et al., 2017). Competition between planktonic algae and benthic algae is one of the key factors affecting shallow aquatic ecosystem dynamics (Flöder et al., 2006; Genkai-Kato et al., 2012) and has become an area of great interest in freshwater ecology (Pastcrnak et al., 2009; Zhang et al., 2013; Jäger and Diehl, 2016).
However, the effects of elevated temperature on resource competition between benthic and planktonic algae in shallow aquatic ecosystems are yet to be fully understood. Here, we hypothesized that elevated temperature would benefit planktonic algae and hamper benthic algae, leading to a deterioration in water quality. To test this hypothesis, we designed a mesocosm experiment with elevated temperatures and compared with controls run at ambient temperature. The effects of elevated temperature on the competition between planktonic algae and benthic algae for resources of light and nutrient were investigated using measurements of light intensity on the sediment surface, nutrient content in overlying water, and the development of benthic and planktonic algal biomass over time. The aim of this study is to evaluate the effect of elevated temperature on resource competition between benthic and planktonic algae in shallow aquatic ecosystems. The results may shed light on the effects of global warming on natural aquatic ecosystems.
The mesocosm system consisted of eight white polyethylene plastic tanks (bottom diameter = 46 cm, upper diameter = 57 cm, and height = 72 cm) placed in the agricultural garden of Anhui Agricultural University, Hefei, China. Sediments were collected from a pond at the campus and were air dried and crushed to remove large particles. The treated sediment was then added to the tanks in order to obtain a 10 cm thick layer (Zhang et al., 2016).
Rainwater (TN = 0.96 mg/L−1, TP = 0.02 mg/L−1) was collected and added to each tank to a depth of 70 cm. All eight mesocosms were allowed to equilibrate for 2 weeks before the experiment started. Then four treatments were covered by black plastic paper on the outside wall to create elevated temperature while the controls were covered by heat insulation film. Both the black paper and the heat insulation film are lightproof. So, sunlight did not enter through the sidewall of either the treatment or the control mesocosms. A Petri dish (diameter of 6 cm) containing treated sediments was inserted into the upper sediment layer of each mesocosm, such that the surface the sediments inside and outside the dishes was at the same level for benthic algae growth. Rainwater was added to the mesocosms as required to maintain the water levels during the experiment. The experiment was then run for 60 days from July 23 to 20 September 2020.
The tanks were sampled at approximately 12 noon every 10 days during the experimental period. Water samples (500 ml) were taken with a clean glass bottle from 10 cm below the water surface from each mesocosm, and analyzed for total nitrogen (TN), total phosphorus (TP), total suspended solids (TSS) and planktonic algal biomass (Chl a). TN was determined by alkaline potassium persulfate UV spectrophotometry and TP was determined by ammonium molybdate UV spectrophotometry (APHA, 1998). 200 ml water was filtered by GF/C grade filter for Chl a of planktonic algal biomass and the Chl a on the filter was determined by UV spectrophotometry after extraction in 90% acetone (Jespersen and Christoffersen, 1987). TSS was calculated by weighing the residue retained on a GF/C grade filter after filtering 200 ml water and drying at 108°C for 2 h (Qu et al., 2019).
Water temperature was measured using a YSI probe from 30 cm below the water surface. Light intensity was measured using an underwater irradiance meter (ZDS-10W) at 50 cm below the water surface before sampling the water.
The Petri dishes with their benthic algae were removed slowly from each mesocosm and the benthic algae were scraped off and diluted with distilled water to 500 ml, then stored in brown glass bottles prior to analysis of algal biomass (Chl a) using the same method as for planktonic algae. After sampling, the Petri dishes and the sediments were re-loaded into each mesocosm for further benthic algae growth.
Independent sample t-tests were used to analyze differences in water temperature, TSS, light intensity, TN, TP and Chl a of both planktonic and benthic algal biomass between the elevated temperature treatment mesocosms and the controls. One-way ANOVA was performed to detect differences between treatments on each sampling occasion. SPSS 19.0 was used for data statistics analysis. All data are presented as mean ± SD and figures were generated by Origin Pro 9.0.
On average, water temperature was 1.7°C higher in the elevated temperature treatments than in the controls (t-test, p = 0.037), at 30.7 ± 0.3°C compared to 29.0 ± 0.2°C (Table 1). A significant difference in temperature was apparent on every sampling occasion except for day 10 and day 60 (one-way ANOVA, treatment effect, p < 0.05).
TN concentrations in the overlying water of the elevated temperature treatments were higher on average than in the controls at 6.43 ± 0.96 mg/L to 5.38 ± 0.42 mg/L respectively (t-test, p = 0.049), and also significantly higher in the elevated temperature treatments on day 40, day 50 and day 60 (one-way ANOVA, treatment effect, p < 0.05, Figure 1).
FIGURE 1. TN (mean ± SD) and TP (mean ± SD) in different treatments over time. Asterisk indicates significant (p < 0.05) differences between treatments.
TP concentrations in overlying water were also higher in the elevated temperature treatments than in the controls (t-test, p = 0.043) at 0.23 ± 0.06 mg/L and 0.16 ± 0.05 mg/L respectively, being significantly higher in the elevated temperature treatments on three out of six sampling occasions (day 30, 40 and 60, one-way ANOVA, p < 0.05, Figure 1).
The biomass of planktonic algae (Chl a) was higher on average in the elevated temperature treatments than in the controls (t-test, p = 0.041) and on each sampling occasion except for day 40 and day 60 (one-way ANOVA, treatment effect, p < 0.05; Figure 2).
FIGURE 2. Chl a of planktonic algae (mean ± SD) in different treatments over time. Asterisk indicates significant (p < 0.05) differences between treatments.
TSS concentrations (Figure 3) were higher on average in the elevated temperature treatments than in the controls (t-test, p = 0.012) at 19.50 ± 3.44 mg/L to 13.46 ± 2.78 mg/L respectively. Significantly higher TSS values were recorded in the elevated temperature treatments on each sampling occasion except for day 10 and day 30 (one-way ANOVA, treatment effect, p < 0.05).
FIGURE 3. TSS (mean ± SD) and light intensity (mean ± SD) in the different treatments over time. Asterisk indicates significant (p < 0.05) differences between treatments.
Light intensity (Figure 3) at the sediment surface was lower on average in the elevated temperature treatments than in the controls (t-test, p = 0.039) at 9565 ± 4403 lx to 15119 ± 3383 lx respectively. Reduced light intensity was recorded in the elevated temperature treatments on all sampling occasions except for day 10 and day 30 (one-way ANOVA, treatment effect, p < 0.05).
The biomass of benthic algae (Chl a) was lower on average in the elevated temperature treatments than in the controls (t-test, p = 0.003), and significantly so on every sampling occasion except for day 10 and day 30 (one-way ANOVA, treatment effect, p < 0.05; Figure 4).
FIGURE 4. Chl a of benthic algae (mean ± SD) in different treatments over time. Asterisk indicates significant (p < 0.05) differences between treatments.
We found that elevated temperature increased the nutrient concentrations of TN and TP in the overlying water, enhanced growth of planktonic algae (Chl a), increased TSS concentration, decreased light intensity at the sediment surface and reduced benthic algal biomass (Chl a).
The water temperature of the black covered mesocosms was 1.2–2.1°C (1.7°C on average) higher than in the controls. According to the Sixth Scientific Assessment Report (IPCC, 2018), global average temperatures increased 1.5°C from 1880 to 2018. This rate of increase has been observed in lakes world-wide (O’Reilly et al., 2015); and in some lakes, summer temperatures has increased 1.5°C in the last decades alone (Hetherington et al., 2015, Oneida Lake, NY, United States). Globally, the water temperature of rivers is expected to increase 0.8–1.6°C more in between 2071 and 2100 than between 1971 and 2000 (van Vliet et al., 2013). Thus, the treatment applied in our study resulted in heating comparable to real-world examples.
The increased nutrients (both TN and TP) in the overlying water of the elevated temperature treatments probably points to increased release from the sediments (Liu et al., 2017). A water temperature increase of 3–4°C, is sufficient to double the release rate of P (Nicholls, 1999). Further inputs of nutrients may be associated with rain events and atmospheric deposition (Liu et al., 2015). However, in this experiment, both the controls and the treatments mesocosms were maintained in the same garden of Anhui Agricultural University, so the N and P deposition from the atmosphere should be the same in both the different treatments. However, we cannot discount the possibility that some atmospheric N was added to the water N fixation by algae, as we did not monitor these algae separately in this study.
That elevated temperature can enhance the growth of planktonic algae is well-established (e.g., Pedersen and Borum, 1996). Blottière et al. (2017) found a 15% increase in planktonic algae for every 1°C increase in average annual temperature. In addition, elevated temperature can enhance the release of nutrients from the sediment (Jensen and Andersen, 1992), which may lead to an increase in the growth of planktonic algae and thus increase in TSS. Further, increases of planktonic algae and TSS can reduce water clarity and thereby limit benthic algae growth due to increased light limitation. Our results are consistent with this literature. We found a reduction in light intensity at the sediment surfaces of the elevated temperature treatments, and a lower benthic algae biomass compared with the controls, indicating increased competitive advantage of planktonic algae over benthic algae. Such a decline or even disappearance of benthic algae will further enhance the release of nutrients from the sediment into overlying water, creating a positive feedback loop promoting planktonic algae growth (Spears et al., 2008; Zhang et al., 2013). Thus, the elevated temperature leads to the transfer of nutrients from benthic to planktonic habitats (Nicholls, 1999; Genkai-Kato et al., 2012; Zhang et al., 2015).
It is important to acknowledge that the effects of elevated temperature on the dynamics of benthic and planktonic algae in natural aquatic ecosystems will be more complex than in our mesocosm system, which excluded fish, benthic animals and submerged macrophytes, all of which may have important roles in the competitive interplay between benthic and planktonic algae (Zhang et al., 2014; Zhang et al., 2017; Razlutskij et al., 2021; Mei et al., 2021). Furthermore, water flow and wave action typical for natural lake ecosystems were not accounted for in our experiments. However, there are calm, fishless or fish poor aquatic ecosystems, where conditions are similar to those simulated here.
The implications are that in similar natural systems, expected increase in temperature resulting from climate change will be conducive to the growth of planktonic algae and enhance their competitive advantage over benthic algae, leading to increased potential for eutrophication and deteriorating water quality. However, further studies at larger scale conditions and different trophic state and trophic structure are needed before general conclusions can be drawn about the effect of climate change on the benthic-pelagic coupling.
In conclusion, in our experimental system simulating shallow lake ecosystems, elevated temperature increased the growth of planktonic algae and enhanced their competitive advantage over the benthic algae, thereby contributing to eutrophication and a decline in water quality.
The original contributions presented in the study are included in the article/Supplementary Material, further inquiries can be directed to the corresponding author.
Conceptualization: XM, XZ, and ZL Investigation: XM, SG, YL, and JH Funding acquisition XM Writing original draft: XM and SG. Writing; editing: XM, VR, LR, EJ, ZL, and XZ.
This research was supported by the National Natural Science Foundation of China (No. 41771100; 42011530017) and the Chinese-Belarusian Joint Project of Belarussian Republican Foundation for Fundamental Research (B18KI-007). EJ was supported by the Tübitak program BIDEB2232 (project 118C250).
The authors declare that the research was conducted in the absence of any commercial or financial relationships that could be construed as a potential conflict of interest.
All claims expressed in this article are solely those of the authors and do not necessarily represent those of their affiliated organizations, or those of the publisher, the editors and the reviewers. Any product that may be evaluated in this article, or claim that may be made by its manufacturer, is not guaranteed or endorsed by the publisher.
American Public Health Association (APHA) (1998). Standard Methods for the Examination of Water and Wastewater. 20th ed. Washington, DC, USA: American Public Health Association.
Amundrud, S. L., and Srivastava, D. S. (2019). Disentangling How Climate Change Can Affect an Aquatic Food Web by Combining Multiple Experimental Approaches. Glob. Change Biol. 25, 3528–3538. doi:10.1111/gcb.14717
Blottière, L., Jaffar-Bandjee, M., Jacquet, S., Millot, A., and Hulot, F. D. (2017). Effects of Mixing on the Pelagic Food Web in Shallow Lakes. Freshw. Biol. 62, 161–177. doi:10.1111/fwb.12859
Butterwick, C., Heaney, S. I., and Talling, J. F. (2010). Diversity in the Influence of Temperature on the Growth Rates of Freshwater Algae, and its Ecological Relevance. Freshw. Biol. 50, 291–300. doi:10.1111/j.1365-2427.2004.01317.x
Chen, M., Fan, M., Liu, R., Wang, X., Yuan, X., and Zhu, H. (2015). The Dynamics of Temperature and Light on the Growth of Phytoplankton. J. Theor. Biol. 385, 8–19. doi:10.1016/j.jtbi.2015.07.039
Claquin, P., Probert, I., Lefebvre, S., and Veron, B. (2008). Effects of Temperature on Photosynthetic Parameters and TEP Production in Eight Species of Marine Microalgae. Aquat. Microb. Ecol. 51, 1–11. doi:10.3354/ame01187
Daufresne, M., Lengfellner, K., and Sommer, U. (2009). Global Warming Benefits the Small in Aquatic Ecosystems. Proc. Natl. Acad. Sci. U.S.A. 106, 12788–12793. doi:10.1073/pnas.0902080106
Flöder, S., Combüchen, A., Pasternak, A., and Hillebrand, H. (2006). Competition between Pelagic and Benthic Microalgae for Phosphorus and Light. Aquat. Sci. 68, 425–433. doi:10.1007/s00027-006-0824-7
Genkai-Kato, M., Vadeboncoeur, Y., Liboriussen, L., and Jeppesen, E. (2012). Benthic-Planktonic Coupling, Regime Shifts, and Whole-Lake Primary Production in Shallow Lakes. Ecology 93, 619–631. doi:10.1890/10-2126.1
Gobiet, A., Kotlarski, S., Beniston, M., Heinrich, G., Rajczak, J., and Stoffel, M. (2014). 21st Century Climate Change in the European Alps-A Review. Sci. Total Environ. 493, 1138–1151. doi:10.1016/j.scitotenv.2013.07.050
Hetherington, A. L., Schneider, R. L., Rudstam, L. G., Gal, G., Degaetano, A. T., and Walter, M. T. (2015). Modeling Climate Change Impacts on the Thermal Dynamics of Polymictic Oneida Lake, New York, United States. Ecol. Model. 300, 1–11. doi:10.1016/j.ecolmodel.2014.12.018
IPCC (2018). “Summary for Policymakers,” in Global Warming of 1.5°C. An IPCC Special Report on the Impacts of Global Warming of 1.5°C Above Pre-Industrial Levels and Related Global Greenhouse Gas Emission Pathways, in the Context of Strengthening the Global Response to the Threat of Climate Change, Sustainable Development, and Efforts to Eradicate Poverty. Editors V. Masson-Delmotte, P. Zhai, H.-O. Pörtner, D. Roberts, J. Skeaet al. Geneva, Switzerland: World Meteorological Organization
Jäger, C. G., and Diehl, S. (2014). Resource Competition across Habitat Boundaries: Asymmetric Interactions between Benthic and Pelagic Producers. Ecol. Monogr. 84, 287–302. doi:10.1890/13-0613.1
Jensen, H. S., and Andersen, F. O. (1992). Importance of Temperature, Nitrate, and pH for Phosphate Release from Aerobic Sediments of Four Shallow, Eutrophic Lakes. Limnol. Oceanogr. 37, 577–589. doi:10.4319/lo.1992.37.3.0577
Jeppesen, E., Meerhoff, M., Holmgren, K., González-Bergonzoni, I., Teixeira-de Mello, F., Declerck, S. A. J., et al. (2010). Impacts of Climate Warming on Lake Fish Community Structure and Potential Effects on Ecosystem Function. Hydrobiologia 646, 73–90. doi:10.1007/s10750-010-0171-5
Jespersen, A. M., and Christoffersen, K. (1987). Measurements of Chlorophyll a from Phytoplankton Using Ethanol as Extraction Solvent. Arch. Hydrobiol. 109, 445–454.
Li, W., Xu, X., Fujibayashi, M., Niu, Q., Tanaka, N., and Nishimura, O. (2016). Response of Microalgae to Elevated CO2 and Temperature: Impact of Climate Change on Freshwater Ecosystems. Environ. Sci. Pollut. Res. 23, 19847–19860. doi:10.1007/s11356-016-7180-5
Liu, C., Zhang, L., Fan, C., Xu, F., Chen, K., and Gu, X. (2017). Temporal Occurrence and Sources of Persistent Organic Pollutants in Suspended Particulate Matter from the Most Heavily Polluted River Mouth of Lake Chaohu, China. Chemosphere 174, 39–45. doi:10.1016/j.chemosphere.2017.01.082
Malmaeus, J. M., Blenckner, T., Markensten, H., and Persson, I. (2006). Lake Phosphorus Dynamics and Climate Warming: A Mechanistic Model Approach. Ecol. Model. 190, 1–14. doi:10.1016/j.ecolmodel.2005.03.017
Marcus, N. H., and Marcus, N. H. (1998). Minireview: The Importance of Benthic-Pelagic Coupling and the Forgotten Role of Life Cycles in Coastal Aquatic Systems. Limnol. Oceanogr. 43, 763–768. doi:10.4319/lo.1998.43.5.0763
Mei, X., Razlutskij, V., Rudstam, L. G., Jeppesen, E., Tang, Y., et al. (2021). Effects of Omnivorous Fish on Benthic-Pelagic Habitats Coupling in Shallow Aquatic Ecosystems: A Minireview. J. Lake Sci. 33, 667–674. doi:10.18307/2021.0304
Nicholls, K. H. (1999). Effects of Temperature and Other Factors on Summer Phosphorus in the Inner Bay of Quinte, Lake Ontario: Implications for Climate Warming. J. Gt. Lakes. Res. 25, 250–262. doi:10.1016/S0380-1330(99)70734-3
O'Reilly, C. M., Sharma, S., Gray, D. K., Hampton, S. E., Read, J. S., Rowley, R. J., et al. (2015). Rapid and Highly Variable Warming of Lake Surface Waters Around the Globe. Geophys. Res. Lett. 42, 10773–10781. doi:10.1002/2015GL066235
Ozaki, N., Fukushima, T., Harasawa, H., Kojiri, T., Kawashima, K., and Ono, M. (2003). Statistical Analyses on the Effects of Air Temperature Fluctuations on River Water Qualities. Hydrol. Process. 17, 2837–2853. doi:10.1002/hyp.1437
Paerl, H. W., Hall, N. S., and Calandrino, E. S. (2011). Controlling Harmful Cyanobacterial Blooms in a World Experiencing Anthropogenic and Climatic-Induced Change. Sci. Total Environ. 409, 1739–1745. doi:10.1016/j.scitotenv.2011.02.001
Paerl, H. W., and Huisman, J. (2008). Blooms like it Hot. Science 320, 57–58. doi:10.1126/science.1155398
Pasternak, A., Hillebrand, H., and Flöder, S. (2009). Competition between Benthic and Pelagic Microalgae for Phosphorus and Light - Long-Term Experiments Using Artificial Substrates. Aquat. Sci. 71, 238–249. doi:10.1007/s00027-009-9143-0
Pedersen, M., and Borum, J. (1996). Nutrient Control of Algal Growth in Estuarine Waters. Nutrient Limitation and the Importance of Nitrogen Requirements and Nitrogen Storage Among Phytoplankton and Species of Macroalgae. Mar. Ecol. Prog. Ser. 142, 261–272. doi:10.3354/meps142261
Qu, X., Mei, X., Razlutskij, V., Rudstam, L. G., and Zhang, X. (2019). Effects of Common Carp (Cyprinus carpio) on Water Quality in Aquatic Ecosystems Dominated by Submerged Plants: a Mesocosm Study. Knowl. Manag. Aquat. Ec. 28, 7. doi:10.1051/kmae/2019017
Razlutskij, V., Mei, X., Maisak, N., Sysova, E., Lukashanets, D., Makaranka, A., et al. (2021). Omnivorous Carp (Carassius gibelio) Increase Eutrophication in Part by Preventing Development of Large-Bodied Zooplankton and Submerged Macrophytes. Water 13 (11), 1497. doi:10.3390/w13111497
Spears, B. M., Carvalho, L., Perkins, R., and Paterson, D. M. (2008). Effects of Light on Sediment Nutrient Flux and Water Column Nutrient Stoichiometry in a Shallow Lake. Water Res. 42, 977–986. doi:10.1016/j.watres.2007.09.012
Takano, K., and Hino, S. (2000). Effect of Temperature and Soluble Reactive Phosphorus on Abundance of Aphanizomenon Flos-aquae (Cyanophyceae). Phycol. Res. 48, 9–13. doi:10.1046/j.1440-1835.2000.00180.x
Thorel, M., Fauchot, J., Morelle, J., Raimbault, V., Le Roy, B., Miossec, C., et al. (2014). Interactive Effects of Irradiance and Temperature on Growth and Domoic Acid Production of the Toxic Diatom Pseudo-nitzschia Australis (Bacillariophyceae). Harmful. Algae. 39, 232–241. doi:10.1016/j.hal.2014.07.010
Vadeboncoeur, Y., Jeppesen, E., Zanden, M. J. V., Schierup, H.-H., Christoffersen, K., and Lodge, D. M. (2003). From Greenland to Green Lakes: Cultural Eutrophication and the Loss of Benthic Pathways in Lakes. Limnol. Oceanogr. 48, 1408–1418. doi:10.2307/359746410.4319/lo.2003.48.4.1408
van Vliet, M. T. H., Franssen, W. H. P., Yearsley, J. R., Ludwig, F., Haddeland, I., Lettenmaier, D. P., et al. (2013). Global River Discharge and Water Temperature under Climate Change. Glob. Environ. Change 23, 450–464. doi:10.1016/j.gloenvcha.2012.11.002
Zhang, P., Zhai, C., Wang, X., Liu, C., Jiang, J., and Xue, Y. (2013). Growth Competition between Microcystis Aeruginosa and Quadrigula Chodatii under Controlled Conditions. J. Appl. Phycol. 25, 555–565. doi:10.1007/s10811-012-9890-5
Zhang, X., Liu, Z., Gulati, R. D., and Jeppesen, E. (2013). The Effect of Benthic Algae on Phosphorus Exchange between Sediment and Overlying Water in Shallow Lakes: a Microcosm Study Using 32P as a Tracer. Hydrobiologia 710, 109–116. doi:10.1007/s10750-012-1134-9
Zhang, X., Liu, Z., Jeppesen, E., and Taylor, W. D. (2014). Effects of Deposit-Feeding Tubificid Worms and Filter-Feeding Bivalves on Benthic-Pelagic Coupling: Implications for the Restoration of Eutrophic Shallow Lakes. Water Res. 50, 135–146. doi:10.1016/j.watres.2013.12.003
Zhang, X., Liu, Z., Jeppesen, E., Taylor, W. D., and Rudstam, L. G. (2016). Effects of Benthic-Feeding Common Carp and Filter-Feeding Silver Carp on Benthic-Pelagic Coupling: Implications for Shallow Lake Management. Ecol. Eng. 88, 256–264. doi:10.1016/j.ecoleng.2015.12.039
Zhang, X., Mei, X., and Gulati, R. D. (2017). Effects of Omnivorous Tilapia on Water Turbidity and Primary Production Dynamics in Shallow Lakes: Implications for Ecosystem Management. Rev. Fish. Biol. Fish. 27, 245–254. doi:10.1007/s11160-016-9458-6
Zhang, X., Mei, X., Gulati, R. D., and Liu, Z. (2015). Effects of N and P Enrichment on Competition between Phytoplankton and Benthic Algae in Shallow Lakes: A Mesocosm Study. Environ. Sci. Pollut. Res. 22, 4418–4424. doi:10.1007/s11356-014-3680-3
Keywords: elevated temperature, planktonic algae, benthic algae, aquatic ecosystem, nutrient
Citation: Mei X, Gao S, Liu Y, Hu J, Razlustkij V, Rudstam LG, Jeppesen E, Liu Z and Zhang X (2022) Effects of Elevated Temperature on Resources Competition of Nutrient and Light Between Benthic and Planktonic Algae. Front. Environ. Sci. 10:908088. doi: 10.3389/fenvs.2022.908088
Received: 30 March 2022; Accepted: 22 April 2022;
Published: 12 May 2022.
Edited by:
Jaan H. Pu, University of Bradford, United KingdomReviewed by:
Mohammad Amir Khan, Galgotias Educational Institutions, IndiaCopyright © 2022 Mei, Gao, Liu, Hu, Razlustkij, Rudstam, Jeppesen, Liu and Zhang. This is an open-access article distributed under the terms of the Creative Commons Attribution License (CC BY). The use, distribution or reproduction in other forums is permitted, provided the original author(s) and the copyright owner(s) are credited and that the original publication in this journal is cited, in accordance with accepted academic practice. No use, distribution or reproduction is permitted which does not comply with these terms.
*Correspondence: Xiufeng Zhang, d2V0bGFuZHhmekAxNjMuY29t
†These authors have contributed equally to this work
Disclaimer: All claims expressed in this article are solely those of the authors and do not necessarily represent those of their affiliated organizations, or those of the publisher, the editors and the reviewers. Any product that may be evaluated in this article or claim that may be made by its manufacturer is not guaranteed or endorsed by the publisher.
Research integrity at Frontiers
Learn more about the work of our research integrity team to safeguard the quality of each article we publish.