- 1State Key Laboratory of Soil and Sustainable Agriculture, Institute of Soil Science, Chinese Academy of Sciences, Nanjing, China
- 2University of Chinese Academy of Sciences, Beijing, China
Excessive application of phosphate fertilizer is common in vegetable fields and causes deterioration of the rhizosphere environment, that is, the soil oxygen (O2) environment, which further constrains root morphology construction and limits vegetable yield. Nevertheless, the interaction between root morphology and the response of the rhizosphere O2 environment to vegetable P utilization has rarely been reported. Therefore, we carried out an experiment applying different concentrations of O2 generator, 10%, 30%, 50%, and 80% urea hydrogen peroxide (as pure nitrogen) instead of urea as a top dressing in the rhizosphere, to study the effect on root morphology and P adsorption, and its mechanism. We found that there were O2-deficient and P-deficient zones in the rhizosphere, and oxygenation could alleviate the rhizosphere O2 and P consumption in roots. The rhizosphere O2 concentration was maintained at approximately 250.6 μmol L−1, which significantly promoted total root length, root volume, average diameter, and root activity by 29.0%, 30.9%, 3.9%, and 111.2%, respectively. Oxygenation promoted organic P mineralization and increased the Olsen-P content in the rhizosphere. The characteristics of root morphology and increased available P in the rhizosphere jointly contributed to high P absorption and utilization, and the P use efficiency was improved by 9.3% and the shoot P accumulation by 10.9% in the 30% urea hydrogen peroxide treatment compared with CK. Moreover, this treatment also improved yield and quality, including vitamin C and the soluble sugar content. However, at a still higher O2 concentration (260.8 μmol L−1), vegetable growth exhibited O2 damage, resulting in reduced yield and quality. Our study provided new insights into constructing efficient root morphology by regulating the rhizosphere O2 environment to improve vegetable yield and quality, as well as to increase P use efficiency in vegetable fields.
Introduction
Driven by economic growth, China has undergone a rapid increase in vegetable production: the area devoted to vegetables has been increased from 3.3 million hm2 in 1976 to 21.5 million hm2 in 2020, which now accounts for 12.8% of the farmland area in China (NBSC 2020). In intensive vegetable production systems, farmers often apply large amounts of fertilizer, especially phosphate fertilizers, to obtain high yields (Liang et al., 2013). The nitrogen (N) fertilization applied to vegetables was 1.26 times that of upland crops per season in China, while the phosphorus (P) fertilization of vegetable fields was 1.85 times that of upland crops per season in China (Wang et al., 2019). Based on the published field studies in China, the P fertilizer applied in greenhouse (571 kg P hm−2) and open-field (117 kg P hm−2) vegetable systems exceeded the P removal in harvested vegetables (44 and 25 kg P hm−2 in greenhouse and open-field vegetable systems, respectively) per season, and organic P application accounted for half of the total P fertilization (Yan et al., 2014). However, most P can be fixed by iron and manganese oxides or locked up in other insoluble forms (Hao et al., 2002; McBeath et al., 2005). The root system, as the main interface between the plant and soil, is important for nutrient and water uptake, and storage (Tian et al., 2014). Vegetable roots are characterized by comparatively low root length densities and a shallow distribution, thus requiring high soil P concentrations in the root zone for optimal production (Yan et al., 2014). However, the diffusion of P to the vegetable root zone is the major way for P absorption; since P uptake occurs at a much higher rate than P diffusion within the root-zone soil, a P depletion zone is quickly established (Morgan and Connolly 2013). Therefore, there is a spatial dislocation between P uptake of vegetable root and P supply, which cannot be completely solved by a large amount of P fertilizer applied.
Moreover, massive and repeated P fertilization could lead to soil compaction because the phosphate ions combine with cations such as Al3+, Fe3+, Ca2+, and Mg2+ in the soil to form insoluble phosphates, and the decreased cation content in the soil solution decreases the soil structure stability. Long-term intensive tillage, such as high-density planting and a high cropping index, also causes soil compaction. Soil compaction reduces the soil air capacity (Schnurr-Putz et al., 2006). The degradation of organic matter accompanied by high consumption of O2 further decreases the soil O2 content since massive amounts of organic fertilizer are customarily applied in vegetable fields (Ratering and Schnell 2001; Li et al., 2014). Indeed, the phenomenon of O2 limitation occurs even within seemingly aerobic, well-drained soils. The lack of soil O2 directly affects root growth because root growth is sensitive to O2 deficiency, which reduces root elongation (Aguilar et al., 2003). The root growth of wheat is suppressed, and yield is substantially decreased when soil O2 levels are below the critical level (Meyer et al., 1985). The poor mobility of P in the soil, accompanied by the decreased P uptake ability resulting from the poor growth of the root system caused by hypoxia, further reduces P use efficiency (PUE) and yield. Therefore, the rhizosphere O2 environment is crucial to efficient P uptake, and vegetables might not reach their maximum biological potential in poor rhizosphere environments, that is, at low O2 concentrations.
Soil aeration is considered the third most important factor, after water and nutrient availability, in affecting soil fertility (Ben-Noah et al., 2021) and accelerating the yield of crops and vegetables (Zhu et al., 2015; Li et al., 2016a; Li et al., 2020). Several techniques for aeration of the root zone have been developed, including physical and chemical oxygenation. Physical oxygenation usually includes pumping pressurized air or sucking air (bubbles) into the irrigation water (Goorahoo et al., 2002; Bhattarai et al., 2004; Bhattarai et al., 2006; Abuarab et al., 2013). Chemical oxygenation is achieved by adding various peroxides (usually considered a kind of O2 fertilizer), such as H2O2, urea hydrogen peroxide (UHP), and potassium peroxide, into the soil or irrigation water (Bryce et al., 1982; Bhattarai et al., 2004; Urrestarazu and Mazuela, 2005). Most studies have concentrated on physical oxygenation in vegetable production (Goorahoo et al., 2002; Li et al., 2016b). However, the larger scale use of physical oxygenation has been limited by oxygenation equipment, non-uniform aeration in the field, and the limited time supersaturated O2 remains dissolved in irrigation water (Lei et al., 2016). Oxygen fertilizer is convenient and targeted, providing O2 in the root zone by direct application to the rhizosphere. Numerous studies have concentrated on the use of O2 fertilizer in flooded conditions, especially the use of UHP in paddy fields. UHP is an environmentally friendly hydrogen peroxide that provides higher O2 concentrations than physical oxygenation by irrigation water venting (Frankenberger, 1997). It has been proven that UHP can increase rice yields by 3.1%–11.5% compared with the control (Zhao et al., 2010). However, there have been few studies on the effect of UHP on the P uptake of vegetables. Thus, the experiments in this study were carried out to study the effect and mechanism of UHP applied to the rhizosphere as an oxygen generator on vegetable yield and P adsorption to realize efficient utilization of vegetable phosphate fertilizer.
Materials and Methods
Soil Collection
Soil samples were collected from open vegetable fields where Chinese cabbage had been cultivated for 7 years in Wuxi, Jiangsu Province (China) (31°23′ N, 119°58′ E). The region has a subtropical monsoon climate with a mean air temperature of 15.6 C and an annual precipitation of 1,100 mm. The soil properties were as follows: 15.6 g kg−1 soil organic matter (SOM), 0.89 g kg−1 total nitrogen (TN), 0.82 g kg−1 total phosphorus (TP), 119.2 mg kg−1 Olsen-P, and 6.35 pH (with a soil: water ratio of 1:2.5). The soil samples were air-dried, ground, sieved through a 0.85-mm mesh, and stored for further incubation experiments.
Experimental Design and Sample Collection
The experiment was carried out to study the effect of optimizing urea hydrogen peroxide (UHP) input on amaranth (A. mangostanus) growth. Based on our pre-experiments, the UHP concentration gradient included five treatments: no UHP(CK), 10% UHP (UHP1), 30% UHP (UHP2), 50% UHP (UHP3), and 80% UHP (UHP4), as a replacement for urea, according to the principle of equal N fertilization in top dressing. A 2.5-kg sample of soil mixed thoroughly with urea (80 mg N kg−1, 50% of the total N fertilization), CaP2H4O8 (80 mg P2O5 kg−1), and K2SO4 (120 mg K2O kg−1) as a base fertilizer, according to the conventional amounts used by the local farmers, was used to fill each pot. All pots were moistened with tap water and equilibrated for 24 h before sowing amaranth by the direct-seeding method. The remaining N fertilizer (50% N) was divided equally and applied twice as top dressing at 13 days and 23 days after sowing. The detailed fertilization strategy is shown in Table 1. UHP was applied in aqueous solution injected by a syringe into the rhizosphere (around the root base) at a depth of 4 cm.
Amaranth seeds were immersed in NaClO (1%) for 20 min and then washed thoroughly with tap water. The seeds were then immersed in water for 3 h before being placed on a moist filter paper to germinate at 25 C overnight in darkness. Fifteen seeds were sown in each pot, and 2-week-old seedlings were thinned to eight with similar growth. To exclude the influence of amaranth roots, one more treatment without amaranth planting was defined as bulk soil. CKbulk represents the treatment without amaranth growth and UHP addition, which reflects the background soil O2 concentration. The moisture content in the soil was maintained at 60% of the field water-holding capacity using daily distilled water replenishment. Sampling was performed at 20 days (seedling stage), 30 days (growth period, 7 days after the first UHP application), and 45 days (harvest, 7 days after the second UHP application) of amaranth growth. The fresh weights of amaranth shoots and roots were determined, and the materials were dried at 105 C for 30 min and then to constant weight at 75 C for 48 h to determine the dry weight. The rhizosphere soil of the amaranth root was gathered by removing the loose soil and collecting the remaining soil that was tightly adhered to the roots, which was then air-dried for chemical analysis.
Soil Property Analysis
Soil pH was measured using a pH meter (Beckman, Inc. in California) with a soil: water ratio of 1:2.5, inorganic P (Pi) was determined by H2SO4 extraction and followed by the Mo–Sb anti-spectrophotometric method, and organic P (PO) was measured by the ignition method (Bao, 2000); Olsen-P was extracted with 0.5 mol L−1 NaHCO3 (pH 8.5) for 30 min on an orbital shaker (180 rpm, 25 C) at a soil solution ratio of 1:20 (Olsen et al., 1954); total P (TP) was measured using the HClO4–H2SO4 digestion and Mo–Sb anti-spectrophotometric methods (Liu, 1996). The P activation coefficient (PAC) was the ratio of Olsen-P to TP, which reflected the soil P availability and was an important indicator of soil fertility (Wu et al., 2017).
Root Morphology and Aboveground Growth Parameters
Root morphological parameters, including total root length, root surface area, root volume, and average diameter, were analyzed using root analysis instrument WinRhizo-LA1600 (Regent Instruments Inc., Quebec, QC, Canada). The aboveground growth was monitored by shoot height, dry weight, and leaf fresh weight (yield). The specific root length (SRL) was calculated as the fresh length of the root sample divided by its dry mass (m g−1). The root activity was monitored using the triphenyltetrazolium chloride (TTC) method (Lindström and Nyström, 1987). TTC is reduced by dehydrogenases, and the dehydrogenase activity is regarded as an indicator of the root activity (Li et al., 2011). The P concentration in shoots was determined after digestion with a mixture of 5 ml of concentrated sulfuric acid and 8 ml of 30% v/v H2O2, followed by spectrophotometric analysis using the molybdovanado phosphate method (UVmini-1240; Shimadzu, Kyoto, Japan) (Johnson and Ulrich, 1959). P accumulation (mg pot−1) and P use efficiency (PUE) (g DW g−1) were calculated by Eq. 1 and Eq. 2. The concentration of leaf soluble sugars was determined using the anthrone method for spectrophotometric determination (Fales, 1951). Ascorbic acid (vitamin C) was measured using the titration method with 2,6-dichloroindophenol (Commission, 2016). The chlorophyll content index (CCI) values of leaves were quantified using a handheld CCM-200 chlorophyll meter (Opti-Sciences, USA), which is a reliable method for estimating chlorophyll contents (Silla et al., 2010).
In Situ Measurements of Soil O2 Concentration
The O2 microelectrode, a miniaturized Clark-type O2 electrode with a guard cathode (OX 50, ø = 40–60 μm, Unisense, Aarhus, Denmark) (Revsbech, 1989), was used to measure soil O2 concentration in situ at harvest (45 days of amaranth growth). The sampling plant for each replication pot was chosen randomly, and the soil O2 profile measurements were performed in the center of the range at a horizontal distance of 1 cm from the plant root base with a depth of 4 cm for all treatments. A micromanipulator (MM33-2, Unisense) with a motor controller (MC-232, Unisense) was used and fixed in a lab stand (LS18, Unisense) to avoid shaking during the microelectrode movement, and treatment was performed with a 1 mm depth interval measurement, and the periods for “wait before measure” and “measure” were both set to 5 s.
Data Analysis
Data were analyzed statistically by statistical software program SPSS version 20 (SPSS Inc., Chicago, IL, USA). Means were compared using one-way analysis of variance (ANOVA) with Duncan’s multiple-range test. Significant differences (p < 0.05) between treatments are indicated by different letters in the figure and table legends. Graphs were produced using Origin Pro 2021.
Results
Distribution of the Soil O2 Concentration
The distribution of the soil O2 concentration in the rhizosphere soil was used to clarify the aeration effect of UHP application. Although certain values at certain depths showed abnormal peaks, the O2 concentration decreased slowly with increasing soil depth and increased with increasing UHP concentration (Figure 1). The average values were approximately 233.4, 242.9, 250.6, 254.3, and 260.8 μmol L−1 in the CK, UHP1, UHP2, UHP3, and UHP4 treatments, respectively (Figure 1). The soil O2 concentration under the CKbulk treatment (no UHP applied and without amaranth growth) was significantly higher than that of the CK treatment (Figure 1). Consumption of O2 by the amaranth root resulted in an “O2-deficient zone” in the rhizosphere.
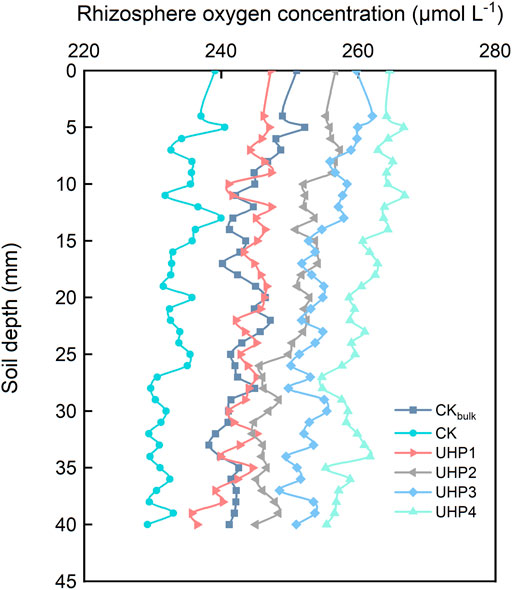
FIGURE 1. Representative graph showing the O2 profiles of the rhizosphere soil (4 cm away from the root surface) harvested at 25°C. CKbulk represents no UHP application and no amaranth growth; CK represents no UHP application. 3 mm from the soil surface was defined as “0” point. The O2 profiles obtained in each replication of the same treatment were generally similar, and results of triple averaging are shown.
Effects of Aeration on the Growth, P Accumulation, and PUE of Amaranth
The shoot dry weight of the UHP2 treatment was significantly higher than that of CK, especially at 20 days, when it was 25.1% higher than that of CK; 30 days, when it was 20.1% higher; and 45 days, when it was 21.0% higher. However, the shoot dry weight showed significant decrease in the UHP3 and UHP4 treatments compared with CK (Figure 2A). The shoot height of all the plants under the UHP1, UHP2, and UHP3 treatments was higher than that under the CK treatment, and the tallest plants were grown under the UHP2 treatment, with an increase of 17.7% compared with CK at harvest (Figure 2B), while there were no significant differences among the UHP3, UHP4, and CK treatments (Figure 2B). The P accumulation in the shoots of the UHP2 treatments was significantly higher than that in CK, with increases of 40.7% at 20 days, 13.4% at 30 days, and 10.9% at 45 days (Figure 2C). The differences in shoot PUE among different treatments at 20 days were not prominent (Figure 2D); PUE in the shoots of UHP2 was significantly increased by 5.8% at 30 days and 9.3% at 45 days compared with that in CK (p < 0.05) (Figure 2D).
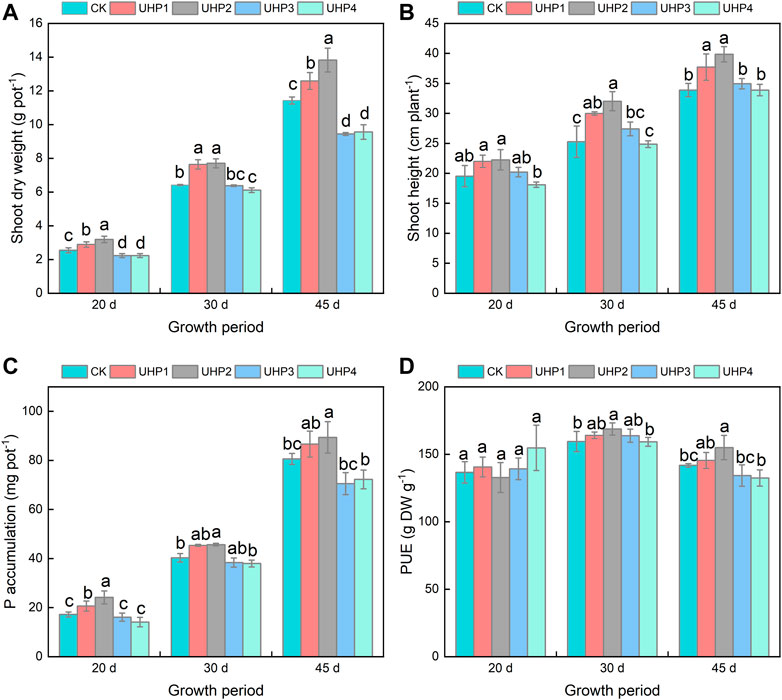
FIGURE 2. Effects of aeration on the growth, absorption, and utilization of P in amaranth at different growth stages. (A) Shoot dry weight, (B) shoot height, (C) P accumulation, and (D) phosphorus use efficiency (PUE). Bars indicate mean ± SD. Different letters on the bars indicate a significant difference (p < 0.05) between different treatments in the same growth period.
Effects of Aeration on the Root Architecture and Activity of Amaranth at Different Growth Stages
The UHP2 treatment resulted in larger root systems than the other treatments, especially total root length and surface area. The total root length under the UHP2 treatment was significantly increased by 38.5% at 20 days, 56.1% at 30 days, and 29.0% at 45 days compared with the CK (p < 0.05) (Table 2). UHP2 treatment also significantly promoted the root surface area by 60.1% at 20 days, 4.5% at 30 days, and 4.1% at 45 days compared with CK (p < 0.05) (Table 2). In contrast, all root indices under the UHP3 and UHP4 treatments were significantly lower than those under CK (p < 0.05), especially root length, which was 19.4% and 39.0% lower than that under CK at 45 days, respectively (Table 2). The specific root length (SRL) in the UHP2 treatment was significantly enhanced by 17.9% at 20 days, 48.9% at 30 days, and 13.8% at 45 days compared with CK (Table 2). We further measured the root activity of different treatments at harvest, indicating that UHP2 treatment had the highest promotional effect, and the root activity was significantly enhanced by 111.2% compared with the CK treatment, while UHP4 treatment decreased root activity by 38.7% compared to CK (Figure 3).
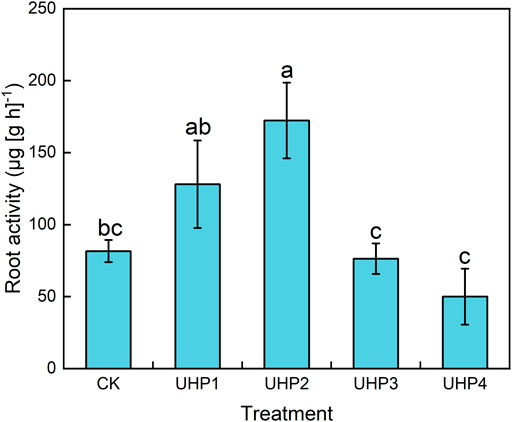
FIGURE 3. Effects of aeration on the root activity of amaranth at harvest. Bars indicate the mean ± SD. Different letters on the bars indicate significant differences (p < 0.05).
Effects of Aeration on Amaranth Yield and Quality
The highest yield and the best quality were both observed in the UHP2 treatment compared with the CK treatment (p < 0.05). The yield of the UHP2 treatment was 12.6% higher than that of CK (Figure 4A). However, the UHP3 and UHP4 treatments exhibited significant inhibitory effects on amaranth yield, which was 8.1% and 11.7% lower than that of CK, respectively (Figure 4A). The soluble sugar contents of the UHP2 treatment were significantly higher than those of CK by 21.1%, and there was no significant difference between other UHP treatments and CK (Figure 4B). The ascorbic acid content of the UHP1, UHP2, and UHP3 treatments increased by 14.6%, 16.1%, and 8.9%, respectively, compared with CK, while it decreased by 5.2% in the UHP4 treatment (Figure 4C). The CCI value of the UHP2 treatment was significantly greater than that of CK by 54.8% (Figure 4D).
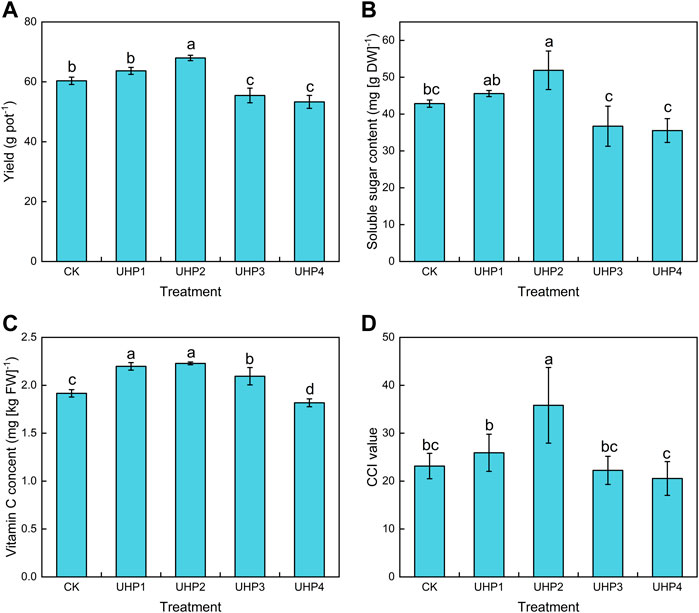
FIGURE 4. Effects of aeration on amaranth yield and quality at harvest. (A) Yield, (B) soluble sugar content, (C) ascorbic acid (vitamin C) content, and (D) chlorophyll content index (CCI). Bars indicate mean ± SD. Different letters on the bars indicate a significant difference between different treatments.
Effects of Aeration on Rhizosphere P Turnover of Amaranth at Different Growth Stages
To investigate the effect of UHP addition on soil P turnover, several main nutritional parameters of soil, including rhizosphere and bulk soil (without vegetable planting), were measured, and the results are shown in Figure 5. The nutrient contents in the bulk soil, including Olsen-P and Pi, were much higher than those in the rhizosphere soil, and P turnover showed an obvious “rhizosphere effect” (Figure 5). Olsen-P, Pi, and PAC showed upward trends as the UHP concentration increased. The Olsen-P contents of all aeration treatments were higher than those of CK, with an increase of 7.4%–13.5% in rhizosphere soil and 6.9%–10.0% in bulk soil (Figure 5C). The Pi contents in the bulk soil were not significantly different among the aeration treatments but were significantly higher than those in CK (Figures 5D–F). In the rhizosphere soil, the Pi content was significantly increased with the addition of UHP at 20 days and 45 days, and the highest content was observed under the UHP4 treatment, with a gap of 3.5% and 5.1% at 20 and 45 days, respectively, compared with CK (Figures 5D,F). Po decreased with the application of UHP, which was opposite to the change in the Pi content. No significant differences in the Po content in the bulk soil were observed between aeration treatments and CK at 30 days and 40 days (Figures 5H,I), but the UHP2, UHP3, and UHP4 treatments had significantly lower Po content than CK at 20 days by 18.3%, 20.8%, and 21.7%, respectively (Figure 5G). Oxygenation had a greater effect on PAC in the rhizosphere than in the bulk soil, especially at harvest, which increased by 7.1%–21.4% compared with that of CK (Figure 5L).
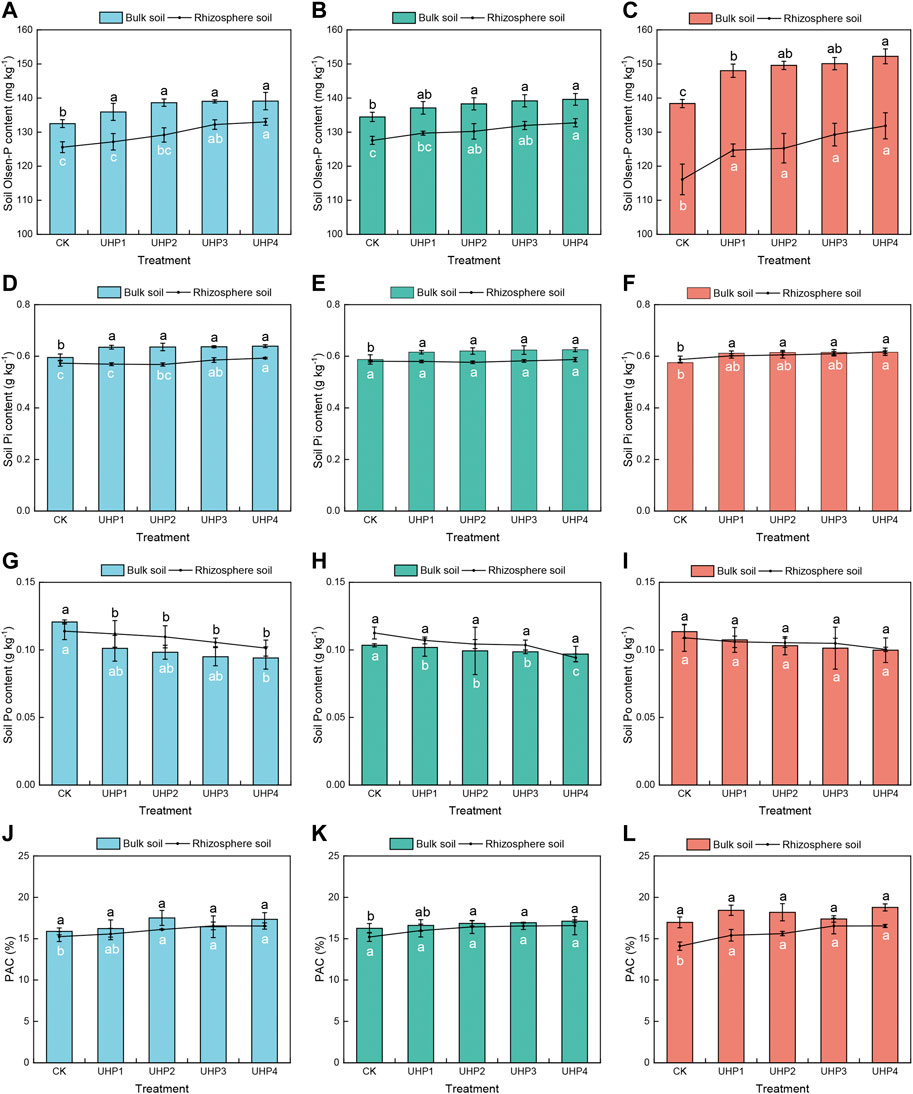
FIGURE 5. Effects of aeration on the P turnover of amaranth rhizosphere soil and bulk soil at different growth stages. (A) Soil Olsen-P content at 20 days, (B) soil Olsen-P content at 30 days, (C) soil Olsen-P content at 45 days, (D) soil inorganic P (Pi) content at 20 days, (E) soil Pi content at 30 days, (F) soil Pi at 45 days, (G) soil organic P (Po) content at 20 days, (H) soil Po content at 30 days, (I) soil Po at 45 days, (J) P activation coefficient (PAC) at 20 days, (K) PAC content at 30 days, and (L) PAC at 45 days. Black letters represent significant differences in bulk soil, while white letters represent rhizosphere soil. Bars indicate mean ± SD. Different letters on the bars indicate a significant difference between different treatments.
Discussion
Alleviation of the Rhizosphere O2-Deficient Zone Coupled With the P-Deficient Zone Under Oxygenation
The soil oxygenation status results from the equilibrium between the physical processes of gas transport between the atmosphere and the soil pores, and the biological processes of O2 consumption and CO2 accumulation (Brzezińska et al., 2001). O2 consumption is caused by root and soil organism respiration; it consumes several tons of O2 per hectare and per year under field conditions; and the presence of plants may elevate O2 consumption more than twofold (Stępniewski, 2011). The dissolved O2 concentration in the soil was approximately 243.6 μmol L−1 without amaranth plants, which was higher than the corresponding control (233.4 μmol L−1 with amaranth) in this study (Figure 1). This confirmed that there was an O2-deficient zone in the rhizosphere soil. There was also a significant change in the rhizosphere ventilation environment with UHP addition, and the dissolved O2 concentration increased with increasing UHP concentration. Soil is heterogeneous with vertical differentiation of the oxygenation status, and the O2 concentration gradually decreases with soil depth (Stepniewski and Stepniewski, 2009), which was also confirmed by this research (Figure 1).
Similar to the rhizosphere anoxic zone, there was also a P-deficient zone in the rhizosphere. Olsen-P in the rhizosphere was lower than that in the corresponding bulk soil, and the phenomenon of P deficiency became increasingly serious with vegetable growth (Figures 5A–C). Indeed, there still existed a relatively P-deficient zone in the rhizosphere, although the bulk soil P maintained a high concentration due to the strong absorption of P by vegetable roots. Oxygenation could alleviate P deficiency in the rhizosphere, promote P turnover, and increase available P in the rhizosphere (Figure 5). Aerobic microorganisms are more active in well-aerated soils, and more nutrients can be released for plants to absorb (Bhattarai, Su et al., 2005). Research has proven that aeration promotes the abundance of microorganisms related to phosphate solubilization, that is, Pseudomonas and Aspergillus (Zhao et al., 2019), which can solubilize insoluble Pi and mineralize Po to available P (Zhang et al., 2018). The reduction in Po compared to Pi (Figures 5D–I) suggested the conversion of Po to Pi, and Pi was the main form for root acquisition by the Pi transporter at plasma membranes (Liao et al., 2019). Increasing the soil air content could effectively increase soil enzyme activity, such as phosphatases (Li et al., 2016b). Soil phosphatase activity directly affects the transformation and utilization of Po (Dodor and Tabatabai, 2003; Wang et al., 2013). The ratio of Olsen-P to TP is defined as the PAC, which was an important indicator of soil fertility, and higher PAC in soils could stimulate plant growth (Xiao et al., 2012; Wu et al., 2017). In this study, the PACs were greater than 2.0% (Figure 5J-L), which was usually defined as the threshold for P effectiveness and bioavailability (Xiao et al., 2012), indicating that soil P was sufficient for plant growth. Furthermore, PAC was higher with UHP added than that in CK treatment (Figure 5J-L), indicating that aeration could accelerate the P transformation process and provide more available P for vegetable growth. Further studies would be conducted for the effect of oxygenation on microbial, as well as enzymatic activity and thus on P turnover processes.
Root Morphology Construction and P-Acquisition Strategies Under Oxygenation
Soil aeration is essential to the normal growth and development of crops. Vegetable roots are the first plant tissue to perceive soil O2 changes and then grow toward the “air-rich” zone (Bhattarai et al., 2005), while there exists reduced root elongation in O2-deficient environment (Aguilar et al., 2003). Compared with the CK treatment, the UHP2 treatment significantly increased the total root length, root surface area, root diameter, and root volume (Table 2), especially root activity at harvest (Figure 3). In other words, increasing O2 supply in the rhizosphere could promote earlier root development. The significant effect of UHP2 treatment on root growth and root activity might be one of the reasons for promoting the formation of amaranth biomass. The yield of the UHP2 treatment increased 12.6% compared with CK (Figure 4A). H2O2 was injected through subsurface drip irrigation at the rate of 5 L hm−2 into a zucchini crop (Cucurbita pepo), and the fruit yield, fruit number, and shoot weight increased by 25%, 29%, and 24%, respectively, compared to the control (Bhattarai et al., 2004). However, it was not the case where higher O2 concentrations led to better plant growth. The total root length, surface area, volume, and average diameter were significantly reduced under the UHP3 and UHP4 treatments compared with CK (p < 0.05) (Table 2). Dunand et al. (2007) found that a high concentration of H2O2 considerably reduced Arabidopsis root length, and the root hardly developed when supplied with 1 mmol L−1 H2O2. A high concentration of UHP damaged root growth due to its strong oxidizing properties, resulting in growth inhibition. Treatment with UHP3 and UHP4 inhibited amaranth growth, and the yield decreased by 8.1% and 11.7%, respectively, compared with those in CK (Figure 4A). The increased O2 concentration could promote the aerobic respiration of vegetables, but under a continuous high O2 environment, amaranth showed different degrees of O2 damage, which affected growth and metabolism. However, a high concentration of UHP damaged root growth (Table 2) due to its strong oxidizing properties, resulting in growth inhibition. Therefore, changes in root morphological characteristics in response to the rhizosphere O2 environment might be one of the main reasons for the difference in amaranth biomass.
Moderately enhanced rhizosphere O2 levels not only were conducive to the development of a more extensive root system but also influenced the P acquisition ability of roots. The P accumulation of amaranth increased significantly under the UHP2 treatment compared with the CK treatment (Figure 2C), and PUE was also significantly higher than that in CK at 30 days and 45 days (Figure 2D). The significant gains in P accumulation in the UHP2 treatment might be attributed to root morphological strategies to increase P acquisition and increase the vigor of root systems. Thicker roots could secrete greater amounts of P-mobilizing exudates to improve soil P availability, like high carboxylate exudation (Wen, Li et al., 2019), and reduced the roots turnover rate to help root effectively maintain P nutrition (De la Fuente Cantó et al., 2020). A larger root length and surface area were observed in the UHP2 treatment (Table 2), which could increase the larger P foraging area and lead to a higher P uptake capacity (Liu, 2021). The root system responds to the changes of O2 environment in the rhizosphere mainly by adjusting root length, therefore leading to changes in SRL. High SRL facilitates faster growth through more rapid acquisition of soil resources (Kramer-Walter et al., 2016). Therefore, moderately enhanced rhizosphere O2 levels (250.6 μmol L−1) could enhance the P bioavailability by increasing the P-mobilizing exudates of thicker roots, expand root foraging for P, and improve the root metabolism, thereby realizing the efficient absorption of P. Improving P acquisition and utilization could enhance P efficiency (Wang et al., 2010), which might be the root P acquisition strategy of PUE increased in the UHP2 treatment (Figure 2D). Therefore, building a well-developed root system is crucial for increasing PUE in vegetable fields.
Improving Vegetable Quality by Regulating the Rhizosphere O2 Environment
Vegetables are rich in essential nutrients such as vitamins and amino acids, and people are increasingly concerned about vegetable quality and safety as living standards improve. Aeration in the rhizosphere could improve the rhizosphere soil O2 environment and physiological metabolism, and has a certain promoting effect on vegetable quality. Vitamin C and soluble sugars, two beneficial substances for the human body, were significantly enhanced under the UHP2 treatment (Figures 4B,C). Root hypoxia led to decreased contents of ascorbic acid (vitamin C), and the low levels of ascorbate might result from a modification of the leaf hormone status caused by disturbed root function (Horchani et al., 2008). The ascorbic acid concentration is reported to be the highest in mature leaves with highest chlorophyll (Gill and Tuteja, 2010), and hypoxia caused leaf epinasty and reduced photosynthetic activity (Pezeshki, 2001). Therefore, the VC content first increased and then decreased with increasing UHP concentration in this study, which was consistent with the change in the CCI value. The CCI value indicated a close positive correlation with the chlorophyll content in leaves (Richardson et al., 2002) and was used to characterize the relative chlorophyll content. Chlorophyll is the main substance that absorbs light energy in plant photosynthesis, and its content was closely related to the photosynthetic rate of plants. A reduction in the chlorophyll content would affect photosynthetic performance and dry matter accumulation, decreasing yield reduction (Figures 4A,D). Soluble sugar content reflects dry matter accumulation, the soluble sugar content of UHP2 treatment was significantly higher than that of CK (Figure 4B), and the dry matter accumulation in leaf tissue by promoting photosynthesis with elevated O2 was another important factor in the increased yield.
Data Availability Statement
The raw data supporting the conclusions of this article will be made available by the authors, without undue reservation.
Author Contributions
RW conducted the experiments, analyzed the data, and wrote the manuscript. YL designed the study and revised the manuscript. WS supervised the manuscript. All authors have read and agreed to the published version of the manuscript.
Funding
This work was supported by the National Natural Science Foundation of China (No. 31872957; 31471948).
Conflict of Interest
The authors declare that the research was conducted in the absence of any commercial or financial relationships that could be construed as a potential conflict of interest.
Publisher’s Note
All claims expressed in this article are solely those of the authors and do not necessarily represent those of their affiliated organizations, or those of the publisher, the editors, and the reviewers. Any product that may be evaluated in this article, or claim that may be made by its manufacturer, is not guaranteed or endorsed by the publisher.
References
Abuarab, M., Mostafa, E., and Ibrahim, M. (2013). Effect of Air Injection under Subsurface Drip Irrigation on Yield and Water Use Efficiency of Corn in a Sandy Clay Loam Soil. J. Adv. Res. 4, 493–499. doi:10.1016/j.jare.2012.08.009
Aguilar, E. A., Turner, D. W., Gibbs, D. J., Armstrong, W., and Sivasithamparam, K. (2003). Oxygen Distribution and Movement, Respiration and Nutrient Loading in Banana Roots (Musa Spp. L.) Subjected to Aerated and Oxygen-Depleted Environments. Plant Soil 253 (1), 91–102. doi:10.1023/a:1024598319404
Ben-Noah, I., Nitsan, I., Cohen, B., Kaplan, G., and Friedman, S. P. (2021). Soil Aeration Using Air Injection in a Citrus Orchard with Shallow Groundwater. Agric. Water Manag. 245, 106664. doi:10.1016/j.agwat.2020.106664
Bhattarai, S. P., Huber, S., and Midmore, D. J. (2004). Aerated Subsurface Irrigation Water Gives Growth and Yield Benefits to Zucchini, Vegetable Soybean and Cotton in Heavy Clay Soils. Ann. Appl. Biol. 144, 285–298. doi:10.1111/j.1744-7348.2004.tb00344.x
Bhattarai, S. P., Pendergast, L., and Midmore, D. J. (2006). Root Aeration Improves Yield and Water Use Efficiency of Tomato in Heavy Clay and Saline Soils. Sci. Hortic. 108, 278–288. doi:10.1016/j.scienta.2006.02.011
Bhattarai, S. P., Su, N., and Midmore, D. J. (2005). Oxygation Unlocks Yield Potentials of Crops in Oxygen‐Limited Soil Environments. Adv. Agron. 88, 313–377. doi:10.1016/s0065-2113(05)88008-3
Bryce, J. H., Focht, D. D., and Stolzy, L. H. (1982). Soil Aeration and Plant Growth Response to Urea Peroxide Fertilization. Soil Sci. 134 (2), 111–116. doi:10.1097/00010694-198208000-00005
Brzezińska, M., Stępniewski, W., Stępniewska, Z., Przywara, G., and Włodarczyk, T. (2001). Effect of Oxygen Deficiency on Soil Dehydrogenase Activity in a Pot Experiment with Triticale Cv. Jago vegetation. Int. Agrophysics 15, 145
Commission, N. H. a. F. P. (2016). “National Food Safety Standard.” in Determination of Ascorbic Acid in Food (GB/T 5009.86—2016). Beijing: China Standard Press, 7
De la Fuente Cantó, C., Simonin, M., King, E., Moulin, L., Bennett, M. J., Castrillo, G., et al. (2020). An Extended Root Phenotype: the Rhizosphere, its Formation and Impacts on Plant Fitness. Plant J. 103, 951. doi:10.1111/tpj.14781
Dodor, D. E., and Tabatabai, M. A. (2003). Effect of Cropping Systems on Phosphatases in Soils. Z. Pflanzenernähr. Bodenk. 166 (1), 7–13. doi:10.1002/jpln.200390016
Dunand, C., Crèvecoeur, M., and Penel, C. (2007). Distribution of Superoxide and Hydrogen Peroxide in Arabidopsis Root and Their Influence on Root Development: Possible Interaction with Peroxidases. New Phytol. 174, 332–341. doi:10.1111/j.1469-8137.2007.01995.x
Fales, F. (1951). The Assimilation and Degradation of Carbohydrates by Yeast Cells. J. Biol. Chem. 193 (1), 113–124. doi:10.1016/s0021-9258(19)52433-4
Frankenberger, W. T. (1997). Factors Affecting the Fate of Urea Peroxide Added to Soil. Bull. Environ. Contam. Toxicol. 59, 50–57. doi:10.1007/s001289900442
Gill, S. S., and Tuteja, N. (2010). Reactive Oxygen Species and Antioxidant Machinery in Abiotic Stress Tolerance in Crop Plants. Plant Physiology Biochem. 48, 909–930. doi:10.1016/j.plaphy.2010.08.016
Goorahoo, D., Carstensen, G., Zoldoske, D. F., Norum, E., and Mazzei, A. (2002). Using Air in Sub-surface Drip Irrigation (SDI) to Increase Yields in Bell Peppers. Int. Water Irrig. 22, 39
Hao, X., Cho, C. M., Racz, G. J., and Chang, C. (2002). Chemical Retardation of Phosphate Diffusion in an Acid Soil as Affected by Liming. Nutrient Cycl. Agroecosyst. 64, 213–224. doi:10.1023/a:1021470824083
Horchani, F., Gallusci, P., Baldet, P., Cabasson, C., Maucourt, M., Rolin, D., et al. (2008). Prolonged Root Hypoxia Induces Ammonium Accumulation and Decreases the Nutritional Quality of Tomato Fruits. J. Plant Physiology 165 (13), 1352–1359. doi:10.1016/j.jplph.2007.10.016
Johnson, C. M., and Ulrich, A. (1959). Analytical Methods for Use in Plant Analysis. Berkeley, CA: USA Agricultural Experiment Station University of California.
Kramer-Walter, K. R., Bellingham, P. J., Millar, T. R., Smissen, R. D., Richardson, S. J., and Laughlin, D. C. (2016). Root Traits Are Multidimensional: Specific Root Length Is Independent from Root Tissue Density and the Plant Economic Spectrum. J. Ecol. 104, 1299–1310. doi:10.1111/1365-2745.12562
Lei, H., Bhattarai, S., Balsys, R., Midmore, D. J., Holmes, T., and Zimmerman, W. (2016). Temporal and Spatial Dimension of Dissolved Oxygen Saturation with Fluidic Oscillator and Mazzei Air Injector in Soil-Less Irrigation Systems. Irrig. Sci. 34, 421–430. doi:10.1007/s00271-016-0512-x
Li, Y., Niu, W., Cao, X., Zhang, M., and Zhang, J. Z. (2020). Growth Response of Greenhouse-Produced Muskmelon and Tomato to Sub-surface Drip Irrigation and Soil Aeration Management Factors. BMC Plant Biol. 20, 141. doi:10.1186/s12870-020-02346-y
Li, Y., Niu, W. Q., Wang, J. W., Xu, J., Zhang, M. Z., and Li, K. Y. (2016a). Review on Advances of Airjection Irrigation. Int. J. Agric. Biol. Eng. 9 (2), 1. doi:10.3965/j.ijabe.20160902.1361
Li, Y., Niu, W., Wang, J., Liu, L., Zhang, M., and Xu, J. (2016b). Effects of Artificial Soil Aeration Volume and Frequency on Soil Enzyme Activity and Microbial Abundance when Cultivating Greenhouse Tomato. Soil Sci. Soc. Am. J. 80, 1208–1221. doi:10.2136/sssaj2016.06.0164
Li, Y., Shi, W., and Wang, X. (2014). New Insights into How Increases in Fertility Improve the Growth of Rice at the Seedling Stage in Red Soil Regions of Subtropical China. Plos One 9 (10), e109161. doi:10.1371/journal.pone.0109161
Li, Z.-j., Xie, X.-y., Zhang, S.-q., and Liang, Y.-c. (2011). Negative Effects of Oxytetracycline on Wheat (Triticum aestivum L.) Growth, Root Activity, Photosynthesis, and Chlorophyll Contents. Agric. Sci. China 10 (10), 1545–1553. doi:10.1016/s1671-2927(11)60150-8
Liang, L. Z., Zhao, X. Q., Yi, X. Y., Chen, Z. C., Dong, X. Y., Chen, R. F., et al. (2013). Excessive Application of Nitrogen and Phosphorus Fertilizers Induces Soil Acidification and Phosphorus Enrichment during Vegetable Production in Yangtze River Delta, China. Soil Use Manage 29 (2), 161–168. doi:10.1111/sum.12035
Liao, Y.-Y., Li, J.-L., Pan, R.-L., and Chiou, T.-J. (2019). Structure-Function Analysis Reveals Amino Acid Residues of Arabidopsis Phosphate Transporter AtPHT1;1 Crucial for its Activity. Front. Plant Sci. 10, 1158. doi:10.3389/fpls.2019.01158
Lindström, A., and Nyström, C. (1987). Seasonal Variation in Root Hardiness in Container Grown Scots Pine, Norway Spruce, and Lodgepole Pine Seedlings. Can. J. For. Res. 17, 787. doi:10.1139/x87-126
Liu, D. (2021). Root Developmental Responses to Phosphorus Nutrition. J. Integr. Plant Biol. 63, 1065–1090. doi:10.1111/jipb.13090
Liu, G. S. (1996). Soil Physical and Chemcial Analysis & Description of Soil Profiles. Beijing: Standards Press of China.
McBeath, T. M., Armstrong, R. D., Lombi, E., McLaughlin, M. J., and Holloway, R. E. (2005). Responsiveness of Wheat (Triticum aestivum) to Liquid and Granular Phosphorus Fertilisers in Southern Australian Soils. Soil Res. 43, 203–212. doi:10.1071/sr04066
Meyer, W., Barrs, H., Smith, R., White, N., Heritage, A., and Short, D. (1985). Effect of Irrigation on Soil Oxygen Status and Root and Shoot Growth of Wheat in a Clay Soil. Aust. J. Agric. Res. 36 (2), 171–185. doi:10.1071/ar9850171
Morgan, J. B., and Connolly, E. L. (2013). Plant-soil Interactions: Nutrient Uptake. Nat. Educ. Knowl. 4 (8), 2.
NBSC (2020). National Bureau of Statistics of China. National Data Available at: https://data.stats.gov.cn/english/.
Olsen, S. R., Cole, C. V., Watanabe, F. S., Dean, L. A., Watanabe, F., and Dean, L. (1954). Estimation of Available Phosphorus in Soils by Extraction with Sodium Bicarbonate. Washington, DC: US Department of Agriculture.
Pezeshki, S. R. (2001). Wetland Plant Responses to Soil Flooding. Environ. Exp. Bot. 46 (3), 299–312. doi:10.1016/s0098-8472(01)00107-1
Ratering, S., and Schnell, S. (2001). Nitrate-dependent Iron(II) Oxidation in Paddy Soil. Environ. Microbiol. 3 (2), 100–109. doi:10.1046/j.1462-2920.2001.00163.x
Revsbech, N. P. (1989). An Oxygen Microsensor with a Guard Cathode. Limnol. Oceanogr. 34, 474–478. doi:10.4319/lo.1989.34.2.0474
Richardson, A. D., Duigan, S. P., and Berlyn, G. P. (2002). An Evaluation of Noninvasive Methods to Estimate Foliar Chlorophyll Content. New phytol. 153 (1), 185–194. doi:10.1046/j.0028-646x.2001.00289.x
Schnurr-Pütz, S., Bååth, E., Guggenberger, G., Drake, H. L., and Küsel, K. (2006). Compaction of Forest Soil by Logging Machinery Favours Occurrence of Prokaryotes. FEMS Microbiol. Ecol. 58 (3), 503–516. doi:10.1111/j.1574-6941.2006.00175.x
Silla, F., González-Gil, A., González-Molina, M. E., Mediavilla, S., and Escudero, A. (2010). Estimation of Chlorophyll in Quercus Leaves Using a Portable Chlorophyll Meter: Effects of Species and Leaf Age. Ann. For. Sci. 67, 108. doi:10.1051/forest/2009093
Stepniewski, W., and Stepniewski, Z. (2009). Selected Oxygen-Dependent Process Response to Soil Management and Tillage. Soil Tillage Res. 102 (2), 193. doi:10.1016/j.still.2008.07.006
Tian, H., De Smet, I., and Ding, Z. (2014). Shaping a Root System: Regulating Lateral versus Primary Root Growth. Trends Plant Sci. 19 (7), 426–431. doi:10.1016/j.tplants.2014.01.007
Urrestarazu, M., and Mazuela, P. C. (2005). Effect of Slow-Release Oxygen Supply by Fertigation on Horticultural Crops under Soilless Culture. Sci. Hortic. 106, 484–490. doi:10.1016/j.scienta.2005.05.010
Wang, R., Min, J., Kronzucker, H. J., Li, Y., and Shi, W. (2019). N and P Runoff Losses in China's Vegetable Production Systems: Loss Characteristics, Impact, and Management Practices. Sci. Total Environ. 663, 971–979. doi:10.1016/j.scitotenv.2019.01.368
Wang, X., Shen, J., and Liao, H. (2010). Acquisition or Utilization, Which Is More Critical for Enhancing Phosphorus Efficiency in Modern Crops? Plant Sci. 179 (4), 302–306. doi:10.1016/j.plantsci.2010.06.007
Wang, Y., Chi, S.-y., Ning, T.-y., Tian, S.-z., and Li, Z.-j. (2013). Coupling Effects of Irrigation and Phosphorus Fertilizer Applications on Phosphorus Uptake and Use Efficiency of Winter Wheat. J. Integr. Agric. 12 (2), 263–272. doi:10.1016/s2095-3119(13)60225-7
Wen, Z., Li, H., Shen, Q., Tang, X., Xiong, C., Li, H., et al. (2019). Tradeoffs Among Root Morphology, Exudation and Mycorrhizal Symbioses for Phosphorus‐acquisition Strategies of 16 Crop Species. New Phytol. 223 (2), 882–895. doi:10.1111/nph.15833
Wu, Q., Zhang, S., Zhu, P., Huang, S., Wang, B., Zhao, L., et al. (2017). Characterizing Differences in the Phosphorus Activation Coefficient of Three Typical Cropland Soils and the Influencing Factors under Long-Term Fertilization. PLoS ONE 12 (5), e0176437. doi:10.1371/journal.pone.0176437
Xiao, R., Bai, J., Gao, H., Huang, L., and Deng, W. (2012). Spatial Distribution of Phosphorus in Marsh Soils of a Typical Land/inland Water Ecotone along a Hydrological Gradient. CATENA 98, 96–103. doi:10.1016/j.catena.2012.06.008
Yan, Z., Liu, P., Li, Y., Ma, L., Alva, A., Dou, Z., et al. (2014). Phosphorus in China's Intensive Vegetable Production Systems: Overfertilization, Soil Enrichment, and Environmental Implications. J. Environ. Qual. 42 (4), 982–989. doi:10.2134/jeq2012.0463
Zhang, L., Ding, X., Peng, Y., George, T. S., and Feng, G. (2018). Closing the Loop on Phosphorus Loss from Intensive Agricultural Soil: A Microbial Immobilization Solution? Front. Microbiol. 9, 104. doi:10.3389/fmicb.2018.00104
Zhao, F., Sun, J., Yu, S., Liu, H., and Yu, K. (2019). Aeration Irrigation Can Improve Growth of Table Grape Cv. Red Globe (Vitis vinifera L.) in GreenhouseRed Globe (Vitis vinifera L.) in Greenhouse. Hort Sci. 54 (4), 732–737. doi:10.21273/hortsci13732-18
Zhao, F., Wang, D.-Y., Xu, C.-M., Zhang, W.-J., Li, F.-B., Mao, H.-J., et al. (2010). Response of Morphological, Physiological and Yield Characteristics of Rice (Oryza Sativa L.) to Different Oxygen-Increasing Patterns in Rhizosphere. Acta. Agronomica. Sinica. 36 (2), 303–312. doi:10.3724/sp.j.1006.2010.00303
Keywords: aeration, vegetable, phosphorus, root morphology, urea hydrogen peroxide
Citation: Wang R, Shi W and Li Y (2022) Link Between Aeration in the Rhizosphere and P-Acquisition Strategies: Constructing Efficient Vegetable Root Morphology. Front. Environ. Sci. 10:906893. doi: 10.3389/fenvs.2022.906893
Received: 29 March 2022; Accepted: 19 April 2022;
Published: 26 May 2022.
Edited by:
Haigang Li, Inner Mongolia Agricultural University, ChinaReviewed by:
Zhihui Wen, China Agricultural University, ChinaLin Zhang, China Agricultural University, China
Copyright © 2022 Wang, Shi and Li. This is an open-access article distributed under the terms of the Creative Commons Attribution License (CC BY). The use, distribution or reproduction in other forums is permitted, provided the original author(s) and the copyright owner(s) are credited and that the original publication in this journal is cited, in accordance with accepted academic practice. No use, distribution or reproduction is permitted which does not comply with these terms.
*Correspondence: Yilin Li, ylli@issas.ac.cn