- Grassland Ecosystem Key Laboratory of Ministry of Education, Sino-U.S. Research Centers for Sustainable Grassland and Livestock Management, Grassland Science College of Gansu Agricultural University, Lanzhou, China
Plant phenotypic and reproductive plasticity is strongly influenced by long-term grazing activities. It is important to understand the life history of dominant plant species, such as Kobresia humilis of alpine meadow, for the stability and sustainable grazing administration meadow on the Qinghai-Tibetan Plateau (QTP). We compared the effects of different grazing treatments (grazing yak, Y; grazing Tibetan sheep, S; and grazing Tibetan sheep and yak, S+Y) on the reproductive and phenotypic plasticity of K. humilis in an alpine meadows on the northeastern margin of the Qilian Mountains in China. The results showed that different grazing treatments had significantly effects on the K. humilis phenotype and reproductive plasticity. The Y treatment significantly reduced the plant height, crown width and K. humilis biomass, but increased the density, which was 1.27 and 1.53 times higher than that in the S+Y and S treatments, respectively. Further, the S+Y treatment significantly increased the crown width, biomass, and future life expectancy of K. humilis. Whereas the S treatment increased the height of K. humilis significantly, which was 1.57 and 1.10 times higher than that in the Y and S+Y treatments, respectively. Both Y and S treatments significantly increased the sexual reproduction efficacy of K. humilis but reduced the storage efficacy. The storage efficacy at S+Y treatment was highest among these treatments. Further, grazing treatments did not change the resource allocation strategy of K. humilis, while the sexual reproductive efficacy was significantly higher than the vegetative reproduction efficacy. The storage efficacy was significantly higher than the growth efficacy among the different grazing treatments. The increase of Cyperaceae indicates the degradation of Cyperaceae—Poaceae meadows. This study showed that grazing Tibetan sheep is a more sustainable grazing method in cold season pastures of alpine meadows on the QTP.
1 Introduction
The Qinghai-Tibet Plateau (QTP) lies between 26° and 39° N and 73°–104° E, most of which are located in southwestern in China. It is not only an important ecological barrier, but also a vital pastoral area. Grazing has existed for thousands of years as the main interference in the alpine meadows on QTP (Huang et al., 2016). In recent years, the alpine meadows have been degraded to varying degrees due to overgrazing (Dai et al., 2021; Wang et al., 2021; Zhang et al., 2021). In response to grazing pressures and environmental factors, phenotypic and reproductive plasticity allows plants to regulate their life history strategies (Sommer, 2020). Previous studies have shown that asexual reproduction facilitates the expansion of populations and the full utilization of environmental resources due to a faster reproduction rate (Pereira and Coimbra, 2019). Sexual reproduction increases the genetic diversity of the plant community and increases the adaptability of the offspring to changing environmental conditions (Dembicz et al., 2018; Wilson Sayres, 2018). Comparing the differential effects of different grazing livestock combinations on the reproduction of dominant forages can provide the basis for grassland grazing management.
The role of grazing in vegetation community succession has been widely studied. Many studies have concluded that grazing affects the vegetation community composition by influencing plant regeneration rate, photosynthetic rate, biomass, and plant diversity, and is an important factor in the community succession (Li X. et al., 2018; Sigcha et al., 2018; Török et al., 2018; Schmitz and Isselstein, 2020; Rysiak et al., 2021). However, few studies have explored vegetation community changes from the perspective of the reproductive strategies of dominant species. Peng Z. et al. (2020) suggested that grazing and rainfall showed a bidirectional regulation of reproductive characteristics of K. humilis. Sexual reproductive index, plant height, and the number of single leaves decreased with decreasing rainfall and increasing trampling intensity (Peng Z. et al., 2020). Xiao et al. (2018) found that trampling by yaks and Tibetan sheep during grazing was the main cause of grass differentiation, and the high hoof pressure of yaks was more detrimental to grass growth (Xiao et al., 2018). Pan et al. (2021) showed that under grazing conditions, the trampling period had the greatest effect on morphological traits of K. humilis, followed by livestock species. Understanding the reproductive strategies of dominant species in alpine meadows can help determine the relationship between livestock population and the ecology of the region. This is also important for understanding the ecological adaptations of plant populations to different livestock grazing.
Kobresia humilis is an important dominant species in alpine meadow. By studying the reproductive and phenotypic plasticity of K. humilis, we determined the impact of grazing treatments on K. humilis populations and Cyperaceae—Poaceae meadow. The specific objectives of this study were to 1) investigate the ways in which grazing treatments affect the vegetative reproduction and asexual reproduction of K. humilis; and 2) select grazing practices that are more suitable for the sustainable use of alpine meadows on the QTP. We hypothesized that: 1) grazing treatments significantly affected the reproductive strategy of K. humilis through the regulation of phenotypic plasticity; and 2) grazing yaks increased sexual reproduction by reducing the storage efficacy of K. humilis.
2 Materials and Methods
2.1 Study Site
The experimental site was located near the Tianzhu Alpine Grassland Ecosystem Experiment Station on the northeastern edge of the QTP in Zhuxixulong Township, Tianzhu Tibetan Autonomous County, Wuwei City, northwestern Gansu Province, China (Figure 1) (Li W. et al., 2018). The experimental site at an altitude of about 2960 m. Its geographical coordinates are 102°40′-102°47′ E longitude and 36°31′-37°55′ N latitude. The climate of the region is characterized by cold and wet conditions, intense sunshine, and large diurnal temperature differences. The summer monsoon brings higher temperatures and precipitation. The average annual temperature ranges between −0.3°C and 0°C. Meanwhile, the cumulative annual temperatures greater than 0°C and 10°C are 1581°C and 1026°C, respectively. The annual precipitation was 416 mm, concentrated in the growing season. The annual evaporation was 1590 mm, four times as much as the precipitation. These data were obtained in 2021. The soils of the study area belong to the alpine Chernozem according to the soil classification proposed by Spaargaren and Deckers (1998). According to the classification of soil types in China, the soil in this region is alpine meadow soil (Bai et al., 2022).
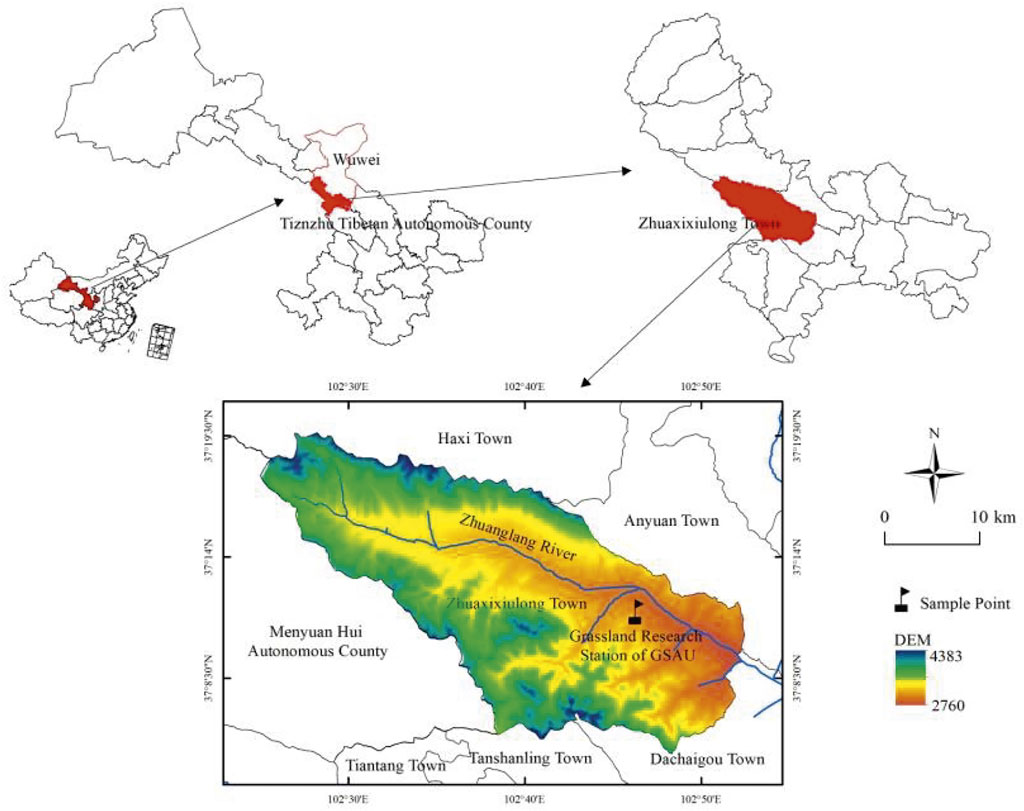
FIGURE 1. Schematic diagram of the experimental site. *Administrative division data were obtained from the Resource and Environment Data Center of the Chinese Academy of Sciences (http://www.resdc.cn), and digital elevation model data, with a spatial resolution of 30 m, were obtained from the Geospatial Data Cloud (http://www.gscloud.cn/).
2.2 Experimental Design
In this study, we selected three connected grasslands of the same type to ensure consistency in soil and vegetation before the grazing treatments were conducted while also conducting spot experiments with grazing treatments. The three pastures were divided into three grazing treatments, namely, grazing yaks (Y), Tibetan sheep and yaks (S+Y), and Tibetan sheep (S). These are cold season pastures, and the grazing time is from October to May of the next year. The grasslands were extensively distributed, and each experimental plot was enclosed by a 1.5 m-high wire fence. In the three plots, a total of 12 squares of 1 m × 1 m were set up. Please refer to Figure 1 for the location of the sample plot.
No non-grazing treatments were used in this study. There are substantial financial costs and logistical difficulties associated with managing Tibetan sheep and yak grazing in repeated grasslands. Therefore, in this study, the experiment was designed using a pseudo-replicate sampling method developed for practicality, meaning samples from the same treatments were sampled in the same meadow. However, the sampling areas in each meadow were sufficiently large, with Y, S+Y, and S measuring 11.33, 10.00, and 18.67 ha, respectively. According to Oksanen (2001), the experimental design used here is statistically sound, thus, the results are reliable. According to a survey of local households conducted during sampling, grazing was continually done for 39 years, i.e., from 1983 to 2021. Grazing rates were not significantly different for each grazing plot (Table 1). Additionally, Figure 2 shows the biomass of the grassland functional group in 2021. The soil nutrients are showed in Table 2. The density of rodents is very low and has no negative impact on the grassland on the meadow.
Different capital letters indicate significant differences in grassland functional group biomass among the treatments (p < 0.05).
2.3 Sample Collection and Measurement Analysis
2.3.1 Sample Collection
K. humilis samples were collected in September 2021, according to the method described by Ren (1998). From a total of 15 samples, 30 representative plants were selected randomly; thus, 150 plants were sampled from each plot, and 450 plants in total were sampled from the three sample plots. The spikes of K. humilis were individually cut, stored in a paper bag, complete plants were subsequently dug up, clean the soil from the roots, then transported to the laboratory in paper bags. The samples were placed in an electro-thermostatic blast oven (Hengzi, GZX-GF101-3-BS- II, Shanghai, China) and were later subjected to 105°C for 0.5 h to acquire dead samples. Subsequently, the samples were dried at 65°C until constant weight was attained and then measured for indices in the next step.
2.3.2 Measurement Indexes and Related Calculations
Plant height: Height from the ground to the plant top.
Crown width: Crown diameter of the plant.
Plant density: Number of K. humilis plants per m2.
Reproductive branches density: Number of branches with spikes per m2.
Reproductive branch height: Height of branches with spikes.
Nutrient branch density: Number of branches without spikes per m2.
Spike length: Length from the base to the tip of the spike.
Number of seeds: Number of seeds on each spike.
Seed size: Length, width, and height of the seeds measured using Vernier calipers.
Effective spike ratio: Proportion of spikes with ≥5 seeds (Yan and Hou, 2018).
The biomass, sexual reproduction efficacy, vegetative reproduction potency, storage efficacy, growth potency, seed yield, and effective spike number ratio were calculated for each trait. The calculation methods are showed in Table 3.
2.4 Data Analysis
Before the data analysis, we performed the normal distribution equal variance test on the relevant data and found that the assumptions were satisfied. One-way analysis of variance was used to analyze significant differences between the different grazing treatments. All data were presented as mean ± standard deviation. SAS was used for all statistical analysis (100 SAS Campus Drive Cary, NC 27513-2414, United States) and Microsoft Excel 2012 (Microsoft Corp., Redmond, WA, United States) was used for graphical representations. The levels of significance were set at p < 0.05.
3 Results
3.1 Effect of Grazing Treatments on Phenotypic Plasticity of K. humilis
The Figures 3A–C shows the effects of grazing treatments on plant height, crown width, and the density of K. humilis, respectively. The Y treatment significantly reduced the plant height (p < 0.05) by 29.93% and 36.20% compared to the S+Y and S treatments, respectively. The maximum height recorded was 14.2 cm in the S treatment, which was significantly higher than that in the other treatments (p < 0.05). The K. humilis crown width was significantly higher in the S+Y treatment (8.8 cm) than that in the Y treatment (5.9 cm) and S treatment (6.3 cm; p < 0.05; Figure 3B). Further, the K. humilis density significantly differed between treatments (p < 0.05), with the highest density in the Y treatment (24.6 individuals/m2) and the lowest density observed in the S treatment (15.84 individuals/m2; Figure 3C).
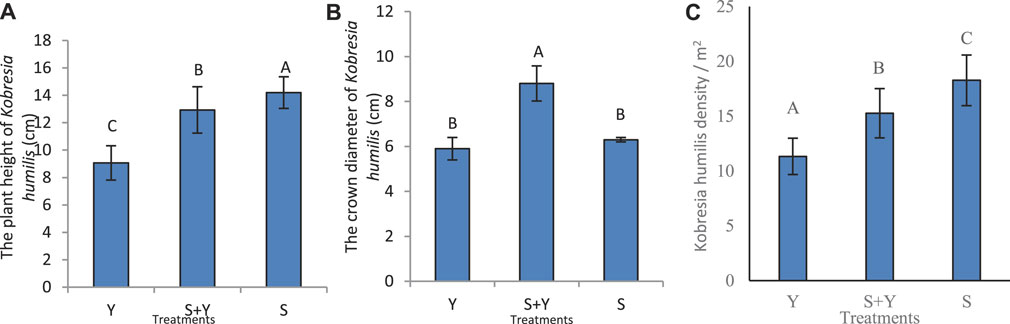
FIGURE 3. Effect of grazing methods on the phenotypic plasticity of K. humilis with the (A) response of plant height, (B) canopy width, and (C) density per m2. Different capital letters in the figure indicate significant differences between grazing methods (p < 0.05).
Figure 4 illustrates the distribution of K. humilis resources among traits due to the grazing treatments. Treatments significantly affected the biomass of K. humilis, with the following trend: S+Y > Y > S (p < 0.05). The biomass of the reproductive branches was significantly higher in the S+Y treatment than in the other treatments (p < 0.05), and significantly higher in the Y treatment than in the S treatment (p < 0.05). Seed biomass was the highest in the S+Y treatment and was not significantly different between the Y and S treatments (p > 0.05), with values ranging from 0.0032 g (Y treatment) to 0.0039 g (S treatment).
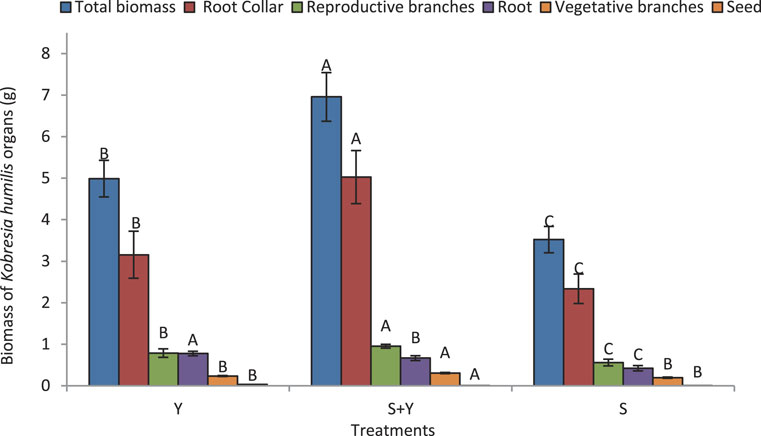
FIGURE 4. Effect of grazing methods on material partitioning patterns of K. humilis. Capital letters indicate significant differences in the biomass of each trait under grazing methods (p < 0.05).
3.2 Effects of Grazing Treatments on the Reproductive Plasticity of K. humilis
3.2.1 Effect of Grazing Treatments on the Sexual Reproductive Characteristics of K. humilis
The grazing treatments caused significant differences (p < 0.05) in the reproductive branch density of K. humilis, with a maximum (248.33 per m2) and minimum (67.5 per m2) values occurring in the S+Y and S treatments, while it was 195.83 per m2 in the Y treatment (Figure 5A). The plants in the S treatment shows a spike length of 1.52 cm (Figure 5C), which was significantly higher than that observed in the S+Y and Y treatments (p < 0.05). Compared to the different treatments, the Y treatment significantly increased the effective spike ratio (Figure 5D), which was significantly higher than that of the other treatments (p < 0.05); the ratio in the S treatment was slightly lower, while the effective spike ratio in the S+Y treatment was significantly lower than that of the other treatments (p < 0.05).
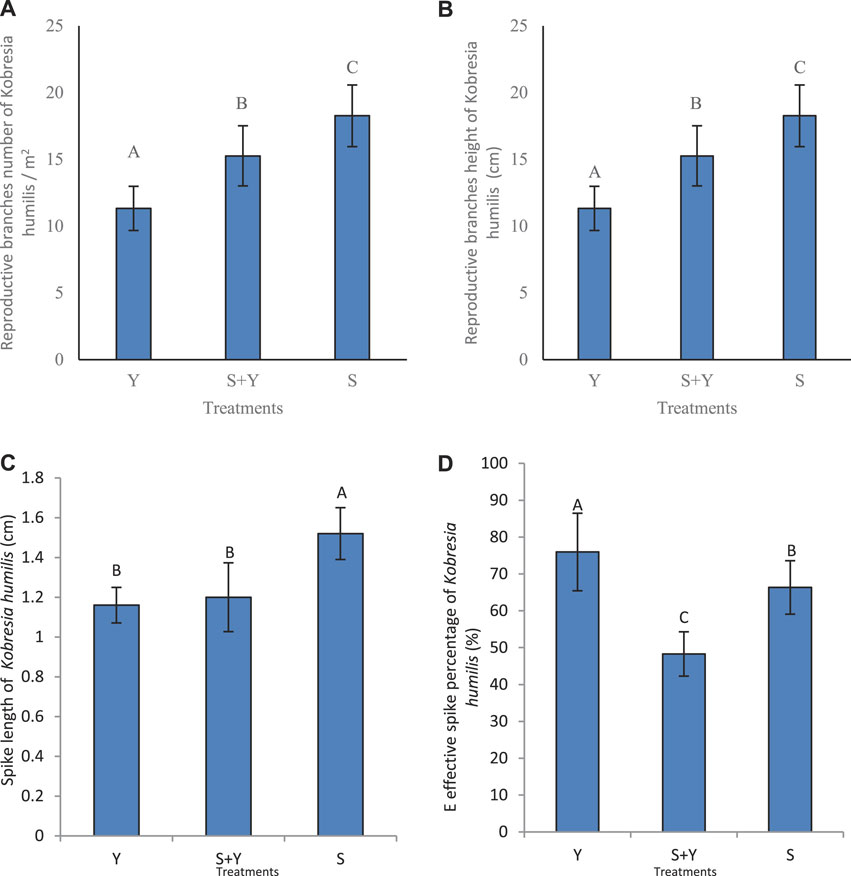
FIGURE 5. Effects of grazing methods on different sexual reproduction characteristics of K. humilis: (A) reproductive branch density, (B) reproductive branch height, (C) spike length, and (D) effective spike number ratio. Capital letters indicate significant differences between grazing methods (p < 0.05).
The Y treatment significantly increased the length and width of K. humilis seeds (p < 0.05), whereas the S treatment significantly increased the seed thickness (p < 0.05) (Figure 6A). Figure 6B shows that the S+Y treatment significantly reduced the 1000-grain weight of K. humilis. The seed number per spike was significantly higher in the S+Y treatment than in the other treatments (p < 0.05) (Figure 6C). Further, the S treatment significantly reduced the seed yield of K. humilis compared to the Y and S+Y treatments (p < 0.05), while there was no significant difference between the Y and S+Y treatments (p > 0.05) (Figure 6D).
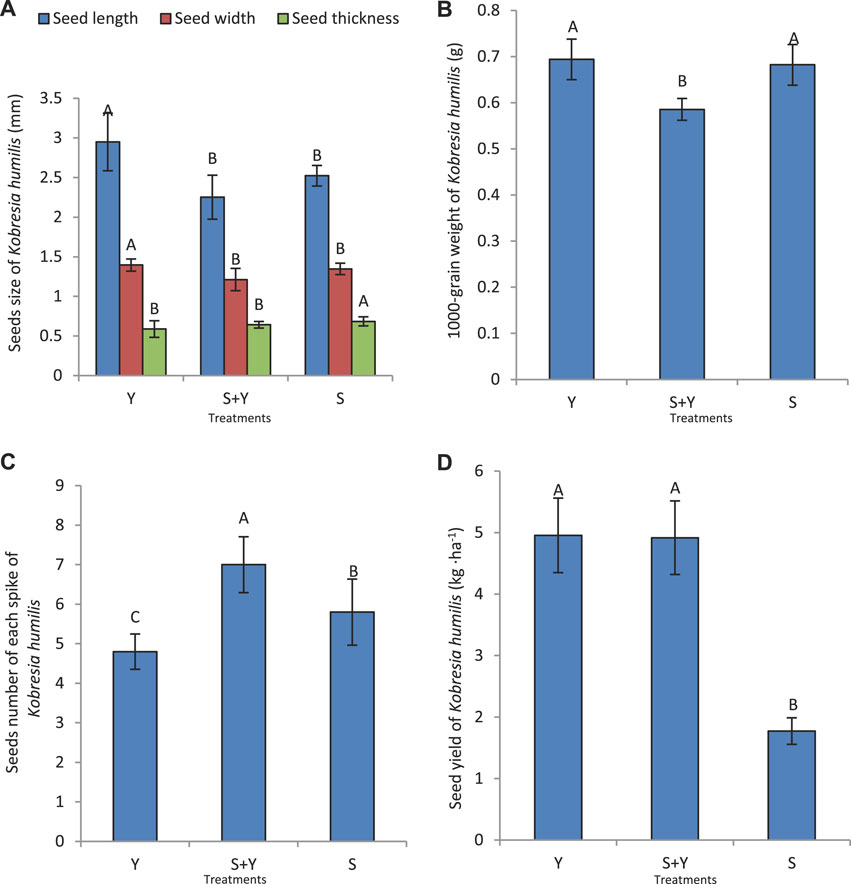
FIGURE 6. Effects of grazing methods on seed indicators of K. humilis: (A) seed size, (B) 1000-seed weight, (C) seed number per spike, and (D) seed yield. Capital letters indicate significant differences between grazing methods (p < 0.05).
3.2.2 Effect of Grazing Treatments on the Vegetative Reproduction Characteristics of K. humilis
Figure 7 depicts the effects of grazing treatments on the vegetative reproduction characteristics of K. humilis. The S+Y treatment significantly increased the nutrient branch density (p < 0.05), which was 3.33 and 1.42 times higher than that in the Y and S treatments, respectively. The Y treatment had the lowest nutrient branch density, which was significantly lower than that in the other treatments (p < 0.05) (Figure 7A). Figure 7B shows that the number of tillers per plant was the highest (12.07) in the Y treatment, which was significantly higher than in the other treatments (p < 0.05), while that in the S+Y treatment (6.07) was significantly higher than in the S treatment (4.8) (p < 0.05). The number of leaves per tiller in the S+Y treatment was significantly higher than the other treatments (p < 0.05), while there was no significant difference between the number of leaves per tiller in the Y and S treatments (p > 0.05) (Figure 7C). Moreover, the relationship between the different treatments was as follows: S+Y > Y > S.
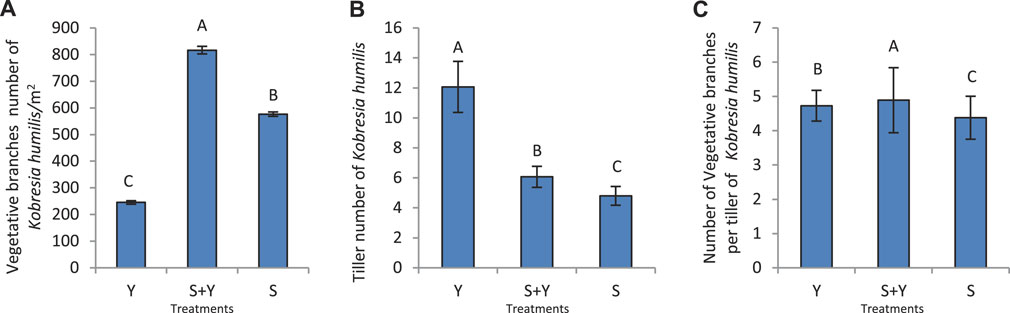
FIGURE 7. Effects of grazing methods on vegetative reproduction of K. humilis in terms of (A) nutrient branch density, (B) number of tillers per plant, and (C) number of nutrient branches per tiller. Capital letters indicate significant differences (p < 0.05) among grazing methods.
3.3 Biomass and Reproduction Efficacy of Each Functional Structure
3.3.1 Reproductive Structure Biomass and Reproductive Efficacy
Grazing treatments significantly changed the biomass of sexual reproductive structures, with the following trend: S+Y > Y > S; additionally, the difference between the vegetative biomass among the treatments was significant (p < 0.05) (Figure 8A). The maximum biomass of vegetative reproductive structures occurred in the S+Y treatment, which was significantly higher than in the Y and S treatments (p < 0.05); however, the differences between the S and Y treatments were not significant (p > 0.05). Figure 8B illustrates the difference between the sexual and vegetative reproduction efficacy. The difference in the sexual reproduction efficacy between the S and Y treatments was not significant (p > 0.05); moreover, the reproduction efficacy in the S+Y treatment (13.76%) was significantly lower than that in other treatments (p < 0.05). Further, the vegetative reproduction between the Y and S+Y treatments was not significant (p > 0.05), and both were significantly lower than the S treatment (p < 0.05).
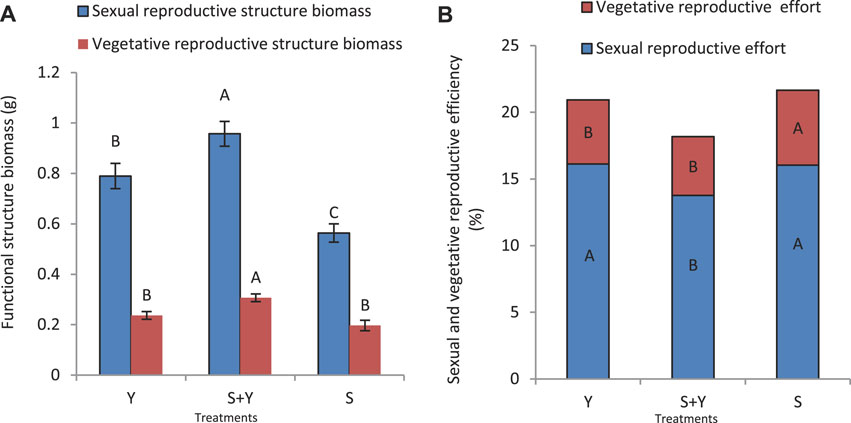
FIGURE 8. Trade-off between grazing methods on sexual and vegetative reproduction of K. humilis in terms of (A) reproductive structure biomass and Nutrition structure biomass and (B) reproductive structure effectiveness and nutritional structure effectiveness. Capital letters indicate significant differences (p < 0.05) among grazing methods.
The S+Y treatment significantly increased the allocation of resources to the storage structure (p < 0.05), and the biomass of the storage structure in the Y treatment was significantly higher than that in the S treatment (p < 0.05) (Figure 9A). Further, the pattern of change in the growth structure was the same as that of the storage structure, with the following trend: S+Y > Y > S, and a significant difference was observed between the treatments (p < 0.05). Figure 9B shows that the S+Y treatment significantly increased the allocation of resources in the storage structure (p < 0.05), and the storage efficacy did not differ significantly between the S and Y treatments (p > 0.05). Additionally, no significant difference was observed in growth effectiveness between the S and Y treatments (p > 0.05).
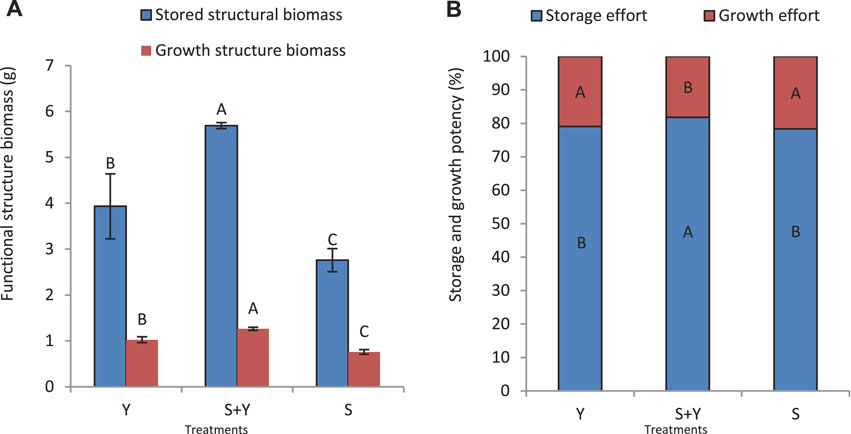
FIGURE 9. Trade-off between grazing methods on the storage and growth of K. humilis in terms of (A) storage structure and growth structure biomass, and (B) storage structure and growth structure effectiveness. Capital letters indicate significant differences among grazing methods (p < 0.05).
4 Discussion
4.1 Morphological Changes of K. humilis in Response to Individual and Population Scales Under Grazing Treatments
K. humilis is one of the dominant species in the alpine meadows of the QTP (Dai et al., 2021). Studying its morphological changes in response to grazing treatments is important for the sustainable utilization and maintenance of species diversity in the QTP alpine meadows (Guo et al., 2017; Zhang Q. et al., 2019). Our results showed that K. humilis developed adaptive strategies in response to different grazing disturbances, with significant differences observed in monocot biomass and material partitioning patterns among the different grazing treatments. The plants improved their adaptation to grazing by adjusting resource allocation to occupy a favorable ecological niche in a continuously disturbed environment (Kruk et al., 2021). In our study, the S+Y treatment resulted in the highest biomass per plant, while the Y treatment showed the lowest biomass per plant. This may be due to several reasons. First, cold season pasture grazing by yaks and Tibetan sheep not only reduced the biomass of K. humilis, but also significantly reduced the height of pasture. As one of the early re-greening forage resources, the lower plant height provided sufficient sunlight for the re-greening of K. humilis in the S treatment. High photosynthetic efficiency increased the material accumulation of K. humilis, which in turn increased the biomass of the individual plants. Li et al. (2021) showed that the physical mechanisms of compensatory growth usually include increased photosynthetic efficiency, release of apical dominance, and redistribution of resources (carbohydrates, water, and nutrients). Thus, the S+Y treatment produced compensatory growth in K. humilis, which could have also increased the biomass. The earlier re-greening also provides an advantage for K. humilis to utilize ecological niches (Zhang L. et al., 2019). This relatively reduced the competitive pressure of K. humilis with other species under S+Y treatment (Wang et al., 2014). Further, higher soil bulk density and lower nutrient content in the Y treatment than that in the S+Y treatment (Table 2) may also lead to the dwarfing of K. humilis. In addition, interspecific competition could also change the phenotypic plasticity. In the S treatment, Pinaceae were the dominant functional group, and interspecific competition due to niche occupancy put great pressure on the growth of K. humilis (Peng F. et al., 2020). To compete for water, light, and nutrients, K. humilis increased its plant height. The root collar is the organ of K. humilis to store nutrients, which plays an important role in completing its life. The biomass of all traits in this experiment indicates that the grazing treatments only promoted the redistribution of material in K. humilis but did not reverse the distribution pattern of material in each trait.
Bradshaw (2006) noted that changes in plant morphology are one of the driving forces of community succession (Kalske and Kessler, 2020), and are a mechanism to increase plant adaptation under high levels of disturbance. This is a result of long-time interaction between plants and domestic animals (Li et al., 2021). In this experiment, we found that although the Y treatment of K. humilis was small, the density per unit area was high at the population scale. The adaptive strategies adopted by plants in response to grazing are divided into two main types: avoidance and tolerance (Kennedy and Barbour, 1992). Our results suggest that plant adaptation to grazing can be understood using both theories together. From the growth hindrance theory, plant dwarfism in the Y treatment is strongly related to hoof pressure of grazing animals. The average hoof pressure of yak and Tibetan sheep is 6.89 kg/cm2 and 3.13 kg/cm2, respectively, and the ratio of average hoof pressure between yak and Tibetan sheep is 2.2:1; additionally, the average trampling intensity of yak is 7.3 times higher than that of Tibetan sheep (Yang H. et al., 2019). The high intensity of trampling increased the compactness of the surface soil, which limits the growth of K. humilis and is detrimental to the storage of nutrients within the plant, resulting in dwarfism. In addition, the reduced plant height may also be a grazing avoidance mechanism developed by K. humilis in response to long term foraging. Although grazing yaks in cold season pasture feed on withered vegetation from forage grass to survive, the loss of yak manure and greater trampling intensity also lead to soil nutrient deficiencies and soil structural damage (Table 2), reduce the number and diversity of beneficial microorganisms in the soil (Wang et al., 2022), which have a profound impact on the soil over time (Yang C. et al., 2019). These conditions have altered K. humilis morphology, forcing K. humilis to adopt a tolerance strategy, choosing to reduce plant height to accommodate reduced soil quality and greater trampling intensity (Zhang et al., 2020). Secondly, the plant height in the Y treatment is relatively high, and dwarf plants are less likely to be foraged by yaks. Reducing the plant height as an avoidance strategy preserves the ability of K. humilis to grow and reproduce and helps K. humilis to maintain its position in the sedge-grass community. Apparently, grazing yaks induced defenses in K. humilis at the population scale. To counteract the negative effects of plant dwarfism in interspecific competition and species survival, K. humilis used its limited resources to increase the number of individuals and thus occupy more ecological niches, with the aim of increasing the survival rate of the population. Previous studies have shown that yak trampling is more likely to stimulate K. humilis buds and tillers than Tibetan sheep (Pan et al., 2021). Changes in morphology may also be due to environmental constraints, including interspecific competition, abiotic limitations to growth and periodic biomass destruction (Buisson et al., 2019). In the S+Y treatment, K. humilis was taller but less dense, which was caused by the competition with the dominant species, Poaceae, due to environmental conditions. Grime and Pierce (2012) and Voile et al. (2012) showed that K. humilis limits intraspecific trait variation and allocates more nutrients to morph establishment to maintain the existing ecological niche width when environmental conditions are harsh.
4.2 Effects of Grazing Treatments on Reproductive and Life History Responses of K. humilis
Trade-offs are a central concept in life history theory (Wolf et al., 2007) wherein grazing intensifies environmental stress in plants, making it difficult for optimal allocation of resources (Hanushek, 1992; Prendergast, 2002). The results of this study showed that the grazing treatments significantly affected the trade-off strategy between sexual and asexual reproduction of K. humilis. Moreover, grazing disturbance in the Y treatment promoted sexual reproduction of K. humilis and reduced the input of vegetative reproduction. Notably, plants in the Y treatment did not use resources to increase the number of reproductive branches and density, but to improve sexual reproduction inputs by increasing the proportion of effective spikes and increasing the seed size and yield. The results of the Y treatment indicated that K. humilis extended its population to more open spaces by increasing its investment in seeds to avoid resource limitation caused by grazing yaks. As a Cyperaceous forage plant, K. humilis uses internal tillers to expand outward to increase its crown width. Thus, its crown width was significantly and positively correlated with K. humilis longevity (Dai et al., 2019).
Wilson and MacArthur (1967) divided the life history strategies of organisms into r-selection (miniaturization of adults, large individual numbers, small body size and high reproductive allocation) and k-selection (slow individual development, small numbers and large individuals). Grime (1979) proposed the concept of c-selection, means that plants to maximize their competitiveness under the condition of low environmental severity and low interference intensity. In our study, the significantly small crown width in the Y treatment suggested that this treatment facilitated the inclination of K. humilis to r-selection in its life history strategy, that is, high reproductive energy allocation and short epoch cycle. This life history strategy allows K. humilis to better adapt to the fluctuating living conditions induced by grazing yaks in the alpine meadows, and shaded and humid environmental conditions, thereby maximizing the population growth rate (Junk and Piedade, 1997). Compared to the other treatments, the K. humilis in S treatment devoted more resources to increasing reproductive branch height, spike length and seed weight, and had the lowest density of the three grazing treatments. This was possibly due to the dominance of Poaceae grass in the S treatment. K. humilis employs c-selection that maximizes individual competitiveness in response to the strong interspecific competition posed by Poaceae Salahuddin et al. (2018). Although the reproductive branch density, seed number, and seed yield in the S+Y treatment were significantly higher than those in the other treatments, sexual reproduction efficacy was significantly lower than that in the other treatments. This may be due to environmental changes that induced K. humilis to select minimal reproductive allocation and to store resources for a longer lifetime (k-selection). Further, the number of tillers in the Y treatment was significantly higher than in the other treatments, which was likely due to the coupling effect of high trampling intensity and foraging by yaks. Pan et al. (2021) showed that grazing yaks fragmented plants and activated their shoots to promote vegetative reproduction. In contrast, the number of tillers as shoot banks of K. humilis increased significantly.
Moreover, heavy foraging of K. humilis by yaks suppressed the plant height, thereby increasing the number of tillers. Both nutrient branch density and number were significantly lower in the Y treatment than in the S+Y treatment. This indicates that although yak grazing activated the K. humilis shoot pool and improved the grazing tolerance of K. humilis (Wang et al., 2017), it did not stimulate further development of shoots into nutrient branches. Many studies have shown that increase investment in sexual reproduction decreases investment in vegetative reproduction, suggesting that there is a significant trade-off between sexual and vegetative reproduction (Wang et al., 2018; Liu, 2020; Endo et al., 2021). Our results support this suggestion. Yuan et al. (2020) showed that simulated grazing of large herbivores favored asexual reproduction of Hordeum brevisubulatum to the detriment of sexual reproduction. This was a result of different simulated grazing intensities at the jointing stage, which increased tiller emergence yield and improved the compensatory growth capacity of tillers. Such findings are contrary to our conclusion that reported sexual reproduction efficacy was significantly higher than vegetative reproduction efficacy in all treatments. The development of shoots into nutritional branches may be related to photosynthetic rate, growth hormone levels, and animal saliva (Zhang et al., 2009). The adaptive strategies of plants to grazing can be reflected not only in the number of resources allocated, but also in the time of resource allocation (Liu et al., 2019; Keep et al., 2021). For perennial plants, a complex trade-off between current reproduction and future growth exists (Quesnel et al., 2018). The reproductive behavior of the perennial plants depends on the potential relationship between the number of resources currently available for growth and the number of resources stored for future reproduction (Friedman, 2020). The rootstocks and roots, act as nutrient storage organs for K. humilis and play crucial roles in the life history strategies and material distribution trade-offs (Song et al., 2020). In our study, storage efficacy was significantly higher than growth efficacy in all treatments, suggesting that future growth investment was more important for K. humilis in the trade-off between the present and the future. Some studies suggest that high storage may be an important factor in maintaining an inter-annual perennial regime in response to changes in abiotic factors, such as moisture and temperature, (Warschefsky et al., 2016; Freund Saxhaug et al., 2020). If future life expectancy is low, the energy allocated to current reproduction is high; conversely, if future life expectancy is high, the investment in reproduction is correspondingly reduced (Lundgren and Des Marais, 2020). The results of our study suggested that the growth effectiveness in the Y and S treatments was significantly higher than that in the S+Y treatment, while the storage efficacy was significantly lower than that in the S+Y treatment. Among the current future choices, the Y and S treatments reduced the future life expectancy of K. humilis, by favoring utilization of limited resources to promote current growth. The Y treatment reduced the crown width, increased the seed size and quality, and sacrificed current individual life length to compete for the utilization of ecological niches and maintenance of genetic diversity. In contrast, K. humilis in the S treatment devoted more resources to maintaining individual height and increasing competition for light resources with the Poaceae forage grass.
Compared with other treatments, S treatment increased K. humilis height, but significantly decreased the density of K. humilis. Cyperaceae—Poaceae meadows is the main vegetation type for alpine meadow. It was showed that the dominance of Cyperaceae in the community tends to increase in a mildly degraded alpine meadows (Han et al., 2019; Bourles et al., 2020), and thus is considered as a sign of decreasing grassland productivity. We found that the increase of yaks during grazing improved the purpose and sexual reproduction efficiency of K. humilis in the vegetation community, resulting in the degradation of alpine meadow. In contrast, Poaceae grasses are conducive to grazing due to their high biomass, good palatability, and benefit to sustainable development of alpine grasslands. Considering the morphological traits and reproductive characteristics of K. humilis in alpine meadows by grazing treatments, mono-grazing Tibetan sheep is more beneficial to grassland health and grazing utilization in alpine meadows.
This study was limited as only adaptive changes of K. humilis morphology and reproduction were explored. In future studies, the comprehensive effects of plant and soil should also be considered to provide a scientific basis for sustainable grazing utilization of alpine meadows.
5 Conclusion
In general, grazing treatments significantly altered the morphological characteristics and reproductive strategies of K. humilis. Grazing yaks alone increased the density and sexual reproductive efficacy of K. humilis, and mixed grazing by yaks and Tibetan sheep increased the canopy and biomass of K. humilis. In addition, sexual reproductive efficacy was significantly higher than nutritional reproductive efficacy and storage efficacy was significantly higher than growth efficacy in all treatments. This indicates that the main reproductive mode of K. humilis in this area is sexual reproduction and as a perennial forage grass, K. humilis stores most of its material to increase its future life expectancy. The Y treatment reduced sexual and nutritional reproduction, which favored the development of meadows to graminoid-grass type meadows. Gramineae meadows are more suitable for grazing utilization of alpine meadows due to their better palatability and biomass. Therefore, considering the health and sustainable utilization of alpine meadows, grazing Tibetan sheep in cool season pastures is a suitable grazing method.
Data Availability Statement
The original contributions presented in the study are included in the article/supplementary materials, further inquiries can be directed to the corresponding author.
Author Contributions
LW collected samples, analyzed data and wrote the manuscript. YJ did a part of sample work. CX selected the sampling location. XY designed the experiment and modified the manuscript.
Funding
This work was financially supported by National Natural Science Foundation of China (31760695).
Conflict of Interest
The authors declare that the research was conducted in the absence of any commercial or financial relationships that could be construed as a potential conflict of interest.
Publisher’s Note
All claims expressed in this article are solely those of the authors and do not necessarily represent those of their affiliated organizations, or those of the publisher, the editors and the reviewers. Any product that may be evaluated in this article, or claim that may be made by its manufacturer, is not guaranteed or endorsed by the publisher.
Acknowledgments
Many thanks to Editage (www.editage.cn) for providing English editing services.
References
Bai, M., Wei, K., Ma, K., Xu, C., and Yu, X. (2022). Rest Grazing from the Critical Period of Soil Thawing Promotes the Propagation of Kobresia Humilis in Alpine Meadow. Ecol. Eng. 179, 106634. doi:10.1016/j.ecoleng.2022.106634
Bourles, A., Guentas, L., Charvis, C., Gensous, S., Majorel, C., Crossay, T., et al. (2020). Co-Inoculation with a Bacterium and Arbuscular Mycorrhizal Fungi Improves Root Colonization, Plant Mineral Nutrition, and Plant Growth of a Cyperaceae Plant in an Ultramafic Soil. Mycorrhiza 30 (1), 121–131. doi:10.1007/s00572-019-00929-8
Bradshaw, A. D. (2006). Unravelling Phenotypic Plasticity - Why Should We Bother? New Phytol. 170 (4), 644–648. doi:10.1111/j.1469-8137.2006.01761.x
Buisson, E., Le Stradic, S., Silveira, F. A. O., Durigan, G., Overbeck, G. E., Fidelis, A., et al. (2019). Resilience and Restoration of Tropical and Subtropical Grasslands, Savannas, and Grassy Woodlands. Biol. Rev. 94 (2), 590–609. doi:10.1111/brv.12470
Dai, L., Fu, R., Guo, X., Ke, X., Du, Y., Zhang, F., et al. (2021). Effect of Grazing Management Strategies on Alpine Grassland on the Northeastern Qinghai-Tibet Plateau. Ecol. Eng. 173, 106418. doi:10.1016/j.ecoleng.2021.106418
Dai, L., Ke, X., Du, Y., Zhang, F., Li, Y., Li, Q., et al. (2019). Nitrogen Controls the Net Primary Production of an Alpine Kobresia Meadow in the Northern Qinghai‐Tibet Plateau. Ecol. Evol. 9 (15), 8865–8875. doi:10.1002/ece3.5442
Dembicz, I., Szczeparska, L., Moysiyenko, I. I., and Wódkiewicz, M. (2018). High Genetic Diversity in Fragmented Iris Pumila L. Populations in Ukrainian Steppe Enclaves. Basic Appl. Ecol. 28, 37–47. doi:10.1016/j.baae.2018.02.009
Endo, H., Sugie, T., Yonemori, Y., Nishikido, Y., Moriyama, H., Ito, R., et al. (2021). Vegetative Reproduction is More Advantageous Than Sexual Reproduction in a Canopy-Forming Clonal Macroalga under Ocean Warming Accompanied by Oligotrophication and Intensive Herbivory. Plants 10 (8), 1522. doi:10.3390/plants10081522
Freund Saxhaug, K., Jungers, J. M., Hegeman, A. D., Wyse, D. L., and Sheaffer, C. C. (2020). Cultivation of Native Plants for Seed and Biomass Yield. Agron. J. 112 (3), 1815–1827. doi:10.1002/agj2.20195
Friedman, J. (2020). The Evolution of Annual and Perennial Plant Life Histories: Ecological Correlates and Genetic Mechanisms. Annu. Rev. Ecol. Evol. Syst. 51, 461–481. doi:10.1146/annurev-ecolsys-110218-024638
Grime, J. P., and Pierce, S. (2012). The Evolutionary Strategies that Shape Ecosystems. Germany: John Wiley & Sons.
Grime, J. P. (1979). Primary Strategies in Plants. Trans. Botanical Soc. Edinb. 43 (2), 151–160. Taylor & Francis Group. doi:10.1080/03746607908685348
Guo, Y., Liu, L.-P., Zheng, L.-L., Yu, F.-H., Song, M.-H., and Zhang, X.-Z. (2017). Long-Term Grazing Affects Relationships between Nitrogen Form Uptake and Biomass of Alpine Meadow Plants. Plant Ecol. 218 (9), 1035–1045. doi:10.1007/s11258-017-0746-6
Han, W., Lu, H., Liu, G., Wang, J., and Su, X. (2019). Quantifying Degradation Classifications on Alpine Grassland in the Lhasa River Basin, Qinghai-Tibetan Plateau. Sustainability 11 (24), 7067. doi:10.3390/su11247067
Hanushek, E. A. (1992). The Trade-Off between Child Quantity and Quality. J. Political Econ. 100 (1), 84–117. doi:10.1086/261808
Huang, K., Zhang, Y., Zhu, J., Liu, Y., Zu, J., and Zhang, J. (2016). The Influences of Climate Change and Human Activities on Vegetation Dynamics in the Qinghai-Tibet Plateau. Remote Sens. 8 (10), 876. doi:10.3390/rs8100876
Junk, W. J., and Piedade, M. T. F. (1997). “Plant Life in the Floodplain with Special Reference to Herbaceous Plants,” in The Central Amazon Floodplain. Editor W. J. Junk (Berlin, Heidelberg: Springer), 147–185. doi:10.1007/978-3-662-03416-3_8
Kalske, A., and Kessler, A. (2020). Population-Wide Shifts in Herbivore Resistance Strategies over Succession. Ecology 101 (11), e03157. doi:10.1002/ecy.3157
Keep, T., Sampoux, J. P., Barre, P., Blanco‐Pastor, J. L., Dehmer, K. J., Durand, J. L., et al. (2021). To Grow or Survive: Which are the Strategies of a Perennial Grass to Face Severe Seasonal Stress? Funct. Ecol. 35 (5), 1145–1158. doi:10.1111/1365-2435.13770
Kennedy, G., and Barbour, J. D. (1992). “Resistance Variation in Natural and Managed Systems,” in Plant Resistance to Herbivores and Pathogens: Ecology, Evolution, and Genetics. Editors R. S. Fritz, and E. L. Simms (Chicago IL: University of Chicago Press), 13–41.
Kruk, C., Piccini, C., Devercelli, M., Nogueira, L., Accattatis, V., Sampognaro, L., et al. (2021). A Trait‐Based Approach Predicting Community Assembly and Dominance of Microbial Invasive Species. Oikos 130 (4), 571–586. doi:10.1111/oik.07694
Li, W., Liu, Y., Wang, J., Shi, S., and Cao, W. (2018a). Six Years of Grazing Exclusion is the Optimum Duration in the Alpine Meadow-Steppe of the North-Eastern Qinghai-Tibetan Plateau. Sci. Rep. 8 (1), 17269. doi:10.1038/s41598-018-35273-y
Li, X., Huang, Q., Mi, X., Bai, Y., Zhang, M., and Li, X. (2018b). Grazing Every Month Minimizes Size but Boosts Photosynthesis in Stipa Grandis in the Steppe of Inner Mongolia, China. J. Arid. Land 10 (4), 601–611. doi:10.1007/s40333-018-0011-4
Li, X., Png, G. K., Li, Y., Jimoh, S. O., Ding, Y., Li, F., et al. (2021). Leaf Plasticity Contributes to Plant Anti-Herbivore Defenses and Indicates Selective Foraging: Implications for Sustainable Grazing. Ecol. Indic. 122, 107273. doi:10.1016/j.ecolind.2020.107273
Liu, M., Gong, J., Yang, B., Ding, Y., Zhang, Z., Wang, B., et al. (2019). Differences in the Photosynthetic and Physiological Responses of Leymus Chinensis to Different Levels of Grazing Intensity. BMC Plant Biol. 19 (1), 558. doi:10.1186/s12870-019-2184-1
Liu, W. (2020). Individual Reproductive Strategy of Stipa Breviflora under Different Grazing Pressures. Grassl. Sci. 66 (3), 174–182. doi:10.1111/grs.12263
Lundgren, M. R., and Des Marais, D. L. (2020). Life History Variation as a Model for Understanding Trade-Offs in Plant-Environment Interactions. Curr. Biol. 30 (4), R180–R189. doi:10.1016/j.cub.2020.01.003
Oksanen, L. (2001). Logic of Experiments in Ecology: Is Pseudoreplication a Pseudoissue? Oikos 94 (1), 27–38. doi:10.1034/j.1600-0706.2001.11311.x
Pan, T., Song, J., Peng, J., Wang, Y., He, X., Zhou, Y., et al. (2021). Effect of Simulated Tibetan Sheep and Yak Trampling at Different Periods on the Reproductive Characteristics, Underground Morphology, and Carbohydrate Content of K. Humilis. Rangel. Ecol. Manag. 79, 126–138. doi:10.1016/j.rama.2021.08.005
Peng, F., Xue, X., You, Q., Sun, J., Zhou, J., Wang, T., et al. (2020a). Change in the Trade‐off between Aboveground and Belowground Biomass of Alpine Grassland: Implications for the Land Degradation Process. Land Degrad. Dev. 31 (1), 105–117. doi:10.1002/ldr.3432
Peng, Z., Xiao, H., He, X., Xu, C., Pan, T., and Yu, X. (2020b). Different Levels of Rainfall and Trampling Change the Reproductive Strategy of K. Humilis in the Qinghai-Tibet Plateau. Rangel. J. 42 (2), 143–152. doi:10.1071/rj19076
Pereira, A. M., and Coimbra, S. (2019). Advances in Plant Reproduction: from Gametes to Seeds. J. Exp. Bot. 70 (11), 2933–2936. doi:10.1093/jxb/erz227
Prendergast, C. (2002). The Tenuous Trade‐off between Risk and Incentives. J. Polit. Econ. 110 (5), 1071–1102. doi:10.1086/341874
Quesnel, L., King, W. J., Coulson, G., and Festa-Bianchet, M. (2018). Tall Young Females Get Ahead: Size-Specific Fecundity in Wild Kangaroos Suggests a Steep Trade-Off with Growth. Oecologia 186 (1), 59–71. doi:10.1007/s00442-017-4003-4
Ren, J. (1998). Research Methods of Pratacultural Science. Beijing: China Agriculture Press. In Chinese.
Rysiak, A., Chabuz, W., Sawicka-Zugaj, W., Jan Zdulski, J., Grzywaczewski, G., and Kulik, M. (2021). Comparative Impacts of Grazing and Mowing on the Floristics of Grasslands in the Buffer Zone of Polesie National Park, Eastern Poland. Glob. Ecol. Conserv. 27, e01612. doi:10.1016/j.gecco.2021.e01612
Rewald, B., Razaq, M., Lixue, Y., Li, J., Khan, F., Jie, Z., et al. (2018). Root Order-Based Traits of Manchurian Walnut & Larch and Their Plasticity Under Interspecific Competition. Sci. Rep. 8 (1), 1–14. doi:10.1038/s41598-018-27832-0
Schmitz, A., and Isselstein, J. (2020). Effect of Grazing System on Grassland Plant Species Richness and Vegetation Characteristics: Comparing Horse and Cattle Grazing. Sustainability 12 (8), 3300. doi:10.3390/su12083300
Sigcha, F., Pallavicini, Y., Camino, M. J., and Martínez-Ruiz, C. (2018). Effects of Short-Term Grazing Exclusion on Vegetation and Soil in Early Succession of a Subhumid Mediterranean Reclaimed Coal Mine. Plant Soil 426 (1), 197–209. doi:10.1007/s11104-018-3629-2
Sommer, R. J. (2020). Phenotypic Plasticity: From Theory and Genetics to Current and Future Challenges. Genetics 215 (1), 1–13. doi:10.1534/genetics.120.303163
Song, M.-H., Zhu, J.-F., Li, Y.-K., Zhou, H.-K., Xu, X.-L., Cao, G.-M., et al. (2020). Shifts in Functional Compositions Predict Desired Multifunctionality along Fragmentation Intensities in an Alpine Grassland. Ecol. Indic. 112, 106095. doi:10.1016/j.ecolind.2020.106095
Spaargaren, O. C., and Deckers, J. (1998). “The World Reference Base for Soil Resources,” in Soils of Tropical Forest Ecosystems (Berlin, Heidelberg: Springer), 21–28. doi:10.1007/978-3-662-03649-5_2
Török, P., Penksza, K., Tóth, E., Kelemen, A., Sonkoly, J., and Tóthmérész, B. (2018). Vegetation Type and Grazing Intensity Jointly Shape Grazing Effects on Grassland Biodiversity. Ecol. Evol. 8 (20), 10326–10335. doi:10.1002/ece3.4508
Violle, C., Enquist, B. J., McGill, B. J., Jiang, L., Albert, C. H., Hulshof, C., et al. (2012). The Return of the Variance: Intraspecific Variability in Community Ecology. Trends Ecol. Evol. 27 (4), 244–252. doi:10.1016/j.tree.2011.11.014
Wang, J., Wang, X., Liu, G., Wang, G., and Zhang, C. (2021). Grazing-to-Fencing Conversion Affects Soil Microbial Composition, Functional Profiles by Altering Plant Functional Groups in a Tibetan Alpine Meadow. Appl. Soil Ecol. 166, 104008. doi:10.1016/j.apsoil.2021.104008
Wang, L., Yu, X., Xu, C., Jing, Y., and Song, M. (2022). Grazing by Tibetan Sheep Enhances Soil Bacterial and Fungal Diversity in Cold Season Pastures of Alpine Meadows on the Northern Qinghai-Tibetan Plateau. J. Soil Sci. Plant Nutr., 1–23. doi:10.1007/s42729-022-00819-7
Wang, P., Li, H., Pang, X.-Y., Wang, A., Dong, B.-C., Lei, J.-P., et al. (2017). Clonal Integration Increases Tolerance of a Phalanx Clonal Plant to Defoliation. Sci. Total Environ. 593-594, 236–241. doi:10.1016/j.scitotenv.2017.03.172
Wang, S., Wang, C., Duan, J., Zhu, X., Xu, G., Luo, C., et al. (2014). Timing and Duration of Phenological Sequences of Alpine Plants along an Elevation Gradient on the Tibetan Plateau. Agric. For. Meteorol. 189-190, 220–228. doi:10.1016/j.agrformet.2014.01.021
Wang, Z., Xie, L., Prather, C. M., Guo, H., Han, G., and Ma, C. (2018). What Drives the Shift between Sexual and Clonal Reproduction of Caragana Stenophylla along a Climatic Aridity Gradient? BMC Plant Biol. 18 (1), 91. doi:10.1186/s12870-018-1313-6
Warschefsky, E. J., Klein, L. L., Frank, M. H., Chitwood, D. H., Londo, J. P., Von Wettberg, E. J. B., et al. (2016). Rootstocks: Diversity, Domestication, and Impacts on Shoot Phenotypes. Trends Plant Sci. 21 (5), 418–437. doi:10.1016/j.tplants.2015.11.008
Wilson, E. O., and MacArthur, R. H. (1967). The Theory of Island Biogeography, 1. Princeton, NJ: Princeton University Press.
Wilson Sayres, M. A. (2018). Genetic Diversity on the Sex Chromosomes. Genome Biol. Evol. 10 (4), 1064–1078. doi:10.1093/gbe/evy039
Wolf, M., van Doorn, G. S., Leimar, O., and Weissing, F. J. (2007). Life-History Trade-Offs Favour the Evolution of Animal Personalities. Nature 447 (7144), 581–584. doi:10.1038/nature05835
Xiao, H., Peng, Z., Xu, C. L., Zhang, G., Chai, J. L., Pan, T. T., et al. (2018). Yak and Tibetan Sheep Trampling Inhibit Reproductive and Photosynthetic Traits of Medicago Ruthenica Var. Inschanica. Environ. Monit. Assess. 190 (9), 507–516. doi:10.1007/s10661-018-6896-8
Yan, B., and Hou, Y. (2018). Effect of Soil Magnesium on Plants: A Review. IOP Conf. Ser. Earth Environ. Sci. 170 (2), 022168. doi:10.1088/1755-1315/170/2/022168
Yang, C., Zhang, Y., Hou, F., Millner, J. P., Wang, Z., and Chang, S. (2019a). Grazing Activity Increases Decomposition of Yak Dung and Litter in an Alpine Meadow on the Qinghai-Tibet Plateau. Plant Soil 444 (1), 239–250. doi:10.1007/s11104-019-04272-x
Yang, H., Sun, J., Xu, C., Zhang, J., Chai, J., Jiao, T., et al. (2019b). Hoof Pressure and Trampling Intensity of Yaks Are Higher Than Those of Tibetan Sheep in a Tianzhu Alpine Meadow. Rangel. J. 41 (2), 125–133. doi:10.1071/rj18073
Yuan, J., Li, H., and Yang, Y. (2020). The Compensatory Tillering in the Forage Grass Hordeum Brevisubulatum after Simulated Grazing of Different Severity. Front. Plant Sci. 11, 792. doi:10.3389/fpls.2020.00792
Zhang, J.-T., Mu, C.-S., Wang, D.-L., Wang, J.-F., and Chen, G.-X. (2009). Shoot Population Recruitment from a Bud Bank over Two Seasons of Undisturbed Growth of Leymus Chinensis. Botany 87 (12), 1242–1249. doi:10.1139/b09-080
Zhang, L., Pang, R., Xu, X., Song, M., and Ouyang, H. (2019a). Three Tibetan Grassland Plant Species Tend to Partition Niches with Limited Plasticity in Nitrogen Use. Plant Soil 441 (3), 601–611. doi:10.1007/s11104-019-04148-0
Zhang, Q., Liu, G., and Chen, Z. (2019b). “Compensatory Growth Pattern of Sheepgrass (Leymus Chinensis),” in Sheepgrass (Leymus Chinensis): An Environmentally Friendly Native Grass for Animals (Singapore: Springer), 181–195. doi:10.1007/978-981-13-8633-6_9
Zhang, T., Chen, X., Guo, R., Degen, A. A., Kam, M., Zhao, J., et al. (2020). Natural Primary Production Mediates the Effects of Nitrogen and Carbon Addition on Plant Functional Groups Biomass and Temporal Stability in the Tibetan Alpine Steppe-Meadow. Agric. Ecosyst. Environ. 302, 107080. doi:10.1016/j.agee.2020.107080
Keywords: alpine meadow, grazing treatments, sexual reproduction, vegetative reproduction, phenotypic plasticity
Citation: Wang L, Jing Y, Xu C and Yu X (2022) Effect of Grazing Treatments on Phenotypic and Reproductive Plasticity of Kobresia humilis in Alpine Meadows of the Qinghai-Tibet Plateau. Front. Environ. Sci. 10:903763. doi: 10.3389/fenvs.2022.903763
Received: 24 March 2022; Accepted: 03 June 2022;
Published: 24 June 2022.
Edited by:
Steve Monfort, Smithsonian Conservation Biology Institute (SI), United StatesReviewed by:
Yu Liu, Northwest A & F University, ChinaZheng Gang Guo, Lanzhou University, China
Shixiong Li, Qinghai University, China
Copyright © 2022 Wang, Jing, Xu and Yu. This is an open-access article distributed under the terms of the Creative Commons Attribution License (CC BY). The use, distribution or reproduction in other forums is permitted, provided the original author(s) and the copyright owner(s) are credited and that the original publication in this journal is cited, in accordance with accepted academic practice. No use, distribution or reproduction is permitted which does not comply with these terms.
*Correspondence: Xiaojun Yu, eXV4akBnc2F1LmVkdS5jbg==