- 1Department of Ecology, University of Innsbruck, Innsbruck, Austria
- 2Research Department for Limnology, Mondsee, University of Innsbruck, Mondsee, Austria
- 3Bayreuth Center of Sport Science, University of Bayreuth, Bayreuth, Germany
Climate warming has multiple effects on an environment. Especially the Alpine region is affected by changing conditions, which do not only have ecological but also economic impacts in respect to winter sports tourism. Due to higher environmental temperatures and less precipitation, artificial snow making is becoming increasingly important and consequently, mountain reservoirs for water storage are built. In these systems, planktonic communities are not only influenced by the naturally harsh environmental conditions of the alpine region, but also by severe changes in water level fluctuations due to water withdrawal and re-filling within short time periods. Information on planktonic communities and species traits in such man-made water bodies is nonexistent. Here, we focused on ciliates, a group of unicellular protists known to adapt and respond rapidly to changing environmental conditions. Simultaneously, we identified abiotic and other biotic factors that shaped these microbial communities. We investigated the species composition, abundance and species traits of ciliates in eleven mountain reservoirs in the Tyrolean Alps, Austria, and hypothesized that these communities differed significantly from natural ones. The mountain reservoirs were investigated twice during the ice-free season and water chemistry, chlorophyll a, bacteria, zooplankton, and ciliates were sampled. We detected 48 ciliate taxa in total, with an average of five taxa per mountain reservoir. A wide range of abundance (summer: 24 to >15,600 Ind L−1; autumn: 38 to ∼7,500 Ind L−1) and no clear pattern in the community composition was found, most likely due to water level fluctuations and the source of water used for filling the mountain reservoirs. The ciliate abundance was significantly affected by pH, nutrients, but also water transparency and potential predators (crustaceans). Planktonic ciliates dominated the mountain reservoirs and, surprisingly, mixotrophic species, typically found in natural (alpine) lakes, were only rarely observed. Our data suggest that in these fast-changing systems, local factors seem to be more important than regional ones.
Introduction
In the Central Alps, tourism affects land use. Due to the importance of winter sports tourism, many new ski lifts were built over the last decades (Vanat, 2020). At the same time, rising temperatures impact the environment and therefore life in mountain areas (Elser et al., 2020). Climate warming causes changes in vegetation zones and the habitability for organisms as well as in the snowfall line and snow cover duration (Breiling and Charamza, 1999). For example, in the Swiss Alps the snow cover duration was shortened for almost 9 days per decade (from 1970 to 2015), mainly driven by early snow melt, but also a decline of the snow depth (Klein et al., 2016). To compensate the lack of snow for touristic activities, artificial snow production became an indispensable tool. For this process, the resource water is taken from mountain streams, lakes, drinking water supplies or man-made mountain reservoirs. The latter are artificial water bodies established close to, or directly in a skiing area ensuring short water transport distances from the source to the slope. These mountain reservoirs are either built in natural troughs or are newly constructed by the use of stones, gravel, concrete and soil. To lower the risk of a dam break or loss of water, they are usually sealed by synthetic membrane liners (e.g., High Density Polyethylene geomembranes) or asphalt concrete. Circulation systems (air injection) keep the water at lower temperatures during summer to prevent algal growth and reduce the onset of ice-building in winter. Some also have thermic elements installed to keep the water at optimal temperatures near the freezing point for snow making during the winter season.
In Austria, there are around 460 of these man-made mountain reservoirs, most of them are located in the counties of Tyrol and Salzburg. They are of different size, depth, morphology, and situated at various elevations. The origin of the water is rather diverse and water levels fluctuate due to water withdrawal for the artificial snow making and refilling. Besides, these water bodies are not directly influenced by local bedrock geology. Apart from that, they have the characteristics of stagnant waters and seem to fulfill the same requirements for the colonization of organisms like natural alpine lakes. New or young aquatic habitats usually show low local biodiversity, which makes it easier for new species to colonize them (Shurin, 2000). The colonization of these ecosystems can be driven by resting stages dispersed by animals or wind (Cáceres and Soluk, 2002; Figuerola and Green, 2002). Ciliates, rotifers and crustaceans are known to encyst or remain in resting eggs and are one of the first organisms to colonize new aquatic habitats (Wetzel, 2001; Foissner, 2006; Blatterer, 2008; Badosa et al., 2017). At the first stage of colonization, regional conditions play a more important role than local ones as shown for newly created ponds. In this stage, they have a common community composition, comparable to nearby natural lakes. However, after a longer time period, local factors primarily shaped the community composition (Audet et al., 2013).
For protists and ciliates in particular, less diversity than in lowland water bodies is typically observed in high mountain lakes because few species are adapted to the unfavorable environmental conditions including low temperatures, high solar radiation and low nutrient availability (Wille et al., 1999; Sonntag et al., 2011a, 2017; Kammerlander et al., 2016), even though studies using molecular methods suggested higher diversities in those environments than prior assumed (ter Braak and Smilauer, 2012; Kammerlander et al., 2015; Ortiz-Álvarez et al., 2018). Amongst lake morphology, the chemical composition of the lake water is crucial for the occurrence of species in high mountain areas (Kernan et al., 2009; Catalan and Donato Rondón, 2016). Phytoplankton and bacterial biomass is also directly related to physico-chemical conditions, which indirectly affect other planktonic organisms such as ciliates and zooplankton (Pace, 1982; Weisse, 2017).
Natural alpine lakes are usually little to none influenced by anthropogenic agents (Rose et al., 2009), which gives them a pristine, but also sensitive character as they are one of the first ecosystems which indicate changes in environmental conditions (Pastorino and Prearo, 2020). In man-made mountain reservoirs, we find the opposite, here, water level instability, artificial mixing by air injection and artificial sealing can influence the whole system. Ciliate assemblages have never been studied in these water bodies before, so we did not know whether the communities differed greatly from those of natural lakes and what factors caused differences in species composition, diversity, distribution, and abundance. Moreover, we were interested in the occurrence of certain functional traits related to local factors prevailing in the artificial systems. Identifying functional traits of ciliates that are key members of aquatic microbial food webs is central in understanding the contribution of these protists to ecosystem stability and dynamics (Weisse, 2017; Xu et al., 2018; Forster et al., 2021; Pröschold et al., 2021; Qu et al., 2021). Therefore, based on a precise morphospecies identification, we focused on two important functional traits: 1) movement: planktonic vs surface-associated morphological adaptations, and, 2) prevailing nutrition modes. Planktonic ciliates have been found predominantly in natural high mountain lakes and they are equipped with membranelles, cirri, bristles or stalks that facilitate (fast) swimming, jumping or attachment to other floating organisms and detritus (for details on planktonic species see compilation of Foissner et al., 1999). In contrast, surface-associated species and communities have cirri (bundles of cilia) to crawl and glide on submersed stones and plants (Foissner et al., 1991, 1994). Consequently, by identifying a ciliate to species level, a good overview of its motion characteristics can be obtained. From the investigation of this trait, we expected to confirm the source of water for filling a mountain reservoir (e.g., stream or stagnant water body) and its influence in shaping the prevailing communities. A second trait that is important in ciliate ecology is the nutrition mode of an individual species, i.e., heterotrophy/phagotrophy or mixotrophy. Mixotrophic ciliates resemble phagotrophic nutrition combined with hosting of algal endosymbionts. In lowland lakes, ciliates living in such a common mutualistic relationship are temporarily dominating the ciliate communities (Foissner et al., 1999; Sonntag et al., 2006; Dziallas et al., 2012). Mixotrophic species have also been frequently observed as a recurring (stable) element in alpine lakes (Wille et al., 1999; Sonntag et al., 2011a; Kammerlander et al., 2016).
Finally, we wanted to investigate potential shifts in species compositions and abundance over time (i.e., summer conditions shortly after ice-out and autumn before freezing) including surveys on water chemistry, potential food sources (i.e., bacteria and chlorophyll a) and predators (i.e., zooplankton). Our hypotheses were that 1) ciliate communities and species pools differed from natural alpine lakes, 2) a higher species diversity and higher abundances were observed during the more productive summer period compared to autumn, 3) the age and artificial disturbance of the water body and the source of the water used for filling played a significant role in shaping the ciliate communities, and, 4) that the fluctuating environmental conditions directly impacted ciliate species traits in respect to feeding types and habitat preferences.
Materials and methods
Study sites
We sampled eleven man-made mountain reservoirs located in the Austrian Central Alps (Figure 1). The reservoirs were constructed between 1999 and 2016 and differed in elevation, maximum depth, volume, and artificial sealing. For bottom sealing, various materials were used; most reservoirs were sealed with synthetic liners (e.g., High Density Polyethylene (HDPE) 2.0–2.5 mm geomembrane) and covered with coarse gravel. Apart from precipitation, the mountain reservoirs were fed with water from nearby located springs, (glacial) streams or other reservoirs (see Table 1 for details).
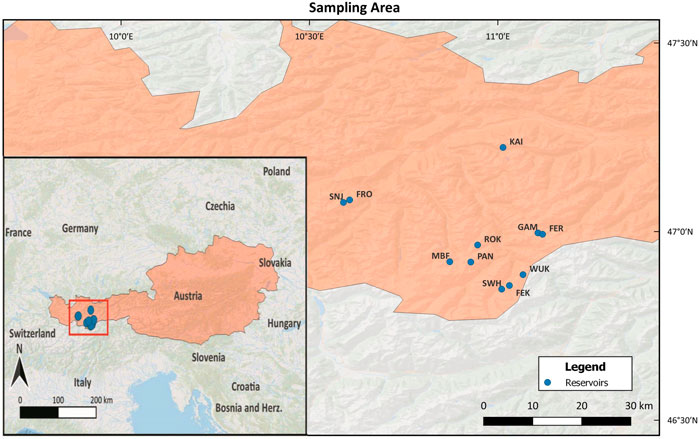
FIGURE 1. Map of the sampling area. Blue dots indicate sampling sites. For reservoir codes see Table 1.
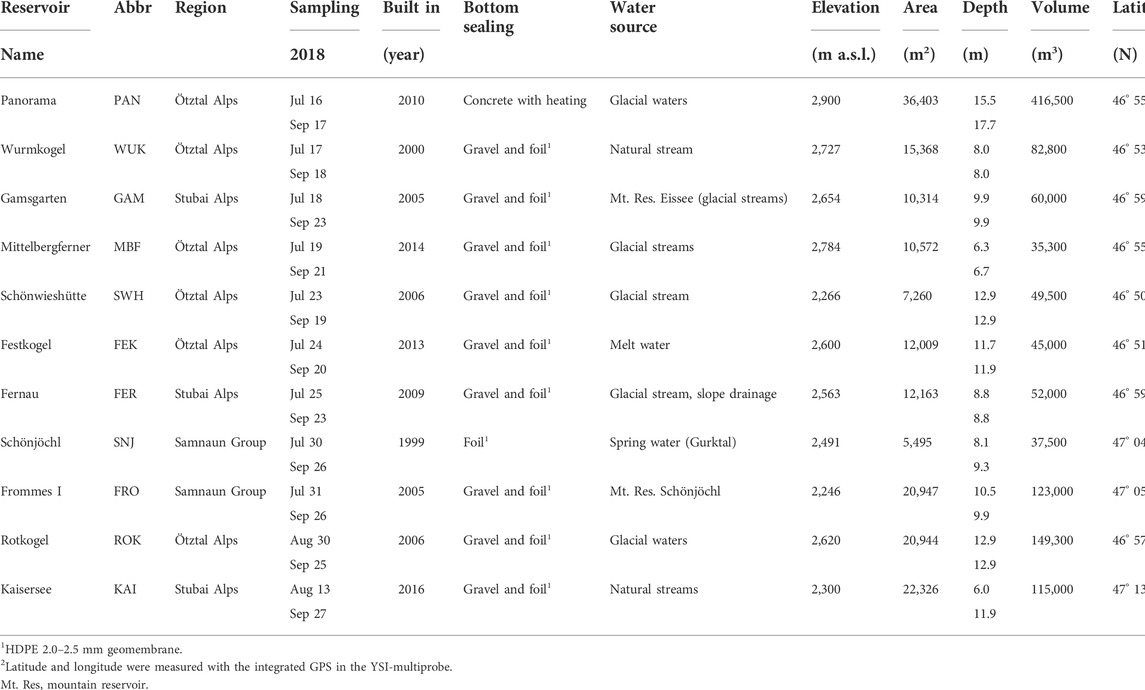
TABLE 1. Main characteristics of the mountain reservoirs including geographic region and coordinates, sampling date, year of construction, type of sealing, water source for filling, elevation, area, maximum depth on the day of sampling, and volume.
Sampling
Each water body was sampled twice during the ice-free season of 2018. The “summer sampling” was carried out shortly after ice-out in July and August, the “autumn sampling” in September. All samples were taken from an inflatable boat at the deepest point of each mountain reservoir. Maximum depth was recorded at each sampling date using a PS-7 portable depth sounder (Hondex Electronics Co., Ltd.). Secchi depth was estimated and temperature, oxygen (O2) concentration and saturation, pH as well as the electrical conductivity were measured with a YSI EXO2 multiprobe (Xylem Analytics) over the whole water column. Water samples were taken with a modified 5-L Schindler-Patalas sampler (Uwitec) for ciliate, bacteria, chlorophyll a (Chl a), turbidity, and water chemistry analyzes and pooled from 2 m depth intervals over the whole water column. When the temperature decreased more than 1°C m−1, two pooled samples were taken for water chemistry, Chl a and turbidity according to epi- and hypolimnion. In one of the lakes (KAI), depth-specific separate samples were taken (summer: 0, 2, 4, 5.5 m; autumn: 0, 2, 6, 11 m; depending on the respective maximum depth/filling level at a time, see Table 1). Ciliate samples (triplicates) were immediately fixed in 500-ml glass bottles (Schott) with freshly prepared Bouin’s solution (5% final concentration) after Skibbe (1994). In addition, vertical net tows for further detailed analysis of the ciliates were taken with a 10-µm plankton net (Uwitec) and a 100 ml aliquot of this concentrated sample was fixed with Bouin’s solution. Bacterial samples (triplicates) were fixed in 50-ml tubes (Greiner) with formalin (2% final concentration). Zooplankton samples were taken with an Apstein plankton net (50-µm mesh; four replicates) over the whole water column and fixed in ethanol (70% final concentration).
Abiotic parameters and chlorophyll a
The raw water samples were analyzed within 24 h after collection. The alkalinity was measured via Gran-Titration with an Orion 960 Titrator (Thermo Fisher Scientific 2008). The concentrations of total (TP) and dissolved (DP) phosphorus were measured via spectrophotometry using molybdate as soluble reactive phosphorus after digestion with potassium persulfate (Vogler, 1966). Dissolved (DOC) and total (TOC) organic carbon concentrations were measured with a total organic carbon analyzer (Shimadzu TOC-VCPH). Dissolved (DN) and total nitrogen (TN) were measured by chemiluminescence using the Total Nitrogen Measuring Unit (Shimadzu TNM-1). Anions and cations were analyzed by ion chromatography (Dionex ICS-1000; Thermo Fisher Scientific). Ammonium (NH4-N) and dissolved reactive silicon (DRSi) were measured with the Indophenol Blue Method (Wagner, 1969) and the Molybdate Method (Smits and Milne, 1981), respectively, using a spectrophotometer (Hitachi-U-2900). Turbidity was determined with a WTW Turb 430 T nephelometer (Xylem Inc.).
For Chl a, between 0.58–13 L were filtered onto GF/F filters (Whatman), extracted with acetone (13 ml), sonicated on ice for 1 min (Bandelin, Sonopuls) and stored in the dark at 4°C overnight. The extracts were cleared over Anodisc filters (0.1 mm pore-size; Whatman) and scanned in a double-beam spectrophotometer (Hitachi-U-2900). To measure the phaeopigment concentration, 0.1 ml of 1 mol L−1 HCl were added and the sample scanned again after 5 min. The Chl a concentration was calculated after Lorenzen (1967).
Biotic parameters
Ciliates were identified and quantified by applying the quantitative protargol staining method (QPS) (Skibbe, 1994; Pfister et al., 1999). Before filtration onto cellulose nitrate filters (0.8 µm pore size, integrated counting grid; Sartorius), the samples were settled for about 1 week before the supernatant was carefully removed with a vacuum pump. Ciliate identification was performed via bright-field microscopy at magnifications of up to ×1,000 (Olympus BX50). Ciliates were determined by using the identification keys of Foissner et al. (1991, 1992, 1994, 1995, 1999). For species traits, we considered nutrition types on the one hand and habitat preference on the other. Feeding modes included heterotrophic/phagotrophic species that primarily feed on prokaryotes (bacteria, cyanobacteria) and other protists (e.g., phytoplankton, heterotrophic nanoflagellates) and mixotrophic species that have either algal endosymbionts or “kleptoplasts” (= chloroplasts acquired by the ciliate from algal food to receive photosynthetic products). Moreover, species traits were also defined after the preferred habitat type of a ciliate, i.e., planktonic life style (floating or swimming species) or surface-associated (crawling or gliding) as it is common for benthic ones found in running waters. All species traits were assigned following Foissner et al. (1991, 1992, 1994, 1995, 1999).
Bacterial abundance was assessed by flow cytometry following the method of Del Giorgio et al. (1996). In short, formaldehyde-fixed cells were stained with the intercalating DNA/RNA stain SYTO13 (final concentration 250 nM) for 20 min in the dark. The cells were identified and counted at 520 nm vs. side scatter of a 488 nm laser on a MoFlo Astrios (Beckman Coulter GmbH, Vienna, Austria). Bacterial abundance was calculated from the ratio of cell counts to a defined number of 1 μm fluorescent reference beads (Sigma Aldrich, Vienna, Austria) added to each sample and adjusted for dilution with fixative and dye.
Zooplankton (crustaceans and rotifers) was counted with an inverted microscope (Leitz, Labovert) after sedimentation in Utermöhl chambers at a magnification of ×100. The species were identified after Flößner (2000), Einsle (1993), Voigt and Koste (1978a) and Voigt and Koste (1978b).
Statistical analyzes
The Shannon-Wiener Diversity Index (H(S)) and the Evenness (E) were calculated according to Mühlenberg (1993). To visualize the variation among the mountain reservoirs at the two sampling occasions, we used Canoco 5 (version 5.12; ter Braak and Smilauer, 2012). We used principal component analyzes (PCA) on independent data sets: 1) based on the environmental data (Supplementary Tables S1, S2, Supplementary Figure S1) and 2) based on the ciliate community (abundance data, Figures 2, 3). Redundancy analyzes (RDA) with forward selection of statistically significant parameters were used to analyze the variation in the ciliate species pattern in relation to the environmental parameters. The first parameter included within the forward selection process typically has the highest significant impact on the ciliate communities. Any parameter co-variant with an already selected parameter will then not be available for selection within the next forward selection step (see ter Braak and Smilauer, 2012). Provided that an alternative explanatory parameter is an equally probable candidate for selection as major parameter, we state this together with the percentage this parameter would explain if chosen as the first parameter. Redundancy analyzes were chosen because of the short gradients observed in the ciliate data. The ciliate abundance data were log(y + 1) transformed, centered for analyzes and the samples were standardized (Chord distance; Legendre and Gallagher, 2001). Explanatory variables were centered and standardized. The RDA was then calculated with the following data: 1) for all ciliates (>1% of total mean abundance in more than one sample) and 2) for the dominant ciliate taxa (Cinetochilum margaritaceum, Halteria spp., Vorticella natans, and Urotricha spp.). Elevation, depth, area, turbidity, Secchi depth, physicochemical parameters (Supplementary Tables S1, S2), abundance of bacteria [log(y + 1) transformed], zooplankton [rotifers and crustaceans >1% of total mean abundance; log(y + 1) transformed] and Chl a were included in the analyzes as explanatory variables. Statistical significance of the explanatory parameters was assessed with 999 unrestricted Monte Carlo permutations and corrected for false discovery rate (Benjamini–Hochberg correction for multiple testing implemented by Canoco 5, with a p < 0.05 considered as significant).
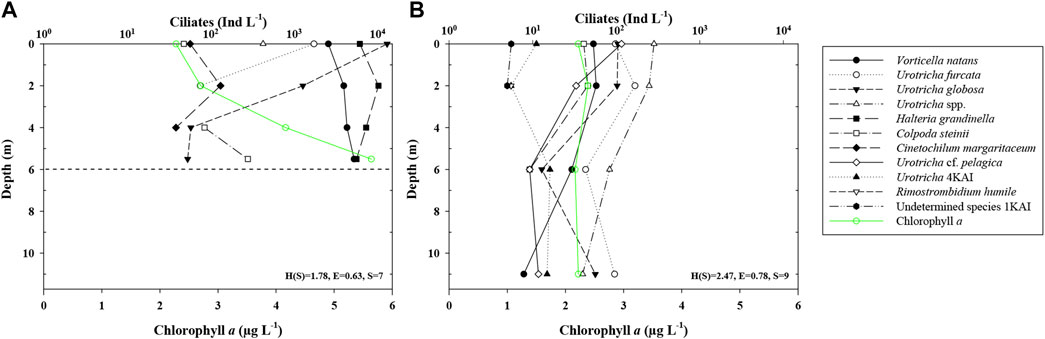
FIGURE 2. Vertical distribution of ciliates (mean abundance) and Chl a in Kaisersee (KAI). The dotted horizontal line marks the maximum filling on the day of sampling. (A) summer sampling (August), (B) autumn sampling (September).
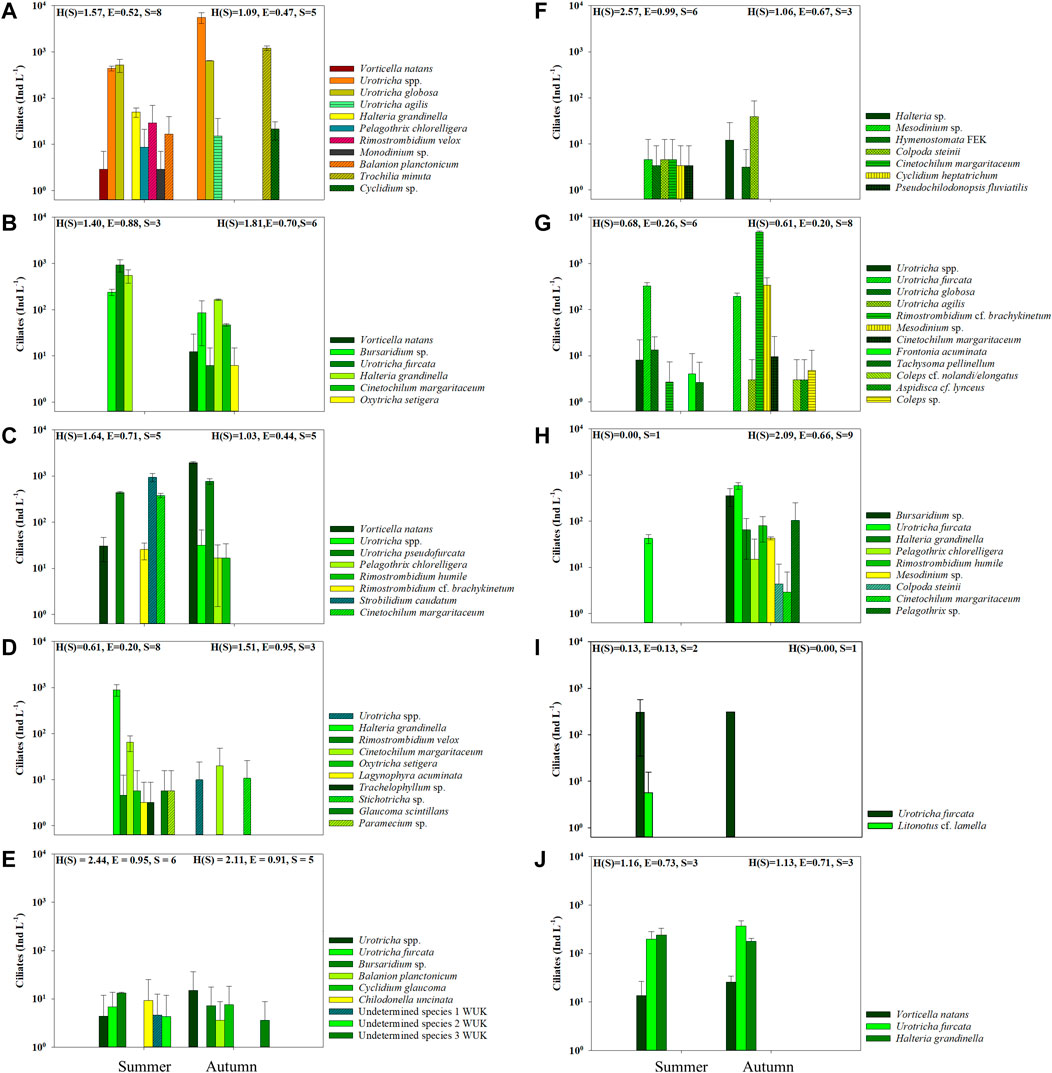
FIGURE 3. Ciliate abundance (mean ± std) during summer and autumn sampling in the mountain reservoirs (A) PAN, (B) FER, (C) ROK, (D) GAM, (E) WUK, (F) FEK, (G) SNJ, (H) FRO, (I) MBF, and (J) SWH. For reservoir codes see Table 1.
Results
Abiotic parameters, chlorophyll a, bacteria, zooplankton, and principal component analyzes
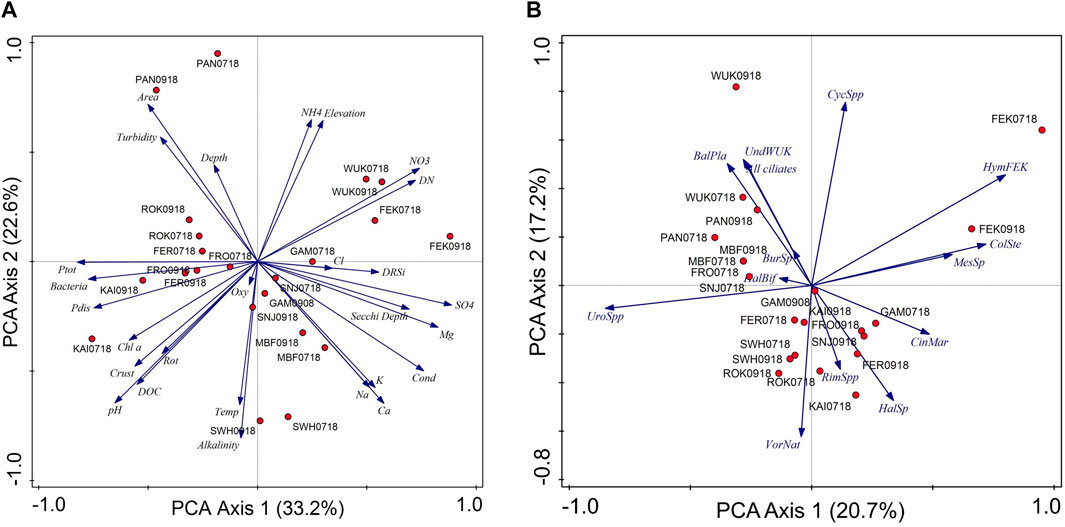
FIGURE 4. Principal component analyzes (PCA) of (A) the abiotic/environmental parameters, and (B) the ciliate communities (based on abundance data; >1% of total mean abundance in more than one sample) for the 11 mountain reservoirs. Percentages indicate amount explained by the individual PCA axes. Cond, conductivity; Crust, crustaceans; Rot, rotifers; Ptot, total phosphorus; Pdis, dissolved phosphorus; Temp, temperature; BalPla, Balanion planctonicum, BurSp., Bursaridium sp., CinMar., Cinetochilum margaritaceum, ColSte, Colpoda steinii, CycSp, Cyclidium spp., HalBif, Halteria cf. bifurcata, HalSp, Halteria sp., HymFEK, Hymenostomata FEK, MesSp., Mesodinium sp., RimSpp, Rimostrombidium spp., UndWUK, Undetermined species WUK, UroSpp, Urotricha spp., VorNat, Vorticella natans. For reservoir codes see Table 1.
The mean water temperature ranged between 5.5°C in PAN in summer and up to 15.9°C in KAI in summer (Supplementary Figure S1). While most mountain reservoirs showed relatively constant temperatures along the entire water column, PAN, SWH and FER had a temperature gradient. MBF was stratified in autumn. There is a trend for lower mean water temperatures during the second sampling period, i.e., 10.4°C vs. 12.0°C in summer. In KAI, we saw the most pronounced decrease in the mean water temperatures between summer and autumn. The mean turbidity ranged from 0.2 in FEK (highly transparent mountain reservoirs with low turbidity <1 nephelometric turbidity units, NTU) up to 7.2 NTU in PAN (turbid reservoirs ≥1.5 NTU). These data are in consensus with the measured Secchi depths, which ranged from 11.9 m (reservoir bottom) in FEK in autumn down to 0.99 m in PAN in autumn (Supplementary Table S1). The pH varied from 5.33 in WUK in autumn up to 8.70 in KAI in summer (Supplementary Table S2). In seven reservoirs (PAN, GAM, MBF, SWH, FER, SNJ, and FRO), the pH was higher in autumn than in summer, whereas no changes were observed in WUK and ROK and lower values were found in FEK and KAI in autumn. While most of the reservoirs were in a neutral range, WUK and FEK had a low pH at both sampling occasions. Accordingly, the alkalinity varied widely from −7 in WUK in summer up to 1,043 µeq L−1 in SWH in summer. The electrical conductivity ranged from 21.80 in PAN in autumn up to 197.30 μS cm−1 in FEK in autumn. Except for the higher values during the autumn sampling in FEK, the values were very similar at both sampling dates.
The values for calcium (Ca2+) ranged from 127.49 in PAN in autumn up to 1,388.31 µeq L−1 in SWH in autumn, with highest values in SWH and MBF (Supplementary Table S2). Here, we saw rather small changes in all mountain reservoirs over time, except for FEK, where Ca2+ was higher in autumn than in summer. The values for magnesium (Mg2+) varied from 36.93 in PAN in autumn up to 706.78 µeq L−1 in FEK in autumn. Five of the mountain reservoirs had Mg2+ concentrations <100 µeq L−1 (PAN, FER, FRO, KAI, and ROK), in four (WUK, GAM, SNJ, and MBF) the concentrations ranged between 100–400 µeq L−1, and in two concentrations were >400 µeq L−1 (SWH, FEK). Overall, the values were similar for both sampling occasions in all reservoirs except for FEK, i.e., ∼400 to >700 µeq L−1, respectively. Sulfate (SO42−) varied from 54.21 in FER in summer up to 1,716.80 µeq L−1 in FEK in autumn. The nitrate (NO3−) concentration ranged from 0.13 µeq L−1 in KAI in summer up to 21.94 µeq L−1 in FEK in autumn and the chloride (Cl−) concentration ranged from 2.30 in FRO in summer up to 23.00 µeq L−1 in GAM in summer.
Total phosphorus (TP) concentrations varied from 0.90 μg L−1 in FEK in autumn up to 8.89 μg L−1 in KAI in summer, with eight reservoirs having higher and three having lower concentrations in summer and autumn, respectively (Supplementary Table S1). The steepest increase was observed in PAN from 3.4–5.5 μg L−1 and the highest decrease in KAI with 8.9–5.8 μg L−1. Dissolved phosphorus (DP) concentrations were lower in autumn than in summer in all mountain reservoirs except for SNJ and FRO. Concentrations of dissolved organic carbon (DOC) ranged from 153.0 in WUK in summer up to 983.0 μg L−1 in FRO in autumn. Except for MBF, FEK and KAI, DOC concentrations were higher during the second sampling date. Dissolved nitrogen (DN) concentrations ranged from 24.5 in MBF in autumn up to 286.0 μg L−1 in FEK in autumn.
Dissolved oxygen (DO) concentrations ranged from 7.2 in KAI in summer up to 9.9 mg L−1 in PAN in summer and an oxygen saturation between 95.0 and 119.8% was observed (Supplementary Table S1). In MBF, SNJ, FRO, KAI, and ROK the oxygen concentrations were higher in autumn than in summer.
The Chl a concentrations varied between 0.02 in FEK and WUK (autumn), respectively, and 3.96 μg L−1 in GAM in autumn (Supplementary Table S1, Supplementary Figure S2A). Five reservoirs had higher concentrations in summer, while in six reservoirs Chl a contents were higher in autumn. PAN (0.53–2.82 μg L−1) and GAM (1.35–3.96 μg L−1) showed the most pronounced differences with higher values in autumn, whereas the opposite trend was found in KAI (3.70–2.25 μg L−1) and ROK (3.53–1.50 μg L−1).
The abundance of bacteria ranged from 0.14 × 106 cells mL−1 in FEK in summer up to 2.10 × 106 cells mL−1 in KAI in summer (Supplementary Table S1, Supplementary Figure S2B). In all mountain reservoirs except for KAI, bacterial numbers were higher in autumn than in summer. Zooplankton abundances ranged from 39 Ind m−3 in WUK in autumn to 1.09 × 106 Ind m−3 in KAI in autumn (Supplementary Figures S2C,D).
The PCA axis one of the environmental data reflects pH, phosphorus (total and dissolved phosphorus) and bacteria versus sulphate, conductivity, magnesium, and nitrate (Figure 4). The mountain reservoir KAI with high pH (specifically during summer), nutrients (phosphorus) and bacteria was separated from reservoirs having low pH and high nitrate, sulphate and magnesium (FEK and WUK). The largest mountain reservoir with high glacial turbidity (PAN) was clearly separated from the other study sites, particularly SWH, which showed high alkalinity and relatively high water temperatures. The difference in ordination space was larger among the different mountain reservoirs than between the sampling occasions.
Ciliate abundance, diversity and relations to abiotic and biotic factors
Total ciliate abundances ranged from 24 (mean; FEK, summer) to >15,600 Ind L−1 (mean; KAI, summer). In autumn, in PAN, SWH, FEK, SNJ, FRO, and ROK abundances were higher than in summer, while in GAM, FER, and KAI they were lower. In MBF and WUK, ciliate abundances were similar at both sampling dates. Overall, ciliate abundances were higher in summer than in autumn and decreased with depth (KAI; Figure 2). We detected 48 ciliate taxa in total with a mean of five taxa per mountain reservoir (1–8 in summer and 1–9 in autumn; min. 2 in MBF up to max. 13 in SNJ) (Figures 2, 3). We could identify 29 taxa to species, 14 to genus level and the remaining taxa were classified higher than genus level. Thirty-two taxa were found only once, 10 occurred in more than two mountain reservoirs. Cinetochilum margaritaceum (Ehrenberg), Halteria grandinella (Müller), Urotricha furcata Schewiakoff, Vorticella natans Fauré-Fremiet, and Urotricha spp. were present in 5–7 mountain reservoirs each. On average, each taxon was found in less than two mountain reservoirs. The species with the highest mean abundances were H. grandinella and V. natans with 7,323 and 4,015 Ind L−1, respectively, in KAI, as well as Rimostrombidium cf. brachykinetum Krainer with 4,765 Ind L−1 in SNJ.
In KAI, 12 ciliate taxa were found, with seven taxa in summer and nine in autumn. The mean abundance was 15,655 Ind L−1 in summer and 453 Ind L−1 in autumn, with decreasing abundances along the water column (Figure 2). Cinetochilum margaritaceum and H. grandinella appeared only in summer (max. abundance of 131 and 10,266 Ind L−1 at 2 m depth). Urotricha globosa Schewiakoff was the species with the highest abundances of up to 13,015 Ind L−1 at 2 m depth in summer. Abundances of the three Urotricha species and of Colpoda steinii Maupas decreased with depth in both summer and autumn. In autumn, four additional taxa (i.e., Rimostrombidium humile Penard, Urotricha cf. pelagica Kahl and two unidentified taxa) were found. The Shannon-Wiener Diversity Index and the Evenness were higher in autumn. Mean bacterial abundances changed from 2.10 × 106 ml−1 in summer to 1.66 × 106 ml−1 in autumn (Supplementary Table S1). Chlorophyll a values ranged from 2.2 in the upper to 5.6 μg L−1 in the lower water layers in summer to concentrations of ∼2 μg L−1 over the entire water column in autumn (Figure 2). The zooplankton abundance was higher in autumn, mainly caused by very high rotifer abundances (Supplementary Figures S2C,D).
Three mountain reservoirs were influenced by glacial turbidity during sampling (i.e., ≥1.5 NTU). In the glacier-fed turbid PAN, 11 ciliate taxa (8 in summer and 5 in autumn) were identified. The most abundant ciliates were U. globosa (526 in summer and 646 Ind L−1 in autumn), other Urotricha spp. (444 and 5,594 Ind L−1) as well as Trochilia minuta (Roux) (1,217 Ind L−1 in autumn), others were only found in low abundances (Figure 3A). While the Chl a concentration was higher in the epilimnion than in the hypolimnion in autumn, it was similar in the two layers and generally lower in summer (Supplementary Figure S2A). Bacterial abundances were twice as high in autumn than in summer and only low crustacean abundances were found in this reservoir (Supplementary Figure S2B–D). Six different ciliate taxa (3 in summer and 6 in autumn) were found in the glacially turbid FER. The total ciliate abundance was ∼5 times higher in summer (1,717 Ind L−1) than in autumn (321 Ind L−1) (Figure 3B). Bursaridium sp. (239 Ind L−1 and 84 Ind L−1 in autumn), U. furcata (929 and 4 Ind L−1) and H. grandinella (549 and 179 Ind L−1) were found during both sampling occasions. In autumn, V. natans (8 Ind L−1) and C. margaritaceum (47 Ind L−1) had higher abundances than U. furcata and Oxytricha setigera Stokes (4 Ind L−1 each). The Shannon-Wiener Diversity Index was higher, while the Evenness was slightly lower in autumn. The Chl a content of the epilimnion and hypolimnion was lower in summer than in autumn (Supplementary Figure S2B). The bacterial abundance was twice as high during the autumn sampling, while the total zooplankton abundance was lower, with rotifers having far higher abundances than crustaceans (Supplementary Figures 2B–D). In ROK, which had glacial turbidity during the autumn sampling, 8 ciliate taxa (5 each in summer and autumn, respectively) were found in total during both sampling periods. Ciliate abundances were higher in autumn (2,784 Ind L−1) than in summer (1,820 Ind L−1) (Figure 3C). Vorticella natans (30 Ind L−1 and 1,954 Ind L−1) and Urotricha pseudofurcata Krainer (442 and 765 Ind L−1) were found in high abundances in autumn or during both sampling dates, while Strobilidium caudatum (Fromentel) had the highest abundance (942 Ind L−1) in summer. The Shannon-Wiener Diversity Index and the Evenness were lower in autumn. The Chl a content was lower in autumn, while the bacterial abundance was slightly higher during the autumn sampling. Zooplankton abundances were also higher in autumn, with lower crustacean, but higher rotifer abundances (Supplementary Figure S2).
In GAM, 10 different ciliate taxa (8 in summer and 3 in autumn) were observed. The most abundant species in summer was H. grandinella (894 Ind L−1), while C. margaritaceum was found at both sampling occasions, with higher abundances in summer (65 Ind L−1) than in autumn (20 Ind L−1) and the highest abundance of the 3 species occurring in autumn (Figure 3D). The Shannon-Wiener Diversity Index and the Evenness were higher during the autumn sampling. The Chl a content was three times higher in autumn, while the bacterial abundance was similar during both sampling dates. Low abundances of crustaceans and rotifers, with higher abundances in autumn, were found (Supplementary Figure S2).
Two of the mountain reservoirs (WUK and FEK) showed low pH, alkalinity and DOC. In total, nine ciliate taxa (6 in summer and 5 in autumn) were found in WUK. Ciliate abundances were low in both summer (42 Ind L−1) and autumn (38 Ind L−1), only Urotricha spp. and Bursaridium sp. were detected at both sampling occasions (Figure 3E). The Shannon-Wiener Diversity Index and the Evenness were slightly lower in autumn, but were still high compared with the other mountain reservoirs studied here. Chlorophyll a concentrations were very low, while bacterial abundance was higher in autumn than in summer. In this mountain reservoir, no crustaceans and only rotifers were found at very low abundances (Supplementary Figure S2). In FEK, 7 ciliate taxa (6 in summer and 3 in autumn) were observed. Low abundances of C. steinii (5 and 26 Ind L−1) and an unidentified hymenostome ciliate (3 and 2 Ind L−1) were found at both sampling occasions, while Halteria sp. was present with 8 Ind L−1 in autumn. In general, ciliate abundances were low in this mountain reservoir (Figure 3F). The Shannon-Wiener Diversity Index was lower in autumn than in summer. The Chl a content was very low during both sampling occasions. The low bacterial abundance was slightly higher in autumn and only very low zooplankton abundances were found.
Two of the mountain reservoirs are connected, the water of SNJ is used to fill FRO. In SNJ, 13 ciliate taxa (6 in summer and 8 in autumn) were found in total. Ciliate abundances were almost 15 times higher in autumn than in summer (364 and 5,319 Ind L−1). Urotricha furcata (328 Ind L−1 and 193 Ind L−1) and Rimostrombidium cf. brachykinetum (3 and 4,765 Ind L−1) were the only two species present at both sampling occasions (Figure 3G). The Chl a content and bacterial abundances were higher in autumn than in summer. The total zooplankton abundance was about eight times higher (more rotifers than crustaceans) in autumn (Supplementary Figure S2). Although a total of nine ciliate taxa were found in FRO, only one ciliate species, U. furcata, was detected in summer. Total abundances were ∼30 times higher during the autumn sampling (42 and 1,265 Ind L−1), mainly caused by U. furcata, having the highest abundance (592 Ind L−1) of all ciliate species in autumn (Figure 3H). Compared with the other mountain reservoirs, the Shannon-Wiener Diversity Index was relatively high in autumn. While the Chl a concentration was similar, the bacterial and zooplankton abundances were almost three times higher during the autumn sampling.
In MBF, only two ciliate species were found. Urotricha furcata (304 and 311 Ind L−1) was observed during both sampling occasions, while Litonotus cf. lamella (Müller) was only present in summer with very low abundances (6 Ind L−1) (Figure 3I). The Shannon-Wiener Diversity Index and the Evenness were very low. The Chl a content was higher during the autumn sampling, specifically in the epilimnion. The bacterial abundance was slightly higher in autumn, while zooplankton abundances were lower and mainly made up of crustaceans (Supplementary Figure S2).
Three ciliate species were present during both sampling dates in SWH. The abundances of V. natans (14 and 26 Ind L−1) and U. furcata (199 and 372 Ind L−1) were higher in autumn, while H. grandinella abundances were higher in summer (242 and 179 Ind L−1) (Figure 3J). The Shannon-Wiener Diversity Index and the Evenness were similar between sampling dates. The Chl a content did not change, whereas bacterial abundances were higher and zooplankton abundances were lower in autumn, with rotifers decreasing more pronounced than crustaceans (Supplementary Figure S2).
The PCA analysis showed that the ciliate communities clearly differed among the mountain reservoirs. Based on the abundance data, the mountain reservoirs were separated along the first PCA axis reflecting the presence of Urotricha spp. versus an unidentified hymenostome ciliate and C. steinii (Figure 4). The latter two taxa were found at high relative abundance in the mountain reservoir with low pH, i.e., FEK. The second PCA axis stands for the presence of V. natans versus Cyclidium spp. In contrast to the abiotic parameters, the composition of the ciliate community was less similar within each mountain reservoir at the two sampling occasions, with the exception of MBF and SWH.
Environmental variables explained 40.6% of the variation in species-environment relationships in the RDA model (p < 0.05). Drivers for the overall ciliate abundance pattern (ciliates >1% mean abundance) appeared to be the pH (explaining 12.3%, p = 0.001), nitrate (12.5%, p = 0.001), Cl− (8.1%, p = 0.024), and NH4 (7.7%, p = 0.032). Alternatively, the abundance of potential predators (crustaceans) significantly explained the ciliate abundance (11.5%, p = 0.045). The pattern of the four most abundant/dominant species was significantly explained by transparency (Secchi depth; 12.7%, p = 0.034).
Ciliate species traits
In respect to the habitat preferences, we found predominantly planktonic ciliate species even in only recently filled mountain reservoirs and fewer surface-associated species (Figure 5, Supplementary Table S3). Regarding nutrition types and food sources, some single individuals of mixotrophic ciliates were observed in the mountain reservoirs (Figure 4B). Most species found could be attributed to a heterotrophic/phagotrophic nutrition mode exploiting various sources including diverse algae, heterotrophic nanoflagellates and (cyano-) bacterial food.
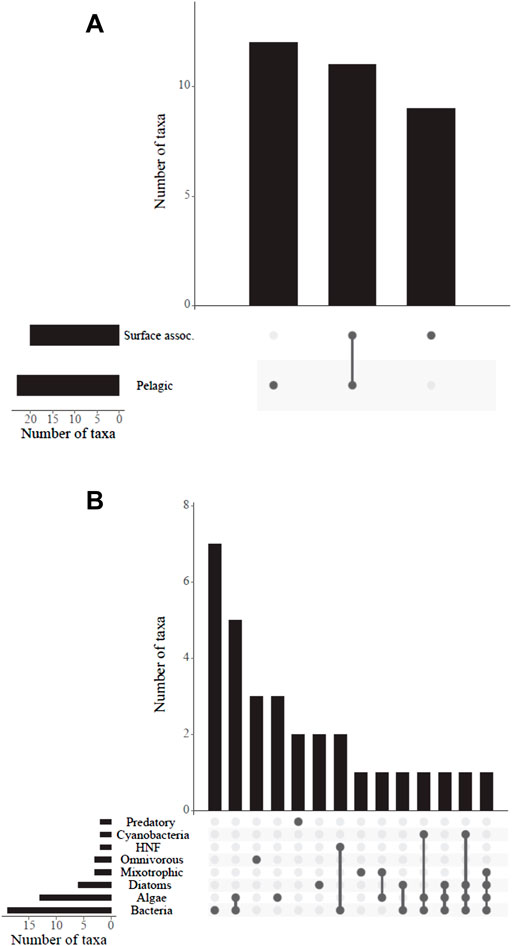
FIGURE 5. (A) Habitat preference (surface associated or pelagic) and (B) food sources and/or nutrition type of ciliates with available information (32 taxa, Foissner et al., 1999) found in the 11 mountain reservoirs. See Supplementary Table S3 for the species considered. HNF, heterotrophic nanoflagellates.
Discussion
Natural lakes are influenced by mixing processes mainly caused by wind or the inflow of water, where seasonal, geographical but also geomorphological attributes play an important role. Alpine lakes are commonly dimictic and usually stratified and the prevailing conditions affect nutrient, ion, and oxygen concentrations as well as the pH, and therefore, the biological activity of organisms (Weyhenmeyer et al., 2009). In such lakes, low water temperatures together with the generally oligotrophic conditions, result in low primary production and Chl a concentrations, accounting for low food availability in the plankton (Sherr and Sherr, 2009). Most aquatic organisms have low metabolic and growth rates at low temperatures, which is also true for ciliate species from alpine lakes (Weisse et al., 2001; Wetzel, 2001; Kammerlander et al., 2018). In man-made mountain reservoirs, temperatures are artificially altered and mixing processes intentionally induced, with yet unknown effects on the plankton communities. In summer, only three out of the eleven mountain reservoirs revealed a thermal stratification (Supplementary Figure S1), however, some natural alpine lakes may be polymictic, particularly when they are subjected to glacial melt water (Peter and Sommaruga, 2017). Glacial turbidity is an important environmental factor in alpine lakes as it not only alters temperature regimes but also protects aquatic organisms from exposure to potentially harmful ultraviolet radiation (UVR) (Sommaruga, 2001). Different turbidity levels (clear to turbid) can affect both ciliate distribution and community composition among otherwise similar alpine lakes (Kammerlander et al., 2015, 2016). While most mountain reservoirs were highly transparent (as indicated by the Secchi depths measured; Supplementary Table S1), high inorganic turbidity was also found, for example, in the glacier-fed PAN (Supplementary Figure S1A,B). Melting processes of a glacier are naturally accelerated during summer and the turbidity increases along with nutrient concentrations (TP) probably driven by an inflow of mineral particles abraded from rocks below the glacier (Slemmons et al., 2013). Interestingly, in the turbid mountain reservoir PAN, Chl a concentrations were higher in autumn than in summer, a sign for higher phytoplankton biomass despite lower solar irradiance, a phenomenon that was also observed in a natural glacier-fed lake in the same region (Kammerlander et al., 2016).
In the mountain reservoirs, both the TP and Chl a concentrations were in the ultra-oligotrophic to oligotrophic range typical for high mountain lakes in the Central Alps (Supplementary Table S1; Camarero et al., 2009; Weyhenmeyer et al., 2009). However, concentrations of Mg2+, Ca2+ and the alkalinity set some of these artificial water bodies apart from natural conditions in this region (Supplementary Table S2; Marchetto et al., 1995; Camarero et al., 2009; Weyhenmeyer et al., 2009; Rogora et al., 2020). For instance, the values for Ca2+ were on average 6.6 times higher and Mg2+ > 200 µeq L−1 were higher than the reported mean values for more than one hundred natural lakes located in the Eastern and Central Alps (Marchetto et al., 1995; Camarero et al., 2009). Also values for Na+, K+ and sulfate (SO42-) concentrations were higher than the mean values typically observed in natural alpine lakes, whereas nitrate (NO3−) and chloride concentrations were similar (Marchetto et al., 1995; Camarero et al., 2009). Our data indicate that several factors probably influenced the water chemistry of the mountain reservoirs, i.e., the artificial bottom sealing with geomembranes and the input of gravel from other geological regions. Consequently, the contact of the water to natural bedrock that is mainly composed of gneiss (Moores and Fairbridge, 2006) was reduced or prevented. In a study on young gravel pits in Canada, which, although located at lower elevations, are comparable to the mountain reservoirs in structure and age, however, significantly lower concentrations of phosphorus and nitrogen were found (Vucic et al., 2019). Another factor influencing the abiotic conditions in the mountain reservoirs was the origin of the water, though this aspect was not directly addressed here. Although the pH range in the mountain reservoirs was similar and the mean alkalinity higher than found in natural alpine lakes, the values for both parameters were still within the range common for this geographic region (Supplementary Table S2; Marchetto et al., 1995; Camarero et al., 2009). Some few ciliate specialists are known from highly acidic mining lakes (Packroff and Woelfl, 2000), but generally, low pH has a negative effect on common species such as U. furcata (pH tolerance of 5.4–9.2) that we detected in the mountain reservoirs (Figures 2, 3; Weisse and Stadler, 2006). Low pH and low Chl a concentrations (i.e., low phytoplankton biomass; Supplementary Tables S1, S2) can together with other factors result in low ciliate abundance (Beklioglu and Moss, 1995). In our study sites, the abundance of the ciliate taxa was also significantly explained by the pH. The electrical conductivity was generally low and typical for lakes at high elevations in the Alps, although levels >100 μS cm−1, as observed in four of the mountain reservoirs (Supplementary Table S2), are rarely found (Camarero et al., 2009). However, ciliate communities from freshwater are tolerant to fluctuations in conductivity as long as the values do not reach brackish or marine conditions (Pfister et al., 2002; Sonntag et al., 2002, 2006).
A low ciliate diversity that does not vary greatly over the warm season and a mean of around five taxa found in these artificial water bodies are not unusual compared to natural alpine lakes (Figures 2, 3; Wille et al., 1999; Sonntag et al., 2011a; Kammerlander et al., 2016). Though, in natural alpine lakes, temporal changes were more noticeable with higher abundances in July than in August (Kammerlander et al., 2016). Local rather than regional factors can directly affect planktonic communities (Audet et al., 2013) and our data indicate that the age of a mountain reservoir played a rather subordinate role on the ciliate diversity and that it is more likely that the frequency of complete emptying and refilling as well as the origin of the water were more influential. Indeed, these artificial systems are constantly affected by strong year-to-year fluctuations with partly very low amounts of water left at the end of the skiing season (March/April) or by complete emptying for construction and maintenance. However, we recorded the highest taxon richness in the oldest mountain reservoir SNJ (Figure 3G) where the original water body had never been completely emptied, providing more favorable conditions for a stable planktonic community (Louette et al., 2008). Moreover, environmental characteristics (e.g., pH and nutrient concentrations) and biological factors such as the presence of predators influenced the ciliate populations. Due to the individual importance and role in aquatic environments as emphasized by Foissner et al. (1991, 1992, 1994, 1995, 1999), we discuss the ciliates' appearance in the mountain reservoirs more in detail on a species-specific trait level including recent literature on their autecology. Such an approach is reasonable in respect to the few ciliate taxa found naturally in alpine lake communities that were supposed to be relatively stable throughout the ice-free period (Sonntag et al., 2011a; Kammerlander et al., 2016).
The most frequent ciliates in the mountain reservoirs were Urotricha species (Figures 2, 3), which occur worldwide in lake plankton (see Foissner and Pfister, 1997; Foissner et al., 1999; Frantal et al., 2022 and references therein, and Sonntag et al., 2022). Urotrichs are typical ciliates in lowland lakes but they are also found in almost any alpine water body (Foissner, 1979; Wille et al., 1999; Sonntag et al., 2011a, 2017; Kammerlander et al., 2016). Their occurrence even in recently filled mountain reservoirs (Figure 2) suggests that they may be considered as primary settlers or members of seed communities. However, it is unknown how urotrichs are dispersed among water bodies as cyst formation has so far never been observed. We therefore exclude wind drift, but a direct transport in water drops or faeces via birds (Szabó et al., 2022) may be possible as birds were occasionally seen at the mountain reservoirs. Alternatively, urotrichs could be washed out from the vegetation cover in the surroundings of the mountain reservoirs as, for example, in seepage and fen areas of the Rotmoos valley near the SWH, urotrichs were frequently observed (Sonntag, unpublished). Foissner et al. (1995) and Foissner and Berger (1996) defined specific ciliate communities serving as ecological indicators for plankton, biofilms in rivers or soil- and moss-influenced habitats. For example, the community of the Marynetum has been designated to mainly cyst-bearing ciliates prevailing in ephemeral small water bodies and interestingly, also Urotricha species were included. Thus, this widely distributed ciliate group indeed may be considered as primary settlers/seeds in alpine aquatic systems. Another planktonic community, i.e., the Oligotrichetea include apart from Urotricha spp., H. grandinella, Balanion planctonicum (Foissner, Oleksiv and Müller), Rimostrombidium spp. or V. natans as type species, some of which were also found dominating our studied systems (Figures 2, 3; Foissner et al., 1995). Halteria grandinella, for example, was recently identified as an important key ciliate in lake microbial food webs feeding on phytoplankton, cyanobacteria and bacteria (Foissner, 1979; Šimek et al., 2019; Forster et al., 2021). The species is very common and widely distributed in diverse habitats and cyst formation is known (Foissner et al., 1999). For V. natans, interestingly, this is the first report on its presence in alpine water bodies although the species can be frequently found in lowland lakes and reservoirs and is able to tolerate also low temperatures (Foissner et al., 1999; Lu et al., 2020). Therefore, mountain reservoirs seem to provide a suitable habitat for this species. Again, as for the urotrichs, we can only speculate about a direct distribution of V. natans among the mountain reservoirs by birds as cyst formation is unknown. Another ciliate that has been found in a glacier-fed turbid lake in high numbers was Mesodinium cf. acarus Stein (Kammerlander et al., 2016), while in our study individuals of this genus were only detected in three transparent mountain reservoirs (Figure 3). Overall, predominantly planktonic species were found in our studied systems (Figure 5A).
Nevertheless, some other ciliate species were identified that can be clearly attributed to a surface-associated life, e.g., T. minuta, Aspidisca spp., Chilodonella uncinata (Ehrenberg) and Litonotus spp. (Figure 3). These ciliates were assigned to the community of the Cyrtophoretea that typically occur in clear streams, pointing to the origin of water used for filling the mountain reservoirs. Another species that is frequently found in both alpine lakes and streams is C. margaritaceum that we detected in seven mountain reservoirs (Figures 2, 3; Foissner et al., 1994; Kammerlander et al., 2016). Although this species has not been designated as planktonic before because its cirri are more feasible for crawling than swimming, we assume that it colonizes detritus and other floating particles to feed on the biofilm.
A further factor known to directly influence the species-specific ciliate distribution along depth gradients in alpine lakes is lake water transparency and therefore the impact of UVR (Sonntag et al., 2011a, 2011b, 2017; Kammerlander et al., 2016). In accordance with former studies (Kammerlander et al., 2016; Kammerlander et al., 2018), transparency (Secchi depth) played a significant role in shaping the ciliate communities here. Heterotrophic ciliates such as one Bursaridium species were found to resist high incident UVR levels by the accumulation of sunscreen compounds from algal food whereas others, e.g., Askenasia chlorelligera Krainer and Foissner hosted algal endosymbionts that synthesized the sunscreen compounds directly (Sonntag et al., 2007; 2017; Summerer et al., 2008; Sonntag and Sommaruga, 2020). Although we detected almost no mixotrophic algal-bearing ciliates in the mountain reservoirs, this functional trait of combining phagotrophy and hosting a symbiotic partner is very common in natural ciliate communities in both alpine and lowland lakes (Wille et al., 1999; Sonntag et al., 2006, 2011a, 2011b; Kammerlander et al., 2015). Though we do not want to overestimate the absence of this common trait from the ciliate plankton, we assume that the ciliate communities in the mountain reservoirs could not reach a certain stability level by lacking mixotrophic species or assemblages. However, relying on a phagotrophic nutrition mode is certainly advantageous, especially in transparent water bodies where sunscreen compounds could be acquired from algal food. Although not tested yet for prostomatid ciliates including urotrichs or B. planctonicum that voraciously forage on phytoplankton, the colpodid Bursaridium could definitely profit from this functional trait (Sonntag et al., 2017).
Moreover, bottom-up control by food sources and top-down control by zooplankton, particularly crustaceans, which were present in most of the mountain reservoirs (Supplementary Figure S2D), affected not only the total ciliate abundance, but likely also the ciliate community composition. Cyclopoid copepods and daphnids, which occurred in seven and three, respectively, of the study sites, but also rotifers are known to feed on small ciliates (Archbold and Berger, 1985; Arndt, 1993; Gilbert and Jack, 1993; Wickham 1995; Weisse and Frahm, 2002; Zingel et al., 2016). Algal-feeding ciliates might also be strong competitors to rotifers as ciliates can release secondary metabolites into the medium inhibiting rotifer reproduction (Pisman, 2009). However, many planktonic ciliate species perform fast jumps to escape predators and such an escape reaction is also typical for ciliates of the genera Urotricha and Halteria (Foissner et al., 1999). Weisse and Frahm (2002), for example, identified species-specific predator-prey relationships among different rotifer species, of which one species (Keratella quadrata Müller) was also found in three of the mountain reservoirs together with Urotricha spp. and B. planctonicum. However, species-specific predator-prey relationships still need to be addressed in future experimental approaches.
In conclusion, man-made mountain reservoirs are systems that show unique physico-chemical and biological features, but also similarities with natural alpine lakes. Mainly planktonic species were found and the ciliate abundances were influenced by the prevailing environmental conditions (pH, nutrient concentrations, water transparency) as well as by biological factors such as the presence and abundance of predators. Water level fluctuations and irregular water withdrawal as well as the process of emptying the mountain reservoirs seemed to play a minor role in shaping the community composition or the abundance of ciliates. Their communities are most likely a reflection of the source of water and colonization processes. Although dispersal by resting cysts was not tested here, formation of cysts is known for some of the species found. Human-induced water level fluctuations up to complete emptying of the mountain reservoirs can cause inhomogeneous conditions over short time periods, which distinguishes those systems from natural alpine lakes.
Data availability statement
The raw data supporting the conclusion of this article will be made available by the authors, without undue reservation.
Author Contributions
BT, MS and BS conceived and designed the study. FS, BT and BS wrote the main manuscript, all co-authors contributed to writing. FS and NR collected the samples. FS investigated the ciliates, NR the zooplankton, MS the bacteria. All authors approved the final manuscript.
Funding
The study was funded by the Swarovski Science Foundation: grant P7430-017-012 (BT) and by the Austrian Science Fund (FWF): I2238-B25 (BS), P28333-B25 (BS).
Acknowledgments
We thank Elias Dechent, Barbara Kammerlander, Gry Larsen, Salvador Moralez-Gomez, Ulrike Scheffel, and Jonas Watterodt for support in the field and in the laboratory. We thank the Bergbahnen Kühtai GmbH and Co KG, Fisser Bergbahnen GmbH, Hochgurgler Liftgesellschaft GmbH and Co KG, Liftgesellschaft Obergurgl GmbH, Ötztaler Gletscherbahn GmbH and Co KG, Pitztaler Gletscherbahnen GmbH and Co KG, Skiliftgesellschaft Sölden-Hochsölden GmbH, and Wintersport Tirol AG for sampling permission and their teams, in particular Thomas Kirschner, Andreas Kleinlercher, Michael Mair, Willi Mareiler, Peter Reich, Alexander Schmid, and Reinhold Streng, for logistical help.
Conflict of interest
The authors declare that the research was conducted in the absence of any commercial or financial relationships that could be construed as a potential conflict of interest.
Publisher’s note
All claims expressed in this article are solely those of the authors and do not necessarily represent those of their affiliated organizations, or those of the publisher, the editors and the reviewers. Any product that may be evaluated in this article, or claim that may be made by its manufacturer, is not guaranteed or endorsed by the publisher.
Supplementary material
The Supplementary Material for this article can be found online at: https://www.frontiersin.org/articles/10.3389/fenvs.2022.903095/full#supplementary-material
References
Archbold, J. H. G., and Berger, J. (1985). A qualitative assessment of some metazoan predators of Halteria grandinella, a common freshwater ciliate. Hydrobiologia 126, 97–102.
Arndt, H. (1993). Rotifers as predators on components of the microbial web (bacteria, heterotrophic flagellates, ciliates) - A review. Hydrobiologia 255/266, 231–246. doi:10.1007/BF00025844
Audet, C., MacPhee, S., and Keller, W. (2013). Colonization of constructed ponds by crustacean zooplankton: Local and regional influences. J. Limnol. 72, 43. doi:10.4081/jlimnol.2013.e43
Badosa, A., Frisch, D., Green, A. J., Rico, C., and Gómez, A. (2017). Isolation mediates persistent founder effects on zooplankton colonisation in new temporary ponds. Sci. Rep. 7, 43983. doi:10.1038/srep43983
Beklioglu, M., and Moss, B. (1995). The impact of pH on interactions among phytoplankton algae, zooplankton and perch (Perca fluviatilis) in a shallow, fertile lake. Freshw. Biol. 33, 497–509. doi:10.1111/j.1365-2427.1995.tb00409.x
Blatterer, H. (2008). Review on in situ studies of ciliates (Protozoa: Ciliophora) from running waters. Denisia 23, 337–359.
Breiling, M., and Charamza, P. (1999). The impact of global warming on winter tourism and skiing: A regionalised model for Austrian snow conditions. Reg. Environ. Change 1, 4–14. doi:10.1007/s101130050003
Cáceres, C. E., and Soluk, D. A. (2002). Blowing in the wind: A field test of overland dispersal and colonization by aquatic invertebrates. Oecologia 131, 402–408. doi:10.1007/s00442-002-0897-5
Camarero, L., Rogora, M., Mosello, R., Anderson, N. J., Barbieri, A., Botev, I., et al. (2009). Regionalisation of chemical variability in European mountain lakes. Freshw. Biol. 54, 2452–2469. doi:10.1111/j.1365-2427.2009.02296.x
Catalan, J., and Donato Rondón, J. C. (2016). Perspectives for an integrated understanding of tropical and temperate high-mountain lakes. J. Limnol. 75, 215–234. doi:10.4081/jlimnol.2016.1372
Del Giorgio, P. A., Bird, D. F., Prairie, Y. T., and Planas, D. (1996). Flow cytometric determination of bacterial abundance in lake plankton with the green nucleic acid stain SYTO 13. Limnol. Oceanogr. 41, 783–789. doi:10.4319/lo.1996.41.4.0783
Dziallas, C., Allgaier, M., Monaghan, M. T., and Grossart, H.-P. (2012). Act together-implications of symbioses in aquatic ciliates. Front. Microbiol. 3, 288. doi:10.3389/fmicb.2012.00288
Einsle, U. (1993). Crustacea Copepoda Calanoida und Cyclopoida. Stuttgart, Jena, New York: Gustav Fischer Verlag.
Elser, J. J., Wu, C., González, A. L., Shain, D. H., Smith, H. J., Sommaruga, R., et al. (2020). Key rules of life and the fading cryosphere: Impacts in alpine lakes and streams. Glob. Chang. Biol. 26, 6644–6656. doi:10.1111/gcb.15362
Figuerola, J., and Green, A. J. (2002). Dispersal of aquatic organisms by waterbirds: A review of past research and priorities for future studies. Freshw. Biol. 47, 483–494. doi:10.1046/j.1365-2427.2002.00829.x
Flößner, D. (2000). Die Haplopoda und Cladocera (ohne Bosminidae) Mitteleuropas. Leiden: Backhuys Publishers.
Foissner, W., and Berger, H. (1996). A user-friendly guide to the ciliates (Protozoa, Ciliophora) commonly used by hydrobiologists as bioindicators in rivers, lakes, and waste-waters, with notes on their ecology. Freshw. Biol. 35, 375–482. doi:10.1111/j.1365-2427.1996.tb01775.x
Foissner, W., Berger, H., and Blatterer, H. (1995). Taxonomische und ökologische Revision der Ciliaten des Saprobiensystems: Band IV: Gymnostomatea, Loxodes, Suctoria. Munich: Informationsberichte des Bayer. Landesamtes für Wasserwirtschaft. Munich: Bayer. Bayer: Landesamtes für Wasserwirtschaft.
Foissner, W., Berger, H., and Kohmann, F. (1992). Taxonomische und ökologische Revision der Ciliaten des Saprobiensystems: Band II: Peritrichia, Heterotrichida, Odontostomatida. Munich: Informationsberichte des Bayer. Landesamtes für Wasserwirtschaft. Munich: Bayer. Bayer: Landesamtes für Wasserwirtschaft.
Foissner, W., Berger, H., and Kohmann, F. (1994). Taxonomische und ökologische Revision der Ciliaten des Saprobiensystems. Band III: Hymenostomata, Prostomatida, Nassulida. Munich: Informationsberichte des Bayer. Bayer: Landesamtes für Wasserwirtschaft.
Foissner, W., Berger, H., and Schaumburg, J. (1999). Identification and ecology of limnetic plankton ciliates. MunichBayer: Landesamt für Wasserwirtschaft.
Foissner, W. (2006). Biogeography and dispersal of micro-organisms: A review emphasizing protists. Acta Protozool. 45, 111–136.
Foissner, W., Blatterer, H., Berger, H., and Kohmann, F. (1991). Taxonomische und ökologische Revision der Ciliaten des Saprobiensystems: Band I: Cyrtophorida, Oligotrichida, Hypotrichia, Colpodea. Munich: Informationsberichte des Bayer. Bayer: Landesamtes für Wasserwirtschaft.
Foissner, W. (1979). Ökologische und systematische Studien über das Neuston alpiner Kleingewässer, mit besonderer Berücksichtigung der Ciliaten. Int. Rev. Ges. Hydrobiol. Hydrogr. 64, 99–140. doi:10.1002/iroh.19790640109
Foissner, W., and Pfister, G. (1997). Taxonomic and ecologic revision of urotrichs (Ciliophora, Prostomatida) with three or more caudal cilia, including a user-friendly key. Limnologica 27, 311–347.
Forster, D., Qu, Z., Pitsch, G., Bruni, E. P., Kammerlander, B., Pröschold, T., et al. (2021). Lake ecosystem robustness and resilience inferred from a climate-stressed protistan plankton network. Microorganisms 9 (3), 549. doi:10.3390/microorganisms9030549
Frantal, D., Agatha, S., Beisser, D., Boenigk, J., Darienko, T., Dirren-Pitsch, G., et al. (2022). Molecular data reveal a cryptic diversity in the genus Urotricha (Alveolata, Ciliophora, Prostomatida), a key player in freshwater lakes, with remarks on morphology, food preferences, and distribution. Front. Microbiol. 12, 787290. doi:10.3389/fmicb.2021.787290
Gilbert, J. J., and Jack, J. D. (1993). Rotifers as predators on small ciliates. Hydrobiologia 255/256, 247–253. doi:10.1007/BF00025845
Kammerlander, B., Breiner, H. W., Filker, S., Sommaruga, R., Sonntag, B., Stoeck, T., et al. (2015). High diversity of protistan plankton communities in remote high mountain lakes in the European Alps and the Himalayan mountains. FEMS Microbiol. Ecol. 91 (4), fiv010. doi:10.1093/femsec/fiv010
Kammerlander, B., Koinig, K. A., Rott, E., Sommaruga, R., Tartarotti, B., Trattner, F., et al. (2016). Ciliate community structure and interactions within the planktonic food web in two alpine lakes of contrasting transparency. Freshw. Biol. 61, 1950–1965. doi:10.1111/fwb.12828
Kammerlander, B., Tartarotti, B., and Sonntag, B. (2018). The impact of UV radiation on Paramecium populations from alpine lakes. J. Euk. Microbiol. 65, 250–254. doi:10.1111/jeu.12463
Kernan, M., Ventura, M., Bitušík, P., Brancelj, A., Clarke, G., Velle, G., et al. (2009). Regionalisation of remote European mountain lake ecosystems according to their biota: Environmental versus geographical patterns. Freshw. Biol. 54, 2470–2493. doi:10.1111/j.1365-2427.2009.02284.x
Klein, G., Vitasse, Y., Rixen, C., Marty, C., and Rebetez, M. (2016). Shorter snow cover duration since 1970 in the Swiss Alps due to earlier snowmelt more than to later snow onset. Clim. Change 139, 637–649. doi:10.1007/s10584-016-1806-y
Legendre, P., and Gallagher, E. (2001). Ecologically meaningful transformations for ordination of species data. Oecologia 129, 271–280. doi:10.1007/s004420100716
Lorenzen, C. J. (1967). Determination of chlorophyll and pheo-pigments: Spectrophotometric equations. Limnol. Oceanogr. 12, 343–346. doi:10.4319/lo.1967.12.2.0343
Louette, G., de Meester, L., and de Declerck, S. (2008). Assembly of zooplankton communities in newly created ponds. Freshw. Biol. 53, 2309. doi:10.1111/j.1365-2427.2008.02052.x
Lu, X., Gao, Y., and Weisse, T. (2020). Functional ecology of two contrasting freshwater ciliated protists in relation to temperature. J. Eukaryot. Microbiol. 68, e12823. doi:10.1111/jeu.12823
Marchetto, A., Mosello, R., Psenner, R., Bendetta, G., Boggero, A., Tait, D., et al. (1995). Factors affecting water chemistry of alpine lakes. Aquat. Sci. 57, 81–89. doi:10.1007/BF00878028
Moores, E. M., and Fairbridge, R. W. (2006). Encyclopedia of European and asian regional geology. Netherlands: Springer.
Mühlenberg, M. (1993). Freilandökologie: UTB für Wissenschaft. Uni-Taschenbücher. Heidelberg u. Wiesbaden: Quelle & Meyer Verlag.
Ortiz-Álvarez, R., Triadó-Margarit, X., Camarero, L., Casamayor, E. O., and Catalan, J. (2018). High planktonic diversity in mountain lakes contains similar contributions of autotrophic, heterotrophic and parasitic eukaryotic life forms. Sci. Rep. 8, 4457. doi:10.1038/s41598-018-22835-3
Pace, M. L. (1982). Planktonic ciliates: Their distribution, abundance, and relationship to microbial resources in a monomictic lake. Can. J. Fish. Aquat. Sci. 39, 1106–1116. doi:10.1139/f82-148
Packroff, G., and Woelfl, S. (2000). A review on the occurrence and taxonomy of heterotrophic protists in extreme acidic environments of pH values ≤ 3. Hydrobiologia 433, 153–156. doi:10.1023/A:1004039309694
Pastorino, P., and Prearo, M. (2020). High-mountain lakes, indicators of global change: Ecological characterization and environmental pressures. Diversity 12, 260. doi:10.3390/d12060260
Peter, H., and Sommaruga, R. (2017). Alpine glacier-fed turbid lakes are discontinuous cold polymictic rather than dimictic. Inland Waters 7, 45–54. doi:10.1080/20442041.2017.1294346
Pfister, G., Auer, B., and Arndt, H. (2002). Pelagic ciliates (Protozoa, Ciliophora) of different brackish and freshwater lakes - A community analysis at the species level. Limnologica 32, 147–168. doi:10.1016/S0075-9511(02)80005-6
Pfister, G., Sonntag, B., and Posch, T. (1999). Comparison of a direct live count and an improved quantitative protargol stain (QPS) in determining abundance and cell volumes of pelagic freshwater protozoa. Aquat. Microb. Ecol. 18, 95–103. doi:10.3354/ame018095
Pisman, T. I. (2009). Experimental and mathematical model of the interactions in the mixed culture of links in the ‘producer-consumer’ cycle. Adv. Space Res. 44, 177–183. doi:10.1016/j.asr.2009.01.022
Pröschold, T., Rieser, D., Darienko, T., Kammerlander, B., Pitsch, G., Bruni, E. P., et al. (2021). An integrative approach sheds new light onto the systematics and ecology of the widespread ciliate genus Coleps (Ciliophora, Prostomatea). Sci. Rep. 11, 5916. doi:10.1038/s41598-021-84265-y
Qu, Z., Forster, D., Bruni, E. P., Frantal, D., Kammerlander, B., Nachbaur, L., et al. (2021). Aquatic food webs in deep temperate lakes: Key species establish through their autecological versatility. Mol. Ecol. 30, 1053–1071. doi:10.1111/mec.15776
Rogora, M., Somaschini, L., Marchetto, A., Mosello, R., Tartari, G. A., Paro, L., et al. (2020). Decadal trends in water chemistry of Alpine lakes in calcareous catchments driven by climate change. Sci. Total Environ. 708, 135180. doi:10.1016/j.scitotenv.2019.135180
Rose, K. C., Williamson, C. E., Saros, J. E., Sommaruga, R., and Fischer, J. M. (2009). Differences in UV transparency and thermal structure between alpine and subalpine lakes: Implications for organisms. Photochem. Photobiol. Sci. 8, 1244. doi:10.1039/b905616e
Sherr, E. B., and Sherr, B. F. (2009). Encyclopedia of microbiology: Microbial food webs. San Diego: Academic Press.
Shurin, J. B. (2000). Dispersal limitation, invasion resistance, and the structure of pond zooplankton communities. Ecology 81, 3074. doi:10.1890/0012-9658(2000)081[3074:DLIRAT]2.0.CO;2
Šimek, K., Grujčić, V., Nedoma, J., Jezberová, J., Šorf, M., Matoušů, A., et al. (2019). Microbial food webs in hypertrophic fishponds: Omnivorous ciliate taxa are major protistan bacterivores. Limnol. Oceanogr. 64, 2295–2309. doi:10.1002/lno.11260
Skibbe, O. (1994). An improved quantitative protargol stain for ciliates and other planktonic protists. Arch. Hydrobiol. 130, 339–347. doi:10.1127/archiv-hydrobiol/130/1994/339
Slemmons, K. E. H., Saros, J. E., and Simon, K. (2013). The influence of glacial meltwater on alpine aquatic ecosystems: A review. Environ. Sci. Process. Impacts 15, 1794. doi:10.1039/c3em00243h
Smits, J. D., and Milne, P. J. (1981). Spectrophotometric determination of siliciate in natural waters by formation of α-molybdosilicic acid and reduction with tin(IV)-ascorbic acid-oxalic acid mixture. Anal. Chim. Acta. 123, 263–270. doi:10.1016/S0003-2670(01)83179-2
Sommaruga, R. (2001). The role of solar UV radiation in the ecology of alpine lakes. J. Photochem. Photobiol. B Biol. 62, 35–42. doi:10.1016/S1011-1344(01)00154-3
Sonntag, B., Frantal, D., Kammerlander, B., Darienko, T., Filker, F., Stoeck, T., et al. (2022). Widespread occurrence of two planktonic ciliate species (Urotricha, Prostomatida) originating from high mountain lakes. Diversity 14 (5), 362. doi:10.3390/d14050362
Sonntag, B., Frantal, D., Kammerlander, B., Darienko, T., Filker, S., Stoeck, T., et al. (2022). Widespread occurrence of two planktonic ciliate species (Urotricha, Prostomatida) originating from high mountain lakes. Diversity 14, 362. doi:10.3390/d14050362
Sonntag, B., Kammerlander, B., and Summerer, M. (2017). Bioaccumulation of ultraviolet sunscreen compounds (mycosporine-like amino acids) by the heterotrophic freshwater ciliate Bursaridium living in alpine lakes. Inland Waters 7, 55–64. doi:10.1080/20442041.2017.1294348
Sonntag, B., Posch, T., Klammer, S., Griebler, C., and Psenner, R. (2002). Protozooplankton in the deep oligotrophic Traunsee (Austria) influenced by discharges of soda and salt industries. Water Air Soil Pollut. Focus 2, 211–226. doi:10.1023/A:1020360125761
Sonntag, B., Posch, T., Klammer, S., Teubner, K., and Psenner, R. (2006). Phagotrophic ciliates and flagellates in an oligotrophic, deep, alpine lake: Contrasting variability with seasons and depths. Aquat. Microb. Ecol. 43, 193–207. doi:10.3354/ame043193
Sonntag, B., and Sommaruga, R. (2020). Effectiveness of photoprotective strategies in three mixotrophic planktonic ciliate species. Diversity 12, 252. doi:10.3390/d12060252
Sonntag, B., Summerer, M., and Sommaruga, R. (2011b). Are freshwater mixotrophic ciliates less sensitive to solar UV radiation than heterotrophic ones? J. Eukaryot. Microbiol. 58, 196–202. doi:10.1111/j.1550-7408.2011.00540.x
Sonntag, B., Summerer, M., and Sommaruga, R. (2011a). Factors involved in the distribution pattern of ciliates in the water column of a transparent alpine lake. J. Plankton Res. 33, 541–546. doi:10.1093/plankt/fbq117
Sonntag, B., Summerer, M., and Sommaruga, R. (2007). Sources of mycosporine-like amino acids in planktonic Chlorellabearing ciliates (Ciliophora). Freshw. Biol. 52, 1476–1485. doi:10.1111/j.1365-2427.2007.01778.x
Summerer, M., Sonntag, B., and Sommaruga, R. (2008). Ciliate-symbiont specificity of freshwater endosymbiotic Chlorella (Trebouxiophyceae, Chlorophyta). J. Phycol. 44, 77–84. doi:10.1111/j.1529-8817.2007.00455.x
Szabó, B., Szabó, A., Vad, C. F., Boros, E., Lukić, D., Ptacnik, R., et al. (2022). Microbial stowaways: Waterbirds as dispersal vectors of aquatic pro- and microeukaryotic communities. J. Biogeogr. 00, 1286–1298. doi:10.1111/jbi.14381
ter Braak, C., and Šmilauer, P. (2012). Canoco reference manual and user’s guide: Software for ordination. Itaca: Microcomputer power. Available at: http://www.canoco.com.version 5.0
Voigt, M., and Koste, W. (1978a). Rotatoria. Die Rädertiere Mitteleuropas. Überordnung Monogononta. I. Textband. Berlin-Stuttgart: Gebrüder Borntraeger.
Voigt, M., and Koste, W. (1978b). Rotatoria. Die Rädertiere Mitteleuropas. Überordnung Monogononta. II. Textband. Berlin-Stuttgart: Gebrüder Borntraeger.
Vucic, J. M., Cohen, R. S., Gray, D. K., Murdoch, A. D., Shuvo, A., Sharma, S., et al. (2019). Young gravel-pit lakes along Canada’s Dempster Highway: How do they compare with natural lakes? Arct. Antarct. Alp. Res. 51, 25–39. doi:10.1080/15230430.2019.1565854
Weisse, T., and Frahm, A. (2002). Direct and indirect impact of two common rotifer species (Keratella spp.) on two abundant ciliate species (Urotricha furcata, Balanion planctonicum). Freshw. Biol. 47, 53–64. doi:10.1046/j.1365-2427.2002.00780.x
Weisse, T. (2017). Functional diversity of aquatic ciliates. Eur. J. Protistol. 61, 331–358. doi:10.1016/j.ejop.2017.04.001
Weisse, T., Karstens, N., Meyer, V. C. L., Janke, L., Lettner, S., Teichgräber, K., et al. (2001). Niche separation in common prostome freshwater ciliates: The effect of food and temperature. Aquat. Microb. Ecol. 26, 167–179. doi:10.3354/ame026167
Weisse, T., and Stadler, P. (2006). Effect of pH on growth, cell volume, and production of freshwater ciliates, and implications for their distribution. Limnol. Oceanogr. 51, 1708–1715. doi:10.4319/lo.2006.51.4.1708
Weyhenmeyer, G. A., Psenner, R., and Tranvik, L. J. (2009). “Lakes of Europe,” in Encyclopedia of inland waters. Editor Likens, G. E. (Amsterdam: Elsevier), 567–576.
Wickham, S. A. (1995). Cyclops predation on ciliates - Species-specific differences and functional responses. J. Plankton Res. 17, 1633–1646. doi:10.1093/plankt/17.8.1633
Wille, A., Sonntag, B., Sattler, B., and Psenner, R. (1999). Abundance, biomass and size structure of the microbial assemblage in the high mountain lake Gossenköllesee (Tyrol, Austria) during the ice-free period. J. Limnol.. 58, 117–126. doi:10.4081/jlimnol.1999.117
Xu, Y., Stoeck, T., Forster, D., Ma, Z., Zhang, L., Fan, X., et al. (2018). Environmental status assessment using biological traits analyses and functional diversity indices of benthic ciliate communities. Mar. Pollut. Bull. 131, 646–654. doi:10.1016/j.marpolbul.2018.04.064
Keywords: plankton, freshwater, artificial lakes, species traits, indicator organisms, alpine region
Citation: Sommer F, Sonntag B, Rastl N, Summerer M and Tartarotti B (2022) Ciliates in man-made mountain reservoirs. Front. Environ. Sci. 10:903095. doi: 10.3389/fenvs.2022.903095
Received: 23 March 2022; Accepted: 27 June 2022;
Published: 22 July 2022.
Edited by:
Juliana Dias, Federal University of Rio Grande do Norte, BrazilReviewed by:
Simone Jaqueline Cardoso, Juiz de Fora Federal University, BrazilS. S. S. Sarma, Universidad Nacional Autónoma de México, Mexico
Copyright © 2022 Sommer, Sonntag, Rastl, Summerer and Tartarotti. This is an open-access article distributed under the terms of the Creative Commons Attribution License (CC BY). The use, distribution or reproduction in other forums is permitted, provided the original author(s) and the copyright owner(s) are credited and that the original publication in this journal is cited, in accordance with accepted academic practice. No use, distribution or reproduction is permitted which does not comply with these terms.
*Correspondence: Barbara Tartarotti, YmFyYmFyYS50YXJ0YXJvdHRpQHVpYmsuYWMuYXQ=