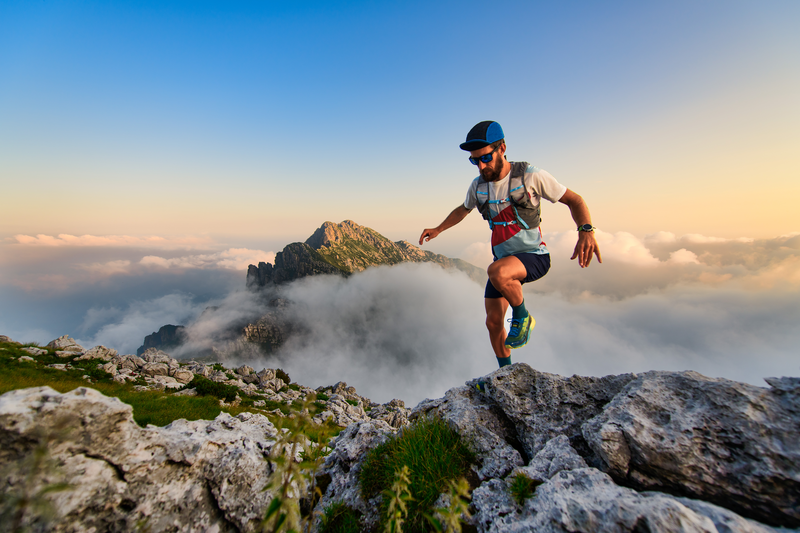
95% of researchers rate our articles as excellent or good
Learn more about the work of our research integrity team to safeguard the quality of each article we publish.
Find out more
MINI REVIEW article
Front. Environ. Sci. , 04 May 2022
Sec. Toxicology, Pollution and the Environment
Volume 10 - 2022 | https://doi.org/10.3389/fenvs.2022.902915
This article is part of the Research Topic High-efficiency Strategies for Bioconversion of Waste to Bioenergy/Resource toward Toxicity Elimination and Carbon Neutralization View all 8 articles
Plant invasion caused due to various human activities has become a serious problem affecting ecosystem diversity and imposes a burden on the economy. In recent years, there have been increasing studies on the application of biochar (BC) in the field of environmental protection. Invasive plants, which are considered as a kind of hazardous waste biomass, can be used as feedstocks to prepare BC. Consumption of invasive plants for BC preparation can achieve a win-win situation in ecology and resources. This can solve a series of ecological problems caused by invasive plants to a certain extent while also realizing the resource utilization of wastes and bringing considerable economic benefits. Based on previous studies, this paper summarizes the progress of preparing and using invasive plant biochar (IPB). This includes the production, modification, merit and demerit of IPB, its application in improving soil quality, the adsorption of pollutants, application in energy storage, and climate change mitigation potential. It provides a basis for further study of IPB based on the currently existing problems and proposes a direction for future development.
Invasive plants are one of the important factors that affect the global ecological environment (Simberloff et al., 2013). Due to the strong adaptability and rapid spreading ability of invasive plants, their negative impact on the local ecosystem cannot be ignored. Many effective methods to limit the invasion of alien plants have been derived, including chemical, biological, and alternative controls (Terry et al., 2021). These treatment methods have certain effects in long-term practice but still require considerable manpower and material resources. Hence, we need to find a more economical and effective way to deal with invasive plants. As an emerging material for environmental protection, biochar (BC) is characterized by its economy and efficiency (Beesley et al., 2011). Studies show that biochar production from invasive plants is suitable for mitigating ecological damage caused by biological invasion (Feng et al., 2021). Biochar production using invasive plants as raw materials realizes the effective utilization of waste and controls the expansion of invasive plants to a certain extent. Thus, this method can potentially alleviate the issue of invasive plants.
Biochar possesses excellent physical and chemical properties. It can be prepared by pyrolysis of waste biomass (Manya, 2012; Tan et al., 2015; Feng et al., 2021). Presently, biochar is often used to improve the soil environment and remove various pollutants in the environment. Hence, it is an important material that aids in environment recovery (Zhang et al., 2013; Ahmad et al., 2014b; Mohan et al., 2014). However, the physical and chemical properties of biochar are greatly affected by raw materials and preparation methods. Hence, finding suitable raw materials and corresponding preparation methods is particularly important.
Invasive plants are extremely diverse and renewable; therefore, using these plants as raw materials for biochar will be more economical and easier to obtain than other biomass wastes (Wang et al., 2021). Some researchers have used invasive plants as raw materials to produce biochar which is mainly used in agricultural soil improvement and pollutant adsorption (Cheng et al., 2017; Beckinghausen et al., 2020). However, the advantages and disadvantages of invasive plant biochar (IPB) and their preparation methods need to be systematically analyzed. Most studies about IPB are focused on its application in environmental protection, while there is little information about its application in the field of energy. There are still many challenges in the implementation of IPB that need further analysis and understanding. Therefore, based on the characteristics of invasive plants and the preparation method of IPB, this paper focuses on the preparation and modification methods of biochar using invasive plants as raw materials, as well as on the application and potential of IPB in the environmental and energy field. Finally, this paper elucidates the current problems facing IPB and the possible future developmental direction of IPB research.
Invasive plants are those that are introduced into a non-native or alien environment and are capable of adept multiplication and can cause damage to the environment, economy, or human health (Prabakaran et al., 2019). Invasive plants usually have the following characteristics: 1) Strong ecological adaptability and high genetic diversity; 2) Strong and rapid reproductive ability, i.e., they can produce offspring in adverse environments; 3) Strong transmission capacity, which is suitable for the transmission of seeds or propagators through media, and a high transmission rate (Wan et al., 2010; Meng et al., 2020).
Some of the most adaptable plants appear in non-native environments due to intentional, unintentional, or accidental human activity (Leprieur et al., 2008; Blackburn et al., 2011; Besnard and Cuneo, 2016). Generally, there are three main pathways of plant invasion: 1) natural invasion; 2) initiative taken to introduce; 3) passive spread (Rahel and Olden, 2008; Hulme, 2009). After successfully entering a new habitat, invasive plants often gain a great competitive advantage over native plants due to the lack of natural enemies and a suitable living environment. Simultaneously, invasive plants may produce various organic acids, allelopathic substances, and hormones, which can destroy the structure of rhizosphere soil microbial community and interrupt the interaction between the soil community and native plants. This results in successful invasion (Simberloff et al., 2013; Jabran et al., 2015; Zhang et al., 2019).
The inherent ability of plants to undergo rapid adaptive evolution on genotypes or exhibit phenotypic plasticity makes them formidable invaders (Prabakaran et al., 2019). Due to the characteristics of strong adaptability and rapid growth, invasive plants can cause significant harm to the local ecological environment (Vila et al., 2011; Eviner et al., 2017). Invasive plants increase the risk of extinction of native species by disrupting biogeographical areas and encroaching on their living space and resources. Many invasive plants also alter ecosystem functions by altering nutrient cycling, habitat structure, and disturbance mechanisms (Pysek et al., 2020). Concurrently, some invasive plants release toxic substances while consuming survival resources, which critically threatens the health and safety of humans and livestock (Weller et al., 2015; Jank and Rath, 2021; Simmons et al., 2021). The economic cost of plant invasion is increasing worldwide, which brings great financial burden to the local economy (Leung et al., 2002; Diagne et al., 2021).
Commonly used methods for the processing of invasive plants are mechanical control (removal by using artificial or mechanical cleaning, shading, and other ways to remove invasive plants), chemical control (through the use of chemicals to kill or control invasive plants), and biological control (introducing other biological agents to control the growth of invasive plants) (Hussner et al., 2017; Weihua Li et al., 2015). Using invasive plants in different ways is a desirable method to reduce the damage caused by them. As shown in Figure 1, common utilization methods of invasive plants include composting, feed, activated carbon, biochar, etc. (Reaser et al., 2007; Atyosi et al., 2019; Feng et al., 2021). Since traditional methods to deal with invasive plants consume significant manpower and material resources, we urgently need more economical and efficient ways to deal with invasive plants.
Currently, the invasive plants most commonly used as raw materials for BC are water hyacinth, Eupatorium Adenophorum, Spartina Alterniflora, Alternanthera Philoxeroides, Solidago Canadensis L, etc. (Nguyen D. T. C. et al., 2021; Cui et al., 2022). These invasive plants are mostly herbaceous, abundant and easily accessible. It is beneficial to collect a large amount of raw materials to prepare BC. The stems of herbaceous plants are densely covered with relatively small vascular bundles, between which are a large number of thin-walled cells (Buranov and Mazza, 2008), which is more conducive to the preparation of BC with large specific surface area and well-developed porosity.
The most common way to prepare biochar is by pyrolysis (Jahirul et al., 2012). Pyrolysis conditions (temperature, heating rate, etc.) have a significant effect on the physicochemical properties of biochar (Mašek et al., 2013). During thermal decomposition, hemicellulose, cellulose, and lignin (components of biomass) are crosslinked, depolymerized, and cleaved at their respective temperatures to produce solid, liquid, and gaseous products (Cha et al., 2016). Biochar preparation by pyrolysis has the advantages of simplicity and low cost.
At present, there are numerous relevant studies on the preparation of biochar using invasive plants as raw materials (GuhaRay et al., 2019; Velez et al., 2018). In addition to the direct preparation of biochar by pyrolysis, modified biochar can also be prepared by pre-treatment of raw materials to improve the relevant properties of IPB (Saravanakumar et al., 2019; Zeng et al., 2019). This can aid in enhancing its applicability in various scenarios. Using invasive plants as the carrier, biochar was modified by acid and base, supported metal and its oxides, oxidizer, etc., and had better performance than the original biochar (Figure 1). Through acid modification, the surface area of BC can be changed to a certain extent while also removing metal and other impurities on the surface of biochar and introducing oxygen-containing functional groups (Shen et al., 2010). Alkali modification can increase the specific surface area (BET) and oxygen-containing functional groups of BC (Ahmed et al., 2016). The modification of BC by metal or metal oxide can enhance the adsorption and catalytic performance and endow it with magnetic properties, which is conducive to recycling (Li et al., 2021). Oxidant modification can increase oxygen-containing functional groups on BC, which helps improve its performance (Uchimiya et al., 2012) (Table 1).
Invasive plants used to prepare biochar often have porous structures (such as water hyacinth). This enables the resulting biochar to have a larger surface area and provide more active sites, which greatly improves the ability of IPB to adsorb pollutants (Zhuang et al., 2020). Pyrolysis temperature also affects the properties of IPB. As high temperatures can remove some impurities in IPB, the porosity of IPB increases with increasing temperature. Simultaneously, high temperatures can increase the carbonization degree of biochar and carbon content of biochar. Eupatorium adenophorum biochar prepared at high temperature has good stability, and the removal effect of heavy metals is better (Fan et al., 2019). Pyrolysis temperature also affects the functional groups on biochar (Xiaoling Dong et al., 2013). At lower temperatures, the degree of carbonization of IPB is not high, so there are more oxygen-containing functional groups on the surface. The specific surface area and pore volume of IPB can be changed by acid-base modification, and some surface functional groups can be introduced to improve the adsorption performance of biochar (Sizmur et al., 2017). The modification of supported metal and its oxides mainly uses the binding force between supported metal elements and biochar to improve adsorption. Metal element oxides attached to the surface of biochar can provide more adsorption sites and can also endow magnetic characteristics to biochar, which is convenient for separation, recovery, and regeneration. Water hyacinth biochar was used as the carrier of Fe3O4 particles to achieve effective separation and recovery of materials (Zhuang et al., 2020). Citric acid was applied for biochar modification (raw material: Anemonis crematis), which introduced extensive carboxyl groups on the surface of biochar and generated numerous active sites (Xu et al., 2016).
The preparation of BC from invasive plants has obvious advantages. Invasive plants are easy to obtain and provide a large number of high quality and cheap raw materials for BC preparation. Consumption of invasive plants for BC preparation can achieve a win-win situation in ecology and resources. Invasive plants have inherent structural advantages, such as the hollow structure of water hyacinth, which can achieve excellent structural characteristics in the initial BC. Most invasive plants are herbaceous and have high cellulose content. In the pyrolysis process, cellulose is easily volatilized by combustion, so BC produced by invasive plants is characterized by abundant pores. The use of invasive plants to prepare BC is still limited. Invasive plant species are abundant, but only a few of them are used to prepare BC. Meanwhile, due to the high content of cellulose and hemicellulose, the yield of BC produced from invasive plants was relatively low. In the actual production process, invasive plants can be selected according to needs. To generate BC with large specific surface area and abundant pores, invasive herbaceous plants with high cellulose content can be used. For those with high yield requirements, the selection range can be expanded to adopt invasive plants with high lignin content.
Biochar is an excellent soil conditioner and can be used for improving soil quality. It ameliorates the soil environment by 1) affecting soil physical and chemical properties and 2) affecting microbial activity. It can increase soil moisture and nutrient retention capacity, promote growth and biological activity of soil microflora, reduce nutrient leaching, increase circulation of soil nutrients, and increase soil organic carbon, which promotes the growth of plants. Biochar is generally alkaline and can be used as a soil modifier to neutralize soil acidity and raise soil pH (Yuan et al., 2011). Similar to other kinds of biochars, when IPB is used to improve soil quality, it can also improve soil fertility, reduce the damage of pollutants on soil quality, and improve the quality and yield of agricultural products, as well as it can reduce the persecution of local species by invasive plants. This IPB application method has good developmental prospects and can be widely used.
It was reported that fine-grained water hyacinth biochar reduced soil macropores and contributed to water retention by capillary action, and the hydrophilic surface bond (OH) of biochar further improved water retention capacity (Bordoloi et al., 2019). The pores of IPB can also provide a certain water retention capacity, which can offer resistance to the development of tensile force of soil cracks (Li et al., 2009). Water hyacinth biochar has higher aromaticity and carbon stability than the initial biomass at 300–350°C. After experimental analysis, it was found that water hyacinth biochar significantly improved soil biological activity, with acid phosphatase and alkaline phosphatase activity increasing by 32 and 22.8%, respectively, thereby increasing soil activity by 3 times (Masto et al., 2013). The abundance of pores in IPB can also provide important habitats for microbes. Through field trials, Lantana camara biochar significantly reduced soil bulk density and exchangeable acidity, while significantly increasing soil total porosity, pH, total nitrogen, organic carbon, and available phosphorus and potassium (Berihun et al., 2017). Organic anions can quickly neutralize soil acidity in acidic soil, so the improvement effect of carbonates in biochar on acidic soil can be long-lasting. The effects of Spartina alterniflora biochar application on the germination and growth of Salinaria salinosa were investigated under three conditions: non-flooding, intermittent flooding, and continuous flooding. IPB had positive effects on the growth of Salinaria and improved the rhizosphere soil quality, which indicated that biochar is feasible for soil remediation in coastal wetlands (Cai et al., 2021). Additionally, biomass and grain yield of maize treated by the Eupatorium adenophorum biochar were significantly improved. This was partly due to changes in soil physical properties, which included improved drainage and air permeability of heavy clay upon the addition of biochar (Obia et al., 2018). IPB can alleviate water, nutrient, and acid stress in soil. Further, the addition of Eupatorium adenophorum biochar improved soil nutrient availability and promoted plant growth by increasing soil pH, Ca/Al ratio, and available phosphorus to keep soil pH stable (Pandit et al., 2018).
With the rapid development of various industries, heavy metals and organic pollutants have increasing influence on the environment and are one of the main challenges to be overcome in the field of environmental sciences and conservation/sustainability (Yang et al., 2018; Hu et al., 2020). At present, IPB is mainly used in environmental remediation to adsorb pollutants in water or soil and reduce the persistence of pollutants in the ecological environment. The IPB has good adsorption and removal effect for some pollutants. Simultaneously, the use of IPB can reduce the ecological harm caused by invasive plants, realize the resource utilization of waste, and reduce the production cost of adsorbents.
The maximum adsorption capacity for Pb(II) of the Alternanthera philoxeroides biochar was 257.12 mg g−1, which was 5.3 times that of common activated carbon (Yang et al., 2014). The adsorption capacity (Kd value) of water hyacinth biochar for heavy metals (Cd, Cu, Pb, and Zn) is greater than 104 L kg−1, and the removal rate is up to 99.9% in the range of low metal concentration. Thus, it shows high adsorption performance and low adsorption reversibility (Doumer et al., 2016). Biochar prepared with Prosopis juliflora as raw material can be effectively separated by a magnetic field from the treated water after modification with doped magnetic NiO, and the adsorption rate of Pb(II) increased (Saravanakumar et al., 2019). Nitrogen-doped biochar prepared from Pistia stratiotes has a large pore structure and abundant surface functional groups. Through hydrogen bonding, Lewis acid-base interaction, and functional group interaction, the adsorption capacity of diethyl phthalate can reach 161.7 mg g−1 (Zhang et al., 2021). Water hyacinth biochar was modified with citric acid, and carboxyl group was added to the surface of the biochar under esterification. The modified water hyacinth biochar had good regeneration adsorption performance and the maximum adsorption capacity for methylene blue was improved (up to 395 mg g−1) (Xu et al., 2016). The removal effect of 2,4,6-trichlorophenol (2,4,6-TCP) in water by nano-iron/nickel bimetal loaded with Eupatorium adenophorum biochar is significant and the degradation rate of 2,4,6-TCP can be increased by 39.7–71.6% under different conditions (Guo Liu et al., 2019).
Contaminants in soil tend to exist in more complex forms than in water and are harder to remove. IPB can complete the adsorption of soil pollutants to some extent. At 600–700°C and pH 6, the Cu(II) removal rates of Spartina alterniflora biochar and water hyacinth biochar were 29.4 and 28.2 mg g−1, respectively. The process of Cu(II) fixation had no significant effect on Na/K/Mg leaching, nutrient cycling (especially K), and heavy metal retention could be achieved simultaneously (Mi Li et al., 2015). Spartina alterniflora biochar prepared under low temperature pyrolysis conditions (350 and 450°C) is beneficial to the passivation of Cd in soil, and the effective Cd content decreases by up to 26.9%, while the increase of soil salinity is not significant. Therefore, Spartina alterniflora biochar can be used to treat Cd pollution in coastal saline-alkali soil (Cai et al., 2020).
Biochar is a promising energy storage material with easily regulated surface chemical properties, multi-purpose porous structure, and abundant surface functional groups. Biochar can play an important role in various energy storage conversion reactions and processes (Wu-Jun Liu et al., 2019). It can be used to produce supercapacitors and batteries (Li-ion, Na-ion, Li-S, and metal-Air) (Saning et al., 2019; Senthil and Lee, 2021). In the recent study, a novel and low cost biochar-phase change materials (PCM) hybrid latent heat energy storage material was developed by the addition of water hyacinth biochar as a supporting matrix. It was found that water hyacinth biochar had a 13.82-fold increased coefficient of thermal conductivity of PCM, and possessed enhanced stability due to the high carbon content and porosity of biochar (Das et al., 2020). Water hyacinth biochar could absorb metal ions, which helped to improve thermal conductivity in the production of PCM and solved the problem of low thermal conductivity in traditional PCM (Muigai et al., 2020). Hierarchical porous carbon based on water hyacinth biochar could realize rapid ion transfer simultaneously by coupling its layered porous structure with high specific surface area, and provide rich active sites for energy storage (Mo et al., 2020). The biochar produced by water hyacinth under high temperature conditions had the characteristics of porous and high graphitization, which contributed to overcome the water degradation of ygroscopic perovskite layer and had better air stability. It could be used to realize the continuous manufacture of perovskite solar cells (Pitchaiya et al., 2020). Water hyacinth absorbed Ni2+ in water through phytoremediation technology and could be used as feedstock to prepare biochar. Metal ions could be introduced naturally, and the electrochemical performance of water hyacinth biochar could be significantly improved (Shell et al., 2021). IPB has great development potential in energy storage, more researches about the application of IPB in energy storage are needed in the future.
Biochar has received increasing attention as a method of almost permanently locking atmospheric carbon in the soil through carbon-negative processes. The pyrolysis of biochar from invasive plants can improve carbon stability and form persistent carbon storage in soil (Gaurav et al., 2020). Applying biochar to agricultural soils can increase soil carbon sequestration, stabilize soil organic carbon pool, inhibit soil CO2 flux, and reduce greenhouse gas emissions to mitigate climate change (Da Dong et al., 2013; Wang et al., 2014). Studies have shown that IBP has a variable impact on greenhouse gas emissions, which is reflected by the significant decrease in soil N2O emissions, increase in soil CH4 uptake, and complex changes (negative, positive, or negligible) in soil CO2 emissions (Li et al., 2017). Meta-analyses from laboratory and potted and field studies found that biochar application significantly affected soil greenhouse gas fluxes and their global warming undercurrent values and reduced soil N2O fluxes by 30.92% (He et al., 2017). IPB could be applied to the soil to reduce nitrous oxide emissions by reducing the use of fertilizers and lime (Simmons et al., 2021). The extent and process of climate change mitigation through IPB application requires further in-depth research.
The ecological damage caused by invasive plants worldwide cannot be ignored, and efficient mitigation policies are necessary to tackle the problems caused by invasive plants. Using invasive plants to prepare biochar can turn problematic weeds into valuable products, thereby taking advantage of them. This method has become an effective way to control invasive plants by reducing the control cost and realizing the sustainable utilization of resources. Invasive plants, especially water hyacinth, Solidago canadas and Alternanthera philoxeroides are excellent raw materials for biochar preparation. Furthermore, the current usage of IPB and modified biochar elucidates that its performance can be enhanced and meet more environmental protection requirements. In this paper, the common methods of biochar preparation by invasive plants and their application in the environmental and energy storage field were discussed based on previous studies. This can provide a basis for the future management of invasive plants. Biochar preparation from invasive plants can solve ecological problems caused by invasive plants to a certain extent while also providing economic value, realizing resource utilization of waste, and meeting the requirements of sustainable utilization. However, there are still some challenges and obstacles in the application of IPB:
1) There are many kinds of invasive plants, but the existed invasive plants are not fully utilized. Therefore, various invasive plants should be considered while selecting raw materials of biochar in the future.
2) The ability of the original IPB to mitigate environmental problems may be insufficient to meet future environmental conservation requirements. Therefore, various modification methods must be considered to significantly improve the performance of IPB.
3) Some biochars produced from invasive plants (such as water hyacinth) showed superior properties and performance in the specific applications. The advantages of invasive plants compared to other raw materials of biochar need further verification.
4) Many invasive plants have desirable structures that can meet the requirements of energy storage materials. Biochar preparation from invasive plants also has great potential in energy storage, but research on this aspect is rare.
5) Fully utilizing invasive plants to prepare biochar can further achieve carbon sequestration. However, there is still some controversy over whether biochar can effectively mitigate climate change, and further research should be carried out in this regard in the future.
LY and XT participate in the outline planning, the data collection, figures design, writing and editing. YD and ZS participated in the writing and editing. QC, HY, and XT participated in the editing and review. XT participated in project administration and funding acquisition. All authors contributed to the article and approved the submitted version.
This research was financially supported by the National Key R&D Program of China (2020YFC1807600), the Natural Science Foundation of Hunan Province, China (Grant Nos. 2021JJ30123).
The authors declare that the research was conducted in the absence of any commercial or financial relationships that could be construed as a potential conflict of interest.
All claims expressed in this article are solely those of the authors and do not necessarily represent those of their affiliated organizations, or those of the publisher, the editors and the reviewers. Any product that may be evaluated in this article, or claim that may be made by its manufacturer, is not guaranteed or endorsed by the publisher.
Ahmad, M., Moon, D. H., Vithanage, M., Koutsospyros, A., Lee, S. S., Yang, J. E., et al. (2014a). Production and Use of Biochar from Buffalo-Weed (Ambrosia trifidaL.) for Trichloroethylene Removal from Water. J. Chem. Technol. Biotechnol. 89 (1), 150–157. doi:10.1002/jctb.4157
Ahmad, M., Rajapaksha, A. U., Lim, J. E., Zhang, M., Bolan, N., Mohan, D., et al. (2014b). Biochar as a Sorbent for Contaminant Management in Soil and Water: a Review. Chemosphere 99, 19–33. doi:10.1016/j.chemosphere.2013.10.071
Ahmed, M. B., Zhou, J. L., Ngo, H. H., Guo, W., and Chen, M. (2016). Progress in the Preparation and Application of Modified Biochar for Improved Contaminant Removal from Water and Wastewater. Bioresour. Technol. 214, 836–851. doi:10.1016/j.biortech.2016.05.057
Atyosi, Z., Ramarumo, L. J., and Maroyi, A. (2019). Alien Plants in the Eastern Cape Province in South Africa: Perceptions of Their Contributions to Livelihoods of Local Communities. Sustainability 11 (18), 5043. doi:10.3390/su11185043
Beckinghausen, A., Reynders, J., Merckel, R., Wu, Y. W., Marais, H., and Schwede, S. (2020). Post-pyrolysis Treatments of Biochars from Sewage Sludge and A. Mearnsii for Ammonia (NH4-n) Recovery. Appl. Energy 271, 115212. doi:10.1016/j.apenergy.2020.115212
Beesley, L., Moreno-Jiménez, E., Gomez-Eyles, J. L., Harris, E., Robinson, B., and Sizmur, T. (2011). A Review of Biochars' Potential Role in the Remediation, Revegetation and Restoration of Contaminated Soils. Environ. Pollut. 159 (12), 3269–3282. doi:10.1016/j.envpol.2011.07.023
Berihun, T., Tadele, M., and Kebede, F. (2017). The Application of Biochar on Soil Acidity and Other Physico‐chemical Properties of Soils in Southern Ethiopia. J. Plant Nutr. Soil Sci. 180 (3), 381–388. doi:10.1002/jpln.201600343
Besnard, G., and Cuneo, P. (2016). An Ecological and Evolutionary Perspective on the Parallel Invasion of Two Cross-Compatible Trees. AoB Plants 8, plw056. doi:10.1093/aobpla/plw056
Blackburn, T. M., Pyšek, P., Bacher, S., Carlton, J. T., Duncan, R. P., Jarošík, V., et al. (2011). A Proposed Unified Framework for Biological Invasions. Trends Ecol. Evol. 26 (7), 333–339. doi:10.1016/j.tree.2011.03.023
Bordoloi, S., Gopal, P., Boddu, R., Wang, Q., Cheng, Y.-F., Garg, A., et al. (2019). Soil-biochar-water Interactions: Role of Biochar from Eichhornia crassipes in Influencing Crack Propagation and Suction in Unsaturated Soils. J. Clean. Prod. 210, 847–859. doi:10.1016/j.jclepro.2018.11.051
Buranov, A. U., and Mazza, G. (2008). Lignin in Straw of Herbaceous Crops. Industrial Crops Prod. 28 (3), 237–259. doi:10.1016/j.indcrop.2008.03.008
Cai, J.-F., Zhang, L., Zhang, Y., Zhang, M.-X., Li, H.-L., Xia, H.-J., et al. (2020). Remediation of Cadmium-Contaminated Coastal Saline-Alkaline Soil by Spartina Alterniflora Derived Biochar. Ecotoxicol. Environ. Saf. 205, 111172. doi:10.1016/j.ecoenv.2020.111172
Cai, J.-F., Fan Jiang, J., Liu, X.-S., Sun, K., Wang, W., Zhang, M.-X., et al. (2021). Biochar-amended Coastal Wetland Soil Enhances Growth of Suaeda Salsa and Alters Rhizosphere Soil Nutrients and Microbial Communities. Sci. Total Environ. 788, 147707. doi:10.1016/j.scitotenv.2021.147707
Cha, J. S., Park, S. H., Jung, S.-C., Ryu, C., Jeon, J.-K., Shin, M.-C., et al. (2016). Production and Utilization of Biochar: A Review. J. Industrial Eng. Chem. 40, 1–15. doi:10.1016/j.jiec.2016.06.002
Chen, X.-L., Li, F., Xie, X. J., Li, Z., and Chen, L. (2019). Nanoscale Zero-Valent Iron and Chitosan Functionalized Eichhornia crassipes Biochar for Efficient Hexavalent Chromium Removal. Int. J. Environ. Res. Public Health 16 (17), 3046. doi:10.3390/ijerph16173046
Cheng, S., Zhang, S., Zhang, L., Xia, H., Peng, J., and Wang, S. (2017). Microwave-Assisted Preparation of Activated Carbon from Eupatorium Adenophorum: Effects of Preparation Parameters. High. Temp. Mat. P. R. 36 (8), 805–814. doi:10.1515/htmp-2015-0285
Cui, X., Wang, J., Wang, X., Khan, M. B., Lu, M., Khan, K. Y., et al. (2022). Biochar from Constructed Wetland Biomass Waste: A Review of its Potential and Challenges. Chemosphere 287 (Pt 3), 132259. doi:10.1016/j.chemosphere.2021.132259
Da Dong, D., Yang, M., Wang, C., Wang, H., Li, Y., Luo, J., et al. (2013). Responses of Methane Emissions and Rice Yield to Applications of Biochar and Straw in a Paddy Field. J. Soils Sediments 13 (8), 1450–1460. doi:10.1007/s11368-013-0732-0
Das, D., Bordoloi, U., Muigai, H. H., and Kalita, P. (2020). A Novel Form Stable PCM Based Bio Composite Material for Solar Thermal Energy Storage Applications. J. Energy Storage 30, 101403. doi:10.1016/j.est.2020.101403
Diagne, C., Leroy, B., Vaissière, A.-C., Gozlan, R. E., Roiz, D., Jarić, I., et al. (2021). High and Rising Economic Costs of Biological Invasions Worldwide. Nature 592 (7855), 571–576. doi:10.1038/s41586-021-03405-6
Dong, L., Li, S., Jin, Y., Hu, B., and Sheng, G. (2021). Enhanced Adsorption of Eu(III) from Wastewater Using Solidago Canadensis-Derived Biochar Functionalized by Ca/Al-LDH and Hydroxyapatite. Appl. Surf. Sci. 567, 150794. doi:10.1016/j.apsusc.2021.150794
Doumer, M. E., Rigol, A., Vidal, M., and Mangrich, A. S. (2016). Removal of Cd, Cu, Pb, and Zn from Aqueous Solutions by Biochars. Environ. Sci. Pollut. Res. 23 (3), 2684–2692. doi:10.1007/s11356-015-5486-3
Du, Y.-d., Feng, Y., Shu, L., Ren, Z.-j., Kong, Q., Xu, F., et al. (2018). A Mesoporous Biochar from Bio-Invasion alligator Weed for Adsorption of Rhodamine B from Aqueous Solution. Desalination Water Treat. 135, 341–350. doi:10.5004/dwt.2018.22616
Eviner, V. T., Garbach, K., Baty, J. H., and Hoskinson, S. A. (2017). Measuring the Effects of Invasive Plants on Ecosystem Services: Challenges and Prospects. Invasive plant Sci. manag. 5 (1), 125–136. doi:10.1614/ipsm-d-11-00095.1
Fan, L., Zhou, X., Liu, Q., Wan, Y., Cai, J., Chen, W., et al. (2019). Properties of Eupatorium Adenophora Spreng (Crofton Weed) Biochar Produced at Different Pyrolysis Temperatures. Environ. Eng. Sci. 36 (8), 937–946. doi:10.1089/ees.2019.0028
Feng, Q., Wang, B., Chen, M., Wu, P., Lee, X., and Xing, Y. (2021). Invasive Plants as Potential Sustainable Feedstocks for Biochar Production and Multiple Applications: A Review. Resour. Conservation Recycl. 164, 105204. doi:10.1016/j.resconrec.2020.105204
Gaurav, G. K., Mehmood, T., Cheng, L., Klemeš, J. J., and Shrivastava, D. K. (2020). Water Hyacinth as a Biomass: A Review. J. Clean. Prod. 277, 122214. doi:10.1016/j.jclepro.2020.122214
GuhaRay, A., Guoxiong, M., Sarkar, A., Bordoloi, S., Garg, A., and Pattanayak, S. (2019). Geotechnical and Chemical Characterization of Expansive Clayey Soil Amended by Biochar Derived from Invasive Weed Species Prosopis Juliflora. Innov. Infrastruct. Solut. 4 (1), 44. doi:10.1007/s41062-019-0231-2
Guo Liu, G., Tang, H., Fan, J., Xie, Z., He, T., Shi, R., et al. (2019). Removal of 2,4,6-trichlorophenol from Water by Eupatorium Adenophorum Biochar-Loaded Nano-Iron/nickel. Bioresour. Technol. 289, 121734. doi:10.1016/j.biortech.2019.121734
He, Y., Zhou, X., Jiang, L., Li, M., Du, Z., Zhou, G., et al. (2017). Effects of Biochar Application on Soil Greenhouse Gas Fluxes: a Meta-Analysis. GCB Bioenergy 9 (4), 743–755. doi:10.1111/gcbb.12376
Hu, B., Ai, Y., Jin, J., Hayat, T., Alsaedi, A., Zhuang, L., et al. (2020). Efficient Elimination of Organic and Inorganic Pollutants by Biochar and Biochar-Based Materials. Biochar 2 (1), 47–64. doi:10.1007/s42773-020-00044-4
Huang, X., Liu, Y., Liu, S., Li, Z., Tan, X., Ding, Y., et al. (2016). Removal of Metformin Hydrochloride by Alternanthera Philoxeroides Biomass Derived Porous Carbon Materials Treated with Hydrogen Peroxide. RSC Adv. 6 (83), 79275–79284. doi:10.1039/c6ra08365j
Hulme, P. E. (2009). Trade, Transport and Trouble: Managing Invasive Species Pathways in an Era of Globalization. J. Appl. Ecol. 46 (1), 10–18. doi:10.1111/j.1365-2664.2008.01600.x
Hussain, R., Kumar Ghosh, K., and Ravi, K. (2021). Impact of Biochar Produced from Hardwood of Mesquite on the Hydraulic and Physical Properties of Compacted Soils for Potential Application in Engineered Structures. Geoderma 385, 114836. doi:10.1016/j.geoderma.2020.114836
Hussner, A., Stiers, I., Verhofstad, M. J. J. M., Bakker, E. S., Grutters, B. M. C., Haury, J., et al. (2017). Management and Control Methods of Invasive Alien Freshwater Aquatic Plants: A Review. Aquat. Bot. 136, 112–137. doi:10.1016/j.aquabot.2016.08.002
Jabran, K., Mahajan, G., Sardana, V., and Chauhan, B. S. (2015). Allelopathy for Weed Control in Agricultural Systems. Crop Prot. 72, 57–65. doi:10.1016/j.cropro.2015.03.004
Jahirul, M., Rasul, M., Chowdhury, A., and Ashwath, N. (2012). Biofuels Production through Biomass Pyrolysis -A Technological Review. Energies 5 (12), 4952–5001. doi:10.3390/en5124952
Jank, B., and Rath, J. (2021). Emerging Tropane Alkaloid Contaminations under Climate Change. Trends Plant Sci. 26 (11), 1101–1103. doi:10.1016/j.tplants.2021.08.001
Leprieur, F., Beauchard, O., Blanchet, S., Oberdorff, T., and Brosse, S. (2008). Fish Invasions in the World's River Systems: when Natural Processes Are Blurred by Human Activities. PLoS Biol. 6 (2), e28. doi:10.1371/journal.pbio.0060028
Leung, B., Lodge, D. M., Finnoff, D., Shogren, J. F., Lewis, M. A., and Lamberti, G. (2002). An Ounce of Prevention or a Pound of Cure: Bioeconomic Risk Analysis of Invasive Species. Proc. R. Soc. Lond. B 269 (1508), 2407–2413. doi:10.1098/rspb.2002.2179
Li, J. H., Zhang, L. M., Wang, Y., and Fredlund, D. G. (2009). Permeability Tensor and Representative Elementary Volume of Saturated Cracked Soil. Can. Geotech. J. 46 (8), 928–942. doi:10.1139/t09-037
Li, Y., Hu, S., Chen, J., Müller, K., Li, Y., Fu, W., et al. (2017). Effects of Biochar Application in Forest Ecosystems on Soil Properties and Greenhouse Gas Emissions: a Review. J. Soils Sediments 18 (2), 546–563. doi:10.1007/s11368-017-1906-y
Li, Y., Gao, L., Wang, Y., Cheng, S., Wu, G., Yang, X., et al. (2021). Development of an Acidized Biochar-Supported Hydrated Fe(III) Oxides for Highly Efficient Cadmium and Copper Sequestration from Water. Sci. Total Environ. 784, 147017. doi:10.1016/j.scitotenv.2021.147017
Liu, D., Tang, Y., Li, J., Hao, Z., Zhu, J., Wei, J., et al. (2021). Eupatorium Adenophorum Derived Adsorbent by Hydrothermal-Assisted HNO3 Modification and Application to Pb2+ Adsorption. J. Environ. Chem. Eng. 9 (5), 105972. doi:10.1016/j.jece.2021.105972
Manyà, J. J. (2012). Pyrolysis for Biochar Purposes: a Review to Establish Current Knowledge Gaps and Research Needs. Environ. Sci. Technol. 46 (15), 7939–7954. doi:10.1021/es301029g
Mašek, O., Brownsort, P., Cross, A., and Sohi, S. (2013). Influence of Production Conditions on the Yield and Environmental Stability of Biochar. Fuel 103, 151–155. doi:10.1016/j.fuel.2011.08.044
Masto, R. E., Kumar, S., Rout, T. K., Sarkar, P., George, J., and Ram, L. C. (2013). Biochar from Water Hyacinth (Eichornia Crassipes) and its Impact on Soil Biological Activity. Catena 111, 64–71. doi:10.1016/j.catena.2013.06.025
Meng, W., Feagin, R. A., Innocenti, R. A., Hu, B., He, M., and Li, H. (2020). Invasion and Ecological Effects of Exotic Smooth Cordgrass Spartina Alterniflora in China. Ecol. Eng. 143, 105670. doi:10.1016/j.ecoleng.2019.105670
Mi Li, M., Lou, Z., Wang, Y., Liu, Q., Zhang, Y., Zhou, J., et al. (2015). Alkali and Alkaline Earth Metallic (AAEM) Species Leaching and Cu(II) Sorption by Biochar. Chemosphere 119, 778–785. doi:10.1016/j.chemosphere.2014.08.033
Mo, C., Zhang, J., and Zhang, G. (2020). Hierarchical Porous Carbon with Three Dimensional Nanonetwork from Water Hyacinth Leaves for Energy Storage. J. Energy Storage 32, 101848. doi:10.1016/j.est.2020.101848
Mohan, D., Sarswat, A., Ok, Y. S., and Pittman, C. U. (2014). Organic and Inorganic Contaminants Removal from Water with Biochar, a Renewable, Low Cost and Sustainable Adsorbent - A Critical Review. Bioresour. Technol. 160, 191–202. doi:10.1016/j.biortech.2014.01.120
Muigai, H. H., Bordoloi, U., Hussain, R., Ravi, K., Moholkar, V. S., and Kalita, P. (2020). A Comparative Study on Synthesis and Characterization of Biochars Derived from Lignocellulosic Biomass for Their Candidacy in Agronomy and Energy Applications. Int. J. Energy Res. 45 (3), 4765–4781. doi:10.1002/er.6092
Nguyen, D. T. C., Le, H. T. N., Nguyen, T. T., Nguyen, T. T. T., Liew, R. K., Bach, L. G., et al. (2021a). Engineering Conversion of Asteraceae Plants into Biochars for Exploring Potential Applications: A Review. Sci. Total Environ. 797, 149195. doi:10.1016/j.scitotenv.2021.149195
Nguyen, T. T. H., Nguyen, X. C., Nguyen, D. L. T., Nguyen, D. D., Vo, T. Y. B., Vo, Q. N., et al. (2021b). Converting Biomass of Agrowastes and Invasive Plant into Alternative Materials for Water Remediation. Biomass Conv. bioref. doi:10.1007/s13399-021-01526-6
Obia, A., Mulder, J., Hale, S. E., Nurida, N. L., and Cornelissen, G. (2018). The Potential of Biochar in Improving Drainage, Aeration and Maize Yields in Heavy Clay Soils. PLoS One 13 (5), e0196794. doi:10.1371/journal.pone.0196794
Pandit, N. R., Mulder, J., Hale, S. E., Martinsen, V., Schmidt, H. P., and Cornelissen, G. (2018). Biochar Improves Maize Growth by Alleviation of Nutrient Stress in a Moderately Acidic Low-Input Nepalese Soil. Sci. Total Environ. 625, 1380–1389. doi:10.1016/j.scitotenv.2018.01.022
Pitchaiya, S., Eswaramoorthy, N., Natarajan, M., Santhanam, A., Asokan, V., Madurai Ramakrishnan, V., et al. (2020). Perovskite Solar Cells: A Porous Graphitic Carbon Based Hole Transporter/Counter Electrode Material Extracted from an Invasive Plant Species Eichhornia Crassipes. Sci. Rep. 10 (1), 6835. doi:10.1038/s41598-020-62900-4
Prabakaran, K., Li, J., Anandkumar, A., Leng, Z., Zou, C. B., and Du, D. (2019). Managing Environmental Contamination through Phytoremediation by Invasive Plants: A Review. Ecol. Eng. 138, 28–37. doi:10.1016/j.ecoleng.2019.07.002
Pyšek, P., Hulme, P. E., Simberloff, D., Bacher, S., Blackburn, T. M., Carlton, J. T., et al. (2020). Scientists' Warning on Invasive Alien Species. Biol. Rev. 95 (6), 1511–1534. doi:10.1111/brv.12627
Rahel, F. J., and Olden, J. D. (2008). Assessing the Effects of Climate Change on Aquatic Invasive Species. Conserv. Biol. 22 (3), 521–533. doi:10.1111/j.1523-1739.2008.00950.x
Raj, F. R. M. S., Boopathi, G., Kalpana, D., Jaya, N. V., and Pandurangan, A. (2022). Sustainable Development through Restoration of Prosopis Juliflora Species into Activated Carbon as Electrode Material for Supercapacitors. Diam. Relat. Mater. 121, 108767. doi:10.1016/j.diamond.2021.108767
Rajapaksha, A. U., Vithanage, M., Ahmad, M., Seo, D.-C., Cho, J.-S., Lee, S.-E., et al. (2015). Enhanced Sulfamethazine Removal by Steam-Activated Invasive Plant-Derived Biochar. J. Hazard. Mater. 290, 43–50. doi:10.1016/j.jhazmat.2015.02.046
Reaser, J. K., Meyerson, L. A., and Von Holle, B. (2007). Saving Camels from Straws: How Propagule Pressure-Based Prevention Policies Can Reduce the Risk of Biological Invasion. Biol. Invasions 10 (7), 1085–1098. doi:10.1007/s10530-007-9186-x
Saning, A., Herou, S., Dechtrirat, D., Ieosakulrat, C., Pakawatpanurut, P., Kaowphong, S., et al. (2019). Green and Sustainable Zero-Waste Conversion of Water Hyacinth (Eichhornia crassipes) into Superior Magnetic Carbon Composite Adsorbents and Supercapacitor Electrodes. RSC Adv. 9 (42), 24248–24258. doi:10.1039/c9ra03873f
Saravanakumar, R., Muthukumaran, K., and Selvaraju, N. (2019). Enhanced Pb (II) Ions Removal by Using Magnetic NiO/Biochar Composite. Mat. Res. Express 6 (10), 105504. doi:10.1088/2053-1591/ab2141
Senthil, C., and Lee, C. W. (2021). Biomass-derived Biochar Materials as Sustainable Energy Sources for Electrochemical Energy Storage Devices. Renew. Sustain. Energy Rev. 137, 110464. doi:10.1016/j.rser.2020.110464
Shell, K. M., Vohra, S. Y., Rodene, D. D., and Gupta, R. B. (2021). Phytoremediation of Nickel via Water Hyacinth for Biocarbon‐Derived Supercapacitor Applications. Energy Technol. 9 (8), 2100130. doi:10.1002/ente.202100130
Shen, W., Li, Z., and Liu, Y. (2010). Surface Chemical Functional Groups Modification of Porous Carbon. Cheng 1 (1), 27–40. doi:10.2174/1874478810801010027
Simberloff, D., Martin, J.-L., Genovesi, P., Maris, V., Wardle, D. A., Aronson, J., et al. (2013). Impacts of Biological Invasions: What's what and the Way Forward. Trends Ecol. Evol. 28 (1), 58–66. doi:10.1016/j.tree.2012.07.013
Simmons, A. T., Cowie, A. L., and Waters, C. M. (2021). Pyrolysis of Invasive Woody Vegetation for Energy and Biochar Has Climate Change Mitigation Potential. Sci. Total Environ. 770, 145278. doi:10.1016/j.scitotenv.2021.145278
Sizmur, T., Fresno, T., Akgül, G., Frost, H., and Moreno-Jiménez, E. (2017). Biochar Modification to Enhance Sorption of Inorganics from Water. Bioresour. Technol. 246, 34–47. doi:10.1016/j.biortech.2017.07.082
Tan, X., Liu, Y., Zeng, G., Wang, X., Hu, X., Gu, Y., et al. (2015). Application of Biochar for the Removal of Pollutants from Aqueous Solutions. Chemosphere 125, 70–85. doi:10.1016/j.chemosphere.2014.12.058
Tang, J., Zhang, S., Zhang, X., Chen, J., He, X., and Zhang, Q. (2020). Effects of Pyrolysis Temperature on Soil-Plant-Microbe Responses to Solidago Canadensis L.-derived Biochar in Coastal Saline-Alkali Soil. Sci. Total Environ. 731, 138938. doi:10.1016/j.scitotenv.2020.138938
Terry, T. J., Madsen, M. D., Gill, R. A., Anderson, V. J., and St. Clair, S. B. (2021). Selective Herbicide Control: Using Furrows and Carbon Seed Coatings to Establish a Native Bunchgrass while Reducing Cheatgrass Cover. Restor. Ecol. 29 (5), 13351. doi:10.1111/rec.13351
Uchimiya, M., Bannon, D. I., and Wartelle, L. H. (2012). Retention of Heavy Metals by Carboxyl Functional Groups of Biochars in Small Arms Range Soil. J. Agric. Food Chem. 60 (7), 1798–1809. doi:10.1021/jf2047898
Velez, T. I., Moonilall, N. I., Reed, S., Jayachandran, K., and Scinto, L. J. (2018). Impact of Melaleuca Quinquenervia Biochar on Phaseolus vulgaris Growth, Soil Nutrients, and Microbial Gas Flux. J. Environ. Qual. 47 (6), 1487–1495. doi:10.2134/jeq2017.12.0484
Vilà, M., Espinar, J. L., Hejda, M., Hulme, P. E., Jarošík, V., Maron, J. L., et al. (2011). Ecological Impacts of Invasive Alien Plants: a Meta-Analysis of Their Effects on Species, Communities and Ecosystems. Ecol. Lett. 14 (7), 702–708. doi:10.1111/j.1461-0248.2011.01628.x
Wan, F., Liu, W., Guo, J., Qiang, S., Li, B., Wang, J., et al. (2010). Invasive Mechanism and Control Strategy of Ageratina Adenophora (Sprengel). Sci. China Life Sci. 53 (11), 1291–1298. doi:10.1007/s11427-010-4080-7
Wang, C., Tu, Q., Dong, D., Strong, P. J., Wang, H., Sun, B., et al. (2014). Spectroscopic Evidence for Biochar Amendment Promoting Humic Acid Synthesis and Intensifying Humification during Composting. J. Hazard. Mater. 280, 409–416. doi:10.1016/j.jhazmat.2014.08.030
Wang, J., Zhao, M., Zhang, J., Zhao, B., Lu, X., and Wei, H. (2021). Characterization and Utilization of Biochars Derived from Five Invasive Plant Species Bidens Pilosa L., Praxelis Clematidea, Ipomoea Cairica, Mikania Micrantha and Lantana Camara L. For Cd2+ and Cu2+ Removal. J. Environ. Manag. 280, 111746. doi:10.1016/j.jenvman.2020.111746
Weihua Li, W., Luo, J., Tian, X., Soon Chow, W., Sun, Z., Zhang, T., et al. (2015). A New Strategy for Controlling Invasive Weeds: Selecting Valuable Native Plants to Defeat Them. Sci. Rep. 5, 11004. doi:10.1038/srep11004
Weller, S., Florentine, S., Sillitoe, J., Grech, C., McLaren, D., and Chauhan, B. S. (2015). The Need for Speed: Timely Prevention of the Dispersal of Noxious Weeds in Relief Fodder Using Efficient Sampling Procedures. Crop Prot. 70, 21–27. doi:10.1016/j.cropro.2014.12.014
Wu-Jun Liu, W.-J., Jiang, H., and Yu, H.-Q. (2019). Emerging Applications of Biochar-Based Materials for Energy Storage and Conversion. Energy Environ. Sci. 12 (6), 1751–1779. doi:10.1039/c9ee00206e
Xiaoling Dong, X., Ma, L. Q., Zhu, Y., Li, Y., and Gu, B. (2013). Mechanistic Investigation of Mercury Sorption by Brazilian Pepper Biochars of Different Pyrolytic Temperatures Based on X-Ray Photoelectron Spectroscopy and Flow Calorimetry. Environ. Sci. Technol. 47 (21), 12156–12164. doi:10.1021/es4017816
Xu, Y., Liu, Y., Liu, S., Tan, X., Zeng, G., Zeng, W., et al. (2016). Enhanced Adsorption of Methylene Blue by Citric Acid Modification of Biochar Derived from Water Hyacinth (Eichornia Crassipes). Environ. Sci. Pollut. Res. 23 (23), 23606–23618. doi:10.1007/s11356-016-7572-6
Yang, Y., Wei, Z., Zhang, X., Chen, X., Yue, D., Yin, Q., et al. (2014). Biochar from Alternanthera Philoxeroides Could Remove Pb(II) Efficiently. Bioresour. Technol. 171, 227–232. doi:10.1016/j.biortech.2014.08.015
Yang, Q., Li, Z., Lu, X., Duan, Q., Huang, L., and Bi, J. (2018). A Review of Soil Heavy Metal Pollution from Industrial and Agricultural Regions in China: Pollution and Risk Assessment. Sci. Total Environ. 642, 690–700. doi:10.1016/j.scitotenv.2018.06.068
Yuan, J.-H., Xu, R.-K., and Zhang, H. (2011). The Forms of Alkalis in the Biochar Produced from Crop Residues at Different Temperatures. Bioresour. Technol. 102 (3), 3488–3497. doi:10.1016/j.biortech.2010.11.018
Zeng, H., Wu, X., Zeng, H., Zhang, H., and Shahab, A. (2019). Preparation and Characteristics of Magnetic Water Hyacinth. IOP Conf. Ser. Earth Environ. Sci. 295 (3), 032062. doi:10.1088/1755-1315/295/3/032062
Zhang, X., Wang, H., He, L., Lu, K., Sarmah, A., Li, J., et al. (2013). Using Biochar for Remediation of Soils Contaminated with Heavy Metals and Organic Pollutants. Environ. Sci. Pollut. Res. 20 (12), 8472–8483. doi:10.1007/s11356-013-1659-0
Zhang, F., Wang, X., Xionghui, J., and Ma, L. (2016). Efficient Arsenate Removal by Magnetite-Modified Water Hyacinth Biochar. Environ. Pollut. 216, 575–583. doi:10.1016/j.envpol.2016.06.013
Zhang, P., Li, B., Wu, J., and Hu, S. (2019). Invasive Plants Differentially Affect Soil Biota through Litter and Rhizosphere Pathways: a Meta‐analysis. Ecol. Lett. 22 (1), 200–210. doi:10.1111/ele.13181
Zhang, H., Xu, F., Xue, J., Chen, S., Wang, J., and Yang, Y. (2020). Enhanced Removal of Heavy Metal Ions from Aqueous Solution Using Manganese Dioxide-Loaded Biochar: Behavior and Mechanism. Sci. Rep. 10 (1), 6067. doi:10.1038/s41598-020-63000-z
Zhang, L., Cheng, H., Pan, D., Wu, Y., Ji, R., Li, W., et al. (2021). One-pot Pyrolysis of a Typical Invasive Plant into Nitrogen-Doped Biochars for Efficient Sorption of Phthalate Esters from Aqueous Solution. Chemosphere 280, 130712. doi:10.1016/j.chemosphere.2021.130712
Zhuang, H., Zhu, H., Zhang, J., Shan, S., Fang, C., Tang, H., et al. (2020). Enhanced 2,4,6-trichlorophenol Anaerobic Degradation by Fe3O4 Supported on Water Hyacinth Biochar for Triggering Direct Interspecies Electron Transfer and its Use in Coal Gasification Wastewater Treatment. Bioresour. Technol. 296, 122306. doi:10.1016/j.biortech.2019.122306
Keywords: biochar, invasive plants, resource utilization, environmental remediation, energy storage
Citation: Yang L, Deng Y, Shu Z, Chen Q, Yang H and Tan X (2022) Application of Invasive Plants as Biochar Precursors in the Field of Environment and Energy Storage. Front. Environ. Sci. 10:902915. doi: 10.3389/fenvs.2022.902915
Received: 23 March 2022; Accepted: 18 April 2022;
Published: 04 May 2022.
Edited by:
Xiaodong Xin, Dongguan University of Technology, ChinaReviewed by:
Hang Yu, Dalian Maritime University, ChinaCopyright © 2022 Yang, Deng, Shu, Chen, Yang and Tan. This is an open-access article distributed under the terms of the Creative Commons Attribution License (CC BY). The use, distribution or reproduction in other forums is permitted, provided the original author(s) and the copyright owner(s) are credited and that the original publication in this journal is cited, in accordance with accepted academic practice. No use, distribution or reproduction is permitted which does not comply with these terms.
*Correspondence: Xiaofei Tan, dGFueGZAaG51LmVkdS5jbg==mailto
Disclaimer: All claims expressed in this article are solely those of the authors and do not necessarily represent those of their affiliated organizations, or those of the publisher, the editors and the reviewers. Any product that may be evaluated in this article or claim that may be made by its manufacturer is not guaranteed or endorsed by the publisher.
Research integrity at Frontiers
Learn more about the work of our research integrity team to safeguard the quality of each article we publish.