- 1Postdoctoral Station of Agricultural Resources and Environment, Land and Environment College, Shenyang Agricultural University, Shenyang, China
- 2National Biochar Institute, Shenyang Agricultural University, Shenyang, China
- 3Key Laboratory of Biochar and Soil Improvement, Ministry of Agriculture and Rural Affairs, Shenyang, China
- 4Agronomy College, Shenyang Agricultural University, Shenyang, China
Turning maize straw into biochar is useful for improving soil fertility and mitigating climate change. However, the difference between straw and straw-derived biochar on soil aggregate associated humic substances has not been sufficiently studied in brown earth soil. The objective of this study was to investigate how different straw management practices affect soil humic substances and aggregate-associated humic substances in the field. As such, an eight-year study (2013–2020) was conducted on brown earth in Northeast China. Three treatments were applied: 1) CK, in which only chemical fertilizer was applied every year; 2) BC, in which biochar was applied at a rate of 2.625 t ha−1 (the same rate of chemical fertilizer as that in the CK was applied); 3) SR, in which straw was returned at a rate of 7.5 t ha−1 (the same rate of chemical fertilizer as that in the CK was applied). Both biochar and straw improved soil aggregate stability that reflected by the mean weight diameter (MWD) and geometric mean diameter (GMD). Compared to the CK treatment, the BC treatment enhanced humic carbon (HMC) both in bulk soil and different aggregate fractions. Biochar decreased the humic acid carbon (HAC) in bulk soil compared to the initial content, but no differences were observed between BC and CK after the eight-year field experiment. All of the aggregate-associated HAC contents were significantly enhanced in response to BC treatment. SR was an effective way to improve soil organic carbon (SOC) and humic substances in bulk soil and aggregate fractions. SR had a relatively small effect on the relative proportions of the C functional groups. Solid-state 13C cross-polarization magic-angle-spinning nuclear magnetic resonance (CPMAS-NMR) spectra of bulk soils revealed that biochar increased the proportion of aromatic C. The ratios of alkyl C/O-alkyl C, aromatic C/aliphatic C, and hydrophobic C/hydrophilic C increased in response to the BC treatment compared to the other treatments. Taken together, biochar amendment can enhance soil aggregate stability, aggregate-associated HMC and stability of SOC on the scale of an eight-year field experiment. Biochar could be an effective approach to sequestrate carbon and improve the quality of brown earth soil.
Introduction
The soil organic carbon (SOC) pool contains approximately 2.4×1012 t of carbon worldwide and is the largest terrestrial carbon pool compared to the atmospheric carbon pool and terrestrial vegetation carbon pool (Stockmann et al., 2013). Minor changes in the SOC pool can markedly affect the concentration of atmospheric CO2, aggravating the greenhouse effect (Han et al., 2016). According to statistics, 1.3×1011 t of global SOC was lost from the top 2 m due to agricultural activities during the past two centuries (Sanderman et al., 2017). Therefore, agricultural practices strongly affect carbon balance. SOC can be considered the most important component, as SOC can maintain fertility and productivity in the field (Diacono and Montemurro, 2010). Intensive land use in recent decades and current agricultural practices have led to soil degradation and a decrease in SOC contents in farmland. Soil degradation has negative effect on the quantity and quality of food production. Decreases in crop yields and agronomy production severely threaten food security for humans (Lal, 2006; Lal, 2009). Therefore, effective farming practices to maintain or enhance SOC are crucial for the sustainable development of agriculture.
Crop residues are an important source of SOC, and SOC plays a predominant role in improving soil fertility and soil functions (Ndzelu et al., 2021). During the decomposition of crop residues, nutrients and energy are released for soil microbes (Cui et al., 2018). Therefore, the return of straw to the soil is a common practice to improve the SOC content and maintain soil fertility (Choudhury et al., 2014; Cui et al., 2018). Straw amendments were shown to sharply enhance SOC contents in the first 3 years, although the rate of increase in the next few years was slower (Wang et al., 2021). However, straw amendment release large amounts of greenhouse gases (Yang et al., 2017). This is not conducive to the realization of “carbon neutralization” from a sustainable perspective. In recent decades, the use of biochar as a soil amendment has been a popular research topic. Biochar is a solid product produced through pyrolysis in an oxygen-limited environment and use used for agriculture and forestry (Chen et al., 2013; IBI, 2013). Biochar particles have an abundance of pores, a large specific surface area and a high cation exchange capacity (Liu et al., 2015; Chen et al., 2019). Biochar is considered an ideal material for carbon sequestration due to its stable properties. Biochar as a soil amendment can enhance crop yield (Mahmoud et al., 2019; Ali et al., 2020), improve soil total porosity and the water-holding capacity (Gao et al., 2019b), and decrease the bio-availabilities of heavy metals and organic pollutions (He et al., 2018; Xia et al., 2019). Biochar contains high amounts of carbon and has considerable potential for increasing the SOC content. Biochar applied to soil could enhance SOC directly through stable carbon; biochar can also promote soil biogeochemical processes and reduce the mineralization of native soil organic carbon, which is referred to as a negative priming effect (Ding et al., 2018). Owing to its inherent characteristics and negative priming effect, biochar can enhance soil carbon sequestration (Weng et al., 2017; Blanco-Canqui et al., 2020; Qianqian Zhang et al., 2021).
Soil aggregates can protect SOC from decomposition by microorganisms via physical barriers (Six et al., 2002). Humic substances are a series of highly acidic, relatively high-molecular-weight, yellow to black colored substances that are generated during the decomposition and transformation of plant and microbial remains (Dou et al., 2020). Humic substances are the largest components of SOC and represent the most stable and microbially recalcitrant components of SOC (Bronick and Lal, 2005; Wang et al., 2016; Dou et al., 2020). Therefore, humic substances play a vital role in soil carbon sequestration and long-term storage. Humic substances can be separated into three fractions according to their acid or alkali solubility, fulvic acid, humic acid, and humin (Waksman, 1925). Human substances are useful in the aggregation process, and soil aggregates can also protect humic substances from long-term persistence (Bongiovanni and Lobartini, 2006). A new soil organic matter (SOM) model named the “soil continuum model” was proposed by Lehmann and Kleber (2015), who considered that the evidence could not support the formation and existence of “humic substances” in soils. However, Dou et al. (2020) argued that humic substances are distinctive and complex, and the traditional humic substances model is still relevant. Baveye and Wander (2019) considered that the key reason for the slow rate of progress in humic substances might be attributed to the extreme compartmentalization of research and education in soil science.
Biochar produced at different pyrolysis temperatures had different impacts on soil humic fractions in a one-year incubation study; biochar produced at 300°C and 400°C increased the HAC content, but biochar produced at 500°C and 600°C decreased the HAC content (Zhao et al., 2018). Based on the 13C isotope method, cotton straw-derived biochar more preferentially formed HMC in a 180-days laboratory incubation experiment (Song et al., 2020). In a tropical sandy loam, biochar increased the SOC content and all humic fractions at 15 t ha−1 and 30 t ha−1 application rates (Amoakwah et al., 2020). In a 3-year field experiment, biochar increased the SOC, HMC, and HAC contents but decreased the FAC content compared to no biochar application (Meng et al., 2016). Distinct effects of biochar on soil humic substances have been reported in previous studies, but how maize straw biochar affects soil humic substances fraction needs systematic research in brown earth areas.
Northeast China is situated in the golden maize belt of the world and is a main grain production area. Maize straw yield is a renewable resource with great potential. According to statistics presented by the Ministry of Agriculture and Rural Affairs, the yield of straw reached approximately 8.6 × 108 t in 2020, and the amount of collectable resources reached 7.2×108 t (Huo et al., 2022). The conventional method for crop residue management is in situ burning in farmland, which cause severe air pollution and a waste of resources (Fang et al., 2015). Suitable straw management practices should be carried out for a large number of straw resources. Turning these resources into biochar is a suitable practice. Previous studies on the effect of biochar on soil humic substances have mainly focused on laboratory conditions, and less attention has been given to long-term effects in the field. In the current study, we aimed to investigate the long-term (8 years) impacts of straw and straw-derived biochar under the same biomass on soil aggregate distribution and stability, soil humic substances and aggregate-associated humic substances. Compared to straw, straw-derived biochar had a distinct composition and concentration of organic carbon. We hypothesize that straw-derived biochar as a soil amendment would have a greater positive effect on the aggregate formation and humic substances of carbon compared to straw return because biochar contains more humic-like substances.
Materials and Methods
Site Description
A long-term field experiment was conducted at the Shenyang Agricultural University biochar trial station in Liaoning Province, China (41°49′N, 123°33′E), and the study was started in May 2013. The site was situated in the northern temperature zone with a semi-humid continental climate. The annual mean temperature is 6.2°C–9.7°C, and the average annual precipitation is between 600 and 800 mm. The soil at the site is a Haplic Luvisol according to the classification system of the world reference base for soil resources (WRB). Before the field experiments, the planting mode in this field was continuous cropping of spring maize; chemical fertilizers were applied annually and no other amendments were applied. The straw was removed after harvest in every autumn.
The basic properties of the soil were measured at the very beginning of the experiment, the results of which are listed in Table 1.
Straw and Biochar Preparation and Experimental Design
Maize straw was collected from the field in autumn after harvest every year and then air dried. Then, the straw was crushed into small segments (5–7 cm) by a grinder. Biochar was subsequently produced by a vertical kiln produced by Jinhefu Agriculture Development Company (Liaoning province, China). The pyrolysis temperature was 450°C, and the pyrolysis duration was 90 min. The basic properties of the straw and biochar are listed in Table 2.
Three treatments were applied in the current research: a control (CK), in which chemical fertilizer only was applied annually; BC, in which biochar was applied at a rate of 2.625 t ha−1 annually in conjunction with the CK treatment; and SR, in which straw was returned at a rate of 7.5 t ha−1 annually in conjunction with the CK treatment. The straw application rate was based on the average yield of maize straw in this region, and the biochar application rate was based on the transformation efficiency (35%) of straw conversion into biochar. All the treatments involved the same chemical fertilizer application rate (120 kg ha−1 N, 60 kg ha−1 P2O5, and 60 kg ha−1 K2O). All the chemical fertilizers were applied once as basal fertilizers before sowing, and the amendments were also applied before sowing annually. The planting pattern was continuous maize cropping. The maize was planted early in May and harvested at the end of September.
Soil Aggregate Separation
A wet sieving method was used to determine the soil aggregate class and stability (Elliott, 1986). The soil aggregates were separated into four size fractions: large macroaggregates (>2000 μm), small macroaggregates (250–2000 μm), microaggregates (53–250 μm), and the aggregates composing the silt + clay fraction (<53 μm). Undisturbed soil samples were sieved through an 8 mm mesh screen during the air drying process. Then, 50 g of the prepared samples was placed on top of a series of sieves. The samples were then immersed in distilled water for 5 min, and the sieves were shifted upward and downward approximately 3 cm and at approximately 30 strokes per minute. All the aggregate fractions were oven dried at 60°C until a constant weight was reached and stored for further analysis.
Humic Substance Fractions
The humic substance fractions were extracted by the sodium hydroxide-hydroxide sodium pyrophosphate mixed solution method (Wang et al., 2016). Both bulk soil samples and aggregate samples were sieved through a 0.25 mm mesh screen. Then, 5.00 g of the bulk samples was weighed in a 100 ml centrifuge tube, and 30 ml of deionized water was added. After shaking in a constant-temperature environment (70°C, 145 r min−1) and centrifuging at 3,500 r min−1, the supernatant was collected and filtered for analysis; this fraction is referred to as dissolved organic carbon (DOC). Then, the residue and soil aggregate samples were extracted by a solution consisting of a mixture of 0.1 M NaOH and 0.1 M Na4P2O7. Then, the supernatants were dissolved in 0.5 M H2SO4, after which the acid-insoluble humic acid carbon (HAC) from acid-soluble fulvic acid carbon could be separated (Falsone et al., 2016). The solid residue remaining in the centrifuge tubes was humin [humic carbon (HMC)]. The DOC, FAC, and HAC were determined by a multi-TOC analyzer (Analytic Jena 3100, Germany), and the SOC and HMC were detected by an Elementar Vario max analyzer (Elementar Macro Cube, Germany).
Solid Nuclear Magnetic Resonance (13C NMR) Analysis
The composition of the SOC was measured by solid-state 13C nuclear magnetic resonance spectroscopy with cross-polarization and magic-angle spinning nuclear magnetic resonance (CPMAS-NMR) (Li et al., 2013). To improve the accuracy of detection, we removed any paramagnetic compounds and minerals by hydrofluoric acid (Chen et al., 2018). We obtained NMR spectra by a 400-MHz Bruker AVANCE Ⅱ spectrometer. Hexamethylbenzene was used to generate an external standard spectrum, and four chemical shift regions were used as specific organic C groups in each NMR spectrum: alkyl C (0–46 ppm), O-alkyl C (46–114 ppm), aromatic C (114–164 ppm), and carbonyl C (164–220 ppm) regions. We obtained the relative contents from the spectra via integration.
Other Properties
Soil pH was measured using a glass electrode (HANNA HI2221, Italy); the solid-water ratio was 1:2.5 (w:v). The total nitrogen contents of soil and amendments were determined by an Elementar Vario max Analyzer (Elementer Macro Cube, Germany). Soil bulk density was determined by the soil core and cutting ring method. The soil phosphorus content was determined by the H2SO4 and HClO4 digestion method. The ash content and volatile matter of biochar and straw were determined by the combustion method (Chen et al., 2018). The specific surface area and average pore size of the amendments were determined by the Brunauer–Emmett–Teller (BET) method (Yang et al., 2017).
Calculations and Statistical Analysis
The mean weight diameter (MWD), geometric mean diameter (GMD), and macroaggregate contents (R > 250 μm) are usually used to indicate aggregate stability. These indexes were calculated using the following equations (Mazurak 1950; van Bavel 1950):
where Wi is the weight percentage of every soil aggregate fraction (%), x is the mean diameter of the soil aggregate fraction (μm), and mi is the weight of the soil aggregate fraction (g).
The stability of SOC was shown by the ratios of alkyl C to O-alkyl C, of aromatic C to aliphatic C, and of hydrophilic C to hydrophilic C. The formulas were calculated as follows:
All the data were calculated and arranged in Office Excel 2016. One-way analysis of variance (ANOVA) was used to analyze the differences among treatments according to the lease significant difference (LSD) test. All the graphs were constructed via Origin 2022 software (Origin Lab, Inc., Northampton, United States).
Results
Soil Aggregate Distribution and Stability
As shown in Figure 1, the soil aggregate size distribution changed after 8 years of applications of straw and biochar as soil amendments. The small macroaggregate (250–2000 μm) size fraction was dominant in the soil, with a proportion of 58.23%–67.43%. The silt + clay size fraction accounted for the lowest proportion, ranging from 4.34%–8.89%. Moreover, the biochar and straw amendments significantly decreased the silt + clay size fraction by 32.25% and 51.16%, respectively. Straw amendments significantly increased the proportion of macroaggregates. The ratio of macroaggregates to microaggregates significantly increased in response to biochar and straw (p < 0.05), and the same trend was also observed for the soil aggregate stability, MWD and GMD; the trend of MWD, GMD and the ratio of macroaggregates to microaggregates was SR > BC > CK (p < 0.05). Compared with biochar, straw amendments had a greater effect on soil aggregate stability.
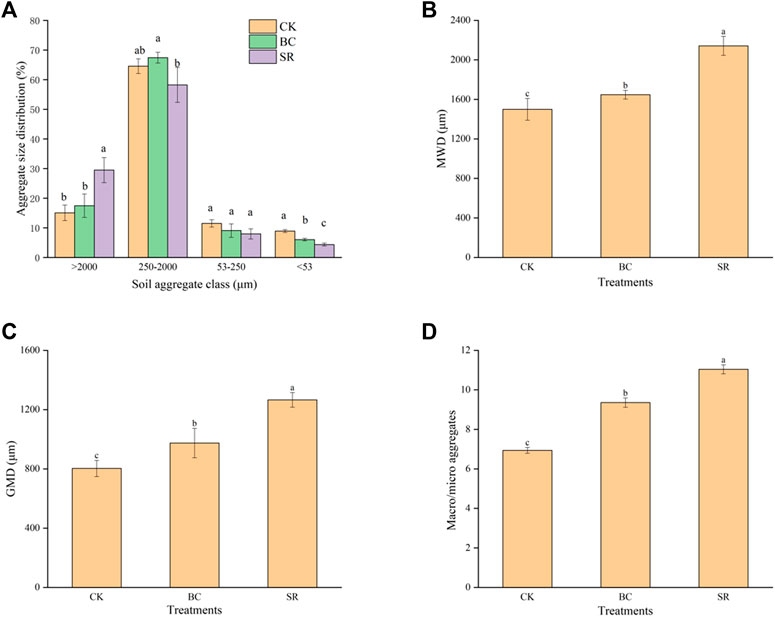
FIGURE 1. Effects of different straw management practices on the soil aggregate distribution (A), mean weight diameter (MWD) (B), and geometric mean diameter (GMD) (C), ratio of macroaggregates to microaggregates (D) in brown earth soil after 8 years. The error bars represent the standard deviations of the mean values (n = 3).
Soil Organic Carbon and Humic Substances in Bulk Soil
As shown in Figure 2, compared with the initial SOC content, the SOC content in the CK decreased (p < 0.05) after 8 years, but the SOC content increased by 53.73% and 51.92% in the BC and SR treatments, respectively, compared to the CK treatment. However, compared to the initial SOC content, the SOC content decreased by 14.92% in the CK treatment, and the SOC content in the BC and SR treatments increased by 30.80% and 29.26%, respectively.
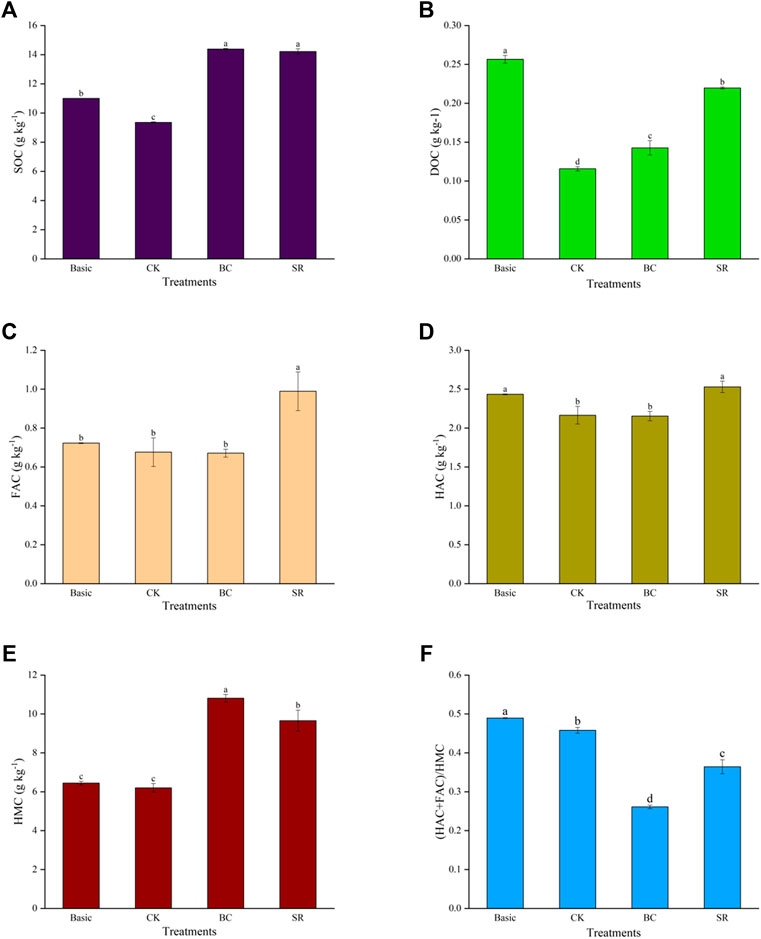
FIGURE 2. Effects of different straw management practices on soil organic carbon (SOC) (A), dissolved organic carbon (DOC) (B), fulvic acid carbon (C), humic acid carbon (HAC) (D), humic carbon (HMC) (E) and the ratio of HAC and FAC to HMC (F) after eight-year field experiments. The error bars represent the standard deviations of the mean values (n = 3). The different lowercase letters indicate significant differences between the different treatments. The initial properties are those measured at the beginning of the experiments.
The DOC contents markedly decreased after 8 years of field experiments. The decrease in DOC in the CK treatment compared with the initial DOC value was the greatest, followed by that in the BC treatment and then that in the SR treatment, and the values in all the treatments reached significant levels (p < 0.05).
The FAC contents were not different between the CK and BC treatments, and the FAC contents of the CK and BC were also not different from the initial FAC contents. Only the SR treatment significantly enhanced the FAC content after 8 years of straw amendment (p < 0.05). Both the CK and BC decreased the HAC contents compared to the initial HAC contents (p < 0.05). Compared with the BC and CK treatments, the SR treatment resulted in higher HAC contents. However, a difference between the FAC content in the SR and the initial FAC contents was observed. The HMC contents were in the following order: BC > SR > CK. Compared with the initial HMC content, the HMC in the CK did not change after 8 years of farming practices. The (HAC + FAC)/HMC ratio was ranked as follows: initial > CK > SR > BC (Figure 2). All the differences in the ratio of (HAC + FAC)/HMC were significant (p < 0.05).
Soil Organic Carbon and Humic Substances in Soil Aggregates
Organic materials increased all aggregate-associated organic carbon compared to that in the CK treatment. In the large macroaggregate (>2000 μm) and microaggregate (53–250 μm) fractions, the aggregate-associated organic carbon contents were in the order of SR > BC > CK, but in the small macroaggregate and silt + clay fractions, the aggregate-associated organic carbon contents were in the order of SR = BC > CK. Biochar and straw increased the SOC content of large macroaggregates by 39.82% and 62.45%; the SOC content of small macroaggregates by 15.46% and 14.98%; the SOC content of microaggregates by 30.73% and 53.98%; and the SOC content of the silt + clay fraction by 68.28% and 70.79%.
The FAC in the different aggregate fractions exhibited the same trend: compared with the CK and BC treatments, the SR treatment presented a higher FAC contents. The HAC contents in all aggregate fractions exhibited the same trend—SR > BC > CK. Compared with the other treatments, the BC treatment resulted in the highest HMC content in the aggregate fractions (excluding the microaggregate fraction), and compared with the CK treatment, the SR treatment also enhanced the HMC contents after 8 years of farming practices.
Solid-State 13C NMR Spectra
The 13C NMR spectra of all treatments are shown in Figure 3. The solid-state 13C NMR spectra showed the carbon functional structures (carbon species) of different treatments. Combined with Table 3, the relative proportions of functional groups showed almost no different between SR and CK; alkyl C ranged from 21.32 to 22.29%, O-alkyl C ranged from 33.12 to 34.46%, aromatic C ranged from 29.90 to 28.31%, and carbonyl C ranged from 15.65 to 14.39%. In contrast, compared with the CK and SR treatments, the BC treatment caused changes in the relative proportion of carbon functional groups. As shown in Table 3, the relative proportion of aromatic C was 52.54% in the BC treatment. The ratios of alkyl C/O-alkyl C, aromatic C/aliphatic C, and hydrophobic C/hydrophilic C were higher in the BC treatment than in the other treatments.

TABLE 3. Relative contents (%) of organic functional groups revealed by solid 13C NMR spectroscopy in different treatments.
Discussion
Effects of Maize Straw and Straw-Derived Biochar on Soil Aggregates and Aggregate Stability
In the current study, both biochar and straw as soil amendments increased the macroaggregate contents and decreased the silt + clay contents (Figure 1). These results suggested that these soil amendments could promote the bonding of smaller soil fractions to form macroaggregates. Compared with the CK treatment, the SR treatment increased both small macroaggregates (250–2000 μm) and large macroaggregates (>2000 μm). In addition, compared with the CK treatment, the BC treatment increased only the small macroaggregate (250–2000 μm) fractions. The macroaggregate contents were also higher in the SR than in the BC, but both were significantly higher than they were in the CK. Biochar and straw application could provide different aggregate binding agents. The effects of biochar on soil aggregate stability have remained controversial until now: biochar as a soil aggregate has been shown to significantly enhance soil aggregate stability (Luo et al., 2020; Han et al., 2021; Zhang et al., 2020; Shuai Zhang et al., 2021), reduce it or have no effect (Rahman et al., 2017; Heikkinen et al., 2019; Zhou et al., 2019). These different results might be caused by many factors, such as differences in soil type, biochar feedstock and climate. However, in the current study, BC had the same effect on soil aggregate stability as did SR. The increase in macroaggregate contents led to the improvement in MWD and GMD in response to biochar and straw applications. The underlying mechanisms of the effects of biochar on soil aggregates might be explained as follows: biochar enhanced the SOC and HMC, which could act as binding agents for aggregates; biochar also contributed to the activity of soil microorganisms, which would also be beneficial for the aggregation process (Sun et al., 2021). Although the level of improvement in aggregate stability was greater in response to straw amendment than to biochar amendment, biochar remains an ideal amendment for soil aggregation.
Effects of Straw and Straw-Derived Biochar on Soil Organic Carbon and Humic Substances in Bulk Soil
The most effective way to increase SOC is returning organic material to the field. In the current study, after eight successive years of biochar and straw amendments, the SOC significantly increased compared to that in response to chemical fertilizer only (Figure 2). The chemical fertilizer only decreased the SOC content compared to the initial content. However, no differences in SOC contents were observed between the BC and SR treatments after 8 years of field farming practices. The SOC content is driven by carbon inputs and outputs (Amundson and Biardeau, 2018). In a previous study, biochar application resulted in higher SOC contents than did straw amendment under equal carbon input (Xiaowei Zhang et al., 2019). In the current study, the amount of carbon inputs was inconsistent (SR > BC), the amount of carbon input due to SR was 3.22 t year−1 in theory, and the amount of carbon input due to BC was 1.73 t year−1 in theory.
However, biochar had the same effect, i.e., increased SOC, after an eight-year field experiment (Figure 2). These results might be attributed to biochar having more stable carbon contents than straw does, and straw amendment could lead to more greenhouse gas emissions (Yang et al., 2017; Huang et al., 2018). Biochar increases the SOC content by direct and indirect effects; the direct effect is mainly due to the stable properties of SOC contained in biochar, and this proportion of SOC could mix with the SOC pool. Biochar also had a negative priming effect, which is the potential reduction in mineralization of the native SOC content (Blanco-Canqui et al., 2020; Han et al., 2020). Therefore, biochar had a higher carbon sequestration efficiency than straw did. These results could be explained by the biochar structure and properties.
Soil HMC accounts for the largest proportion of SOC (Dou et al., 2020). Traditional organic materials have the capacity to improve the formation of soil humic substances (Yang et al., 2019; Jiuming Zhang et al., 2019). Biochar as a kind of organic amendment has received little attention in previous studies. Mazie straw biochar was shown to enhance soil HMC and aggregate-associated organic carbon in Mollisols (Jinjing Zhang et al., 2019). In the current study, compared with the CK treatment, the SR enhanced DOC, FAC, HAC, and HMC after an eight-year field experiment. In addition, compared with the CK treatment, the BC treatment also increased DOC and HMC contents, but no differences were observed in FAC and HAC contents (Figure 2). Although biochar contains humic-like compounds (Graber et al., 2015; Zhang et al., 2015), biochar still had no effect on HAC or FAC in brown earth. The potential underlying mechanisms still need further research. Moreover, compared with the other two treatments in the current study, the BC treatment resulting in the highest HMC content. These results could be explained by biochar containing more alkyl C and aromatic C than the other treatment components did (Figure 3 and Table 3). The straw decomposition process could release labile carbon such as polysaccharides, peptides and other small molecules; these active substances could be beneficial for the formation of DOC, HAC, and FAC. Therefore, SR enhanced almost all the humic substances after 8 years application. Biochar also partly consists of labile carbon and could be useful for the humification process. However, in this study, only DOC and HMC changed in response to biochar application. Biochar applications increased HAC and FAC in a Mollisol in a four-year field experiment (Jinjing Zhang et al., 2019). The results of our study were different from the results of Xiaowei Zhang et al. (2019). These results might be due to the different soil types and biochar application rates. The ratio of (HAC + FAC)/HMC reflects the contribution and accumulation of amendments on the humic fractions (Wang et al., 2016). The results indicated that BC was more advantageous for the formation of HMC but that SR was beneficial for the formation of HAC and FAC. However, the results were different from those of the study by Jinjing Zhang et al. (2019). Both biochar and straw were favorable for the formation of HAC in that study. Our results were similar to the results of another study by Zhang et al. (2022), in which biochar application was found to be most beneficial for the enhancement of HMC.
SR treatment had little effect on the chemical composition of SOC compared to that of the CK after the eight-year field experiment (Figure 3 and Table 3). However, biochar clearly altered the chemical composition of the SOC (Table 3). Biochar as a soil amendment increased the amounts of alkyl C and aromatic C. The stability of SOC was also enhanced by biochar application, as reflected by the ratios of alkyl C/O-alkyl C, aromatic C/aliphatic C, and hydrophobic C/hydrophilic C. In conclusion, biochar could enhance SOC stability for long-term storage.
Effects of Biochar and Maize Straw on Soil Aggregate Organic Carbon and Aggregate-Associated Humic Substances
Both biochar and straw were shown to increase the aggregate-associated organic carbon contents in previous studies (Zhang et al., 2017; Dai et al., 2019; Jiuming Zhang et al., 2019). Similarly, all aggregate fractions associated with the organic carbon contents were increased by biochar and straw in the current study. Straw decomposed by soil microorganisms could benefit the soil aggregation process, as more binding agents for soil aggregation would be produced by soil microorganisms. Biochar also has the capacity to promote the formation of macroaggregates. Because of its large specific area and cation exchangeable capacity, biochar has the potential to form organo-mineral complexes, which would facilitate the soil aggregation process (Liu et al., 2017). Macroaggregates have been shown to protect SOC, enabling increased storage duration (Six et al., 2002). Macroaggregates usually contain more SOC compared to microaggregates (Mehmood et al., 2020; Shuai Zhang et al., 2021); our results are consistent with the findings of these studies. Because the small macroaggregate fraction dominated in the study, the small macroaggregate-associated organic carbon dominated in the study. However, microaggregates were reported to contain the highest SOC contents in black soil in a previous study (Xiaowei Zhang et al., 2019), and the silt + clay fraction associated organic carbon contents were also higher in a sandy soil (Dai et al., 2019). Therefore, the different results among different studies might be due to different soil types.
All aggregate fraction-associated humic substances in the SR treatment were higher than those in the CK treatment (Figure 4). These results indicated that straw residue carbon incorporated into humic fractions was distributed in different aggregate fractions. Although biochar and soil humic substances are completely different, biochar applied to soil could be depolymerized by special microorganisms for the formation of humic substances (Placido et al., 2016). Biochar also had the same effect on HMC and HAC. These results showed that biochar had the potential to become incorporated into the HMC and HAC fractions, which means that biochar comprised more stable components. Although the total HAC contents in the bulk soil were not different between the CK and BC treatments, the HAC within water-stable aggregates increased in response to biochar application. This phenomenon might be explained by the absence of the sieving process provided by water-stable aggregates. The free particles might be washed away during the sieving process, but more particles were enclosed by aggregates. HAC might also be adsorbed by biochar particles. Moreover, compared with the other treatments, biochar application was more conducive to the formation of HMC. No difference in HMC between the BC and SR treatments was observed in the microaggregate fraction. Mukherjee et al. (2014) suggested that biochar resulted in the formation of FAC prior to that of HAC in a 1.5-year field experiment. Our results indicated a different trend. The difference might be due to the different test years and conditions. Some HAC or FAC can be utilized by soil microorganisms during a long-term field experiment (Zhao et al., 2018). These results indicated that the microaggregate fraction was the most stable fraction and that the organic carbon associated with microaggregates was also more stable than that associated with the other fractions.
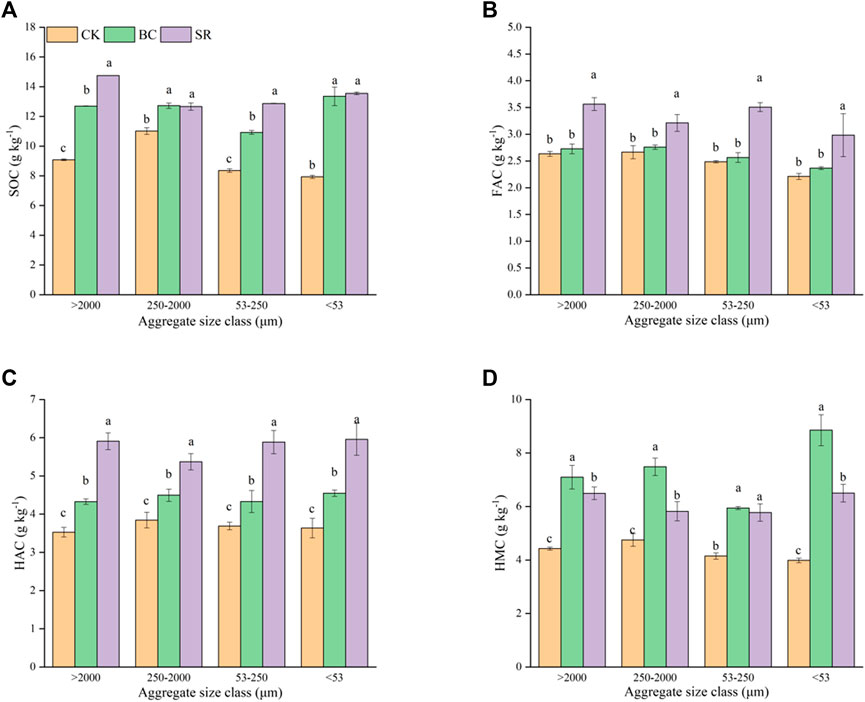
FIGURE 4. Effects of different straw management practices on aggregate-associated organic carbon (A), fulvic acid carbon (B), humic acid carbon (HAC) (C) and humic carbon (HMC) (D) after eight-year field experiments. The error bars represent the standard deviations of the mean values (n = 3). The different lowercase letters indicate significant differences between the different treatments.
Conclusion
This eight-year study demonstrated that, compared with chemical fertilizer alone, both maize straw and straw-derived biochar enhanced macroaggregate contents and soil aggregate stability. Both biochar and straw promoted the silt + clay fraction to form macroaggregates. The contents of SOC and different humic fractions contents in bulk soil were also generally higher after the application of maize straw. However, biochar enhanced only the DOC and HMC in the bulk soil. The contents of SOC and humic substances fractions in the aggregate fractions also increased in response to SR trentment, and biochar also enhanced the aggregate-associated SOC, HMC, and HAC contents. Moreover, biochar obviously altered the chemical composition of the SOC. The ratios of alkyl C/O-alkyl C, aromatic C/aliphatic C, and hydrophobic C/hydrophilic C increased in response to BC treatment compared to SR and CK. Our findings suggested that biochar amendment could enhance the long-term storage of SOC both via macroaggregate protection and via the stability of SOC (Gao et al., 2019b).
Data Availability Statement
The raw data supporting the conclusions of this article will be made available by the authors, without undue reservation.
Author Contributions
QS: conceptualization; data curation; formal analysis; investigation; methodology; resources; software; writing-original draft. XY: data curation; resources; writing-review and editing. JM: supervision, writing—review and editing, project administration, funding acquisition. YL: supervision; writing-review and editing. WC: supervision; writing-review and editing. XH: supervision; writing-review and editing. YH: supervision; writing-review and editing.
Funding
This study was financially supported by the Earmarked Fund for Modern Agroindustry Technology Research System (No. CARS-01-51), the Innovative Talents Promotion Plan of the Ministry of Science and Technology of the People’s Republic of China (No. 2017RA2211), and the Project of Standardization Subsidy, Shenyang, China (2021-57).
Conflict of Interest
The authors declare that the research was conducted in the absence of any commercial or financial relationships that could be construed as a potential conflict of interest.
Publisher’s Note
All claims expressed in this article are solely those of the authors and do not necessarily represent those of their affiliated organizations, or those of the publisher, the editors and the reviewers. Any product that may be evaluated in this article, or claim that may be made by its manufacturer, is not guaranteed or endorsed by the publisher.
References
Ali, I., He, L., Ullah, S., Quan, Z., Wei, S., Iqbal, A., et al. (2020). Biochar Addition Coupled with Nitrogen Fertilization Impacts on Soil Quality, Crop Productivity, and Nitrogen Uptake under Double‐cropping System. Food Energy Secur 9 (3), 208. doi:10.1002/fes3.208
Amoakwah, E., Arthur, E., Frimpong, K. A., Parikh, S. J., and Islam, R. (2020). Soil Organic Carbon Storage and Quality Are Impacted by Corn Cob Biochar Application on a Tropical Sandy Loam. J. Soils Sediments 20 (4), 1960–1969. doi:10.1007/s11368-019-02547-5
Amundson, R., and Biardeau, L. (2018). Soil Carbon Sequestration Is an Elusive Climate Mitigation Tool. Proc. Natl. Acad. Sci. U.S.A. 115, 11652–11656. doi:10.1073/pnas.1815901115
Baveye, P. C., and Wander, M. (2019). The (Bio)Chemistry of Soil Humus and Humic Substances: Why Is the “New View” Still Considered Novel after More Than 80 years? Front. Environ. Sci. 7, 27. doi:10.3389/fenvs.2019.00027
Blanco‐Canqui, H., Laird, D. A., Heaton, E. A., Rathke, S., and Acharya, B. S. (2020). Soil Carbon Increased by Twice the Amount of Biochar Carbon Applied after 6 years: Field Evidence of Negative Priming. GCB Bioenergy 12, 240–251. doi:10.1111/gcbb.12665
Bongiovanni, M. D., and Lobartini, J. C. (2006). Particulate Organic Matter, Carbohydrate, Humic Acid Contents in Soil Macro- and Microaggregates as Affected by Cultivation. Geoderma 136, 660–665. doi:10.1016/j.geoderma.2006.05.002
Bronick, C. J., and Lal, R. (2005). Soil Structure and Management: a Review. Geoderma 124, 3–22. doi:10.1016/j.geoderma.2004.03.005
Chen, W., Zhang, W., and Meng, J. (2013). Advances and Prospects in Research of Biochar Utilization in Agriculture. Sci. Agric. Sin. 46, 3324–3333. doi:10.3864/j.issn.0578-1752.2013.16.003
Chen, J., Chen, D., Xu, Q., Fuhrmann, J. J., Li, L., Pan, G., et al. (2018). Organic Carbon Quality, Composition of Main Microbial Groups, Enzyme Activities, and Temperature Sensitivity of Soil Respiration of an Acid Paddy Soil Treated with Biochar. Biol. Fertil. Soils 55, 185–197. doi:10.1007/s00374-018-1333-2
Chen, W., Meng, J., Han, X., Lan, Y., and Zhang, W. (2019). Past, Present, and Future of Biochar. Biochar 1, 75–87. doi:10.1007/s42773-019-00008-3
Cui, S., Liang, S., Zhang, X., Li, Y., Liang, W., Sun, L., et al. (2018). Long-term Fertilization Management Affects the C Utilization from Crop Residues by the Soil Micro-food Web. Plant Soil 429, 335–348. doi:10.1007/s11104-018-3688-4
Dai, H., Chen, Y., Liu, K., Li, Z., Qian, X., Zang, H., et al. (2019). Water-stable Aggregates and Carbon Accumulation in Barren Sandy Soil Depend on Organic Amendment Method: A Three-Year Field Study. J. Clean. Prod. 212, 393–400. doi:10.1016/j.jclepro.2018.12.013
Diacono, M., and Montemurro, F. (2010). Long-term Effects of Organic Amendments on Soil Fertility. A Review. Agron. Sustain. Dev. 30 (2), 401–422. doi:10.1051/agro/2009040
Ding, F., Van Zwieten, L., Zhang, W., Weng, Z., Shi, S., Wang, J., et al. (2018). A Meta-Analysis and Critical Evaluation of Influencing Factors on Soil Carbon Priming Following Biochar Amendment. J. Soils Sediments 18 (4), 1507–1517. doi:10.1007/s11368-017-1899-6
Dou, S., Shan, J., Song, X., Cao, R., Wu, M., Li, C., et al. (2020). Are Humic Substances Soil Microbial Residues or Unique Synthesized Compounds? A Perspective on Their Distinctiveness. Pedosphere 30, 159–167. doi:10.1016/S1002-0160(20)60001-7
Elliott, E. T. (1986). Aggregate Structure and Carbon, Nitrogen, and Phosphorus in Native and Cultivated Soils. Soil Sci. Soc. Am. J. 50, 627–633. doi:10.2136/sssaj1986.03615995005000030017x
Falsone, G., Celi, L., Stanchi, S., and Bonifacio, E. (2016). Relative Importance of Mineralogy and Organic Matter Characteristics on Macroaggregate and Colloid Dynamics in MG‐Silicate Dominated Soils. Land Degrad. Dev. 27 (7), 1700–1708. doi:10.1002/ldr.2516
Fang, F., Li, X., Shi, Z., Wang, F., Chang, Z., Zhang, S., et al. (2015). Analysis on Distribution and Use Structure of Crop Straw Resources in Huang-Huai-Hai Plain of China. Trans. Chin. Soc. Agric. Eng. 31 (2), 228–234. doi:10.3969/j.issn.1002-6819.2015.02.032
Gao, J., Dou, S., and Wang, Z. (2019a). Structural Analysis of Humic Acid in Soil at Different Corn Straw Returning Modes through Fluorescence Spectroscopy and Infrared Spectroscopy. Int. J. Anal. Chem. 2019, 1–9. doi:10.1155/2019/1086324
Gao, J., Zhao, Y., Zhang, W., Sui, Y., Jin, D., Xin, W., et al. (2019b). Biochar Prepared at Different Pyrolysis Temperatures Affects Urea-Nitrogen Immobilization and N2O Emissions in Paddy Fields. Peer J. 7, e7027. doi:10.7717/peerj.7027
Graber, E. R., Tsechansky, L., Mayzlish-Gati, E., Shema, R., and Koltai, H. (2015). A Humic Substances Product Extracted from Biochar Reduces Arabidopsis Root Hair Density and Length under P-Sufficient and P-Starvation Conditions. Plant Soil 395, 21–30. doi:10.1007/s11104-015-2524-3
Gupta Choudhury, S., Srivastava, S., Singh, R., Chaudhari, S. K., Sharma, D. K., Singh, S. K., et al. (2014). Tillage and Residue Management Effects on Soil Aggregation, Organic Carbon Dynamics and Yield Attribute in Rice-Wheat Cropping System under Reclaimed Sodic Soil. Soil Tillage Res. 136, 76–83. doi:10.1016/j.still.2013.10.001
Han, L., Sun, K., Jin, J., and Xing, B. (2016). Some Concepts of Soil Organic Carbon Characteristics and Mineral Interaction from a Review of Literature. Soil Biol. Biochem. 94, 107–121. doi:10.1016/j.soilbio.2015.11.023
Han, L., Sun, K., Yang, Y., Xia, X., Li, F., Yang, Z., et al. (2020). Biochar's Stability and Effect on the Content, Composition and Turnover of Soil Organic Carbon. Geoderma 364, 114184. doi:10.1016/j.geoderma.2020.114184
Han, L., Zhang, B., Chen, L., Feng, Y., Yang, Y., and Sun, K. (2021). Impact of Biochar Amendment on Soil Aggregation Varied with Incubation Duration and Biochar Pyrolysis Temperature. Biochar 3, 339–347. doi:10.1007/s42773-021-00097-z
He, L., Fan, S., Müller, K., Wang, H., Che, L., Xu, S., et al. (2018). Comparative Analysis Biochar and Compost-Induced Degradation of Di-(2-ethylhexyl) Phthalate in Soils. Sci. Total Environ. 625, 987–993. doi:10.1016/j.scitotenv.2018.01.002
Heikkinen, J., Keskinen, R., Soinne, H., Hyväluoma, J., Nikama, J., Wikberg, H., et al. (2019). Possibilities to Improve Soil Aggregate Stability Using Biochars Derived from Various Biomasses through Slow Pyrolysis, Hydrothermal Carbonization, or Torrefaction. Geoderma 344, 40–49. doi:10.1016/j.geoderma.2019.02.028
Huang, R., Tian, D., Liu, J., Lv, S., He, X., and Gao, M. (2018). Responses of Soil Carbon Pool and Soil Aggregates Associated Organic Carbon to Straw and Straw-Derived Biochar Addition in a Dryland Cropping Mesocosm System. Agric. Ecosyst. Environ. 265, 576–586. doi:10.1016/j.agee.2018.07.013
Huo, L., Yao, Z., Zhao, L., Luo, J., and Zhang, P. (2022). Contribution and Potential of Comprehensive Utilization of Straw in GHG Emission Reduction and Carbon Sequestration. J. Agric. Mach. 53 (1), 349–360. doi:10.6401/j.issn.1000-1298.2022.01.038
IBI (2013). Standardized Product Definition and Product Testing Guidelines for Biochar that Is Used in Soil (Aka IBI Biochar Standards. IBI-STD-01.1.
Jinjing Zhang, J., Wei, Y., Liu, J., Yuan, J., Liang, Y., Ren, J., et al. (2019). Effects of Maize Straw and its Biochar Application on Organic and Humic Carbon in Water-Stable Aggregates of a Mollisol in Northeast China: A Five-Year Field Experiment. Soil Tillage Res. 190, 1–9. doi:10.1016/j.still.2019.02.014
Jiuming Zhang, J., Chi, F., Wei, D., Zhou, B., Cai, S., Li, Y., et al. (2019). Impacts of Long-Term Fertilization on the Molecular Structure of Humic Acid and Organic Carbon Content in Soil Aggregates in Black Soil. Sci. Rep. 9 (1), 11908. doi:10.1038/s41598-019-48406-8
Lal, R. (2006). Managing Soils for Feeding a Global Population of 10 Billion. J. Sci. Food Agric. 86, 2273–2284. doi:10.1002/jsfa.2626
Lal, R. (2009). Soil Degradation as a Reason for Inadequate Human Nutrition. Food Sec. 1, 45–57. doi:10.1007/s12571-009-0009-z
Lehmann, J., and Kleber, M. (2015). The Contentious Nature of Soil Organic Matter. Nature 528, 60–68. doi:10.1038/nature16069
Li, Y., Zhang, J., Chang, S. X., Jiang, P., Zhou, G., Fu, S., et al. (2013). Long-term Intensive Management Effects on Soil Organic Carbon Pools and Chemical Composition in Moso Bamboo (Phyllostachys Pubescens) Forests in Subtropical China. For. Ecol. Manag. 303, 121–130. doi:10.1016/j.foreco.2013.04.021
Liu, W.-J., Jiang, H., and Yu, H.-Q. (2015). Development of Biochar-Based Functional Materials: Toward a Sustainable Platform Carbon Material. Chem. Rev. 115, 12251–12285. doi:10.1021/acs.chemrev.5b00195
Liu, X., Sun, J., Duan, S., Wang, Y., Hayat, T., Alsaedi, A., et al. (2017). A Valuable Biochar from Poplar Catkins with High Adsorption Capacity for Both Organic Pollutants and Inorganic Heavy Metal Ions. Sci. Rep. 7 (1), 10033. doi:10.1038/s41598-017-09446-0
Luo, C., Yang, J., Chen, W., and Han, F. (2020). Effect of Biochar on Soil Properties on the Loess Plateau: Results from Field Experiments. Geoderma 369, 114323. doi:10.1016/j.geoderma.2020.114323
Mahmoud, E., El-Beshbeshy, T., El-Kader, N. A., El Shal, R., and Khalafallah, N. (2019). Impacts of Biochar Application on Soil Fertility, Plant Nutrients Uptake and Maize (Zea mays L.) Yield in Saline Sodic Soil. Arab. J. Geosci. 12 (23), 1–9. doi:10.1007/s12517-019-4937-4
Mazurak, A. P. (1950). Effect of Gaseous Phase on Water-Stable Synthetic Aggregates. Soil Sci. 69, 135–148. doi:10.1097/00010694-195002000-00005
Mehmood, I., Qiao, L., Chen, H., Tang, Q., Woolf, D., and Fan, M. (2020). Biochar Addition Leads to More Soil Organic Carbon Sequestration under a Maize-Rice Cropping System Than Continuous Flooded Rice. Agric. Ecosyst. Environ. 298, 106965. doi:10.1016/j.agee.2020.106965
Meng, F., Dou, S., Yin, X., Zhang, G., and Zhong, S. (2016). Effects of Maize Stalk Biochar on Humus Composition and Humic Acid Structure in Black Soil. J. Agro-Environment Sci. 35 (1), 122–128. doi:10.11654/jaes.2016.01.017
Mukherjee, A., Lal, R., and Zimmerman, A. R. (2014). Impacts of 1.5-Year Field Aging on Biochar, Humic Acid, and Water Treatment Residual Amended Soil. Soil Sci. 179 (7), 333–339. doi:10.1097/SS.0000000000000076
Ndzelu, B. S., Dou, S., and Zhang, X. (2021). Corn Straw Return Can Increase Labile Soil Organic Carbon Fractions and Improve Water-Stable Aggregates in Haplic Cambisol. J. Arid. Land 12 (6), 1018–1030. doi:10.1007/s40333-020-0024-7
Placido, J., Capareda, S., and Karthikeyan, R. (2016). Production of Humic Substances from Cotton Stalks Biochar by Fungal Treatment with Ceriporiopsis Subvermispora. Sustain. Energy Technol. Assessments 13, 31–37. doi:10.1016/j.seta.2015.11.004
Qianqian Zhang, Q., Duan, P., Gunina, A., Zhang, X., Yan, X., Kuzyakov, Y., et al. (2021). Mitigation of Carbon Dioxide by Accelerated Sequestration from Long-Term Biochar Amended Paddy Soil. Soil Tillage Res. 209, 104955. doi:10.1016/j.still.2021.104955
Rahman, M. T., Zhu, Q. H., Zhang, Z. B., Zhou, H., and Peng, X. (2017). The Roles of Organic Amendments and Microbial Community in the Improvement of Soil Structure of a Vertisol. Appl. Soil Ecol. 111, 84–93. doi:10.1016/j.apsoil.2016.11.018
Sanderman, J., Hengl, T., and Fiske, G. J. (2017). Soil Carbon Debt of 12,000 Years of Human Land Use. Proc. Natl. Acad. Sci. U.S.A. 114, 9575–9580. doi:10.1073/pnas.1706103114
Shuai Zhang, S., Cui, J., Wu, H., Zheng, Q., Song, D., Wang, X., et al. (2021). Organic Carbon, Total Nitrogen, and Microbial Community Distributions within Aggregates of Calcareous Soil Treated with Biochar. Agric. Ecosyst. Environ. 314, 107408. doi:10.1016/j.agee.2021.107408
Six, J., Conant, R. T., Paul, E. A., and Paustian, K. (2002). Stabilization Mehcanisms of Soil Organic Matter: Implications for C-Saturation of Soils. Plant Soil 241, 155–176. doi:10.1023/A:1016125726789
Song, X., Yang, J., Hussain, Q., Liu, X., Zhang, J., and Cui, D. (2020). Stable Isotopes Reveal the Formation Diversity of Humic Substances Derived from Different Cotton Straw-Based Materials. Sci. Total Environ. 740, 140202. doi:10.1016/j.scitotenv.2020.140202
Stockmann, U., Adams, M. A., Crawford, J. W., Field, D. J., Henakaarchchi, N., Jenkins, M., et al. (2013). The Knowns, Known Unknowns and Unknowns of Sequestration of Soil Organic Carbon. Agric. Ecosyst. Environ. 164, 80–99. doi:10.1016/j.agee.2012.10.001
Sun, Q., Meng, J., Lan, Y., Shi, G., Yang, X., Cao, D., et al. (2021). Long-term Effects of Biochar Amendment on Soil Aggregate Stability and Biological Binding Agents in Brown Earth. Catena 205, 105460. doi:10.1016/j.catena.2021.105460
van Bavel, C. H. M. (1950). Mean Weight-Diameter of Soil Aggregates as a Statistical Index of Aggregation. Soil Sci. Soc. Am. J. 14, 20–23. doi:10.2136/sssaj1950.036159950014000C0005x
Waksman, S. A. (1925). What Is Humus? Proc. Natl. Acad. Sci. U.S.A. 11, 463–468. doi:10.1073/pnas.11.8.463
Wang, Y., Gao, S., Li, C., Zhang, J., and Wang, L. (2016). Effects of Temperature on Soil Organic Carbon Fractions Contents, Aggregate Stability and Structural Characteristics of Humic Substances in a Mollisol. J. Soils Sediments 16, 1849–1857. doi:10.1007/s11368-016-1379-4
Weng, Z., Van Zwieten, L., Singh, B. P., Tavakkoli, E., Joseph, S., Macdonald, L. M., et al. (2017). Biochar Built Soil Carbon over a Decade by Stabilizing Rhizodeposits. Nat. Clim. Change 7, 371–376. doi:10.1038/nclimate3276
Wang, X., Jing, Z.-H., He, C., Liu, Q.-Y., Qi, J.-Y., Zhao, X., et al. (2021). Temporal Variation of SOC Storage and Crop Yield and its Relationship - A Fourteen Year Field Trial about Tillage Practices in a Double Paddy Cropping System, China. Sci. Total Environ. 759, 143494. doi:10.1016/j.scitotenv.2020.143494
Xia, S., Song, Z., Jeyakumar, P., Shaheen, S. M., Rinklebe, J., Ok, Y. S., et al. (2019). A Critical Review on Bioremediation Technologies for Cr(VI)-contaminated Soils and Wastewater. Crit. Rev. Environ. Sci. Technol. 49 (12), 1027–1078. doi:10.1080/10643389.2018.1564526
Xiaowei Zhang, X., Dou, S., Ndzelu, B. S., Guan, X. W., Zhang, B. Y., and Bai, Y. (2019). Effects of Different Corn Straw Amendments on Humus Composition and Structural Characteristics of Humic Acid in Black Soil. Commun. Soil Sci. Plant Analysis 51, 107–117. doi:10.1080/00103624.2019.1695827
Yang, X., Meng, J., Lan, Y., Chen, W., Yang, T., Yuan, J., et al. (2017). Effects of Maize Stover and its Biochar on Soil CO 2 Emissions and Labile Organic Carbon Fractions in Northeast China. Agric. Ecosyst. Environ. 240, 24–31. doi:10.1016/j.agee.2017.02.001
Yang, Y., Wang, L., Zhang, Y., Li, L., Shi, X., Liu, X., et al. (2019). Transformation of Corn Stalk Residue to Humus-like Substances during Solid-State Fermentation. Sustainability 11, 6771. doi:10.3390/su11236771
Zhang, Q., Du, Z., Lou, Y., and He, X. (2015). A One-Year Short-Term Biochar Application Improved Carbon Accumulation in Large Macroaggregate Fractions. Catena 127, 26–31. doi:10.1016/j.catena.2014.12.009
Zhang, M., Cheng, G., Feng, H., Sun, B., Zhao, Y., Chen, H., et al. (2017). Effects of Straw and Biochar Amendments on Aggregate Stability, Soil Organic Carbon, and Enzyme Activities in the Loess Plateau, China. Environ. Sci. Pollut. Res. 24, 10108–10120. doi:10.1007/s11356-017-8505-8
Zhang, Q., Song, Y., Wu, Z., Yan, X., Gunina, A., Kuzyakov, Y., et al. (2020). Effects of Six-Year Biochar Amendment on Soil Aggregation, Crop Growth, and Nitrogen and Phosphorus Use Efficiencies in a Rice-Wheat Rotation. J. Clean. Prod. 242, 118435. doi:10.1016/j.jclepro.2019.118435
Zhang, G., Dou, S., Meng, F., Yin, X., and Zhou, X. (2022). Transformation of Biochar into Extracted Humic Substances under Short-Term Laboratory Incubation Conditions: Evidence from Stable Carbon Isotopes. Soil Tillage Res. 215, 105189. doi:10.1016/j.still.2021.105189
Zhao, S., Ta, N., Li, Z., Yang, Y., Zhang, X., Liu, D., et al. (2018). Varying Pyrolysis Temperature Impacts Application Effects of Biochar on Soil Labile Organic Carbon and Humic Fractions. Appl. Soil Ecol. 123, 484–493. doi:10.1016/j.apsoil.2017.09.007
Keywords: soil aggregate, soil humic substances, biochar, 13C solid NMR, maize straw, soil organic carbon sequestration
Citation: Sun Q, Yang X, Meng J, Lan Y, Han X, Chen W and Huang Y (2022) Long-Term Effects of Straw and Straw-Derived Biochar on Humic Substances and Aggregate-Associated Humic Substances in Brown Earth Soil. Front. Environ. Sci. 10:899935. doi: 10.3389/fenvs.2022.899935
Received: 19 March 2022; Accepted: 02 May 2022;
Published: 26 May 2022.
Edited by:
Yuxue Liu, Zhejiang Academy of Agricultural Sciences, ChinaReviewed by:
Jining Zhang, Shanghai Academy of Agricultural Sciences, ChinaHao Zheng, Ocean University of China, China
Copyright © 2022 Sun, Yang, Meng, Lan, Han, Chen and Huang. This is an open-access article distributed under the terms of the Creative Commons Attribution License (CC BY). The use, distribution or reproduction in other forums is permitted, provided the original author(s) and the copyright owner(s) are credited and that the original publication in this journal is cited, in accordance with accepted academic practice. No use, distribution or reproduction is permitted which does not comply with these terms.
*Correspondence: Jun Meng, mengjun1217@syau.edu.cn; Xiaori Han, hanxr@syau.edu.cn
†These authors have contributed equally to this work and share first authorship