- 1Key Laboratory of Mariculture (Ocean University of China), Ministry of Education, Qingdao, China
- 2The Marine Ecological Civilization Comprehensive Experimental Area of Changdao, Yantai, China
Prolongation of light duration is one of the effective methods to improve the current seagrass propagation technique. We subjected plants of eelgrass Zostera marina to different prolonged light durations [0 (control), 2, 4, 6, 8 h d−1] for 6 weeks under controlled laboratory conditions. We measured plant response in terms of survivorship, growth, productivity, leaf pigment and carbohydrate concentrations. Survival analysis combined with growth assessment suggested that the optimum range of prolonged light durations for the establishment of Z. marina plants is 3.9–4.6 h d−1. The propagation coefficient of Z. marina plants exposed to 4 h d−1 was 1.4 times higher than that of plants under the control. Pearson and Mantel correlation analysis indicated that the promotion of prolonged light duration to the survival and growth of Z. marina plants mainly depended on the increase of chlorophyll content and the accumulation and synthesis of non-structural carbohydrate. The total chlorophyll content of leaves and soluble sugar content of aboveground tissues of Z. marina plants exposed to 4 h d−1 were 1.6 times and 2.9 times higher than those of plants under the control, respectively. The results will provide data that could prove helpful in the development of efficient artificial propagation technology of Z. marina plants.
1 Introduction
Seagrasses, a kind of angiosperms, evolved from land plants to fully adapt to the marine environment (Ooi et al., 2011; Olsen et al., 2016). Seagrass beds are usually composed of one or several seagrass species, which has strong ecological functions including water purification, climate regulation and maintenance of marine living resources (Aguilar-Perera and Appeldoorn, 2007; Misturini and Colling, 2021). However, seagrass beds are severely degraded worldwide due to natural and anthropogenic disturbances. Since 1993, 18% of the global seagrass beds have completely disappeared, and the degradation rate is still accelerating, which is one of the serious problems facing to the coastal ecosystems (Castellanos-Iglesias et al., 2021; Stockbridge et al., 2021; Yue et al., 2021; Zoffoli et al., 2021).
Plant transplantation is a relatively mature method for seagrass bed restoration, which includes sod method, plug method, and rhizome method (Park and Lee, 2007; Lee and Park, 2008). The transplants of donor plants could produce plenty of lateral branches through clonal reproduction, which would increase obviously the plant density and biomass of the seagrass beds (Li et al., 2021a; Park et al., 2021). However, the required donor plants are mostly collected from natural seagrass beds, which may not only have a great negative impact on the donor beds, but also limit the large-scale development of plant transplantation. Therefore, establishing an efficient artificial propagation technology of plants is extremely important for the large-scale seagrass bed restoration.
The absorption of nutrients for plants is promoted by light, including increasing the amount of carbon capture, synthesizing organic matter, and providing an essential nutrient for seagrass growth, development, and propagation. The effect of light, especially light quality and intensity, on the growth and survival of seagrass has been studied widely (Davey et al., 2018; Martin et al., 2018). For example, the seedling survival of Halophila ovalis was 100% under the red light, which was higher than that of seedlings under the full-spectrum and yellow light (Wilhelm et al., 2010). Lee et al. (2005) found that the productivity of Zostera marina varied seasonally, and the photosynthetic rate was maximum with saturation light intensity ranging from 100 to 200 μmol photons m−1 s−1.
It has been well known that prolonged light duration significantly affects the vegetive growth and survival of plants. The growth and yield enhancements are associated with increases in leaf photosynthetic rate, chlorophyll content and non-structure carbohydrate during prolonged light duration (Demers and Gosselin, 2002). However, studies on the effect of prolonged light durations on growth, biomass and photosynthetic rate of plants mainly focused on the terrestrial plants, e.g., Lactuca sativa and Solanum lycopersicum (Fukuda et al., 2000; Bertelli and Unsworth, 2018; Martin et al., 2018). Data on the most suitable prolonged light duration for survival, growth and promotion of seagrasses are still unknown.
Photosynthesis is the most important metabolic activity of submerged plants. The photochemical activity of photosynthetic pigments is enhanced though the plant leaves received light, and a series of redox reactions is carried out to achieve the transformation of light energy and electric energy (Ge and Zhao, 2019). During this process, plants absorb external carbon sources to achieve carbon sequestration, so as to accumulate organic substances such as starch and soluble sugar as necessary energy and substances for plant growth and survival (Schmid and Clauss, 1975). Some studies indicated that appropriately prolonged light duration could improve photosynthetic efficiency and C storage efficiency by promoting the accumulation of photosynthetic pigments of plants (Matsuda et al., 2014; Yamamoto et al., 2016). For example, the accumulation of photosynthetic pigment content promoted the accumulation of sugar and the distribution of dry matter, and the growth rate of plants increased by 2–3 times (Dong et al., 2015).
The eelgrass Z. marina has been the focus of most of the seagrass restoration activities along temperate coasts of Europe, North America, and the northwest Pacific (Zhang et al., 2015). Many studies have proved that the growth and yield of terrestrial plants could be enhanced by suitable prolongation of light duration, thus we hypothesize that the appropriate prolongation of light duration may also promote the growth and propagation of Z. marina plants. To test this hypothesis, this study aims to test experimentally the response of plants of Z. marina to prolonged light duration and to obtain the optimum range of prolonged light duration for growth and promotion of Z. marina plants. The responses of plants of Z. marina to different prolonged light durations were studied through a cultivation experiment focusing on plant survival, growth, and photosynthetic pigment and carbohydrate concentrations under controlled laboratory conditions.
2 Materials and Methods
2.1 Plant Collection
The cuttings of plants with attached roots and rhizomes were gently collected individually by hand from bottom sediments of a 100 × 100 m donor bed in Swan Lake on the eastern coast of Shandong Peninsula, China (37.3382°–37.3588°N, 122.5551°–122.5793°E) in June 2019. Intact shoots were selected and then rinsed thoroughly in seawater to remove sediments, shells and other debris. The shoots were immediately placed in plastic boxes and transported to aquaculture farm within 4 h.
For shoot acclimatization, shoots were held in the farm for 7 days in a 12.5 m × 1.5 m × 1.0 m (length × width × height) tank with flowing seawater at temperature (20 ± 1.2°C), natural light condition and seawater salinity (30 PSU) until prepared for the experiment.
2.2 Experimental Design and Procedure
In this experiment, four different prolonged light durations 2, 4, 6, and 8 h d−1 were produced by using light-emitting diode (LED) plant lamps. Natural light duration (average 14 h d−1) was used as reference prolonged light duration (0 h d−1).
A total of 360 cuttings were randomly selected from those that had been acclimated to laboratory conditions over 7 days, and then divided into 20 groups of 18 cuttings each. Each treatment was replicated four times (four groups). All cuttings used in this study were carefully selected to obtain the similar morphological characteristics with rhizomes of 5–7 cm, height of 25 ± 2 cm, and numbers of leaves (three to four). All selected cuttings were marked for leaf and rhizome growth estimations using the leaf punching method and rhizome binding method (Short and Duarte, 2001).
A 20.5 cm × 14.3 cm × 9 cm plastic box, filled with sediments, was used as planting container for each group. Filling sediments were collected at the beach just outside the experimental site. The mixture of 25% fine sand (sediment particle size between 63 and 250 μm) and 75% silt and clay (sediment particle size <63 μm) in weight percentages of sediments were obtained using a standard series of sieves according to Zhang et al. (2015). Each box was filled with the sediment at an 8 cm thick layer and then was kept submerged in sea water for at least 48 h until the shoots were planted.
The 18 cuttings in each group were evenly anchored by carefully inserting the rhizome meristem into the sediment at a depth of 4 cm. Then the four boxes for each treatment were placed at random in a 90 × 60 × 75 cm polyethylene tank with an effective water volume of 300 L. The experiment was conducted in a circulating-water system, which consisted of five polyethylene tanks (experimental tanks), a transport tank with a 200 W aquarium pump for the recycling of the water at an exchange rate of 900 L h−1 and a programmed temperature controller with a heater and a refrigerator for the holding of the water temperature (Figure 1). The position of the four boxes in the same tank was changed randomly every day to reduce the error in light intensity. The experiment lasted for 42 days.
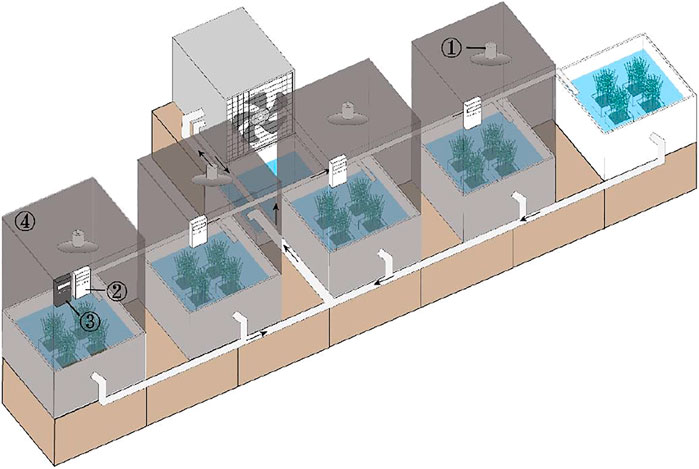
FIGURE 1. Schematic diagram of experimental apparatus with a circulating-water system, a temperature controller and light controllers. Note: 1: LED plant lamp; 2: time control switch; 3: light control switch; 4: enclosure barrier.
2.3 Experimental Apparatus and Conditions
LED plant lamps (red: blue = 7 : 3) were used as the supplementary light source to keep the light intensity over 2000 lx. Light control switch and time control switch were used to produced different prolonged light duration. Enclosure barriers were used in the treatments of prolonged light durations to separate the treatments each other and to avoid interaction effects between different treatments (Figure 1).
During the course of the experiment, water temperature and salinity of the water in the tank were maintained at 20.0 ± 1.0°C, 30.0 ± 1.2 PSU, respectively. Temperature and salinity were checked daily using a mercury thermometer and a portable refractometer (Atago). The water in the tank was renewed daily at a rate of 30%.
2.4 Sample Collection and Calculations
2.4.1 Survival Rate
The total number of plants in each group was counted, and the percentage of plants that survived each treatment at the end of the experiment was calculated. The survival rate (SR, %) of Z. marina was calculated as the number of plants that were still alive after the experiment over the number of plants planted at the beginning of the experiment.
2.4.2 Propagation and Growth
2.4.2.1 Propagation
At the end of the experiment, all the plants in each treatment were collected and washed with tap water. The number of ramets (PT), stock plants producing ramets (SP) and stock plants (ST) in each replicate group were counted.
Propagation coefficient (PC, shoots shoot−1 d−1) of Z. marina plants was calculated as PC =
2.4.2.2 Growth
Newly formed leaves and rhizome segments (those without the mark) were separated from the rest, gently cleaned with gauze and washed with tap water. Morphometrics of new plants were analysed by measuring leaf length, leaf width, and internode length (cm). The total leaf area (TLA, cm2 shoot−1) was determined as the sum of all the leaf areas of each plant, which was calculated by multiplying length and width of each leaf. Leaf elongation rate (LER, mm day−1) and internode elongation rate (IER, cm shoot−1 mm day−1) were also calculated to estimate the plant dynamic properties according to Xu et al. (2019). After morphological measurements, subsamples were dried at 60 °C for 96 h to obtain leaf and rhizome weight. Aboveground productivity (ADP, mg DW shoot−1 day−1) was calculated as leaf weight divided by experimental days, and belowground productivity (BDP, mg DW shoot−1 day−1) was determined as rhizome weight divided by experimental days.
2.4.3 Physiological Parameters
2.4.3.1 Pigment Content Analysis
Exactly six cuttings were randomly selected from each treatment, gently cleaned with gauze, and washed with tap water. All the second or third fresh leaf blades of each plant were selected and a leaf area of 2 cm2 for each blade was cut to measure the pigment content of Z. marina using the extraction method. Subsequently, the pigment was extracted from the leaf segment using 5 ml of N, N-Dimethyl formamide for 72 h at 4°C in darkness. Absorbance of the supernatant was measured spectrophotometrically at 470, 649, 665, and 652 nm and then the pigment contents were calculated following the protocol by Xiao and Wang (2005).
2.4.3.2 Soluble Sugar and Starch Analysis
Exactly three cuttings were randomly selected from the surviving plants in each treatment. The leaf and rhizome materials were separated from the plants, and then were pre-treated by high temperature desiccation under 105°C. Subsequently, these materials were dried at 60°C for 96 h, then weighed and grounded to a power. Fifty milligrams of the powder from each group were analyzed for soluble sugar and starch following the method of anthrone reagent. Briefly, sugars were removed from the ground tissue, with two sequential extractions. The soluble sugar content was determined spectrophotometrically at 625 nm following the protocol by Xiao and Wang (2005). Following extraction of the soluble sugar, the starch content of the remaining material was extracted using perchloric acid according to Xiao and Wang (2005).
2.5 Statistical Analysis
Statistical analyses of all data were performed using the SPSS Windows Program (Release 25.0, SPSS Inc.). All data were reported as means ± SD, and were analysed by one-way analysis of variance (ANOVA) to test for the effect of different light durations on the responses of Z. marina plants. All data were tested for normality and homogeneity of variance to meet the assumptions of parametric statistical analysis. Post hoc mean comparisons (Student-Newman-Keuls; S-N-K) were performed to identify the significance of differences among treatments when the one-way ANOVAs were significant at α = 0.05.
Principal component analysis (PCA) was conducted using the FactoMine package in R software (version 4.1.1) to reveal the relationship between the variables of Z. marina plants. The result of PCA showed the explanation and contribution of ADP, BDP, IER and RF to each variable.
The quadratic function curves were fitted according to the relationship between the prolonged light durations and SR, ADP, BDP, IER, and RF, and the optimal suitable growth and propagation periods were obtained according to the fitting relationship.
Pearson and Mantel correlation analysis were conducted using the ggcor package in R software (version 4.1.1) to identify the degree of potential correlations between the variables, including survival, growth, propagation and physiological of Z. marina plants.
3 Results
3.1 Survival Rate of Z. marina Plants
The survival rate of Z. marina plants exposed to different prolonged light durations increased firstly from 0 h d−1–4 h d−1 up to 97.2% and then decreased to 93.1% at 8 h d−1 (Figure 2A). The result of one-way ANOVA indicated that the prolonged light duration had a significant effect on the survival of Z. marina plants (p < 0.05; Table 1). The survival attained under the 4 h d−1 treatment was 1.1 and 1.0 times significantly higher than those attained under control and 8 h d−1 treatments. The relationship between survival rate (S) and prolonged light duration (PLD) could be expressed as quadratic function through curve estimation. The estimated maximum survival rate (Smax) was achieved at 96.9%, i.e., PLDopt= 4.1 h d−1 and Smax= 96.9%, and the range of prolonged light duration within which survival rate is >90% of the maximum were estimated between 3.7 and 4.6 h d−1.
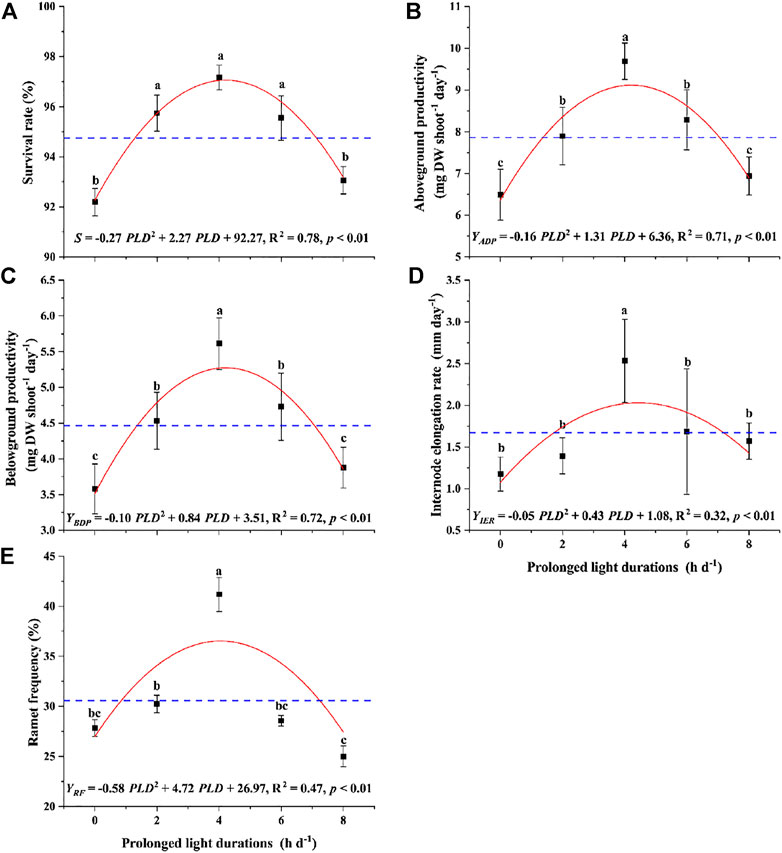
FIGURE 2. Change in the (A) survival rate (S) (B) aboveground productivity (ADP) (C) internode elongation rate (IER) (D) belowground productivity (BDP), and (E) ramet frequency (RF)of Z. marina plants exposed to different prolonged light durations (PLD). Note: The blue dotted line represents the average of survival rate. Data are expressed as the mean ± SD. Different letters above error bars indicate significant differences (p < 0.05).
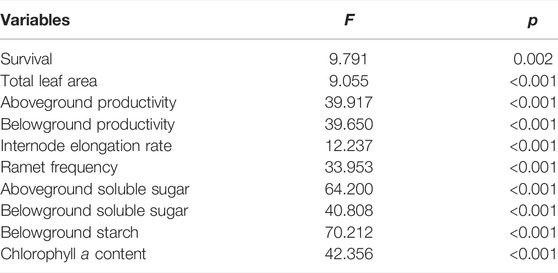
TABLE 1. Results of ANOVA showing the effects of different prolonged light durations on survival, growth and physiology of the Z. marina plants. F-values and p-values are included in the table.
3.2 The Relationship Between Growth and Propagation of Z. marina Plants
The PCA using the seven indices of the growth and propagation of Z. marina plants accounted for 70% of the variance in the data with the first two dimensions, and allowed for clear separation of the treatment groups (Figure 3A; Table 2). PC1 accounted for 59.8% of the total variance, and was defined primarily by ADP, BDP, RF and IER, which had the highest positive loadings, respectively. PC2 explained 15.0% of the variance in Z. marina growth and propagation, and was primarily defined by LA (highest positive loadings). The treatment of 4 h d−1 produced more ramet (RF) and faster growth (ADP, BDP and IER) than other treatments.
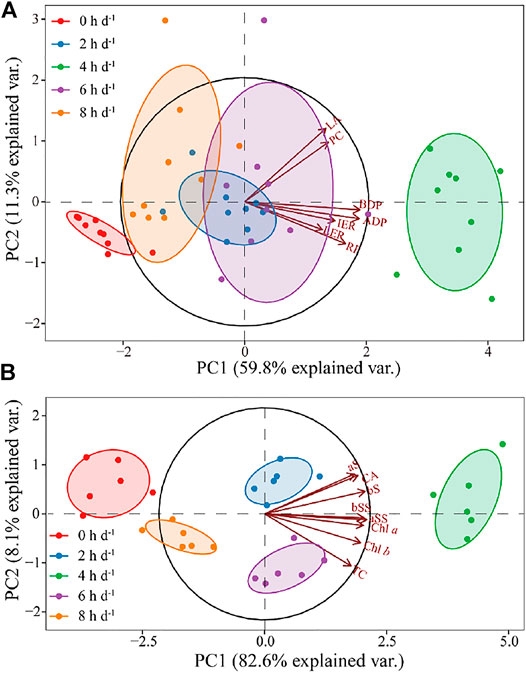
FIGURE 3. Principal component analysis between growth and propagation variables (A) and between chlorophyll, carotenoid, soluble sugar and starch variables (B) exposed to different prolonged light durations. Note: TLA: total leaf area; LER: leaf elongation rate; IER: internode elongation rate; ADP: aboveground productivity; BDP: belowground productivity; PC: propagation coefficient; RF: ramet frequency; aS: aboveground starch; aSS: aboveground soluble sugar; bS: belowground starch; bSS: belowground soluble sugar; Chl a: chlorophyll a content; Chl b: chlorophyll b content; TC: total chlorophyll content; CA: carotenoids content.
The ADP, BDP, IER and RF of Z. marina plants exposed to different prolonged light durations increased firstly from 0 h d−1–4 h d−1 and then decreased at 8 h d−1 (Figures 2B–E). The result of one-way ANOVA indicated that the prolonged light duration had a significant effect on the ADP, BDP, IER and RF of Z. marina plants (p < 0.05; Table 1). The greatest ADP, BDP, IER and RF attained were 9.7 mg DW shoot−1 day−1, 5.6 mg DW shoot−1 day−1, 2.5 mm/day and 41.2% under the 4 h d−1 treatment, which was 1.5, 1.6, 2.1, and 1.5 times significantly higher than those attained under control. The curve fitting showed that there was a significant quadratic relation between the growth (ADP, BDP and IER) and propagation (RF) and the light prolonged durations (p < 0.01).
3.3 Range of Optimum Prolonged Light Durations
The range of prolonged light duration within which growth and propagation are >90% of the maximum were estimated, and the lower limit of IER and the upper limit of RF or SR were selected as the optimum durations range, that is, 3.9–4.6 h d−1 (Figure 4).
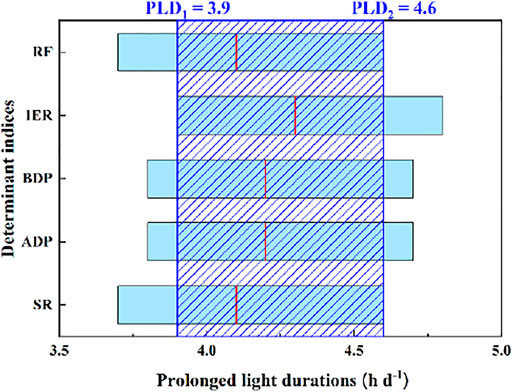
FIGURE 4. Map of optimum prolonged light durations (PLD). Note: The red lines represent the average prolonged light durations for the growth and propagation of Z. marina. PLD1 to PLD2 was the range of optimum prolonged light durations.
3.4 Relationship Between Chlorophyll, Carotenoid, Soluble Sugar and Starch on the Leaves of Z. marina Plants
The PCA using the eight indices of the physiological of Z. marina plants accounted for 90.7% of the variance in the data with the first two dimensions, and allowed for clear separation of the treatment groups (Figure 3B; Table 3). PC1 accounted for 82.6% of the total variance, and was defined primarily by aSS, bSS, bS and Chl a, which had the highest positive loadings, respectively. PC2 explained 8.1% of the variance in physiology of Z. marina. The content of chlorophyll and non-structural carbohydrate of Z. marina at 4 h d−1 were higher than those at other treatments.
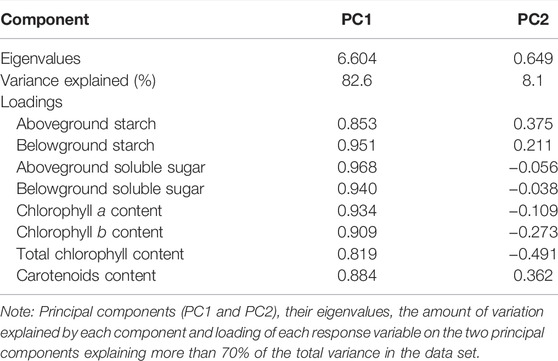
TABLE 3. Principal component analysis between chlorophyll, carotenoid, soluble sugar and starch variables.
One-way ANOVAs indicated that prolonged light durations significantly affected the aSS, bSS, bS and Chl a of Z. marina plants (p < 0.05; Figure 5; Table 1). The aSS, bSS, bS and Chl a varied with durations of prolonged light, which were all the lowest at control (0 h d−1). The highest values were observed at 4 h d−1, which were 1.6, 1.5, 1.8, and 1.3 times higher than the average contents (p < 0.05).
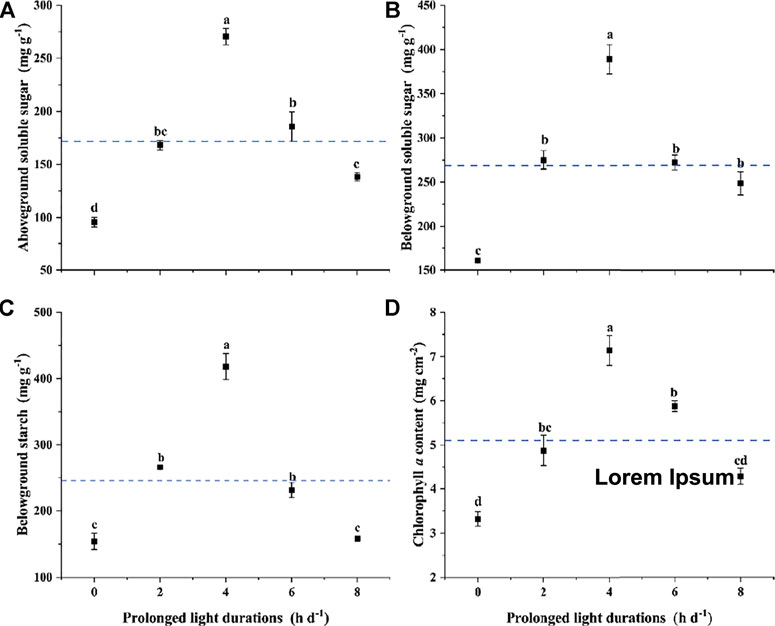
FIGURE 5. Changes in (A) aboveground soluble sugar (aSS) (B) belowground soluble sugar (bSS) (C) belowground starch (bS) and (D) chlorophyll a content (Chl a) of Z. marina plants exposed to different prolonged light durations. Note: The blue dotted lines represent the average values. Data are expressed as the mean ± SD. Different letters above error bars indicate significant differences (p < 0.05).
3.5 Correlation Analysis of Survival, Growth, Propagation, and Physiological of Z. marina Plants
Pearson correlation analysis showed a significantly positive correlation between the physiological indicators of Z. marina plants exposed to different prolonged light durations (Figure 6; Table 4). Mantel analysis showed that the survival rate and content of chlorophyll and starch of leaves were significantly affected by soluble sugar and starch of belowground tissues of Z. marina plants (p < 0.05).
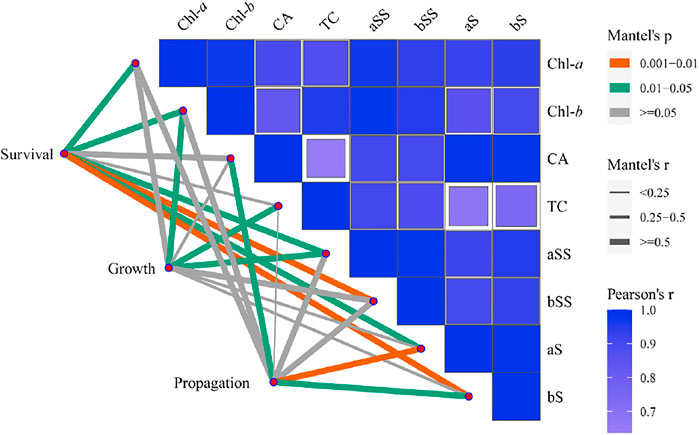
FIGURE 6. Correlation analysis between survival, growth, propagation and physiological index of Z. marina plants and chlorophyll, carotenoid and soluble sugar contents under different prolonged light durations. Note: aS: aboveground starch; aSS: aboveground soluble sugar; bS: belowground starch; bSS: belowground soluble sugar; Chl-a: chlorophyll a; Chl-b: chlorophyll b; TC: total chlorophyll; CA: carotenoids; Survival was the data of survival rate of Z. marina plants; Growth was the data of total leaf area, leaf elongation rate, internode elongation rate, aboveground productivity and belowground productivity of Z. marina plants; Propagation was the data of propagation coefficient and ramet frequency of Z. marina plants.
4 Discussion
4.1 Suitability Analysis of Prolonged Light Durations for Growth and Survival of Z. marina
Our observation results show that the promotion effect of seagrass growth and propagation is more significant under the condition of 4 h d−1. Especially, the greatest internode elongation rate attained was 2.5 mm day−1 at 4 h d−1, which was 2.1 times significantly higher than that attained under control. Survival analysis combined with growth assessment indicated that the optimum range of prolonged light durations for the establishment of Z. marina plants is 3.9–4.6 h d−1. Especially, the propagation coefficient of Z. marina plants exposed to the optimum range of prolonged light durations were 1.9–2.1 times higher than that under the control. Some studies on terrestrial vegetations demonstrated that prolonged light duration had a positive impact on the growth of plants. For example, leaves elongation and biomass of Nicotiana tabacum increased with the increase of prolonged light durations based on the natural light durations, in which the leaf length and plant height of plants exposed to 2 h d−1 increased 7.2 and 5.5% compare to the control (0 h d−1), respectively (Xu et al., 2019). Li et al. (2009) indicated that the seedlings of Cucurbita pepo obtained a higher dry weight growth rate (6.6 mg shoot−1) under full sunlight (24 h d−1), which was 2.0 times higher than that of plants under the treatment of 14 h d−1.
Similar results are also found on submerged plants. For example, Dennison and Alberte (1985) conducted a field experiment and demonstrated that the leave biomass of Z. marina plants under prolonged light duration of 3.0–5.0 h d−1 was 1.1 times higher than that of plants under the prolonged light duration of 4.0–6.0 h d−1. These results suggest that appropriate prolonged light duration has an important role in promoting the growth and development of plants, yet overlong duration of prolonged light has no obvious effect on the growth of plants, even limits the plant growth. Our results found that no marked differences in survival rate, internode elongation rate, ramet frequency and productivity were found between the treatments of 8 h d−1 and 0 h d−1.
Some studies have found that the photosynthetic function, photosynthetic rate and dry matter synthesis of terrestrial plants reduced due to the light suppression under the excessive light duration, mostly limiting the extension of underground tissues (Digby and Dyson, 1973; Zhang et al., 2012; Nitschke et al., 2017). For example, root of Vitis champinii exhibited higher growth rate when they grew under 10 h d−1 (up to 7.2 mm day−1) than those under the 14 h d−1 (Mahmud et al., 2018). The stem diameter of Vigna angularis exposed to the 12 h d−1 was up to 1.8 cm, which was lower than that under natural light duration (Dong et al., 2015).
In addition, these results also found that the optimum range of prolonged light duration range of seagrasses was larger than that of terrestrial plants. To adapt the marine low light environment, seagrasses have lower light exploitation rate (3–10 mg O2 DW−1 h−1; Vermaat et al., 1997), lower light requirement (no less than 10%; Duarte, 1991) and lower light compensation point compare with terrestrial plants (Jiang et al., 2013). Moreover, seagrasses can reach net photosynthetic production at lower light compensation point and make them grow normally (Vermaat et al., 1997; Alcoverro et al., 2001). Appropriate prolonged light durations could significantly promote the growth and development of plants when incident light intensity is above the light compensation point of plants (Birch et al., 1998; Searle and Coupland, 2014; Liu et al., 2021).
4.2 Physiological Response of Seagrass to Different Prolonged Light Duration
The results of our study revealed that an increase in the content of non-structural carbohydrates (starch and soluble sugar) and chlorophyll was found in the response of Z. marina plants to suitable prolonged light duration, which promoted the survival, growth and propagation. Especially, the aboveground soluble sugar and chlorophyll a content were 1.4–1.6 and 1.2–1.3 times higher than those under the control. Some studies on terrestrial vegetation demonstrated that non-structural carbohydrates, especially soluble sugars, not only exist widely in plants as signal molecules and regulate physiological processes such as growth and development of plants (Dennison and Alberte, 1985; Zhang et al., 2021), but also can be used as energy supply to promote growth and development of plants (Oberhuber et al., 1993; Hao and Papadopoulos, 1999). And it also provides a carbon skeleton for the synthesis of organic matter such as plant tissue proteins (Oliveira and Coruzzi, 1999). For example, these studies on the Cucumis sativus and Lycopersicon esculentum have proved that the increase of photosynthetic efficiency and the accumulation of starch and sugar promoted the growth of plants (Digby and Dyson, 1973; Demers et al., 1998; Pettersen, et al., 2010; Doğru, 2020). The soluble sugar of Helianthus annuus irradiated by red light for 20 h d−1 increased by 38.6% compared with 12 h d−1, indicating that the sugar content of plant leaves affected starch synthesis by regulating the activity of caramel acidification enzyme (AGPase) to regulate the growth of plants (Zhang et al., 2012).
Similar results are also found on submerged plants. For example, the longer photoperiod in summer could accumulate higher soluble sugar (as higher as 96.2 mg g−1) for Thalassia hemprichii, which allowed it to adapt quickly to the environment (Jiang et al., 2013). Dennison and Alberte (1985) indicated that increased prolonged light durations had a positive impact on the maximum net photosynthetic rate (Pmax) and chlorophyll content of Z. marina leaves under 3.0–5.0 h d−1, and had a negative effect on Pmax under 4.0–6.0 h d−1. Consequently, the appropriate durations of prolonged light can improve the accumulation of soluble sugar in submerged plants and promote significantly their growth and respiration.
However, excessive prolonged light duration resulted in a lower photosynthetic efficiency and amount of soluble sugar synthesis of plants (Li et al., 2021b). The leaves of plants expressed photoinhibition during the excessive prolonged light durations with lower accumulation of photosynthetic pigments and synthesis of dry matter such as starch, resulting in slow growth and reducing ramet frequency of plants (Ruiz and Romero, 2003; Campbell et al., 2012). For example, the plants of Nicotiana tabacum exposed to the light duration of 3 h d−1 indicated an obvious photoinhibition, in which the net photosynthetic rate was significantly lower than that of plants under the 2 h d−1 (Xu et al., 2019).
5 Conclusion
Plant transplantation is considered one of the relatively mature methods for seagrass bed restoration, however, the large-scale acquisition of donor plants is still a bottleneck for this method. A propagation approach that has been used for large-scale production of donor plants of Z. marina involves collecting stock plants and growing ramets in controlled culture systems until they can be out-planted to restoration sites in recent restoration projects. Light, temperature, inorganic nutrients and salinity affect biochemical processes of organisms, and are considered as major factors controlling seagrass growth (Lee et al., 2007; Touchette, 2007). To date, the effects of temperature and inorganic nutrients on the growth and propagation of seagrasses have been well studied to improve the propagation method of Z. marina plants (Howard et al., 2016). However, the response of the plants of Z. marina to prolonged light duration is still unknown. We have demonstrated that prolonged light displayed a significant effect on the growth and development of Z. marina plants. We have found that prolonged light duration of 3.9–4.6 h d−1 may be the optimal range to establish plants of Z. marina. Our results demonstrate that the prolongation of light can be an effective method to produce plenty of ramets and the method should be considered for future large-scale acquisition of donor plants of Z. marina in the restoration projects. And we have also found that the increase of chlorophyll content and accumulation and synthesis of non-structural carbohydrate may attribute to be the promotion of prolonged light duration to the survival and growth of Z. marina plants. However, successful propagation of seagrasses is highly affected by many environmental factors. Repeated experiments at more potential influence factors may be necessary.
Data Availability Statement
The original contributions presented in the study are included in the article/supplementary material, further inquiries can be directed to the corresponding author.
Author Contributions
Y-H Z analysed the data and wrote the manuscript. J-D L, S-J Y, and Z-X W designed the study, performed the experiment, and helped perform the experiment and contributed substantially to revisions. P-D Z and W-T L designed the study, performed the experiment, and contributed substantially to revisions.
Funding
This study was supported by the funds from the National Key R&D Program of China (2019YFD0901302), National Natural Science Foundation of China (42076100), and Joint Funds of the National Natural Science Foundation of China (U2006214).
Conflict of Interest
The authors declare that the research was conducted in the absence of any commercial or financial relationships that could be construed as a potential conflict of interest.
Publisher’s Note
All claims expressed in this article are solely those of the authors and do not necessarily represent those of their affiliated organizations, or those of the publisher, the editors and the reviewers. Any product that may be evaluated in this article, or claim that may be made by its manufacturer, is not guaranteed or endorsed by the publisher.
Acknowledgments
We thank Shi-Qi Li, Chao Li, and Hong-Yu Zhang for their assistance during experimental apparatus constructed, and we thank Kun-Xiu Xie and Jia Lu for their assistance during sample collection and calculations.
References
Aguilar-Perera, A., and Appeldoorn, R. S. (2007). Variation in Juvenile Fish Density along the Mangrove–Seagrass–Coral Reef Continuum in SW Puerto Rico. Mar. Ecol. Prog. Ser. 348, 139–148. doi:10.3354/meps07082
Alcoverro, T., Cerbiān, E., and Ballesteros, E. (2001). The Photosynthetic Capacity of the Seagrass Posidonia Oceanica: Influence of Nitrogen and Light. J. Exp. Mar. Biol. Ecol. 261 (1), 107–120. doi:10.1016/S0022-0981(01)00267-2
Bertelli, C. M., and Unsworth, R. K. F. (2018). Light Stress Responses by the Eelgrass, Zostera Marina (L). Front. Environ. Sci. 6, 39. doi:10.3389/fenvs.2018.00039
Birch, C. J., Hammer, G. L., and Rickert, K. G. (1998). Temperature and Photoperiod Sensitivity of Development in Five Cultivars of Maize (Zea mays L.) from Emergence to Tassel Initiation. Field Crops Res. 55, 93–107. doi:10.1016/S0378-4290(97)00062-2
Campbell, J. E., Yarbro, L. A., and Fourqurean, J. W. (2012). Negative Relationships between the Nutrient and Carbohydrate Content of the Seagrass Thalassia Testudinum. Aquat. Bot. 99, 56–60. doi:10.1016/j.aquabot.2012.02.002
Castellanos-Iglesias, S., Siret-Martínez, S. L., Di Domenico, M., Martínez-Daranas, B., and Haddad, M. A. (2021). Epiphytic Hydroid Community as Sentinels of Seagrass Condition and Human Impacts. Mar. Pollut. Bull. 173, 112939. doi:10.1016/j.marpolbul.2021.112939
Davey, P. A., Pernice, M., Ashworth, J., Kuzhiumparambil, U., Szabó, M., Dolferus, R., et al. (2018). A New Mechanistic Understanding of Light-Limitation in the Seagrass Zostera Muelleri. Mar. Environ. Res. 134, 55–67. doi:10.1016/j.marenvres.2017.12.012
Demers, D.-A., Dorais, M., Wien, C. H., and Gosselin, A. (1998). Effects of Supplemental Light Duration on Greenhouse Tomato (Lycopersicon esculentum Mill.) Plants and Fruit Yields. Sci. Hortic. 74, 295–306. doi:10.1016/S0304-4238(98)00097-1
Demers, D. A., and Gosselin, A. (2002). Growing Greenhouse Tomato and Sweet Pepper under Supplemental Lighting: Optimal Photoperiod, Negative Effects of Long Photoperiod and Their Causes. Acta Hortic. 580, 83–88. doi:10.17660/ActaHortic.2002.580.9
Dennison, W., and Alberte, R. (1985). Role of Daily Light Period in the Depth Distribution of Zostera Marina (Eelgrass). Mar. Ecol. Prog. Ser. 25, 51–61. doi:10.3354/meps025051
Digby, J., and Dyson, P. W. (1973). A Comparison of the Effects of Photoperiod, and of a Growth Retardant (CCC) on the Control of Stem Extension in Potato. Potato Res. 16, 159–167. doi:10.1007/BF02360617
Doğru, A. (2020). Photochemical Responses of Cucumber (Cucumis Sativus L.) Plants to Heat Stress. Not. Sci. Biol. 12, 829–835. doi:10.15835/nsb12410815
Dong, W., Zhang, Y., Zhang, Y., Ren, S., Wei, Y., and Zhang, Y. (2016). Short-day Photoperiod Effects on Plant Growth, Flower Bud Differentiation, and Yield Formation in Adzuki Bean (Vigna Angularis L). Int. J. Agric. Biol. 18 (2), 337–345. doi:10.17957/IJAB/15.0091
Duarte, C. M. (1991). Seagrass Depth Limits. Aquat. Bot. 40, 363–377. doi:10.1016/0304-3770(91)90081-f
Fukuda, N., Suzuki, K., and Ikeda, H. (2000). Effects of Supplemental Lighting from 23:00 to 7:00 on Growth of Vegetables Cultured by NFT. J. Japan Soc. Hort. Sci. 69 (1), 76–83. doi:10.2503/jjshs.69.76
Ge, G., and Zhao, Z. (2019). Combining Iodic Acid and Nitric Acid to Fabricate Carbon Nitride Tubes for Enhanced Hydrogen Evolution under Visible Light. Catal. Sci. Technol. 9 (2), 266–270. doi:10.1039/c8cy02006j
Hao, X., and Papadopoulos, A. P. (1999). Effects of Supplemental Lighting and Cover Materials on Growth, Photosynthesis, Biomass Partitioning, Early Yield and Quality of Greenhouse Cucumber. Sci. Hortic. 80, 1–18. doi:10.1016/S0304-4238(98)00217-9
Howard, J. L., Perez, A., Lopes, C. C., and Fourqurean, J. W. (2016). Fertilization Changes Seagrass Community Structure but Not Blue Carbon Storage: Results from a 30-year Field Experiment. Estuar. Coast 39 (5), 1422–1434. doi:10.1007/s12237-016-0085-1
Jiang, Z., Huang, X., and Zhang, J. (2013). Dynamics of Nonstructural Carbohydrates in Seagrass Thalassia Hemprichii and its Response to Shading. Acta Oceanol. Sin. 32 (8), 61–67. doi:10.1007/s13131-013-0342-0
Lee, K.-S., and Park, J.-I. (2008). An Effective Transplanting Technique Using Shells for Restoration of Zostera Marina Habitats. Mar. Pollut. Bull. 56, 1015–1021. doi:10.1016/j.marpolbul.2008.02.010
Lee, K.-S., Park, S. R., and Kim, J.-B. (2005). Production Dynamics of the Eelgrass, Zostera Marina in Two Bay Systems on the South Coast of the Korean Peninsula. Mar. Biol. 147, 1091–1108. doi:10.1007/s00227-005-0011-8
Lee, K.-S., Park, S. R., and Kim, Y. K. (2007). Effects of Irradiance, Temperature, and Nutrients on Growth Dynamics of Seagrasses: A Review. J. Exp. Mar. Biol. Ecol. 350, 144–175. doi:10.1016/j.jembe.2007.06.016
Li, H., Liu, J., and Che, X. (2021a). Establishing Healthy Seedlings of Enhalus Acoroides for the Tropical Seagrass Restoration. J. Environ. Manag. 286, 112200. doi:10.1016/j.jenvman.2021.112200
Li, H. Y., Han, G. H., Ren, Q. P., and Lv, F. T. (2009). The Effect of Different Photoperiod on Growth of Cucumber Seedling. Acta. Agric. Boreal. Occident. Sin. 18 (3), 201–203. (in Chinese). doi:10.3969/j.issn.1004-1389.2009.03.045
Li, J., Lu, Y., Chen, H., Wang, L., Wang, S., Guo, X., et al. (2021b). Effect of Photoperiod on Vitamin E and Carotenoid Biosynthesis in Mung Bean (Vigna Radiata) Sprouts. Food Chem. 358, 129915. doi:10.1016/j.foodchem.2021.129915
Liu, W., Feke, A., Leung, C. C., Tarté, D. A., Yuan, W., Vanderwall, M., et al. (2021). A Metabolic Daylength Measurement System Mediates Winter Photoperiodism in Plants. Dev. Cell 56, 2501–2515. doi:10.1016/j.devcel.2021.07.016
Mahmud, K. P., Holzapfel, B. P., Guisard, Y., Smith, J. P., Nielsen, S., and Rogiers, S. Y. (2018). Circadian Regulation of Grapevine Root and Shoot Growth and Their Modulation by Photoperiod and Temperature. J. Plant Physiol. 222, 86–93. doi:10.1016/j.jplph.2018.01.006
Martin, B. C., Statton, J., Siebers, A. R., Grierson, P. F., Ryan, M. H., and Kendrick, G. A. (2018). Colonizing Tropical Seagrasses Increase Root Exudation under Fluctuating and Continuous Low Light. Limnol. Oceanogr. 63, 381–391. doi:10.1002/lno.10746
Matsuda, R., Ozawa, N., and Fujiwara, K. (2014). Leaf Photosynthesis, Plant Growth, and Carbohydrate Accumulation of Tomato under Different Photoperiods and Diurnal Temperature Differences. Sci. Hortic. 170, 150–158. doi:10.1016/j.scienta.2014.03.014
Misturini, D., and Colling, L. A. (2021). Can Short-Term Meteorological Events Alter Subtropical Estuarine Macrobenthic Assemblages in Seagrass Meadows (Patos Lagoon Estuary - Southern Brazil)? Estuar. Coast. Shelf Sci. 261, 107532. doi:10.1016/j.ecss.2021.107532
Nitschke, S., Cortleven, A., and Schmülling, T. (2017). Novel Stress in Plants by Altering the Photoperiod. Trends Plant Sci. 22 (11), 913–916. doi:10.1016/j.tplants.2017.09.005
Oberhuber, W., Dai, Z.-Y., and Edwards, G. E. (1993). Light Dependence of Quantum Yields of Photosystem II and CO2 Fixation in C3 and C4 Plants. Photosynth. Res. 35, 265–274. doi:10.1007/bf00016557
Oliveira, I. C., and Coruzzi, G. M. (1999). Carbon and Amino Acids Reciprocally Modulate the Expression of Glutamine Synthetase in Arabidopsis. Plant Physiol. 121, 301–310. doi:10.1104/pp.121.1.301
Olsen, J. L., Rouzé, P., Verhelst, B., Lin, Y.-C., Bayer, T., Collen, J., et al. (2016). The Genome of the Seagrass Zostera Marina Reveals Angiosperm Adaptation to the Sea. Nature 530, 331–335. doi:10.1038/nature16548
Ooi, J. L. S., Kendrick, G. A., Van Niel, K. P., and Affendi, Y. A. (2011). Knowledge Gaps in Tropical Southeast Asian Seagrass Systems. Estuar. Coast. Shelf Sci. 92, 118–131. doi:10.1016/j.ecss.2010.12.021
Park, H. J., Park, T. H., Kang, H. Y., Lee, K.-S., Kim, Y. K., and Kang, C.-K. (2021). Assessment of Restoration Success in a Transplanted Seagrass Bed Based on Isotopic Niche Metrics. Ecol. Eng. 166, 106239. doi:10.1016/j.ecoleng.2021.106239
Park, J.-I., and Lee, K.-S. (2007). Site-specific Success of Three Transplanting Methods and the Effect of Planting Time on the Establishment of Zostera Marina Transplants. Mar. Pollut. Bull. 54, 1238–1248. doi:10.1016/j.marpolbul.2007.03.020
Pettersen, R. I., Torre, S., and Gislerød, H. R. (2010). Effects of Leaf Aging and Light Duration on Photosynthetic Characteristics in a Cucumber Canopy. Sci. Hortic. 125, 82–87. doi:10.1016/j.scienta.2010.02.016
Ruiz, J. M., and Romero, J. (2003). Effects of Disturbances Caused by Coastal Constructions on Spatial Structure, Growth Dynamics and Photosynthesis of the Seagrass Posidonia Oceanica. Mar. Pollut. Bull. 46, 1523–1533. doi:10.1016/j.marpolbul.2003.08.021
Schmid, R., and Clauss, H. (1975). Multiplication and Protein Content of Chloroplasts ofAcetabularia Mediterranea in Blue Light after Prolonged Irradiation with Red Light. Protoplasma 85, 315–325. doi:10.1007/BF01567956
Searle, I., and Coupland, G. (2004). Induction of Flowering by Seasonal Changes in Photoperiod. Embo. J. 23, 1217–1222. doi:10.1038/sj.emboj.7600117
Short, F. T., and Duarte, C. M. (2001). “Methods for the Measurement of Seagrass Growth and Production,” in Global Seagrass Research Methods. Editors F. T. Short, and R. G. Coles (Amsterdam: Elsevier), 155–182. doi:10.1016/b978-044450891-1/50009-8
Stockbridge, J., Jones, A. R., Gaylard, S. G., Nelson, M. J., and Gillanders, B. M. (2021). Evaluation of a Popular Spatial Cumulative Impact Assessment Method for Marine Systems: A Seagrass Case Study. Sci. Total Environ. 780, 146401. doi:10.1016/j.scitotenv.2021.146401
Touchette, B. W. (2007). Seagrass-salinity Interactions: Physiological Mechanisms Used by Submersed Marine Angiosperms for a Life at Sea. J. Exp. Mar. Biol. Ecol. 350, 194–215. doi:10.1016/j.jembe.2007.05.037
Vermaat, J. E., Agawin, N. S. R., Fortes, M. D., Uri, J. S., Duarte, C. M., and Marba, N. (1997). The Capacity of Seagrasses to Survive Increased Turbidity and Siltation: The Significance of Growth Form and Light Use. Ambio 26 (8), 499–504.
Wilhelm, C., Kramer, P., and Wild, A. (1985). Effect of Different Light Qualities on the Ultrastructure, Thylakoid Membrane Composition and Assimilation Metabolism of Chlorella Fusca. Physiol. Plant 64, 359–364. doi:10.1111/j.1399-3054.1985.tb03353.x
Xiao, L. T., and Wang, S. G. (2005). Experimental Techniques of Plant Physiology. Beijing: China Agricultural Press, 262. (in Chinese).
Xu, C. H., Li, J. Y., Cui, M. K., Ma, E. D., Huang, G. B., and Gong, M. (2019). Effects of Supplemental Lighting on Growth and Photosynthesis of Tobacco Leaves. Acta. Bot. Boreal. Occident. Sin. 33 (4), 763–770. (in Chinese). doi:10.3969/j.issn.1000-4025.2013.04.018
Yamamoto, H., Horiuchi, Y., Ogura, R., Sakai, H., Sato, H., and Kato, K. (2016). Identification and Molecular Mapping of Flowering Date1 (FD1), a Major Photoperiod Insensitivity Gene in the Adzuki Bean (Vigna Angularis). Plant Breed. 135, 1–7. doi:10.1111/pbr.12412
Yue, S., Zhang, X., Xu, S., Liu, M., Qiao, Y., Zhang, Y., et al. (2021). The Super Typhoon Lekima (2019) Resulted in Massive Losses in Large Seagrass (Zostera Japonica) Meadows, Soil Organic Carbon and Nitrogen Pools in the Intertidal Yellow River Delta, China. Sci. Total Environ. 793, 148398. doi:10.1016/j.scitotenv.2021.148398
Zhang, H., Zhang, L. L., Li, W., Xing, Z. N., Zhang, D., and Cui, J. (2012). Effects of Photoperiod under Red LED on Growth and Quality of Sunflower Sprouts. Acta horticul. Sin. 39 (2), 297–304. (in Chinese). doi:10.1007/s11033-012-1527-x
Zhang, Q., Liu, J., Zhang, P.-D., Liu, Y.-S., and Xu, Q. (2015). Effect of Silt and Clay Percentage in Sediment on the Survival and Growth of Eelgrass Zostera Marina: Transplantation Experiment in Swan Lake on the Eastern Coast of Shandong Peninsula, China. Aquat. Bot. 122, 15–19. doi:10.1016/j.aquabot.2015.01.001
Zhang, Y., Zhao, P., Yue, S., Liu, M., Qiao, Y., Xu, S., et al. (2021). New Insights into Physiological Effects of Anoxia under Darkness on the Iconic Seagrass Zostera Marina Based on a Combined Analysis of Transcriptomics and Metabolomics. Sci. Total Environ. 768, 144717. doi:10.1016/j.scitotenv.2020.144717
Keywords: Zostera marina, light, survival, propagation, chlorophyll, carbohydrate
Citation: Zhang Y-H, Li J-D, Wu Z-X, Yuan S-J, Li W-T and Zhang P-D (2022) Effects of Different Prolonged Light Durations on Survival, Growth and Physiology of the Eelgrass Zostera marina. Front. Environ. Sci. 10:893377. doi: 10.3389/fenvs.2022.893377
Received: 10 March 2022; Accepted: 30 May 2022;
Published: 20 June 2022.
Edited by:
Orsolya Valkó, Centre for Ecological Research, HungaryReviewed by:
Virginia Menicagli, University of Pisa, ItalyJianxiang Feng, Sun Yat-Sen University, China
Copyright © 2022 Zhang, Li, Wu, Yuan, Li and Zhang. This is an open-access article distributed under the terms of the Creative Commons Attribution License (CC BY). The use, distribution or reproduction in other forums is permitted, provided the original author(s) and the copyright owner(s) are credited and that the original publication in this journal is cited, in accordance with accepted academic practice. No use, distribution or reproduction is permitted which does not comply with these terms.
*Correspondence: Pei-Dong Zhang, emhhbmdwZHNnQG91Yy5lZHUuY24=