- 1College of Resources and Environment, Shandong Agricultural University, Taian, China
- 2National Engineering Research Center for Efficient Utilization of Soil and Fertilizer Resources, Taian, China
- 3Key Laboratory of Land Surface Pattern and Simulation, Institute of Geographic Sciences and Natural Resources Research, CAS, Beijing, China
- 4College of Resources and Environment, University of Chinese Academy of Sciences, Beijing, China
- 5Wells Fargo, San Francisco, CA, United States
With global urbanization and industrialization, environmental pollution and food safety problems caused by soil heavy metal pollution occur frequently. To realize the safe utilization of cultivated land resources in high-risk areas of heavy metal pollution, we present an approach to safe utilization classification and management in this study. A typical agricultural area around industrial and mining enterprises located in the economic belt of the Yangtze River Delta was chosen as the research area with cultivated soil as the research object. A total of 1,139 geochemical survey sampling sites and soil survey data were used for this research. Initially, the potential ecological risk index was used to assess the potential ecological risk of heavy metals in cultivated soil, and key soil physical and chemical indicators were chosen to assess soil resilience. Next, the safe utilization classification of cultivated land was carried out by combining the potential ecological risk of soil heavy metals with soil resilience. Then, the specific classification management strategy was developed according to ecological risk factor types and crop types. The results showed that the production and operation activities of industrial and mining enterprises in the study area contributed significantly to soil Cd and Hg pollution, and the potential ecological risk of heavy metals in the surrounding soil was high. The soil resilience of cultivated land in the study area was generally not high. The clay content and cation exchange capacity (CEC) were the main strong restrictive indicators, while CaO, soil organic carbon (SOC), and pH were the main medium restrictive indicators. Natural conditions such as topography, hydrogeology, and soil-forming parent material differed by region, resulting in different restrictive factors. There were 16 safe utilization types in the study area. Different management strategies were proposed based on various soil potential ecological risks, soil resilience, ecological risk factor types, and crop types. This study can offer fresh perspectives on the safe utilization classification and management of land resources in high-risk areas of soil pollution and serve as a reference for sustainable intensification.
1 Introduction
In parallel with economic development, air, water, and soil pollution have increased due to excessive use of non-renewable energy and climate change (Elahi et al., 2021a; Khan et al., 2021; Elahi et al., 2022a; Elahi et al., 2022b; Tang et al., 2022). Heavy metals (HM) provide a direct and indirect threat to food safety, the ecological environment, and human health because of their characteristics of difficult degradation, mobility, easy enrichment, and potential toxicity (He et al., 2013; Wang et al., 2016; de Lima et al., 2022; Wei et al., 2022). Heavy metal accumulation in soils is significantly influenced by human activities connected to urbanization and industrialization, including industrial manufacturing, agricultural inputs, sewage irrigation, waste treatment, and vehicle exhaust (Li et al., 2008; Foucault et al., 2013; Doabi et al., 2018; Xiao et al., 2019; Hu et al., 2020). Therefore, the issue of soil heavy metal pollution has gained increased attention as a result of the rapid rise of urbanization and industrialization. The soil environment status was not favorable, according to China’s “National Soil Pollution Survey Bulletin,” which was issued in 2014. In China, the exceedance rate of all soil sampling sites was 16.1% and the exceedance rate of cultivated soil sampling sites was 19.4%, primarily due to inorganic pollution, with Cd severely exceeding the limit (Zhao et al., 2015). Soil heavy metal pollution has caused great concern around the world, especially for cultivated land. In 2013, the FAO designated December 5 as “World Soil Day” and 2015 as the “International Year of Soils,” with the theme of “healthy soil for a healthy life.” In 2015, China hosted a forum titled “Soil and Ecological Environment Security—International Year of Soils,” which emphasized the importance of monitoring and warning about heavy metal pollution in agricultural product–producing areas, strengthening agricultural planting structure adjustment, carrying out heavy metal pollution repair and treatment in agricultural product–producing areas, implementing strict hierarchical management, and delimiting prohibited production areas for agricultural products. In 2016, the State Council issued an action plan for soil pollution prevention and control, which calls for the implementation of the safe utilization classification and management of cultivated land (China State Council, 2016).
A challenge that needs to be solved is how to scientifically carry out the safe utilization classification of cultivated land. Some pertinent research has been conducted. Based on the requirements of the action plan (China State Council, 2016), according to the pollutant risk screening value and control value in GB15618 (Ministry of Ecology and Environment of People’s Republic of China, 2018; Li et al., 2019), farmlands are classified as follows: priority protection (HMsoil < risk screening value), safe utilization (risk screening value ≤ HMsoil ≤ risk intervention value), and strict control (HMsoil > risk intervention value). Kong and Zhang (2021) classified the farmland around the Beijing Plain into safe, low-risk, medium-risk, and high-risk utilization areas based on the geoaccumulation index and potential ecological risk index and proposed control strategies according to the core pollution sources in each area. Liu et al. (2016) proposed a safe utilization classification and management strategy based on the single factor pollution index, Nemero pollution index, and potential ecological risk index. Gao et al. (2021) determined the redevelopment priority of abandoned industrial and mining land through the comprehensive pollution risk rating of soil heavy metals. However, the aforementioned classification scheme only considers the total amount and exceedance of heavy metals in soil, ignoring the actual impact of heavy metals on crop safety and the ecological environment. The concentration of heavy metals in crop particles and the total amount of heavy metals in the soil are not always significantly correlated (Gu et al., 2018). Therefore, there is the possibility of “false positives” or “mistaken judgments” (Yu et al., 2020), leading to bias in safe utilization classification. For instance, the soil in a priority protection area may have a high ecological risk, or the strictly controlled area may squander land resources as a result of an overestimation of the soil ecological risk (Yang Q. et al., 2021; Yang Y. et al., 2021). To address this issue, the researchers proposed an improved classification scheme, which primarily incorporates crop safety status into the classification basis. Yu et al. (2020) developed a model of safe utilization classification based on the link between crop and human biomarker concentrations and crop and topsoil concentrations, which was then applied to the safe utilization of Se-rich farmland with Cd. Yang Q. et al. (2021) proposed the recommended threshold values for the safe utilization and risk control of Cd in karst areas with a high geochemical background, based on the relationship between soil pH and Cd in the study area and Cd in rice particles. Li et al. (2021) and Yang Y. et al. (2021) proposed a farmland safe utilization scheme using the Cd concentration in topsoil and the predicted Cd concentration in crop grains.
The absorption, accumulation, and redistribution of heavy metals by crops depend not only on the total amount of heavy metals in soil but also on the bioavailability of heavy metals. For example, karst soils in Jamaica and central and eastern Guangxi, China, are rich in heavy metals, but the concentrations and exceedance values in local rice grains are low due to limited bioavailability (Zhang and Lalor, 2002; Grant et al., 2013; Gu et al., 2018; Wen et al., 2020). Similarly, if heavy metals’ potential mobility is low, their impact on the surrounding environment, such as the groundwater, will be limited. It is well known that the bioavailability and potential mobility of soil heavy metals are closely related to soil properties, such as the pH, organic matter content, and clay content (Mcbride, 2002; Usman et al., 2004; Zeng et al., 2011; Mahar et al., 2016). These key soil physical and chemical properties form the filtering, buffering, and biochemical transformation capabilities (Blum, 2005), which provide the soil with some resilience in the face of heavy metal pollution (Blum, 2000; Schiefer et al., 2015; Schaeffer et al., 2016). Soil resilience is the capacity of a system to return to (a new) equilibrium after disturbance (Blum and Aguilar, 1994; Ludwig et al., 2018). Resilient soils have a high rate of recovery, a high resilience, high buffering capacity, and low malleability and may also be hysteretic because of intrinsic soil properties that influence the recovery path (Schiefer et al., 2016). Thus, soil resilience can reduce heavy metal bioavailability and potential mobility, lowering the threat of heavy metal pollution to crop safety, the ecological environment, and human health. In this sense, we can combine the pollution status reflected by the total amount of soil heavy metals with soil resilience to carry out safe utilization classification of land.
In summary, we chose Yixing County, which is located in the Yangtze River Delta’s economic belt, as the research area and cultivated soil as the research object to carry out the following research: 1) evaluate the potential ecological risk of cultivated soil heavy metals using total soil heavy metal data; 2) select key soil indicators to evaluate the soil resilience of cultivated land; and 3) carry out safe utilization classification of cultivated land by combining the potential ecological risk of soil heavy metals with soil resilience and propose classification management strategies based on ecological risk factor types and crop types. It is hoped that this study will provide a reference for the safe utilization classification and management of cultivated land in high-risk areas. The article is divided into five sections: Section 1 deals with the study background, problem identification, and research gap. Section 2 describes the study area, data sources and processing, and the methods used to address the study objectives. Section 3 relates to the explanation of the study results. Section 4 discusses the value of the study and suggests some policies and future research recommendations. Section 5 summarizes the main findings.
2 Materials and methods
2.1 Study area
The study site is located in Yixing County, southwest of Jiangsu Province, China (31°07′–31°37′ N and 119°31′–120°03′ E) (Figure 1). The region is part of the Taihu Lake Basin in the Yangtze River Delta, bordering Taihu Lake in the east and Gehu Lake in the north, and has a total area of 197,700 hm2 with a permanent population of 1.29 million. The regional climate is warm and humid, belonging to the subtropical monsoon climate, with an annual average temperature of 16.5°C and an average annual precipitation of 1,200 mm. The terrain of the region is high in the south and low in the north, with a variety of soil types. Paddy soil is dominant on the plains, which is formed by the alternation of iron and manganese reduction leaching and oxidation deposition after long-term flooding under rice planting. The hilly areas are mostly covered with acidic soils with allitization characteristics, including yellow-brown soil and red soil; red soil is more acidic and shows more obvious desilicification and allitization.
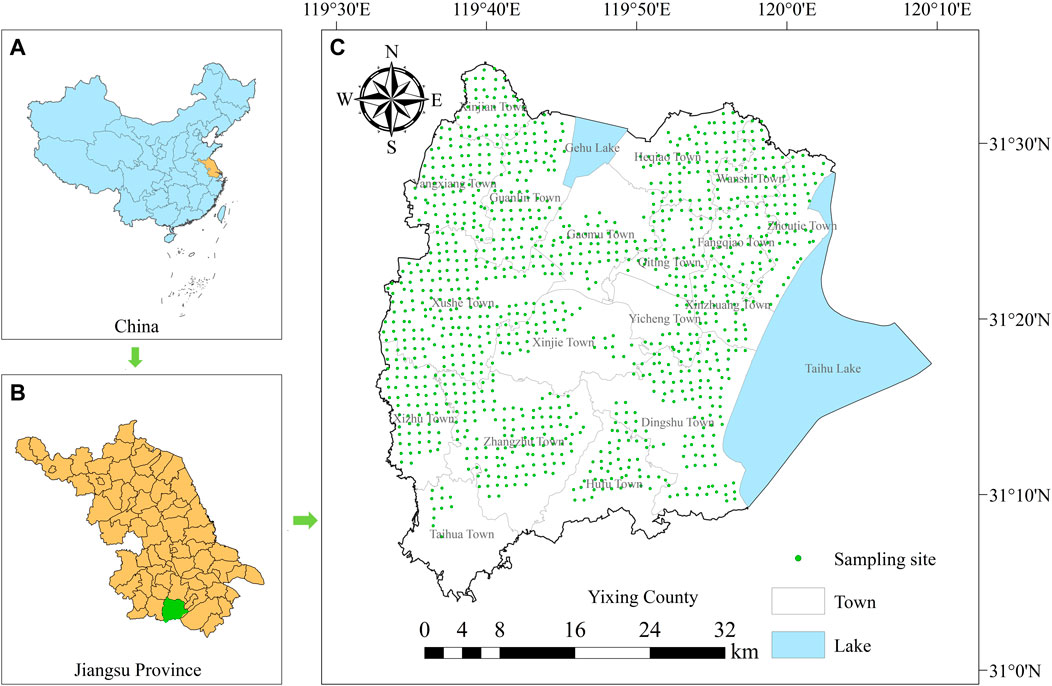
FIGURE 1. Location of the study area and distribution of sampling sites. (A,B) show the location of the study area (Yixing County) in Jiangsu Province, China, and (C) shows the administrative division of Yixing County and the distribution of sampling sites.
The region is one of the most important commodity grain production bases in the Taihu Lake Basin, with dense rivers and convenient irrigation and transportation. The region is part of the Yangtze River Delta’s economic development zone, where industrialization and agricultural intensification are prevalent, and the accumulation and exceedance of soil heavy metals due to industrial and agricultural production activities are becoming increasingly serious and have received widespread attention.
2.2 Data sources and processing
Data on the content of heavy metals (Cd, Hg, As, Pb, Cu, and Zn) in the topsoil of Yixing County were derived from the results of a land quality geochemical survey. According to the “Specification of Land Quality Geochemical Assessment” (DZ/T 0295–2016) (Ministry of Natural Resources of the People’s Republic of China, 2016), 1,139 sampling sites (including 614 cultivated land sampling sites) were distributed across the entire area using a grid structure. The sampling density was around 2 sites/km2, the sampling depth was 0–20 cm, and GPS was used for precise positioning. Pb and Cd in soil samples were determined by graphite furnace atomic absorption spectrophotometry, Hg and As by atomic fluorescence spectrometry, and Cu and Zn by flame atomic absorption spectrophotometry. The sample measurement deviation was kept to less than ±10%, and 10% of the samples were chosen for the repeated test, with a relative error of less than ±5%. Data for soil resilience indicators were derived from the results of the soil survey.
The spatial interpolation method was used to turn the sampling point data into spatial continuous data. This study focuses on soil heavy metal exceedances, especially the maximum content. Compared with other interpolation methods, inverse distance weighted (IDW) interpolation can retain information on the local peaks or troughs of the spatial distribution of soil heavy metals. The method is based on the principle of “the nearer, the more similar,” which coincides with the spatial distribution characteristics of soil heavy metals. Therefore, the IDW method was chosen for spatial interpolation. The reliability of the interpolation results was tested by cross-validation and compared with the ordinary kriging (OK) method and radial basis function (RBF) method. The mean error (ME) and root mean square error (RMSE) were used as evaluation indicators. In general, the higher the prediction accuracy, the closer the ME to 0 and the smaller the RMSE. Furthermore, if the sample sites cover the entire area evenly, the interpolation accuracy will be high. Therefore, instead of only 614 cultivated land sampling sites, a total of 1,139 sampling sites from the entire area were used for interpolation (Figure 1). For the statistical analysis of heavy metal content and the potential ecological risk assessment, we also used 1,139 sampling sites.
2.3 Evaluation method of heavy metal pollution
Accurate assessment of the heavy metal pollution status of cultivated soil has become the foundation for ensuring safe utilization of cultivated land and food security. Currently, there are various methods to evaluate heavy metal pollution, each with its own focus. There are methods for assessing single heavy metal element pollution, such as the single factor pollution index, enrichment factor method, and geoaccumulation index, as well as multi-element comprehensive assessment methods, such as the Nemero comprehensive pollution index, fuzzy mathematics method, and potential ecological risk index (Wu et al., 2015; Kong and Zhang, 2021; Wang H. et al., 2021). The potential ecological risk index proposed by Hakanson evaluates the degree of heavy metal pollution based on heavy metal toxicity and environmental response by introducing a toxicity response coefficient (Hakanson, 1980). This method is suitable for evaluating and comparing the potential ecological risk of soil over a vast area and is of great significance for the prevention and control of soil pollution. At present, this method is widely used in ecological risk assessment of soil heavy metal pollution (Cao et al., 2009; Sun et al., 2010; Iqbal and Shah, 2011; Wang et al., 2012). The potential ecological risk index (RI) is calculated as follows:
where i represents 6 heavy metal elements involved in the calculation (Cd, Hg, As, Pb, Cu, and Zn); the value range is 1–6; Ei is the potential ecological risk factor (risk coefficient of a particular heavy metal); RI is the potential ecological risk index calculated as the sum of the risk factors; Ti is the “toxic-response” factor for each heavy metal element (Cd = 30, Hg = 40, As = 10, Pb = 5, Cu = 5, and Zn = 1) (Hakanson, 1980); Pi is the pollution factor of heavy metal element i; Ci is the actual measured concentration of the element in the measured sample (mg/kg); Si is the environmental background value of the sample element i (mg/kg). The background values of Cd, Hg, As, Pb, Cu, and Zn in Yixing County are 0.19, 0.12, 10.6, and 24.8 mg/kg, respectively.
According to the different values of Ei and RI, the potential ecological risks are divided into different levels, as shown in Table 1.
2.4 Definition and identification of the soil resilience index
Soil sustainability is achieved through coordination of its various environmental, social, and economic functions, which include 1) biomass production, 2) protection of humans and the environment, 3) gene reservoirs, 4) physical basis of human activities, 5) sources of raw materials, and 6) geogenic and cultural heritage (Blum, 2005). We cannot ensure the cleanliness and safety of agricultural production or prevent groundwater and food chain pollution, unless we consider the ability of soil to provide other ecological functions besides food and biomass. Therefore, in addition to considering the function of soil biomass production, we should also consider the function of protecting humans and the environment. Soil function is founded on soil ecosystem services, which are composed of a series of soil processes (Zhao et al., 2021). On many solid, liquid, or gaseous inorganic or organic depositions, soil reacts through mechanical filtration, physicochemical absorption, and precipitation or micro-biochemical and biochemical mineralization and metabolization of organic compounds, resulting in filtering, buffering, and transformation functions (Blum, 2005). By lowering the bioavailability and potential mobility of heavy metals, these functions can reduce the risk of heavy metals to crop safety, the environment, and human health, allowing the soil to show some resilience in the face of heavy metal pollution.
To summarize, soil resilience to pollution can be reflected by the key physical and chemical indicators of the soil. Indicators provide information for understanding and managing heavy metal–contaminated land according to soil resilience. Indicators must be easily measurable and understandable for specialists, as well as for politicians, decision-makers, and farmers at the grass-root level (Schiefer et al., 2015). Based on the existing literature, expert knowledge, and data availability, we selected seven specific soil indicators, including soil organic carbon (SOC), clay content, pH, cation exchange capacity (CEC), soil depth, TFe2O3, and CaO, determined the threshold levels, and scored them as poor (1), medium (2), good (3), and excellent (4) (Table 2). Soil pH directly affects the solubility of soil heavy metal hydroxides, carbonates, and phosphates, as well as heavy metal hydrolysis, organic component dissolution, and the characteristics of soil surface charges, making it an important factor in the heavy metal adsorption process. It is generally believed that as pH increases, the adsorption capacity of soil for heavy metals increases and the effective heavy metal content decreases (Li et al., 2013). The pH of the soil system is mainly controlled by CaO (Mahar et al., 2016), and Ca ions have a buffering effect on the soil pH, which means that in an environment with high Ca2+ content, the pH value decreases slowly. Studies in the Yangtze River Delta and karst areas show that when the CaO content drops below 1%, soil acidification occurs quickly (Wang et al., 2015; Li et al., 2021). CEC is a key factor that influences soil buffering capacity. The higher the CEC, the stronger the adsorption and fixation of cations in soil and the lower the solubility and mobility of heavy metals (Zhang et al., 2018). Soil organic matter affects the migration characteristics and bioavailability of heavy metals by changing the occurrence form of soil heavy metals via the specific adsorption of heavy metal elements (Zhou et al., 2020). Clay particles are the core part of soil inorganic colloids, with small particle size, large specific surface area, and high charge density, which can increase the effective adsorption site of soil and enhance the adsorption and fixation ability of soil for heavy metals (Damian et al., 2019). Li et al. (2021) found a significant negative correlation between TFe2O3 and the ability of rice to absorb and accumulate Cd from the soil. Iron oxide in soil has a strong adsorption capacity for heavy metals and can effectively fix heavy metals (Ran et al., 2014; Qiao et al., 2020). Only under extremely acidic conditions (pH ≤ 3) can heavy metals in Fe-Mn nodules be dissolved (Ji et al., 2021). Iron spots formed by the strong iron redox reaction in the rice rhizosphere can isolate heavy metals on the root surface (Zhang et al., 2012; Hu et al., 2015). Furthermore, thick soil with superior physical and chemical properties can also prevent heavy metal migration and groundwater pollution (Schiefer et al., 2015).
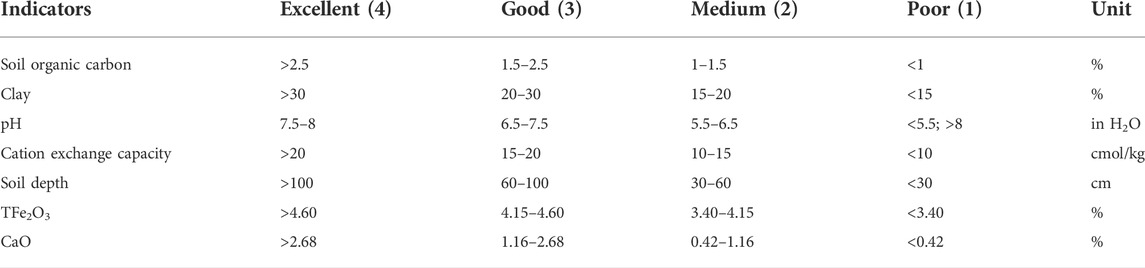
TABLE 2. Threshold levels and scoring of soil indicators (the score for each indicator is given in parentheses).
By summing up all the scores, the minimum and maximum scores for land units were 7 and 28, respectively. Based on the score, all land units were divided into five soil resilience types. Poor units scored between 7 and 14, indicating that in the face of soil heavy metal pollution, most of the inherent soil characteristics cannot support cleaner agricultural product production and a healthy groundwater environment. Moderate units scored between 15 and 21, indicating medium soil resilience, and some factors were restrictive. Based on the performance of each indicator, the moderate type was divided into two subtypes. M1-type units had at least one strong restrictive indicator (score = 1), while M2-type units did not. High units scored between 22 and 28, indicating better soil resilience to soil pollution, since these can assure the sustainability and safety of agricultural production and can maintain a healthy groundwater environment. Similarly, according to the performance of each indicator, the high type was divided into two subtypes. The H1 type only had one strong restrictive indicator. Others were of the H2 type, and the performance of each indicator was generally excellent.
3 Results
3.1 Analysis of heavy metal content
The results of the Kolmogorov–Smirnov (K-S) test showed that the soil heavy metal content data from 1,139 sampling sites in the study area showed a skewed distribution (Table 3). Therefore, the median was used to describe the average level of each heavy metal. Moreover, from the perspective of the standard deviation (SD), the SDs of Cd and Hg were small, indicating that the representation of the mean value was also good. The median values of As, Cd, Cu, Hg, Pb, and Zn were 8.48, 0.19, 27.60, 0.12, 36.09, and 69.00 mg kg−1, respectively. The median value of Pb exceeded the background value, whereas the median values of Cd and Hg were equivalent to the background values, but the mean values exceeded the background values. The order of the coefficients of variation (CVs) of the six heavy metal elements was Cd > Cu > Hg > Pb > Zn > As. The mean Cd content was higher than the background value, with a CV greater than 100%, showing a strong variability, with some sampling sites having a very high Cd content. This indicated that the Cd content was affected by external factors, resulting in uneven cumulative distribution, with anthropogenic input possibly being the primary source (Wang and Lu, 2011).
3.2 Analysis of the evaluation results of heavy metal pollution
The potential ecological risk factors (Ei-values) and potential ecological risk indexes (RI-values) of the soil heavy metals at the 1,139 sample sites were calculated using the Hakanson method.
As shown in Table 4, the mean Ei-values of the six heavy metals were in the following order: Hg > Cd > As > Pb > Cu > Zn. Hg had the highest mean value, 47.68, indicating a moderate risk level. In total, 58 sampling sites were at considerable risk, 4 sites were at high risk, and 1 site was at very high risk. The mean values of the remaining five elements were all less than 40, which denotes a low-risk level. Although Cd was at a low-risk level, the mean value exceeded 30; 192 sampling sites were still at moderate risk, 25 sites were at considerable risk, 8 sites were at high risk, and 4 sites were at very high risk. All sample sites had low-risk levels for As and Zn. Most sites for Cu and Pb were at low risk, and only a few were at moderate or considerable risk. The survey showed that 22 of the 37 sampling sites with Cd above a considerable risk level were distributed near industrial and mining enterprises, including those producing ceramics, glass, metal, cement, and stone. This finding further confirmed the important contribution of human activities to Cd accumulation in the above analysis. Moreover, the three sampling sites where Cu and Pb were at moderate or considerable risk also had industrial and mining enterprises nearby.
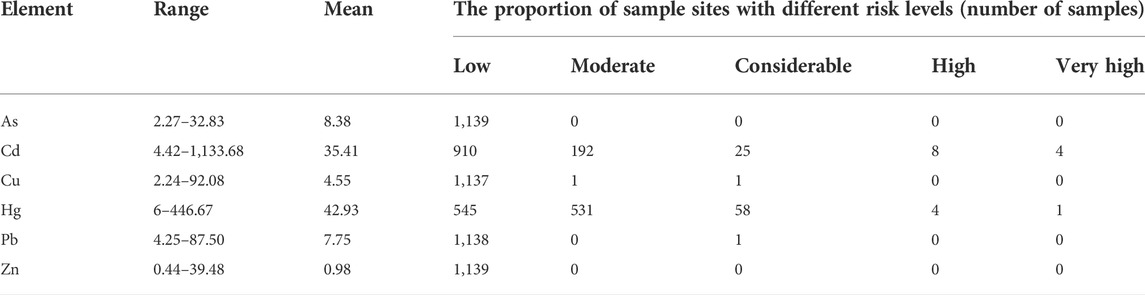
TABLE 4. Evaluation results of potential ecological risk factors (Ei-values) for heavy metals in soil.
As shown in Table 5, the RI-values of soil heavy metals ranged from 27.01 to 1,361.07, with a mean value of 100, indicating a generally low risk in the study area. In total, 94.47% of the sampling sites were at low risk, but 54 sites were still at moderate risk, 7 sites were at high risk, and 2 sites were at severe risk. At the sampling site with the highest RI-value, Cd was at high risk, Pb was at considerable risk, and Hg was at moderate risk. This site was located in Zhanzhu Town, which has a developed quarrying industry (Figure 2A), and large quarries were distributed nearby. At the sampling site with the second highest RI-value, Cd was at high risk and Hg was at moderate risk. This site was located in Dingshu Town, which has a developed pottery industry, and was surrounded by several purple clay pot workshops. Among the 7 high-risk sampling sites, 4 sites were surrounded by pottery and metal processing factories, and 3 sites were located in Dingshu Town, near the heavy-risk sample sites. Therefore, the waste gas, wastewater, and wastes released by industrial and mining enterprises in Yixing County made a significant contribution to the heavy metal pollution in the surrounding soil. Cd and Hg were the main potential risk factors (Figure 3).

TABLE 5. Evaluation results of the potential ecological risk index (RI-values) for heavy metals in soil.
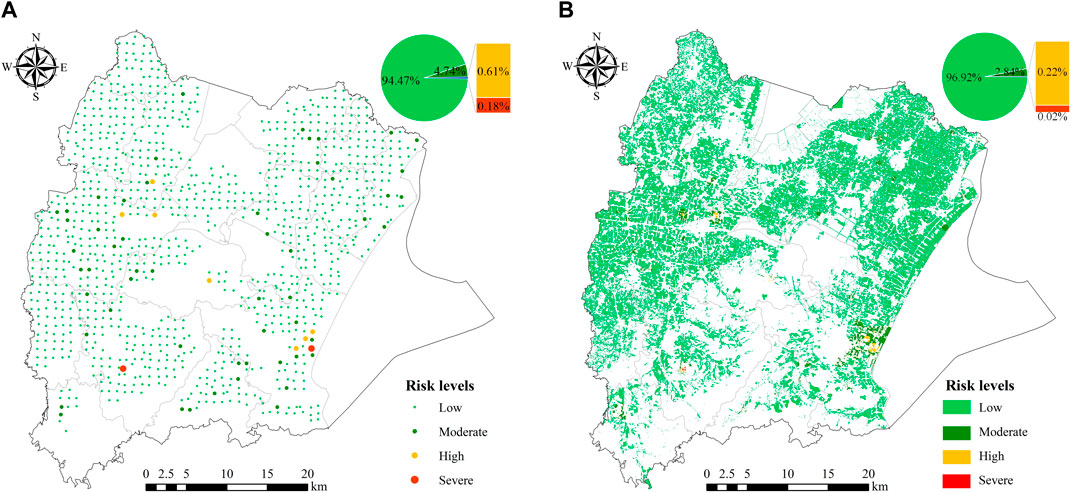
FIGURE 2. Distribution of potential ecological risk index evaluation results. (A) shows the evaluation results of the sampling sites. (B) shows the evaluation results of the cultivated land obtained by interpolation.
The RI-values of the 1,139 sample sites were interpolated using the IDW method. The cross-validation results showed that the ME was 0.00017, close to 0, and the RMSE was 55.0673 (Table 6). Compared with other methods, the ME and RMSE of IDW were less than those of OK and RBF, indicating that the IDW method had the best interpolation ability and the highest interpolation accuracy for the RI-values.
The interpolation result map was superimposed upon the cultivated land map of Yixing County and reclassified according to the classification standard of the potential ecological risk. Figure 2B shows the distribution map of the potential ecological risk level of heavy metals in the cultivated land areas of Yixing County: 96.92% of the cultivated land was at low risk, but 2.84% was still at moderate risk. Moreover, 0.22% of the cultivated land was at high risk, mainly in Dingshu Town, with a small portion near the residential area of Xushe Town, and another 0.02% of the cultivated land was at severe risk, mainly in Zhangzhu Town.
3.3 Analysis of the soil resilience of cultivated land
The overall distribution characteristics of cultivated soil resilience in Yixing County were high in the north and low in the south, and the region along the lake in the east was lower than that in the middle and the west (Figure 4). In total, 8.88% of the cultivated land had high soil resilience, mainly the H2 type distributed in low-lying polder areas in the west. The predominant soil type was paddy soil, which was formed from lacustrine sediments. The paddy soil had a deep soil layer, heavy viscosity, poor permeability, more than 30% clay content, good water and fertilizer retention, a high organic matter content, high cation exchange capacity, neutral and alkaline pH, and no strong restrictive indicators. Therefore, it could effectively filter, buffer, and undergo biochemical transformation to deal with soil heavy metal pollution. The cultivated land with high resilience and strong restrictive indicators (mainly SOC and CaO) only accounted for 0.03% of the total area, which was concentrated in the low-lying polder areas in the east. In total, 85.12% of the cultivated land had moderate soil resilience and was widely distributed in Yixing County. The northern plain area was mainly distributed with paddy soil developed from fluvial and lacustrine alluvial sediments, with a deep soil layer and staggered silt layer and clay layer. It belonged to the region with high scores in the moderate resilience area, which was primarily made up of M2-type units. Most of the units scored only 2 for SOC and pH. The low mountain and hilly areas in the south were mainly distributed with yellow-brown soil and red soil developed from acidic parent material, and the soil was generally acidic. In this area, CaO, SOC, pH, clay content, and CEC were generally strong restrictive indicators, and M1-type units were widely distributed. The beach areas along Taihu Lake and Gehu Lake were mainly distributed with paddy soils developed from lacustrine alluvium and fluvo-aquic soils developed from river-lake facies alluvium. In this area, the strong restrictive indicator was the clay content. In total, 6.01% of the cultivated land had low soil resilience and performed poorly on all indicators. Heavy metals imported into the soil in such areas often have high bioavailability and potential mobility. Low resilience units were concentrated in the beach area along Taihu Lake, with strong restrictive indicators of the clay content, CEC, and TFe2O3, while they were scattered in the southern hilly area, with strong restrictive indicators of the pH, clay content, SOC, CEC, CaO, and TFe2O3.
Through the above analysis, the restrictive indicators of soil resilience in the various geomorphic regions of Yixing County were found to vary due to the diversity of the topography, hydrology, and soil parent materials. By summarizing the low and medium graded areas of each indicator, we found that the strong restrictive indicators were mainly the clay content and CEC, which were too low in 9.00 and 7.03% of the cultivated land, respectively, and the medium restrictive indicators were mainly CaO, SOC, and pH, which were low in 87.86, 82.96, and 66.38% of the cultivated land, respectively (Figure 5).
3.4 Analysis of safe utilization of heavy metals in cultivated soil
The safe utilization classification of cultivated land in Yixing County was carried out by combining the potential ecological risk of soil heavy metals with soil resilience. The classification results showed that there were 16 types of safe utilization of cultivated land in Yixing County, as shown in Figure 6. In the classification code, the potential ecological risk grade code was at the front and the soil resilience level code was at the back.
The area of the L-H type was 4,592.45 hm2, accounting for 8.54% of the total cultivated land. This type had a low potential ecological risk of heavy metals, high soil resilience, and high coincidence with the high resilience area. The L-H2 type accounted for 4,574.76 hm2, while the L-H1 type accounted for 17.69 hm2. This type was mainly distributed in low-lying polder areas and could maintain clean production of cultivated land and a good groundwater environment for a long time. New pollution should be avoided by strictly controlling new polluting industrial and mining enterprises, strengthening irrigation water quality monitoring, and strictly controlling excessive fertilization and pesticide abuse. The degradation of key soil physical and chemical properties could be prevented by promoting the combination of planting and maintenance, returning straw to the field, increasing the application of organic fertilizer, and using less tillage or no tillage. Qualified cultivated land could be considered for inclusion in the permanent prime farmland to strictly control the occupation of non-agricultural construction. For the L-H1 type, it is necessary to focus on the improvement of strong restrictive indicators.
The area of the L-M type was 44,572.22 hm2, accounting for 82.87% of the total cultivated land. This type was widespread in Yixing County and was highly coincident with the moderate resilience area. The L-M2 type accounted for 38,572.15 hm2, while the L-M1 type accounted for 6,000.07 hm2. This type had a low potential ecological risk of heavy metals, moderate soil resilience, and some limiting key physical and chemical properties. As discussed earlier, the limiting factors in different geomorphic areas were different, so targeted soil improvement is needed to improve soil resilience, especially for the L-M1 type.
The area of the L-L type was 2,966.93 hm2, accounting for 5.52% of the total cultivated land, with a high coincidence with the low resilience area. This type had a low potential ecological risk of heavy metals and low soil resilience. Most of the key soil physical and chemical properties were severely limited. Once imported, exogenous heavy metals will have high bioavailability and potential mobility. Comprehensive soil improvement is needed to improve soil resilience.
The area of the M-H type was 169.54 hm2, accounting for 0.32% of the total cultivated land. This type was mainly distributed in the beach area of Taihu Lake east of Dingshu Town, the low-lying polder area west of Xushe Town, and the hilly area southwest of Taihua Town. The potential ecological risk of heavy metals in this type was moderate. The main ecological risk factor in the low-lying polder area was Hg, with Cd in other areas. The only subtype was M-H2, with a high soil resilience and no strong restrictive indicator. In the medium and long term, it could maintain the clean production of cultivated land and pose little threat to the groundwater environment. It is necessary to identify the specific sources of Cd and Hg, control further pollution input, and prevent the degradation of key soil physical and chemical properties.
The area of the M-M type was 1,140.92 hm2, accounting for 2.12% of the total cultivated land. This type was mainly distributed in the beach area of Taihu Lake east of Dingshu Town and the low-lying polder area west of Xushe Town, with a small amount scattered in other areas, mainly rice- and wheat-growing areas. The M-M2 type accounted for 949.17 hm2, while the M-M1 type accounted for 191.76 hm2. The M-M type had moderate potential ecological risk of heavy metals and moderate soil resilience, with Cd as the main risk factor. Pollution source control and targeted soil improvement are needed, particularly for the M-M1 type. On this basis, alternative planting measures for rice and wheat varieties with low Cd accumulation could be implemented to reduce the transfer of Cd from soil to crops, especially the edible part.
The area of the M-L type was 217.47 hm2, accounting for 0.40% of the total cultivated land. This type was scattered in the beach area of Taihu Lake and the southern hilly area, mainly the rice-growing area. The potential ecological risk of heavy metals in this type was moderate, with Cd as the main risk factor. Due to the low soil resilience, Cd has a high bioavailability and potential mobility. Based on pollution source control and comprehensive improvement of soil properties, rice varieties with low accumulation of Cd could be planted instead. Meanwhile, rice leaf regulation (spraying beneficial elements such as Si and Se to improve rice stress resistance) could inhibit the transfer of Cd from rice roots to edible parts. For the acidic soil in the southern hilly area, lime could be used for regulation.
The area of the H-H type was 7.40 hm2, accounting for 0.01% of the total cultivated land. This type was mainly distributed in the low-lying polder area east of Xushe Town, mainly the rice-growing area, while a small amount occurred in the southern hilly area, where mainly wheat and rape are grown. The potential ecological risk of heavy metals in this type was high, with Cd as the main risk factor. However, the soil resilience was also high, and the only subtype was the H-H2 type, without strong restrictive indicators. Heavy metals accumulated due to the immobilization effect, and their bioavailability and potential mobility were limited. Pollution source control and necessary agronomic control measures are needed to control the import of heavy metals and the transfer to agricultural products. It is also necessary to prevent the degradation of key soil physical and chemical properties. Considering the small area, high-cost phytoremediation technology could be implemented to reduce the concentration of heavy metals in a short time. Furthermore, agricultural product samples should be collected regularly to monitor the concentration of heavy metals in these products.
The area of the H-M type was 63.07 hm2, accounting for 0.12% of the cultivated land. This type was mainly distributed in the beach area of Taihu Lake east of Dingshu Town and the western low-lying polder area, where mainly rice and wheat are grown. The H-M2 type accounted for 59.48 hm2, while the H-M1 type accounted for 3.59 hm2. This type had a high potential ecological risk of heavy metals, with Cd as the main risk factor, some restrictive key soil properties, and a high risk of the food chain and groundwater pollution. Pollution source control and targeted soil improvement are needed, particularly for the H-M1 type. On this basis, regulation and remediation technologies could be comprehensively applied. While implementing agronomic regulation measures such as low accumulation variety substitution, flooding irrigation, and lime regulation, remediation measures such as soil conditioners, passivators, and organic fertilizers could be implemented to ensure the agricultural product quality meets the standard.
The area of the H-L type was 44.76 hm2, accounting for 0.08% of the total cultivated land. This type was concentrated in the beach area of Taihu Lake east of Dingshu Town, where mainly rice and wheat are grown. The high potential ecological risk of heavy metals and the limitation of key soil properties made this type unsuitable for the production of most edible agricultural products. It is necessary to adjust the planting structure, delimit the prohibited production areas of specific agricultural products, and consider alternative planting of non-edible cash crops and ornamental agroforestry crops.
The area of the S-H type was 6.59 hm2, accounting for 0.01% of the total cultivated land. This type was concentrated in the sample area with very high potential ecological risk factors in Zhangzhu Town. The only subtype was the S-H2 type. This type had severe potential ecological risk and high soil resilience. Cd accumulated significantly in the soil due to its limited bioavailability and potential mobility. However, considering the severe potential ecological risk, planting structure adjustment should be implemented.
The area of the S-M type was 5.27 hm2, accounting for 0.01% of the total cultivated land. This type was concentrated in the sample area with high potential ecological pollution risk factors in Zhangzhu Town. The only subtype was the S-M2 type. The potential ecological risk of heavy metals in this type was severe, with Cd as the main risk factor. Some key soil physical and chemical properties were limited. This type could not ensure the clean production of agricultural products and a good groundwater environment. The cost of soil improvement and pollution control is high. Measures such as fallowing or returning farmland to forest and grassland could be taken.
The area of the S-L type was 0.97 hm2, accounting for 0.002% of the total cultivated land. This type was concentrated in the sample area with very high potential ecological risk factors in Dingshu Town. The potential ecological risk of heavy metals in this type was severe, and the soil resilience was worse than that of the S-M type. Therefore, the measures of returning farmland to forest and grassland could be taken directly.
4 Discussion
Scientific and reasonable safe utilization classification and management of land is an important way to realize the safe utilization of land resources in high-risk areas of soil heavy metals, such as acidic soil rice-planting areas, agricultural areas around typical industrial and mining enterprises, sewage irrigation areas, suburbs of large and medium-sized cities, highly intensive vegetable bases, and areas with a high background of geological elements. In light of this, we studied the method of safe utilization classification of land. As discussed earlier, classification based only on the content of heavy metals in soil easily results in “false positives” or “mistaken judgments,” leading to bias in classification and management (Yu et al., 2020). Therefore, researchers incorporated the crop safety status into the classification basis for improvement and obtained a more reasonable classification scheme for the safe utilization of cultivated land (Yu et al., 2020; Li et al., 2021; Yang Q. et al., 2021; Yang Y. et al., 2021). We recognize that crop safety is closely related to the bioavailability of heavy metals in soil, and bioavailability is closely related to the soil resilience formed by soil buffering, filtering, and biochemical transformation functions. In addition, the collection of crop safety data is not as extensive as that of soil survey data (Yu et al., 2020; Li et al., 2021; Yang Q. et al., 2021). It is common practice to build a model through limited crop grain data to predict the crop safety situation of the whole region (Gu et al., 2019; Wen et al., 2020; Yu et al., 2020; Wang Y. et al., 2021), but there may be limitations in the accuracy of prediction. Therefore, it may be a simple and quick method to directly select the key soil physical and chemical properties closely related to soil buffering, filtering, and biochemical transformation functions to evaluate soil resilience and then combine soil resilience with the degree of soil heavy metal pollution for the safe utilization classification of land. Although this study only focuses on cultivated land, this method can also be used for other agricultural land or land without crops. Moreover, it can also be used as a reference for the identification of cultivated land reserve resources and the determination of the direction of soil improvement and restoration. We also acknowledge that the classification method of this study may be more effective for regional macro decision-making and can form a regional comparable basic classification. On this basis, further research is required to develop a more detailed classification and management system that takes into account different heavy metals, pollution sources, and crops.
Soil resilience essentially reflects the ecological function and sustainable performance of soil and should be an important condition for the sustainable intensification (SI) of agricultural production (Schiefer et al., 2015). The goal of SI is to increase agricultural production under sustainable conditions to meet the needs created by population growth. It is a possible solution to meet the growing food needs of future generations without damaging the environment (Garnett et al., 2013; Struik and Kuyper, 2017). The realization of SI should take into account both the productive and ecological functions of cultivated land. For high-risk areas of soil pollution, such as highly intensive vegetable bases, in addition to considering soil resilience, sustainability also needs to consider the degree of soil pollution. Therefore, the final classification results of this study can also be used as a judgment basis for SI in high-risk areas of soil pollution.
At present, China’s soil pollution control work is still relatively difficult and the treatment cost is relatively high (Liu et al., 2021; Qin et al., 2021). For cultivated land with a high clean production capacity, priority protection, long-term monitoring, and soil improvement should be taken as the management principles. This type of cultivated land can be included in the functional areas of grain production and the production protection areas of important agricultural products. The concentrated and contiguous cultivated land can be preferentially classified into permanent prime farmland, which should be strictly protected, and priority should be given to the construction of high-standard farmland. It is necessary to strictly control the emergence of new pollution sources around and actively implement measures to protect and improve soil resilience, such as the return of straw to the field, increasing the application of organic fertilizer, less tillage or no-tillage, grain and bean rotation, reduction of pesticides and fertilizers, and the reduction and recycling of agricultural film (Elahi et al., 2021b). For cultivated land with a low clean production capacity, strengthening early warning, safe utilization, and improvement and restoration should be the management principles. It is necessary to reduce the intervention of known pollution sources and implement the corresponding economic and feasible measures. In light of the different pollution risks and soil resilience, and with consideration for the main crop varieties and planting habits in the region, priority should be given to implementing low-cost agronomic control measures for safe utilization to prevent or reduce the entry of heavy metals into crops, especially the edible parts. Lime regulation, optimized fertilization, variety adjustment, water regulation, leaf regulation, and deep tillage are some of the feasible agronomic control methods. It is necessary to regularly carry out agricultural product quality monitoring, investigation, and evaluation, allowing for the timely optimization and adjustment of agronomic control measures. In addition, bioremediation-type measures that do not affect agricultural production and do not reduce the productive function of the soil can be selected. Physical and chemical treatment and remediation measures, such as the application of passivators and soil conditioners, can be used as supplementary measures. In addition, it is also necessary to strengthen the technical guidance and training for farmers and farmers’ cooperatives. When the condition of cultivated land makes it difficult to ensure that the quality of edible agricultural products is up to standard, specific agricultural production areas should be designated, with strict prohibition of the planting of edible agricultural products. If necessary, measures such as planting structure adjustment, fallow land, and restoring farmland to forest and grassland can be implemented.
5 Conclusion
We chose the agricultural areas around typical industrial and mining enterprises located in the economic belt of the Yangtze River Delta as the study area and cultivated soil as the research object. The results showed that the accumulation of heavy metals in the study area was significantly affected by human activities. The waste gas, wastewater, and waste discharged from the production and operation activities of industrial and mining enterprises had a significant contribution to the Cd and Hg pollution of the surrounding soil. The potential ecological risk of heavy metals in the cultivated soil around the industrial and mining enterprises was high. The soil resilience of cultivated land in the study area was generally not high. The strong restrictive indicators were mainly the clay content and CEC, and the medium restrictive indicators were mainly CaO, SOC, and pH. The differences in natural conditions such as the topography, hydrogeology, and soil-forming parent material in different regions led to differences in the restrictive factors. The safe utilization classification of regional cultivated land was carried out with respect to the soil potential ecological risk and soil resilience, and different management strategies were suggested. It is hoped that this study can provide new ideas for the safe utilization classification and management of land resources in high-risk areas of soil pollution, as well as provide a reference for SI.
Data availability statement
The raw data supporting the conclusions of this article will be made available by the authors, without undue reservation.
Author contributions
WS: conceptualization, methodology, software, and writing—original draft. HZ: data curation and writing—original draft. XL: conceptualization and investigation. HS: visualization. BN: software and visualization. XS: formal analysis. JL: writing—reviewing and editing.
Funding
This study was supported by the National Natural Science Foundation of China (41771324 and 41807004).
Acknowledgments
We would like to thank the editor and reviewers for their valuable suggestions, which helped to improve the quality of the article greatly. We thank LetPub (www.letpub.com) for its linguistic assistance during the preparation of this manuscript.
Conflict of interest
Author XS was employed by Wells Fargo.
The remaining authors declare that the research was conducted in the absence of any commercial or financial relationships that could be construed as a potential conflict of interest.
Publisher’s note
All claims expressed in this article are solely those of the authors and do not necessarily represent those of their affiliated organizations, or those of the publisher, the editors, and the reviewers. Any product that may be evaluated in this article, or claim that may be made by its manufacturer, is not guaranteed or endorsed by the publisher.
References
Blum, W. E. H., and Aguilar, S. A. (1994). “A concept of sustainability and resilience based on soil functions: The role of the ISSS in promoting sustainable land use,” in Soil Resilience and Sustainable Land Use: Proceedings of a Symposium Held in Budapest, 28 September to 2 October 1992, Including the Second Workshop On the Ecological Foundations of Sustainable Agriculture Wefsa Ii, 535–542.
Blum, W. (2005). Functions of soil for society and the environment. Rev. Environ. Sci. Biotechnol. 4, 75–79. doi:10.1007/s11157-005-2236-x
Blum, W. (2000). Soil resilience–the capacity of soil to react on stress. Boll. Della Soc. Ital. Della Sci. Del Suolo 49, 7–13.
Cao, H. C., Luan, Z. Q., Wang, J. D., and Zhang, X. L. (2009). Potential ecological risk of cadmium, lead and arsenic in agricultural black soil in Jilin Province, China. Stoch. Environ. Res. Risk Assess. 23, 57–64. doi:10.1007/s00477-007-0195-1
China State Council (2016). The action plan for soil pollution prevention and control. Beijing: People’s Publishing House., 2016–2031.
Damian, G., Szakacs, Z., Iepure, G., and Damian, F. (2019). Distribution of heavy metals in granulometric fractions and on soil profiles. Carpathian J. Earth Environ. Sci. 14, 343–351. doi:10.26471/cjees/2019/014/085
de Lima, M. W., Da Silveira Pereira, W. V., de Souza, E. S., Teixeira, R. A., Palheta, D. D. C., Freitas Faial, K. D. C., et al. (2022). Bioaccumulation and human health risks of potentially toxic elements in fish species from the southeastern Carajas Mineral Province, Brazil. Environ. Res. 204, 112024. doi:10.1016/j.envres.2021.112024
Doabi, S. A., Karami, M., Afyuni, M., and Yeganeh, M., 2018. Pollution and health risk assessment of heavy metals in agricultural soil, atmospheric dust and major food crops in Kermanshah province, Iran. Ecotoxicol. Environ. Saf. 163, 153–164. doi:10.1016/j.ecoenv.2018.07.057
Elahi, E., Khalid, Z., Tauni, M. Z., Zhang, H., and Lirong, X. (2021a). Extreme weather events risk to crop-production and the adaptation of innovative management strategies to mitigate the risk: A retrospective survey of rural Punjab. Pakistan: Technovation, 102255. doi:10.1016/j.technovation.2021.102255
Elahi, E., Khalid, Z., and Zhang, Z. (2022a). Understanding farmers’ intention and willingness to install renewable energy technology: A solution to reduce the environmental emissions of agriculture. Appl. Energy 309, 118459. doi:10.1016/j.apenergy.2021.118459
Elahi, E., Zhang, H., Lirong, X., Khalid, Z., and Xu, H. (2021b). Understanding cognitive and socio-psychological factors determining farmers’ intentions to use improved grassland: Implications of land use policy for sustainable pasture production. Land Use Policy 102, 105250. doi:10.1016/j.landusepol.2020.105250
Elahi, E., Zhang, Z., Khalid, Z., and Xu, H. (2022b). Application of an artificial neural network to optimise energy inputs: An energy-and cost-saving strategy for commercial poultry farms. Energy 244, 123169. doi:10.1016/j.energy.2022.123169
Foucault, Y., Durand, M. J., Tack, K., Schreck, E., Geret, F., Leveque, T., et al. (2013). Use of ecotoxicity test and ecoscores to improve the management of polluted soils: Case of a secondary lead smelter plant. J. Hazard. Mat. 246, 291–299. doi:10.1016/j.jhazmat.2012.12.042
Gao, X., Tian, J. P., Huo, Z., Wu, Y. B., and Li, C. X. (2021). Evaluation of redevelopment priority of abandoned industrial and mining land based on heavy metal pollution. Plos One 16, e0255509. doi:10.1371/journal.pone.0255509
Garnett, T., Appleby, M. C., Balmford, A., Bateman, I. J., Benton, T. G., Bloomer, P., et al. (2013). Sustainable intensification in agriculture: Premises and policies. Science 341, 33–34. doi:10.1126/science.1234485
Grant, C. N., Dennis, H. T., Antoine, J., Hoo-Fung, L. A., and Lalor, G. C. (2013). Agglomerative hierarchical clustering analysis of twenty-six rice samples analysed by instrumental neutron activation analysis and other techniques. J. Radioanal. Nucl. Chem. 297, 233–239. doi:10.1007/s10967-012-2379-5
Gu, Q., Yang, Z., Yu, T., Ji, J., Hou, Q., and Zhang, Q. (2019). Application of ecogeochemical prediction model to safely exploit seleniferous soil. Ecotoxicol. Environ. Saf. 177, 133–139. doi:10.1016/j.ecoenv.2019.03.084
Gu, Q., Yang, Z., Yu, T., Yang, Q., Hou, Q., and Zhang, Q. (2018). From soil to rice – A typical study of transfer and bioaccumulation of heavy metals in China. Acta Agric. Scand. Sect. B — Soil & Plant Sci. 68, 631–642. doi:10.1080/09064710.2018.1455218
Hu, M., Li, F., Liu, C., and Wu, W. (2015). The diversity and abundance of As(III) oxidizers on root iron plaque is critical for arsenic bioavailability to rice. Sci. Rep. 5, 13611. doi:10.1038/srep13611
Hakanson, L. (1980). An ecological risk index for aquatic pollution control.a sedimentological approach. Water Res. 14, 975–1001. doi:10.1016/0043-1354(80)90143-8
He, B., Yun, Z., Shi, J., and Jiang, G. (2013). Research progress of heavy metal pollution in China: Sources, analytical methods, status, and toxicity. Chin. Sci. Bull. 58, 134–140. doi:10.1007/s11434-012-5541-0
Hu, B., Zhou, Y., Jiang, Y., Ji, W., Fu, Z., Shao, S., et al. 2020. Spatio-temporal variation and source changes of potentially toxic elements in soil on a typical plain of the Yangtze River Delta, China (2002–2012). J. Environ. Manag. 271, 110943. doi:10.1016/j.jenvman.2020.110943
Iqbal, J., and Shah, M. H., 2011. Distribution, correlation and risk assessment of selected metals in urban soils from Islamabad, Pakistan. J. Hazard. Mater. 192, 887–898. doi:10.1016/j.jhazmat.2011.05.105
Ji, W., Yang, Z., Yu, T., Yang, Q., Wen, Y., and Wu, T. (2021). Potential ecological risk assessment of heavy metals in the Fe–Mn nodules in the karst area of Guangxi, southwest China. Bull. Environ. Contam. Toxicol. 106, 51–56. doi:10.1007/s00128-020-02837-6
Khan, S., Naushad, M., Lima, E., Zhang, S., Shaheen, S., and Rinklebe, J. (2021). Global soil pollution by toxic elements: Current status and future perspectives on the risk assessment and remediation strategies – a review. J. Hazard. Mater. 417, 126039. doi:10.1016/j.jhazmat.2021.126039
Kong, C., and Zhang, S. (2021). Security regional division of farmland soil heavy metal elements in north of the north China plain. Front. Environ. Sci. 9, 639460. doi:10.3389/fenvs.2021.639460
Li, C., Yang, Z., Yu, T., Hou, Q., Liu, X., Wang, J., et al. 2021. Study on safe usage of agricultural land in karst and non-karst areas based on soil Cd and prediction of Cd in rice: A case study of heng county, Guangxi. Ecotoxicol. Environ. Saf. 208, 111505. doi:10.1016/j.ecoenv.2020.111505
Li, T., Liu, Y., Lin, S., Liu, Y., and Xie, Y. (2019). Soil pollution management in China: A brief introduction. Sustainability 11, 556. doi:10.3390/su11030556
Li, Y., Kang, C., Chen, W., Ming, L., Zhang, S., and Guo, P. (2013). Thermodynamic characteristics and mechanisms of heavy metals adsorbed onto urban soil. Chem. Res. Chin. Univ. 29, 42–47. doi:10.1007/s40242-013-2200-1
Liu, P., Wu, K., Luo, M., Li, C., Zhu, P., Zhang, Q., et al. (2016). Evaluation of agricultural land soil heavy metal elements exceed standards and safe utilization zones. Trans. Chin. Soc. Agric. Eng. 32, 254–262. doi:10.11975/j.issn.1002-6819.2016.23.035
Liu, S., Wang, X., Guo, G., and Yan, Z. (2021). Status and environmental management of soil mercury pollution in China: A review. J. Environ. Manag. 277, 111442. doi:10.1016/j.jenvman.2020.111442
Ludwig, M., Wilmes, P., and Schrader, S. (2018). Measuring soil sustainability via soil resilience. Sci. Total Environ. 626, 1484–1493. doi:10.1016/j.scitotenv.2017.10.043
Mahar, A., Wang, P., Ali, A., Guo, Z., Awasthi, M. K., Lahori, A. H., et al. (2016). Impact of CaO, fly ash, sulfur and Na2S on the (im)mobilization and phytoavailability of Cd, Cu and Pb in contaminated soil. Ecotoxicol. Environ. Saf. 134, 116–123. doi:10.1016/j.ecoenv.2016.08.025
Mcbride, M. B. (2002). Cadmium uptake by crops estimated from soil total Cd and Ph. Soil Sci. 167, 62–67. doi:10.1097/00010694-200201000-00006
Ministry of Ecology and Environment of People's Republic of China (2018). Soil environmental quality - risk control standards for soil contamination of agricultural land (GB 15618–2018). Beijing: China Environmental Science Press.
Ministry of Natural Resources of the People's Republic of China (2016). Specification of land quality geochemical assessment (DZ/T 0295-2016). China: University of Geosciences Press.
Qiao, Q., Yang, X., Liu, L., Luo, Y., Qiu, G., Liu, C., et al. (2020). Electrochemical adsorption of cadmium and arsenic by natural Fe-Mn nodules. J. Hazard. Mater. 390, 122165. doi:10.1016/j.jhazmat.2020.122165
Qin, G., Niu, Z., Yu, J., Li, Z., Ma, J., and Xiang, P. (2021). Soil heavy metal pollution and food safety in China: Effects, sources and removing technology. Chemosphere 267, 129205. doi:10.1016/j.chemosphere.2020.129205
Ran, L., Altschul, E. B., Hedin, R. S., Nakles, D. V., and Dzombak, D. A. (2014). Sequestration enhancement of metals in soils by addition of iron oxides recovered from coal mine drainage sites. Soil Sediment Contam. Int. J. 23, 374–388. doi:10.1080/15320383.2014.831027
Schaeffer, A., Amelung, W., Hollert, H., Kaestner, M., Kandeler, E., Kruse, J., et al. (2016). The impact of chemical pollution on the resilience of soils under multiple stresses: A conceptual framework for future research. Sci. Total Environ. 568, 1076–1085. doi:10.1016/j.scitotenv.2016.06.161
Schiefer, J., Lair, G. J., and Blum, W. E. H. (2015). Indicators for the definition of land quality as a basis for the sustainable intensification of agricultural production. Int. Soil Water Conservation Res. 3, 42–49. doi:10.1016/j.iswcr.2015.03.003
Schiefer, J., Lair, G. J., and Blum, W. (2016). Potential and limits of land and soil for sustainable intensification of European agriculture. Agric. Ecosyst. Environ. 230, 283–293. doi:10.1016/j.agee.2016.06.021
Struik, P. C., and Kuyper, T. W. (2017). Sustainable intensification in agriculture: The richer shade of green. A review. Agron. Sustain. Dev. 37, 39. doi:10.1007/s13593-017-0445-7
Sun, Y., Zhou, Q., Xie, X., and Liu, R. (2010). Spatial, sources and risk assessment of heavy metal contamination of urban soils in typical regions of Shenyang, China. J. Hazard. Mater. 174, 455–462. doi:10.1016/j.jhazmat.2009.09.074
Tang, W., Pei, Y., Zheng, H., Zhao, Y., Shu, L., and Zhang, H., 2022. Twenty years of China's water pollution control: Experiences and challenges. Chemosphere 295, 133875. doi:10.1016/j.chemosphere.2022.133875
Usman, A. R. A., Kuzyakov, Y., and Stahr, K. (2004). Sorption, desorption, and immobilization of heavy metals by artificial soil. Stuttgart: University of Hohenhiem. PhD Dissertation.
Wang, C., Li, W., Yang, Z., Chen, Y., Shao, W., and Ji, J. (2015). An invisible soil acidification: Critical role of soil carbonate and its impact on heavy metal bioavailability. Sci. Rep. 5, 12735. doi:10.1038/srep12735
Wang, C., Yang, Z., Zhong, C., and Ji, J. (2016). Temporal–spatial variation and source apportionment of soil heavy metals in the representative river–alluviation depositional system. Environ. Pollut. 216, 18–26. doi:10.1016/j.envpol.2016.05.037
Wang, H., Li, W., Zhu, C., and Tang, X. (2021). Analysis of heavy metal pollution in cultivated land of different quality grades in Yangtze River Delta of China. Int. J. Environ. Res. Public Health 18, 9876. doi:10.3390/ijerph18189876
Wang, H., and Lu, S. (2011). Spatial distribution, source identification and affecting factors of heavy metals contamination in urban-suburban soils of Lishui city, China. Environ. Earth Sci. 64, 1921–1929. doi:10.1007/s12665-011-1005-0
Wang, M., Bai, Y., Chen, W., Markert, B., Peng, C., and Ouyang, Z., 2012. A GIS technology based potential eco-risk assessment of metals in urban soils in Beijing, China. Environ. Pollut. 161, 235–242. doi:10.1016/j.envpol.2011.09.030
Wang, Y., Yu, T., Yang, Z., Bo, H., Lin, Y., Yang, Q., et al. (2021). Zinc concentration prediction in rice grain using back-propagation neural network based on soil properties and safe utilization of paddy soil: A large-scale field study in Guangxi, China. Sci. Total Environ. 798, 149270. doi:10.1016/j.scitotenv.2021.149270
Wei, J., Li, H., and Liu, J. (2022). Heavy metal pollution in the soil around municipal solid waste incinerators and its health risks in China. Environ. Res. 203, 111871. doi:10.1016/j.envres.2021.111871
Wen, Y., Li, W., Yang, Z., Zhuo, X., Ji, J., Song, Y., et al. (2020). Evaluation of various approaches to predict cadmium bioavailability to rice grown in soils with high geochemical background in the karst region, Southwestern China. Environ. Pollut. 258, 113645. doi:10.1016/j.envpol.2019.113645
Wu, S., Peng, S., Zhang, X., Wu, D., Luo, W., Zhang, T., et al. (2015). Levels and health risk assessments of heavy metals in urban soils in Dongguan, China. J. Geochem. Explor. 148, 71–78. doi:10.1016/j.gexplo.2014.08.009
Xiao, R., Guo, D., Ali, A., Mi, S., Liu, T., Ren, C., et al. 2019. Accumulation, ecological-health risks assessment, and source apportionment of heavy metals in paddy soils: A case study in hanzhong, shaanxi, China. Environ. Pollut. 248, 349–357. doi:10.1016/j.envpol.2019.02.045
Yang, Q., Yang, Z., Zhang, Q., Liu, X., Zhuo, X., Wu, T., et al. (2021). Ecological risk assessment of Cd and other heavy metals in soil-rice system in the karst areas with high geochemical background of Guangxi, China. Sci. China Earth Sci. 64, 1126–1139. doi:10.1007/s11430-020-9763-0
Yang, Y., Li, C., Yang, Z., Yu, T., Jiang, H., Han, M., et al. (2021). Application of cadmium prediction models for rice and maize in the safe utilization of farmland associated with tin mining in Hezhou, Guangxi, China. Environ. Pollut. 285, 117202. doi:10.1016/j.envpol.2021.117202
Li, Y., Gou, X., Wang, G., Zhang, Q., Su, Q., and Xiao, G. (2008). Heavy metal contamination and source in arid agricultural soil in central Gansu Province, China. J. Environ. Sci. 20, 607–612. doi:10.1016/S1001-0742(08)62101-4
Yu, T., Hou, W., Hou, Q., Ma, W., Xia, X., Li, Y., et al. (2020). Safe utilization and zoning on natural selenium-rich land resources: A case study of the typical area in enshi county, China. Environ. Geochem. Health 42, 2803–2818. doi:10.1007/s10653-020-00519-0
Zeng, F., Ali, S., Zhang, H., Ouyang, Y., Qiu, B., Wu, F., et al. (2011). The influence of pH and organic matter content in paddy soil on heavy metal availability and their uptake by rice plants. Environ. Pollut. 159, 84–91. doi:10.1016/j.envpol.2010.09.019
Zhang, C., and Lalor, G. (2002). Multivariate relationships and spatial distribution of geochemical features of soils in Jamaica. Chem. Speciat. Bioavailab. 14, 57–65. doi:10.3184/095422902782775326
Zhang, C., Ying, G. E., Yao, H., Chen, X., and Minkun, H. U. (2012). Iron oxidation-reduction and its impacts on cadmium bioavailability in paddy soils: A review. Front. Environ. Sci. Eng. 6, 509–517. doi:10.1007/s11783-012-0394-y
Zhang, L., Zhu, G., Ge, X., Xu, G., and Guan, Y. (2018). Novel insights into heavy metal pollution of farmland based on reactive heavy metals (RHMs): Pollution characteristics, predictive models, and quantitative source apportionment. J. Hazard. Mater. 360, 32–42. doi:10.1016/j.jhazmat.2018.07.075
Zhao, F. J., Ma, Y. B., Zhu, Y. G., Tang, Z., and McGrath, S. P. (2015). Soil contamination in China: Current status and mitigation strategies. Environ. Sci. Technol. 49, 750–759. doi:10.1021/es5047099
Zhao, R., Li, J., Wu, K., and Kang, L. (2021). Cultivated land use zoning based on soil function evaluation from the perspective of black soil protection. Land 10, 605. doi:10.3390/land10060605
Keywords: soil heavy metal pollution, potential ecological risk index, soil resilience, safe utilization classification of cultivated land, sustainable intensification
Citation: Song W, Zhang H, Li X, Song H, Niu B, Shi X and Li J (2022) Safe utilization of cultivated land in high-risk areas of soil heavy metal pollution based on soil resilience. Front. Environ. Sci. 10:889069. doi: 10.3389/fenvs.2022.889069
Received: 04 March 2022; Accepted: 18 July 2022;
Published: 26 August 2022.
Edited by:
Chen Zeng, Huazhong Agricultural University, ChinaReviewed by:
Yiyun Chen, Wuhan University, ChinaEhsan Elahi, Shandong University of Technology, China
Copyright © 2022 Song, Zhang, Li, Song, Niu, Shi and Li. This is an open-access article distributed under the terms of the Creative Commons Attribution License (CC BY). The use, distribution or reproduction in other forums is permitted, provided the original author(s) and the copyright owner(s) are credited and that the original publication in this journal is cited, in accordance with accepted academic practice. No use, distribution or reproduction is permitted which does not comply with these terms.
*Correspondence: Beibei Niu, YmJud2h1QHNkYXUuZWR1LmNu; Junying Li, bGp5MzA2OUAxNjMuY29t
†These authors share first authorship