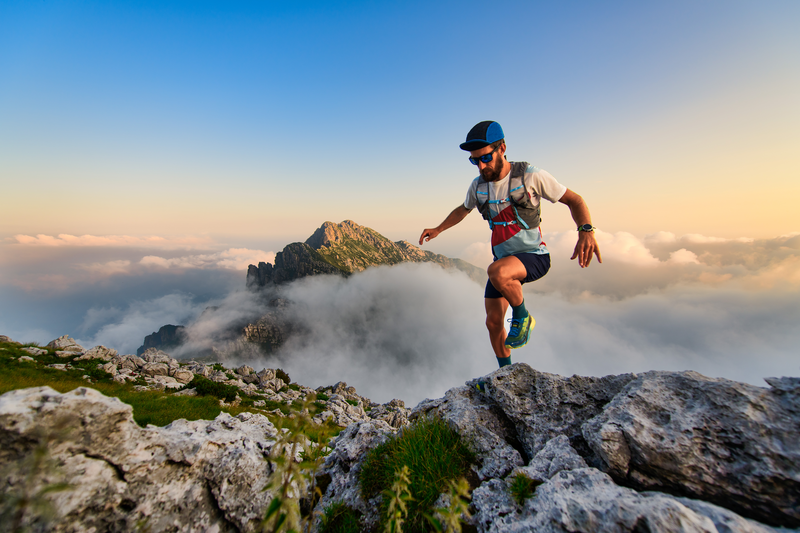
94% of researchers rate our articles as excellent or good
Learn more about the work of our research integrity team to safeguard the quality of each article we publish.
Find out more
ORIGINAL RESEARCH article
Front. Environ. Sci. , 25 August 2022
Sec. Toxicology, Pollution and the Environment
Volume 10 - 2022 | https://doi.org/10.3389/fenvs.2022.887837
This article is part of the Research Topic Novel Insights into Mercury Sources and Behavior in the Surface Earth Environment View all 5 articles
Mercury (Hg)-laden coal fly ash is an environmental concern when it is exposed to precipitation or surface/groundwater under natural conditions. In this study, fly ash samples collected from fifteen coal-fired power plants in Guizhou province of southwest China were subjected to examine the different Hg binding forms. The results showed that total Hg in fly ash from these CFPPs ranged from 30 to 870 ng/g. The percentage of different Hg binding forms in coal fly ash decreased in the following order: strong complexed form (64%–91%) > acid-soluble form (1%–25%) and sulfide form (3.4%–14.8%) > ion-exchangeable form (0.01%–8.1%), and water-soluble form (0.01%–4.4%). The low proportion of water-soluble and ion-exchangeable forms indicated that Hg was not easily removed under natural conditions. Furthermore, fly ash samples from three out of fifteen CFPPs were carried out the leaching experiments to disclose the leachability of Hg and the transmedia migration potential of this element. The results indicated pH dependence of Hg leachability, with more than 4-times fold higher Hg been leached out under acidic (pH < 5.5) and strong alkaline (pH > 13) conditions than under neutral and weakly alkaline conditions (pH = 7–12). In addition, Hg was leached out more in higher liquid/solid ratio than lower ratios. Nevertheless, Hg concentration in extract (<7 ng/L) of these three CFPPs under all conditions was well below the applicable regulation limits, and less than 0.11% of total Hg was leached out. This study demonstrated that Hg in the CFPP fly ashes was more stable under natural conditions when exposed to surface/groundwaters and had a negligible Hg leachability.
Mercury (Hg) is a global pollutant due to its toxicity, environmental persistence, atmospheric transport, and bioaccumulation characteristics (World Health Organization, 1991; UNEP, 2013; Huang et al., 2021). Since the Industrial Revolution, human activities like gold/silver extraction, nonferrous metal smelting, and coal combustion have significantly changed the global Hg cycle (Streets et al., 2011; Opiso et al., 2018, 2021), resulting in a significant increase in Hg concentration in different environmental media; for example, atmospheric Hg and surface ocean Hg are 3–5 times and 2 times higher than in the past, respectively, and surface soil Hg has increased by 20% (Driscoll et al., 2013). Since the outbreak of the Minamata disease in Japan in the 1950s, Hg has attracted much public attention (World Health Organization, 1991; UNEP, 2013).
Coal combustion has been an important atmospheric Hg source over the past half century (Streets et al., 2011), since during the coal combustion, nearly all Hg in coal will been released into flue gas, which creates approximately half of the global anthropogenic atmospheric Hg emissions during the early 2000s (UNEP, 2002). As the world’s largest coal consumer and highest number of coal-fired power generators, China has faced tremendous challenges in reducing its Hg emissions from coal combustion, especially from coal-fired power plants (CFPPs), which consume approximately 50% of its national usage. CFPPs in China have upgraded their air pollution control devices to create lower particulate, SO2, and NOx emissions over the past two decades (Wu et al., 2016), which has an obvious co-benefit of Hg emission reduction (Wang et al., 2010a). For example, through field investigations, Zheng et al. (2018) found the atmospheric Hg emissions from four ultra-low emission power plants were only 0.4%–14% of total Hg input, which were much lower than figures (64%–78%) in China in middle 1990s when no SO2 and NOx emission controls for CFPPs (Wang et al., 2000). In Guizhou province, Hg removal efficiency from different air pollution control devices for pulverised coal (PC) furnace power plants averaged 94%, and that from circulating fluidised bed (CFB) utility boilers was 98% in middle 2010s (Tang et al., 2016; Li et al., 2019). For CFB utility boilers, 97%–99% of Hg in the feed coal was ultimately removed into captured fly ash (Li et al., 2019), whereas for 11 of the pulverised CFPPs, 40% of the Hg in the feed coal ended up in the fly ash and 54% in desulfurisation gypsum in Guizhou province after the upgradation of SO2, NOx, and particulate emission control devices (Guizhou Environmental Monitoring Center Station and Institute of Geochemistry CAS, 2016).
Coal fly ash discharge are increasing, but under 50% of world production is utilised (Izquierdo and Querol, 2012). The annual generation capacity of coal-fired fly ash in the United States and India in 2015 was about 130 and 190 million tonnes, respectively (Yao et al., 2015). In 2019, fly ash yields from CFPPs in China were approximately 748 million (China Powder Network, 2021), and the utilisation rate of fly ash was ∼70% in recent years (NDRC, 2014). Unutilised fly ash is typically piled, where Hg might be released into the environment by leaching out with water or be re-emitted into the atmosphere. Therefore, the environmental behaviour of Hg in this kind of flue gas cleaning by-products of CFPPs cannot be ignored. However, there are few studies been carried out for the Hg secondary release from coal fly ash under nature conditions. In United States, Gustin and Ladwig (2004) found that Hg concentrations of coal fly ash extracts derived using United States Environmental Protection Agency Method 1312 were ≤14.4 ng/L. In Slovenia, a lake with coal fly ash dumping had resulted in high pH (10–12) and high concentrations of heavy metals (Kotnik et al., 2002). In China, Zhao et al. (2018) found Hg concentrations in extracts of four Chinese coal fly ash leached by deionised water were as high as 11-302 ng/L, while, Wang and Meng (2013) found the figures for other four CFPPs was only 4.11-13.26 ng/L. The results obtained by different researchers seems varied a great. As the fourth-largest coal-producing province in China (Bai et al., 2018), Guizhou discharges 35 million tonnes of fly ash every year, and its cumulative stock exceeds 150 million tonnes, covering an area of more than 1300 ha (Chen et al., 2012; Chen and Zhang, 2017). The fly ash utilisation rate (approximately 40%) of this region is much lower than the average for China (Chen et al., 2012). In addition, Guizhou province is located in the center of China’s karst landscape, which covers 62% of the province’s land area (Li, 2011). The abundant rainfall (ca. 1200 mm/year) and slight acidity of precipitation (Lv et al., 2017), and the low soil formation rate and high permeability of carbonate rocks make the local environment fragile (Jiang et al., 2014).
Therefore, studying the fate of Hg in fly ash when stockpiled in such a vulnerable environment is essential. Although Gong et al. (2010) found that the leaching of Zn from the fly ash yard of a CFPP in Guizhou province was more significant than Cd and Pb, Hg was not studied in this case. Only one report found that the Hg concentration in extracts of two (fine and coarse) coal fly ash samples from a CFPP in Guizhou was in the range of 8.12-13.24 ng/L when deionized water was used as the leaching reagent (Wang and Meng, 2013). To date, relevant research on Guizhou province is limited, scattered, and not systematic. The stockpiles, landfill, and utilisation (for example, as road base and agricultural use) of fly ash during the ash treatment process might involve water washing and leaching, which transfer unstable Hg from the solid to the liquid phase, and contaminate the surface/ground waters.
In the present study, we collected coal fly ash samples from 15 CFPPs representing approximately 40% of provincial coal-powered generators and covering different coal qualities, furnace types, and combinations of pollution control facilities in Guizhou. We then performed indoor leachability experimental research on Hg. The aims of the present study were to 1) explore the binding forms of Hg in the fly ash from the CFPPs and 2) determine the leaching characteristics of Hg in the fly ash under different situations, such as varying pH conditions and solid-liquid ratios. We then assessed the total amount of Hg lost during the leaching process and the potential environmental impacts of Hg from the fly ash after exposure to surface/groundwaters. The result would provide scientific basis for evaluating Hg cycling in coal fly ash under nature environment.
A total of 34 samples from 15 CFPPs in Guizhou province were subjected to the Hg binding forms experiment. All CFPPs were fed with local coal, which has much higher ash yield (range: 30%–46%, average 38%) and sulfur content (range 0.5%–3.4%, average 2.0%) than the national average (16.8% for ash yield and 1.1% for sulfur content, Li and Zhai, 1994). The fly ash samples were collected from the storage hopper of the air pollution control devices during a Hg atmospheric emission study programme (Tang et al., 2016; Li et al., 2019), and represented a mixed sample of fly ash from the plant. At each CFPP, fly ash samples were collected 3–6 times with a half-day interval between each sampling, and each sample had a minimum weight of 1 kg. Most of the CFPPs were distributed in central to western areas of the Guizhou province (Supplementary Figure S1), which are the main coal production areas in this province. Detailed information about the CFPPs and the samples identification is shown in Table 1.
TABLE 1. Detailed information about the CFPPs in Guizhou Province, China and the sample ID for coal fly ash samples.
Measuring the total Hg value in the fly ash samples involved aqua regia digestion in a water bath, the digest was then reduced with SnCl2, and the produced Hg0 vapour was preconcentrated in a gold trap and detected using cold vapour atomic fluorescence spectrometry (Brooks Rand MERX Model, United States). Each solid sample was measured at three times to obtain a mean value. Certified reference materials of coal fly ash (NIST SRM 1633c; GBW 08401) and soil (GSS-5) were measured to guarantee the analytical quality. The difference between measured Hg and the recommended values was less than 10%.
A modified five-step sequential extraction procedure was employed to identify the Hg binding forms in all fly ash samples (Table 2), as this method have been successively applied for Hg binding forms of different solid environmental matrix (Wei et al., 2011; Diao et al., 2018). The binding forms of Hg in the fly ash were classified as water-soluble (F1), ion-exchangeable (F2), acid-soluble (F3), strong complexed (F4), and sulfide (F5) forms.
TABLE 2. Five-step sequential chemical extraction procedure and attributed Hg forms in each step (modified from Diao et al., 2018; O'Connor et al., 2019).
A sample of 1.0 g of fly ash from each CFPP was used for the extraction procedure. A total of 20 ml of deionised water was added to a centrifuge tube and shaken on an end-to-end shaker at 300 rpm at room temperature. The extraction was then centrifuged at 4000 rpm for 30 min, the supernatant was removed with a pipette, and the extract solution was weighed and recorded. After each extraction step, the extract was centrifuged, filtered through a 0.45 µm nylon filter, and stored at 4°C before determination. The solid residue from each step was subjected to the next extraction step by adding the corresponding solvent. All supernatant samples were supplemented with hydrochloride and SnCl2 for Hg reduction, and the total Hg was quantified using cold vapour atomic fluorescence spectrometry (Brooks Rand MERX Model, United States). To check the accuracy of the sequential extraction, the sum of all of the extraction steps 1–5 (Table 2) for Hg was compared with that found using direct digestion with aqua regia. For two certified reference materials of coal fly ash (NIST 1633c) and soil (GSS-5), the agreement between the values obtained using these two methods was satisfactory, with recoveries of 85%–115%.
Fly ash samples from three CFPPs (CFPPs #1, #2, and #3) in central to western Guizhou were selected for the Hg leaching experiment (P1E1 and P1E2 from CFPP #1; P2F1 and P2F2 from CFPP #2; and P3E1 and P3E2 from CFPP #3). The three CFPPs have different geographical locations, boiler types, and pollution control devices, and the Hg concentration in the fly ash has a gradient (110–390 ng/g).
The leaching experiments followed the methods recommended by the United States Environmental Protection Agency (USEPA) for general industrial waste, namely, the Leaching Environmental Assessment Framework method (USEPA Method 1316, 2012). The specific procedure used is shown in Figure 1. The extraction procedures were conducted using 150 ml polypropylene bottles with a horizontal oscillator with an adjustable frequency. Milli-Q water (18.25 Ω) and different chemical reagent solutions were used as the extraction reagent. The membrane for filtering supernatant samples was 0.45 μm polypropylene. All extracts had hydrochloride and SnCl2 added for Hg reduction, and Hg was quantified using cold vapour atomic fluorescence spectrometry (Brooks Rand MERX Model, United States).
For the Hg liquid-solid partitioning experiment as a function of extract pH (USEPA Method 1313), 5 g of fly ash from three selected CFPPs was leached with Milli-Q water and pH adjusted to 2, 4, 5.5, 7, 8, 9, 10.5, 12, and 13, and the solid-liquid ratio was 1:10. The pH was adjusted using HNO3 (guaranteed reagent) and 0.1 N KOH. A pH of 5.5 means that the raw Milli-Q water did not have HNO3 or KOH added. The mixture was oscillated at 180 rpm for 24 h at room temperature (approximately 25°C). Then, the supernatant was filtered and 3% HCl was added and the sample was stored at 4°C before determination. The Hg leachability was assessed by the total Hg lost in the leachate, calculated by multiplying the Hg concentration in the leachate (ng/L) and the leachate volume (L). Triplicate samples were used and the average value is shown.
For the Hg liquid-solid partitioning as a function of the solid-liquid ratio (USEPA Method 1316, 2012), a weight of 40 g, 20 g, 10 g, 5 g, and 5 g of fly ash samples were added with Milli-Q water to reach solid-liquid ratios of 1:1, 1:2, 1:5, 1:10, and 1:20, respectively. Oscillation, filtration, and Hg determination procedures were the same as discussed above for USEPA Method 1313, and the experiment was conducted in triplicate.
The measured total Hg in fly ash from the present study is shown in Figure 2. A considerable variation was found in Hg concentration between CFPPs, for example, Hg concentration in CFPP #5 and CFPP #6 which using PC was 833 ng/g and 44 ng/g, respectively, with the difference nearly 20 times. Hg concentration in fly ash of three CFB power plants ranged from 158 to 870 ng/g (Figure 2).
Hg concentration of fly ash in the present study was close to most previous studies for CFB plants (Table 3; Duan et al., 2008; Lopez-Anton et al., 2011a). However, Hg in PC fly ash from the present study was much higher than in a previous study in China (Wang et al., 2010b). This difference might be caused by the variation in the Hg concentration in the feed coal (Goodarzi, 2005), flue gas components (such as SO2 concentration) that might inhibit Hg absorption (Kellie et al., 2005), fly ash properties (e.g., unburnt carbon concentration and the existence of other metal oxides) that might enhance Hg absorption (Gibb et al., 2000; Zhao et al., 2019), and the types of dust removal devices (electrostatic precipitator (ESP) or fabric filter (FF)) (Pavlish et al., 2003). In addition, the relative higher ash yield (38%–42%) in CFPP #5 and #10 led to much higher Hg concentration in fly ash samples (655–833 ng/kg) and higher Hg removal efficiency (80%–85%) by dust collectors than the other PC boilers, e.g., the average Hg removal efficiency by dust collectors for other PC boilers was only 32% (Guizhou Environmental Monitoring Center Station and Institute of Geochemistry CAS, 2016). In addition, the X-ray fluorescence spectroscopy (XRF) results of the fly ash samples are shown in Supplementary Table S1, it indicated that the main components of fly ash are SiO2, Al2O3 and Fe2O3 both for PC and CFB boilers, while, CaO may be a main component of fly ash for CFB plant (such as CFPP#4) due to CaO injection into the boiler.
Figure 3 presents the results of Hg forms in the fly ash samples. The proportion of the five Hg binding forms varied in different fly ash samples. Generally, Hg was in the following order: strong complexed form (F4) > acid-soluble form (F3) and sulfide form (F5) > ion-exchangeable form (F2) and water-soluble form (F1).
Detailed information regarding the Hg binding forms in each fly ash sample is shown in Supplementary Table S2 in the Supporting Information. F4 made up the largest proportion with 64%–91%, followed by F3 and F5, accounting for 1%–25% and 3–15%, respectively, and F1 (<4.5%) and F2 (<8%) occupied a smaller proportion overall. There was a large difference in the proportions between different samples. For example, the maximum proportion of F1 was 4.5% in P11E1, whereas the minimum was <0.01% in P2F2 (Supplementary Table S2). Similarly, there was a considerable gap between the maximum and minimum values of the other forms.
Hg concentration in extracts under different pH conditions are shown in Figure 4. It revealed that Hg in fly ash was more easily been released under acidic conditions (pH = 2–5.5) and strong alkaline conditions (pH = 13) than under neutral and weakly alkaline conditions (pH = 7–12), the difference of mean Hg concentrations in extracts between pH = 2–5.5 plus 13 relative to pH = 7–12 was significantly by the one-way variance (ANOVA) analysis (Supplementary Table S3). This indicated that Hg leaching from coal fly ash was pH dependent, which was different from other’s report (Izquierdo and Querol, 2012). Nevertheless, total Hg concentrations in extracts of all samples from these three CFPPs under a pH range of 2–13 were less than 5 ng/L (Supplementary Table S3). The release fraction of Hg from the fly ash under varying pH conditions was extremely low, ranging from 0.000% to 0.036% (Supplementary Table S4).
FIGURE 4. Leaching characteristics of Hg from coal fly ash with varying pH and a constant solid-liquid ratio of 1:10.
During the actual disposal process, the leaching of fly ash can occur under different solid-liquid ratios; therefore, the effect of the solid-liquid ratio on the leaching of Hg was investigated in the present study. Hg release fraction was calculated based on the Hg mass that been leached out relative to the total Hg in fly ash. The results are shown in Figure 5.
The Hg release fraction from coal fly ash of CFPP #1 was higher than that of CFPP #2 and CFPP #3, and the difference was larger as the solid-liquid ratio decreased (such as solid-liquid ratio 1:10 and 1:20) (Figure 5). However, all three CFPPs showed a similar pattern of variation, with the Hg release fraction increasing with a decreasing solid-liquid ratio (Figure 5). Even so, the release fraction of Hg from fly ash remained considerably low (0.000%–0.110%) for different solid-liquid ratio conditions, especially at solid-liquid ratios of 1:1, 1:2, and 1:5 (<0.005% in all CFPPs; Figure 5, Supplementary Table S4).
These results were consistent when comparing the Hg concentrations in F1 in the Hg binding forms experiment. Specifically, the aqueous dissolved Hg concentrations with sequential extraction experiment for CFPP #1, #2, and #3 were 8.0, 1.2, and 0.5 ng/L, respectively, when extracted with Milli-Q water at a solid-liquid ratio of 1:20. The corresponding leaching experiments with Milli-Q water at a solid-liquid ratio of 1:20 with USEPA method 1316 resulted in fly ash leachate Hg concentrations of 6.4, 1.0, and 0.7 ng/L for CFPP #1, #2 and #3, respectively. Therefore, these results were comparable and reliable. The details of Hg concentrations in the extract and the loss rate of each sample are provided in Supplementary Tables S3, S4 in the Supporting Information, respectively.
The ultra-high percentage of F4 (extracted by 8 M HNO3) in the present study was comparable to the results obtained by Wei et al. (2011), who found that Hg in coal fly ashes from Tianjin city, China, predominantly (85%–91%) existed in the form that extracted with 12 M HNO3. Using the temperature-programmed decomposition technique, Lopez-Anton et al. (2011b) found that HgCl2 and HgSO4 were the primary forms of Hg in fly ashes for CFB power plants and HgCl2 and Hg0 for PC power plants. Zheng et al. (2018) showed that Hg in fly ash was mainly in HgCl2 (41%–47%) and HgS (43%–47%) using the same method. While, HgCl2 has much higher solubility in water (up to 73 g/L at 25°C, O'Connor et al., 2019) than other Hg compounds, hence, the low proportion of water soluble forms (F1) of this study suggests HgCl2 might not be the main Hg forms in coal fly ash. According to the results indicated by the sequential extraction experiment in the present study (Table 2), the possible forms of F4 were either Hg exist in fly ash mineral lattice, or in Hg (0), or in Hg2Cl2. A recent study probed by mild 2-mercaptoethanol extraction and HPLC-ICP-MS analysis revealed the widely existence of Hg(I) or Hg2Cl2 in coal fly ash (Wang et al., 2020). There has obvious difference in the primary Hg forms obtained by these two methods (sequential extraction and temperature-programmed decomposition technique), one possible reason might be a portion of HgCl2 in the fly ash been reduced to Hg2Cl2 under acidic conditions (pH < 5) during sequential extraction (Wang et al., 2022). Hence, both the natural occurrence of Hg2Cl2 in fly ash and the secondary formed Hg2Cl2 might account for the large portion of F4 form extracted by HNO3. The rapid release of Hg from coal fly ash has a specific correlation with water-soluble Hg in the environment (Sun et al., 2014) because Hg in this form (F1) was labile and ready to be taken up by plants or animals with high environmental risks. The extremely low percentage of F1 in the present study reflected the low release potential of fly ash for Hg. Hg in F3 can be released into the environment under specific conditions, and should be regarded as dangerous because it can be released under acidic conditions and is sensitive to pH. Hg in F3 form in fly ash occupied a considerable proportion (up to 25%, second only to F4), creating a substantial environmental risk (Figure 3). For F5 that exist in sulfide form (such as HgS), the proportion of this form in total Hg was low (3%–15%) and considered to be insoluble (O'Connor et al., 2019); therefore, it was difficult for this form to affect the environment under natural conditions.
The leaching experiments indicated only a small fraction of Hg was leached out, which confirmed previous researches. For example, Wang and Meng (2013) conducted ultrapure water leaching experiments on fly ash samples from several regions in China and showed extract Hg concentrations of 4–13 ng/L and fly ash Hg release fraction of 0.02%–0.2%. Our results indicated a much lower rate of Hg leaching (extract Hg concentration of <6.4 ng/L and Hg release fractions of less than 0.11%; Figures 4, 5, Supplementary Table S3, S4).
pH and solid-liquid ratio affected the leaching fraction of Hg from fly ash. Hg concentration in extracts increased sharply as pH decreased (acidification) (Figure 3). When pH decreases, the fine particulate matter in the ash dissolves, whereas the inorganic component reacts with the acid, resulting in the release of Hg from fly ash. In contrast, Hg concentration in extract was maintained at a very low level in a weak alkaline environment (Figure 3) because Hg in fly ash reacted with OH− in extract to form Hg(OH)+, which is very easily adsorbed on the solid surface and does not release Hg (Mac Naughton and James, 1974; Newton et al., 1976; Kinniburgh and Jackson, 1978). Under strong alkaline conditions (pH = 13), Hg concentration in extract increased sharply (Figure 3), due to the formation of Hg(OH)2 under strong alkaline conditions, which had a smaller adsorption potential than Hg(OH)+, leading to more Hg being leached (Bonnissel-Gissinger et al., 1999; Sarkar et al., 1999).
The phenomenon that the release fraction of fly ash Hg increased with decreasing solid-liquid ratio resulted from the dissolution effect. The release of fly ash Hg was low due to the limited amount of dissolved Hg in F1 and F2 (Figure 3). As the solid-liquid ratio decreased, the dissolved fraction of fly ash increased.
According to the United States federal government, the maximum Hg concentration in drinking water should not be higher than 2000 ng/L and the USEPA state that Hg concentration in water from water sources should not be higher than 1400 ng/L (Lopez-Anton et al., 2010). China’s implementation of the “Sanitation Standards for Drinking Water” stated that Hg concentration in drinking water should not be higher than 1000 ng/L (GB-5749 2006, 2006). Regardless of changes to pH and the solid-liquid ratio, Hg concentration of fly ash extract in the present study (<6.5 ng/L; Supplementary Table S3) was much lower than the drinking water standard. Even in pH 2, the leachate Hg concentration was approximately 3 ng/L (Figure 3). The acid rain pH in some areas of Guizhou was approximately 3.8 in recent years (Yan et al., 2020), in such extreme acid rain environments, Hg concentration in fly ash extract was well below the applicable water standard and would not cause a Hg hazard to the environment.
Our research showed that the leaching effect only accounted for a small percentage of Hg in fly ash being washed out into the natural environment, and Hg concentration in extract was far below the applicable policy requirements. China is a large coal-consuming country, and the annual fly ash production reached 748 million tonnes in 2019. Approximately 200 million tonnes (or 30%) of the fly ash goes through the pile storage process. Based on the data of the average total Hg in fly ash (ca. 300 ng/g), and the average Hg loss rate (0.02%) under real conditions (pH = 4–5.5; solid-liquid ratio 1:10–1:20) (Supplementary Table S4) of the present study, a rough estimation of the amount of Hg been released into the natural environment from piled CFPPs fly ash in China was 0.013 tonnes per year. This is tiny compared to the Hg input by wet deposition in China (210 tonnes per year, Wang et al., 2018); therefore, the amount of Hg released from fly ash leaching can be ignored. The present study showed that only under highly acidic (pH < 5.5) or alkaline (pH > 13) conditions could Hg in fly ash be weakly released. However, the released Hg concentration (1.74–4.22 ng/L; Supplementary Table S3) was far below the guideline in surface and groundwater in China (1000 ng/L) and only slightly above the Hg levels in natural surface water bodies in Guizhou (<1.6 ng/L, Yao et al., 2011) and lower than local rainfall Hg levels (4–12 ng/L, Fu et al., 2016). Therefore, the loss of Hg from fly ash through leaching under natural conditions is negligible.
In the present study, we explored the binding forms and leaching characteristics of Hg in coal fly ash from southwest China. Although Hg in fly ash exists mainly in the F4 form that extracted by HNO3, the proportion of quickly released Hg in F1 (0–4.45%) and F2 (0.01–8.08%) forms was very small. The leaching experiments with different pH and solid-liquid ratios for selected samples indicated that less than 0.110% was leached out, and Hg concentration in extracts under all conditions was well below the applicable policy limits. Therefore, the present study demonstrated that the leaching of Hg in fly ash under natural conditions was negligible. Although the direct emissions of Hg from stack flue gas of CFPPs in China have been dramatically reduced in recent decades, and Hg leaching from the fly ash under natural conditions is minimal, the cross-media emissions of Hg from other fly ash utilisation processes, such as brick-making that involves heating processes, require further investigation.
The original contributions presented in the study are included in the article/Supplementary Material, further inquiries can be directed to the corresponding author.
ZL involved in the conception of the study; ZL, YH and GW performed the field investigation; YH, GW were involved in the experimental analysis; JL, BZ and ZX provided the resources; JL and ZL supervised this work; YH wrote the main manuscript; JL, ZL, GS and QW, edited and revised the manuscript. All authors have read and approved the final manuscript.
This work is financially supported by the Natural Science Foundation of China (No. 41967044); Talent Base for Environmental Protection and Mountain Agricultural in Chishui River Basin; Science and Technology Talent Project of Guizhou Provincial Department of Education (No. Qian-Jiao-He KY Zi [2019]057).
The authors declare that the research was conducted in the absence of any commercial or financial relationships that could be construed as a potential conflict of interest.
All claims expressed in this article are solely those of the authors and do not necessarily represent those of their affiliated organizations, or those of the publisher, the editors and the reviewers. Any product that may be evaluated in this article, or claim that may be made by its manufacturer, is not guaranteed or endorsed by the publisher.
The Supplementary Material for this article can be found online at: https://www.frontiersin.org/articles/10.3389/fenvs.2022.887837/full#supplementary-material
Bai, X., Ding, H., Lian, J., Ma, D., Yang, X., Sun, N., et al. (2018). Coal production in China: past, present, and future projections. Int. Geol. Rev. 60 (5-6), 535–547. 535–547. doi:10.1080/00206814.2017.1301226
Bonnissel-Gissinger, P., Alnot, M., Lickes, J. P., Ehrhardt, J. J., and Behra, P. (1999). Modeling the adsorption of mercury (II) on (Hydr)oxides II: α-FeOOH (goethite) and amorphous silica. J. Colloid Interface Sci. 215 (2), 313–322. doi:10.1006/jcis.1999.6263
Chen, C. L., Fang, K. H., Zhao, Z. H., and Ye, W. (2012). Research on the quality and comprehensive utilization of fly ash in Guizhou Province. Compr. Util. Fly Ash 4, 22–26. (In Chinese).
Chen, H. F., and Zhang, J. G. (2017). Study on comprehensive utilization of solid waste and countermeasures for thermal power enterprises in Guizhou region. Guizhou Electr. Power Technol. 20 (7), 84–86. (In Chinese). doi:10.19317/j.cnki.1008-083x.2017.07.026
China Powder Network (CPN) (2021). From industrial solid waste to "urban mineral" fly ash diversified treatment plan, do you know everything? (In Chinese Available at: https://news.cnpowder.com.cn/60017.html (Accessed on Feb 25, 2022).
Diao, X., Yuan, C. G., Wu, J. J., Gui, B., and Zhang, C. (2018). Mercury release and fraction transformation during desulfurization gypsum aging process (UV irradiation). Fuel 217, 522–528. doi:10.1016/j.fuel.2017.12.120
Driscoll, C. T., Mason, R. P., Hing Man, C., Jacob, D. J., and Nicola, P. (2013). Mercury as a global pollutant: Sources, pathways, and effects. Environ. Sci. Technol. 47, 4967–4983. doi:10.1021/es305071v
Duan, Y. F., Jiang, Y. M., Yang, L. G., and Wang, Y. J. (2008). Experimental study on mercury emission and adsorption in circulating fluidized bed boiler. Proc. CSEE 28 (32), 1–5. (In Chinese with English abstract).
Fu, X., Yang, X., Lang, X., Zhou, J., Zhang, H., Yu, B., et al. (2016). Atmospheric wet and litterfall mercury deposition at urban and rural sites in China. Atmos. Chem. Phys. 16, 11547–11562. doi:10.5194/acp-16-11547-2016
GB 5749-2006 (2006). Sanitary standard for drinking water. Beijing: Environmental Protection Agency of China. (In Chinese).
Gibb, W. H., Clarke, F., and Meht, A. K. (2000). The fate of coal mercury during combustion. Fuel Process. Technol. 65–66, 365–377. doi:10.1016/s0378-3820(99)00104-6
Gong, X., Wu, T., Qiao, Y., and Xu, M. H. (2010). In situ leaching of trace elements in a coal ash dump and time dependence laboratory evaluation. Energy fuels. 24, 84–90. doi:10.1021/ef9005115
Goodarzi, F. (2005). Petrology of subbituminous feed coal as a guide to the capture of mercury by fly ash-influence of depositional environment. Int. J. Coal Geol. 61, 1–12. doi:10.1016/j.coal.2004.06.001
Guizhou Environmental Monitoring Center Station (GEMCS) and Institute of Geochemistry CAS (IGCAS) (2016). Study on the characteristics of atmospheric mercury emission from coal-fired power plants and cement plants in Guizhou Province. A final programme report. Guiyang, China. (in Chinese).
Gustin, M. S., and Ladwig, K. (2004). An assessment of the significance of mercury release from coal fly ash. J. Air & Waste Manag. Assoc. 54 (3), 320–330. doi:10.1080/10473289.2004.10470900
Huang, X., Yu, H., Zhao, X., Guo, X., Ye, Y., and Xu, Z. (2021). Spatial variation in cadmium and mercury and factors influencing their potential ecological risks in farmland soil in Poyang Lake Plain, China. Front. Environ. Sci. 9, 641497. doi:10.3389/fenvs.2021.641497
Izquierdo, M., and Querol, X. (2012). Leaching behaviour of elements from coal combustion fly ash: An overview. Int. J. Coal Geol. 94, 54–66. doi:10.1016/j.coal.2011.10.006
Jiang, Z., Lian, Y., and Qin, X. (2014). Rocky desertification in Southwest China: impacts, causes, and restoration. Earth-Science Rev. 132, 1–12. doi:10.1016/j.earscirev.2014.01.005
Kellie, S., Cao, Y., Duan, Y., Li, L., Chu, P., Mehta, A., et al. (2005). Factors affecting mercury speciation in a 100-MW coal-fired boiler with low-NOx burners. Energy fuels. 19 (3), 800–806. doi:10.1021/ef049769d
Kinniburgh, D. G., and Jackson, M. L. (1978). Adsorption of mercury(II) by iron hydrous oxide gel. Soil Sci. Soc. Am. J. 42, 45–47. doi:10.2136/sssaj1978.03615995004200010010x
Kotnik, J., Horvat, M., Fajon, V., and Logar, M. (2002). Mercury in small freshwater lakes: A case study: Lake velenje, Slovenia. Water Air Soil Pollut., 7–337. doi:10.1023/A:1014192906185
Li, W. H., and Zhai, J. (1994). Ash, sulfur and calorific value of Chinese thermal coal. Coal Convers. 1, 15–25. (In Chinese).
Li, X. Y., Li, Z. G., Fu, C. C., Tang, L., Chen, J., Wu, T. T., et al. (2019). Fate of mercury in two CFB utility boilers with different fueled coals and air pollution control devices. Fuel 251, 651–659. doi:10.1016/j.fuel.2019.04.071
López-Antón, M. A., Dḯaz-SomoanoM, DiazL., Martḯnez-Tarazona, M. R., and Martinez-Tarazona, M. R. (2011a). Avoiding mercury emissions by combustion in a Spanish circulating fluidized-bed combustion (CFBC) plant. Energy fuels. 25, 3002–3008. doi:10.1021/ef200572x
Lopez-Anton, M. A., Perry, R., Abad-Valle, P., Díaz-Somoano, M., Martínez-Tarazona, M. R., and Maroto-Valer, M. M. (2011b). Speciation of mercury in fly ashes by temperature programmed decomposition. Fuel Process. Technol. 92, 707–711. doi:10.1016/j.fuproc.2010.12.002
Lopez-Anton, M. A., Yuan, Y., Perry, R., and Maroto-Valer, M. M. (2010). Analysis of mercury species present during coal combustion by thermal desorption. Fuel 89 (3), 629–634. doi:10.1016/j.fuel.2009.08.034
Lv, P., Ha, G., and Wu, Q. (2017). Chemical characteristics of rainwater in karst rural areas, Guizhou Province, Southwest China. Acta Geochim. 36 (3), 572–576. doi:10.1007/s11631-017-0238-3
Mac Naughton, M. G., and James, R. O. (1974). Adsorption of aqueous mercury (II) complexes at the oxide/water interface. J. Colloid Interface Sci. 47 (2), 431–440. doi:10.1016/0021-9797(74)90275-6
National Development, and Reform Commission (NDRC) (2014). Annual report on comprehensive utilization of Resources in China. (In Chinese) Available at: https://www.ndrc.gov.cn/xwdt/xwfb/201410/W020190905399746121257.pdf (Accessed on Jan 10, 2022).
Newton, D. W., Ellis, J. R., and Paulsen, G. M. (1976). Effect of pH and complex formation on mercury (II) adsorption by bentonite. J. Environ. Qual. 5 (3), 251–254. doi:10.2134/jeq1976.00472425000500030007x
O'Connor, D., Hou, D., Ok, Y. S., Mulder, J., Duan, L., Wu, Q., et al. (2019). Mercury speciation, transformation, and transportation in soils, atmospheric flux, and implications for risk management: A critical review. Environ. Int. 125, 747–761. doi:10.1016/j.envint.2019.03.019
Opiso, E. M., Aseneiro, J. P. J., Banda, M. H. T., and Tabelin, C. B. (2018). Solid-phase partitioning of mercury in artisanal gold mine tailings from selected key areas in Mindanao, Philippines, and its implications for mercury detoxification. Waste Manag. Res. 36 (3), 269–276. doi:10.1177/0734242x17753534
Opiso, E. M., Tabelin, C. B., Maestre, C. V., Aseneiro, J. P. J., Park, I., and Villacorte-Tabelin, M. (2021). Synthesis and characterization of coal fly ash and palm oil fuel ash modified artisanal and small-scale gold mine (ASGM) tailings based geopolymer using sugar mill lime sludge as Ca-based activator. Heliyon 7 (4), e06654. doi:10.1016/j.heliyon.2021.e06654
Pavlish, J. H., Sondreal, E. A., Mann, M. D., Olson, E. S., Galbreath, K. C., Laudal, D. L., et al. (2003). Status review of mercury control options for coal-fired power plants. Fuel Process. Technol. 82, 89–165. doi:10.1016/s0378-3820(03)00059-6
Sarkar, D., Essington, M. E., and Misra, K. C. (1999). Adsorption of mercury(II) by variable charge surfaces of quartz and gibbsite. Soil Sci. Soc. Am. J. 63 (6), 1626–1636. doi:10.2136/sssaj1999.6361626x
Streets, D. G., Devane, M. K., Lu, Z., Bond, T. C., Sunderland, E. M., and Jacob, D. J. (2011). All-time releases of mercury to the atmosphere from human activities. Environ. Sci. Technol. 45 (24), 10485–10491. doi:10.1021/es202765m
Sun, M. Y., Hou, J. A., Cheng, G. H., Baig, S. A., Tan, L. S., and Xu, X. H. (2014). The relationship between speciation and release ability of mercury in flue gas desulfurization (FGD) gypsum. Fuel 125, 66–72. doi:10.1016/j.fuel.2014.02.012
Tang, L., Liu, H. Y., Feng, X. B., Li, Z. G., Fu, C. C., Wang, H., et al. (2016). Mercury emissions from a coal-fired power plant burning high ash concentration anthracite coal. Chin. J. Ecol. 35 (5), 1351–1357. (In Chinese with English abstract). doi:10.13292/j.1000-4890.201605.015
United Nations Environment Programme (UNEP), (2013). Minamata convention on mercury. Geneva, Switzerland: UNEP.
U.S. Environmental Protection Agency U.S. EPA (2012). USEPA Method 1313. Liquid-solid partitioning as a function of extract pH using a parallel batch extraction procedure. Available at: https://www.epa.gov/hw-sw846/sw-846-test-method-1313-liquid-solid-partitioning-function-extract-ph-using-parallel-batch (Accessed February 20, 2022).
USEPA Method 1316 (2012). Liquid-solid partitioning as a function of liquid-to-solid ratio in solid materials using a parallel batch procedure. Available at: https://www.epa.gov/hw-sw846/sw-846-test-method-1316-liquid-solid-partitioning-function-liquid-solid-ratio-solid (Accessed February 20, 2022).
Wang, Q., Li, Z., Zeng, B., and Wang, D. 2022. The influence mechanisms of wet flue gas desulfurization system conditions on mercury species in gypsum. Zunyi, China. in preparation.
Wang, Q., Shen, W., and Ma, Z. (2000). Estimation of mercury emission from coal combustion in China. Environ. Sci. Technol. 34, 2711–2713. doi:10.1021/es990774j
Wang, S., and Meng, Y. (2013). Mercury leaching potential in fly ash. J. Tsinghua Univ. Sci. Technol. (5), 673–678. (In Chinese with English abstract). doi:10.16511/j.cnki.qhdxxb.2013.05.011
Wang, S. X., Zhang, L., Li, G. H., Wu, Y., Hao, J. M., Pirrone, N., et al. (2010b). Mercury emission and speciation of coal-fired power plants in China. Atmos. Chem. Phys. 10, 1183–1192. doi:10.5194/acp-10-1183-2010
Wang, S., Zhang, L., Wu, Y., Ancora, M. P., Zhao, Y., and Hao, J. (2010a). Synergistic mercury removal by conventional pollutant control strategies for coal-fired power plants in China. J. Air Waste Manag. Assoc. 60, 722–730. doi:10.3155/1047-3289.60.6.722
Wang, X., Lin, C.-J., Feng, X., Yuan, W., Fu, X., Zhang, H., et al. (2018). Assessment of regional mercury deposition and emission outflow in Mainland China. J. Geophys. Res. Atmos. 123, 9868–9890. doi:10.1029/2018jd028350
Wang, Y., Liu, G., Li, Y., Liu, Y., Guo, Y., Shi, J., et al. (2020). Occurrence of mercurous [Hg(I)] species in environmental solid matrices as probed by mild 2-mercaptoethanol extraction and HPLC-ICP-MS analysis. Environ. Sci. Technol. Lett. 7, 482–488. doi:10.1021/acs.estlett.0c00329
Wei, Z., Wu, G., Su, R., Li, C., and Liang, P. (2011). Mobility and contamination assessment of mercury in coal fly ash, atmospheric deposition, and soil collected from Tianjin, China. Environ. Toxicol. Chem. 30 (9), 1997–2003. doi:10.1002/etc.605
World Health Organization (Who), (1991). Environmental health criteria. Inorganic mercury. Geneva: World Health Organization.
Wu, Q., Wang, S., Li, G., Liang, S., Lin, C. J., Wang, Y., et al. (2016). Temporal trend and spatial distribution of speciated atmospheric mercury emissions in China during 1978–2014. Environ. Sci. Technol. 50 (24), 13428–13435. doi:10.1021/acs.est.6b04308
Yan, Z., Han, X., Lang, Y., Guo, Q., and Li, S. (2020). The abatement of acid rain in Guizhou province, southwestern China: Implication from sulfur and oxygen isotopes. Environ. Pollut. 267, 115444. doi:10.1016/j.envpol.2020.115444
Yao, H., Feng, X., Guo, Y., Yan, H., Fu, X., Li, Z., et al. (2011). Mercury and methylmercury concentrations in two newly constructed reservoirs in the Wujiang River, Guizhou, China. Environ. Toxicol. Chem. 30 (3), 530–537. doi:10.1002/etc.413
Yao, Z. T., Ji, X. S., Sarker, P. K., Tang, J. H., Ge, L. Q., Xia, M. S., et al. (2015). A comprehensive review on the applications of coal fly ash. Earth-Science Rev. 141, 105–121. doi:10.1016/j.earscirev.2014.11.016
Zhao, S., Duan, Y., Lu, J., Gupta, R., Pudasainee, D., Liu, S., et al. (2018). Chemical speciation and leaching characteristics of hazardous trace elements in coal and fly ash from coal-fired power plants. Fuel 232, 463–469. doi:10.1016/j.fuel.2018.05.135
Zhao, S., Pudasainee, D., Duan, Y., Gupta, R., Liu, M., and Lu, J. (2019). A review on mercury in coal combustion process: Content and occurrence forms in coal, transformation, sampling methods, emission and control technologies. Prog. Energy Combust. Sci. 73, 26–64. doi:10.1016/j.pecs.2019.02.001
Keywords: mercury, coal fly ash, leachability, sequential extraction, influencing factors
Citation: Huang Y, Liu J, Wang G, Wang Q, Zeng B, Xiao Z, Sun G and Li Z (2022) Leachability of mercury in coal fly ash from coal-fired power plants in southwest China. Front. Environ. Sci. 10:887837. doi: 10.3389/fenvs.2022.887837
Received: 02 March 2022; Accepted: 01 August 2022;
Published: 25 August 2022.
Edited by:
Larissa Schneider, Australian National University, AustraliaReviewed by:
Meihua Deng, Zhejiang Academy of Agricultural Sciences, ChinaCopyright © 2022 Huang, Liu, Wang, Wang, Zeng, Xiao, Sun and Li. This is an open-access article distributed under the terms of the Creative Commons Attribution License (CC BY). The use, distribution or reproduction in other forums is permitted, provided the original author(s) and the copyright owner(s) are credited and that the original publication in this journal is cited, in accordance with accepted academic practice. No use, distribution or reproduction is permitted which does not comply with these terms.
*Correspondence: Zhonggen Li, bGl6aG9uZ2dlbmNuQDEyNi5jb20=
Disclaimer: All claims expressed in this article are solely those of the authors and do not necessarily represent those of their affiliated organizations, or those of the publisher, the editors and the reviewers. Any product that may be evaluated in this article or claim that may be made by its manufacturer is not guaranteed or endorsed by the publisher.
Research integrity at Frontiers
Learn more about the work of our research integrity team to safeguard the quality of each article we publish.