- 1University Paris-Saclay, INRAE, AgroParisTech, UMR ECOSYS—Ecotoxicology Team, Versailles, France
- 2INERIS, Unit ETES, Parc Technologique ATALA, Verneuil-en-Halatte, France
- 3Ecotox Centre, EPFL ENAC IIE, GE, Lausanne, Switzerland
- 4Ecotox Centre, Dübendorf, Switzerland
Soils can be sinks of pollutant mixtures, whose effects on terrestrial ecosystems are not of obvious interpretation. Risk assessment is rather codified and many approaches can be used. Nevertheless, there are still uncertainties remaining when dealing with diffuse pollution, including chronic inputs of low, sublethal, concentrations of mixtures of micropollutants. In this paper, we reviewed through a comprehensive literature analysis one of the latest promising methodologies, the triad approach, in order to understand its area of application in terrestrial ecosystems, the ways of applicability and the reported actual usage. In the case of diffuse pollution, where all the criteria of the triad approach can differ in their indication, we show that some improvements have to be made in either the chemical, ecological or ecotoxicological approaches to be able to clearly identify the risk and to address the uncertainties linked to the low, sublethal contents of contaminants.
1 Introduction
Soils are continuously subjected to the pressure of different stressors, which make them vulnerable to degradation. In Europe, the prioritized soil threats (Commission of the European Communities, 2006) span across a wide array of physical and chemical processes, which can be independent or related to human activities (Stolte et al., 2015). Anthropogenic pressure on soil ecosystems, however, is prominent and mainly takes shape into a number of industrial and agricultural activities, which cause the release of toxic substances via multiple contamination routes (Leclerc et al., 2019). Main contaminants include: 1) potentially toxic elements (PTE) such as metals, 2) organic compounds, and 3) compounds of emerging environmental concern (Vodyanitskii and Yakovlev, 2016; Snow et al., 2019) such as pharmaceuticals and personal care products (Boxall Alistair et al., 2012; Williams-Nguyen et al., 2016), 4) material weathering byproducts (Ng et al., 2018; Sieber et al., 2020), 5) engineered nanoparticles (Gottschalk et al., 2013; Adam et al., 2018) and 6) plastics (Rillig et al., 2017).
To face the widespread problem of land contamination, different practices have been adopted. Management of contaminated land has been developed in a systematic way from the 1980s and involves different forms of ecological risk assessment (ERA), which is the process that aims at indicating ongoing or future harms sustained by one or more components of an ecosystem (US EPA, 1992). Various procedures fall in such a broad definition and it is not possible to identify a fit-for-all approach. In general, they involve the analysis of collected evidence and characterization of the risk associated to contamination, in order to undertake management actions (Weeks and Comber, 2005), according to national environmental policies. Measurements of contaminant concentrations are compared to benchmark values, when these are present in national policies, as in Dutch and Italian legislations (Lgs, 2006; Swartjes et al., 2012). These countries enforce soil-screening values that, if exceeded, trigger risk assessment procedures to inform on necessary actions. On the one hand, benchmark values are difficult to construct and not universally adopted, and on the other hand, when they exist, they can be different between countries, depending of various factors like the type of soil, the geochemical background or agricultural and industrial practices.
Moreover, the form and spatial distribution of contamination greatly varies according to the source of emission and characteristics of the receiving environment. Local point-source contamination due to human activities, for instance related to industrial plants and landfills, usually affects a defined area interested by a single hotspot source, hence being easily identified. Inputs of contaminants in the environment from multiple spatially unconfined sources, such as atmospheric deposition, runoff from urban areas, farmlands and road systems, lead to diffuse contamination involving large areas of land affected by mixtures of contaminants (Biasioli et al., 2012; Giandon, 2015; Fabian et al., 2017; Cachada et al., 2018). Although diffuse contamination chronically affecting terrestrial ecosystems represents a well-known environmental issue, it received less attention than hotspot like contamination. This is partly due to the complicated determination of its potential consequences to soil systems and because of the practical difficulties of managing diffusely contaminated areas (Posthuma et al., 2008). For instance, this could be due to the inadequacy of simple soil benchmark values to capture the risk posed by mixture of contaminants, whose concentration falls below such thresholds. Diffuse contamination presents other challenges, such technical ones related to the identification of low levels of target chemicals in heterogeneous soil matrices. The magnitude of diffusely polluted areas may equally make it impractical to attain a full chemical characterization of compounds of interest in all of the concerned soils. Moreover, adverse effects stemming from diffuse contamination might not be manifest or take longer times to clearly emerge, hence being difficult to identify, as chronic inputs of low levels of contaminants may lead to a concentration buildup in soils, until resulting in adverse effects to terrestrial ecosystems (Cachada et al., 2018).
In the case of terrestrial systems chronically receiving inputs of contaminants from different sources, the occurrence of adverse ecological effects is particularly influenced by the characteristics of the receiving environment. It was pointed out that the characterization of such effects is different from the case of local source pollution and, as a consequence, soil diffuse contamination requires site-specific instruments to study the exposure and effects (Posthuma et al., 2008). Intuitively, a multidisciplinary approach better suits the complexity of diffusely contaminated soil and the variability of associated effects. Moreover, it is a valuable effort in transitioning from a chemically-anchored to a more inclusive risk assessment. The combination of different scientific inputs within a weight of evidence (WoE) framework, as reported in the triad procedure (Chapman, 1990), seems a valuable tool to deal with site-specific scenarios. The triad ERA framework is based on the underlying concept that only the integration of different yet complementary information of chemical, ecological and ecotoxicological lines-of-evidence (LoE), organized in a tiered approach, can improve risk assessment of low contaminated soils. Given the vast and complex nature of soil pollution, the triad is a versatile tool that can be adapted to site-specific situations and potentially overcome the limitation of classical ERA in face of diffuse contamination.
In order to help advancing the risk assessment and management of the more generally encountered chronic and diffuse low contaminated sites, the aim of this review is threefold: 1) to describe the state of the art of the general triad approach, 2) to provide an analysis of its strengths and pitfalls emerging from its reported applications and 3) to examine the suitability of triad to address the challenges of diffuse contamination and propose improvements of the approach. The result of this analysis will summarize knowledge on triad approaches and aid to direct efforts in addressing the gaps in risk assessment of contaminated soil using such procedures.
2 Conceptualization of the Triad Approach
Soil consists of highly heterogeneous but structured environmental compartments and their characteristics largely determine the bioavailability of the received chemical contaminants (Lanno et al., 2019). It follows that effects of soil contamination are site-specific (Posthuma et al., 2008) and impose matrix-based approaches instead of compound-based ones (Pivato et al., 2017) for a realistic ERA. The deployment of a site-specific approach implies the usage of methodologies from different fields, organized in distinct LoEs, which group a set of measurements tackling one aspect of the considered risk assessment (Swartjes et al., 2012). The use of complementary and corroborative analyses has been initially proposed by (Long and Chapman, 1985) in the Sediment Quality Triad, in order to compensate for an approach confined to a single discipline. The underlying idea of the three-faceted procedure stems from the shortcomings of chemical measurements of environmental samples, as total contaminant levels alone may not be ideal indicators of biological effects (Chapman, 1990). In particular, biological endpoints should be accounted for by flanking chemical analyses with relevant measurements during ERA, as they can elucidate potential adverse outcomes and provide a causal nexus. Nonetheless, even if ecotoxicity assays reflect the toxic potential of a soil, they do not associate it with a specific compound or mixture (Cachada et al., 2018). At this point, chemical analyses should include information on the availability of the compounds they identify in soil. Indeed, for the presence of contaminants in the environment to translate into toxic effects, their bioavailability is a prerequisite. Bioavailability can be viewed as a concept made up of three interdependent latent variables (Beaumelle et al., 2016), which can be inferred through different measurable endpoints (Harmsen et al., 2005; ISO 17402, 2008). The first one is defined as the soil availability, which is the potentially accessible fraction of a compound present in soil. Environmental and toxicological bioavailability then represent the amount taken up by a soil organism and the one actually capable of initiating a toxic effect, respectively (Harmsen, 2007). Several proxies exist to infer the different bioavailability components, chemical and biological, of mineral and organic toxic compounds in soil (Peijnenburg et al., 2007; Ortega-Calvo et al., 2015), yet the proposed framework for bioavailability (ISO 17402, 2008) provides no standardized set of methods to be use in practice. Despite providing flexibility to adapt to case-specific needs, this can limit the systematic application of bioavailability as a tool to advance risk assessment (Harmsen and Naidu, 2013; Naidu et al., 2015). Indeed, examples proposing an actual integration of bioavailability into soil quality criteria and as an integral part in ERA are sparse (Brand et al., 2013; Kördel et al., 2013). Besides bioavailability, shifts in the biota composition assessed in situ, and therefore a change in the ecological functions of an interested area, have been progressively included in the triad approach, as indicative of an occurred negative alteration.
Hence, the underlying principle of triad is that none of the considered parameter is per se adequate to describe the environmental health but these are all complementary for an integrated stance (Chapman et al., 1987). Thus, ERA have increasingly relied on the integration of chemical data with additional ecological and ecotoxicological measurements, to increase realism and provide grounds for a more tailored appraisal of environmental deterioration. Above all, the conceptualization of the triad procedure marked an important shift towards an effect-based approach to ERA, where the type and origin of contamination revealed by chemical analyses must be robustly integrated to highlight an actual correspondence with biological effects. Such an integration of inherently different measures has been made by quantitatively synthesizing chemical, toxicological and ecological data into a final index, after their normalization with corresponding values from a reference unpolluted area. This approach enabled both the comparison among different areas and the monitoring of the evolution of the risk associated with a peculiar site in time, making the triad approach adaptable (Chapman, 1989; Chapman, 1990). Indeed, one of the strengths of the triad is that it can be used in a retrospective way when applied to assess the consequences of pollution, but also proactively, when contamination has not yet led to environmental degradation. The former approach can be used for the identification of sites needing remediation actions or simply to classify different areas according to the level of contamination. The latter approach can be incorporated in monitoring strategies of, for instance, environments likely to be affected by anthropogenic activities or sites that underwent remediation. The triad paradigm has been recognized internationally. Different site-specific ERA frameworks have been declined from the original Sediment Quality Triad applied by (Long and Chapman, 1985) into national legislations (Pereira et al., 2018) over the years, especially in the US, Canada, UK and the Netherlands (Breure and Peijnenburg, 2003; Smith et al., 2005; Swartjes et al., 2012). Additionally, the structure of triad procedure for soil quality assessment has recently been the object of standardization efforts, as outlined in Figure 1 (Jensen and Mesman, 2006; ISO 19204, 2017), in order to streamline its application and provide a clear guide for site-specific risk assessment (Rutgers and Jensen, 2011). As of today, ERA procedures do not systematically embrace the triad multidisciplinary concept, even though a standard framework exists. Rather, we argue that the possibility to increase the quality and quantity of input data is a key feature to allow including pertinent analyses to broaden the applicability of triad.
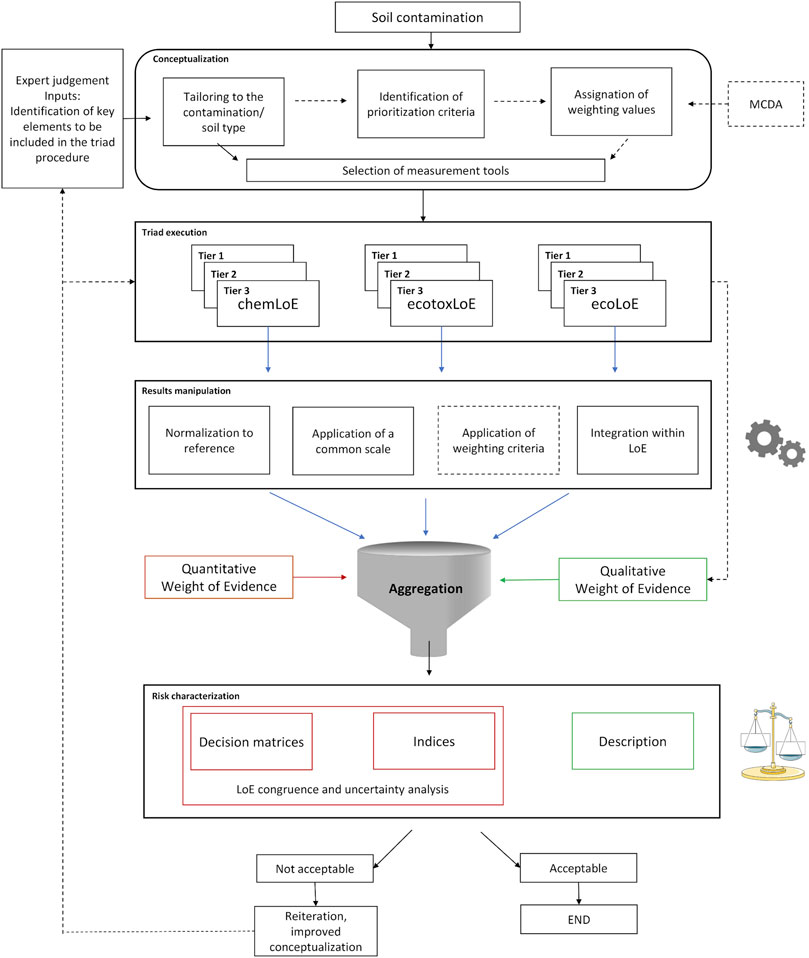
FIGURE 1. Layout of the main steps involved in the triad procedure, from the problem formulation, to execution and integration phases. Solid lines represent the minimal workflow composing the triad approach. Dashed lines symbolize additional steps that can integrate the different parts of the procedure.
3 Systematic Literature Search
A comprehensive bibliographic research was performed to identify studies reporting the application of triad approaches in soil. The literature search was last updated in August 2021. The Scopus database was queried using a combination of the keywords “Triad” AND “Risk” AND (“ERA” OR “Environmental” OR “Ecological” OR “Procedure”) as a search equation. The search comprised paper titles, keywords and abstracts, spanning from 2000 to 2020, and resulted in 239 items. Even though the Sediment Quality Triad approach was theorized as early as 1985 (Long and Chapman, 1985), we chose to restrict the time frame for the literature search as more structured frameworks for the application of triad appeared only later (Jensen and Mesman, 2006; ISO 19204, 2017). The publications were pooled in a Zotero library to screen paper titles and abstracts in order to exclude those clearly not relevant to the field. After this elimination the resulting 29 papers dealing with the triad were further sorted according to 1) pertinence to the subject, 2) originality and 3) completeness of the applied triad procedure. Likewise, review papers, as well as viewpoints or methodological descriptions of the triad procedure were not considered. Following this step, only papers focusing on triad-based ERA applications were finally retained. Furthermore, the addressed environmental compartment in each study was considered an additional selection criterion, as the focus was on the application of triad to terrestrial environments. This further screening reduced the number of journal articles to 11. Using these, we aimed to highlight patterns in the application of the triad approach by focusing on:
(1) The study area and contamination type, such as hotspot or diffuse soil contamination and the type of pollutant
(2) The type and complexity of the methodologies used.
(3) The conclusion of the study and the main issues encountered
Owing to these criteria, we broke down the practical triad applications to soil contamination into their defining characteristics by obtaining relevant information concerning the nature of contaminants, the origin of soil contamination, the chemical analyses of soil, the bioassays and the ecological approaches used, and the main outcomes of the procedure. In most cases, the specific methodologies listed in the studies within each LoE were already assigned to a specific tier by the authors. However, when this was not done, we used the standard frameworks found in the literature (ISO 19204, 2017; Jensen and Mesman, 2006; Rutgers and Jensen, 2011) as guidelines. Moreover, we chose to classify the specific methodologies not listed in the ISO guideline ultimately in term of their level of complexity.
4 The Pillars of the Triad Structure
The inputs for triad risk assessment encompass chemical, ecotoxicological and ecological investigations, organized in three separate LoEs. A classification of the recommended tests and methodologies to be employed in each LoE is now presented in standard guidelines (ISO 19204, 2017) Within each LoE, tests and measurements are organized in three sequential tiers of increasing complexity and labor-intensity, in order to improve cost-effectiveness of the procedure (Weeks and Comber, 2005). If the result of a tier is associated with an unacceptable level of uncertainty, the triad is repeated under the next tier, until a satisfactory risk assessment can be derived (Figure 1). The triad tiers have been described and used in various contexts by different authors over the years (Rutgers et al., 2000; Jensen and Mesman, 2006; Swartjes et al., 2012). The first tier generally includes rapid and cheap methodologies for a simple screening, while tier two is a “refined screening,” where the specificity of test increases. The third tier is often named “detailed assessment,” where more sophisticated tests and analyses are used to increasingly tailor the assessment to a specific terrestrial ecosystem, in order to reduce residual uncertainties. The choice of assays to include in tiers is not limited to a certain set of tests defined a priori for each LoE. Thus, chemicals, toxicological and ecological parameters, other than the ones listed in standard documents (ISO 19204, 2017), can be chosen to tune triad approach according to the specific objectives.
4.1 Chemistry LoE
Preliminary knowledge of the anthropogenic stressors affecting the studied soil is required to inform on the presence of compounds of interest. As in tier one the level of refinement of chemical analysis is low, only total soil concentrations are generally considered, enabling an initial screening from which useful information can be gained. The first one is the comparison of chemical levels in soils with environmental quality standards or background values (Dagnino et al., 2008), when these are provided. An interesting use of the tier one chemical screening is linked to the species sensitivity distributions (SSD) (Posthuma et al., 2001; Signore et al., 2016). SSDs are used to derive generic quality criteria, such as the hazard concentration for 5% of the species (HC5) based on no observed effect concentrations (NOECs). Thus, starting from measured total concentrations, SSDs can be used to determine local risk (Posthuma et al., 2008). Total soil concentrations can then be used to derive the toxic pressure of a chemical by calculating the potentially affected fraction (PAF) from SSDs retrieved from ecotoxicity databases of, for instance, 50% effect concentration (EC50) for the considered chemical (Posthuma and Suter, 2011). Finally, PAFs from locally occurring contaminants can be combined in a single multi-substance PAF to summarize the site-specific toxic pressure (Jensen and Mesman, 2006; Pit et al., 2018).
Though widely employed in the first triad tier, such an indirect approach was questioned (Gutiérrez et al., 2015), and amendments to integrate chemical bioavailability instead of considering total soil concentration in site-specific ERA have been proposed (Janssen et al., 2003). Thus, chemistry LoE at tier two differentiates between various chemical fractions or speciation associated to soil components (Kumpiene et al., 2017). As of PTEs, for instance, the dissolved fraction in pore water is considered readily available for soil dwelling organisms, and more indicative of potential toxic effects than the total soil contents (Luo et al., 2014). Together with the concentration of dissolved metals, at this stage extractive methods can be used to estimate metal bioavailability. Methods with different extraction power can analytically define easily or hardly accessible metal fractions in the soil compartment (Peijnenburg et al., 2007). Deionized water and CaCl2 extractions are often used in this regard to define the readily accessible metal pool, while extraction through chelating agents (Beaumelle et al., 2014) or acid treatments account for tightly-bound fractions more scarcely available for soil organisms. Even though such operational definitions of soil metal pools represent only proxies of actual bioavailable metals (ISO 17402, 2008), they provide additional information for a more realistic assessment of contamination that mainly considers chemical species likely available to biota. Accounting for the contribution of site-specific soil characteristics, such as pH, organic carbon content and soil texture, enables a more accurate determination of the exposure pathways to a contaminant, overall contributing to reduce uncertainties regarding the risk for defined ecological receptors. For PTEs, additional details can be gained in the third tier employing generic mechanistic models in addition to empirical ones to predict, for instance, the speciation or amount of free metal ions according to the parameters of a specific soil (Beaumelle et al., 2015; Beaumelle et al., 2016). The output data of such modelling in terms of available pools have been shown to be better correlated to the impact on soil organisms than total or extractible contents, in relation with the pathways of soil organisms exposure (Lofts et al., 2013) and generally to better express the causal relationship between contamination and effects on biota.
4.2 Ecotoxicology LoE
Bioassays provide a means for a direct toxicity assessment, hence relating to the toxicologically available fraction of a studied substance or mixture in soil (Smolders et al., 2009; ISO 17616, 2019). Rapid standardized bioassays and toxicity tests are often the starting point of ecotoxicological evaluation and belong to the first tier (ISO 19204, 2017), fitting in the purpose of a preliminary screening. Cell-based assays or bacterial bioreporter strains are often employed at this stage, providing a generic measure of toxicity. Similarly, ecotoxicity tests such as earthworm avoidance assays (Amorim et al., 2005; Loureiro et al., 2005) and plant germination tests represent a suitable options for fast ecotoxicological screening of soil samples. The methodologies employed at this stage lack the representativeness of the studied site while providing generic toxicity endpoints not aiming to capture the biological significance of chemical contamination, yet being useful owing to their rapidity and relative ease of use. In the second tier a higher level of ecological relevance is seeked with increasing site-specificity of biological analysis, and this often translates into the choice of representative soil organisms for ecotoxicity testing. Bioassays in tier two may encompass endpoints responding to lower contaminant concentrations, hence being more adapted to diffuse soil pollution. The time span of ecotoxicological tests is also longer as chronic effects are often privileged over acute ones. Consequently, endpoints such as soil invertebrate reproduction impairment are preferred over lethality, and plant physiological endpoints, such as root length and biomass production are adopted, resulting in higher sensitivity. According to the purpose of a refined investigation and a more exhaustive biological assessment, ecologically relevant test organisms may be chosen, such as different species of soil oligochaetes (Spurgeon et al., 2003), isopods (Drobne et al., 2008; Udovic et al., 2009; Lavtižar et al., 2016) and springtails (González et al., 2011) that have been extensively used in soil ecotoxicology. The biological tests in the third tier are carried out in the case of remaining uncertainties. While the causality of toxic effects due to soil contamination has already been established, the investigations carried out at this point shift the focus from the characterization of the exposure to the investigation of specific effects. The resulting information concern contaminant mode of action and are achieved via tests focused on specific sublethal toxicity mechanisms (Environment Agency, 2004). For instance, these can be biomarkers, such as the activities of antioxidant enzymes or the activity of ATP-binding cassette (ABC) transporters. In addition, the expression of genes inducible by certain chemical classes or specific tests, such as the inhibition of acetylcholinesterase, can pinpoint the effects of a compound towards exposed organisms. Of particular note is the use of multi-species tests at the last triad stage, such as microcosms and mesocosms (de Zwart and Posthuma, 2005) employing at the same time plants and animals. This can result in complex test setups, which can nonetheless cover the interaction of different organisms across multiple levels of the trophic web, and increase the ecological relevance of ecotoxicology LoE, drawing closer the measured responses to the assessment endpoints that set out the ERA.
4.3 Ecology LoE
A wide array of ecological procedures of sequentially increasing complexity can be included in the triad approach (Jensen and Mesman, 2006; ISO 19204, 2017). The estimation of the vegetation cover of studied sites is commonly used in the first tier. Instead, tier two procedures encompass the determination of abundance and richness of soil fauna, usually considering arthropods, earthworms or microfaunal specie, such as nematodes and collembolans. Field ecological observations are relatively simple to perform yet they rely heavily on the presence of accurate “control” sites to be used as a reference, whose characteristic are similar to the studied one but unaffected by contamination. It was reported that methods based on site surveys can generate misleading results when compared to inappropriate reference areas due to the heterogeneity of factors that can influence the composition of plant and faunal communities, often independent from contamination (Sorvari et al., 2013). Additionally, soil functional parameters can be considered in tier two. The ability of a soil to contribute to essential ecosystem services, such as nutrient cycling and carbon storage, is assessed by highlighting an impairment of the processes underlying such functions due to contamination. Moreover, such endpoints reflect an actual disruption of biological processes in situ, providing evidence of an occurred negative effect sustained by soil ecosystem. To this end, soil feeding activities, such as litterbag decomposition and bait lamina assays (ISO 18311, 2016) are proposed. Being important from a functional standpoint, measurements of different microbial enzymes, such as alkaline phosphatase and urease, for instance involved in nutrient acquisition or major element cycles, are also suitable for inclusion in tier two toolboxes (Dick et al., 1997; Gianfreda et al., 2005; Xian et al., 2015). It was shown that these respond to soil contamination earlier than other microbial parameters, which could make changes in enzyme activities useful in the case of diffuse pollution (Winding et al., 2005). Basal and soil substrate-induced respiration, together with carbon mineralization and nitrification, have also been considered at tier two both as a proxies for microbial activity in soil and for their capacity to contribute to carbon and nitrogen cycles (Winding et al., 2005). The analyses proposed for incorporation in the second tier require increased time and resources together with major technical skills yet can improve the refinement of the ecological LoE results and provide insight on the status of soil ecological functioning. Soil genetic materials is collected and studied when tier three evaluation is needed. In this regard, metagenomic analyses are a method of choice to generate genetic profiles of soil microbial communities in order to assess their diversity or the presence/absence of specific bacterial or fungal phyla. Long term ecological monitoring can also be advisable in the case of a tier three detailed assessment (Jensen and Mesman, 2006; Rutgers and Jensen, 2011). As such, they follow the evolution of an ecological endpoint identified as possibly impacted by contamination affecting the studied soil. For instance, the disappearance of a top species performing key ecological functions in the considered ecosystem can be regarded as a loss of site-specific ecological value consequent to human activities. These analyses require a follow-up on the studied site taking into account possible confounding factors including the sampling season, key soil properties, temperature and humidity around the sampling area and a good knowledge of ecologically relevant species in a non-contaminated part of the site. Hence, ecology LoE implies a general preliminary insight on the site in order to account for confounding factors and adapt the experimental strategy accordingly.
5 Pitfalls Between the Theoretical and Practical Application of the Triad Approach
Over the years, the triad approach has been performed in different ways. From the original formulation of the concept (Long and Chapman, 1985) the execution has evolved from a simple compilation of experiments making up chemical, ecotoxicological and ecological LoEs, to a more structured approach (Figure 1). Moreover, analytical advances were progressively translated into the enrichment of the methods able to provide data within individual LoEs. As conducting an ERA procedure implies selecting, organizing and making sense of a wealth of different information, structured approaches such as frameworks and decision support systems set out to instruct and homogenize such tasks (Jensen and Mesman, 2006). A compelling reason to adopt the triad approach as a framework for ERA resides in the potential for optimization of resource use and allocation. Indeed, while the open nature of the framework allows the end user to implement each LoE according to specific needs, their sequential application through tiers can rule out the need for time and resource demanding analyses, if an acceptable outcome is reached early in the process.
Scientifically, the strength of the triad approach stems from the adoption of operationally independent pathways to reach a common conclusion on risk assessment. In theory, such approach should produce unbiased and structurally sound results, associated with a low level of uncertainty. The practical application, however, is faced with the organization of the whole process and the integration of heterogeneous results, in a way as to minimize loss of information. To this end, WoE approaches have been developed to work with different types of information for the ecological risk characterization (Burton et al., 2002; Smith et al., 2002; Linkov et al., 2009; Suter et al., 2017). In the triad, the whole set of LoE data need to be normalized before aggregation, in order to be projected on a common scale, without losing their quantitative value. Once scaled, information produced within LoEs are conveyed into the same risk assessment framework and synthesized following qualitative or quantitative approaches. The simplest approach is to combine qualitatively LoE inputs, without an integration, and to present the results drawing conclusions on the ecological risks based on the listed evidence (Linkov et al., 2009). While being rapidly performed and adaptable, such process lacks transparency and it was pointed out that a simple qualitative evaluation is more appropriate when the effect of contamination are clearly identifiable (Burton et al., 2002). This is particularly the case of acute contamination near hotspots, when an agreement of chemical, toxicological and ecological LoEs is expected, facilitating expert decision. Conversely, in soils subject to diffuse chemical inputs or in cases where aging reduced contaminants bioavailability, indications of related impacts may neither be easily identified nor disentangled from confounding factors (Smolders et al., 2009). The triad approach thus really makes sense when LoE information tend to diverge, so that more structured quantitative methods are needed to evaluate the results and reach a conclusion on risk assessment. Therefore, in most scenarios, a well-defined and articulated approach is suited, capable of integrating information form site-specific tests to draw objective conclusions (Rutgers and Jensen, 2011; Swartjes et al., 2011; Swartjes et al., 2012).
The final stage consists in combining the individual test results within a LoE and subsequently performing an overall integration into a final index, such as a risk number (IR), normally ranging from 0 to 1, or a decision matrix. This is essentially the product of the WoE approach and summarizes the site-specific information collected during the process. As such, it can be viewed as a proxy synthesizing soil health, according to three different but complementary points of view, which enables the comparison with impairment thresholds. These can be user-defined limits indicating the risk at a site as for instance low, moderate or high when the IR falls within a defined range (Jensen and Mesman, 2006; Semenzin et al., 2008; Rutgers and Jensen, 2011). Consequently, the risk posed by contamination at a certain site can be deemed acceptable or not according to the envisaged land uses. For example, an IR falling in the 0.51 to 0.75 range could be acceptable for an industrial use but not for an agricultural one. The extent to which chemical, toxicological and ecological information agree in the final risk estimate is also evaluated at the integration stage to ascertain individual LoE contributions to the IR. Discrepancies in the magnitude of risk indicated by the different LoEs undermine the reliability of the IR, and thus the significance of the triad outcome. Theoretically, with an a priori knowledge of a specific case, one could reduce the need to reiterate the process by adapting LoE strategies from the beginning, which can explain the scarcity of published studies reporting repeated triad application to a same site. However, if the difference between the results acquired in each LoE is too high, it is recommended to use the next tier.
Owing to the complementarity of LoEs, the results of site-specific measurements should contribute equally to risk assessment. However, it may be decided to give more importance to certain aspects over others. Weighting of components within a LoE, or of an entire LoE over the others, can be advisable in some situations. For instance, weighting can be useful to compensate for the inherent uncertainties of a particular test or LoE, by lowering their decisional power. In other cases, weighting may be needed to balance results deviating from the control ones, produced at the reference site, due to factors other than the studied stressor. This is particularly true, for example, in the case of significant differences of ecological communities between control and study areas simply due to a different biotype, and ultimately comes down to the difficulty of finding appropriate reference sites. Finally, the use of weighting factors can reflect the expert judgement of risk assessors applied to the specific case of study. For instance, this can be driven by the nature of the soil or the type of contamination encountered. More simply, they can be applied following an a priori evaluation of the relevance of different triad information. One systematic approach consists in organizing expert inputs and categorizing triad tools in terms of their contribution to ERA (Semenzin et al., 2009; Sorvari et al., 2013; Gutiérrez et al., 2015). For instance, the sensitivity of ecological tests for ecosystems effects, or the endpoint sensitivity of ecotoxicological assays, were reported similar valid parameters to estimate the ability of a measurement endpoint to capture impairment at the ecosystem level due to soil contamination (Critto et al., 2007). Chemistry LoE parameters suitability can also be compared in a similar way (Semenzin et al., 2007). Indeed, different approaches for identifying soil chemical contamination can take into account different variables involved in the dynamic exposure to contaminants. As bioavailability is determined by a concurrence of factors inherent to soil type, contaminant chemical characteristics and the biology of exposed organisms (Lanno et al., 2004), chemical tools may incorporate such variables to a different extent. As such, they can be compared based on criteria reflecting their inclusivity of the aforementioned aspects of bioavailability and their relevance to a specific soil. Moreover, criteria accounting for test standardization, time and cost can define a tool as mostly suitable for a tier or another, this being in line with the triad as an efficiency-enhancing framework for ERA. Likewise, according to such metrics the weight of each measurement endpoint is defined and used in the final integration of the results within each LoE, and eventually into a final risk index (Semenzin et al., 2008). To make sense of such elements in the decision making process, multi-criteria decision analysis (MCDA) was recently reported to provide a valuable method when applied to environmental WoE procedures (Linkov et al., 2006, 2009, 2011). In fact, MCDA allowed the aggregation of information concerning the attributes of each test according to a user-defined set of values, tailored to the site-specific scenario. Reported outputs of MCDA applied to ERA aimed to support the decision-making and in general the development of a shared strategy by clearly ranking the possible alternatives for each LoE, therefore deducting from the subjectivity of the decisional process. Hence, the outcome of ERA being highly dependent on the practical experimental means, this could represent a double opportunity for a mindful choice of tests and the derivation of their weighting factors, in a reproducible way. In practice, however, weighting is seldom performed and few concrete examples are found in relevant literature (Dagnino et al., 2008; Terekhova et al., 2014). The lack of detailed instructions or of a reference frame in international standards for classifying tests according to a defined set of attributes makes deriving weighting factors a bias-prone and laborious process, and could hamper its systematic adoption in the triad. The feasibility of this can be considered crucial, as the choice of LoE parameters necessarily influences the whole downstream process. Thus, between theory and application, one of the main issues is the subjectivity of the criteria that must be sought, using also the flexibility that the triad approach allows.
6 Real Life Applications of the Triad Approach
Here, we summarize the most relevant applications of triad-based approaches to real-case scenarios of soil contamination, and broke the procedures down to their components. Using literature to compile data on the already used triad approaches, we selected the following items: the targeted contaminants, the type of site destination, the type of contamination between diffuse and hotspot, the endpoints and tiers chosen for each LoE, as summarized in Table 1. Among the discarded studies, some showed an incomplete triad approach, where experiments belonging to a specific LoE were not performed due to technical challenges (Volchko et al., 2020). One interesting example is the study from (Pereira et al., 2017), which applied a tier 1 chemical and ecotoxicological LoEs to Antarctic soils. The authors did not provide a reason for the exclusion of ecological assays from the triad procedure yet we can suppose that the peculiar nature of the polar environment may have limited the feasibility of ecology LoE. In some other instances, an entire LoE was replaced with different type of analyses. For example, this was the case of groundwater (Crévecoeur et al., 2011), where LoE for ecology was substituted by physico-chemical measurements (i.e., pH, conductivity and nutrient levels), due to the impossibility to perform an ecological assessment. In another study, genotoxicity and endocrine disruption biomarkers on exposed fishes served as proxies to make up for the lack of ecological data for surface waters (Mendes et al., 2017). These examples highlight the difficulty to capture ecological features in water matrices for exploitation within an ERA framework, as opposed to land-oriented approaches where plants or soil organisms can be more easily studied. Nonetheless, among the works conforming to the selection criteria, some dealt with risk assessment of water systems. As originally the triad approach was theorized for sediment quality appraisal, its application to sediments collected from contaminated freshwater and marine ecosystem was consistent among the results of our search. Furthermore, there was a clear distinction between studies dealing either with riverine or estuarine environments. The formers showed a pattern of a localized contaminant inputs due to past industrial activities, involving the discharge of mercury (Flanders et al., 2019), DDT (Marziali et al., 2017; Harwood et al., 2019), metals (Marziali et al., 2017) and organochlorines (Iannuzzi et al., 2008). Usually, a reference area was chosen upstream, while different points were characterized downstream along a contamination gradient with increasing distance from the source (Silva et al., 2016). The geographic breadth of studies dealing with estuarine ecosystems was generally larger, as is the case of (Moreira et al., 2019), where sampling points encompassed a large costal area interested by land-driven contaminants via river discharges. Similar yet smaller in size was the case described by (Lee et al., 2018), concerning a bay historically impacted by different anthropic activities. In these last studies, multiple contaminants inputs, such as production plants and increased urbanization, were the motive behind the realization of a triad procedure. Diffuse contamination was also at the base of the case reported by (Iwinski et al., 2016), due to the repeated usage of copper-based biocides in a lake system. To date, however, studies reporting on the usage of a complete triad approach specifically to tackle soil contamination are scarce in the published literature. In this context, the addressed environmental compartment in each study was considered a further selection criterion for the publication summarized in Table 1.
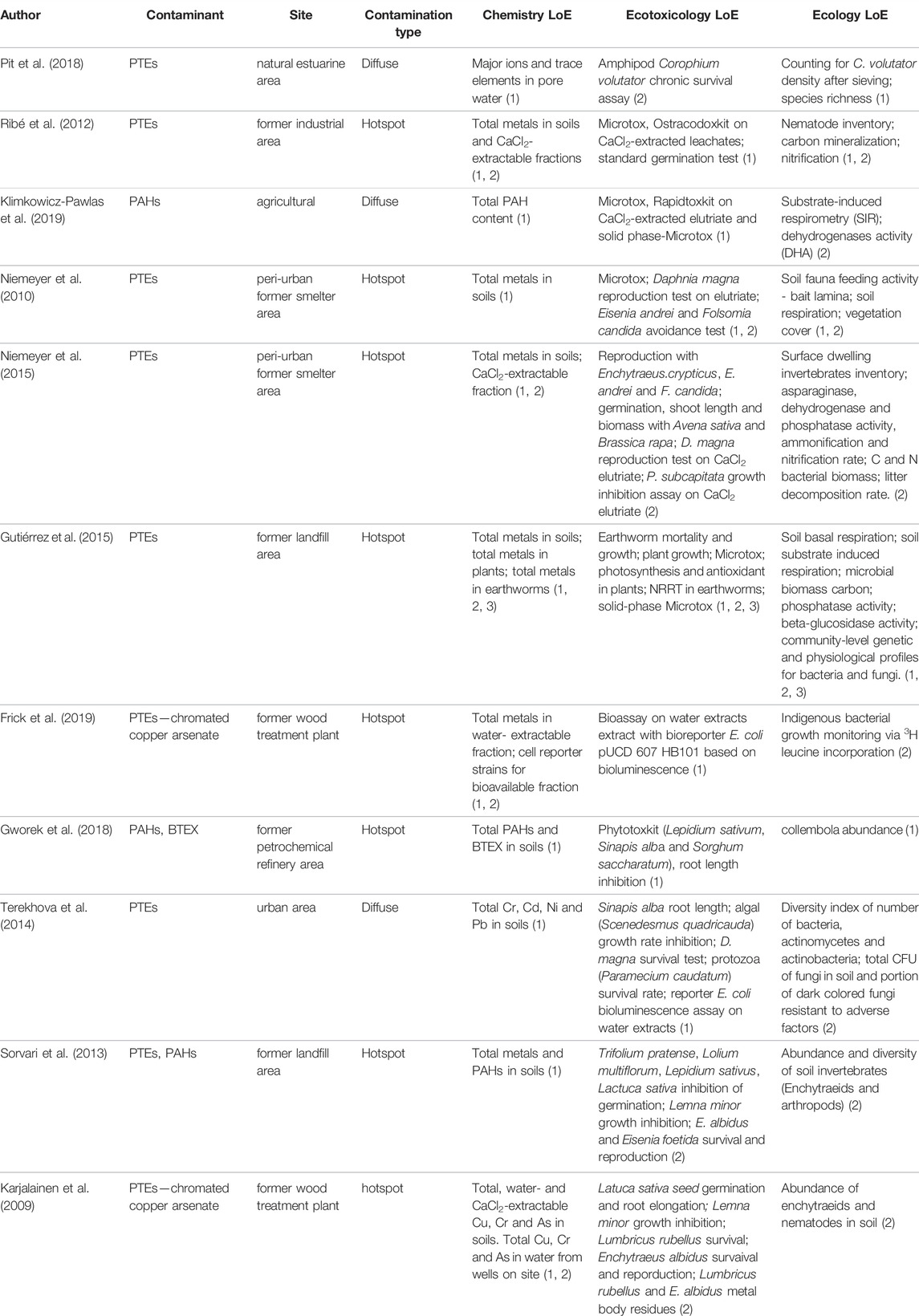
TABLE 1. Summary of the main characteristics extracted from studies reporting a triad approach in contaminated soil. The reported triad level of each LoE was either already stated in the literature or designated in the present study, when possible according to standard guidelines or to the authors’ judgement. Numbers in brackets represent the assigned tier of each LoE.
6.1 Study Area and Contamination Type
There was an evident predisposition in the analyzed literature for sites with an industrial past, with 8 studies out of 11 dealing either with discontinued manufacturing plants or closed waste disposal sites. When activities related to a production plant cause the localized emission of chemicals, actions to prevent or remediate the contamination can be implemented. Together with being of easier identification, events where contamination is usually circumscribed to a specific area can be easier to remediate. On the same note, due to dealing with a peculiar industrial process, production plants may cause the discharge of contaminants belonging to the same chemical class, which facilitates monitoring and remediation actions. The prevalence of studies targeting such contamination type (Table 1) could be an indication of these factors. In fact, papers reporting on diffuse contamination scenarios of soils were limited to vehicular traffic and urbanization (Terekhova et al., 2014) and a combination of local and neighboring energy production activities (Klimkowicz-Pawlas et al., 2019). In line with this, legacy contaminants were most represented among the literature. Mainly PTEs and PAHs in soils were the object of the triad approaches analyzed here, while none of the studies dealt with terrestrial contamination by substances of emerging concern. Being among the most common mineral and organic contaminants, it is intuitive that they would figure as primary targets for risk assessment and remediation. Moreover, conventional methods for chemical characterization of total burdens and available fractions of such compounds in soils are more readily accessible and routinely employed, while analytical techniques for emerging contaminants may pose additional challenges and necessitate specific tuning. This could limit the applicability of triad for novel soil threats, such as the emerging issue of microplastics and associated chemicals in the terrestrial environment (Billings et al., 2021). It can also be argued that for chemical substances of emerging concern the scientific interest in their fate and effects is still growing, which leaves chemical and toxicological methods currently open for implementation and standardization. Thus, their application in an ERA framework can be more challenging, compared to legacy contaminants (Koelmans et al., 2022). In addition, as literature mainly reports triad application to historically contaminated sites due to, among others, past production plants, it appears that retrospective ERA would find fewer opportunities for application to chemicals of emerging concern.
6.2 Type and Complexity of the Methodologies Used
Table 1 reports the experimental procedures used to contribute to chemical, ecotoxicological and ecological LoEs. Even though the categorization as belonging to specific tier is not comprehensive, we assigned the listed methodologies to three tiers of increasing complexity, specificity and cost, according to the existing frameworks (ISO 19204, 2017) and based on our judgement.
6.2.1 Chemistry: Methods Used to Quantify the Contents and Availability of Pollutants in Soil
The complexity of chemical analyses carried out varied greatly, as the main distinction was in the focus on total or available soil contaminant fractions. Half of the considered studies employed only generic quantification approaches, such as total soil burdens for organic and mineral compounds, with the rest aiming to refine the analysis by focusing on certain analytically accessible fractions as well. Generally, measuring total soil concentration was the first step also for studies additionally using higher tier methods. A screening of the presence of one or more contaminant of interest is straightforward in a generic assessment of soil status and allows for the quick comparison with threshold values, when applicable, in order to decide if further investigations are needed. However, these do not provide information on their availability to soil biota. Hence, despite serving the purpose for a tier 1 assessment, one can argue that their utility resides mainly in the comparison with soil quality standards, while their usefulness in predicting deleterious effects on soil biota has been questioned (Janssen et al., 2003). In the case of metals, in order to incorporate environmental availability in risk assessment, mobile and readily exchangeable pools can be estimated with chemical methods such as soil incubation with water or CaCl2solutions, while ethylenediaminetetraacetic acid (EDTA) extraction can account for potentially available fractions (Harmsen, 2007; ISO 17402, 2008). When moving to tier 2 (Niemeyer et al., 2015), applied CaCl2 extraction to metal contaminated soils, while the measurement of total concentrations was performed in the case of a tier 1 chemical LoE, applied on the same site for a preliminary evaluation (Niemeyer et al., 2010). Being generally low cost and rapid, these methods were used in most papers reporting a refined assessment for PTEs. An exception was (Gutiérrez et al., 2015), where chemistry LoE encompassed the metal concentrations in exposed plants and earthworms for tier 2 and 3, respectively, as a direct bioavailability measure. On the same note (Frick et al., 2019), incubated Pseudomonas fluorescens and Escherichia coli coli luciferase reporter strains with water soil extracts to estimate As and Cu biovailability. Concerning PAHs, the analyzed studies focused only on the total soil concentration, generally by means of exhaustive extraction techniques (Gworek et al., 2018; Klimkowicz-Pawlas et al., 2019; Sorvari et al., 2013). It is known that such fraction does not reflect the bioavailability of organic compounds in soils and may not be as relevant for risk assessment as concentrations determined via extractive techniques involving, for instance, tenax, cyclodextrines, or mild solvents (Cui et al., 2013). It was argued that these should rather be employed to inform on the bioaccessible fraction in soils (Puglisi et al., 2007). Similarly, equilibrium partitioning methods (Niu et al., 2020), such as passive samplers like solid-phase micro-extraction fibers (ter Laak et al., 2006; Lu et al., 2011), can assess freely dissolved organic contaminant concentrations in soil pore water, which is highly relevant in determining exposure to soil organisms by being readily available. A suite of different in vitro bioanalytical tools exists, targeting the activity of various organic compounds in environmental samples, thus representing the toxicologically available fraction. When these were applied to crude extracts, they showed higher bioanalytical equivalent concentrations compared to non-exhaustive extraction techniques reflecting the bioaccessible fraction (Puglisi et al., 2007; Li et al., 2013), and proving that bulk soil extracts largely overlook soil processes responsible for the attenuation of bioavailability of organic contaminants, and possibly overestimate risk assessment. Accurate assessment is crucial as soil properties are key drivers of contaminant bioavailability. As an example, binding to soil organic matter is known to reduce contaminant bioavailability over time, which makes aging very important in certain soil types. The absence of such considerations, particularly in the case of organic compounds, interrogates on the suitability of total concentrations alone to reflect the influence of soil processes in determining the exposure to contaminants (Cachada et al., 2014). This seems of particular interest given the history of soil contamination reported in the triad approaches analyzed here (Table 1), specifically in the cases of (Sorvari et al., 2013; Gworek et al., 2018; Klimkowicz-Pawlas et al., 2019). Indeed, as most of the publications in relevant literature dealt with past, concluded inputs of chemicals in soils, attenuation of pollution due to biological and chemical processes can be hypothesized (Cachada et al., 2016). Despite their usefulness, to the best of our knowledge non-exhaustive and biomimetic extraction methods were not included in chemistry LoE of triad approaches, therefore missing out on the opportunity for a refined assessment based on bioavailability of organics in soils (Cachada et al., 2014, 2016; Titaley et al., 2020). We argue that the relative ease of use of such methods could represent a viable way to determine bioavailable and bioaccessible organic contaminant fractions in soil, and as such, they could improve the accuracy of a tier 2 stage.
6.2.2 Ecotoxicology: Type of Bioassays Used
Different ecotoxicity tests were used in the literature to assess the toxic potential of sampled soils. All of the 11 studies investigated here made use of some standardized assays, yet the breadth of test batteries varied greatly, as the considered endpoints. General toxicity was often assessed by means of the Microtox assay, based on bioluminescent Vibrio fischeri exposed either to soil solid phase (Klimkowicz-Pawlas et al., 2019) or leachates (Niemeyer et al., 2010; Ribé et al., 2012). Such tests fitted well in a tier 1 triad screening due to its broad applicability, rapidity and cost effectiveness. On the downside, they provide low soil ecological relevance and give no information on the toxic mode of action. Elutriates were also used for a number of tests involving crustaceans, with Daphnia magna being the most represented, assessing mortality and reproduction, and growth inhibition assays for green algae (Terekhova et al., 2014; Niemeyer et al., 2015). Using aquatic organisms, these necessitated the production of soil elutriates, either made by water or CaCl2 extractions, hence reflecting the bioavailable fraction of soil contaminants. It could be argued, however, that aquatic organisms are not adequate to reflect soil complexity and to translate soil contamination into meaningful biological effects, due to the specific interplay of soil matrix and terrestrial organisms on the resulting toxic effects. Nonetheless, according to (Critto et al., 2007; Gutiérrez et al., 2015), these are particularly suited for a screening phase due to their reproducibility and broad applicability, criteria highly valued in tier 1. This was also true for standardized tests with soil organisms, such as plants, oligochaetes and collembolans, whose ecological relevance is nevertheless higher. Survival upon exposure to contaminated soils was commonly assessed, whereas sublethal effects on growth or reproduction, were considered as higher sensitivity endpoints (van Gestel et al., 2001) and viewed as tier 2 tools. Moreover, such longer term sublethal endpoints can increase the ecological relevance of ecotoxicology LoE, also integrating diverse exposure routes to contaminants by employing different soil organisms (González et al., 2011). In fact, while some studies analyzed here showed absence of acute toxicity in soils with measured levels of contaminants, in general chronic tests better captured the effects of contamination. Reproduction tests with Enchytraeus crypticus, Eisenia Andrei and Folsomia candida were performed on metal contaminated soil in tier 2 by (Niemeyer et al., 2015) and, while reinforced the results obtained from the first tier ecotoxicology LoE, they reduced the associated uncertainty. Only two additional authors reported second tier ecotoxicological tests, involving oligochaete reproduction tests and phytotoxicity assays (Karjalainen et al., 2009; Sorvari et al., 2013), while ecotoxicology LoE was limited to tier 1 in the rest of the studies. This is in contrast to the discrepancies highlighted by some of these studies between the results of ecotoxicology LoEs and chemical analyses, the former not matching the reported presence of soil contaminants with effects on selected endpoints. For example, (Klimkowicz-Pawlas et al., 2019), applied a first tier ecotoxicology LoE on an agricultural soil impacted by multiple diffuse PAH sources, by means of the Microtox and Rapidoxkit assays (Table 1), showing low toxic effects, as opposed to the high risk index derived from chemisty LoE. Although it should be noted that exhaustive PAH extractions were performed, the outcomes of this study highlight the importance of the chronic and sublethal of bioassays and the choice of relevant endpoints to the soil matrix particularly in the case of diffuse contamination. The choice of different toxicity bioassays could improve the reliability of the indications provided by ecotoxicology LoE, if sublethal, chronic and more sensitive endpoints were prioritized over acute ones, although owing to the scarcity of their application, whether this holds true in practice remains unclear. Strikingly, only one study reported the use of sub-cellular biomarkers (Gutiérrez et al., 2015), namely lysosomal membrane stability of earthworms immune cells. The use of biomarkers to highlight soil toxicity due to different contaminants has broadly been discussed in ecotoxicology (Weeks et al., 2004; Forbes et al., 2006; van Gestel et al., 2009) and a suite of biochemical and molecular approaches tailored for soil organisms has long existed (Scott-Fordsmand and Weeks, 2000; Environment Agency, 2004; Spurgeon et al., 2005b). Their absence in triad-based approaches could stem from the inherent complexity and lack of standardized procedures. Responses at low levels of biological organization can be regarded as high sensitivity tools for elucidating toxicity mechanisms and discriminate contaminant exposure and effects, in order to reduce uncertainty for a detailed assessment. Tier 3 for ecotoxicology is a costly and time-consuming endeavor, rarely performed in the literature (Table 1) and this could be the underlying cause for the scarcity of biomarker-based approaches in triad procedures. Additionally, the interpretation of biomarker responses in the framework of regulatory ecotoxicology can be challenging, as the link between suborganism toxicity and deleterious environmental effects is not easy to make (Spurgeon et al., 2005a) and still a subject of research in modelling, for instance related to adverse outcome pathways (AOP). Therefore, the problem of the interpretation of biomarkers remains actual, especially in a context where their contribution needs to be clearly formalized to weigh in for risk assessment. Hence, integrating such approaches in ERA is still not routinely done, as demonstrated by recent literature.
6.2.3 Ecology: Approaches Used for the Ecological Characterization of the Studied Area
Even though specificity and complexity of the methodologies used in the literature for ecology LoE varied, it was possible to delineate a trend from the analyzed publications. Generally, the studies analyzed here relied either on soil fauna inventories and vegetation surveys or on the estimation of soil functional traits. Soil fauna inventories were prominent among the measurements reported in ecology LoEs (n = 7). Nematodes, oligochaetes and collembolans were chosen to evaluate abundance and diversity of soil invertebrates communities sampled in contaminated areas (Karjalainen et al., 2009; Sorvari et al., 2013; Niemeyer et al., 2015; Gworek et al., 2018). Decrease in the number of taxa or in the total abundance of organisms could be viewed as a direct indication of contamination impacts on population and communities. Being time consuming, these tools were categorized as tier 2, while simple vegetation cover inspections were employed in first tier screening. Some of the reported studies highlighted the difficulty to dissociate the result of these ecological parameters from soil properties other than contamination. For instance, differences in soil fauna communities could be as well related to divergent soil properties or to other confounding factors at the study site, compared to a reference area, rather than to some degree of contamination. More commonly, such ecological indices could respond to the interaction of both and a decrease in species richness could be the cumulative result of, for instance, chemical, physical and biological stresses. Thus the choice of a control site, when possible, with overall similar characteristics with respect to the targeted ones is of primary importance in a triad procedure (van Gestel et al., 2021). Another well-represented approach in ecology LoE consisted in measuring the impact of contamination on soil functioning. The preservation of key soil functions was identified as a primary protection goal and their evaluation has a recognized place in risk assessment of contaminated soils (van Gestel et al., 2009; Römbke et al., 2018). Functional assays such as basal respiration, organic matter decomposition or bait lamina feeding activity were used to estimate the deterioration of key soil processes, like nutrient turnover, to due chemical contamination. Similarly, activities of microbial enzymes included dehydrogenase, alkaline phosphatase and β-glucosidase and informed on the effects of soil contamination on the loss of functions related to biogeochemical cycles of major elements. Only the study from (Gutiérrez et al., 2015) included a tier 3 ecological assessment as part of a simultaneous multi-tiered approach. The authors performed a genetic screening of bacterial and fungal communities, to characterize microbial diversity in soils. In addition, community-level physiological profiles of soil microorganisms were obtained through metabolic activity plate assays. While this was performed in parallel to tier 1 and 2 analyses, to the best of our knowledge no other study reported a tier 3 approach in a triad procedure as part of the classical tier progression strategy. Overall, the detailed triad assessment for ecology is seldom performed, with a clear predisposition for tier 1 and 2.
7 Challenges and Future Recommendations
To date, research into the use of triad approach for ERA of contaminated soil is sparse. Most studies analyzed in this review reported ERA procedures applied to local and hotspot contamination scenarios, mainly caused by PTEs and PAHs. To the best of our knowledge, the available literature on triad used in the case of diffuse contamination is limited to two research articles. This seems insufficient to fully conclude on the outcomes of triad approaches for terrestrial diffuse contamination. Nonetheless, different critical points emerged from the analysis of all the studies, which need to be addressed to improve triad quality. Based on currently available information, some recommendation can be drawn to implement triad approaches, also in view of their applicability on diffusely contaminated soil. The outcomes of the publications covered in this review, with particular regard to strengths and drawbacks of the analyzed approaches, are summarized in Table 2.
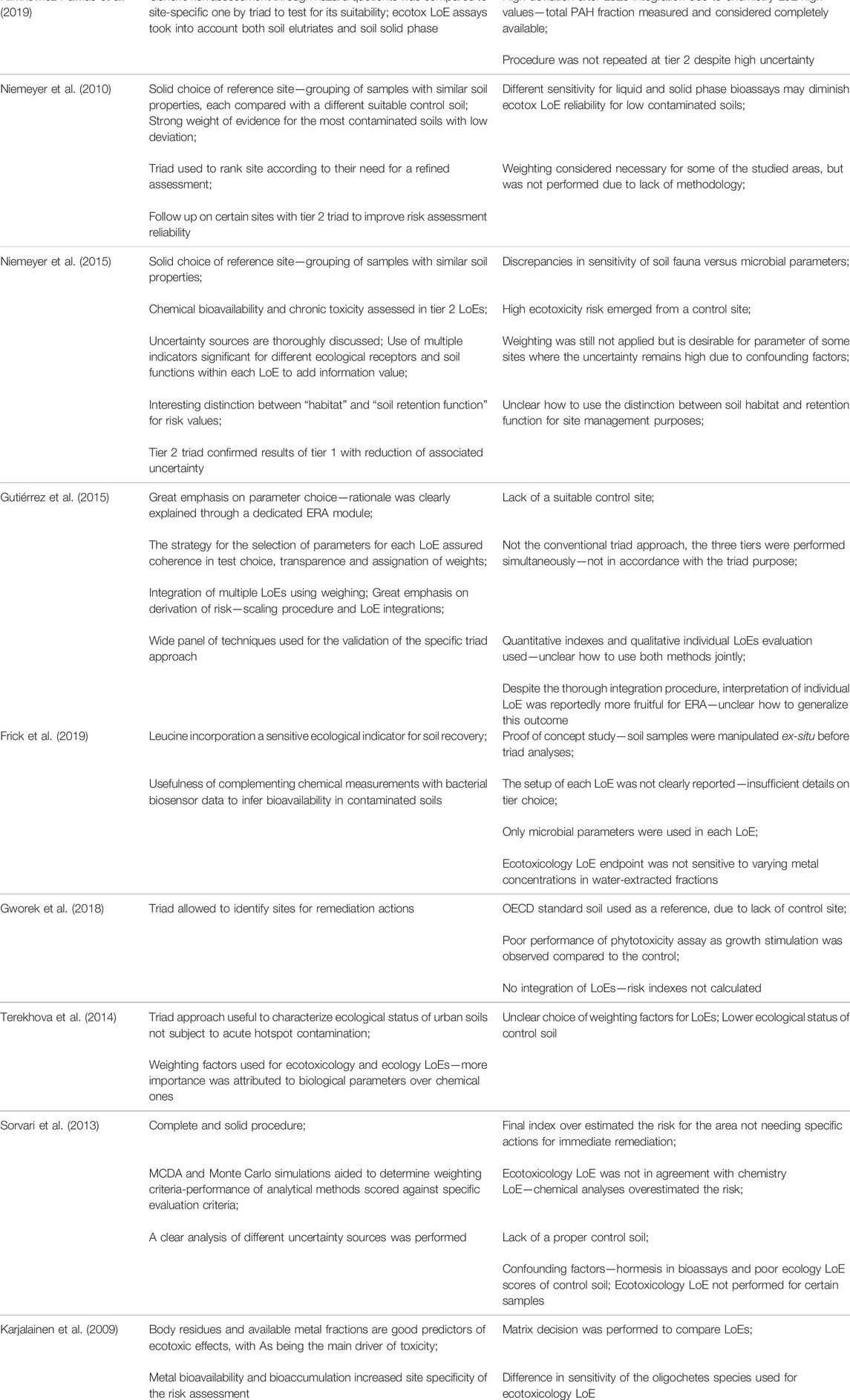
TABLE 2. Main outcomes identified from analyzed literature. Each considered study was screened to evaluate the results of triad and summarize the strengths and weaknesses highlighted by the authors, both in terms of the quality and solidity of the applied procedure, and of possible drawbacks that could hamper risk assessment.
7.1 Loss of Information
Loss of information from individual LoEs following their integration into a final triad risk value has emerged as a common drawback from the analyzed studies. Generally, the production of indexes to summarize heterogeneous and multidisciplinary sets of data implies a trade-off between the synthesizing process and the amount of information possibly excluded from these. This issue encompasses the fields of ecotoxicology and environmental sciences as well (Diaz et al., 2004; Chapman, 2011; Green and Chapman, 2011), and in the triad approach a loss of specific information produced by integration, compared to the individual analysis of LoEs, can be expected to some degree (Silva et al., 2016). Additionally, in some cases the synthesis of distinct results from different fields may prove inconsistent and increase the overall uncertainty. This contrasts with the envisaged increase of reliability of the evaluation promised by the triad approach, as opposed to the information solely provided by one discipline. A major objective of the triad is the optimization of the process, where the use of different LoEs serves to corroborate the final ERA result. In line with this, loss of information should be kept to a minimum and not translate into unreliable risk values. The frameworks for triad offer solutions to cope with uncertainty in index risk (Jensen and Mesman, 2006), when the integration of LoE is done in a quantitative way, even though this is not specified in the ISO guideline (ISO 19204, 2017). In practice, when the standard deviation of the three LoEs is higher than the predefined value of 0.4, the final risk index is considered inconclusive and it triggers a higher tier procedure. One could argue that such a value may give way to a non-conservative interpretation of environmental risk, and that a hard boundary of acceptability might not be scientifically defensible. However, this issue is also inherent to the proposed usage of the triad approach, whether it is for research purposes or merely for management ones. In the latter case, the use of a (pre)defined threshold can be considered functional for clearly stating when the risk justifies more in-depth characterization or the outcome needs to be retained. It was proposed that more complex methods, including probability distributions and correlations, should be used to quantify the likelihood of ecological alterations occurring (Silva et al., 2016), normally not embedded in traditional risk assessment. In this optic, the probabilistic dimension of ERA could be included by using Bayesian inference (Landis, 2021; Moe et al., 2021). Even though it has never been implemented in the context of triad, Bayesian networks seem promising tools for managing the variability and uncertainty stemming from multiple LoEs and to represent causal relationships between ERA inputs (LoEs) and outputs (risk index).
7.2 Adaptations for Low Contaminated Soils
The triad approach tends to give clearer outcomes when applied to heavily contaminated sites, while in the cases of diffuse pollution the different LoEs have been reported to give conflicting results, producing uncertainty when integrated and making the process inconclusive. Diffuse contamination in soil ecosystems usually entails more challenges for ERA, and some adaptations of the triad approach can be proposed to improve its usefulness in this context. It can be argued that soil parameters influencing the bioavailability of studied compounds are largely overlooked in the relevant literature. Combining the assessment of important soil properties, such as cation exchange capacity and the content of soil organic matter, with methods to estimate the environmental availability of contaminants, could advance chemical assessment towards the fractions of concern, instead of using total soil concentrations. Examples of their application to PTE contamination exist in tier 2, while none was reported for organic chemicals in soils. These should be more largely used in chemistry LoE for soils impacted by continuous, low inputs of contaminants, where second tier tools appear more appropriate, since aging of mineral and organic chemicals in soil can greatly alter theirs bioavailability. However, the choice of bioavailability tests is not trivial and depends on many factors and considers different aspects, for instance related to their feasibility or their accuracy, which can be used to score their suitability for inclusion in one tier (Semenzin et al., 2007). Another opportunity for improvement of triad in the case of low contaminated soil due to multiple and diffuse sources could be the implementation of ecotoxicology LoE. Although acute toxicity was mainly investigated, chronic endpoints were also present in the literature. Their addition in ecotoxicology LoE could highlight effects induced by sublethal levels of contaminants that otherwise would not be captured by acute toxicity tests, as lethality tends to emerge following high concentration of soil pollutants, and was seldom responsive in the cases covered in this review. Moreover, we argue that negative alterations of terrestrial ecosystems can accumulate overtime with the continuous inputs of contaminants in soil systems and progressively undermine key soil functions, when certain tipping points are attained. In this light, acute toxicity might not be an useful parameter to capture such long term effects, as they can be viewed as an indication of an already undergone damage. Hence, ecotoxicology and ecology LoE should prefer chronic endpoints that can be related to the future loss or impairment of soil functions. This is in line with the increased attention in terrestrial ecotoxicology to soil functions sustaining key ecosystem services. For instance, soil contamination could influence organic matter turnover and major element cycles. A shift of paradigm, from soil structure being the primary target of soil health assessment, to the continuity in assuring key function, could be welcomed in cases when the effects due to low continuous contaminations are more subtle and difficult to capture. The use of different species from standardized ecotoxicity assays (bacteria, microalgae, crustaceans etc.) can provide a multifaceted set of information more ecologically representative of the studied area and is often opposed to the use of a single species. Accordingly, it is of primary importance that future works use ecologically relevant soil species and, when possible, different organisms (e.g., oligochaetes and collembolans) targeting chronic endpoints, in order to integrate different exposure pathways to soil contaminants for a more inclusive and site-specific assessment.
The use of exposure and effect biomarkers in response to environmental stressors, contextualized to soil contamination, was included only in one study (Gutiérrez et al., 2015). Biomarkers and responses at the molecular level can be viewed as tools to improve the sensitivity of ecotoxicology LoE to mildly contaminated scenarios and possibly reduce of the uncertainty associated with the application of standardized assays-based procedures in triad for diffuse contamination. For instance, the enforcement of a microarray-based transcriptomic approach has been proposed for inclusion in the triad procedure, using as a model the arthropod Folsomia candida (Chen et al., 2014, 2015). Such study illustrated the potential of transcriptomic analysis without, however, including it in an applicative example of the triad approach. Nonetheless, it can serve as a basis to discuss an actual routinely implementation of gene expression analysis for triad-based environmental risk assessment (Chen et al., 2014, 2015; Pitombeira de Figueirêdo et al., 2019). However, in practice the causal link between biomarker responses in soil organisms and an impairment of the ecological function of such species in terrestrial ecosystem is still hard to demonstrate. A set of factors for the inclusion of biomarkers in the assessment of ecosystem health ought to be considered and the classical vision of biomarkers as early warning signals still needs to find its place in the practical application of triad. Interestingly, a WoE approach applied to diffuse soil contamination illustrates a possible use of biomarkers along the classical chemical, ecotoxicological and ecological LoEs (Dagnino et al., 2008). The results from a comprehensive battery of general stress and genotoxicity biomarkers on model organisms exposed to sampled soils was funneled into a separate biological vulnerability index. The authors envisaged to carry out monitoring activities when a certain threshold was reached, even if no environmental threat was identified, in parallel with the classical three-LoEs approach.
(Weeks et al., 2004; Spurgeon et al., 2005a) comprehensively summarized studies enforcing biological tests at different levels of organization, in metal contaminated areas. They identified a continuum of metal-related biological endpoints, ranging from the molecular to the organism level, which virtually describes a sequence of responses along a gradient of contamination with increasing influence at the ecosystem level. For instance, biomarkers such gene expression, the levels of metallothioneins or different classes of Heat Shock Proteins are intuitively difficult to connect to soil ecosystem effects but tend to be present when the level of contamination is low (e.g., with increasing distance from the pollution source or due to diffuse contamination). Other biomarkers occupy a higher level on a virtual ecosystem effect scale and their ecological significance is therefore higher, such as the activity of different classes of esterases and the decrease of energy reserves (e.g., lipid, glycogen and protein levels), which better capture the physiological stress at the organism level. Overall, the interconnectivity of the effect continuum along an increasing gradient of biological organization postulates a latent effect on soil ecosystem. Further studies should explore the concept that biomarkers are truly predictive, for instance through AOP construction, meaning that responses are induced at concentrations below the ones affecting key life parameters (e.g., reproduction), before their use in practical triad approaches is widely accepted.
The biomarkers at the molecular levels, representing an early response to levels of soil contamination that would fail to provoke tangible effects directly determinable in an ecologically appreciable way, can be nonetheless viewed as indicators of the exposure of soil ecosystem to toxicants. In fact, these can be fruitfully employed to detect soil contamination at an early stage or within moderately contaminated contexts, where standardized ecotoxicity approaches (e.g., growth inhibition, mortality, reproduction etc.) would lack the needed sensitivity and accuracy, hence underestimating the risk. Based on the principle of the hierarchical cascade of biological events, the response of biomarkers at the molecular level is indicative of a potential harm to higher levels of organization, hence being a foundational principle of regulatory ecotoxicology (Spurgeon et al., 2005a). Given such premises, a place for biomarkers analysis in the triad procedure shall be found. Some examples are nonetheless present in ERA of aquatic ecosystems, which could also be applicable to the field of terrestrial risk assessment, where biomarkers are less represented. Compared to the biological assays normally included in the triad approach a weighting procedure was envisaged for scoring the selected biomarkers during the phase of the synthesis of results, within and across different LoEs, to arrive to the final risk index (Piva et al., 2011). In this light, biomarkers were weighted differently according to their biological significance in indicating an ecosystem effect (e.g., molecular biomarkers should have a lower weight compared to organism level ones). Such integration of biomarkers within risk indexes, based on WoE approaches, has been carried out in studies regarding aquatic environments impacted by different contamination sources, yet providing a convincing elaboration of different LoEs (Mezzelani et al., 2016; Barjhoux et al., 2018; Pittura et al., 2018; Regoli et al., 2019). Weighting approaches are considered necessary to reduce high uncertainty associated with the integration of the LoEs, by emphasizing the contribution of a single LoE or piece of information over the rest, based on the user evaluation on the peculiar study area. While this could harmonize the information from different LoEs, hence achieving a clear indication at the end of the triad approach, is based on the user judgement and could introduce bias in the process. As subjectivity remains an issue, it can be reduced with multicriteria procedures for weighting and in general benefits the overall risk evaluation (Silva et al., 2016). Additionally, the necessity to expand triad with supplementary LoE or sets of data has been widely expressed (Chapman and Hollert, 2006; Backhaus et al., 2019). Based on these experiences, the translation of such an approach to soil triad procedures is desirable, by taking into account the peculiarities and conditions of soil contamination compared to waterborne one.
7.3 Selection of Reference Sites
The inaccurate selection of the reference site can rebound on the outcomes of the single LoEs, as they are scaled against the control values obtained from such areas. Underestimation of the risk from the study areas is often associated with the selection of an inappropriate reference site. Some of the papers analyzed here reported (Table 2) higher toxicity effects from bioassays on control, supposedly unpolluted soils, compared to the ones targeted by the triad. In some cases, an uncontaminated site is not available in the proximity of the study area, or it may present different physical-chemical characteristics that could act as confounding factors for the interpretation of ecotoxicological bioassays or ecology LoE. For instance, the effect of soil pH can largely determine the outcomes of tests performed on soil elutriates. Thus, these can provide inaccurate baselines for the scaling of results obtained on targeted sites where soil pollution actually elicited ecotoxicological effects. It has been proposed to prioritize, where possible, tests that do not need transformations to fit in a 0 to 1 scale, as the ones expressing percentage responses (Critto et al., 2007). Another interesting approach was proposed by (Dagnino et al., 2008), as a standard artificial soil was used to decide on the usability of the soil collected at the reference site, whereby only ectoxicological assays showing limited differences (i.e., < 20%) when tested on both samples were finally included in ecotox LoE. While this is an interesting solution, its feasibility may be limited by the increased experimental efforts it requires. More generally, when a reference site is not present, environmental variations affecting the targeted ecotoxicological endpoints should be accounted for, to attribute the observed effects exclusively to contamination. On this note, using historical and newly produced data, (Barrick et al., 2016) attempted to standardize the effect of possible confounding factors, such as salinity or temperature, on the energy reserves of the polychaete Hediste diversicolor, in order to create a baseline for toxicity. Interestingly this was possible for oxidative stress and neurotoxicity biomarkers (Barrick et al., 2018), introducing an effect threshold for such responses to be associated with anthropogenic stressors.
To the best of our knowledge, no such efforts have been produced in the field of terrestrial ecotoxicology and applied to the triad approach. Nonetheless, the integration of natural variability could represent a solution when direct comparison with an appropriate reference is not possible (Devin et al., 2014).
8 Conclusion
Growing concerns over soil pollution has prompted the development of tools to evaluate the probability of adverse effects in terrestrial ecosystems and prioritize management actions. The introduction of triad was an important step towards site-specific ERA, and the use of a WoE approach based on chemical, (eco)toxicological and ecological LoEs increased the reliability of the process. In this study, we analyzed the status of the triad approach, by describing its evolution from a practical point of view and we reviewed its utilization for soil contamination. Currently, a very limited amount of studies reported the application to contaminated terrestrial ecosystems, using the procedure described in standardized guidelines. Main deviations consisted in bypassing one LoE or modifying the data integration procedure. Additionally, the application of the triad approach to terrestrial ecosystems was mostly limited to hotspot-like soil contamination, mainly caused by dismantled industrial activities, and the targeted pollutants remain confined to PTEs and PAHs. Scenarios characterized by diffuse contamination are still scarce in scientific literature concerning triad-based ERA. This may be indicative of the challenges associated to handling low, diffuse soil contamination by triad procedure. Although the triad was conceived to be an increasingly site-specific process when needed, environmental traits and confounding soil factors can complicate the interpretation of ecological risks under such conditions. Moreover, some studies did not upgrade to higher tier, as required, when uncertainties compromised the evaluation, making it difficult to determine if an acceptable outcome would be reached under the triad framework. This systematic literature review highlighted different shortcomings of the triad. These concern LoE technical ameliorations, and general aspects regarding both the layout of the procedure and the interpretation of the results. We identified the former to be mainly ascribed to: 1) the integration of tools to measure contaminant bioavailability in order to refine chemical risk assessment; 2) an increase in the sensitivity for sublethal endpoints using exposure and effect biomarkers for ecotoxicology LoE; 3) the more extensive use of chronic endpoints to identify long-term effects of low soil contamination and better link those to effects at higher levels of biological organization. Moreover, given the challenge of applying a composite procedure to heterogeneous environmental compartments, the organization of the process can be adapted. Areas or amelioration includes the use of weighting factors for the prioritization of key parameters, in line with their relevance for case-specific ERA needs, as in the case of diffuse contamination. This would allow exploiting the flexibility of triad and meaningfully integrating the LoEs with new information. Funneling data toward a single, final risk metric could entail loss of information and not be well suited to depict the complexity of environmental problems. Although attempts to include such elements are proposed as alternatives to the guidelines, they are scarce in relevant literature and ultimately rely on expert judgement. Overall, different aspects of the triad are open for implementation, which could expand its applicability. The ever-increasing need to deal with soil contamination from multiple sources stresses the need of risk assessment procedures up to this challenge. This should drive efforts for extensive research on triad itself and encourage its use to gather feedbacks from real scenarios and strengthen its applicability to diffusely contaminated terrestrial ecosystems.
Author Contributions
IL, JF and GG designed the study. GG performed the bibliographic research and wrote the original draft. IL, JF, BJDF and NP revised the manuscript. All authors contributed to the interpretation of results and approved the submitted version.
Funding
This work was financially supported by the European Life program, through the Adsorb project (LIFE17ENV/000398 LIFE ADSORB).
Conflict of Interest
The authors declare that the research was conducted in the absence of any commercial or financial relationships that could be construed as a potential conflict of interest.
Publisher’s Note
All claims expressed in this article are solely those of the authors and do not necessarily represent those of their affiliated organizations, or those of the publisher, the editors and the reviewers. Any product that may be evaluated in this article, or claim that may be made by its manufacturer, is not guaranteed or endorsed by the publisher.
Acknowledgments
GG acknowledges funding from the Adsorb project that supported his postdoctoral work.
References
Adam, V., Caballero-Guzman, A., and Nowack, B. (2018). Considering the Forms of Released Engineered Nanomaterials in Probabilistic Material Flow Analysis. Environ. Pollut. 243, 17–27. doi:10.1016/j.envpol.2018.07.108
Amorim, M. J. B., Römbke, J., and Soares, A. M. V. M. (2005). Avoidance Behaviour of Enchytraeus Albidus: Effects of Benomyl, Carbendazim, Phenmedipham and Different Soil Types. Chemosphere 59, 501–510. doi:10.1016/j.chemosphere.2005.01.057
Backhaus, T., Brack, W., Van den Brink, P. J., Deutschmann, B., Hollert, H., Posthuma, L., et al. (2019). Assessing the Ecological Impact of Chemical Pollution on Aquatic Ecosystems Requires the Systematic Exploration and Evaluation of Four Lines of Evidence. Environ. Sci. Eur. 31, 98. doi:10.1186/s12302-019-0276-z
Barjhoux, I., Fechner, L. C., Lebrun, J. D., Anzil, A., Ayrault, S., Budzinski, H., et al. (2018). Application of a Multidisciplinary and Integrative Weight-Of-Evidence Approach to a 1-year Monitoring Survey of the Seine River. Environ. Sci. Pollut. Res. 25, 23404–23429. doi:10.1007/s11356-016-6993-6
Barrick, A., Châtel, A., Marion, J.-M., Perrein-Ettajani, H., Bruneau, M., and Mouneyrac, C. (2016). A Novel Methodology for the Determination of Biomarker Baseline Levels in the marine Polychaete Hediste Diversicolor. Mar. Pollut. Bull. 108, 275–280. doi:10.1016/j.marpolbul.2016.04.056
Barrick, A., Marion, J.-M., Perrein-Ettajani, H., Châtel, A., and Mouneyrac, C. (2018). Baseline Levels of Biochemical Biomarkers in the Endobenthic Ragworm Hediste Diversicolor as Useful Tools in Biological Monitoring of Estuaries under Anthropogenic Pressure. Mar. Pollut. Bull. 129, 81–85. doi:10.1016/j.marpolbul.2018.02.006
Beaumelle, L., Gimbert, F., Hedde, M., Guérin, A., and Lamy, I. (2015). Subcellular Partitioning of Metals in Aporrectodea Caliginosa along a Gradient of Metal Exposure in 31 Field-Contaminated Soils. Sci. Total Environ. 520, 136–145. doi:10.1016/j.scitotenv.2015.03.021
Beaumelle, L., Lamy, I., Cheviron, N., and Hedde, M. (2014). Is There a Relationship between Earthworm Energy Reserves and Metal Availability after Exposure to Field-Contaminated Soils? Environ. Pollut. 191, 182–189. doi:10.1016/j.envpol.2014.04.021
Beaumelle, L., Vile, D., Lamy, I., Vandenbulcke, F., Gimbert, F., and Hedde, M. (2016). A Structural Equation Model of Soil Metal Bioavailability to Earthworms: Confronting Causal Theory and Observations Using a Laboratory Exposure to Field-Contaminated Soils. Sci. Total Environ. 569–570, 961–972. doi:10.1016/j.scitotenv.2016.06.023
Biasioli, M., Fabietti, G., Barberis, R., and Ajmone-Marsan, F. (2012). An Appraisal of Soil Diffuse Contamination in an Industrial District in Northern Italy. Chemosphere 88, 1241–1249. doi:10.1016/j.chemosphere.2012.03.081
Billings, A., Jones, K. C., Pereira, M. G., and Spurgeon, D. J. (2021). Plasticisers in the Terrestrial Environment: Sources, Occurrence and Fate. Environ. Chem. 18, 111–130. doi:10.1071/EN21033
Boxall Alistair, B. A., Rudd Murray, A., Brooks Bryan, W., Caldwell Daniel, J., Choi, K., Silke, H., et al. (2012). Pharmaceuticals and Personal Care Products in the Environment: What Are the Big Questions? Environ. Health Perspect. 120, 1221–1229. doi:10.1289/ehp.1104477
Brand, E., Lijzen, J., Peijnenburg, W., and Swartjes, F. (2013). Possibilities of Implementation of Bioavailability Methods for Organic Contaminants in the Dutch Soil Quality Assessment Framework. J. Hazard. Mater. 261, 833–839. doi:10.1016/j.jhazmat.2012.11.066
Breure, T., and Peijnenburg, W. (2003). Developments in Soil protection in the Netherlands. J. Soils Sediments 3, 248–249. doi:10.1007/BF02988674
Burton, G. A., Chapman, P. M., and Smith, E. P. (2002). Weight-of-Evidence Approaches for Assessing Ecosystem Impairment. Hum. Ecol. Risk Assess. Int. J. 8, 1657–1673. doi:10.1080/20028091057547
Cachada, A., Ferreira da Silva, E., Duarte, A. C., and Pereira, R. (2016). Risk Assessment of Urban Soils Contamination: The Particular Case of Polycyclic Aromatic Hydrocarbons. Sci. Total Environ. 551–552, 271–284. doi:10.1016/j.scitotenv.2016.02.012
Cachada, A., Pereira, R., da Silva, E. F., and Duarte, A. C. (2014). The Prediction of PAHs Bioavailability in Soils Using Chemical Methods: State of the Art and Future Challenges. Sci. Total Environ. 472, 463–480. doi:10.1016/j.scitotenv.2013.11.038
Cachada, A., Rocha-Santos, T., and Duarte, A. C. (2018). “Chapter 1 - Soil and Pollution: An Introduction to the Main Issues,” in Soil Pollution. Editor A. C. Duarte, A. Cachada, and T. Rocha-Santos (Academic Press), 1–28.
Chapman, P. M. (1989). Current Approaches to Developing Sediment Quality Criteria. Environ. Toxicol. Chem. 8, 589–599. doi:10.1002/etc.5620080706
Chapman, P. M., Dexter, R. N., and Long, E. R. (1987). Synoptic Measures of Sediment Contamination, Toxicity and Infaunal Community Composition (The Sediment Quality Triad) in San Francisco Bay. Mar. Ecol. Prog. Ser. 37, 75–96. doi:10.3354/meps037075
Chapman, P. M., and Hollert, H. (2006). Should the Sediment Quality Triad Become a Tetrad, a Pentad, or Possibly Even a Hexad? J. Soils Sediments 6, 4–8. doi:10.1065/jss2006.01.152
Chapman, P. M. (2011). Indices: Attractive Delusions. Integr. Environ. Assess. Manag. 7, 313. doi:10.1002/ieam.197
Chapman, P. M. (1990). The Sediment Quality Triad Approach to Determining Pollution-Induced Degradation. Sci. Total Environ. 97–98, 815–825. doi:10.1016/0048-9697(90)90277-2
Chen, G., Boer, T. E. d., Wagelmans, M., Gestel, C. A. M. v., Straalen, N. M. v., and Roelofs, D. (2014). Integrating Transcriptomics into Triad-Based Soil-Quality Assessment. Environ. Toxicol. Chem. 33, 900–909. doi:10.1002/etc.2508
Chen, G., den Braver, M. W., van Gestel, C. A. M., van Straalen, N. M., and Roelofs, D. (2015). Ecotoxicogenomic Assessment of Diclofenac Toxicity in Soil. Environ. Pollut. 199, 253–260. doi:10.1016/j.envpol.2015.01.032
Commission of the European Communities (2006). Soil Thematic Strategy, COM(2006) 231. Available at: https://www.eea.europa.eu/policy-documents/soil-thematic-strategy-com-2006-231 (Accessed June 1, 2020).
Crévecoeur, S., Debacker, V., Joaquim-Justo, C., Gobert, S., Scippo, M.-L., Dejonghe, W., et al. (2011). Groundwater Quality Assessment of One Former Industrial Site in Belgium Using a TRIAD-like Approach. Environ. Pollut. 159, 2461–2466. doi:10.1016/j.envpol.2011.06.026
Critto, A., Torresan, S., Semenzin, E., Giove, S., Mesman, M., Schouten, A. J., et al. (2007). Development of a Site-specific Ecological Risk Assessment for Contaminated Sites: Part I. A Multi-Criteria Based System for the Selection of Ecotoxicological Tests and Ecological Observations. Sci. Total Environ. 379, 16–33. doi:10.1016/j.scitotenv.2007.02.035
Cui, X., Mayer, P., and Gan, J. (2013). Methods to Assess Bioavailability of Hydrophobic Organic Contaminants: Principles, Operations, and Limitations. Environ. Pollut. 172, 223–234. doi:10.1016/j.envpol.2012.09.013
Dagnino, A., Sforzini, S., Dondero, F., Fenoglio, S., Bona, E., Jensen, J., et al. (2008). A Weight-Of-Evidence Approach for the Integration of Environmental “Triad” Data to Assess Ecological Risk and Biological Vulnerability. Integr. Environ. Assess. Manag. 4, 314–326. doi:10.1897/IEAM_2007-067.1
de Zwart, D., and Posthuma, L. (2005). Complex Mixture Toxicity for Single and Multiple Species: Proposed Methodologies. Environ. Toxicol. Chem. 24, 2665–2676. doi:10.1897/04-639R.1
Devin, S., Giamberini, L., and Pain-Devin, S. (2014). Variation in Variance Means More Than Mean Variations: What Does Variability Tell Us about Population Health Status? Environ. Int. 73, 282–287. doi:10.1016/j.envint.2014.08.002
Diaz, R. J., Solan, M., and Valente, R. M. (2004). A Review of Approaches for Classifying Benthic Habitats and Evaluating Habitat Quality. J. Environ. Manage. 73, 165–181. doi:10.1016/j.jenvman.2004.06.004
Dick, R. P., Breakwell, D. P., and Turco, R. F. (1997). “Soil Enzyme Activities and Biodiversity Measurements as Integrative Microbiological Indicators,” in Methods for Assessing Soil Quality (John Wiley & Sons), 247–271. doi:10.2136/sssaspecpub49.c15
Drobne, D., Blažič, M., Van Gestel, C. A. M., Lešer, V., Zidar, P., Jemec, A., et al. (2008). Toxicity of Imidacloprid to the Terrestrial Isopod Porcellio scaber (Isopoda, Crustacea). Chemosphere 71, 1326–1334. doi:10.1016/j.chemosphere.2007.11.042
Environment Agency (2004). Application of Sublethal Ecotoxicological Tests for Measuring Harm in Terrestrial Systems. Bristol: Environment Agency.
Fabian, K., Reimann, C., and de Caritat, P. (2017). Quantifying Diffuse Contamination: Method and Application to Pb in Soil. Environ. Sci. Technol. 51, 6719–6726. doi:10.1021/acs.est.7b00741
Flanders, J. R., Long, G., Reese, B., Grosso, N. R., Clements, W., and Stahl, R. G. (2019). Assessment of Potential Mercury Toxicity to Native Invertebrates in a High-Gradient Stream. Integr. Environ. Assess. Manag. 15, 374–384. doi:10.1002/ieam.4133
Forbes, V. E., Palmqvist, A., and Bach, L. (2006). The Use and Misuse of Biomarkers in Ecotoxicology. Environ. Toxicol. Chem. 25, 272. doi:10.1897/05-257R.1
Frick, H., Tardif, S., Kandeler, E., Holm, P. E., and Brandt, K. K. (2019). Assessment of Biochar and Zero-Valent Iron for In-Situ Remediation of Chromated Copper Arsenate Contaminated Soil. Sci. Total Environ. 655, 414–422. doi:10.1016/j.scitotenv.2018.11.193
Giandon, P. (2015). “Soil Contamination by Diffuse Inputs,” in Environmental Indicators. Editors R. H. Armon, and O. Hänninen (Dordrecht: Springer Netherlands), 331–341. doi:10.1007/978-94-017-9499-2_21
Gianfreda, L., Antonietta Rao, M., Piotrowska, A., Palumbo, G., and Colombo, C. (2005). Soil Enzyme Activities as Affected by Anthropogenic Alterations: Intensive Agricultural Practices and Organic Pollution. Sci. Total Environ. 341, 265–279. doi:10.1016/j.scitotenv.2004.10.005
González, V., Díez-Ortiz, M., Simón, M., and van Gestel, C. A. M. (2011). Application of Bioassays with Enchytraeus Crypticus and Folsomia candida to Evaluate the Toxicity of a Metal-Contaminated Soil, before and after Remediation. J. Soils Sediments 11, 1199–1208. doi:10.1007/s11368-011-0391-y
Gottschalk, F., Kost, E., and Nowack, B. (2013). Engineered Nanomaterials in Water and Soils: A Risk Quantification Based on Probabilistic Exposure and Effect Modeling. Environ. Toxicol. Chem. 32, 1278–1287. doi:10.1002/etc.2177
Green, R., and Chapman, P. M. (2011). The Problem with Indices. Mar. Pollut. Bull. 62, 1377–1380. doi:10.1016/j.marpolbul.2011.02.016
Gutiérrez, L., Garbisu, C., Ciprián, E., Becerril, J. M., Soto, M., Etxebarria, J., et al. (2015). Application of Ecological Risk Assessment Based on a Novel TRIAD-Tiered Approach to Contaminated Soil Surrounding a Closed Non-Sealed Landfill. Sci. Total Environ. 514, 49–59. doi:10.1016/j.scitotenv.2015.01.103
Gworek, B., Baczewska-Dąbrowska, A. H., Kalinowski, R., Górska, E. B., Rekosz-Burlaga, H., Gozdowski, D., et al. (2018). Ecological Risk Assessment for Land Contaminated by Petrochemical Industry. PLOS ONE 13, e0204852. doi:10.1371/journal.pone.0204852
Harmsen, J. (2007). Measuring Bioavailability: From a Scientific Approach to Standard Methods. J. Environ. Qual. 36, 1420–1428. doi:10.2134/jeq2006.0492
Harmsen, J., and Naidu, R. (2013). Bioavailability as a Tool in Site Management. J. Hazard. Mater. 261, 840–846. doi:10.1016/j.jhazmat.2012.12.044
Harmsen, J., Rulkens, W., and Eijsackers, H. (2005). Bioavailability: Concept for Understanding or Tool for Predicting? Land Contam. Reclam. 13, 161–171. doi:10.1061/9780784408155.ch01
Harwood, A. D., Sutherland, G. E., Woller-Skar, M. M., Lydy, M. J., and Borrello, M. C. (2019). Evaluating Toxicity Risk in Sediments after Remediation at a Superfund Megasite Using a Triad Approach. Environ. Monit. Assess. 191, 665. doi:10.1007/s10661-019-7870-9
Iannuzzi, T. J., Armstrong, T. N., Long, E. R., Iannuzzi, J., and Ludwig, D. F. (2008). Sediment Quality Triad Assessment of an Industrialized Estuary of the Northeastern USA. Environ. Monit. Assess. 139, 257–275. doi:10.1007/s10661-007-9832-x
ISO 17402 (2008). Soil Quality. Requirements and Guidance for the Selection and Application of Methods for the Assessment of Bioavailability of Contaminants in Soil and Soil Materials. Geneva, Switzerland: International Standard organization. Available at: https://www.iso.org/cms/render/live/en/sites/isoorg/contents/data/standard/03/83/38349.html (Accessed May 18, 2020).
ISO 17616 (2019). Soil Quality. Guidance on the Choice and Evaluation of Bioassays for Ecotoxicological Characterization of Soils and Soil Materials. Geneva, Switzerland. Available at: https://shop.bsigroup.com/ProductDetail/?pid=000000000030363588 (Accessed May 15, 2020).
ISO 18311 (2016). Soil Quality. Method for Testing Effects of Soil Contaminants on the Feeding Activity of Soil Dwelling Organisms — Bait-Lamina Test. Geneva, Switzerland. Available at: https://www.iso.org/cms/render/live/en/sites/isoorg/contents/data/standard/06/21/62102.html (Accessed February 3, 2022).
ISO 19204 (2017). Soil Quality. Procedure for Site-specific Ecological Risk Assessment of Soil Contamination (Soil Quality TRIAD Approach). GenevaSwitzerland. Available at: https://www.iso.org/cms/render/live/en/sites/isoorg/contents/data/standard/06/39/63989.html (Accessed April 7, 2020).
Iwinski, K. J., McQueen, A. D., Kinley, C. M., Calomeni, A. J., Geer, T. D., and Rodgers, J. H. (2016). Sediment Copper Concentrations, In Situ Benthic Invertebrate Abundance, and Sediment Toxicity: Comparison of Treated and Untreated Coves in a Southern Reservoir. Water Air Soil Pollut. 227, 85. doi:10.1007/s11270-016-2778-2
Janssen, C. R., Heijerick, D. G., De Schamphelaere, K. A. C., and Allen, H. E. (2003). Environmental Risk Assessment of Metals: Tools for Incorporating Bioavailability. Environ. Int. 28, 793–800. doi:10.1016/S0160-4120(02)00126-5
Jensen, J., and Mesman, M. (2006). Ecological Risk Assessment of Contaminated Land: Decision Support for Site Specific Investigations. Bilthoven: RIVM. Available at: http://www.rivm.nl/bibliotheek/rapporten/711701047.pdf (Accessed April 10, 2020).
Karjalainen, A.-M., Kilpi-Koski, J., Väisänen, A. O., Penttinen, S., Gestel, C. A. v., and Penttinen, O.-P. (2009). Ecological Risks of an Old wood Impregnation Mill: Application of the Triad Approach. Integr. Environ. Assess. Manag. 5, 379–389. doi:10.1897/IEAM_2008-072.1
Klimkowicz-Pawlas, A., Maliszewska-Kordybach, B., and Smreczak, B. (2019). Triad-based Screening Risk Assessment of the Agricultural Area Exposed to the Long-Term PAHs Contamination. Environ. Geochem. Health 41, 1369–1385. doi:10.1007/s10653-018-0220-y
Koelmans, A. A., Redondo-Hasselerharm, P. E., Nor, N. H. M., de Ruijter, V. N., Mintenig, S. M., and Kooi, M. (2022). Risk Assessment of Microplastic Particles. Nat. Rev. Mater. 7, 138–152. doi:10.1038/s41578-021-00411-y
Kördel, W., Bernhardt, C., Derz, K., Hund-Rinke, K., Harmsen, J., Peijnenburg, W., et al. (2013). Incorporating Availability/bioavailability in Risk Assessment and Decision Making of Polluted Sites, Using Germany as an Example. J. Hazard. Mater. 261, 854–862. doi:10.1016/j.jhazmat.2013.05.017
Kumpiene, J., Giagnoni, L., Marschner, B., Denys, S., Mench, M., Adriaensen, K., et al. (2017). Assessment of Methods for Determining Bioavailability of Trace Elements in Soils: A Review. Pedosphere 27, 389–406. doi:10.1016/S1002-0160(17)60337-0
Landis, W. G. (2021). The Origin, Development, Application, Lessons Learned, and Future Regarding the Bayesian Network Relative Risk Model for Ecological Risk Assessment. Integr. Environ. Assess. Manag. 17, 79–94. doi:10.1002/ieam.4351
Lanno, R. P., Oorts, K., Smolders, E., Albanese, K., and Chowdhury, M. J. (2019). Effects of Soil Properties on the Toxicity and Bioaccumulation of Lead in Soil Invertebrates. Environ. Toxicol. Chem. 38, 1486–1494. doi:10.1002/etc.4433
Lanno, R., Wells, J., Conder, J., Bradham, K., and Basta, N. (2004). The Bioavailability of Chemicals in Soil for Earthworms. Ecotoxicol. Environ. Saf. 57, 39–47. doi:10.1016/j.ecoenv.2003.08.014
Lavtižar, V., Berggren, K., Trebše, P., Kraak, M. H. S., Verweij, R. A., and van Gestel, C. A. M. (2016). Comparative Ecotoxicity of Chlorantraniliprole to Non-target Soil Invertebrates. Chemosphere 159, 473–479. doi:10.1016/j.chemosphere.2016.06.036
Leclerc, A., Sala, S., Secchi, M., and Laurent, A. (2019). Building National Emission Inventories of Toxic Pollutants in Europe. Environ. Int. 130, 104785. doi:10.1016/j.envint.2019.03.077
Lee, J., Hong, S., Kwon, B.-O., Cha, S. A., Jeong, H.-D., Chang, W. K., et al. (2018). Integrated Assessment of Persistent Toxic Substances in Sediments from Masan Bay, South Korea: Comparison between 1998 and 2014. Environ. Pollut. 238, 317–325. doi:10.1016/j.envpol.2018.02.064
Li, J.-Y., Tang, J. Y. M., Jin, L., and Escher, B. I. (2013). Understanding Bioavailability and Toxicity of Sediment-Associated Contaminants by Combining Passive Sampling with In Vitro Bioassays in an Urban River Catchment. Environ. Toxicol. Chem. 32, 2888–2896. doi:10.1002/etc.2387
Linkov, I., Loney, D., Cormier, S., Satterstrom, F. K., and Bridges, T. (2009). Weight-of-evidence Evaluation in Environmental Assessment: Review of Qualitative and Quantitative Approaches. Sci. Total Environ. 407, 5199–5205. doi:10.1016/j.scitotenv.2009.05.004
Linkov, I., Satterstrom, F. K., Kiker, G., Batchelor, C., Bridges, T., and Ferguson, E. (2006). From Comparative Risk Assessment to Multi-Criteria Decision Analysis and Adaptive Management: Recent Developments and Applications. Environ. Int. 32, 1072–1093. doi:10.1016/j.envint.2006.06.013
Linkov, I., Welle, P., Loney, D., Tkachuk, A., Canis, L., Kim, J. B., et al. (2011). Use of Multicriteria Decision Analysis to Support Weight of Evidence Evaluation. Risk Anal. 31, 1211–1225. doi:10.1111/j.1539-6924.2011.01585.x
Lofts, S., Criel, P., Janssen, C. R., Lock, K., McGrath, S. P., Oorts, K., et al. (2013). Modelling the Effects of Copper on Soil Organisms and Processes Using the Free Ion Approach: Towards a Multi-Species Toxicity Model. Environ. Pollut. 178, 244–253. doi:10.1016/j.envpol.2013.03.015
Long, E. R., and Chapman, P. M. (1985). A Sediment Quality Triad: Measures of Sediment Contamination, Toxicity and Infaunal Community Composition in Puget Sound. Mar. Pollut. Bull. 16, 405–415. doi:10.1016/0025-326X(85)90290-5
Loureiro, S., Soares, A. M. V. M., and Nogueira, A. J. A. (2005). Terrestrial Avoidance Behaviour Tests as Screening Tool to Assess Soil Contamination. Environ. Pollut. 138, 121–131. doi:10.1016/j.envpol.2005.02.013
Lu, X., Skwarski, A., Drake, B., and Reible, D. D. (2011). Predicting Bioavailability of PAHs and PCBs with Porewater Concentrations Measured by Solid-phase Microextraction Fibers. Environ. Toxicol. Chem. 30, 1109–1116. doi:10.1002/etc.495
Luo, W., Verweij, R. A., and van Gestel, C. A. M. (2014). Determining the Bioavailability and Toxicity of lead Contamination to Earthworms Requires Using a Combination of Physicochemical and Biological Methods. Environ. Pollut. 185, 1–9. doi:10.1016/j.envpol.2013.10.017
Marziali, L., Rosignoli, F., Drago, A., Pascariello, S., Valsecchi, L., Rossaro, B., et al. (2017). Toxicity Risk Assessment of Mercury, DDT and Arsenic Legacy Pollution in Sediments: A Triad Approach under Low Concentration Conditions. Sci. Total Environ. 593–594, 809–821. doi:10.1016/j.scitotenv.2017.03.219
Mendes, M. P., Salomão, A. L. S., Niemeyer, J. C., and Marques, M. (2017). Ecological Risk Assessment in a Tropical Wetland Contaminated with Gasoline: Tier 1. Hum. Ecol. Risk Assess. 23, 992–1007. doi:10.1080/10807039.2017.1294477
Mezzelani, M., Gorbi, S., Da Ros, Z., Fattorini, D., d’Errico, G., Milan, M., et al. (2016). Ecotoxicological Potential of Non-steroidal Anti-inflammatory Drugs (NSAIDs) in marine Organisms: Bioavailability, Biomarkers and Natural Occurrence in Mytilus galloprovincialis. Mar. Environ. Res. 121, 31–39. doi:10.1016/j.marenvres.2016.03.005
Moe, S. J., Carriger, J. F., and Glendell, M. (2021). Increased Use of Bayesian Network Models Has Improved Environmental Risk Assessments. Integr. Environ. Assess. Manag. 17, 53–61. doi:10.1002/ieam.4369
Moreira, L. B., Leite, P. R. B. D., Dias, M. D. L., Martins, C. D. C., and Abessa, D. M. D. S. (2019). Sediment Quality Assessment as Potential Tool for the Management of Tropical Estuarine Protected Areas in SW Atlantic, Brazil. Ecol. Indic. 101, 238–248. doi:10.1016/j.ecolind.2018.12.052
Naidu, R., Channey, R., McConnell, S., Johnston, N., Semple, K. T., McGrath, S., et al. (2015). Towards Bioavailability-Based Soil Criteria: Past, Present and Future Perspectives. Environ. Sci. Pollut. Res. 22, 8779–8785. doi:10.1007/s11356-013-1617-x
Ng, E.-L., Huerta Lwanga, E., Eldridge, S. M., Johnston, P., Hu, H.-W., Geissen, V., et al. (2018). An Overview of Microplastic and Nanoplastic Pollution in Agroecosystems. Sci. Total Environ. 627, 1377–1388. doi:10.1016/j.scitotenv.2018.01.341
Niemeyer, J. C., Moreira-Santos, M., Nogueira, M. A., Carvalho, G. M., Ribeiro, R., Da Silva, E. M., et al. (2010). Environmental Risk Assessment of a Metal-Contaminated Area in the Tropics. Tier I: Screening Phase. J. Soils Sediments 10, 1557–1571. doi:10.1007/s11368-010-0255-x
Niemeyer, J. C., Moreira-Santos, M., Ribeiro, R., Rutgers, M., Nogueira, M. A., Silva, E. M. da., et al. (2015). Ecological Risk Assessment of a Metal-Contaminated Area in the Tropics. Tier II: Detailed Assessment. PLOS ONE 10, e0141772. doi:10.1371/journal.pone.0141772
Niu, L., Carmona, E., König, M., Krauss, M., Muz, M., Xu, C., et al. (2020). Mixture Risk Drivers in Freshwater Sediments and Their Bioavailability Determined Using Passive Equilibrium Sampling. Environ. Sci. Technol. 54, 13197–13206. doi:10.1021/acs.est.0c05124
Ortega-Calvo, J.-J., Harmsen, J., Parsons, J. R., Semple, K. T., Aitken, M. D., Ajao, C., et al. (2015). From Bioavailability Science to Regulation of Organic Chemicals. Environ. Sci. Technol. 49, 10255–10264. doi:10.1021/acs.est.5b02412
Peijnenburg, W. J. G. M., Zablotskaja, M., and Vijver, M. G. (2007). Monitoring Metals in Terrestrial Environments within a Bioavailability Framework and a Focus on Soil Extraction. Ecotoxicol. Environ. Saf. 67, 163–179. doi:10.1016/j.ecoenv.2007.02.008
Pereira, J. L., Pereira, P., Padeiro, A., Gonçalves, F., Amaro, E., Leppe, M., et al. (2017). Environmental hazard Assessment of Contaminated Soils in Antarctica: Using a Structured Tier 1 Approach to Inform Decision-Making. Sci. Total Environ. 574, 443–454. doi:10.1016/j.scitotenv.2016.09.091
Pereira, R., Cachada, A., Sousa, J. P., Niemeyer, J., Markwiese, J., and Andersen, C. P. (2018). “Chapter 8 - Ecotoxicological Effects and Risk Assessment of Pollutants,” in Soil Pollution. Editors A. C. Duarte, A. Cachada, and T. Rocha-Santos (Academic Press), 191–216. doi:10.1016/B978-0-12-849873-6.00008-X
Pit, I. R., van Egmond, E. M., Dekker, S. C., Griffioen, J., Wassen, M. J., and van Wezel, A. P. (2018). Ecotoxicological Risk of Trace Element Mobility in Coastal Semiartificial Depositional Areas Near the Mouth of the River Rhine, the Netherlands: Ecotoxicological Risk of Trace Element Mobility in the River Rhine. Environ. Toxicol. Chem. 37, 2933–2946. doi:10.1002/etc.4262
Pitombeira de Figueirêdo, L., Daam, M. A., Mainardi, G., Mariën, J., Espíndola, E. L. G., van Gestel, C. A. M., et al. (2019). The Use of Gene Expression to Unravel the Single and Mixture Toxicity of Abamectin and Difenoconazole on Survival and Reproduction of the Springtail Folsomia candida. Environ. Pollut. 244, 342–350. doi:10.1016/j.envpol.2018.10.077
Pittura, L., Avio, C. G., Giuliani, M. E., d’Errico, G., Keiter, S. H., Cormier, B., et al. (2018). Microplastics as Vehicles of Environmental PAHs to Marine Organisms: Combined Chemical and Physical Hazards to the Mediterranean Mussels, Mytilus galloprovincialis. Front. Mar. Sci. 5. doi:10.3389/fmars.2018.00103
Piva, F., Ciaprini, F., Onorati, F., Benedetti, M., Fattorini, D., Ausili, A., et al. (2011). Assessing Sediment hazard through a Weight of Evidence Approach with Bioindicator Organisms: A Practical Model to Elaborate Data from Sediment Chemistry, Bioavailability, Biomarkers and Ecotoxicological Bioassays. Chemosphere 83, 475–485. doi:10.1016/j.chemosphere.2010.12.064
Pivato, A., Lavagnolo, M. C., Manachini, B., Vanin, S., Raga, R., and Beggio, G. (2017). Ecological Risk Assessment of Agricultural Soils for the Definition of Soil Screening Values: A Comparison between Substance-Based and Matrix-Based Approaches. Heliyon 3, e00284. doi:10.1016/j.heliyon.2017.e00284
Posthuma, L., Eijsackers, H. J. P., Koelmans, A. A., and Vijver, M. G. (2008). Ecological Effects of Diffuse Mixed Pollution Are Site-specific and Require Higher-Tier Risk Assessment to Improve Site Management Decisions: A Discussion Paper. Sci. Total Environ. 406, 503–517. doi:10.1016/j.scitotenv.2008.06.065
Posthuma, L., Glenn, W. S., and Traas, T. P. (2001). Species Sensitivity Distributions in Ecotoxicology. Boca raton, FL: CRC Press.
Posthuma, L., and Suter, G. W. (2011). “Ecological Risk Assessment of Diffuse and Local Soil Contamination Using Species Sensitivity Distributions,” in Dealing with Contaminated Sites: From Theory towards Practical Application. Editor F. A. Swartjes (Dordrecht: Springer Netherlands), 625–691. doi:10.1007/978-90-481-9757-6_14
Puglisi, E., Murk, A. J., Berg, H. J. v. d., and Grotenhuis, T. (2007). Extraction and Bioanalysis of the Ecotoxicologically Relevant Fraction of Contaminants in Sediments. Environ. Toxicol. Chem. 26, 2122–2128. doi:10.1897/06-581R.1
Regoli, F., d’Errico, G., Nardi, A., Mezzelani, M., Fattorini, D., Benedetti, M., et al. (2019). Application of a Weight of Evidence Approach for Monitoring Complex Environmental Scenarios: the Case-Study of Off-Shore Platforms. Front. Mar. Sci. 6. doi:10.3389/fmars.2019.00377
Ribé, V., Aulenius, E., Nehrenheim, E., Martell, U., and Odlare, M. (2012). Applying the Triad Method in a Risk Assessment of a Former Surface Treatment and Metal Industry Site. J. Hazard. Mater. 207–208, 15–20. doi:10.1016/j.jhazmat.2011.07.120
Rillig, M. C., Ingraffia, R., and de Souza Machado, A. A. (2017). Microplastic Incorporation into Soil in Agroecosystems. Front. Plant Sci. 8. doi:10.3389/fpls.2017.01805
Römbke, J., Bernard, J., and Martin-Laurent, F. (2018). Standard Methods for the Assessment of Structural and Functional Diversity of Soil Organisms: A Review. Integr. Environ. Assess. Manag. 14, 463–479. doi:10.1002/ieam.4046
Rutgers, M., Faber, J. H., Postma, J. F., and Eijsackers, H. (2000). Site-specific Ecological Risks: A Basic Approach to Function-specific Assessment of Soil Pollution. Wageningen, Netherlands: Netherlands Integrated Soil Research Programme, 28.
Rutgers, M., and Jensen, J. (2011). “Site-Specific Ecological Risk Assessment,” in Dealing with Contaminated Sites. Editor F. A. Swartjes (Dordrecht: Springer Netherlands), 693–720. doi:10.1007/978-90-481-9757-6_15
Scott-Fordsmand, J. J., and Weeks, J. M. (2000). “Biomarkers in Earthworms,” in Reviews of Environmental Contamination and Toxicology. Editor G. W. Ware (New York, NY: Springer New York), 117–159. doi:10.1007/978-1-4612-1172-3_3
Semenzin, E., Critto, A., Carlon, C., Rutgers, M., and Marcomini, A. (2007). Development of a Site-specific Ecological Risk Assessment for Contaminated Sites: Part II. A Multi-Criteria Based System for the Selection of Bioavailability Assessment Tools. Sci. Total Environ. 379, 34–45. doi:10.1016/j.scitotenv.2007.02.034
Semenzin, E., Critto, A., Rutgers, M., and Marcomini, A. (2009). “DSS-ERAMANIA: Decision Support System for Site-specific Ecological Risk Assessment of Contaminated Sites,” in Decision Support Systems for Risk-Based Management of Contaminated Sites. Editors A. Marcomini, G. W. SuterII, and A. Critto (Boston, MA: Springer US), 1–33. doi:10.1007/978-0-387-09722-0_10
Semenzin, E., Critto, A., Rutgers, M., and Marcomini, A. (2008). Integration of Bioavailability, Ecology and Ecotoxicology by Three Lines of Evidence into Ecological Risk Indexes for Contaminated Soil Assessment. Sci. Total Environ. 389, 71–86. doi:10.1016/j.scitotenv.2007.08.032
Sieber, R., Kawecki, D., and Nowack, B. (2020). Dynamic Probabilistic Material Flow Analysis of Rubber Release from Tires into the Environment. Environ. Pollut. 258, 113573. doi:10.1016/j.envpol.2019.113573
Signore, A. D., Hendriks, A. J., Lenders, H. J. R., Leuven, R. S. E. W., and Breure, A. M. (2016). Development and Application of the SSD Approach in Scientific Case Studies for Ecological Risk Assessment. Environ. Toxicol. Chem. 35, 2149–2161. doi:10.1002/etc.3474
Silva, C., Yáñez, E., Martín-Díaz, M. L., and DelValls, T. A. (2016). GIS-based Ecological Risk Assessment for Contaminated Sites by Fish Farm Effluents Using a Multicriteria Weight of Evidence Approach. Aquac. Res. 47, 524–539. doi:10.1111/are.12512
Smith, E. P., Lipkovich, I., and Ye, K. (2002). Weight-of-Evidence (WOE): Quantitative Estimation of Probability of Impairment for Individual and Multiple Lines of Evidence. Hum. Ecol. Risk Assess. Int. J. 8, 1585–1596. doi:10.1080/20028091057493
Smith, R., Pollard, S. J. T., Weeks, J. M., and Nathanail, C. P. (2005). Assessing Significant Harm to Terrestrial Ecosystems from Contaminated Land. Soil Use Manag. 21, 527–540. doi:10.1079/SUM2005345
Smolders, E., Oorts, K., Sprang, P. V., Schoeters, I., Janssen, C. R., McGrath, S. P., et al. (2009). Toxicity of Trace Metals in Soils as Affected by Soil Type and Aging after Contamination: Using Calibrated Bioavailability Models to Set Ecological Soil Standards. Environ. Toxicol. Chem. 28, 1633. doi:10.1897/08-592.1
Snow, D. D., Cassada, D. A., Biswas, S., Malakar, A., D’Alessio, M., Carter, L. J., et al. (2019). Detection, Occurrence, and Fate of Emerging Contaminants in Agricultural Environments. Water Environ. Res. 91, 1103–1113. doi:10.1002/wer.1204
Sorvari, J., Schultz, E., and Haimi, J. (2013). Assessment of Ecological Risks at Former Landfill Site Using TRIAD Procedure and Multicriteria Analysis. Risk Anal. 33, 203–219. doi:10.1111/j.1539-6924.2012.01858.x
Spurgeon, D. J., Ricketts, H., Svendsen, C., Morgan, A. J., and Kille, P. (2005a). Hierarchical Responses of Soil Invertebrates (Earthworms) to Toxic Metal Stress. Environ. Sci. Technol. 39, 5327–5334. doi:10.1021/es050033k
Spurgeon, D. J., Svendsen, C., and Hankard, P. K. (2005b). “Biological Methods for Assessing Potentially Contaminated Soils,” in Environmental Toxicity Testing. Editors K. C. Thompson, K. Wadhia, and A. P. Loibner (Oxford, UK: Blackwell Publishing Ltd.), 163–205. doi:10.1002/9781444305531.ch6
Spurgeon, D. J., Weeks, J. M., and Van Gestel, C. A. M. (20032002). A Summary of Eleven Years Progress in Earthworm Ecotoxicology: The 7th International Symposium on Earthworm Ecology. Cardiff · Wales ·Pedobiologia 47, 588–606. doi:10.1078/0031-4056-0023410.1016/s0031-4056(04)70243-7
Stolte, J., Tesfai, M., Øygarden, L., Kværnø, S., Keizer, J., Verheijen, F., et al. (2015). Soil Threats in Europe. Luxembourg: Publications Office.
Suter, G., Cormier, S., and Barron, M. (2017). A Weight of Evidence Framework for Environmental Assessments: Inferring Qualities. Integr. Environ. Assess. Manag. 13, 1038–1044. doi:10.1002/ieam.1954
Swartjes, F. A., Breure, A. M., and Beaulieu, M. (2011). “Introduction to Ecological Risk Assessment,” in Dealing with Contaminated Sites: From Theory towards Practical Application. Editor F. A. Swartjes (Dordrecht: Springer Netherlands), 573–624. doi:10.1007/978-90-481-9757-6_13
Swartjes, F. A., Rutgers, M., Lijzen, J. P. A., Janssen, P. J. C. M., Otte, P. F., Wintersen, A., et al. (2012). State of the Art of Contaminated Site Management in The Netherlands: Policy Framework and Risk Assessment Tools. Sci. Total Environ. 427–428, 1–10. doi:10.1016/j.scitotenv.2012.02.078
ter Laak, T. L., Agbo, S. O., Barendregt, A., and Hermens, J. L. M. (2006). Freely Dissolved Concentrations of PAHs in Soil Pore Water: Measurements via Solid-Phase Extraction and Consequences for Soil Tests. Environ. Sci. Technol. 40, 1307–1313. doi:10.1021/es0514803
Terekhova, V. A., Pukalchik, M. A., and Yakovlev, A. S. (2014). The Triad Approach to Ecological Assessment of Urban Soils. Eurasian Soil Sci. 47, 952–958. doi:10.1134/S1064229314090129
Titaley, I. A., Simonich, S. L. M., and Larsson, M. (2020). Recent Advances in the Study of the Remediation of Polycyclic Aromatic Compound (PAC)-Contaminated Soils: Transformation Products, Toxicity, and Bioavailability Analyses. Environ. Sci. Technol. Lett. 7, 873–882. doi:10.1021/acs.estlett.0c00677
Udovic, M., Drobne, D., and Lestan, D. (2009). Bioaccumulation in Porcellio scaber (Crustacea, Isopoda) as a Measure of the EDTA Remediation Efficiency of Metal-Polluted Soil. Environ. Pollut. 157, 2822–2829. doi:10.1016/j.envpol.2009.04.023
US EPA (1992). Framework for Ecological Risk Assessment. Available at: https://www.epa.gov/risk/framework-ecological-risk-assessment (Accessed April 26, 2020).
van Gestel, C. A. M., Koolhaas, J. E., Hamers, T., van Hoppe, M., van Roovert, M., Korsman, C., et al. (2009). Effects of Metal Pollution on Earthworm Communities in a Contaminated Floodplain Area: Linking Biomarker, Community and Functional Responses. Environ. Pollut. 157, 895–903. doi:10.1016/j.envpol.2008.11.002
van Gestel, C. A. M., Mommer, L., Montanarella, L., Pieper, S., Coulson, M., Toschki, A., et al. (2021). Soil Biodiversity: State-Of-The-Art and Possible Implementation in Chemical Risk Assessment. Integr. Environ. Assess. Manag. 17, 541–551. doi:10.1002/ieam.4371
van Gestel, C. A. M., Waarde, J. J. v. d., Derksen, J. G. M., Hoek, E. E. v. d., Veul, M. F. X. W., Bouwens, S., et al. (2001). The Use of Acute and Chronic Bioassays to Determine the Ecological Risk and Bioremediation Efficiency of Oil-Polluted Soils. Environ. Toxicol. Chem. 20, 1438–1449. doi:10.1002/etc.5620200705
Vodyanitskii, Yu. N., and Yakovlev, A. S. (2016). Contamination of Soils and Groundwater with New Organic Micropollutants: A Review. Eurasian Soil Sci. 49, 560–569. doi:10.1134/S1064229316050148
Volchko, Y., Berggren Kleja, D., Back, P.-E., Tiberg, C., Enell, A., Larsson, M., et al. (2020). Assessing Costs and Benefits of Improved Soil Quality Management in Remediation Projects: A Study of an Urban Site Contaminated with PAH and Metals. Sci. Total Environ. 707, 135582. doi:10.1016/j.scitotenv.2019.135582
Weeks, J. M., and Comber, S. D. W. (2005). Ecological Risk Assessment of Contaminated Soil. Mineral. Mag. 69, 601–613. doi:10.1180/0026461056950274
Weeks, J. M., Spurgeon, D. J., Svendsen, C., Hankard, P. K., Kammenga, J. E., Dallinger, R., et al. (2004). Critical Analysis of Soil Invertebrate Biomarkers: A Field Case Study in Avonmouth, UK. Ecotoxicology 13, 817–822. doi:10.1007/s10646-003-4479-z
Williams‐Nguyen, J., Sallach, J. B., Bartelt‐Hunt, S., Boxall, A. B., Durso, L. M., McLain, J. E., et al. (2016). Antibiotics and Antibiotic Resistance in Agroecosystems: State of the Science. J. Environ. Qual. 45, 394–406. doi:10.2134/jeq2015.07.0336
Winding, A., Hund-Rinke, K., and Rutgers, M. (2005). The Use of Microorganisms in Ecological Soil Classification and Assessment Concepts. Ecotoxicol. Environ. Saf. 62, 230–248. doi:10.1016/j.ecoenv.2005.03.026
Keywords: integrative risk assessment, ecotoxicology, biomarkers, exposure, terrestrial pollution
Citation: Grassi G, Lamy I, Pucheux N, Ferrari BJD and Faburé J (2022) State of the Art of Triad-Based Ecological Risk Assessment: Current Limitations and Needed Implementations in the Case of Soil Diffuse Contamination. Front. Environ. Sci. 10:878238. doi: 10.3389/fenvs.2022.878238
Received: 17 February 2022; Accepted: 04 April 2022;
Published: 12 May 2022.
Edited by:
Andrew Hursthouse, University of the West of Scotland, United KingdomReviewed by:
Agnieszka Klimkowicz-Pawlas, Institute of Soil Science and Plant Cultivation, PolandJulia Carina Niemeyer, Federal University of Santa Catarina, Brazil
Copyright © 2022 Grassi, Lamy, Pucheux, Ferrari and Faburé. This is an open-access article distributed under the terms of the Creative Commons Attribution License (CC BY). The use, distribution or reproduction in other forums is permitted, provided the original author(s) and the copyright owner(s) are credited and that the original publication in this journal is cited, in accordance with accepted academic practice. No use, distribution or reproduction is permitted which does not comply with these terms.
*Correspondence: Giacomo Grassi, Z2lhY29tby5ncmFzc2lAaW5yYWUuZnI=